- 1Bristol Veterinary School, University of Bristol, Bristol, United Kingdom
- 2Department of Veterinary Medicine and Animal Science, University of Milan, Milan, Italy
- 3Institute of Food, Nutrition and Health, University of Reading, Reading, United Kingdom
Consumption of animal-derived foods (ADFs), particularly red meat, is declining in high-income countries because of concerns over health and the effects on climate change but is increasing in low- and middle-income countries. As a group of foods, ADFs are high in good-quality protein and several key vitamins and minerals (notably vitamin B12, iron and zinc). There is evidence, though, that processed red meat poses risks of cardiovascular disease (CVD) and colorectal cancer and the same risks, although not so strong, are apparent for unprocessed red meat. Milk and milk products generally have a neutral disease risk and there is evidence of reduced risks of CVD and colorectal cancer. Similarly, white meat (chicken and fish) is not associated with disease risk whilst eggs have been linked with increased CVD risk because of their cholesterol content. The risks of chronic disease seem higher in high-income than in low- and middle-income countries, possibly due to different levels of consumption. Production of ADFs results in high greenhouse gas emissions per unit of output compared with plant proteins. Ruminant meat production has particularly high costs but wide variation between farms in different regions of the world suggests costs can be significantly lowered by changes to production systems. Reducing ADF consumption to benefit health and the environment has been proposed but in low-income countries, current levels of consumption of ADFs may be compatible with health and climate targets.
1 Introduction
Animal-derived foods (ADFs) (meat and meat products, milk and milk products, fish and seafood, and eggs) have been important constituents of the diets of people around the world for millions of years and are part of the culture of many ethnic groups. They provide high-quality protein and a range of nutrients important for the maintenance of health and they contribute unique tastes and flavours to meals and dishes. However, since the 1950s, their consumption has been linked with the incidence of chronic diseases and more recently with climate change. In low- and middle-income countries, the production and consumption of ADFs have increased rapidly in recent years and are expected to increase further in the next 10 years. In high income countries consumption may have reached a peak and there are calls to reduce consumption for health and environmental reasons. Alternative forms of dietary protein based on plants and microbes are now widely available and others are emerging (e.g., cell-cultured meat and insects). There are therefore many questions surrounding the future of ADFs and this overview aims to summarise the current state of thinking.
2 Trends in consumption of ADFs
Although plants provide most (57%) of the protein in diets globally (Henchion et al., 2017), ADFs provide a high percentage of the dietary protein in many countries, for example 59% in the 19-64 age group in the UK (Public Health England, 2020). Of this, 34% is from meat and meat products, 13% from milk and milk products, 7% from fish and seafood and 5% from eggs. Individual countries vary in the relative contributions of the different ADFs, depending on historical and cultural factors (Auestad et al., 2015).
In the last 50 years (1961-2020), global production of ADFs has greatly increased: meat and meat products by 403% to 352 Mt, milk and milk products by 167% to 918 Mt, fish and seafood by 355% to 176 Mt and eggs by 513% to 92 Mt (FAO, 2021). Low- and middle-income countries, particularly in Asia, have shown the greatest growth, driven by population increases and per capita consumption, the latter explained by increasing incomes. There were wide variations in the growth of per capita consumption between countries and regions of the world (Table 1). Per capita consumption of all ADFs increased markedly in China, milk and milk products by 956% and meat by 1747%. Increases were much smaller in Europe and North America and consumption of milk fell by 23% in the latter. Africa had the lowest levels of consumption and consumption of milk declined in the 50-year period. In China, consumption of all meat types increased, beef by 7388%. In Europe and North America, consumption of beef fell by 13% and 20%, respectively but levels were still higher than in China in 2020. In Africa, sheepmeat and beef consumption declined. Poultry showed the biggest increase among meat types in all regions and increased by 467% in the world as a whole.
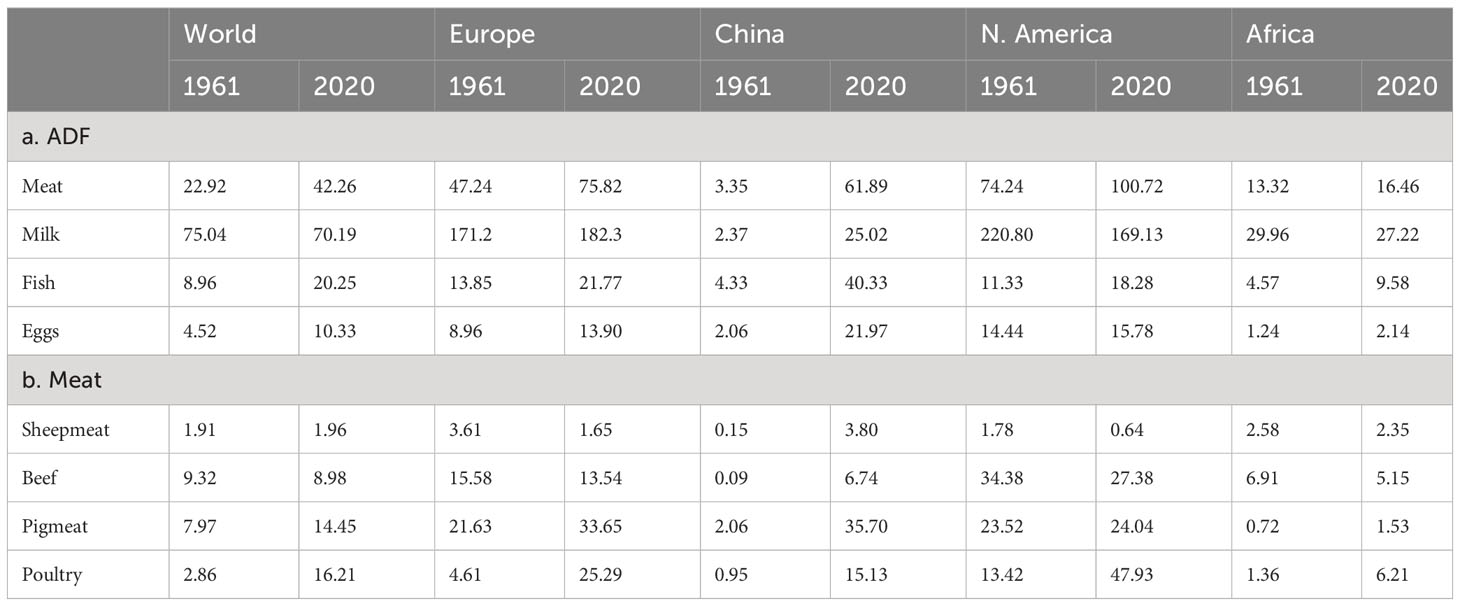
Table 1 Consumption (kg/person/yr) of a. ADFs and b. Meat types, for World, Europe, China, North America and Africa in 1961 and 2020 (FAO, 2021).
According to the agricultural outlook published by OECD/FAO (2023), global meat production is expected to increase by a further 12% between 2022 and 2032, with most of the growth occurring in Asia. Poultry production will show the greatest growth globally (13%), poultry accounting for half of the additional meat production in the next 10 years. Pigmeat production will outstrip poultry production in China as the country recovers from the African Swine Fever outbreak in 2018. Beef production will show the least growth (9%). Meat consumption per capita is predicted to increase by 2% globally in the next 10 years with most of the growth occurring in low- and middle-income countries. Poultry will show the greatest increase and will provide 41% of protein from meat sources in 2032. The Outlook notes that concerns about some aspects of meat production (animal welfare, environmental costs and human health) is now global and is partly responsible for the switch from beef to poultry consumption in many countries, poultry having lower feed and environmental costs and white meat perceived as having less harmful effects on human health than red meats (see sections 4-6 in this review). These consumer concerns are causing moves towards veganism and vegetarianism in some countries. In UK, a recent poll by YouGov (2023) showed that 3% of adults now consider themselves vegan, 5% vegetarian and 71% meat-eaters. Consumers can now purchase a variety of non-meat protein foods including plant-based and microbial proteins and foods from non-traditional animal sources such as insects and cell-cultured meat (Frank et al., 2022).
World milk production is expected to grow by 15% between 2022 and 2032 (OECD/FAO, 2023). India is the largest milk-producing country and production will increase by 30% in this period. Production in Europe and North America will remain static. Per capita consumption will increase by 7% globally, India having the highest per capita consumption at 52kg per year, most of which is liquid milk. Europe and North America consume more than half of their milk as processed products (butter and cheese) and the share of processed products is also increasing in other regions. As with meat, there is increasing availability of plant-based milk-like products.
Global fish production is expected to increase by 17% between 2022 and 2032 (OECD/FAO, 2023). Aquaculture will account for 54% of fish production in 2032, with production from capture fisheries not expected to increase. China continues to dominate world aquaculture production and will have a 56% share of global production in 2032. Per capita consumption of fish is expected to increase by 4% in the 10-year period with the greatest level of consumption (24.5 kg/year) occurring in Asia.
Global egg production is expected to increase by 12% between 2022 and 2032 (OECD/FAO, 2023) and by 2032, 76% of production will occur in low- and middle-income countries, 35% in China. Per capita consumption is expected to increase by 5% globally, with China having the highest level of consumption in 2032 (24.7 kg/year).
In conclusion, global production and consumption of all ADFs are expected to increase in the next 10 years, particularly in low- and middle-income countries where populations and incomes are increasing. There are downward trends in consumption in high-income countries, particularly for red meat, but per capita consumption levels are still relatively high.
3 Environmental effects of production of ADFs
The climate of the Earth is changing, with temperatures increasing and rainfall and weather events becoming more extreme (Henry et al., 2018). It is now recognised that the production of ADFs contributes significantly to climate change by increasing greenhouse gas emissions (GHGEs), the main cause of climate change and increasing pressure on other environment indicators such as land use, biodiversity loss, depletion of freshwater resources and pollution of aquatic and terrestrial ecosystems (Springmann et al., 2018; Scarborough et al., 2023). Production of ADFs in many parts of the world is negatively affected by climate change, for example production from ruminants in tropical and sub-tropical regions where people depend on livestock for income and nutrition (Henry et al., 2018).
It is estimated that globally the food chain from farm to retail is responsible for around 30% of anthropogenic GHGEs (Crippa et al., 2021; Scarborough et al., 2023), with meat, dairy, fish and eggs contributing about 56% of these emissions (Poore and Nemecek, 2018). The farm stage dominates the production of GHGEs, with post-farm gate activities, including processing, making negligible contributions in comparison. Estimates of GHGEs from the farm to retail stages per kg food product, based on data from 38,700 farms in 119 countries in Table 2 show that animal products are associated with much higher emissions than plant products, the lowest-impact animal products typically exceeding those of vegetable substitutes (Poore and Nemecek, 2018). The biggest emissions are from ruminant production, with beef and cattle milk production estimated to account for 41% and 20% respectively of the livestock sector’s emissions (Gerber et al., 2013). Beef production from dairy herds is associated with about 30% of the GHGEs of those from beef herds because the costs are shared between both meat and milk production. The main source of emissions (44% of livestock emissions) is methane from enteric fermentation and manure handling. Atmospheric amounts of methane have increased rapidly over the last decade and although methane remains in the atmosphere for a much shorter time than CO2 it has approximately 30 times the climate-warming effect when measured over a 100-year period (UN, 2021).
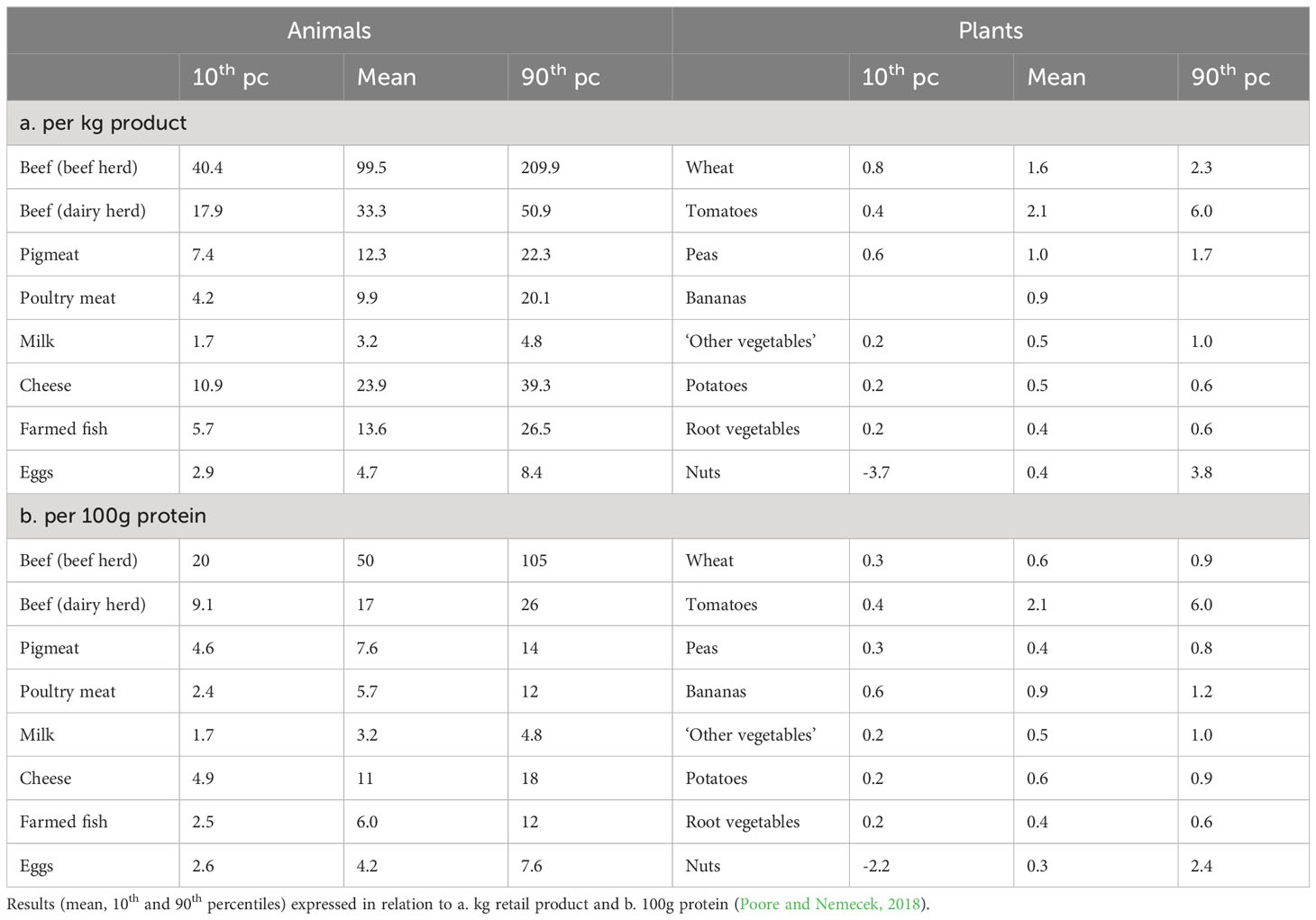
Table 2 Estimates of greenhouse gas emissions (kg CO2 equivalents) from production to retail of some agricultural products.
ADFs are major sources of protein in the diet and when expressed per 100g protein, the difference in emission intensity between animals and plants, when expressed per kg, narrows but is still substantial (Table 2). It is argued that expressing GHGEs per 100g protein still overestimates emissions from ADFs compared with plant foods and the unit of comparison should allow for the ideal balance of amino acids in ADFs, their inclusion of all the essential amino acids and their higher digestibility (McAuliffe et al., 2023). Others have suggested that emissions from ruminants should take account of the other valuable nutrients provided in their products such as calcium and iodine in milk (Hobbs et al., 2020) and B vitamins, iron, selenium and zinc in meat (Lee et al., 2021).
The global analysis by Poore and Nemecek (2018) (Table 2) showed that among the 38,700 farms studied, there was wide variation in GHGEs. It was estimated that for beef production from beef herds, 25% of producers were responsible for 56% of GHGEs. There is therefore much scope for mitigation of emissions through changes in practices on farms. In ruminant production there is a strong correlation between productivity and emission intensity so the greatest potential for mitigation is in systems operating at low productivity in areas such as South Asia, South America and Africa (Gerber et al., 2013; Rivero et al., 2021). Better feeding, herd management, animal health and genetics can all improve productivity and reduce emission intensity. An important characteristic of ruminants is their ability to utilise grass-based (forage) diets although production from grain-based (concentrate) diets results in greater productivity and lower GHGEs per kg. Improvements are also possible through changes in feed constituents which reduce methane production (e.g., ionophores, plant bioactive compounds, condensed tannins and fatty acids), changes to manure handling and carbon sequestration in which land use change is reduced and depleted pastures are replenished. Gerber et al. (2013) concluded that because of the high current costs to the environment of ruminant production, even modest improvements in systems can yield substantial gains in emission intensities and food security. Rivero et al. (2021), using data from a global network of farms, showed that key changes in genetic and nutritional approaches can make important contributions to the sustainability of global ruminant livestock production.
Efforts to reduce GHGEs in the production of ADFs has mainly focussed on ruminants, especially beef, because of the evidence that emissions from this group are so high (Table 2). Pigs, poultry and aquaculture are each responsible for about 7-10% of emissions from the livestock sector, mainly in the form of CO2 (from the production, processing and transport of feed) and N2O (from fertilizers and manure) (Gerber et al., 2013; MacLeod et al., 2020). Mitigation in these sectors involves the adoption of better practices, including reducing land use change (e.g., in the production of cereals and soyabeans) and improving efficiency (MacLeod et al., 2020; UN, 2021).
Springmann et al. (2018) modelled the effects of different mitigating influences on GHGEs from agriculture. The results showed that GHGEs could be reduced by 6% if food waste was halved, 10% if technological improvements were introduced on farms and by 29-56% if consumers changed their diets. The much lower environmental cost of vegetarian and vegan diets compared with meat-based diets was shown by Scarborough et al. (2023) in a UK study of 55,504 participants which linked food consumption and nutrient composition data with the environment indicators database of Poore and Nemecek (2018) (Table 2). The analysis accounted for variations in sourcing and production within each food group. The results for global warming potential (GWP), which accounts for emissions from CO2, N2O and methane are in Figure 1. They show that vegan diets had 24% of the GWP of the diets of high meat eaters (140g/d meat and meat products). The diets of low meat eaters (28.3g/d meat and meat products) had 52% of the GWP of those of high meat eaters. The emissions from the diets of low meat eaters were only slightly greater than those from fish eaters (who also consumed 2g meat and meat products per day).
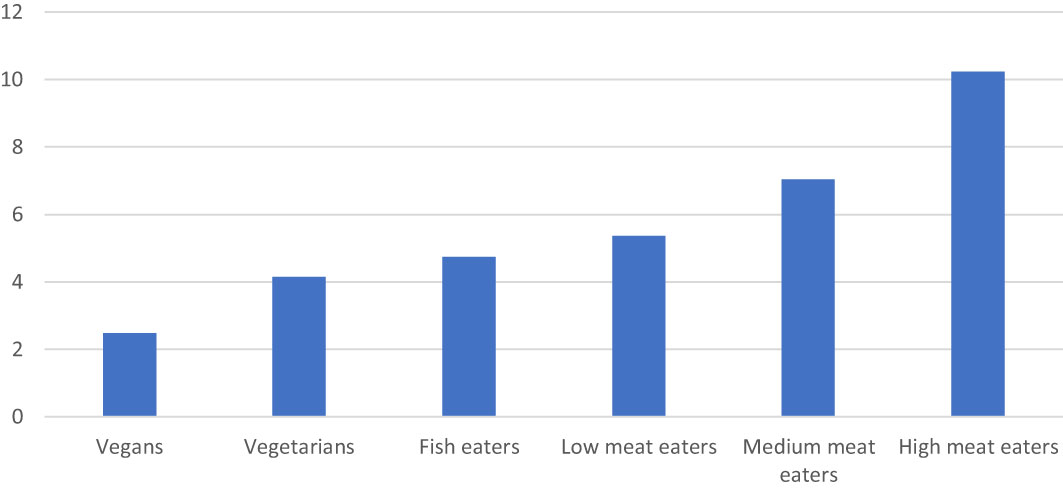
Figure 1 GHGEs (GWP 100 kg CO2 equivalents/day) associated with the diets of vegans, vegetarians, fish eaters and low, medium and high meat eaters in UK. 2.5th and 97.5th percentiles were 2.09-3.36 for vegans and 7.04-15.95 for high meat eaters (Scarborough et al., 2023).
Examples of diets modified to reduce GHGEs are given in WHO/FAO (2003), USDA (2020) and Willett et al. (2019). Groups in several countries have established ‘food-based dietary guidelines’ which call for substantial reductions in the consumption of ADFs, particularly in ruminant products (Steenson and Buttriss, 2021; Scarborough et al., 2023). Green et al. (2015) found in a modelling study that a reduction of 30% in GHGEs from UK diets was possible by reducing the contributions from some ADFs and switching between others. Reductions of more that 30% risked impairing nutritional value or required ‘non-trivial’ dietary shifts. Action at government level will be required to bring about these large changes in consumption and in UK, the Committee on Climate change has called for a 20% reduction in the consumption of beef, sheepmeat and dairy products by 2050 to help meet the UKs commitment to achieving ‘net zero’ (where emissions produced and removed from the atmosphere are in balance) by 2050 (CCC, 2020; Stewart et al., 2021).
Calls for reductions in the production and consumption of ADFs in high-income countries contrast with the projections that both production and consumption of ADFs in low- and middle-income countries will increase in the coming years as incomes and demand grow (OECD/FAO, 2023). There are also social considerations which suggest that strategies for dealing with climate change will differ between countries. In some low- and middle-income countries, livestock are required for income, transport and draught power and their ownership increases social inclusiveness (Parlasca and Qaim, 2022). The plant foods available in these countries are deficient in key nutrients such as iron, zinc and vitamin B12 which are provided in meat, milk and fish (Adesogan et al., 2020). It is also probable that the low levels of production and consumption of ADFs in some of these countries results in emissions which are compatible with climate targets (Springmann et al., 2018). Scarborough et al. (2023) suggested that the UK could meet its emissions reduction targets if consumption of ADFs fell to a level above that currently consumed in low-income countries.
In all countries, production from ruminants often occurs in areas which are unsuitable for other forms of agriculture because of topography and soil type. Lee et al. (2021) showed that land use suitable for human-edible food production was much lower for ruminant production than for the production of pigmeat and chicken when expressed in relation to the nutrients produced. Ruminants can also utilise forages (e.g., hay and silages), crop residues (e.g., cereal straw) and food industry by-products (e.g., sugar beet pulp and brewers grains) which are not suitable for other types of animal production or indeed for human food. These benefits of ruminant production are often not accounted for when assessing the overall costs (Van Kernebeek et al., 2016).
It must also be recognised that the alternative forms of protein production suggested to replace production from ruminants and other ADFs (e.g., plant-based foods, microbial protein, cell-cultured meat and insects) are at a relatively early stage of development and have not been fully evaluated regarding their environmental costs and chemical/nutritional profiles (Henchion et al., 2017). Steenson and Buttriss (2021) concluded that the environmental benefits of some meat and milk alternatives have been overstated, for example by underestimating their energy, water and land use costs.
4 Nutrients in ADFs and daily intakes
The different types of ADFs contain nutrients important for the maintenance of health as shown in Tables 3–5. Many databases exist and the data in the Tables 3–5 are from the UK database McCance and Widdowson’s Composition of Foods (Public Health England, 2021). The foods shown are chosen to represent the range of ADFs: lean muscle from red (beef) and white (chicken) meat, liver from beef and chicken representative of organ meats, white (cod) and oily (mackerel) fish, chicken eggs and whole and semi-skimmed milk. The amounts per 100g are compared with the reference intake and ‘significant amount’ figures published by the European Food Safety Authority (EFSA) (EU, 2011) for the purposes of food labelling and making nutrient claims. All the foods are ‘high in protein’ (Table 3) because they contain more than 20% of the energy as protein, ranging from 22% in whole milk to 93% in cod. The UK rolling National Diet and Nutrition Survey (NDNS) (Public Health England, 2020) shows that ADFs contribute about 59% of dietary protein intake in men and women aged 19-64. The protein is of high nutritional value, having a favourable balance of amino acids, including the nine essential amino acids and has high bioavailability from the diet in contrast to plant proteins (McAuliffe et al., 2023).
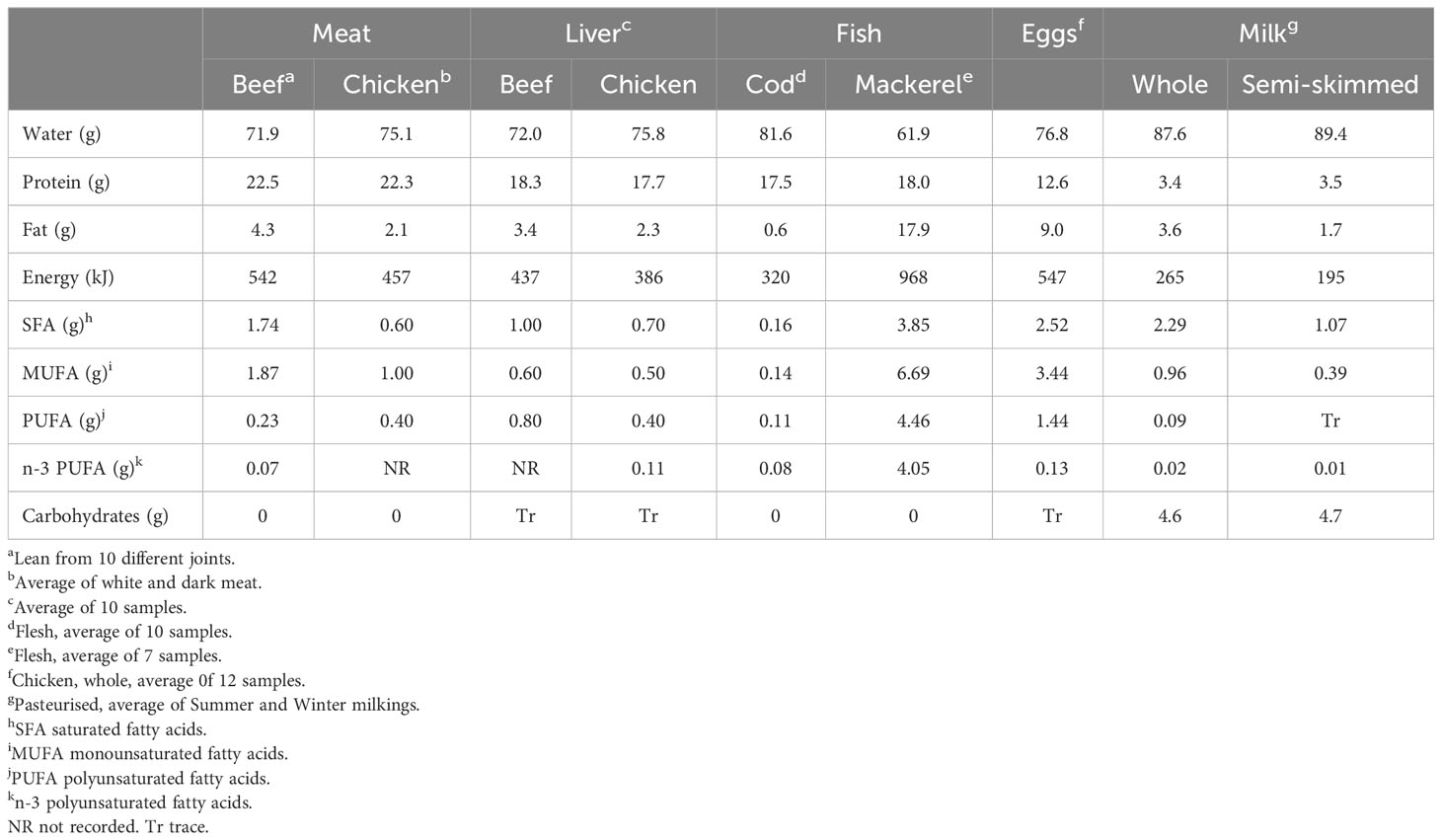
Table 3 Macronutrient content (per 100g) of some ADFs (Public Health England, 2021).
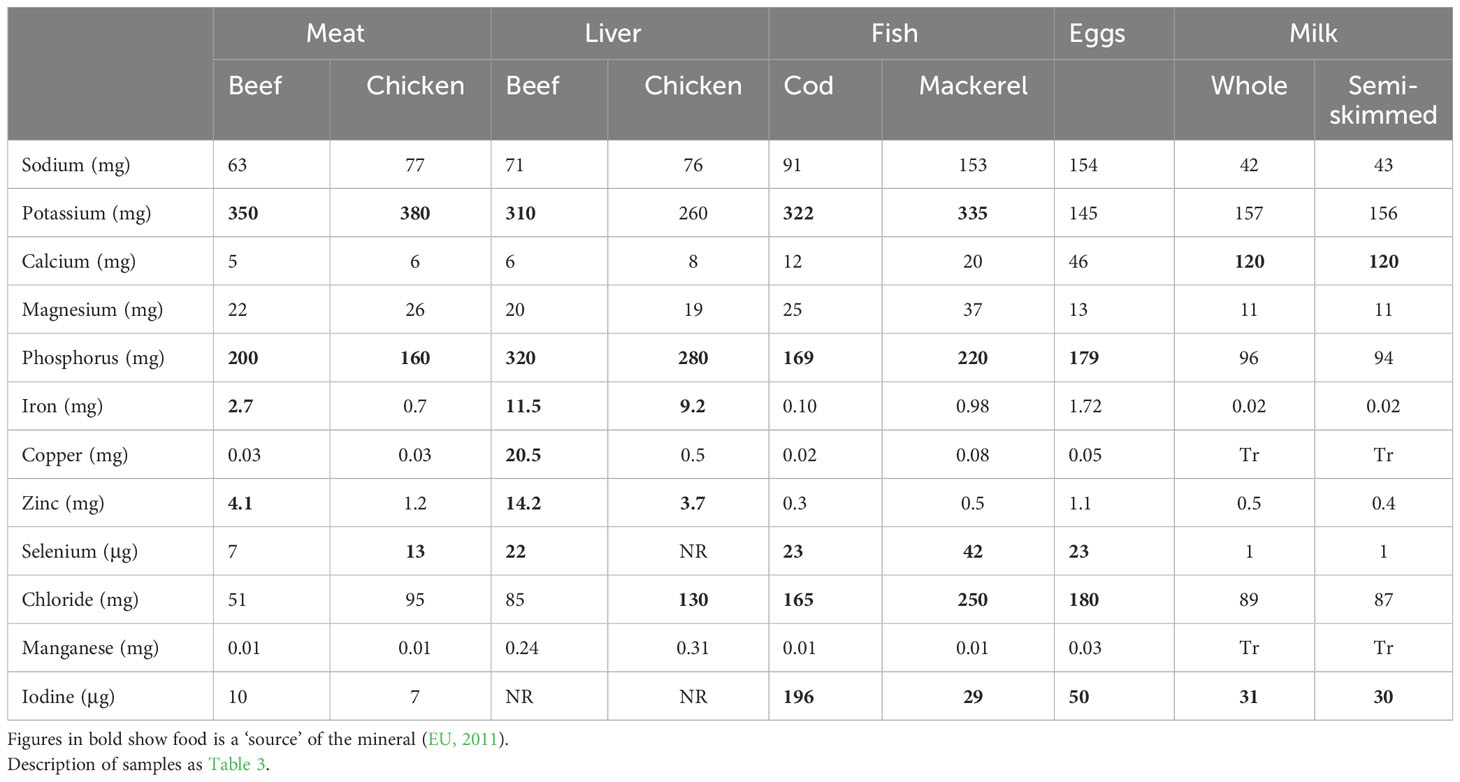
Table 4 Mineral content of some ADFs (Public Health England, 2021).
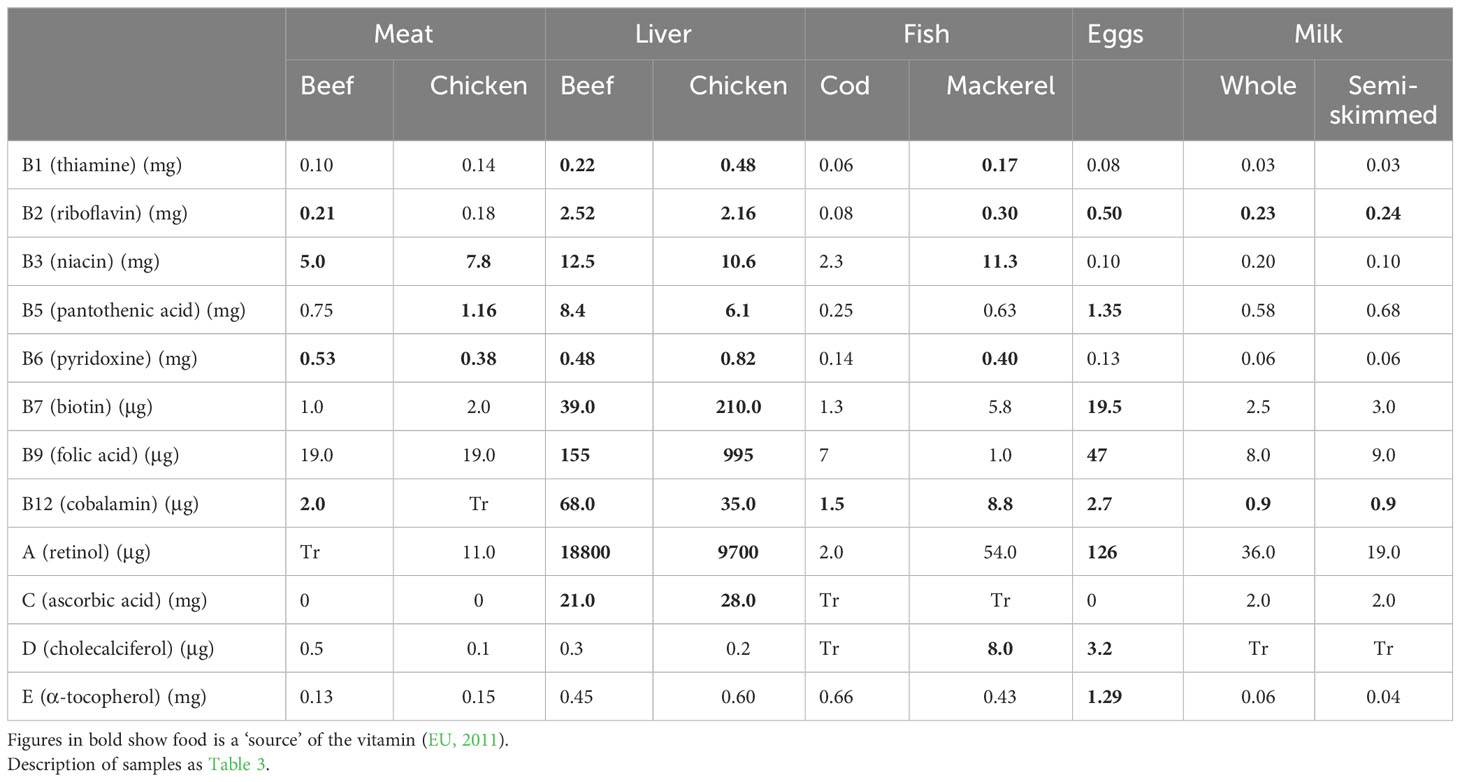
Table 5 Vitamin content (per 100g) of some ADFs (Public Health England, 2021).
Fat is a major source of energy in foods but over-consumption has been linked with obesity, coronary heart disease and some cancers (USDA, 2020). The samples of chicken muscle, chicken liver, cod and semi-skimmed milk in Table 3 are ‘low in fat’ (defined as <3g/100g for solids and <1.5g/100g for liquids). These same foods are ‘low in saturated fat’, saturated fat (SFA) being a risk factor for cardiovascular disease (CVD), the leading cause of mortality and morbidity worldwide (USDA, 2020), although the role of SFA in the aetiology of CVD is being increasingly challenged (Givens, 2023; Teicholz, 2023). The fat and SFA content of meat is greatly increased when adipose tissue is included in the food. For example, the sample of beef sausages listed in Public Health England (2021) has 19.5 g/100g fat and 7.6 g/100g SFA and is considered ‘high in fat’. The NDNS survey results show that men and women aged 19-64 obtain 49% and 56% of their fat and SFA intake, respectively, from ADFs (22% of fat and 21% SFA from meat and meat products) (Public Health England, 2020). The definition of ‘low energy’ foods in EU (2011) is <170 kJ/100g so none of the ADFs meet this threshold. Neither can any of the ADFs can be described as ‘high in’ MUFA or PUFA. The foods differ in the percentages of total fatty acids in the three main classes, beef muscle and milk (ruminant foods) having the highest percentages SFA and liver and fish the highest percentages of PUFA. Mackerel had the highest content of n-3 PUFA. About 60% of n-3 PUFA in mackerel are the long-chain fatty acids eicosapentaenoic acid (EPA) and docosahexaenoic acid (DHA) (Strobel et al., 2012) so 100g mackerel provides much more EPA+DHA than the 250mg/d recommended by EFSA (2017) or the 450mg/d recommended in UK (SACN, 2004) to benefit cardiovascular health. Most ADFs have little carbohydrate but both milk products contain intrinsic sugars – these do not contribute to ‘free sugar’ intake which is higher in most UK age groups than the government recommendations (Public Health England, 2020).
Table 4 lists the major minerals in foods and their concentrations in ADFs. There is concern over high intakes of sodium from salt in the diet because this is a risk factor for high blood pressure and CVD (Micha et al., 2012). All the raw ADFs except mackerel and eggs can be described as ‘low in salt’ (< 120mg/100g, EU, 2011). However, the sodium content of foods in which salt is used for curing can greatly exceed this value, for example the sample of cured bacon listed in Public Health England (2021) has 1140mg sodium/100g. The NDNS results show that ADFs provide about 46% of sodium intake for UK men and women in the 19-64 age groups, 26% from meat and meat products (Public Health England, 2020). For the other minerals, low intakes are of greater concern and concentrations which exceed the ‘significant amount’ or ‘source’ level, defined as 15% of the reference intake per 100g, are shown in bold. Phosphorus is at ‘source’ levels in seven of the nine ADFs, potassium, selenium and iodine in five, iron and zinc in three and calcium in two. The NDNS survey results show that ADFs make important contributions to UK intakes of several minerals. For the 19-64 age group, meat and meat products provide 31% of zinc, 29% of selenium and 19% of iron intakes (Public Health England, 2020). Milk and milk products provide 34% of calcium and 32% of iodine intakes. Fish and fish products provide 16% of selenium and 10% of iodine. Intakes of some minerals are considerably below the Lower Reference Nutrient Intake (LRNI) level in several groups in the population, particularly in girls aged 11-18 in which 49% had intakes below the LRNI for iron, 47% for magnesium, 37% for potassium, 41% for selenium, 16% for zinc and 28% for iodine (Public Health England, 2020). It is probable that low intakes of ADFs are responsible for these shortfalls (Givens, 2018) and there is concern that this cohort will become more deficient if ADF intakes are reduced further.
Iron deficiency is a major public health concern in many countries, including UK, in which 9% of girls aged 11-18 have iron deficiency anaemia (Fairweather-Tait, 2023). The haem iron in meat is more bioavailable than the iron in vegetables in which phytates and polyphenols may inhibit absorption. Haem iron concentrations in meat differ between the species. Lombardi-Boccia et al. (2002) found values of 2.64, 2.25, 0.56 and 0.42 mg/100g in beef sirloin, lamb chop, pork chop and chicken leg, respectively.
Table 5 lists the major vitamins in foods and their concentrations in ADFs. The figures in bold indicate the ADF contains a ‘significant amount’ or is a ‘source’ of the vitamin (EU, 2011). As with the minerals, all the ADFs have important levels of some vitamins, with beef and chicken liver being highest in all except vitamins D and E. Intakes of organ meats such as liver have declined in UK and other countries but their high content of micronutrients is invaluable when nutrient density is important, for example in young children, especially in low-income countries with poor access to plant sources of protein and micronutrients (Miller et al., 2023). The NDNS survey results show that ADFs contribute 83% of cobalamin (vitamin B12) and 70% of vitamin D intakes in the 19-64 age groups in UK, more than 50% of riboflavin and niacin and more than 30% of vitamin A, thiamine, pyridoxine and vitamin E (Public Health England, 2020). The results show that intakes of some vitamins are below the LRNI, especially in girls aged 11-18 and women aged 19-64. For these groups, riboflavin intake is particularly low, with 12-21% of participants below the LRNI in girls and women.
Measurement of serum 25-hydroxyvitamin D showed that in 2016-2019 in UK, 19% of children aged 11-18, 16% of adults aged 19-64 and 13% of adults over 65 had levels below which there is a risk to musculoskeletal health (Public Health England, 2020). USDA (2020) reported that 90% of Americans do not consume enough vitamin D. Again, low intakes of ADFs probably contributed to the UK shortfalls (Givens, 2018).
Animal-derived foods also contain many bioactive compounds present in small amounts that have a range of physiological and metabolic functions in the body which are not accounted for in standard nutritional studies. These bioactive compounds include creatine, taurine, carnitine, carnosine, choline, α-lipoic acid, conjugated linoleic acid, glutathione, coenzyme Q10 and bioactive peptides (Park and Nam, 2015; Kulczynski et al., 2019). The properties of these compounds include antioxidant, lipid-lowering, antihypertensive, anti-inflammatory and immunomodulatory effects. Peptides derived from hydrolysis of proteins and studied mainly in milk include the angiotensin 1-converting enzyme (ACE) inhibitors which are antihypertensive (Park and Nam, 2015).
Studies have shown that in some low- to middle-income countries, ADFs provide essential nutrients that are not present in the plant foods available (Adesogan et al., 2020; Parlasca and Qaim, 2022). ADFs, which in general are nutrient-dense, play a vital role in the nutrition of children, especially in the complementary feeding period (6-23 months) when nutrient requirements are high and gastric capacity low (Ortenzi and Beal, 2021). In some parts of the world, where diets are inadequate in several key nutrients, including iron, zinc, folate, vitamin B12 and calcium, ADFs can address the nutritional gaps (Miller et al., 2023). In a meta-analysis of randomised control trials, Pimpin et al. (2019) found that animal protein supplementation during infancy and early childhood increased child weight and height-for-age and reduced the risk of stunting. Nutrient deficiencies in early life can also lead to poor cognitive development and brain-related disorders which can influence health in adult life, increasing the risks of CVD and type-2 diabetes (Adesogan et al., 2020).
5 Consumption of ADFs and chronic disease
Reports from some international organisations have stated that the diets commonly consumed in many developed countries are associated with increased risks from obesity, CVD, type-2 diabetes and some cancers (WCRF, 2018; Willett et al., 2019; USDA, 2020). These bodies advocate changes in dietary patterns that address the nutritional causes of disease in addition to the climate change costs of food production. In many of the dietary patterns suggested, there is an important place for some ADFs (poultry, fish, eggs and low-fat milk) but lower intakes of red meat (beef, sheepmeat and pigmeat), and particularly processed meat, are advocated. The most extreme proposals are those of the EAT-Lancet Commission (Willett et al., 2019) whose ‘healthy reference diet’ contains 14g/day red meat (there is also 29g chicken, 13g eggs and 28g fish). This contrasts with the 50.5g/day intake of red and processed meat currently consumed in the UK (Public Health England, 2020). The WCRF (2018) report recommends ‘consumers eat no more than moderate amounts of red meat and little, if any, processed meat’ in 3 portions per week, amounting to 350-500g (50-71g/day).
The basis for these recommendations on health grounds is evidence from prospective cohort studies and meta-analyses of such studies showing that consumption of processed meat (red and white processed meat are usually not examined separately) is associated with mortality from CVD and all causes (Sinha et al., 2009; Rohrmann et al., 2013; Wang et al., 2016; Zhong et al., 2020; Iqbal et al., 2021). WCRF (2018) has concluded that processed meat is a convincing cause of colorectal cancer. Higher consumption of unprocessed red meat has been linked with CVD and all-cause mortality in some studies (Sinha et al., 2009; Zhong et al., 2020) but not others (Rohrmann et al., 2013; Iqbal et al., 2021). WCRF (2018) have concluded that red meat consumption is probably a cause of colorectal cancer but Lescinsky et al. (2022), noting high heterogeneity between studies, found a weak association between consumption of unprocessed red meat and colorectal cancer in their meta-analysis.
A wide range of meta-analyses of prospective studies indicate that consumption of milk and milk products has a neutral association with CVD risk overall, although some indicate a negative association with CVD, stroke in particular (summarised by Givens, 2023). In addition, there is increasing evidence that yoghurt consumption is associated with a reduced risk of type-2 diabetes (Gijsbers et al., 2016; Soedamah-Muthu and de Goede, 2018; Drouin-Chartier et al., 2019). The overall findings of a neutral or beneficial association of dairy foods with CVD from prospective cohort studies is counterintuitive to many, being contrary to the long-held hypothesis of the link between SFA consumption, LDL-cholesterol and atherosclerotic CVD. There are, however, a range of emerging factors in dairy foods which may explain this, some of which are independent of changes in blood lipids and some which moderate blood lipid effects (Givens, 2023). WCRF (2018) concluded that dairy consumption reduced the risk of colorectal cancer but indicated that there was limited-suggestive evidence that it increased the risk of prostate cancer.
No associations between poultry consumption and CVD or all-cause mortality have been found (Rohrmann et al., 2013; Abete et al., 2014; Zhong et al., 2020; Iqbal et al., 2021) and poultry consumption is not linked with colorectal cancer (WCRF, 2018). For fish consumption, Zhong et al. (2020) found no associations with CVD or all-cause mortality and Mohan et al. (2021) found a lower risk of CVD and overall mortality with a fish intake of 175g (2 servings) per week but only among people at risk of and having CVD, not in the general population. In a study of meat-eaters, fish-eaters and vegetarians, Key et al. (2014) found a lower risk of incident cancers in fish-eaters and vegetarians compared with meat-eaters. However, WCRF (2018), although noting limited evidence that fish consumption reduced the risks of liver and colorectal cancer, concluded that consumption of salted fish may increase the risk of nasopharyngeal cancer. Many studies have investigated the links between egg consumption, cholesterol consumption and the risk of CVD with some showing no risk except in individuals with type-2 diabetes (Rong et al., 2013; Guo et al., 2018; Drouin-Chartier et al., 2020) and some showing increased risks (Song et al., 2016; Zhong et al., 2019; Zhao et al., 2022). Although it is generally agreed that there is only a weak link between dietary and serum cholesterol, high cholesterol intakes can lead to plaque formation and inflammation, increasing the risk of CVD (Zhao et al., 2022).
Huang et al. (2022) reported high relative risks from egg protein in a prospective cohort study of 416,000 men and women with a follow-up period of 16 years. These authors examined the effects of replacing 3% protein from several animal sources (red meat protein, white meat protein, dairy protein and egg protein) with plant protein. Replacing white meat protein did not affect the risk of mortality overall or from cancer or CVD. The biggest reductions in these risks were seen by replacing egg protein.
In contrast to diets containing animal protein, diets containing fruit, vegetables, whole grains, nuts and fibre have been shown to be protective against the risks of cardiometabolic disease outcomes (Huang et al., 2022; Miller et al., 2022). Shah and Iyengar (2022) in a literature review found that a vegetarian plant-based diet was associated with a lower cancer risk than a ketogenic diet based on meat, dairy, fish and eggs. The ketogenic diet had some positive outcomes such as a reduction in obesity, inflammation and insulin levels.
Zhang et al. (2021) noted that globally, the prevalence of dementia is increasing, especially in low- and middle-income countries where meat consumption is also increasing. These authors investigated associations between meat consumption and the risk of dementia in the UK Biobank cohort and found that higher processed meat consumption increased the risk of dementia but increased red meat consumption reduced the risk. Trends were non-significant for poultry and all-meat.
In conclusion, evidence from several sources shows that higher intakes of processed meat are associated with mortality from CVD, colorectal cancer and possibly dementia and supports the suggestion that consumption of processed meat should be at a low level. The evidence is less clear for red meat and Rohrmann et al. (2013), who showed no links between red meat consumption and CVD or cancer in 448,568 people across 10 European countries, contrasted European results with those in the US where meat intake is higher. Also, some US studies have included processed meat within the red meat category (Sinha et al., 2009; Huang et al., 2022). Iqbal et al. (2021) conducted their study in 21 low-, middle- and high-income countries and found that a high daily consumption of red meat (>37g vs 7g) was not associated with all-cause or CVD mortality. Hur et al. (2019) showed that a high proportion of the evidence linking processed and red meat intake with colorectal cancer was from Western countries and that there is no evidence of these associations in Asian countries, including Korea, where rates of colorectal cancer are high. Possible explanations for this discrepancy between countries could be genetic variation and/or differences in the daily amounts of red and processed meat consumed, which have been higher over a long period in Western countries.
Poultry and fish consumption are not linked with CVD or cancer risks and are seen as possible replacements for some processed red meat protein in the diet. The general conclusion is that milk and milk products do not impact on CVD and some studies point to a protective effect of dairy products against colorectal cancer (WCRF, 2018). The data supports advice to maintain but not increase egg consumption but the risk of CVD mortality from extra egg consumption differed between country cohorts in the meta-analysis of Zhao et al. (2022). It was high in US cohorts (RR 1.08, 95% CI, 1.02-1.14), tended to be high in European cohorts (RR 1.05, 95% CI, 0.98-1.14) and was non-significant in Asian cohorts (RR 0.96, 95% CI, 0.87-1.06) (Zhao et al., 2022).
6 Constituents of ADFs and roles in disease
Several possible mechanisms underlying the effects of ADFs on chronic disease have been investigated. For processed meat, high concentrations of sodium, which increase blood pressure, could partly explain the higher risk of CVD (Micha et al., 2012). Nitrite/nitrate in cured meat can react with breakdown products of amino acids to form N-nitroso compounds which increase the risk of insulin resistance, CVD and cancer (Habermeyer et al., 2015). Haem iron catalyses the formation of N-nitroso compounds and increases their production in the gastro-intestinal tract (Sodring et al., 2022). Red meat consumption stimulates the production of N-nitroso compounds in the intestine but white meat, with a lower haem content, does not (Bingham et al., 2002). Both nitrite/nitrate and haem iron are pro-oxidants which can promote oxidative damage and inflammation in different organs (Etemadi et al., 2017). Another compound formed in the gut after the consumption of ADFs is trimethylamine N-oxide. Plasma concentrations of this choline metabolite have been shown to be positively associated with the risk of CVD and to have adverse effects on cholesterol metabolism and oxidative stress (Fretts et al., 2022).
The role of processed red meat in the development of colorectal cancer has been explained by the presence of many known mutagens including heterocyclic aromatic amines (HAA) and polycyclic aromatic hydrocarbons (PAH) produced during cooking (De Smet and Vossen, 2016). The N-nitroso compounds formed from haem iron in red meat are also carcinogenic. Hur et al. (2019) showed that the amounts and types of the N-nitroso compounds HAA and PAH depend on cooking methods and temperature and are sometimes found in other Korean foods at much higher levels than in processed meat. In a review, they found no evidence of correlations between the intakes of processed and red meat and the incidence of colorectal cancer in Asian studies.
Since the 1950s, long-chain (C12-C18) SFA in foods have been seen as risk factors for type-2 diabetes and CVD (WHO/FAO, 2003; USDA, 2020). Saturated fatty acids, particularly C14:0 and C16:0, raise total- and LDL-cholesterol levels in blood with a small HDL-raising effect. SFA increase coagulation, insulin resistance and inflammation, all of which increase the risk of type-2 diabetes and CVD (Calder, 2015). These effects are countered by PUFA and the correct balance of SFA to PUFA in the diet are considered important for cardiovascular health (Hooper et al., 2020).
Despite this evidence of the harmful effects of SFA, debate continues about their direct effects on CVD. Systematic reviews and meta-analyses of controlled trials have concluded there is no evidence to support the view that dietary SFA are associated with CVD (Siri-Tarino et al., 2010; Chowdhury et al., 2014). Others have concluded that advice from governments to limit SFA intake is unwarranted (Astrup et al., 2021; Teicholz, 2023). However, this advice continues to be given. For example, current UK government advice is to limit SFA to 10% of total dietary energy from the current 13% (Public Health England, 2020).
There is evidence that the source of SFA is important in relation to the effects on CVD. For example, the prospective cohort studies of de Oliveira Otto et al. (2012) and Vissers et al. (2019) showed a positive association between CVD risk and SFA from meat but not with SFA from dairy. These conclusions are supported by results from the meta-analysis of prospective cohort studies by Bechthold et al. (2019). In support of these results, Forouhi et al. (2014) and Prada et al. (2021) showed that plasma concentrations of the odd-chain SFA 15:0 and 17:0, which are markers for dairy fat intake, had inverse associations with the risk of type-2 diabetes. Perna and Hewlings (2023) showed that CVD risk was low or neutral for dietary levels of short and medium-chain fatty acids (C4-C10), which are also at higher concentrations in milk than meat.
Givens (2018) noted that hypertension is a risk factor for CVD and that bioactive peptides from milk proteins have hypotensive effects. The high concentration of calcium in dairy products may also reduce CVD risk (Lorenzen and Astrup, 2011).
The consensus view until recently has been that dietary cholesterol is not a factor in blood cholesterol concentrations (USDA, 2020) but recent cohort studies have found that increased egg consumption (0.5-1 egg/day) with its extra cholesterol, significantly increases the risk of CVD mortality (Zhong et al., 2019; Zhao et al., 2022).
Fish have many constituents which impact positively on health including high quality protein, minerals and vitamins (Tables 3–5) (Zheng et al., 2012). Their high content of long-chain n-3 fatty acids is also important. These fatty acids benefit many CVD risk factors including blood triglyceride levels, blood pressure, heart rate, endothelial function and myocardial oxygen demand (Del Gobbo et al., 2016) and have recently been associated with reduced CVD incidence and mortality over 10- and 20-year follow-up periods (Critselis et al., 2023). Randomised clinical trials of fish oil supplements have found mixed effects on CVD events but a meta-analysis of 13 trials concluded that supplementation with marine algae was associated with lower CVD mortality (RR 0.93, 95% CI, 0.88-0.99) and total CVD (RR 0.97, 95% CI, 0.94-0.99) (Hu et al., 2019). It is of concern that farmed salmon, the main oily fish consumed in the UK and elsewhere, has been shown to contain considerably lower concentrations of EPA+DHA (1.2g/100g, Henriques et al., 2014) than the value of 2.5g/100g used by SACN (2004) to set the UK dietary guidelines which would result in an EPA+DHA intake of 450mg/day.
7 Concluding remarks
Animal-derived foods have made important contributions to the diets of consumers over many years and are widely enjoyed as components of a balanced diet. However, high levels of consumption, especially of meat, are now widely seen as a factor in climate change and increasing levels of chronic disease.
Ruminant meat production poses the greatest risks to the environment, from GHGEs, water use, land use and eutrophication of water courses, but wide variation in these indicators suggests average costs can be lowered. The greater use of marginal land and lower use of arable land for beef and sheepmeat production in many countries are mitigating factors. Milk production from ruminants is associated with lower environmental costs than meat production and crossbred beef production from dairy herds lowers costs compared with purebred beef production. The costs of pigmeat, chicken, fish and egg production are lower than those of beef and sheepmeat but still higher than plant-based alternative sources of protein.
In terms of chronic disease, meat production from ruminants, especially when processed, poses the greatest risks. It is not clear how processing increases the risks of CVD or colorectal cancer: nitrites/nitrates, salt and carcinogens introduced during cooking have been implicated. Processed meat from chicken is probably less damaging than that from red meat but red and white processed meats have not been studied separately in most studies. Meat products containing low levels of nitrites/nitrates, salt and fat are now widely available.
Milk and milk products are associated with low or negative risks of CVD and cancer compared with beef and sheepmeat. The high haem content of ruminant meats could explain part of the disease risk and this poses the question why pigmeat is included in most studies as a red meat. Apart from a low haem content, it shares other characteristics with chicken, including a similar nutrient profile and similar balance between SFA and PUFA (Wood, 2023). Penkert et al. (2021) noted that the separate effects of pigmeat on health have been examined in very few studies.
The disease risks associated with chicken and fish are low but we draw attention to the risk of CVD from high egg consumption, with it’s extra cholesterol. Chicken has had the greatest success among ADFs and is forecast to provide 41% of protein from meat sources globally by 2030.
The reductions in the consumption of red and processed meat called for in some reports to improve environmental and health outcomes are substantial. The EAT-Lancet Commission called for a greater than 50% reduction in the global consumption of red meat (Willett et al., 2019). Governments respond to such advice in different ways. The UK Government recommended consumption of 70g/day red and processed meat in the Eatwell Guide and present figures are around 50.5g/day (Stewart et al., 2021). The figure of 14g red meat per day included by Willett et al. (2019) in their ‘healthy reference diet’ would be hard to achieve. The assumption that red and processed meat will be replaced in diets by vegetables, fruit, beans and nuts (Willett et al., 2019) may also be problematic. Benefits to health and the environment would not occur if consumers switched to alternatives like ultra-processed foods, sales of which have recently increased (Srour et al., 2019). The use of a ‘ruminant meat tax’ to limit consumption would be difficult politically and could result in unexpected costs (Lee et al., 2021).
Calls for lower levels of ADF consumption in high-income countries contrast with the increases in consumption predicted in low- and middle-income countries in the next 10 years. Some reports have shown lower risks of CVD and colorectal cancer associated with consumption of ADFs in these countries. This may be due to lower levels of consumption of ADFs and as these increase the risks may increase.
This overview has outlined the current (2023-24) position of ADFs as foods in our diets and has described the challenges facing their production. This is a rapidly advancing story for society and the agricultural industry and we await further developments.
Author contributions
JW: Writing – original draft. CG: Writing – original draft. DIG: Writing – original draft.
Funding
The author(s) declare that no financial support was received for the research, authorship, and/or publication of this article.
Conflict of interest
The authors declare that the research was conducted in the absence of any commercial or financial relationships that could be construed as a potential conflict of interest.
The author(s) declared that they were an editorial board member of Frontiers, at the time of submission. This had no impact on the peer review process and the final decision.
Publisher’s note
All claims expressed in this article are solely those of the authors and do not necessarily represent those of their affiliated organizations, or those of the publisher, the editors and the reviewers. Any product that may be evaluated in this article, or claim that may be made by its manufacturer, is not guaranteed or endorsed by the publisher.
References
Abete I., Romaguera D., Vieira A. R., de Munain A. L., Norat T. (2014). Association between total, processed, red and white meat consumption and all-cause, CVD and IHD mortality: a meta-analysis of cohort studies. Br. J. Nutr. 112, 762–775. doi: 10.1017/S000711451400124X
Adesogan A. T., Havelaar A. H., McKune S. L., Eilittä M., Dahl G. E. (2020). Animal source foods: Sustainability problem or malnutrition and sustainability solution? Perspective matters. Global Food Secur. 25, 100325. doi: 10.1016/j.gfs.2019.100325
Astrup A., Teicholz N., Magkos F., Bier D. M., Brenna J. T., King J. C., et al. (2021). Dietary saturated fats and health: Are the US guidelines evidence-based? Nutrients 13, 3305. doi: 10.3390/nu13103305
Auestad N., Hurley J. S., Fulgoni V. L., Schweitzer C. M. (2015). Contribution of food groups to energy and nutrient intakes in five developed countries. Nutrients 7, 4593–4618. doi: 10.3390/nu7064593
Bechthold A., Boeing H., Schwedhelm C., Hoffmann G., Sven Knuppel S., Iqbal K., et al. (2019). Food groups and risk of coronary heart disease, stroke and heart failure: A systematic review and dose-response meta-analysis of prospective studies. Crit. Rev. Food Sci. Nutr. 59, 1071–1090. doi: 10.1080/10408398.2017.1392288
Bingham S. A., Hughes R., Cross A. J. (2002). Effect of white versus red meat on endogenous N-nitrosation in the human colon and further evidence of a dose response. J. Nutr. 132, 3522S–3525S. doi: 10.1093/jn/132.11.3522S
Calder P. C. (2015). Functional roles of fatty acids and their effects on human health. J. Parenteral Enteral Nutr. 39, 18S–32S. doi: 10.1177/0148607115595980
CCC (Committee on Climate Change) (2020) Land use: policies for a net zero UK. Available online at: https://www.theccc.org.uk/publication/land-use-policies-for-a-net-zero-uk.
Chowdhury R., Warnakula S., Kunutsor S., Crowe F., Ward H. A., Johnson L., et al. (2014). Association of dietary, circulating, and supplement fatty acids with coronary risk. A systematic review and meta-analysis. Ann. Internal Med. 160, 398–406. doi: 10.7326/M13-1788
Crippa M., Solazzo E., Guizzardi D., Monforti-Ferrario F., Leip A. (2021). Food systems are responsible for a third of global anthropogenic GHG emissions. Nat. Food. 2, 198–209. doi: 10.1038/s43016-021-00225-9
Critselis E., Tsiampalis T., Damigou E., Georgousopoulou E., Barkas F., Chrysohoou C., et al. (2023). High fish intake rich in n-3 polyunsaturated fatty acids reduces cardiovascular disease incidence in healthy adults: The ATTICA cohort study, (2002-2022). Front. Physiol. 14, 1158140. doi: 10.3389/fphys.2023.1158140
Del Gobbo L. C., Imamura F., Aslibekyan S., Marklund M., Virtanen J. K., Wennberg M., et al. (2016). ω-3 polyunsaturated fatty acid biomarkers and coronary heart disease. Pooling project of 19 cohort studies. JAMA Internal Med. 176, 1155–1166. doi: 10.1001/jamainternmed.2016.2925
de Oliveira Otto M. C., Mozaffarian D., Kromhout D., Bertoni A. G., Sibley C. T., Jacobs D. R., et al. (2012). Dietary intake of saturated fat by food source and incident cardiovascular disease: the Multi-Ethnic Study of Atherosclerosis. Am. J. Clin. Nutr. 96, 397–404. doi: 10.3945/ajcn.112.037770
De Smet S., Vossen E. (2016). Meat: The balance between nutrition and health. A review Meat Sci. 120, 145–156. doi: 10.1016/j.meatsci.2016.04.008
Drouin-Chartier J. P., Chen S., Li Y., Schwab A. L., Stampfer M. J., Sacks F. M., et al. (2020). Egg consumption and risk of cardiovascular disease: three large prospective US cohort studies, systematic review and updated meta-analysis. BMJ 368, m513. doi: 10.1136/bmj.m513
Drouin-Chartier J.-P., Li Y., Korat A. V. A., Ding M., Lamarche B., Manson J. E., et al. (2019). Changes in dairy product consumption and risk of type 2 diabetes: results from 3 large prospective cohorts of US men and women. Am. J. Clin. Nutr. 110, 1201–1212. doi: 10.1093/ajcn/nqz180
EFSA (European Food Standards Authority) (2017). Dietary Reference Values for Nutrients. Summary Report (Parma, Italy: EFSA Supporting Publication), 98. doi: 10.2903/sp.efsa.2017.e15121
Etemadi A., Sinha R., Ward M. H., Graubard B. I., Inoue-Choi M., Dawsey S. M., et al. (2017). Mortality from different causes associated with meat, heme iron, nitrates, and nitrites in the NIH-AARP Diet and Health Study: population -based cohort study. BMJ 357, j1957. doi: 10.1136/bmj.j1957
EU (European Union) (2011). Commission Regulation (EU) No. 1169/2011 of the European Parliament and of the Council of 25 October 2011 on the provision of food information to consumers. Off. J. Eur. Union 304, 18–63.
Fairweather-Tait S. (2023). The role of meat in iron nutrition of vulnerable groups of the UK population. Front. Anim. Sci. 4. doi: 10.3389/fanim.2023.1142252
FAO (Food and Agriculture Organisation of the United Nations) (2021). Available online at: http://www.fao.org/faostat/en/#data/FBS.
Forouhi N. G., Koulman A., Sharp S. J., Imamura F., Kroger J., Schulze M. B., et al. (2014). Differences in the prospective association between individual plasma phospholipid saturated fatty acids and incident type 2 diabetes: the EPIC-InterAct case-cohort study. Lancet Diabetes Endocrinol. 2, 810–818. doi: 10.1016/S2213-8587(14)70146-9
Frank D., Oytam Y., Hughes J., McDonnell C. K., Bucklow R. (2022). “Sensory perceptions and new consumer attitudes to meat,” in New Aspects of Meat Quality. Ed. Purslow P. P. (Woodhead Publishing Ltd, Cambridge), 853–886.
Fretts A. M., Hazen S. L., Jensen P., Budoff M., Sitlani C. M., Wang M., et al. (2022). Association of trimethylamine N-oxide and metabolites with mortality in older adults. JAMA Network Open 5, e2213242. doi: 10.1001/jamanetworkopen.2022.13242
Gerber P. J., Steinfeld H., Henderson B., Mottet A., Opio C., Dijkman J., et al. (2013). Tackling climate change through livestock – A global assessment of emissions and mitigation opportunities (Rome: Food and Agriculture Organization of the United Nations (FAO).
Gijsbers L., Ding E. L., Malik V. S., de Goede J., Geleijnse J. M., Soedamah-Muthu S. S. (2016). Consumption of dairy foods and diabetes, incidence: a dose-response meta-analysis of observational studies. Am. J. Clin. Nutr. 103, 1111–1124. doi: 10.3945/ajcn.115.123216
Givens D. I. (2018). Review: Dairy foods, red meat and processed meat in the diet: implications for health at key life stages. Animal 12, 1709–1721. doi: 10.1017/S1751731118000642
Givens D. I. (2023). Dairy foods and cardiometabolic diseases: an update and a reassessment of the impact of saturated fatty acids. Proc. Nutr. Society 82, 320–345. doi: 10.1017/S0029665123000083
Green R., Milner J., Dangour A. D., Haines A., Chalabi Z., Markandya A., et al. (2015). The potential to reduce greenhouse gas emissions in the UK through healthy and realistic dietary change. Climatic Change 129, 253–265. doi: 10.1007/s10584-015-1329-y
Guo J., Hobbs D. A., Cockcroft J. R., Elwood P. C., Pickering J. E., Lovegrove J. A., et al. (2018). Association between egg consumption and cardiovascular disease events, diabetes and all-cause mortality. Eur. J. Nutr. 57, 2943–2952. doi: 10.1007/s00394-017-1566-0
Habermeyer M., Roth A., Guth A., Diel P., Engel K-H., Epe B., et al. (2015). Nitrate and nitrite in the diet: how to assess their benefit and risk for human health. Mol. Nutr. Food Res. 59, 106–128. doi: 10.1002/mnfr.201400286
Henchion M., Hayes M., Mullen A. M., Fenelon M., Brijesh Tiwari B. (2017). Future protein supply and demand: strategies and factors influencing a sustainable equilibrium. Foods 6, 53. doi: 10.3390/foods6070053
Henriques J., Dick J. R., Tocher D. R., Bell J. G. (2014). Nutritional quality of salmon products available from major retailers in the UK: content and composition of n-3 long-chain PUFA. Br. J. Nutr. 112, 964–975. doi: 10.1017/S0007114514001603
Henry B. K., Eckard R. J., Beauchemin K. A. (2018). Review: Adaptation of ruminant livestock production systems to climate changes. Animal 12, s445–s456. doi: 10.1017/S1751731118001301
Hobbs D. A., Durrant C., Elliott J., Givens D. I., Lovegrove J. A. (2020). Diets containing the highest levels of dairy products are associated with greater eutrophication potential but higher nutrient intakes and lower financial cost in the United Kingdom. Eur. J. Nutr. 59, 895–908. doi: 10.1007/s00394-019-01949-y
Hooper L., Martin N., Jimoh O. F., Kirk C., Foster E., Abdelhamid A. S. (2020). Reduction in saturated fat intake for cardiovascular disease. Cochrane Database Systematic Rev. (8), CD011737.
Hu Y., Hu F. B., Manson J. E. (2019). Marine omega-3 supplementation and cardiovascular disease: an updated meta-analysis of 13 randomized control trials involving 127 477 participants. J. Am. Heart Assoc. 119, 013543. doi: 10.1161/JAHA.119.013543
Huang J., Liao L. M., Weinstein S. J., Sinha R., Graubard B. I., Albanes D. (2022). Association between plant and animal protein intake and overall and cause-specific mortality. JAMA Internal Med. 180, 1173–1184. doi: 10.1001/jamainternmed.2020.2790
Hur S. J., Jo C., Yoon Y., Jeong J. Y., Lee K. T. (2019). Controversy on the correlation of red and processed meat consumption with colorectal cancer risk: an Asian perspective. Crit. Rev. Food Sci. Nutr. 59, 3526–3537. doi: 10.1080/10408398.2018.1495615
Iqbal R., Dehghan M., Mente A., Rangarajan S., Wielgosz A., Avezum A., et al. (2021). Associations of unprocessed and processed meat intake with mortality and cardiovascular disease in 21 countries [Prospective Urban Rural Epidemiology (PURE) Study]: a prospective cohort study. Am. J. Clin. Nutr. 114, 1049–1058. doi: 10.1093/ajcn/nqaa448
Key T. J., Appleby P. N., Crowe F. L., Bradbury K. E., Schmidt J. A., Travis R. C. (2014). Cancer in British vegetarians: updated analyses of 4998 incident cancers in a cohort of 32,491 meat eaters 8612 fish eaters, 18,298 vegetarians, and 2246 vegans. Am. J. Clin. Nutr. 100, 378S–385S. doi: 10.3945/ajcn.113.071266
Kulczynski B., Sidor A., Gramza-Michałowska A. (2019). Characteristics of selected antioxidative and bioactive compounds in meat and animal origin products. Antioxidants 8, 335. doi: 10.3390/antiox8090335
Lee M. R. F., Domingues J. P., McAuliffe G. A., Tichit M., Accatino F., Takahashi T. (2021). Nutrient provision capacity of alternative livestock farming systems per area of arable farmland required. Sci. Rep. 11, 14975. doi: 10.1038/s41598-021-93782-9
Lescinsky H., Afshin A., Ashbaugh C., Bisignano C., Brauer M., Ferrara G., et al. (2022). Health effects associated with consumption of unprocessed red meat: a Burden of Proof study. Nat. Med. 28, 2075–2082. doi: 10.1038/s41591-022-01968-z
Lombardi-Boccia G., Martinez-Dominguez B., Aguzzi A. (2002). Total heme and non-heme iron in raw and cooked meats. J. Food Sci. 67, 1738–1741.
Lorenzen J. K., Astrup A. (2011). Dairy calcium intake modifies responsiveness of fat metabolism and blood lipids to a high-fat diet. Br. J. Nutr. 105, 1823–1831. doi: 10.1017/S0007114510005581
MacLeod M. J., Hasan M. R., Robb D. H. F., Rashid M. M. U. (2020). Quantifying greenhouse gas emissions from global aquaculture. Sci. Rep. 10, 11679. doi: 10.1038/s41598-020-68231-8
McAuliffe G. A., Takahashi T., Beal T., Huppertz T., Leroy F., J. Buttriss J., et al. (2023). Protein quality as a complementary functional unit in life cycle assessment (LCA). Int. J. Life Cycle Assessment 28, 146–155. doi: 10.1007/s11367-022-02123-z
Micha R., Michas G., Mozaffarian D. (2012). Unprocessed red and processed meats and risk of coronary artery disease and type 2 diabetes – an updated review of the evidence. Curr. Atheroscl. Rep. 14, 515–524. doi: 10.1007/s11883-012-0282-8
Miller V., Micha R., Choi E., Karageorgou D., Webb P., Mozaffarian D. (2022). Evaluation of the quality of evidence of the association of foods and nutrients with cardiovascular disease and diabetes. A systematic review. JAMA Network Open 5, e2146705. doi: 10.1001/jamanetworkopen.2021.46705
Miller V., Webb P., Cudhea F., Zhang J., Reedy J., Shi P., et al. (2023). Children’s and adolescents’ rising animal-source food intakes in 1990–2018 were impacted by age, region, parental education and urbanicity. Nat. Food. 4, 305–319. doi: 10.1038/s43016-023-00731-y
Mohan D., Mente A., Dehghan M., Rangarajan S., O’Donnell M., Hu W., et al. (2021). Associations of fish consumption with risk of cardiovascular disease and mortality among individuals with or without vascular disease from 58 Countries. JAMA Internal Med. 181, 631–649. doi: 10.1001/jamainternmed.2021.0036
OECD-FAO (Organisation of Economic Cooperation and Development-Food and Agriculture Organisation of the United Nations) (2023). Agricultural Outlook 2022-2032 (Paris: OECD Publishing).
Ortenzi F., Beal T. (2021). Priority micronutrient density of foods for complementary feeding of young children (6–23 months) in south and Southeast Asia. Front. Nutr. 8, 785227. doi: 10.3389/fnut.2021.785227
Park Y. W., Nam M. S. (2015). Bioactive peptides in milk and dairy products: a review. Korean J. Food Sci. Anim. Resources 35, 831–840. doi: 10.5851/kosfa.2015.35.6.831
Parlasca M. C., Qaim M. (2022). Meat consumption and sustainability. Annu. Rev. Resource Economics 14, 17–41. doi: 10.1146/annurev-resource-111820-032340
Penkert L. P., Ruogu L., Huang J., Gurcan A., Chung M., Wallace T. C. (2021). Pork consumption and its relationship to human nutrition and health: a scoping review. Meat Muscle Biol. 5, 1–22. doi: 10.22175/mmb.12953
Perna M., Hewlings S. (2023). Saturated fatty acid chain length and risk of cardiovascular disease: A systematic review. Nutrients 15, 30.
Pimpin L., Kranz S., Liu E., Shulkin M., Karageorgou D., Miller V., et al. (2019). Effects of animal protein supplementation of mothers, preterm infants, and term infants on growth outcomes in childhood: a systematic review and meta-analysis of randomized trials. Am. J. Clin. Nutr. 110, 410–429. doi: 10.1093/ajcn/nqy348
Poore J., Nemecek T. (2018). Reducing food’s environmental impacts through producers and consumers. Science 360, 987–992. doi: 10.1126/science.aaq0216
Prada M., Wittenbecher C., Eichelmann F., Wernitz A., Drouin-Chartier J.-P., Schulze M. B. (2021). Association of the odd-chain fatty acid content in lipid groups with type 2 diabetes risk: A targeted analysis of lipidomics data in the EPIC-Potsdam cohort. Clin. Nutr. 40, 4988–4999. doi: 10.1016/j.clnu.2021.06.006
Public Health England (2020). National Diet and Nutrition Survey Rolling Programme Years 9 to 11 (2016/2017 to 2018/2019) (London: Public Health England).
Public Health England (2021). McCance and Widdowson’s The Composition of Foods. Integrated Dataset (London: Public Health England).
Rivero M. J., Lopez-Villalobos N., Evans A., Berndt A., Cartmill A., Neal A. L., et al. (2021). Key traits for ruminant livestock across diverse production systems in the context of climate change: perspectives from a global platform of research farms. Reproduction Fertility Dev. 33, 1–19. doi: 10.1071/RD20205
Rohrmann S., Overvad K., Bueno-de-Mesquita H. B., Jakobsen M. U., Egeberg R., Tjønneland A., et al. (2013). Meat consumption and mortality - results from the European Prospective Investigation into Cancer and Nutrition. BMC Med. 11, 63–75. doi: 10.1186/1741-7015-11-63
Rong Y., Chen L., Zhu T., Song Y., Yu M., Shan Z., et al. (2013). Egg consumption and risk of coronary heart disease and stroke: dose-response meta-analysis of prospective cohort studies. BMJ 346, e8539. doi: 10.1136/bmj.e8539
SACN (Scientific Advisory Committee on Nutrition) (2004). Advice on fish consumption: benefits and risks (London: The Stationery Office).
Scarborough P., Clark M., Cobiac L., Papier K., Knuppel A., Lynch J., et al. (2023). Vegans, vegetarians, fish-eaters and meat-eaters in the UK show discrepant environmental impacts. Nat. Food. 4, 565–574. doi: 10.1038/s43016-023-00795-w
Shah U. A., Iyengar N. M. (2022). Plant-based and ketogenic diets as diverging paths to address cancer. A Review. JAMA Oncol. 8, 1201–1208. doi: 10.1001/jamaoncol.2022.1769
Sinha R., Cross A. J., Graubard B. I., Leitzmann M. F., Schatzkin A. (2009). Meat intake and mortality: A prospective study of over half a million people. Arch. Intern. Med. 169, 562–571. doi: 10.1001/archinternmed.2009.6
Siri-Tarino P. W., Sun Q., Hu F. B., Krauss R. M. (2010). Meta-analysis of prospective cohort studies evaluating the association of saturated fat with cardiovascular disease. Am. J. Clin. Nutr. 91, 535–546. doi: 10.3945/ajcn.2009.27725
Sodring M., Dragsted L. O., Muller M. H. B., Paulsen J. E., Haug A., Egelandsdal B. (2022). “Meat and cancer evidence for and against,” in New Aspects of Meat Quality. Ed. Purslow P. P. (Woodhead Publishing Ltd, Cambridge), 579–607.
Soedamah-Muthu S. S., de Goede J. (2018). Dairy consumption and cardiometabolic diseases: systematic review and updated meta-analyses of prospective cohort studies. Curr. Nutr. Rep. 7, 171–182. doi: 10.1007/s13668-018-0253-y
Song M., Fung T. T., Hu F. B., Willett W. C., Longo V. D., Chan A. T., et al. (2016). Association of animal and plant protein intake with all-cause and cause-specific mortality. JAMA Internal Med. 176, 1453–1463. doi: 10.1001/jamainternmed.2016.4182
Springmann M., Clark M., Mason-D’Croz D., Wiebe K., Bodirsky B. L., Lassaletta L., et al. (2018). Options for keeping the food system within environmental limits. Nature 562, 519–525. doi: 10.1038/s41586-018-0594-0
Srour B., Fezeu L. K., Kesse-Guyot E., Alles B., Mejean C., Andrianasolo R. M., et al. (2019). Ultra-processed food intake and risk of cardiovascular disease:prospective cohort study (NutriNet-Santé). BMJ 365, l1451. doi: 10.1136/bmj.l1451
Steenson S., Buttriss J. L. (2021). Healthier and more sustainable diets: What changes are needed in high-income countries? Nutr. Bulletin 46, 279–309. doi: 10.1111/nbu.12518
Stewart C., Piernas C., Cook B., Jebb S. A. (2021). Trends in UK meat consumption: analysis of data from years 1–11 (2008–09 to 2018–19) of the National Diet and Nutrition Survey rolling programme. Lancet Planet Health 5, e699–e708. doi: 10.1016/S2542-5196(21)00228-X
Strobel C., Jahreis G., Kuhnt K. (2012). Survey of n-3 and n-6 polyunsaturated fatty acids in fish and fish products. Lipids Health Disease 11, 144–154. doi: 10.1186/1476-511X-11-144
Teicholz N. (2023). A short history of saturated fat: the making and unmaking of a scientific consensus. Curr. Opin. Endocrinol. Diabetes Obes. 30, 65–71. doi: 10.1097/MED.0000000000000791
UN (United Nations Environment Programme and Climate and Clean Air Coalition) (2021). Global Methane Assessment: Benefits and Costs of Mitigating Methane Emissions (Nairobi: United Nations Environment Programme).
USDA (United States Department of Agriculture) (2020). Scientific Report of the 2020 Dietary Guidelines Advisory Committee. U.S. Department of Agriculture (Washington, DC: Agricultural Research Service).
Van Kernebeek H. R. J., Oosting S. J., Van Ittersum M. K., Bikker P., De Boer I. J. M. (2016). Saving land to feed a growing population: consequences for consumption of crop and livestock products. Int. J. Life Cycle Assessment 21, 677–687. doi: 10.1007/s11367-015-0923-6
Vissers L. E. T., Rijksen J., Boer J. M. A., Verschuren W. M. M., van der Schouw Y. T., Sluijs I. (2019). Fatty acids from dairy and meat and their association with risk of coronary heart disease. Eur. J. Nutr. 58, 2639–2647. doi: 10.1007/s00394-018-1811-1
Wang X., Lin X., Ouyang Y. Y., Liu J., Zhao G., Pan A., et al. (2016). Red and processed meat consumption and mortality: dose–response meta-analysis of prospective cohort studies. Public Health Nutr. 19, 893–905. doi: 10.1017/S1368980015002062
WCRF (World Cancer Research Fund). American Institute for Cancer Research (2018). Diet, Nutrition, Physical Activity and Cancer (London, UK: A Global Perspective. Continuous Update Project Expert Report. World Cancer Research Fund).
WHO/FAO (World Health Organisation/Food and Agriculture Organisation of the United Nations) (2003). Diet, nutrition and the prevention of chronic diseases: report of a joint WHO/FAO expert consultation (Geneva: World Health Organisation).
Willett W., Rockström J., Loken B., Springmann M., Lang T., Vermeulen S., et al. (2019). Food in the Anthropocene: the EAT–Lancet Commission on healthy diets from sustainable food systems. Lancet 393, 447–492. doi: 10.1016/S0140-6736(18)31788-4
Wood J. D. (2023). “Meat composition and nutritional value” in Lawrie’s Meat Science 9th edition. Ed. Toldra F. (Cambridge: Woodhead Publishing Ltd), 665–685.
YouGov (2023). Dietary choices of Brits (eg vegetarian, flexitarian, meat-eater etc) (London: YouGov poll. Biannual tracker. YouGov PLC).
Zhang H., Greenwood D. C., Risch H. A., Bunce D., Hardie L. J., Cade J. E. (2021). Meat consumption and risk of incident dementia: cohort study of 493,888 UK Biobank participants. Am. J. Clin. Nutr. 114, 175–184. doi: 10.1093/ajcn/nqab028
Zhao B., Gan L., Graubard B. I., Männistö S., Albanes D., Huang J. (2022). Associations of dietary cholesterol, serum cholesterol, and egg consumption with overall and cause-specific mortality: systematic review and updated meta-analysis. Circulation 145, 1506–1520. doi: 10.1161/CIRCULATIONAHA.121.057642
Zheng J., Huang T., Yu Y., Hu X., Yang B., Li D. (2012). Fish consumption and CHD mortality: an updated meta-analysis of seventeen cohort studies. Public Health Nutr. 15, 725–737. doi: 10.1017/S1368980011002254
Zhong V. W., Van Horn L., Cornelis M. C., Wilkins J. T., Ning H., Carnethon M. R., et al. (2019). Associations of dietary cholesterol or egg consumption with incident cardiovascular disease and mortality. JAMA 321, 1081–1095. doi: 10.1001/jama.2019.1572
Zhong V. W., Van Horn L., Greenland P., Carnethon M. R., Ning H., Wilkins J. T., et al. (2020). Associations of processed meat, unprocessed red meat, poultry, or fish intake with incident cardiovascular disease and all-cause mortality. JAMA Internal Med. 180, 503–512. doi: 10.1001/jamainternmed.2019.6969
Keywords: red meat, white meat, fish, eggs, milk, milk products, global warming, cardiovascular disease
Citation: Wood JD, Giromini C and Givens DI (2024) Animal-derived foods: consumption, composition and effects on health and the environment: an overview. Front. Anim. Sci. 5:1332694. doi: 10.3389/fanim.2024.1332694
Received: 03 November 2023; Accepted: 15 February 2024;
Published: 01 March 2024.
Edited by:
Marzena Helena Zajac, University of Agriculture in Krakow, PolandReviewed by:
Piotr Kulawik, University of Agriculture in Krakow, PolandMonika Modzelewska-Kapituła, University of Warmia and Mazury in Olsztyn, Poland
Copyright © 2024 Wood, Giromini and Givens. This is an open-access article distributed under the terms of the Creative Commons Attribution License (CC BY). The use, distribution or reproduction in other forums is permitted, provided the original author(s) and the copyright owner(s) are credited and that the original publication in this journal is cited, in accordance with accepted academic practice. No use, distribution or reproduction is permitted which does not comply with these terms.
*Correspondence: J. D. Wood, amVmZi53b29kQGJyaXN0b2wuYWMudWs=