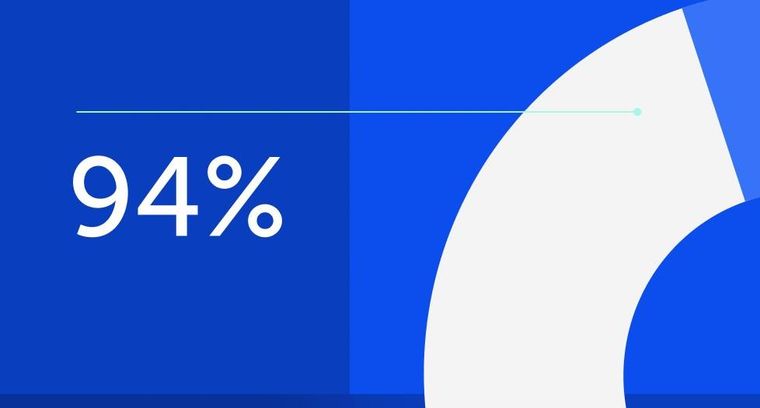
94% of researchers rate our articles as excellent or good
Learn more about the work of our research integrity team to safeguard the quality of each article we publish.
Find out more
BRIEF RESEARCH REPORT article
Front. Anim. Sci., 22 March 2024
Sec. Animal Nutrition
Volume 5 - 2024 | https://doi.org/10.3389/fanim.2024.1349499
Immune function plays a pivotal role in dictating the overall health and productivity of cattle. In a proficient immune system, the liver assumes an integral function in detoxification and metabolic processes and contributes substantially to overall production and immunity. In this study, we evaluated the hepatic mRNA expression of genes involved in innate and adaptive immunity in crossbred beef steers with positive or negative residual body weight gain (RADG). Positive-RADG beef steers (n = 8; RADG = 0.73 kg/d) and negative-RADG beef steers (n = 8; RADG = -0.69 kg/d) were identified from a group of 108 growing crossbred beef steers (average BW = 556 ± 38 kg) after a 56-d performance testing period. At the end of the 56-d period, liver tissue samples were collected from the beef steers for RNA extraction and cDNA synthesis. The mRNA expression of 84 genes involved in innate and adaptive immunity were analyzed using pathway-focused PCR-based arrays. The mRNA expression of genes with false discovery rate-adjusted P-values (FDR) ≤ 0.05 and absolute fold change (FC) ≥ 1.2 were determined to be differentially expressed. Out of the 84 genes analyzed, four genes (IL-2, MYD88, CD-80, NFkB-1) were differentially expressed and were all upregulated in positive compared with negative-RADG beef steers. IL-2 is a cytokine that plays a critical role in the immune response by activating and proliferating T-cells, which are important for fighting infections. MYD88 is an adaptor protein that is essential for signaling by toll-like receptors, which are involved in pathogen recognition. CD80 is a protein that is expressed on the surface of antigen-presenting cells and plays a critical role in the initiation of an immune response. The activation of NF-κB leads to the production of cytokines and chemokines that help to recruit immune cells to the site of infection. The upregulation of the aforementioned genes in positive-RADG beef steers suggests that they had a better ability than negative-RADG beef steers to quickly recognize pathogens and initiate appropriate responses to effectively fight off infections without causing inflammatory reactions, potentially contributing to their better feed efficiency.
In beef production, the cost of feeding is a crucial factor that significantly impacts the overall cost of production. Studies have demonstrated that feed cost accounts for 60 to 70% of the total expenses in animal production (Becker, 2008; Greenwood, 2021). Given this, improving feed efficiency holds the potential to mitigate costs and enhance the economic viability of farms. Moreover, improved feed efficiency can lead to reduced environmental impacts by reducing nutrient wastes and mitigating greenhouse gas emissions (Llonch et al., 2017). There is a growing need to understand the biological mechanisms associated with feed efficiency in beef production systems, considering the complex sustainability factors such as the overall impact of feed cost and environmental footprints. Residual feed intake (RFI) and residual body weight gain (RADG) have been explored for several years as measures of feed efficiency in beef cattle (Crews, 2005; Berry and Crowley, 2012). Residual body weight gain is the difference between the actual daily gain and predicted gain based on body weight and dry matter intake of beef cattle (Northcutt and Bowerman, 2010). Positive RADG values signify more desirable feed efficient animals, while negative RADG values indicate inefficient animals (Crowley et al., 2011).
Several studies have sought to understand how selection for divergent RFI or RADG affects important traits such as metabolism, fertility, rumen microbiome, and carcass characteristics (Cantalapiedra-Hijar et al., 2018; Taiwo et al., 2022; Idowu et al., 2023). In our recent study, Taiwo et al. (2023) investigated expression of immunity-associated genes in the liver and whole blood of crossbred beef cattle with divergent RFI. The results revealed differences in the expression of genes associated with several pathways including pattern recognition receptor activity and immune cell differentiation; however, little is known about how selection based on RADG affects immune gene expression in beef cattle.
The liver functions as a frontline immune organ strategically positioned to detect and clear pathogens entering the body through the gut, underscoring its significant role in the immune response (Loor et al., 2005; Yan et al., 2014). Notably, variations in the expression of immune-related genes in this organ can profoundly affect metabolic function, nutrient availability, and feed efficiency in animals (Vigors et al., 2019; Cheng et al., 2021). Thus, in this study, we hypothesized that the expression of some immunity genes would vary in beef steers with divergent positive or negative RADG. Our objective was to determine the differences in mRNA expression of certain innate and adaptive immune genes in the liver of beef steers with divergent negative or positive RADG phenotypes.
The research procedures described in this study received approval from the Institutional Animal Care and Use Committees of West Virginia (protocol number 2204052569). A total of 108 growing crossbred (Angus × Hereford) beef steers (average body weight (BW) = 556 ± 38 kg; 529 ± 22 d of age), were fed a high-forage total mixed ration (TMR) consisting primarily of corn silage, ground hay, and a ration-balancing supplement (Table 1) for a total of 56 days after a 15-d adaptation period to the feeding facilities and diet. The steers were housed in five dry lot pens (20-22 steers per pen). Two GrowSafe 8000 intake nodes (GrowSafe Systems Ltd., Airdrie, Alberta, Canada) were installed in each pen to monitor individual feed intake. Daily BW measurements were obtained using In-Pen Weighing Positions (IPW, Vytelle LLC). At the end of the 56-d period, the beginning BW, mid-test metabolic BW, and average daily gain (ADG) were calculated by regressing the daily BW for each animal using a simple linear regression. The ADG of each steer was regressed against their daily dry matter intake (DMI) and mid-test metabolic BW (MMTW = mid-test BW0.75), and the RADG was calculated as the residual or the difference between the predicted value of the regression and the actual measured value using the following regression equation: Y = β0 + β1X1 + β2X2 + ε, where Y represents the ADG (kg/d), β0 is the regression intercept, β1 and β2 are the partial regression coefficients, X1 is the MMTW (kg), X2 is the observed DMI (kg/d) (Koch et al., 1963; Berry and Crowley, 2012). Upon completion of the feeding trial, the beef steers were ranked based on their RADG coefficients and the most efficient (positive-RADG = +0.76 kg/d, n = 8) and least efficient (negative-RADG = -0.65 kg/d, n = 8) were identified for further analysis.
Liver tissues were obtained from the 8 positive-RADG and 8 negative-RADG beef steers using a needle biopsy under local anesthesia. An incision was made in the skin and liver tissue was harvested from the 10th intercostal space through a 14-gauge biopsy needle (Tru-Core-II Automatic Biopsy Instrument: Angiotech, Lausanne, Switzerland). Approximately 1 g of liver tissue sample was obtained from each of the 16 steers by puncture and was immediately transferred into RNA-Protect tubes (cat. No: 76104; Qiagen) and stored at −80°C for further analysis. A sub-sample of the liver tissue (5 mg each) was used for total RNA extraction using RNeasy Micro kit (cat. no. 74004; Qiagen) following the manufacturer’s instructions. Samples with >100 ng/µL total RNA were used. RNA concentration was measured using a NanoDrop 2000 spectrophotometer with an A260:A280 ratio from 1.8 to 2.0 (Thermo Fisher Scientific, Waltham, MA, USA). All samples had RNA integrity numbers > 8.0 analyzed using Agilent 2100 Bioanalyzer (Agilent Technologies). Complementary DNA (cDNA) synthesis was then carried out using the extracted, purified RNA. To obtain transcript abundance, cDNA was synthesized via reverse transcription (RT) using the RT2 first strand kit (cat. no. 330401; Qiagen). RT2 Profiler cow innate and adaptive immune responses Polymerase chain reaction (PCR) Array (PABT-052ZA; Qiagen) was used for mRNA expression analysis of 84 genes related to innate and adaptive immunity. Each array was a 96-well plate containing 84 adaptive and innate immune-related genes together with five housekeeping genes (β-actin, hypoxanthine phosphoribosyl transferase 1, glyceraldehyde-3-phosphate dehydrogenase, tyrosine 3-monooxygenase, and TATA box-binding protein), three RT, three positive PCR controls, and one genomic DNA control (Supplementary Table S2). Real-time PCR was performed using the Quant Studio 5 real-time PCR system (Applied Biosystems, Foster City, CA). The PCR cycle conditions were as follows: 40 denaturation cycles of 95°C for 10 min, 95°C for 15 s, and 60°C for 1 min.
Variables such as initial and final BW, ADG, DM intake, and RADG values were analyzed using the GLIMMIX procedure of SAS version 9.4 (SAS Institute Inc., Cary, NC), with RADG status included as a fixed effect. Significant effects were declared at P ≤ 0.05. Values of initial body weight were included as a covariate for the final body weight. We used the Gene Globe data analysis center (https://geneglobe.qiagen.com) to analyze the mRNA expression data. The comparative cycle threshold (Ct) method was used for the relative quantification of the gene expression (Pfaffl, 2001). To determine the differences in mRNA expression between the positive- and negative-RADG beef steers, the delta-delta Ct (2−ΔΔCt) method was employed. The raw data were normalized using the geometric mean of the five housekeeping genes (Pfaffl, 2001). The stability of the housekeeping genes was confirmed using ΔCt and NormFinder (Andersen et al., 2004). The PCR arrays employed in this study have an average amplification efficiency of 99%, with a 95% confidence interval ranging from 90 to 110%, enabling accurate simultaneous analysis of multiple genes using the 2−ΔΔCt method. Differentially expressed genes were identified using absolute fold change (FC) ≥ 1.2 and false discovery rate-adjusted P-values of 0.05.
The results showing the growth performance of the beef steers with divergent RADG phenotypes are presented in Table 2. The average RADG values of positive- and negative-RADG beef steers were 0.73 and -0.69 kg/d, respectively (P = 0.01). Dry matter intake and initial BW of the two groups were similar (P > 0.05). Compared to negative-RADG, final BW and ADG were greater (P ≤ 0.05) in positive- RADG than negative-RADG beef steers.
The mRNA expression analysis of the 84 innate and adaptive immune genes is shown in Supplementary Table S3. Among the 84 genes analyzed, the mRNA expression of 4 genes encoding interleukin 2 (IL-2), nuclear factor NF-kappa-B (NF-κB-1), myeloid differentiation primary response 88 (MYD88), and the cluster of differentiation 80 (CD80) were differentially expressed and were all upregulated in beef steers with positive RADG (FC ≥ 1.2, P ≤ 0.05; Table 3). The upregulation of IL-2, MYD88, and NFκB-1 in positive-RADG beef steers suggests a robust immune interaction between the innate and adaptive cells of these animals. Myeloid differentiation primary response 88 (MYD88) plays a crucial role in innate immune signaling, mediating the innate immune response and cytokine production to combat pathogens and stimulate adaptive immunity (Arnold-Schrauf et al., 2014; Li et al., 2020). Upon lipopolysaccharide recognition, MYD88 plays a critical adapter protein role in the TLR4 signaling pathway, which in turn activates downstream signaling molecules that express genes involved in the inflammatory response during bacterial infection (Fitzgerald et al., 2004). Interleukin 2 contributes to immune balance and long-lasting cell-mediated immunity (Liao et al., 2011; Wrenshall et al., 2014). Nuclear factor kappa B is vital for the development, activation, and survival of adaptive immune cells (Lee and Kleiboeker, 2005; Tergaonkar, 2006). It also plays a pivotal role in the innate immune response to pathogens through pattern recognition receptor signaling (Lee and Kim, 2007). Prolonged inhibition of NF-κB-1 can result in inappropriate immune cell development or delayed cell growth (Hinz et al., 1999; Graham et al., 2010).
The increased expression of CD80-1 in the positive-RADG beef steers indicates effective adaptive immune responses. CD80, primarily expressed on antigen-presenting cells like macrophages and dendritic cells, interacts with its receptor CD28 on T cells, providing co-stimulatory signals necessary for sustained T cell activation and proliferation (Lenschow et al., 1996; Sharpe and Freeman, 2002). Moreover, CD80 plays a role in regulating the balance between immune activation and tolerance (Martínez-Méndez et al., 2021). The upregulation of CD80 in beef steers with positive RADG suggest a competent immunological memory, enabling rapid response upon re-exposure to previously encountered pathogens.
The results of this study revealed the upregulation of key immune-related genes like IL-2, CD-80, MYD88, and NFκB-1 in the liver of beef steers with positive RADG, suggesting a robust interaction between innate and adaptive immune cells, potentially contributing to their improved feed efficiency. Future research should focus on assessing the immunocompetence of beef steers classified as positive or negative RADG when exposed to pathogen challenges. Such investigations are crucial in unveiling the intricate links between immunocompetence and feed efficiency, thereby enabling targeted interventions to improve livestock health and growth performance.
The original contributions presented in the study are included in the article/Supplementary Material. Further inquiries can be directed to the corresponding author.
The animal study was approved by The Institutional Animal Care and Use Committees of West Virginia. The study was conducted in accordance with the local legislation and institutional requirements.
DO: Data curation, Formal analysis, Investigation, Methodology, Visualization, Writing – original draft, Writing – review & editing. MI: Data curation, Formal analysis, Investigation, Methodology, Visualization, Writing – original draft, Writing – review & editing. GT: Data curation, Investigation, Methodology, Visualization, Writing – review & editing. TS: Data curation, Investigation, Methodology, Visualization, Writing – review & editing. ET: Investigation, Methodology, Visualization, Writing – review & editing. FE: Formal analysis, Investigation, Methodology, Visualization, Writing – review & editing. FB: Formal analysis, Investigation, Resources, Writing – review & editing. IO: Conceptualization, Data curation, Formal analysis, Funding acquisition, Investigation, Methodology, Resources, Supervision, Visualization, Writing – original draft, Writing – review & editing.
The author(s) declare that financial support was received for the research, authorship, and/or publication of this article. The study was funded by West Virginia University Experimental Station in support of U.S. Department of Agriculture hatch multi-state regional project W-3010.
The authors declare that the research was conducted in the absence of any commercial or financial relationships that could be construed as a potential conflict of interest.
All claims expressed in this article are solely those of the authors and do not necessarily represent those of their affiliated organizations, or those of the publisher, the editors and the reviewers. Any product that may be evaluated in this article, or claim that may be made by its manufacturer, is not guaranteed or endorsed by the publisher.
The Supplementary Material for this article can be found online at: https://www.frontiersin.org/articles/10.3389/fanim.2024.1349499/full#supplementary-material
Andersen C. L., Jensen J. L., Ørntoft T. F. (2004). Normalization of Real-Time Quantitative Reverse Transcription-PCR data: a Model-Based variance estimation approach to identify genes suited for normalization, applied to bladder and colon cancer data sets. Cancer Res. 64, 5245–5250. doi: 10.1158/0008-5472.CAN-04-0496
Arnold-Schrauf C., Dudek M., Dielmann A., Pace L., Swallow M., Kruse F., et al. (2014). Dendritic Cells Coordinate Innate Immunity via MyD88 Signaling to Control Listeria monocytogenes Infection. Cell Rep. 6, 698–708. doi: 10.1016/j.celrep.2014.01.023
Becker G. S. (2008). Livestock Feed Costs: Concerns and Options (Washington, DC, USA: Congressional Research Service, Library of Congress).
Berry D., Crowley J. (2012). Residual intake and body weight gain: A new measure of efficiency in growing cattle. J. Anim. Sci. 90, 109–115. doi: 10.2527/jas.2011-4245
Cantalapiedra-Hijar G., Abo-Ismail M., Carstens G. E., Guan L. L., Hegarty R., Kenny D. A., et al. (2018). Biological determinants of between-animal variation in feed efficiency of growing beef cattle. Animal 12, s321–s335. doi: 10.1017/S1751731118001489
Cheng M., Nakib D., Perciani C. T., MacParland S. A. (2021). The immune niche of the liver. Clin. Sci. 135, 2445–2466. doi: 10.1042/CS20190654
Crews D. H. (2005). Genetics of efficient feed utilization and national cattle evaluation: a review. PubMed 4, 152–165.
Crowley J., Evans R., Hugh N. M., Pabiou T., Kenny D. A., McGee M., et al. (2011). Genetic associations between feed efficiency measured in a performance test station and performance of growing cattle in commercial beef herds. J. Anim. Sci. 89, 3382–3393. doi: 10.2527/jas.2011-3836
Fitzgerald K. A., Rowe D. C., Golenbock D. T. (2004). Endotoxin recognition and signal transduction by the TLR4/MD2-complex. Microbes Infect. 6, 1361–1367. doi: 10.1016/j.micinf.2004.08.015
Graham J. G., Tullai J. W., Cooper G. M. (2010). GSK-3 represses growth factor-inducible genes by inhibiting NF-KB in quiescent cells. J. Biol. Chem. 285, 4472–4480. doi: 10.1074/jbc.M109.053785
Greenwood P. L. (2021). Review: An overview of beef production from pasture and feedlot globally, as demand for beef and the need for sustainable practices increase. Animal 15, 100295. doi: 10.1016/j.animal.2021.100295
Hinz M., Krappmann D., Eichten A., Heder A., Scheidereit C., Strauss M. (1999). NF-κB function in growth control: regulation of cyclin D1 expression and G0/G1-to-S-phase transition. Mol. Cell. Biol. 19, 2690–2698. doi: 10.1128/MCB.19.4.2690
Idowu M., Taiwo G. A., Sidney T., Olanrewaju M., Pech-Cervantes A. A., Estrada-Reyes Z. M., et al. (2023). The differential plasma and ruminal metabolic pathways and ruminal bacterial taxa associated with divergent residual body weight gain phenotype in crossbred beef steers. Trans. Anim. Sci. 7. doi: 10.1093/tas/txad054
Koch R. M., Swiger L. A., Chambers D. T., Gregory K. E. (1963). Efficiency of feed use in beef cattle. J. Anim. Sci. 22, 486–494. doi: 10.2527/jas1963.222486x
Lee M. S., Kim Y. (2007). Signaling pathways downstream of Pattern-Recognition receptors and their cross talk. Annu. Rev. Biochem. 76, 447–480. doi: 10.1146/annurev.biochem.76.060605.122847
Lee S., Kleiboeker S. B. (2005). Porcine arterivirus activates the NF-κB pathway through IκB degradation. Virology 342, 47–59. doi: 10.1016/j.virol.2005.07.034
Lenschow D. J., Walunas T. L., Bluestone J. A. (1996). Cd28/b7 system of t cell costimulation. Annu. Rev. Immunol. 14, 233–258. doi: 10.1146/annurev.immunol.14.1.233
Li Q., Wang F., Wang Q., Zhang N., Zheng J., Zheng M., et al. (2020). SPOP promotes ubiquitination and degradation of MyD88 to suppress the innate immune response. PloS Pathog. 16, e1008188. doi: 10.1371/journal.ppat.1008188
Liao W., Lin J., Leonard W. J. (2011). IL-2 family cytokines: new insights into the complex roles of IL-2 as a broad regulator of T helper cell differentiation. Curr. Opin. Immunol. 23, 598–604. doi: 10.1016/j.coi.2011.08.003
Llonch P., Haskell M., Dewhurst R., Turner S. (2017). Current available strategies to mitigate greenhouse gas emissions in livestock systems: An animal welfare perspective. Animal 11, 274–284. doi: 10.1017/S1751731116001440
Loor J. J., Dann H., Everts R. E., Oliveira R., Green C. A., Guretzky N., et al. (2005). Temporal gene expression profiling of liver from periparturient dairy cows reveals complex adaptive mechanisms in hepatic function. Physiol. Genomics 23, 217–226. doi: 10.1152/physiolgenomics.00132.2005
Martínez-Méndez D., Mendoza L., Villarreal C. C., Huerta L. (2021). Continuous modeling of T CD4 lymphocyte activation and function. Front. Immunol. 12. doi: 10.3389/fimmu.2021.743559
Northcutt S., Bowerman B. (2010). Angus feed efficiency selection tool: RADG. By the Numbers. Angus J., 170–172.
Pfaffl M. W. (2001). A new mathematical model for relative quantification in real-time RT-PCR. Nucleic Acids Res. 29, 45e–445. doi: 10.1093/nar/29.9.e45
Sharpe A. H., Freeman G. J. (2002). The B7–CD28 superfamily. Nat. Rev. Immunol. 2, 116–126. doi: 10.1038/nri727
Taiwo G., Idowu M., Pech-Cervantes A. A., Estrada-Reyes Z. M., Ogunade I. M. (2022). Hepatic mRNA Expression of Nutrient and Mitochondrial Energy Metabolism Genes in Beef Steers Selected for low or High Residual Feed Intake. J. Anim. Sci. 100, 384. doi: 10.1093/jas/skac247.702
Taiwo G., Morenikeji O. B., Idowu M., Sidney T., Adekunle A., Pech-Cervantes A. A., et al. (2023). Characterization of rumen microbiome and immune genes expression of crossbred beef steers with divergent residual feed intake phenotypes. Res. Square. 25, 245. doi: 10.21203/rs.3.rs-2793700/v1
Tergaonkar V. (2006). NFκB pathway: A good signaling paradigm and therapeutic target. Int. J. Biochem. Cell Biol. 38, 1647–1653. doi: 10.1016/j.biocel.2006.03.023
Vigors S., O’Doherty J. V., Bryan K., Sweeney T. (2019). A comparative analysis of the transcriptome profiles of liver and muscle tissue in pigs divergent for feed efficiency. BMC Genomics 20. doi: 10.1186/s12864-019-5740-z
Wrenshall L. E., Clabaugh S. E., Cool D. R., Arumugam P., Grunwald W. C., Smith D. R., et al. (2014). Identification of a cytotoxic form of dimeric interleukin-2 in murine tissues. PloS One 9, e102191. doi: 10.1371/journal.pone.0102191
Keywords: feed efficiency, immunity, gene expression, liver, beef cattle
Citation: Ologunagba D, Idowu M, Taiwo G, Sidney T, Treon E, Eichie F, Bebe F and Ogunade IM (2024) Hepatic mRNA expression of innate and adaptive immune genes in beef steers with divergent residual body weight gain. Front. Anim. Sci. 5:1349499. doi: 10.3389/fanim.2024.1349499
Received: 04 December 2023; Accepted: 07 March 2024;
Published: 22 March 2024.
Edited by:
James Levi Klotz, United States Department of Agriculture, United StatesReviewed by:
Nicole C. Burdick Sanchez, Agricultural Research Service (USDA), United StatesCopyright © 2024 Ologunagba, Idowu, Taiwo, Sidney, Treon, Eichie, Bebe and Ogunade. This is an open-access article distributed under the terms of the Creative Commons Attribution License (CC BY). The use, distribution or reproduction in other forums is permitted, provided the original author(s) and the copyright owner(s) are credited and that the original publication in this journal is cited, in accordance with accepted academic practice. No use, distribution or reproduction is permitted which does not comply with these terms.
*Correspondence: Ibukun M. Ogunade, aWJ1a3VuLm9ndW5hZGVAbWFpbC53dnUuZWR1
Disclaimer: All claims expressed in this article are solely those of the authors and do not necessarily represent those of their affiliated organizations, or those of the publisher, the editors and the reviewers. Any product that may be evaluated in this article or claim that may be made by its manufacturer is not guaranteed or endorsed by the publisher.
Research integrity at Frontiers
Learn more about the work of our research integrity team to safeguard the quality of each article we publish.