- 1Stockholm Environment Institute, US, Somerville, MA, United States
- 2Brooks McCormick Jr. Animal Law and Policy Program, Harvard Law School, Cambridge, MA, United States
- 3Stockholm Environment Institute, Oxford, United Kingdom
- 4Department of Nutrition, Harvard School of Public Health, Boston, MA, United States
- 5Stockholm Environment Institute York, University of York, York, United Kingdom
- 6Division Animals in Science and Society, Department Population Health Sciences, Faculty of Veterinary Medicine, Utrecht University, Utrecht, Netherlands
- 7Department of Environmental Studies, New York University, New York, NY, United States
- 8Laboratório de Etologia Aplicada e Bem-Estar Animal, Universidade Federal de Santa Catarina, Florianópolis, Brazil
Animal agriculture contributes significantly to global greenhouse gas (GHG) emissions—an estimated 12%-20% of total anthropogenic emissions. This has led both governmental and private actors to propose various ways to mitigate those climate impacts. This paper applies a One Health lens to the issue, arguing that the choice of solutions should not only consider the potential to reduce GHG emissions—which is not always a given—but also the implications for public health and animal welfare. With this perspective, we examine the potential public health and animal welfare impacts of three types of strategies that are often proposed: (1) “sustainable intensification” methods, aimed at maintaining or increasing production while limiting emissions and avoiding further land conversion; (2) “species shift” approaches, which focus on changing diets to consume meat from animals produced with lower GHG emissions instead of that of animals associated with higher emissions; and (3) “systemic dietary change” approaches that promote shifts towards whole plant-based foods or novel alternatives to conventional animal products. We discuss how some approaches—particularly those associated with sustainable intensification and species shift—could introduce new and significant risks to public health and animal welfare. Promoting systemic dietary change helps to overcome some of these challenges, but requires careful attention to equity to ensure that vulnerable populations still have access to the nutrients they need. We end with recommendations for a more holistic approach to reducing emissions from farmed animals that can help avoid trade-offs and increase synergies with other societal goals.
1 Introduction
The farming of land animals is responsible for an estimated 12%-20% of global greenhouse gas (GHG) emissions (Xu et al., 2021; FAO, 2023a). There is strong evidence that even if all emissions from the fossil fuel sector are eliminated, it will be difficult, if not impossible, to reach global climate targets without significant changes in animal farming (Clark et al., 2020; Ivanovich et al., 2023).
In response, many governments and private sector actors have begun exploring ways to reduce emissions from farmed animals. Numerous strategies have been proposed: from “sustainable intensification” to maximize the efficiency and productivity of animal farming systems (Campbell et al., 2014), to novel types of fodder and additives, masks that capture burped methane (Palangi et al., 2022), and gene-editing to change cows’ microbiomes (Sicard, 2023), to the promotion of diets that rely less on animal-sourced foods and promote the development of novel plant-based and cultivated alternatives (Banach et al., 2023). A growing body of literature discusses the climate implications of these different approaches, which are not always straightforward or intuitive. For instance, increased efficiencies may also lead to lower prices, which could result in higher consumption through the “rebound effect,” limiting the environmental benefits (Ceddia et al., 2013; Pellegrini and Fernández, 2018). Such issues warrant serious attention.
It is also essential to consider the broader societal implications of efforts to reduce emissions from the farmed animal sector. At the 28th UN Climate Change Conference (COP 28) in 2023, over 140 governments endorsed a One Health approach to environmental challenges (COP 28 UAE Declaration on Climate and Health, 2023). The One Health framework stresses that the health of people, animals, and the environment are intertwined (OHHLEP et al., 2022), and there is substantial evidence linking the treatment of farmed animals to human health impacts. For instance, confining animals in close quarters can heighten the risk of emergence of infectious diseases that can spill over to humans (Hayek, 2022).
Animal welfare is another important consideration, as recognized by the UN’s One Health Expert Panel (OHHLEP et al., 2022) and by scientists, ethicists, and citizens worldwide. However, neither animal welfare nor the wide-ranging human health implications of animal farming have received much attention in the climate discourse. For example, the Sixth Assessment Report of the Intergovernmental Panel on Climate Change highlights the importance of reducing emissions from animal agriculture, but does not mention One Health in this context or consider the impact of mitigation strategies on animal welfare (IPCC, 2023; see also McShane, 2018). This omission creates a risk that some climate actions will inadvertently harm animal health and welfare—and, by doing so, undermine public support for those actions (Sinclair et al., 2022; Perino and Schwickert, 2023).
Aiming to inform more sustainable and broadly beneficial solutions to the climate crisis, this Policy and Practice Review explores how different kinds of strategies to reduce emissions from animal farming could affect public health and animal welfare. This is not meant to be an exhaustive assessment of all possible interventions, or of each measure’s advantages and disadvantages, which can be complex and depend on the context. Instead, the objective is to encourage policymakers and stakeholders to take a more holistic approach in considering climate interventions, and aim to ensure they are not only sustainable but also aligned with broader public health goals as well as being ethically and publicly acceptable.
With regard to public health impacts, we focus mainly on implications for nutrition, infectious disease emergence, and antimicrobial resistance. These are widespread and often-overlooked challenges linked to animal farming, but we recognize there are other urgent public health considerations as well, including complex interactions with food security (Reynolds et al., 2015; Sun et al., 2022), occupational risks (Krumel and Goodrich, 2023), and health problems linked to hormone use, and air, water, and soil pollution (Hu et al., 2017).
Our analysis of animal welfare issues follows the World Organisation for Animal Health definition of welfare as “the mental and physical state of an animal in relation to the conditions in which [the animal] lives and dies” (WOAH, 2021). Following the Five Domains model, we consider how nutrition, health, physical environment, behavioral interactions, and other elements can affect how animals feel (Mellor et al., 2020). All else being equal, we consider animals as better off when they experience more pleasure, satisfaction, and other such positive states, and worse off when they experience pain, suffering, frustration, and other such negative states. We focus only on farmed animals, but recognize that the welfare of wild animals may also be affected by climate interventions (Sebo, 2022); that is an important emerging area of research.
The next section provides more context on the One Health approach and the links between human and animal health, as well as animal welfare as a stand-alone societal concern. Section 3 then presents an analysis of the public health and animal welfare implications of three types of interventions that are commonly promoted to reduce emissions from animal agriculture: 1) “sustainable intensification,” focused on maintaining or increasing production while reducing emissions and avoiding further land conversion; 2) “species shift” approaches, focused on reducing consumption of products from animals associated with high emissions, such as cows, and replacing them with products from animals associated with lower emissions, such as chickens, fishes, and insects; and 3) “systemic dietary change” approaches, which promote reduced consumption of animal-sourced foods in favor of plant-based foods and/or alternative proteins. Section 4 provides recommendations for policymakers and other stakeholders for a more holistic approach to reducing emissions from farmed animals. Section 5 presents closing reflections and briefly highlights some other environmental policy areas that would benefit from a more holistic approach.
2 Integrating public health and animal welfare into climate mitigation strategies: a One Health approach
The One Health framework stresses that human, animal, and environmental health are inextricably linked, and that interventions to promote any of the three should recognize those linkages in order to achieve synergies and prevent or mitigate trade-offs (Mackenzie and Jeggo, 2019; OHHLEP et al., 2022). The diverse ways in which we interact with animals consumed for food clearly illustrates how the three elements interact. For example, hunting and the capture and sale of wild animals for human consumption have been linked to biodiversity loss and to the emergence of infectious diseases that affect humans as well as farmed and wild animals (Wiebers and Feigin, 2020; Verkuijl et al., 2023).
Such risks to public health can be significant. An estimated 60–75% of newly emerging infectious diseases have an animal origin (Taylor et al., 2001; Jones et al., 2008), and such zoonoses are responsible for some 2.5 billion cases of human illness and 2.7 million human deaths each year (Grace et al., 2012). In addition, in 2019 alone, there were an estimated 1.27 million deaths attributable to bacterial antimicrobial resistance (AMR), and another 3.57 million associated with AMR (Murray et al., 2022). The contribution of animal agriculture to AMR is difficult to quantify, but we know that almost three-quarters of the antibiotics sold globally are used in animals raised for food (van Boeckel et al., 2019). This practice contributes to AMR by creating a reservoir of resistant bacteria in the animals, which can be transferred to humans through close physical contact (including consumption), or indirectly, such as through contaminated soil. The risk is increasing with the growing number of farmed animals raised for consumption worldwide (Bartlett et al., 2022).
The One Health Expert Panel has called for solutions that “recognize the importance of animal welfare and the integrity of the whole ecosystem” (OHHLEP et al., 2022, p.3). This is important for several reasons. First, an emphasis on animal welfare acknowledges that good health is not just the absence of disease or injury, but also good mental health. Second, when animals are stressed and kept in poor conditions, they can be more susceptible to health issues, including diseases they could potentially transmit to humans (Albernaz-Gonçalves et al., 2022). Pre-slaughter (dis)stress is also associated with lower meat quality (Kumar et al., 2023). Similarly, higher welfare on-farm has been associated with lower levels of antimicrobial use in animals (Diana et al., 2020), suggesting a potential relationship between animal welfare and AMR. A holistic approach to One Health that includes animal welfare can thus benefit human health by promoting more structural and effective solutions to public health challenges (Goldberg, 2016; Coghlan et al., 2021).
Animal welfare also matters to people for its own sake. A 2021 survey of adults in a geographically and culturally diverse group of countries (including Australia, Bangladesh, China, Pakistan, Sudan, Thailand, and the United States) found 87% agreed that the welfare of farmed animals in their country was important (Sinclair et al., 2022). Similarly, 84% of Europeans believe that the welfare of farmed animals should be better protected in their country than it is now (Eurobarometer, 2023). Those views may be linked to growing agreement among scientists and ethicists that many animals are sentient—that is, they demonstrate the capacity to experience positive and negative states such as pleasure and pain—and thus deserve moral consideration (Coghlan et al., 2021). This applies not only to vertebrates such as mammals, fish, and birds, but also to invertebrates such as lobsters, octopuses, and crabs (Broom, 2014; Sneddon and Brown, 2020; Gov.uk, 2021; Wickens, 2022), and possibly insects (Crump et al., 2023).
Many countries have formally recognized an obligation to protect animal welfare (Simonin and Gavinelli, 2019; European Commission n.d.a; Interafrican Bureau For Animal Resources, 2019), or have otherwise taken some regulatory steps to protect animal welfare. Notably, public concern over animal welfare may sometimes trump environmental concerns. For instance, consumers in various high-income countries are more likely to support price premiums on meat for animal welfare concerns, rather than sustainability concerns (Denver et al., 2023; Perino and Schwickert, 2023). However, food and agriculture policies overall still do not yet reflect the multiple interconnections among humans, animals, and the environment.
Several publications have highlighted some of the policy deficiencies. Hayek (2022) describes the mechanisms through which intensified animal production can decrease GHG emissions while increasing other zoonotic disease risks. Shields and Orme-Evans (2015) discuss how key emissions abatement strategies can negatively affect farmed animals, while Garnett et al. (2013) highlight the need to ensure that sustainable intensification approaches safeguard acceptable animal welfare standards. Similarly, Bracke et al. (2022) emphasize the need to integrate animal welfare considerations into circular agriculture approaches. To date, however, there is little if any research considering the potential public health and animal welfare impacts of climate mitigation strategies together, and both issues remain overlooked in policy and practice.
Several recent developments suggest growing momentum for reducing emissions from the food system, including the first installment of the UN Food and Agriculture Organization’s (FAO) roadmap for ending hunger while keeping global warming below 1.5°C (FAO, 2023b) and the Global Methane Pledge, through which more than 110 countries have pledged to reduce their methane emissions by 30% by 2030 (Global Methane Pledge, 2023). These policy developments may have a range of public health and animal welfare implications in both the short and long term: considering their impacts holistically is crucial for the development of a more sustainable, healthy, and compassionate food system.
3 Mapping public health and animal welfare impacts of common climate mitigation interventions in animal agriculture
In this section we examine seven examples of climate mitigation measures in animal agriculture and their potential impacts on public health and animal welfare. We consider three options for “sustainable intensification”: intensification of animal farming operations, feed changes to reduce methane emissions, and genetic selection for productivity. We then examine two “species shift” approaches: replacing consumption of beef cattle with meat from chickens and/or fishes, and insect farming. Lastly, we discuss two “systemic dietary change” approaches: promoting a shift to more plant-based diets, and investing in alternative proteins. Figure 1 provides an overview. For each intervention, we provide concrete examples and review evidence of potential tradeoffs and/or synergies with public health and animal welfare.
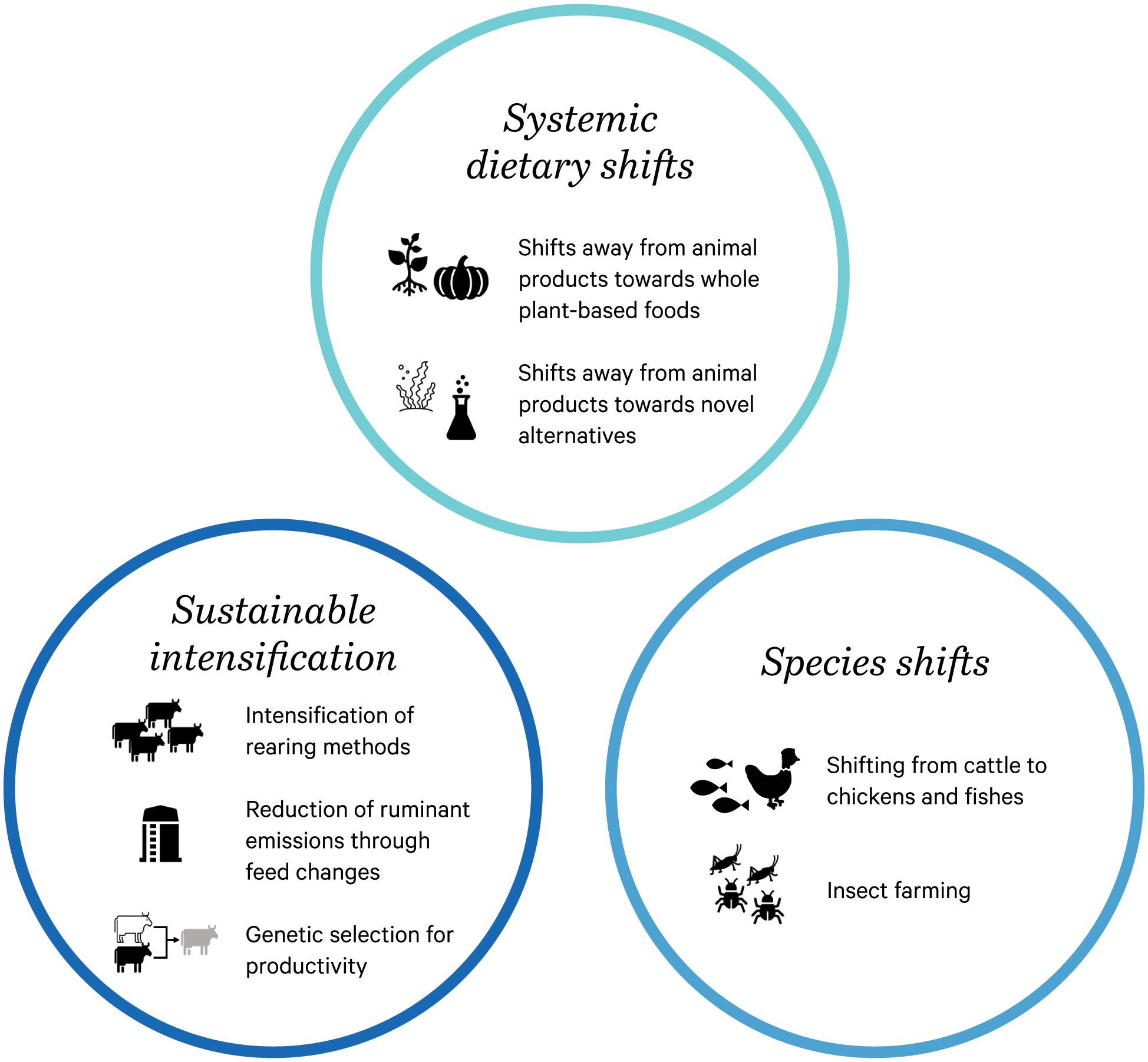
Figure 1 Three approaches to reducing GHG emissions from farmed animals. Climate change policy has typically failed to consider broad public health and animal welfare impacts of such approaches, risking missed synergies and creating trade-offs.
3.1 “Sustainable intensification” interventions
There is no universally accepted definition of sustainable intensification in animal agriculture (Godfray, 2015), but it is broadly understood to refer to measures to maintain or increase productivity while minimizing environmental impact. Below, we discuss three examples of sustainable intensification measures that are commonly discussed and promoted.
3.1.1 Intensification of rearing methods
Intensification of rearing methods typically means keeping larger numbers of animals in a given space, typically within an enclosure or indoors. This reduces land requirements, and the confined animals can gain weight quickly on less fodder than if they were foraging on open land. While this is sometimes proposed as a way to reduce the environmental impacts of animal farming, it should be noted that it requires high calorific-value feed, such as soy and maize, which requires arable land. Producing feed for farmed animals has led to large areas of forest and natural habitat loss (Merry and Soares-Filho, 2017).
Animal farming systems are already highly intensified in much of the Global North, and they are increasingly intensive in the Global South. Governments in countries such as Brazil, China, India, Mexico, Türkiye, and Vietnam have introduced policies to encourage intensification of animal agriculture (Lam et al., 2019; Gilbert et al., 2021). The first installment of the FAO roadmap for ending hunger without breaching the 1.5°C threshold also explicitly calls for intensification of animal agriculture systems in relevant locations (FAO, 2023b). While this approach could reduce environmental impacts per animal, rebound effects could offset the benefits (Ceddia et al., 2013; Pellegrini and Fernández, 2018). Specifically, as noted earlier, if intensification leads to lower meat prices, it may drive up meat consumption, which would lead to more animals being raised and killed for food. “Sustainable intensification can thus spur greater environmental impacts, undermining its sustainable aims” (Hayek, 2022, p.3).
Some practices associated with intensification can have significant implications for public health. Antimicrobials are given prolifically to densely housed animals to maintain acceptable health status, and this contributes to antimicrobial resistance (van Boeckel et al., 2015, 2019). Intensification can also accelerate the transmission of diseases among farmed animals, thus creating significant public health risks (Hayek, 2022). Infectious diseases are likelier to spread rapidly in crowded intensive farms (Bartlett et al., 2022). The stress experienced by animals in intensive, confined systems can also affect the immune system and is associated with higher antimicrobial use and resistance (Albernaz-Gonçalves et al., 2022).
Extensive farming systems present their own animal welfare challenges (Sherwin et al., 2010). Keeping animals outdoors (Jori et al., 2021), as well as the habitat loss associated with extensive farming (Bartlett et al., 2022), may bring farmed animals and farm workers in closer contact with wild animals and make them more vulnerable to disease spillover. Veterinary care for animals in large extensive systems may also be delayed (Temple and Manteca, 2020). In addition, animals may be exposed to extreme weather, parasites, and predation (Balcão et al., 2016; Bestman and Bikker-Ouwejan, 2020). In some places, the availability and quality of food and drinking water may be limited (Daros et al., 2019).
Raising animals in more intensive conditions can thus offer some potential benefits (Garnett et al., 2013). However, highly intensified housing systems in particular can be detrimental to animals’ well-being. They may not be able to move freely and have limited space to exhibit natural behaviors. Social interactions may be limited, especially related to maternal care (Beaver et al., 2019). Many problematic and destructive behaviors can result, such as tail-biting in pigs (Henry et al., 2021), feather-pecking in chickens (Rodenburg et al., 2013; von Eugen et al., 2019) and cross-suckling in cattle (Vaughan et al., 2016). In dairy cattle, lack of space can lead to agonistic social interactions. Problematic behaviors often lead farmers to trim the beaks of poultry, dock the tails of pigs, and dehorn cattle (Nordquist et al., 2017). Air quality in confined housing can also be poor, leading to respiratory issues (Wang et al., 2019; Ni et al., 2021).
3.1.2 Reduction of ruminant emissions through feed changes
Cattle account for an estimated 62% of global emissions from animal agriculture, an estimated 3.8 Gt CO2e in 2015 (FAO, 2023a), with methane from enteric fermentation making up well over half of the GHGs associated with beef and dairy alike. As the climate impacts of beef and dairy have drawn increased attention, producers have looked for potential ways to reduce methane emissions, particularly from beef cattle.
Concentrated grain-finishing is an effective method to increase feed utilization and meat production per animal. In order to bring cattle up to market weight before slaughter, they are transitioned from a forage-based diet to a concentrated diet of cereals and cereal by-products in finishing lots. The animals gain weight rapidly, as the high-starch diet results in more efficient feed utilization, and they also emit less methane from enteric fermentation; as a result, all else being equal, grain-finished cattle have a lower GHG footprint than grass-finished cattle (Beauchemin et al., 2022). Feedlot-finishing strategies are widely adopted in intensive cattle production systems in the US and increasingly in other parts of the world, such as Brazil, where the number of cattle raised in feedlots doubled between 2009 and 2019 (Silvestre and Millen, 2021), though total levels remain low (zu Ermgassen et al., 2020).
In addition to grain-finishing, several feed additives have been developed to reduce enteric methanogenesis in ruminants, including methane inhibitors, nitrates, ionophores, and dietary lipids (Honan et al., 2021). There are also novel feeds, such as seaweed and seaweed-derived products (Spillias et al., 2023). Some governments are offering carbon offsets to farmers and producers who use certain approved feed additive strategies, and some are investing in the development and regulatory approval of novel feed additives. Australia and Canada, for instance, have approved methodologies for using feed additives or supplements to reduce methane emissions and claim carbon credits (Haugen-Kozyra, 2021; Clean Energy Regulator, 2022). Reducing enteric methane production is attractive for producers as it is considered an improvement of production efficiency and economic returns, as a meaningful percent of gross energy in feed can be lost via eructated methane (Llonch et al., 2017).
The adoption of feed change strategies that reduce enteric methane production in ruminants can offer both environmental and productivity benefits (Beauchemin et al., 2022). However, it is worth considering how changing cattle feed might affect human health. For example, compared with grass-fed cattle, the meat of grain-fed cattle tends to have a higher content of cholesterol-elevating saturated fatty acids and a lower concentration of precursors for vitamins A and E (Daley et al., 2010). In addition, seaweed-derived feed may raise concerns related to presence of inorganic elements and heavy metals such as iodine, bromine, and arsenic, which at high concentrations could lead to toxicity in animals and humans, as well as risk of carcinogenicity (Vijn et al., 2020; de Bhowmick and Hayes, 2023). More research is needed to fully understand the impact of methane-reducing feed additives on the nutritional characteristics of meat and dairy products.
Limited research has looked at the animal welfare implications of feed changes. However, there are some indications that there could be adverse effects, depending on the type of feed used. For instance, the use of highly fermentable, carbohydrate-rich feeds can lead to digestive and metabolic disorders in cattle, such as ruminal acidosis, which can be painful and may cause other health problems, like liver abscesses, rumenitis, or laminitis (Constable, 2022; Plaizier et al., 2022). Cattle are at particularly high risk of suffering from these disorders when they are transitioned suddenly from forage to a high-grain diet. A recent review also pointed to possible health concerns such as ulcers and inflammation in cattle fed seaweed-based additives (Camer-Pesci et al., 2023). Moreover, precision feeding strategies for methane mitigation involving the delivery of concentrates or lipids, or compounds such as algae, chemical inhibitors of methanogenesis, nitrates, or essential oils, are not well-suited to pasture-based systems (Beauchemin et al., 2022). They may therefore need to go hand-in-hand with more intensification and its associated welfare challenges.
3.1.3 Genetic selection for productivity
Genetic manipulation and/or selective breeding of farmed animals for maximum production efficiency is a popular mitigation option: it is a particularly cost-effective technology that produces permanent and cumulative changes in performance (Wall et al., 2010), as well as bringing economic benefits. With animals who utilize feed more efficiently, producers can use less feed per unit of output, and potentially raise fewer animals to produce the same amount of meat and other animal products (Llonch et al., 2017). This lowers GHG emissions embedded in the production, processing, and transport of animal feed (including land-use change, fertilizers and pesticide use), and the lower number of animals reduces methane emissions. Genetic selection can be intertwined with feed interventions, with some breeds (especially poultry) bred to optimize high feed conversion ratios, and different feeding strategies generally requiring different genetics (Sell-Kubiak et al., 2017).
Genetic breeding for efficiency is exemplified in dairy cows. The US dairy industry once used several different breeds, but now relies almost exclusively on Holsteins (Shields and Orme-Evans, 2015). Holstein cows made up just under 40% of the US dairy herd in 1944, but 90% in 2007 (Capper et al., 2009). At the same time, selectively breeding hyper-productive cows allowed the industry to go from 26 million dairy cows producing 53 billion kg of milk per year in 1944, to 9 million cows producing 84 billion kg of milk per year by 2007 (i.e. from an average of about 2,000 kg per cow, to around 9,000 kg). These gains allowed the total carbon footprint of the US dairy industry to decline from 194 Mt CO2e in 1944, to 114 Mt CO2e by 2007 (Capper et al., 2009; Shields and Orme-Evans, 2015).
In addition to selecting for maximum production efficiency, breeding strategies to select for low methane-emitting animals have been considered as well, as the heritability of absolute methane production is moderate in some animals, such as cattle and sheep (Beauchemin et al., 2022). This approach may be particularly attractive to producers, as the resulting methane reduction is considered to be cost-effective, permanent, and cumulative (de Haas et al., 2021). However, there is uncertainty as to whether breeding for low methane traits will negatively impact other traits of interest, such as productivity (Beauchemin et al., 2022), and existing methods of measuring methane emissions from animals (e.g. respiration chambers) are difficult to implement on a large scale (de Haas et al., 2021).
Selective breeding may have indirect public health impacts. As noted earlier, high-density confined environments can be breeding grounds for zoonotic pathogens (Brozek and Falkenberg, 2021; Gilbert et al., 2021). It is not yet known, however, whether the continued narrowing of genetic diversity among these animals via selective breeding will exacerbate risk of pathogen spillover and/or of emerging infectious diseases. Some researchers have warned that high stocking densities and genetic uniformity may amplify the risk of zoonotic outbreak (Shepon et al., 2023). Conversely, some have argued that intensive farming approaches may also help to reduce infectious risk by identifying and introducing key resistance genes into high-yielding breeds (Bartlett et al., 2022).
All else being equal, more efficient raising of animals would lead to fewer animals farmed and killed, with conceivable animal welfare benefits. However, there is again a risk of rebound effects, if prices drop and demand for animal-sourced foods grows. Moreover, the genetic conditions brought about through breeding for efficiency can also result in significant harms to animal welfare. In many cases, animals that have been selected for their high productivity may be at higher risk for immunological, behavioral, and psychological problems (van der Most et al., 2011; Hartcher and Lum, 2020), and the intensive selection for certain productivity traits (thereby reducing genetic diversity) can result in unfavorable consequences to animal health and longevity (Brito et al., 2021; van Marle-Köster and Visser, 2021). Illustrative examples are included in Table 1.
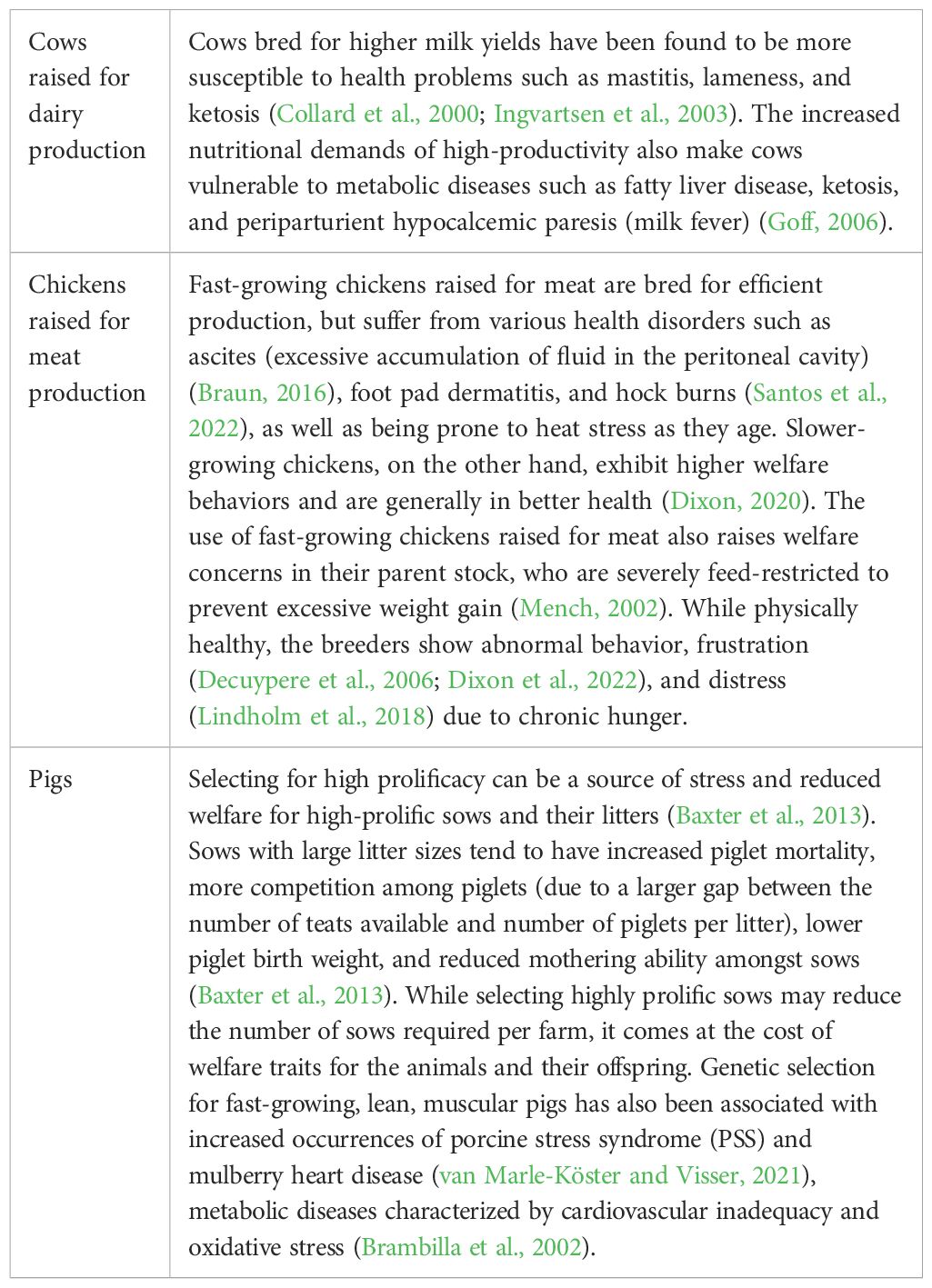
Table 1 Illustrative examples of animal welfare impacts associated with breeding animals for efficient production.
3.2 Species shifts
A second approach to reducing emissions from farmed animals that has gained traction is to replace consumption of the meat of animals associated with high GHG emissions with meat from animals with lower emissions. Below we discuss two such shifts that have been promoted by policymakers and the private sector.
3.2.1 Shifting from cattle to chickens and fishes
The environmental advantage of smaller monogastric animals compared with cattle is well established: they have greater feed efficiency, lower enteric methane emissions, and faster rates of reproduction (Xu et al., 2021; Ritchie et al., 2022). It takes 17 times as much land to produce one kilogram of beef as it does to produce one kilogram of pork or chicken (Poore and Nemecek, 2018). Overconsumption of red meat can also have negative health consequences. Red meat is high in saturated fats, which can increase LDL cholesterol levels and has been linked to higher risk of ischemic heart disease (Papier et al., 2023). Red meat contains trimethylamine N-oxide (TMAO), too, which has been linked to cardiovascular disease (Abbasi, 2019; Wang et al., 2022). In addition, red meat consumption has been associated with heightened risk of type 2 diabetes (Pan et al., 2013; Qian et al., 2020).
For all these reasons, efforts to reduce the climate impacts of meat consumption have tended to target beef most of all. Some countries, such as Canada, Sweden, and Brazil, have included environmental considerations in formulating their national dietary guidelines, which now recommend limiting consumption of red and processed meat, and instead eating more plant-based sources of protein (nuts, legumes, seeds, tofu, and fortified soy beverage), fish, eggs, and poultry (Government of Canada n.d.; Ministério da Saúde, 2014; Monteiro et al., 2015; Swedish Food Agency, 2023). Ethiopia has included a shift from beef to chicken production in its nationally determined contribution under the Paris Agreement (Federal Democratic Republic of Ethiopia, 2021). The first installment of the FAO roadmap for ending hunger without breaching the 1.5°C threshold calls for a shift from beef to poultry, as well as for an at least 75% increase in aquaculture by 2040 (FAO, 2023b).
Consumption of poultry products has already risen in the Global North, while red meat consumption has stagnated or declined over the past decades. In the US, beef and veal consumption dropped 15% per capita between 2000 and 2019, while poultry consumption increased by a similar percentage (Whitton et al., 2021). New Zealand saw a 50% decrease in beef consumption per capita in 2019 relative to 2000, while poultry consumption per capita nearly doubled (Whitton et al., 2021). Across Organisation for Economic Co-operation and Development (OECD) countries, beef and veal consumption dropped by 9.4% in that period, while poultry consumption increased by 36.8% (Whitton et al., 2021).
Fish consumption has been linked with a reduction in premature mortality and prevention of certain cancers (Lloret et al., 2016; Clark et al., 2019), though ingestion of contaminants such as mercury may form a risk for some subgroups, such as pregnant women and children (Thomsen et al., 2021). The evidence on poultry consumption is more mixed: several studies have found no health effects, or positive health effects (Clark et al., 2019); however, there may be a link between poultry consumption and some health risks, such as incident cardiovascular disease (Zhong et al., 2020).
That said, shifting away from beef farming and increasing poultry farming may still be associated with other public health risks. Cattle farming has previously contributed to the emergence of zoonotic disease such as bovine spongiform encephalopathy (BSE) (Smith and Bradley, 2003); and cattle farming can contribute to deforestation which may trigger zoonotic spillover from wild animal populations to humans, although more research is needed to understand under what circumstances such spillover is likely to occur (Hayek, 2022). However, poultry also harbor zoonotic diseases and their intensive housing conditions in close proximity to humans contribute to zoonotic potential of diseases such as avian influenza, which poses an ongoing risk to farmed animals, humans, and wild species (Sooksawasdi Na Ayudhya and Kuiken, 2021; Adloch and Baldinelli, 2023; WHO, 2023).
Moreover, antibiotics are used routinely in a range of farmed animals, including both cattle and poultry. This appears to be linked to AMR in humans, although more research is needed to understand the complexities of this dynamic (Ardakani et al., 2023). The use of antibiotics in poultry farming has sharply decreased in recent years due to husbandry factors including using slower-growing genetic lines and the introduction of ionophores (Mulchandani et al., 2023). Nevertheless, it remains a serious concern given the scale, the fact that antibiotics can still be present in chicken meat upon consumption (even after cooking), the ongoing presence of resistant bacteria infections in humans that originate from chicken production and that chicken farms are major emitters of particles and materials that may contain or promote antibiotic-resistant strains of bacteria (Zhuge et al., 2021; Gržinić et al., 2023). Antibiotic use that raises risks of antimicrobial resistance is also a concern in aquaculture, but it is currently poorly monitored (Preena et al., 2020; Hossain et al., 2022).
From an animal welfare perspective, a dietary shift from cows to smaller animals would entail a dramatic increase in the number of animals raised and killed for meat (Scherer et al., 2018). Consuming a given amount of calories of chicken meat requires 18–40 times as many days of animals living in intensive farms compared to beef, and the number is even greater for a shift from beef to fish (Mathur, 2022). In addition to implicating more animal lives, current intensive poultry farming systems create a myriad of welfare issues at many levels in the production chain that push animals’ physiological limits (Korver, 2023).
Fish farming is also associated with welfare risks, and fish are generally not yet protected by the same types of welfare legislation, or even the same level of knowledge about the welfare needs, as other farmed animals (Franks et al., 2021). On the other hand, some forms of aquaculture are likely associated with significantly reduced animal welfare concerns, such as farming of bivalves (Jacquet et al., 2017).
3.2.2 Insect farming
Certain insect species have been consumed by humans for millennia, including across Africa, Asia, and Latin America. Recently, several jurisdictions, including the European Union, have begun to promote insects as a potential new source of food to help address the impacts of conventional animal products (e.g., European Commission CORDIS, n.d.), with several insects now approved as novel foods in the region (European Commission n.d.b). Insect protein is also gaining increased attention for its potential as a “climate-smart” feedstock ingredient for farmed land animals and fish, which currently rely heavily on environmentally costly soy and corn feeds (Little, 2021). As of 2021, the EU has authorized the use of processed animal protein derived from insects in poultry, pig, and aquaculture feed (Official Journal of the European Union, 2021). Other countries are expected to follow the EU’s approach. The Rabobank has predicted that the 10,000 metric tons of insects currently farmed per year could climb to 500,000 metric tons annually by 2030 (de Jong and Nikolik, 2021).
A range of insects are generally considered a nutritious and healthy source of protein, essential amino acids, and various vitamins and minerals such as calcium, magnesium, manganese, phosphorus, and selenium (Hawkey et al., 2021; Liceaga et al., 2022). Compared with conventional feed for farmed animals, insect production systems are land and resource-use efficient; vertical insect farms require minimal arable land, water, or feed (thereby reducing pesticide and fertilizer use embedded within food production) (Moruzzo et al., 2021). Their resource efficiency is primarily driven by the fact that insects do not expend energy maintaining high body temperatures, leading to a high feed conversion ratio. Crickets, for example, which are among the species deemed safe for consumption by humans and animals in the EU, need 12 times less feed than cattle, and half as much as pigs and chickens raised for meat to produce the same amount of protein (van Huis et al., 2013). Insects are also able to convert low-value food waste into protein and fat-rich biomass. Because of this, some researchers expect insect protein cultivation to provide an even more efficient and economical pathway for food waste treatment than composting (Ites et al., 2020).
In practice, however, the diversion of waste streams into insect feed has yet to happen at a meaningful scale. Most insect farming practices feed insects a grain-based diet, and occasionally some fruits and vegetables (Lundy and Parrella, 2015). Moreover, currently, most farmed insects are fed to other animals, including pets, fish, and poultry (Sogari et al., 2023). If insects destined for animal feed are eating the same grain or soy that would otherwise be fed to cattle or chickens, the environmental benefits of insect-based feed over conventional feed are limited. Insect farming will be an industry to support other kinds of animal farming, rather than replacing them (Sogari et al., 2023). It may also increase competition for agricultural products that would otherwise be fed to poultry and pigs, exacerbating pressures on arable land.
The welfare implications of insect farming at large scale are also important to consider, particularly given the number of animals affected (Scherer et al., 2018) and the speed at which industrialized forms of insect farming are projected to emerge in global markets. In 2020 over 1 trillion insects per year were farmed for animal feed and direct human consumption and this number may increase significantly going forward (Rowe, 2020; Barrett and Fischer, 2023). There is also uncertainty about whether insects are sentient in a morally relevant way. Evidence is accumulating to suggest that a range of insect species are plausibly or likely sentient (Giurfa, 2015; Haberkern and Jayaraman, 2016; Klein and Barron, 2016; Lambert et al., 2021; Crump et al., 2023).
Generating high-welfare farm environments may be easier to achieve at scale for insects than has been the case for vertebrates: factory farms more closely resemble insects’ natural habitats —crowded, damp, and dark—than the forest habitats pigs and chickens evolved in (Bollard, 2021). However, such arguments are still speculative, and the welfare implications of practices such as genetic modification of insects are unknown (Barrett and Fischer, 2023). An additional consideration is the current lack of regulatory welfare protections for insects. Several guidelines regarding the production of insects exist; however, these are not legally binding (Hanboonsong and Durst, 2020; Delvendahl et al., 2022). Of the guidelines that do exist, few specifically mention welfare or offer species-specific regulations.
3.3 Promoting systemic dietary change
A third approach to reducing the climate impacts of animal-sourced foods that is widely discussed is to support a more systemic shift towards plant-based foods and alternative protein sources. That is the focus of the last two strategies we explore in our analysis.
3.3.1 Replacement of animal products with whole plant-based foods
There is broad scientific consensus regarding the significant environmental benefits of shifting away from high levels of animal-sourced foods and transitioning towards more plant-based diets. Such dietary changes could lead to reduced GHG emissions, decreased deforestation and biodiversity loss, and more efficient use of water and land resources (Poore and Nemecek, 2018; IPCC, 2022). Against this backdrop, several governments have begun to support a shift towards lower meat consumption. France’s Climate and Resilience Law mandates vegetarian meal options in school cafeterias and public canteens once a week by 2023 (Pistorius, 2021). “Meatless Monday” and “Green Monday” campaigns have been launched globally, and several city councils including Amsterdam and Oxford have implemented vegetarian or fully plant-based catering policies at council events (The Monday Campaigns n.d.; Darroch, 2019; Vegconomist, 2023).
From a health perspective, whole, unprocessed or minimally processed plant-based foods such as grains, vegetables, fruits, nuts, seeds, and legumes are associated with reduced risks of premature mortality and various non-communicable diseases, including cardiovascular disease, type 2 diabetes, and some cancers (Afshin et al., 2019; Kim et al., 2019). Although concerns exist regarding the adequacy of certain micronutrients in completely plant-based diets, such as vitamin B12, zinc, calcium, and selenium (Bakaloudi et al., 2021), this is more of a concern for strictly plant-based (vegan) diets, not for those that simply limit animal products. Moreover, vegans can generally obtain all necessary nutrients by planning properly and consuming some fortified foods (Niklewicz et al., 2023). Compared with animal farming, food crop production uses less land per calorie and avoids high stocking densities of animals, thus reducing the risk of zoonotic spillovers (Shepon et al., 2023). Similarly, antibiotic use is not a significant concern in plant-based food systems.
Even so, not everyone may have access to sufficient plant-based food options to forgo or sharply reduce consumption of animal-sourced foods. In lower-income and food-insecure communities, animal protein sometimes provides access to essential nutrients that are difficult to obtain elsewhere (Godfray et al., 2018). Realizing the potential benefits of a shift towards whole plant-based foods requires ensuring better accessibility and affordability for groups who currently lack access.
In some regions, particularly in developing countries, pastoralism has a long history and a very different environmental footprint than industrialized animal farming in the Global North, and animal-sourced products are currently regarded as essential for food security (Houzer and Scoones, 2021). Pushing for a dietary shift among these communities, particularly without ensuring that appropriate alternatives are available, raises important equity and social justice concerns.
From an animal welfare perspective, the benefits are clear and straightforward: Reduced demand for animal-sourced foods would mean that fewer animals would be raised and killed for human consumption, which could help alleviate the suffering currently experienced by tens of billions of animals each year (Singer, 2023). Moreover, if consumption of animal-sourced foods is reduced significantly, the remaining demand could potentially be met by raising animals in less crowded conditions that are more conducive to their welfare.
3.3.2 Replacement of animal products with novel alternatives
In recent years, several high-profile meat and dairy alternatives have been developed that closely mimic the sensory experience of animal products, but contain no animal-derived ingredients. Impossible Foods has created a plant-based burger that “bleeds” like a traditional beef burger, while Beyond Meat used plant proteins to replicate the taste, texture, and juiciness of meat. Plant-based milks made from almonds, soy, oats, pea protein, and various blends offer alternatives to cow’s milk and other dairy products (e.g., cheese and yogurt). In the US, plant-based milk accounts for 15% of all dollar sales of total milk retail (UNEP, 2023). In addition to these plant-based alternatives new fermentation techniques are being developed to produce animal-free proteins, such as biomass fermentation and precision fermentation, including through use of fungus protein as a main input (UNEP, 2023). The Perfect Day company is using precision fermentation to produce animal-free dairy proteins that can be used to make milk, cheese, and ice cream (GFI, 2022).
Cell-based or “cultivated” meat is another proposed pathway to reduce agriculture emissions and other environmental impacts by replacing conventional animal meat with cell-grown analogues. The first cultivated meat went on sale in Singapore in 2020 (Woodyatt and Wiener-Bronner, 2020), although the extent to which cultivated meat can be culturally accepted and viably commercially scaled up remains to be seen (Bryant and Barnett, 2018; Dullaghan, 2021).
The environmental impacts of alternatives to animal products vary depending on a range of factors, including whether such products are produced with fossil or low-carbon energy (Sinke et al., 2023). Nevertheless, in many cases such products confer several environmental advantages compared with conventional animal products, such as reduced GHG emissions (particularly when low-carbon energy is used) and reduced land use (UNEP, 2023).
Against this backdrop, many governments have begun to support the development and commercialization of these products and technologies For example, both China and the US have identified novel alternatives as investment priorities (UNEP, 2023). The EU has also been increasingly supportive of cellular agriculture in recent years, awarding a €2 million grant to Mosa Meat’s “Feed for Meat” project, and a €2.7 million grant to the cultured meat research program Meat4all (ProVeg, 2022). Singapore has made millions of dollars available to the alternative protein sector through the “30 by 30” resilient food production initiative (Shu, 2020; Singapore Food Agency, 2020). The Indian government granted over US$600,000 to the establishment of a Centre of Excellence in cultured meat agriculture (Ramamurthy, 2019), and the Japanese Agency of Science and Technology has granted US$2 million to Integriculture to help accelerate the development of technologies for the application and mass production of cultivated meat (Integriculture, 2022).
From a public health perspective, alternatives to conventional animal protein are likely to confer a lower risk of infectious disease outbreak as well as lower risk of antimicrobial resistance compared to conventional animal sourced foods (UNEP, 2023). From a nutritional perspective, it is not clear to what extent replacing meat with novel plant- or fungus-based proteins would convey the same benefits as eating unprocessed or minimally processed plant-based foods. Many of these products are highly processed, and there are ongoing debates about the health implications of consuming them regularly (Flint et al., 2023). One recent long-term follow-up study found that although higher consumption of ultra-processed foods generally increased the risk of cancer and cardiometabolic multimorbidity, plant-based alternatives were not associated with such risks (Cordova et al., 2023). There is limited information about the impacts of cultivated meat, since these products are not yet widely available. A recent United Nations report called for more research to understand the nutritional health implications of regularly consuming novel meat alternatives as a main source of protein (UNEP, 2023). Access to such alternatives is moreover geographically imbalanced, meaning they may not be a feasible solution in all contexts. More research is also needed into the equity dimensions of novel alternatives (UNEP, 2023).
From an animal welfare perspective, replacing conventional animal products with plant-based or cultivated products would imply significant gains for animals, as it would reduce or even eliminate reliance on animals that are raised and slaughtered for food. However, cultivated meat production currently still relies on fetal bovine serum (FBS) as a growth serum. This is conventionally obtained from fetuses taken from slaughtered pregnant cows (Lee et al., 2022). Moreover, producing cultivated meat currently requires biopsies from animals. Although the number of animals needed to provide cells for cultivated meat is vastly lower than that required for conventional animal products, and the procedure of biopsy relatively trivial compared with many commonplace animal farming interventions, this still raises questions about the ethical treatment of animals used to make cultivated meat (Dutkiewicz and Abrell, 2021; UNEP, 2023). However, promising developments for animal-free alternatives to FBS for use in cultured meat production are underway, while cultured meat producers are also exploring the genetic immortalization of cells to eliminate the need for biopsies from animals (UNEP, 2023).
4 Recommendations: towards more holistic solutions
The preceding sections have shown that common climate mitigation interventions targeting animal agriculture have implications for public health and animal welfare. Our findings are summarized in Figure 2. The impacts can be positive, negative, or mixed, but they are often overlooked in climate policy and practice. When seeking to reduce the climate impacts of animal farming, it is thus crucial to look beyond GHG emissions alone: a wider set of social goods merits serious attention.
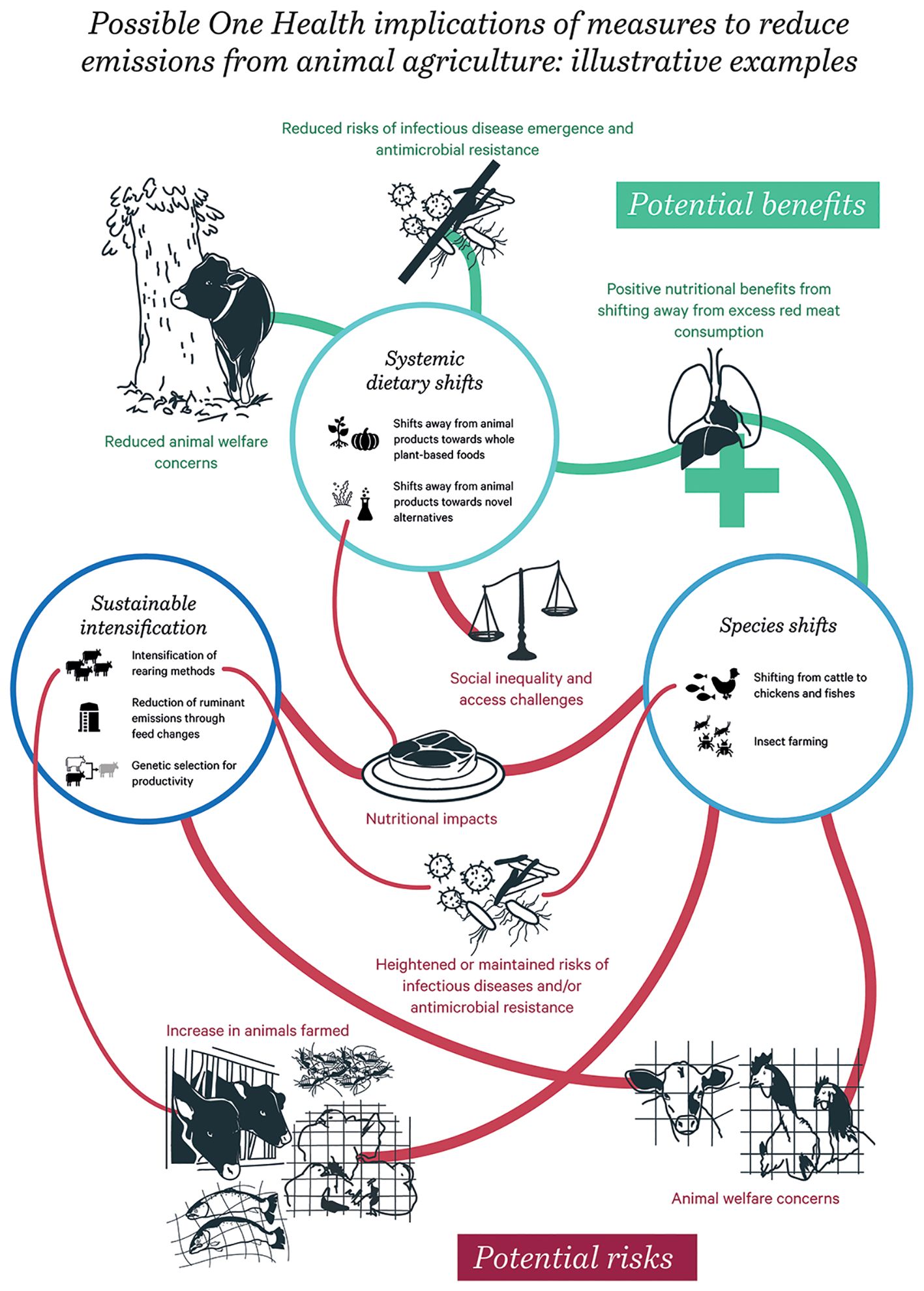
Figure 2 A One Health approach towards food systems transformation reveals risks and benefits of different interventions.
There are multiple, complementary entry points for giving broader consideration to public health and animal welfare in climate change policy. The Sustainable Development Goals (SDGs) provide a useful framework for a more holistic approach to sustainability (United Nations, 2015). The potential for both synergies and trade-offs across different sustainable development outcomes is well documented (Nilsson et al., 2016). Considering these potential outcomes explicitly can contribute to policy coherence: helping to maximize benefits and prevent or reduce trade-offs (Verkuijl et al., 2022). Likewise, the One Health framework recognizes the interconnectedness of human, environmental, and animal health, and the need to approach these issues holistically (OHHLEP et al., 2022).
Animal welfare is currently not mentioned explicitly in the SDGs, but hundreds of experts have recognized that giving attention to animal welfare in sustainable development policy can benefit humans, non-humans, and the environment alike (Sebo et al., 2022). In this light, the UN OHHLEP’s explicit recognition of the importance of animal welfare in the context of One Health is an important development (OHHLEP et al., 2022). Governments and the UN system could recognize these important interlinkages more strongly—for instance, in international statements and commitments related to reducing emissions from the food system, efforts to implement the Global Methane Pledge, the FAO’s 1.5°C roadmap for the food system, and the IPCC’s synthesis work.
At a national policy level, stronger collaboration among different ministries and departments can also help achieve more holistic outcomes. Ministries of health, agriculture, and the environment can work together to identify and evaluate the impacts of climate mitigation strategies across various societal sectors. This collaboration can facilitate the integration of public health, animal welfare, and environmental concerns into climate policies, leading to more effective and balanced outcomes. Policymakers could introduce impact assessments that consider the broader implications of climate mitigation strategies, including for animal welfare (McCulloch and Reiss, 2017; Blattner, 2020), with quantitative tools increasingly emerging to understand magnitudes of animal welfare loss (e.g., Welfare Footprint Project n.d.) and bring animal welfare into policy analysis (Budolfson et al., 2023). Such approaches can inform governments and the private sector in taking into account a broader set of considerations in their climate mitigation strategies.
Both nationally and internationally, the financial sector—including investors, banks and insurers— can consider broader societal implications in their funding decisions (see e.g., van der Mark and Nedeff, 2021). Evaluating the potential trade-offs and risks associated with different strategies is crucial to make informed investment decisions. For instance, measures that reduce GHG emissions but increase the risk of infectious disease outbreaks may have detrimental societal and financial consequences (FAIRR, 2016). Integrating these considerations into financial assessments can promote more responsible and sustainable investment. International development banks, too, can ensure their funding decisions take a more holistic approach. Financial, technology, and capacity building support and international knowledge exchange can ensure that different regions are able to integrate a wider set of considerations such as public health and animal welfare in their sustainable development and climate policy decisions (Verkuijl et al., 2022).
Finally, greater collaboration among scientific disciplines is needed to advance our understanding of the complex interactions between climate change, animal agriculture, public health, and animal welfare. Current research efforts often focus narrowly on the impacts of interventions on one societal goal such as climate mitigation, potentially overlooking important co-benefits and trade-offs between different domains. By fostering interdisciplinary collaboration, researchers can generate knowledge that informs policy decisions and guides the development of effective and sustainable climate mitigation strategies (Sebo, 2022). A better understanding of the circumstances under which significant public health risks such as zoonotic disease and AMR are likely to emerge through our food system should be a research priority.
5 Closing reflections
The growing attention to the role of animal agriculture in climate change is encouraging. A transformation of the animal agriculture sector is needed to meet climate change goals, and in pursuing emission reductions, we also have a prime opportunity to improve human health and animal welfare. Concerningly, however, most discussions to date about how to reduce GHG emissions in this sector have failed to consider key public health and animal welfare impacts. As a result, important risks and ethical challenges are not widely recognized.
By applying a One Health lens, this paper has shown that common approaches to reduce emissions from farmed animals, including various strategies related to sustainable intensification and shifting consumption to different animal species, can create new public health risks and exacerbate animal welfare challenges. However, there is also potential for interventions to be synergistic with these different goals. For instance, interventions to support a shift towards more plant-based diets can be significantly co-beneficial by reducing emissions, supporting public health, and benefiting animal welfare, although ensuring sufficient accessibility remains a challenge in some settings. Alternative protein innovations also show promise in terms of reduced risks for animal welfare and public health, although more work is needed to understand their nutritional implications.
There are a range of approaches available at the international and national level that can help governments, the UN system, and financial institutions to minimize trade-offs and maximize synergies between these different social goals. These include paying more attention to such interconnections in policy documents, improving collaboration between relevant ministries, considering interlinkages through impact assessments, and supporting cross-disciplinary science.
While the article has focused on animals raised for food, climate policies can also affect animals in other ways that merit increased policy attention. Wild animals, for instance, could be affected by climate adaptation infrastructure or land clearance for mineral mining (Sebo, 2022). Animals are also affected by a range of other interventions that are pursued to further sustainable development, including biodiversity policy, in which questions around their welfare are frequently overlooked (Coghlan and Cardilini, 2022). By considering the impacts of these and other sustainable development and climate change interventions together, policymakers and practitioners can help support better outcomes for human health, animals, and the environment alike.
Author contributions
CV: Conceptualization, Supervision, Visualization, Writing – original draft, Writing – review & editing. JSm: Writing – original draft, Writing – review & editing, Conceptualization. JG: Writing – review & editing. RN: Visualization, Writing – review & editing, Writing – original draft. JSe: Writing – review & editing. MH: Conceptualization, Writing – review & editing. MH: Conceptualization, Writing – original draft, Writing – review & editing.
Funding
The author(s) declare financial support was received for the research, authorship, and/or publication of this article. Open access APC charges were funded by Utrecht University, the Netherlands.
Acknowledgments
The authors thank Linnéa Haviland and Mia Shu for their support with the figures and Marion Davis for support with copy editing.
Conflict of interest
The authors declare that the research was conducted in the absence of any commercial or financial relationships that could be construed as a potential conflict of interest.
The author(s) declared that they were an editorial board member of Frontiers, at the time of submission. This had no impact on the peer review process and the final decision.
Publisher’s note
All claims expressed in this article are solely those of the authors and do not necessarily represent those of their affiliated organizations, or those of the publisher, the editors and the reviewers. Any product that may be evaluated in this article, or claim that may be made by its manufacturer, is not guaranteed or endorsed by the publisher.
References
Abbasi J. (2019). TMAO and heart disease: the new red meat risk? JAMA 321, 2149–2151. doi: 10.1001/jama.2019.3910
Adloch C., Baldinelli F. (2023). Avian influenza, new aspects of an old threat. Euro Surveill 28 (19), 2300227. doi: 10.2807/1560-7917.ES.2023.28.19.2300227
Afshin A., Sur P. J., Fay K. A., Cornaby L., Ferrara G., Salama J. S., et al. (2019). Health effects of dietary risks in 195 countries 1990–2017: A systematic analysis for the global burden of disease study 2017. Lancet 393, 1958–1972. doi: 10.1016/S0140-6736(19)30041-8
Albernaz-Gonçalves R., Olmos Antillón G., Hötzel M. J. (2022). Linking animal welfare and antibiotic use in pig farming: A review. Animals 12, 216. doi: 10.3390/ani12020216
Ardakani Z., Canali M., Aragrande M., Tomassone L., Simoes M., Balzani A., et al. (2023). Evaluating the contribution of antimicrobial use in farmed animals to global antimicrobial resistance in humans. One Health 17, 100647. doi: 10.1016/j.onehlt.2023.100647
Bakaloudi D. R., Halloran A., Rippin H. L., Oikonomidou A. C., Dardavesis T. I., Williams J., et al. (2021). Intake and adequacy of the vegan diet. A systematic review of the evidence. Clin. Nutr. 40, 3503–3521. doi: 10.1016/j.clnu.2020.11.035
Balcão L., Longo C., Costa J. H., Uller-Gmez C., Pinheiro MaChado Filho L., Hötzel M. (2016). Characterisation of smallholding dairy farms in southern Brazil. Anim. Production Sci. 57, 735–745. doi: 10.1071/AN15133
Banach J. L., van der Berg J. P., Kleter G., van Bokhorst-van de Veen H., Bastiaan-Net S., Pouvreau L., et al. (2023). Alternative proteins for meat and dairy replacers: Food safety and future trends. Crit. Rev. Food Sci. Nutr. 63, 11063–11080. doi: 10.1080/10408398.2022.2089625
Barrett M., Fischer B. (2023). Challenges in farmed insect welfare: Beyond the question of sentience. Anim. Welfare 32, e4. doi: 10.1017/awf.2022.5
Bartlett H., Holmes M. A., Petrovan S. O., Williams D. R., Wood J. L. N., Balmford A. (2022). Understanding the relative risks of zoonosis emergence under contrasting approaches to meeting livestock product demand. R. Soc. Open Sci. 9, 2115735. doi: 10.1098/rsos.211573
Baxter E., Rutherford K., D'eath R., Arnott G., Turner S., Sandøe P., et al. (2013). The welfare implications of large litter size in the domestic pig II: management factors. Anim. Welfare 22, 219–238. doi: 10.7120/09627286.22.2.219
Beauchemin K. A., Ungerfeld E. M., Abdalla A. L., Alvarez C., Arndt C., Becquet P., et al. (2022). Invited review: current enteric methane mitigation options. J. Dairy Sci. 105, 9297–9326. doi: 10.3168/jds.2022-22091
Beaver A., Meagher R. K., Keyserlingk M. A. G., Weary D. M. (2019). Invited review: A systematic review of the effects of early separation on dairy cow and calf health. J. Dairy Sci. 102, 5784–5810. doi: 10.3168/jds.2018-15603
Bestman M., Bikker-Ouwejan J. (2020). Predation in organic and free-range egg production. Animals 10, 177. doi: 10.3390/ani10020177
Blattner C. (2020). “Animal impact assessments: contesting denial, changing the future?,” in What Can Animal Law Learn From Environmental Law?, 2d ed. Ed. Abate R. S. (ELI Press, Washington DC), 95–120.
Bollard L. (2021). “The promise and perils of insect farming,” in Open Philanthropy Farm Animal Welfare Newsletter. Available at: https://mailchi.mp/0d9c51072475/the-promise-and-perils-of-insect-farming.
Bracke M. B. M., Boumans I. J. M. M., Nijland H. J., Bokkers E. A. M. (2022). Connecting circularity to animal welfare calls for a ‘novel’ conceptual framework based on integrity. Animal 17, 100694. doi: 10.1016/j.animal.2022.100694
Brambilla G., Civitareale C., Ballerini A., Fiori M., Amadori M., Archetti L. I., et al. (2002). Response to oxidative stress as a welfare parameter in swine. Redox Rep. 7, 159–163. doi: 10.1179/135100002125000406
Braun U. (2016). Ascites in cattle. Veterinary Clinics North America: Food Anim. Pract. 32, 55–83. doi: 10.1016/j.cvfa.2015.09.004
Brito L. F., Bedere N., Douhard F., Oliveira H. R., Arnal M., Peñagaricano F., et al. (2021). Review: Genetic selection of high-yielding dairy cattle toward sustainable farming systems in a rapidly changing world. Animal Sustain. livestock Syst. high-producing Anim. 15, 100292. doi: 10.1016/j.animal.2021.100292
Broom D. M. (2014). Sentience and animal welfare (Wallingford, Oxfordshire: Cabi). doi: 10.1079/9781780644035.0000
Brozek W., Falkenberg C. (2021). Industrial animal farming and zoonotic risk: COVID-19 as a gateway to sustainable change? A scoping study. Sustainability 13, 9251. doi: 10.3390/su13169251
Bryant C., Barnett J. (2018). Consumer acceptance of cultured meat: A systematic review. Meat Sci. 143, 8–17. doi: 10.1016/j.meatsci.2018.04.008
Budolfson M., Fischer B., Scovronick N. (2023). Animal welfare: Methods to improve policy and practice. Science 381, 32–34. doi: 10.1126/science.adi0121
Camer-Pesci B., Laird D. W., van Keulen M., Vadiveloo A., Chalmers M., Moheimani N. R. (2023). Opportunities of Asparagopsis sp. cultivation to reduce methanogenesis in ruminants: A critical review. Algal Res. 76, 103308. doi: 10.1016/j.algal.2023.103308
Campbell B. M., Thornton P., Zougmoré R., van Asten P., Lipper L. (2014). Sustainable intensification: What is its role in climate smart agriculture? Curr. Opin. Environ. Sustainability SI: Sustainability governance transformation 8, 39–43. doi: 10.1016/j.cosust.2014.07.002
Capper J. L., Cady R. A., Bauman D. E. (2009). The environmental impact of dairy production: 1944 compared with 2007. J. Anim. Sci. 87, 2160–2167. doi: 10.2527/jas.2009-1781
Ceddia M. G., Sedlacek S., Bardsley N. O., Gomez-y-Paloma S. (2013). Sustainable agricultural intensification or jevons paradox? The role of public governance in tropical South America. Global Environ. Change 23, 1052–1063. doi: 10.1016/j.gloenvcha.2013.07.005
Clark M. A., Domingo N. G. G., Colgan K., Thakrar S. K., Tilman D., Lynch J., et al. (2020). Global food system emissions could preclude achieving the 1.5° and 2°C climate change targets. Science 370, 705–708. doi: 10.1126/science.aba7357
Clark M. A., Springmann M., Hill J., Tilman D. (2019). Multiple health and environmental impacts of foods. Proc. Natl. Acad. Sci. 116, 23357–23362. doi: 10.1073/pnas.1906908116
Clean Energy Regulator (2022). About the Emissions Reduction Fund. Available online at: https://www.cleanenergyregulator.gov.au/ERF/About-the-Emissions-Reduction-Fund.
Coghlan S., Cardilini A. P. (2022). A critical review of the compassionate conservation debate. Conserv. Biol. 36, e13760. doi: 10.1111/cobi.13760
Coghlan S., Coghlan B. J., Capon A., Singer P. (2021). A bolder one health: expanding the moral circle to optimize health for all. One Health Outlook 3, 215. doi: 10.1186/s42522-021-00053-8
Collard B. L., Boettcher P. J., Dekkers J. C. M., Petitclerc D., Schaeffer L. R. (2000). Relationships between energy balance and health traits of dairy cattle in early lactation. J. Dairy Sci. 83, 2683–2690. doi: 10.3168/jds.S0022-0302(00)75162-9
Constable P. D. (2022). Grain Overload in Ruminants - Digestive System (Merck Veterinary Manual). Available at: https://www.merckvetmanual.com/digestive-system/diseases-of-the-ruminant-forestomach/grain-overload-in-ruminants.
COP 28 UAE Declaration on Climate and Health (2023). Available online at: https://www.who.int/publications/m/item/cop28-uae-declaration-on-climate-and-health.
Cordova R., Viallon V., Fontvieille E., Peruchet-Noray L., Jansana A., Wagner K. H., et al. (2023). Consumption of ultra-processed foods and risk of multimorbidity of cancer and cardiometabolic diseases: a multinational cohort study. Lancet Regional Health–Europe 35, 100771. doi: 10.1016/j.lanepe.2023.100771
Crump A., Gibbons M., Barrett M., Birch J., Chittka L. (2023). Is it time for insect researchers to consider their subjects’ welfare? PloS Biol. 21, e3002138. doi: 10.1371/journal.pbio.3002138
Daley C. A., Abbott A., Doyle P. S., Nader G. A., Larson S. (2010). A review of fatty acid profiles and antioxidant content in grass-fed and grain-fed beef. Nutr. J. 9. doi: 10.1186/1475-2891-9-10
Daros R. R., Bran J. A., Hötzel M. J., von Keyserlingk M. A. G. (2019). Readily available water access is associated with greater milk production in grazing dairy herds. Animals 9, 48. doi: 10.3390/ani9020048
Darroch G. (2019). Neither fish nor fowl: Amsterdam council moves towards 100% vegetarian catering. DutchNews.nl.
de Bhowmick G., Hayes M. (2023). Potential of seaweeds to mitigate production of greenhouse gases during production of ruminant proteins. Global Challenges 7, 2200145. doi: 10.1002/gch2.202200145
Decuypere E., Hocking P. M., Tona K., Onagbesan O., Bruggeman V., Jones E. K. M., et al. (2006). Broiler breeder paradox: a project report. World's Poultry Sci. J. 62, 443–453. doi: 10.1079/WPS2005107
de Haas Y., Veerkamp R. F., de Jong G., Aldridge M. N. (2021). Selective breeding as a mitigation tool for methane emissions from dairy cattle. Animal 15, 100294. doi: 10.1016/j.animal.2021.100294
de Jong B., Nikolik G. (2021). EU food watchdog approves mealworms for human consumption. Bloomberg.
Delvendahl N., Rumpold B. A., Langen N. (2022). Edible insects as food–insect welfare and ethical aspects from a consumer perspective. Insects, 13 (2), 121. Insects 13, 121. doi: 10.3390/insects13020121
Denver S., Christensen T., Lund T. B., Olsen J. V., Sandøe P. (2023). Willingness-to-pay for reduced carbon footprint and other sustainability concerns relating to pork production–A comparison of consumers in China, Denmark, Germany and the UK. Livestock Sci. 276, 105337. doi: 10.1016/j.livsci.2023.105337
Diana A., Lorenzi V., Penasa M., Magni E., Alborali G. L., Bertocchi L., et al. (2020). Effect of welfare standards and biosecurity practices on antimicrobial use in beef cattle. Sci. Rep. 10, 20939. doi: 10.1038/s41598-020-77838-w
Dixon L. M. (2020). Slow and steady wins the race: The behaviour and welfare of commercial faster growing broiler breeds compared to a commercial slower growing breed. PloS One 15, e0231006. doi: 10.1371/journal.pone.0231006
Dixon L. M., Dunn I. C., Brocklehurst S., Baker L., Boswell T., Caughey S. D., et al. (2022). The effects of feed restriction, time of day, and time since feeding on behavioral and physiological indicators of hunger in broiler breeder hens. Poultry Sci. 101, 101838. doi: 10.1016/j.psj.2022.101838
Dutkiewicz J., Abrell E. (2021). Sanctuary to table dining: Cellular agriculture and the ethics of cell donor animals. Politics Anim. 7, 1–15.
Eurobarometer (2023). Attitudes of Europeans towards animal welfare. Available online at: https://europa.eu/eurobarometer/surveys/detail/2996.
European Commission Animal Welfare. Available online at: https://food.ec.europa.eu/animals/animal-welfareen.
European Commission Approval of fourth insect as a Novel Food. Available online at: https://food.ec.europa.eu/safety/novel-food/authorisations/approval-insect-novel-food_en.
European Commission CORDIS. “Horizon 2020,” in SUStainable INsect CHAIN. Available at: https://cordis.europa.eu/project/id/861976. Project Description.
FAIRR (2016). Factory Farming. Assessing investment risks. Available online at: https://www.fairr.org/resources/reports/factory-farming-assessing-investment-risks.
FAO (2023a). Pathways towards lower emissions – A global assessment of the greenhouse gas emissions and mitigation options from livestock agrifood systems (Rome: Food and Agriculture Organization of the United Nations). doi: 10.4060/cc9029en
FAO (2023b). Achieving SDG 2 without breaching the 1.5 °C threshold: A global roadmap, Part 1: How agrifood systems transformation through accelerated climate actions will help achieving food security and nutrition, today and tomorrow, In brief (Rome, Italy: FAO). doi: 10.4060/cc9113en
Federal Democratic Republic of Ethiopia (2021). Ethiopia’s updated NDC. Available online at: https://unfccc.int/sites/default/files/NDC/2022-06/Ethiopia%27s%20updated%20NDC%20JULY%202021%20Submission_.pdf.
Flint M., Bowles S., Lynn A., Paxman J. R. (2023). Novel plant-based meat alternatives: future opportunities and health considerations. Proc. Nutr. Soc. 82, 1–16. doi: 10.1017/S0029665123000034
Franks B., Ewell C., Jacquet J. (2021). Animal welfare risks of global aquaculture. Sci. Adv. 7, eabg0677. doi: 10.1126/sciadv.abg0677
Garnett T., Appleby M. C., Balmford A., Bateman I. J., Benton T. G., Bloomer P., et al. (2013). Sustainable intensification in agriculture: premises and policies. Science 341, 33–34. doi: 10.1126/science.1234485
GFI (2022). 2021 State of the Industry Report, Plant-based meat, seafood, eggs and dairy. Good Food Institute.
Gilbert W., Thomas L. F., Coyne L., Rushton J. (2021). Review: mitigating the risks posed by intensification in livestock production: the examples of antimicrobial resistance and zoonoses. Animal 15, 100123. doi: 10.1016/j.animal.2020.100123
Giurfa M. (2015). Learning and cognition in insects: learning and insect cognition. Wiley Interdiscip. Reviews: Cogn. Sci. 6, pp 383–395. doi: 10.1002/wcs.1348
Global Methane Pledge (2023). Available online at: https://www.globalmethanepledge.org/resources/global-methane-pledge.
Godfray H. C. J. (2015). The debate over sustainable intensification. Food Secur. 7, 199–208. doi: 10.1007/s12571-015-0424-2
Godfray H. C. J., Aveyard P., Garnett T., Hall J. W., Key T. J., Lorimer J., et al. (2018). Meat consumption, health, and the environment. Science 361, eaam5324. doi: 10.1126/science.aam5324
Goff J. P. (2006). Macromineral physiology and application to the feeding of the dairy cow for prevention of milk fever and other periparturient mineral disorders. Anim. Feed Sci. Technol. 126, 237–257. doi: 10.1016/j.anifeedsci.2005.08.005
Goldberg A. M. (2016). Farm animal welfare and human health. Curr. Environ. Health Rep. 3, 313–321. doi: 10.1007/s40572-016-0097-9
Government of Canada Section 1. Foundation for healthy eating. Canada Food Guide. https://food-guide.canada.ca/en/guidelines/section-1-foundation-healthy-eating/.
Gov.uk (2021). Lobsters, octopus and crabs recognised as sentient beings. Wi.GOV.UK. 2021. Available at: https://www.gov.uk/government/news/lobsters-octopus-and-crabs-recognised-as-sentient-beings.
Grace D., Mutua F., Ochungo P., Kruska R., Jones K., Brierley L., et al. (2012). “Mapping of poverty and likely zoonoses hotspots,” in Zoonoses Project 4. Report to the UK Department for International Development (Nairobi: International Livestock Research Institute). Available at: https://assets.publishing.service.gov.uk/media/57a08a63ed915d622c0006fd/ZooMapDFIDreport18June2012FINALsm.pdf.
Gržinić G., Piotrowicz-Cieślak A., Klimkowicz-Pawlas A., Górny R. L., Ławniczek-Wałczyk A., Piechowicz L., et al. (2023). Intensive poultry farming: A review of the impact on the environment and human health. Sci. Total Environ. 858, 160014. doi: 10.1016/j.scitotenv.2022.160014
Haberkern H., Jayaraman V. (2016). Studying small brains to understand the building blocks of cognition. Curr. Opin. Neurobiology 37, 59–65. doi: 10.1016/j.conb.2016.01.007
Hanboonsong Y., Durst P. (2020). Guidance on sustainable cricket farming – A practical manual for farmers and inspectors. FAO. doi: 10.4060/cb2446en
Hartcher K. M., Lum H. K. (2020). Genetic selection of broilers and welfare consequences: a review. World’s Poultry Sci. J. 76, 154–167. doi: 10.1080/00439339.2019.1680025
Haugen-Kozyra K. (2021). Market-based tools for accelerating cattle sustainability in Canada. Anim. Front. 11, 17–25. doi: 10.1093/af/vfab038
Hawkey K. J., Lopez-Viso C., Brameld J. M., Parr T., Salter A. M. (2021). Insects: a potential source of protein and other nutrients for feed and food. Annu. Rev. Anim. Biosci. 9, 333–354. doi: 10.1146/annurev-animal-021419-083930
Hayek M. N. (2022). The infectious disease trap of animal agriculture. Sci. Adv. 8, eadd6681. doi: 10.1126/sciadv.add6681
Henry M., Jansen H., Amezcua M., del R., O’Sullivan T. L., Niel L., et al. (2021). Tail-Biting in pigs: A scoping review. Animals 11. doi: 10.3390/ani11072002
Honan M., Feng X., Tricarico J. M., Kebreab E. (2021). Feed additives as a strategic approach to reduce enteric methane production in cattle: modes of action, effectiveness and safety. Anim. Production Sci. 62, 1303–1317. doi: 10.1071/AN20295
Hossain A., Habibullah-Al-Mamun M., Nagano I., Masunaga S., Kitazawa D., Matsuda H. (2022). Antibiotics, antibiotic-resistant bacteria, and resistance genes in aquaculture: Risks, current concern, and future thinking. Environ. Sci. pollut. Res. 29, 1–22. doi: 10.1007/s11356-021-17825-4
Houzer E., Scoones I. (2021). “Are livestock always bad for the planet?,” in Rethinking the Protein Transition and Climate Change Debate (PASTRES, Brighton). doi: 10.19088/STEPS.2021.003
Hu Y., Cheng H., Tao S. (2017). Environmental and human health challenges of industrial livestock and poultry farming in China and their mitigation. Environ. Int. 107, 111–130. doi: 10.1016/j.envint.2017.07.003
Ingvartsen K. L., Dewhurst R. J., Friggens N. C. (2003). On the relationship between lactational performance and health: is it yield or metabolic imbalance that cause production diseases in dairy cattle? A position paper. Livestock Production Sci. 83, 277–308. doi: 10.1016/S0301-6226(03)00110-6
Integriculture (2022) IntegriCulture to accelerate cultivated meat commercialization capability with proprietary cell agriculture system, supported by Japanese authority grant. Available online at: https://integriculture.com/en/news/4754/.
Interafrican Bureau For Animal Resources (2019). Animal welfare strategy for africa (AWSA) executive summary. Available online at: https://rr-africa.woah.org/wp-content/uploads/2019/05/awsa_executive_summary_layout_eng_2017.pdf.
IPCC (2022). AR6 climate change 2021: impacts, adaptation and vulnerability. Available online at: https://www.ipcc.ch/report/sixth-assessment-report-working-group-ii/.
IPCC (2023). AR6 synthesis report: climate change 2023. Available online at: https://www.ipcc.ch/report/ar6/syr/.
Ites S., Smetana S., Toepfl S., Heinz V. (2020). Modularity of insect production and processing as a path to efficient and sustainable food waste treatment. J. Cleaner Production 248, 119248. doi: 10.1016/j.jclepro.2019.119248
Ivanovich C. C., Sun T., Gordon D. R., Ocko I. B. (2023). Future warming from global food consumption. Nat. Climate Change 13, 1–6. doi: 10.1038/s41558-023-01605-8
Jacquet J., Sebo J., Elder. M. (2017). Seafood in the future: bivalves are better. Solutions 8, 27–32.
Jones K. E., Patel N. G., Levy M. A., Storeygard A., Balk D., Gittleman J. L., et al. (2008). Global trends in emerging infectious diseases. Nature ;451, 990–993. doi: 10.1038/nature06536
Jori F., Hernandez-Jover M., Magouras I., Dürr S., Brookes V. J. (2021). Wildlife–livestock interactions in animal production systems: what are the biosecurity and health implications? Anim. Front. 11, 8–19. doi: 10.1093/af/vfab045
Kim H., Caulfield L. E., Garcia-Larsen V., Steffen L. M., Coresh J., Rebholz C. M. (2019). Plant-based diets are associated with a lower risk of incident cardiovascular disease, cardiovascular disease mortality, and all-cause mortality in a general population of middle-aged adults. J. Am. Heart Assoc. 8, e012865. doi: 10.1161/JAHA.119.012865
Klein C., Barron A. B. (2016). Insects have the capacity for subjective experience. Anim. Sentience 1. doi: 10.51291/2377-7478.1113
Korver D. R. (2023). Review: Current challenges in poultry nutrition, health, and welfare. animal 17, 100755. Selected keynote lectures of the 73rd Annual Meeting of the European Federation of Animal Science (Porto, Portugal). doi: 10.1016/j.animal.2023.100755
Krumel T. P., Goodrich C. (2023). Meatpacking working conditions and the spread of COVID-19. Appl. Economics 55, 3637–3660. doi: 10.1080/00036846.2022.2117776
Kumar P., Ahmed M. A., Abubakar A. A., Hayat M. N., Kaka U., Ajat M., et al. (2023). Improving animal welfare status and meat quality through assessment of stress biomarkers: A critical review. Meat Sci. 197, 109048. doi: 10.1016/j.meatsci.2022.109048
Lam Y., Fry J. P., Nachman K. E. (2019). Applying an environmental public health lens to the industrialization of food animal production in ten low-and middle-income countries. Globalization Health 15, 1–20. doi: 10.1186/s12992-019-0479-5
Lambert H., Elwin A., D’Cruze N. (2021). Wouldn’t hurt a fly? A review of insect cognition and sentience in relation to their use as food and feed. Appl. Anim. Behav. Sci. 243, 105432. doi: 10.1016/j.applanim.2021.105432
Lee D. Y., Lee S. Y., Yun S. H., Jeong J. W., Kim J. H., Kim H. W., et al. (2022). Review of the current research on fetal bovine serum and the development of cultured meat. Food Sci. Anim. Resour. 42, 775–799. doi: 10.5851/kosfa.2022.e46
Liceaga A. M., Aguilar-Toalá J. E., Vallejo-Cordoba B., González-Córdova A. F., Hernández-Mendoza A. (2022). Insects as an alternative protein source. Annu. Rev. Food Sci. Technol. 13, 19–34. doi: 10.1146/annurev-food-052720-112443
Lindholm C., Johansson A., Middelkoop A., Lees J. J., Yngwe N., Berndtson E., et al. (2018). The quest for welfare-friendly feeding of broiler breeders: effects of daily vs. 5:2 feed restriction schedules. Poultry Sci. 97, 368–377. doi: 10.3382/ps/pex326
Little A. (2021). Why Bugs Must Be a Bigger Part of the Human Food Chain (Bloomberg.Com). Available at: https://www.bloomberg.com/opinion/articles/2021-12-17/why-bugs-must-be-a-bigger-part-of-the-human-food-chain.
Llonch P., Haskell M. J., Dewhurst R. J., Turner S. P. (2017). Current available strategies to mitigate greenhouse gas emissions in livestock systems: an animal welfare perspective. Animal 11, 274–284. doi: 10.1017/S1751731116001440
Lloret J., Rätz H.-J., Lleonart J., Demestre M. (2016). Challenging the links between seafood and human health in the context of global change. J. Mar. Biol. Assoc. United Kingdom 96, 29–42. doi: 10.1017/S0025315415001988
Lundy M. E., Parrella M. P. (2015). Crickets are not a free lunch: protein capture from scalable organic side-streams via high-density populations of acheta domesticus. PloS One 10, e01187855. doi: 10.1371/journal.pone.0118785
Mackenzie J. S., Jeggo M. (2019). The one health approach—Why is it so important? Trop. Med. Infect. Dis. 4, 88. doi: 10.3390/tropicalmed4020088
Mathur M. B. (2022). Ethical drawbacks of sustainable meat choices. Science 375, 1362–1362. doi: 10.1126/science.abo2535
McCulloch S. P., Reiss M. J. (2017). The development of an Animal Welfare Impact Assessment (AWIA) tool and its application to bovine tuberculosis and badger control in England. J. Agric. Environ. Ethics 30, 485–510. doi: 10.1007/s10806-017-9684-5
McShane K. (2018). Why animal welfare is not biodiversity, ecosystem services, or human welfare: toward A more complete assessment of climate impacts. Les Ateliers l’éthique / Ethics Forum 13, 43–64. doi: 10.7202/1055117ar
Mellor D. J., Beausoleil N. J., Littlewood K. E., McLean A. N., McGreevy P. D., Jones B., et al. (2020). The 2020 five domains model: including human-animal interactions in assessments of animal welfare. Anim. an Open Access J. MDPI 10, 1870. doi: 10.3390/ani10101870
Mench J. A. (2002). Broiler breeders: feed restriction and welfare. World’s Poultry Sci. J. 58, 23–29. doi: 10.1079/WPS20020004
Merry F., Soares-Filho B. (2017). Will intensification of beef production deliver conservation outcomes in the Brazilian Amazon? Elementa. Sci. Anthropocene 5, 24. doi: 10.1525/elementa.224
Ministério da Saúde (2014). Guia alimentar para a população brasileira. Available online at: https://bvsms.saude.gov.br/bvs/publicacoes/guia_alimentar_populacao_brasileira_2ed.pdf.
Monteiro C. A., Cannon G., Moubarac J.-C., Martins A. P. B., Martins C. A., Garzillo J., et al. (2015). Dietary guidelines to nourish humanity and the planet in the twenty-first century. A blueprint from Brazil. Public Health Nutr. 18, 2311–2322. doi: 10.1017/S1368980015002165
Moruzzo R., Mancini S., Guidi A. (2021). Edible insects and sustainable development goals. Insects 12, 557. doi: 10.3390/insects12060557
Mulchandani R., Wang Y., Gilbert M., Boeckel T. P. V. (2023). Global trends in antimicrobial use in food-producing animals: 2020 to 2030. PloS Global Public Health 3, e0001305. doi: 10.1371/journal.pgph.0001305
Murray C. J., Ikuta K. S., Sharara F., Swetschinski L., Aguilar G. R., Gray A., et al. (2022). Global burden of bacterial antimicrobial resistance in 2019: a systematic analysis. Lancet 399, Pp 629–655. doi: 10.1016/S0140-6736(21)02724-0
Ni J. Q., Erasmus M. A., Croney C. C., Li C., Li Y. (2021). A critical review of advancement in scientific research on food animal welfare-related air pollution. J. Hazardous Materials 408, 124468. doi: 10.1016/j.jhazmat.2020.124468
Niklewicz A., Smith A. D., Smith A., Holzer A., Klein A., McCaddon A., et al. (2023). The importance of vitamin B12 for individuals choosing plant-based diets. Eur. J. Nutr. 62, 1551–1559. doi: 10.1007/s00394-022-03025-4
Nilsson M., Griggs D., Visbeck M. (2016). Policy: map the interactions between Sustainable Development Goals. Nature 534, 320–322. doi: 10.1038/534320a
Nordquist R. E., van der Staay F. J., Van Eerdenburg F. J. C. M., Velkers F. C., Fijn L., Arndt S. S. (2017). Mutilating procedures, management practices, and housing conditions that may affect the welfare of farm animals: implications for welfare research. Animals 7, 12. doi: 10.3390/ani7020012
Official Journal of the European Union (2021) Commission Regulation (EU) 2021/1372 of 17 August 2021 amending Annex IV to Regulation (EC) No 999/2001 of the European Parliament and of the Council as regards the prohibition to feed non-ruminant farmed animals, other than fur animals, with protein derived from animals. Available at: https://eur-lex.europa.eu/legal-content/EN/TXT/PDF/?uri=OJ:L:2021:295:FULL&from=EN.
OHHLEP, Adisasmito W. B., Almuhairi S., Behravesh C. B., Bilivogui P., Bukachi S. A., et al. (2022). One Health: A new definition for a sustainable and healthy future. PloS Pathog 18 (6), e1010537. doi: 10.1371/journal.ppat.1010537
Palangi V., Taghizadeh A., Abachi S., Lackner M. (2022). Strategies to mitigate enteric methane emissions in ruminants: A review. Sustainability 14, 13229. doi: 10.3390/su142013229
Pan A., Sun Q., Bernstein A. M., Manson J. E., Willett W. C., Hu F. B. (2013). Changes in red meat consumption and subsequent risk of type 2 diabetes: three cohorts of US men and women. JAMA Internal Med. 173, 1328–1335. doi: 10.1001/jamainternmed.2013.6633
Papier K., Knuppel A., Syam N., Jebb S. A., Key T. J. (2023). Meat consumption and risk of ischemic heart disease: A systematic review and meta-analysis. Crit. Rev. Food Sci. Nutr. 63, 1–12. doi: 10.1080/10408398.2021.1949575
Pellegrini P., Fernández R. J. (2018). Crop intensification, land use, and on-farm energy-use efficiency during the worldwide spread of the green revolution. Proc. Natl. Acad. Sci. 115, (10) 2335–2340. doi: 10.1073/pnas.1717072115
Perino G., Schwickert H. (2023). Animal welfare is a stronger determinant of public support for meat taxation than climate change mitigation in Germany. Nat. Food 4, 160–169. doi: 10.1038/s43016-023-00696-y
Plaizier J. C., Mulligan F. J., Neville E. W., Guan L. L., Steele M. A., Penner G. B. (2022). Invited review: Effect of subacute ruminal acidosis on gut health of dairy cows. J. Dairy Sci. 105, 7141–7160. doi: 10.3168/jds.2022-21960
Poore J., Nemecek T. (2018). Reducing food’s environmental impacts through producers and consumers. Science. 360, 987–992. doi: 10.1126/science.aaq0216
Preena P. G., Swaminathan T. R., Kumar V. J. R., Singh I. S. B. (2020). Antimicrobial resistance in aquaculture: a crisis for concern. Biologia 75, 1497–1517. doi: 10.2478/s11756-020-00456-4
Qian F., Riddle M. C., Wylie-Rosett J., Hu F. B. (2020). Red and processed meats and health risks: how strong is the evidence? Diabetes Care 43, 265–271. doi: 10.2337/dci19-0063
Ramamurthy R. (2019). Indian government grants over $600,000 to cell-based meat research. Good Food Institute.
Reynolds L. P., Wulster-Radcliffe M. C., Aaron D. K., Davis T. A. (2015). Importance of animals in agricultural sustainability and food security. J. Nutr. 145, 1377–1379. doi: 10.3945/jn.115.212217
Ritchie H., Rosado P., Roser M. (2022). Environmental Impacts of Food Production. Available at: https://ourworldindata.org/environmental-impacts-of-food.
Rodenburg T. B., Van Krimpen M. M., De Jong I. C., De Haas E. N., Kops M. S., Riedstra B. J., et al. (2013). The prevention and control of feather pecking in laying hens: identifying the underlying principles. World’s Poultry Sci. J. 69, 361–374. doi: 10.1017/S0043933913000354
Rowe A. (2020). Insects raised for food and feed — Global scale, practices, and policy. Rethink Priorities.
Santos M. N., Widowski T. M., Kiarie E. G., Guerin M. T., Edwards A. M., Torrey S. (2022). In pursuit of a better broiler: walking ability and incidence of contact dermatitis in conventional and slower growing strains of broiler chickens. Poultry Sci. 101, 101768. doi: 10.1016/j.psj.2022.101768
Scherer L., Tomasik B., Rueda O., Pfister S. (2018). Framework for integrating animal welfare into life cycle sustainability assessment. Int. J. Life Cycle Assess. 23, 1476–1490. doi: 10.1007/s11367-017-1420-x
Sebo J. (2022). Saving Animals, Saving Ourselves: Why animals matter for pandemics, climate change, and other catastrophes (New York: Oxford University Press). doi: 10.1093/oso/9780190861018.001.0001
Sebo J., Verkuijl C., Hotzel M., Achakulwisut P., Lima M., Green J. (2022). Sustainable development matters for animals too: governments have a responsibility to recognize that. CABI One Health. doi: 10.1079/cabionehealth.2022.0002
Sell-Kubiak E., Wimmers K., Reyer H., Szwaczkowski T. (2017). Genetic aspects of feed efficiency and reduction of environmental footprint in broilers: a review. J. Appl. Genet. 58, 487–498. doi: 10.1007/s13353-017-0392-7
Shepon A., Wu T., Kremen C., Dayan T., Perfecto I., Fanzo J., et al. (2023). Exploring scenarios for the food system–zoonotic risk interface. Lancet Planetary Health 7, e329–e335. doi: 10.1016/S2542-5196(23)00007-4
Sherwin C. M., Richards G. J., Nicol C. J. (2010). Comparison of the welfare of layer hens in 4 housing systems in the UK. Br. poultry Sci. 51, 488–499. doi: 10.1080/00071668.2010.502518
Shields S., Orme-Evans G. (2015). The impacts of climate change mitigation strategies on animal welfare. Animals 5, 361–945. doi: 10.3390/ani5020361
Shu C. (2020). Eat just to sell lab-grown meat in Singapore after gaining ‘world first’ Regulatory approval. TechCrunch(blog).
Sicard C. (2023). Can CRISPR Cut Methane Emissions From Cow Guts? (Davis: University of California). Available at: https://www.ucdavis.edu/food/news/can-crispr-cut-methane-emissions-cow-guts.
Silvestre A. M., Millen D. D. (2021). The 2019 Brazilian survey on nutritional practices provided by feedlot cattle consulting nutritionists. Rev. Bras. Zootecnia 50, e20200189. doi: 10.37496/rbz5020200189
Simonin D., Gavinelli A. (2019). The European Union legislation on animal welfare: state of play, enforcement and future activities. La Fondation Droit Animal Ethique Sci, 59–70.
Sinclair M., Lee N. Y., Hötzel M. J., de Luna M. C., Sharma A., Idris M., et al. (2022). International perceptions of animals and the importance of their welfare. Front. Anim. Sci. 3. doi: 10.3389/fanim.2022.960379
Singapore Food Agency (2020). Singapore Food Story R&D Programme Vol. 2020 (SFA). Available at: https://www.sfa.gov.sg/food-farming/singapore-food-story/r-and-d-programme.
Singer P. (2023). Animal Liberation Now: The Definitive Classic Renewed (New York: Diversion Books).
Sinke P., Swartz E., Sanctorum H., van der Giesen C., Odegard I. (2023). Ex-ante life cycle assessment of commercial-scale cultivated meat production in 2030. Int. J. Life Cycle Assess. 28, 234–254. doi: 10.1007/s11367-022-02128-8
Smith P. G., Bradley R. (2003). Bovine spongiform encephalopathy (BSE) and its epidemiology. Br. Med. Bull. 661, 185–198. doi: 10.1093/bmb/66.1.185
Sneddon L. U., Brown C. (2020). Mental capacities of fishes. In: Johnson L., Fenton A., Shriver A. (eds) Neuroethics and Nonhuman Animals. Advances in Neuroethics (Springer: Cham), 401–439. doi: 10.1007/978-3-030-31011-0_4
Sogari G., Bellezza Oddon S., Gasco L., van Huis A., Spranghers T., Mancini S. (2023). Review: Recent advances in insect-based feeds: from animal farming to the acceptance of consumers and stakeholders. animal 17, 100904. doi: 10.1016/j.animal.2023.100904
Sooksawasdi Na Ayudhya S., Kuiken T. (2021). Reverse zoonosis of COVID-19: lessons from the 2009 influenza pandemic. Veterinary Pathol. 58, 234–242. doi: 10.1177/0300985820979843
Spillias S., Valin H., Batka M., Sperling F., Havlík P., Leclère D., et al. (2023). Reducing global land-use pressures with seaweed farming. Nat. Sustainability 6, 380–390. doi: 10.1038/s41893-022-01043-y
Sun Z., Scherer L., Zhang Q., Behrens P. (2022). Adoption of plant-based diets across Europe can improve food resilience against the Russia–Ukraine conflict. Nat. Food 3, 905–910. doi: 10.1038/s43016-022-00634-4
Swedish Food Agency (2023). Eating habits and dietary guidelines – Adults. Available online at: https://www.livsmedelsverket.se/en/food-habits-health-and-environment/dietary-guidelines/adults.
Taylor L. H., Latham S. M., Woolhouse M. E. (2001). Risk factors for human disease emergence. Philos. Trans. R. Soc. London. Ser. B: Biol. Sci. 356, 983–989. doi: 10.1098/rstb.2001.0888
Temple D., Manteca X. (2020). Animal welfare in extensive production systems is still an area of concern. Front. Sustain. Food Syst. 4. doi: 10.3389/fsufs.2020.545902
The Monday Campaigns The global movement. In: (The Monday Campaigns). Available online at: https://www.mondaycampaigns.org/meatless-monday/the-global-movement (Accessed April 3, 2023).
Thomsen S. T., Assunção R., Afonso C., Boué G., Cardoso C., Cubadda F., et al. (2021). Human health risk–benefit assessment of fish and other seafood: a scoping review. Crit. Rev. Food Sci. Nutr. 62, 1–22. doi: 10.1080/10408398.2021.1915240
UNEP (2023). “Frontiers,” in What’s Cooking? An assessment of the potential impacts of selected novel alternatives to conventional animal products (Nairobi: United Nations Environment Programme). doi: 10.59117/20.500.11822/44236
United Nations (2015). Transforming our world. The 2030 agenda for sustainable development. Available online at: https://sustainabledevelopment.un.org/post2015/transformingourworld/publication.
van Boeckel T. P., Brower C., Gilbert M., Grenfell B. T., Levin S. A., Robinson T. P., et al. (2015). Global trends in antimicrobial use in food animals. Proc. Natl. Acad. Sci. United States America 112, 5649–5654. doi: 10.1073/pnas.1503141112
van Boeckel T. P., Pires J., Silvester R., Zhao C., Song J., Criscuolo N. G., et al. (2019). Global trends in antimicrobial resistance in animals in low- and middle-income countries. Science 365 (6459), eaaw1944. doi: 10.1126/science.aaw1944
van der Mark M., Nedeff E. (2021) Financial institutions and animal welfare. Sinergia Animal's review of the status of animal welfare from financial institutions in the Global North and South. Available online at: https://banksforanimals.org/media/filer_public/98/44/98441e7c-d7b8-4c82-b386-8c83bb8e1e61/sinergia_animal_2021-financial_institutions_and_animal_welfare.pdf.
van der Most P. J., de Jong B., Parmentier H. K., Verhulst S. (2011). Trade-off between growth and immune function: a meta-analysis of selection experiments. Funct. Ecol. 25, 74–80. doi: 10.1111/j.1365-2435.2010.01800.x
van Huis A., van Itterbeeck J., Klunder H., Mertens E., Halloran A., Muir G., et al. (2013). “Edible insects: future prospects for food and feed security,” in FAO Forestry Paper 171 (Food and Agriculture Organization of the United Nations, Rome). Available at: https://www.fao.org/3/i3253e/i3253e.pdf.
van Marle-Köster E., Visser C. (2021). Unintended consequences of selection for increased production on the health and welfare of livestock. Arch. Anim. Breed. 64, 177–185. doi: 10.5194/aab-64-177-2021
Vaughan A., Miguel-Pacheco G. G., de Passillé A. M., Rushen J. (2016). Reciprocated cross sucking between dairy calves after weaning off milk does not appear to negatively affect udder health or production. J. Dairy Sci. 99, 5596–5603. doi: 10.3168/jds.2015-9504
Vegconomist (2023). Oxford city council votes to make all internal events fully plant-based. Available online at: https://vegconomist.com/politics-law/oxford-city-council-internal-events-plant-based/.
Verkuijl C., Sebo J., Achakulwisut P., Lima M. G. B., Green J. (2022). “Mainstreaming animal welfare in sustainable development: a policy agenda,” in Stockholm+50 background paper series (Stockholm: Stockholm Environment Institute). Available at: https://www.sei.org/publications/mainstreaming-animal-welfare/.
Verkuijl C., Strambo C., Hocquet R., Butterfield R., Achakulwisut P., Boyland M., et al. (2023). A just transition in animal agriculture is necessary for more effective and equitable One Health outcomes. CABI One Health 2023). p.ohcs202300021. doi: 10.1079/cabionehealth.2023.0021
Vijn S., Compart D. P., Dutta N., Foukis A., Hess M., Hristov A. N., et al. (2020). Key considerations for the use of seaweed to reduce enteric methane emissions from cattle. Front. Veterinary Sci. 7, 1135. doi: 10.3389/fvets.2020.597430
von Eugen K., Nordquist R. E., Zeinstra E., van der Staay F. J. (2019). Stocking density affects stress and anxious behavior in the laying hen chick during rearing. Animals 53 (2), 53. doi: 10.3390/ani9020053
Wall E., Simm G., Moran D. (2010). Developing breeding schemes to assist mitigation of greenhouse gas emissions. animal 4, 366–376. doi: 10.1017/S175173110999070X
Wang T., He Q., Yao W., Shao Y., Li J., Huang F. (2019). The variation of nasal microbiota caused by low levels of gaseous ammonia exposure in growing pigs. Front. Microbiol. 10. doi: 10.3389/fmicb.2019.01083
Wang M., Wang Z., Lee Y., Lai H. T. M., de Oliveira Otto M. C., Lemaitre R. N., et al. (2022). Dietary meat, trimethylamine N-oxide-related metabolites, and incident cardiovascular disease among older adults: the cardiovascular health study. Arteriosclerosis Thrombosis Vasc. Biol. 42, e273–e288. doi: 10.1161/ATVBAHA.121.316533
Welfare Footprint Project. Available online at: http://www.welfarefootprint.org.
Whitton C., Bogueva D., Marinova D., Phillips C. J. (2021). Are we approaching peak meat consumption? Analysis of meat consumption from 2000 to 2019 in 35 countries and its relationship to gross domestic product. Animals 11, 3466. doi: 10.3390/ani11123466
WHO (2023). Ongoing avian influenza outbreaks in animals pose risk to humans. Situation analysis and advice to countries from FAO, WHO, WOAH. Available online at: https://www.who.int/news/item/12-07-2023-ongoing-avian-influenza-outbreaks-in-animals-pose-risk-to-humans (Accessed November 27, 2023).
Wickens S. (2022). Review of the evidence of sentience in cephalopod molluscs and decapod crustaceans. Anim. Welfare 31, 155–156. doi: 10.1017/S0962728600009866
Wiebers D. O., Feigin V. L. (2020). What the COVID-19 crisis is telling humanity. Neuroepidemiology 54, 283–286. doi: 10.1159/000508654
WOAH. (2021). Terrestrial Code Online Access. Available online at: https://www.woah.org/en/what-we-do/standards/codes-and-manuals/terrestrial-code-online-access/?id=169&L=1&htmfile=chapitre_aw_introduction.htm.
Woodyatt A., Wiener-Bronner D. (2020). Singapore Becomes First Country to Approve Lab-Grown Meat | CNN Business (CNN). Available at: https://www.cnn.com/2020/12/02/business/lab-grown-chicken-intl-scli-scn/index.html.
Xu X., Sharma P., Shu S., Lin T.-S., Ciais P., Tubiello F. N., et al. (2021). Global greenhouse gas emissions from animal-based foods are twice those of plant-based foods. Nat. Food 2, 724–732. doi: 10.1038/s43016-021-00358-x
Zhong V. W., Van Horn L., Greenland P., Carnethon M. R., Ning H., Wilkins J. T., et al. (2020). Associations of processed meat, unprocessed red meat, poultry, or fish intake with incident cardiovascular disease and all-cause mortality. JAMA Internal Med. 180, 503–512. doi: 10.1001/jamainternmed.2019.6969
Zhuge X., Zhou Z., Jiang M., Wang Z., Sun Y., Tang F., et al. (2021). Chicken-source Escherichia coli within phylogroup F shares virulence genotypes and is closely related to extraintestinal pathogenic E. coli causing human infections. Transboundary emerging Dis. 68, 880–895. doi: 10.1111/tbed.13755
Keywords: climate policy, One Health, public health, animal welfare, sustainable development, animal agriculture, mitigation
Citation: Verkuijl C, Smit J, Green JMH, Nordquist RE, Sebo J, Hayek MN and Hötzel MJ (2024) Climate change, public health, and animal welfare: towards a One Health approach to reducing animal agriculture’s climate footprint. Front. Anim. Sci. 5:1281450. doi: 10.3389/fanim.2024.1281450
Received: 22 August 2023; Accepted: 26 March 2024;
Published: 15 May 2024.
Edited by:
Ruth C. Newberry, Norwegian University of Life Sciences, NorwayReviewed by:
Peter Sandøe, University of Copenhagen, DenmarkMarc B. M. Bracke, Wageningen University and Research, Netherlands
Copyright © 2024 Verkuijl, Smit, Green, Nordquist, Sebo, Hayek and Hötzel. This is an open-access article distributed under the terms of the Creative Commons Attribution License (CC BY). The use, distribution or reproduction in other forums is permitted, provided the original author(s) and the copyright owner(s) are credited and that the original publication in this journal is cited, in accordance with accepted academic practice. No use, distribution or reproduction is permitted which does not comply with these terms.
*Correspondence: Rebecca E. Nordquist, ci5lLm5vcmRxdWlzdDFAdXUubmw=
†ORCID: Rebecca E. Nordquist, orcid.org/0000-0002-8541-5285
Maria José Hötzel, orcid.org/0000-0003-1034-3202