- 1DairyNZ Ltd, Hamilton, New Zealand
- 2DairyNZ Ltd, Lincoln, New Zealand
Producers in New Zealand’s pasture-based, seasonal dairy sector are striving to reduce nitrogen (N) losses to the environment whilst maintaining or increasing farm profitability. This study examined the cost-effectiveness of stacking different combinations of five N leaching mitigation strategies within the whole farm system; 1) reduced N fertilizer input, 2) off-paddock infrastructure, 3) recycling N by growing maize silage on a dedicated area on the farm using effluent as a fertilizer source followed by a catch-crop, 4) dietary salt supplementation to dilute urinary N, and 5) applying a nitrification inhibitor (NI) to slow the release of nitrate in the soil. The reference point (baseline) was a typical current dairy farm (CF) system in the Waikato region of New Zealand. We modelled four Future Farm scenarios by stacking mitigation strategies as follows: baseline plus reduced N fertilizer input, reduced stocking rate, and off-paddock infrastructure (FF); FF plus a dedicated maize block (FFP); FFP plus dietary salt (FFPS); and FFPS plus NI (FFPSNI). These systems were modelled using the Whole Farm Model coupled with the Urine Patch Framework, and APSIM models, using observed climate and economic input data over five consecutive years from 2013-2018. Relative to CF, the FF system achieved a N leaching reduction of 31% with a reduction in profit of 16%. The FFP system had a smaller N leaching reduction (22%), but the reduction in profit was smaller (11%). The fully stacked system (FFPSNI) demonstrated the largest leaching reduction of 33%, but also the largest profit reduction of 27%, compared with the CF. Stacking these five N mitigation strategies can achieve substantial N leaching reductions at the farm-scale. Including a dedicated, effluent-fertilized maize block followed by a catch-crop as part of the stack can reduce the negative impact on profitability but has a trade-off in N leaching. Farmers will have to weigh up these compromises between profit and leaching, considering risk factors not modelled here.
1 Introduction
Livestock production can exert many pressures on waterways - from habitat loss and increases in the amount of sediment, nutrients and microbial pathogens transferred to waterways (Steinfeld et al., 2006). Of the main contaminants, reducing the loss of nitrogen (N) is a particularly challenging problem for pasture-based dairy farmers because of the amounts of N brought into the farm system mainly in the form of fertilizer and feed (Monaghan and de Klein, 2014). Mitigating N losses from a dairy enterprise generally comes with cost implications to the farm business (Beukes et al., 2017). The challenge, therefore, is to identify strategies to reduce dairying’s N footprint, while minimizing the impact on farm profitability.
Pastoral 21 (P21), a set of national farm systems trials in New Zealand, took place between 2011- 2016 and evaluated various management strategies anticipated to reduce the environmental impact of dairying while maintaining profitability (Beukes et al., 2017). The P21 trial in the Waikato region compared two farm systems over the five-year period: 1) a typical farm (Current Farm = CF) with 3.2 cows/ha, average genetic merit, replacement rate 22%, 150 kg N fertilizer/ha, no off-paddock infrastructure, and 2) a mitigated future farm (Future Farm = FF) with 2.6 cows/ha, high genetic merit, replacement rate 18%, 50 kg N fertilizer/ha, and a standoff pad (or loafing pad where no feeding occurs) was used from March to June (autumn to early-winter) for removing some urinary N loading from pastured areas. The measured average annual N leaching loss from the FF was 43% less than from the CF. However, less N cycling through the system also meant lower pasture production, resulting in 4% less milk production from the FF and a 13% reduction in profit (Clark et al., 2019). Although the P21 trial demonstrated that substantial N leaching reductions are possible, the loss of profit (13%) was a concern and generated questions about the future resilience of farm businesses under environmental regulation. In many regulatory situations, there is no need for such large reductions (e.g., 43%), so the question was posed on how the system can be managed for a more balanced outcome for N leaching and profitability.
After conducting a literature review (e.g., Monaghan and de Klein, 2014; McDowell et al., 2021) and interviews with subject matter experts (e.g., Ross Monaghan and Richard McDowell, AgResearch, personal communication) and farmers in New Zealand, we identified eight key mitigation strategies that showed promise for effective N leaching reduction that was broadly applicable to dairy farms across New Zealand. These were in no order of priority; 1) genetically engineered (GE) ryegrass, 2) dietary salt supplementation, 3) lower N fertilizer inputs, 4) growing maize crop(s) fertilized with effluent, 5) applying a nitrification inhibitor (NI), 6) using off-paddock infrastructure (feed or standoff pad), 7) protecting natural attenuation assets on-farm (restored and constructed wetlands and riparian zones), and 8) capturing urine in-paddock at certain dedicated sites (via “cow toilets”).
One of these strategies, growing a maize crop and feeding it as silage, is becoming common practice on dairy farms in the North Island of New Zealand, e.g., the Waikato region. The extra feed grown is used to fill feed deficits that may develop in the drier summer months when temperate pastures (mainly perennial ryegrass-clover) can have low growth rates, or through winter when the slow growth from perennial ryegrass is not sufficient to sustain the non-lactating cows, or the extra feed can be used for more milk production. The land area used to grow these crops is frequently rotated around the farm and forms part of the farmers’ pasture renewal process where poor-performing pastures are first cropped before being returned to permanent pasture (Wall et al., 2020). Maize (Zea mays L.) is a high yielding (18-28 t DM/ha) crop that requires a significant amount of N to grow. Every ton of DM produced, requires 12 kg of N, so a 20 t DM/ha maize silage crop will require 240 kg N/ha (Scharf et al., 2002; Worku et al., 2007). It is a deep rooting plant with roots recorded at depths of 1.8 m (Kovács et al., 1995; Kristensen and Thorup-Kristensen, 2004), capable of utilizing N, and phosphorus, well below the ryegrass root depth. Further, maize silage is a low crude protein (7-8% CP) feed, which can be used to dilute excess protein from a pasture-dominant diet. The feeding of maize silage is considered as one of the mitigation strategies available to pastoral dairy farmers to reduce N excreted and loss from their farms (Romera et al., 2007). When effluent is used on the maize silage crop, more N is recycled inside the farm gate, which can reduce the need for imported synthetic N fertilizer. So, it was of particular interest to investigate the cost-effectiveness of including home-grown maize silage fed on a feed pad (normally a concrete surface so not suitable for extended periods of cow loafing) as one of the strategies in the stacked system. Previous work by Williams et al. (2019) indicated that a Waikato system with an effluent-fertilized crop area (“crop block”), which comprised 15% of the farm and grew maize silage in the summer and annual ryegrass in the winter, appeared promising in reducing N leaching while increasing milk production. However, they concluded that a more comprehensive analysis was needed to include the effect of climate variability on crop yield and the effect of milk and supplement price variation on profitability.
Two strategies that were identified as promising but have not been evaluated in farm systems investigations before were salt supplementation and nitrification inhibitor (NI). Salt supplementation increases cattle water intake and urination frequency, resulting in a lower urine N deposition rate and potentially a decrease in urine N leaching from urine patches. Strategic salt supplementation in autumn/early winter with feed has been identified as a potential mitigation option to decrease N leaching in grazed pastures (Ledgard et al., 2015). Nitrification inhibitors can be added to fertilizer or deposited directly onto grazed pasture. They are widely available overseas and, until 2011, an inhibitor called dicyandiamide (DCD) was used on some New Zealand farms (Di and Cameron, 2002). However, the discovery of trace residues in milk led to the withdrawal of DCD from the New Zealand market. Work funded by the New Zealand Agricultural Greenhouse Gas Research Consortium in recent years has identified a potential novel inhibitor in both field and laboratory trials that has demonstrated similar efficacy to DCD, but without the same risks. Preliminary information shows that it reduces N leaching losses by allowing more plant N uptake and reducing the need for imported fertilizer N. The advantage of this inhibitory product is that it is already widely used for other purposes, is approved for use in both humans and animals and has internationally agreed residue limits in food (NZAGRC, 2021).
Two strategies, lower N fertilizer and off-paddock infrastructure were already part of the P21 mitigation package and embedded in the FF system. Three of the eight strategies were not modelled. GE grass and “cow toilets” were identified as strategies that were not technologically mature enough i.e., they are classified as developing mitigations. Wetlands and riparian zones were not modelled because they are generally not applicable to all farming landscapes in New Zealand and require spatial data for individual farms when deciding on their size, location, and cost-efficiency (Tanner et al., 2022).
With continued sector focus on reducing N loss from grazed pastoral dairy land, the objective of this study was to build upon the work conducted in P21 and augment it through the modelling of key emerging stacked N mitigations. A particular focus was finding a stacked farm system that could deliver a more balanced outcome for N leaching reduction and impact on profitability i.e., worthwhile N leaching reductions (generally anything more than 10%) with smaller, and if possible zero negative impacts on profitability.
2 Materials and methods
2.1 Modelling approach
The DairyNZ Whole Farm Model (WFM), linked to APSIM (Agricultural Production Systems Simulator, Holzworth et al., 2014) via the Urine Patch Framework (Beukes et al., 2011) was used to simulate a typical Waikato Farm (CF) and a Future Farm (FF) as used in the P21 trial (Clark et al., 2019). The FF then became the new starting point from where alternative stacked systems (combining mitigation strategies) were evaluated for N leaching reductions and profitability. First, the FF was altered by adding a dedicated crop block comprising 15% of the farm (maize silage followed by a catch-crop) and using farm dairy effluent as the fertilizer source, and by replacing the standoff pad (no facilities for feeding) in FF with a feed pad for feeding the maize silage to the herd (FFP).Two further mitigations were added to FFP. Dietary salt supplementation (giving FFPS), and NI (giving FFPSNI). Key model inputs are summarized in Table 1. Each system was simulated over five consecutive seasons (farm season as defined from June one year to May the next; from 2013/14 to 2017/18; average 1,187 ± 281 mm rainfall/year) using observed daily climate (daily min. and max. temperature, solar radiation, potential evapotranspiration, and rainfall) driving the pasture model in WFM, and milk price data ($6.06 ± 1.84/kg fat + protein (milksolids; MS), range $4.06 – 8.68) for those seasons. The model predicting pasture growth rates (kg DM/ha/day) and yield (t DM/ha), crop yield (t DM/ha) and milk production (kg MS/cow/year), N leaching (kg/ha) under pasture (below 55 cm) and maize crop areas (below 162 cm because of deeper rooted maize), and operating profit (NZ$/ha). All relative changes and efficiencies were calculated for each of the five simulated seasons first, then averages and standard deviations were calculated across the seasons.
2.2 Soil, climate, and economic inputs
The WFM was initialized using a dominant soil under dairy in the Waikato region, a Horotiu silt loam soil (allophanic, moderately well drained) (Hewitt, 1998), and daily climate data from the NIWA meteorological station at Ruakura, Hamilton, Waikato region. For each of the five production seasons, farm operating costs (all in NZ$) from Economic Farm Surveys (www.dairynz.co.nz) were used as inputs to the model. In the FFP system, the feed pad was costed at $600/cow for construction and interest on borrowed capital at 5%, with depreciation over 25 years ($77/ha/year was added to farm working expenses). Feed pad maintenance was estimated at $3/cow/year (~$10/ha/year) and feeding out costed at $45/t DM supplement. In the FF system the standoff pad was costed at $875/cow ($112/ha) and $69/cow ($222/ha/year) for maintenance (Beukes et al., 2017). The higher maintenance cost of the standoff pad was mainly driven by the cost of replacing the woodchip bedding (required for extended hours of loafing). Feed and standoff pad costs were kept constant across the five simulated seasons. Feeding fine agricultural salt (Agsalt) was costed $39/ha (Farmlands, personal communication) and two applications of NI assuming the same price as DCD were costed $220/ha (Romera et al., 2017).
2.3 Cropping
The crop rotation on the FFP farm consisted of maize being directly drilled in October and harvested in March. Annual ryegrass was directly drilled in early April, harvested, and ensiled with the last cut in September, before the block went back into maize. No synthetic N fertilizer, other than effluent collected from the dairy shed and feed pad, was applied to the crop block (approximately 230 kg N/ha/year). All feed from the crop block was cut-and-carried and fed on the feed pad with assumed losses of 13% for maize and 15% for pasture silage. Maize yields were climate-driven in both WFM and APSIM models, which used daily input of actual climate data. Maize growing, plus harvesting costs, were assumed to be $3230/ha (for high fertility land) and for annual ryegrass it was $530/ha (Ian Williams, Pioneer Brand Products, personal communication). These cropping costs were kept constant across the simulated seasons. Maize silage yields ranged between 18 and 22 t DM/ha over the five years. Since there is no annual ryegrass model in the WFM, a user-defined yield of 5 t DM/ha was selected (Densley et al., 2006).
2.4 Dietary salt
The use of salt feeding (between Feb-May) coincides with the time when urinary N onto paddocks is most vulnerable to leaching loss (Shepherd et al., 2018). It is not recommended to feed salt to dairy cows in the month preceding calving because it can interfere with the calcium metabolism of the animal (Stewart Ledgard, pers. comm., AgResearch, April 2022). The effect of salt on urine volume and, therefore, the reduction in the urinary N concentration in cattle, can vary from 17 to 59% depending on breed, age, intake and environmental conditions (Ledgard et al., 2015). For our work we assumed a reduction of 20% (supported by Brendon Welten, pers. comm., AgResearch, March 2022). The effect of salt was implemented in the model by scaling the urine volumes deposited onto paddocks in the months February to May by 1.25, but keeping the amount of N excreted the same, which resulted in urinary N concentrations decreasing by 20%.
2.5 Nitrification inhibitor
The treatment with NI was simulated based on recommended best management practice for the use of DCD, which equates to one application in late April or early May, and one in late July or August (Romera et al., 2017), with the assumption that the novel NI will behave very similar to DCD and break down slower in the colder months of the year. Modelling the effects of NI on N leaching has shown that the month of application is important and also the number of days after urine was deposited, i.e., after the cows leave the paddock (Romera et al., 2017). Romera et al. (2017) demonstrated that the N leaching risk can be reduced by up to 35% if NI is applied immediately following the cows in late April/early May, and by 43% in late July/early August. Nevertheless, an application immediately following the cows is not always practical, so we assumed a delay of up to five days after the cows had left the paddock before the NI is applied. This resulted in assumed reductions in leaching risk of 32 and 40% for April and July applications, respectively (Romera et al., 2017). There is no NI module in APSIM, so the effects of NI were implemented by reducing the amount of urinary N that was actually deposited onto the paddocks by 32 and 40% for April and July, respectively. This was done by adding another set of calculations in the data flow between WFM where urinary N output per cow is simulated, and the Urine Patch Framework and APSIM where N leaching from urine patches is simulated. This adaptation had the same effect as NI slowing the release of N from the ammonium to nitrate form.
3 Results
Average annual pasture yield for the FF was 1.8 t DM/ha lower than for the CF due primarily to reduced N fertilizer use and stocking rate (Table 2). Pasture yield for the FFP was 0.7 t DM/ha/yr higher than for the FF. Although similar amounts of synthetic N fertilizer were used, stocking rate on the FFP was higher than on the FF and 15% of the pasture area was taken out of pasture production for the dedicated maize crop block. This combination of higher stocking rate and smaller pasture area resulted in a higher grazing pressure on the FFP with resultant lower post-grazing pasture masses (residuals). Within a certain range, lower post-grazing residuals allow pastures to remain in the photosynthetic efficient state and produce more biomass (Lee et al., 2008). The addition of the maize cropping increased annual production on the FFP by 141 kg MS/ha compared with the CF, and 275 kg MS/ha compared with the FF. This was due to the FFP having the same stocking rate as CF but higher genetic merit cows producing more MS per cow. Whereas compared with the FF the genetic merit of the cows was the same, but FFP had a higher stocking rate (3.2 cf 2.6 cows/ha), supported by the extra feed grown on the crop block.
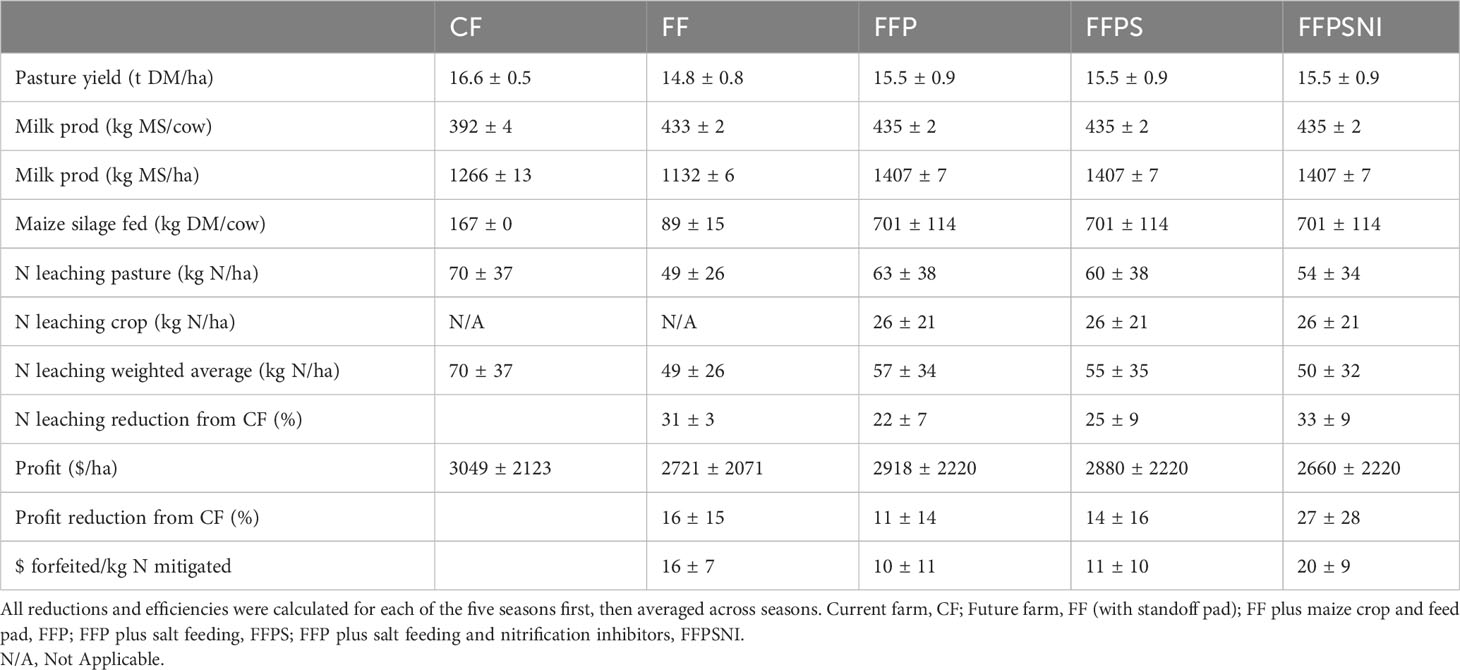
Table 2 Modelled results (mean ± SD) for five consecutive seasons from 2013/14 to 2017/18 for the Waikato dairy system.
Nitrogen leaching from the pasture block was highest for the CF (70 kg N/ha) and lowest for the FF (49 kg N/ha) because of the combination of a reduction in N fertilizer input and the removal of substantial amounts of urinary N from the paddocks due to restricted grazing (standoff infrastructure) in the FF. Because of the ability of the maize to capture more recycled N and keep it on-farm in the crop biomass, more N cycled through the FFP herd and a proportion of it was deposited on the pasture resulting in higher leaching via urine patches compared with the FF (63 kg N/ha). In the case of the FFP, the feed pad contributed to the capture and recycling of N because of the time cows spent on this structure (3 h/day) and the assumption that 84% of urinary N deposited in the dairy parlor (2 h/day) and on the feed pad was recycled. In FFPS, leaching from pasture was reduced, by using dietary salt supplementation, to 60 kg/ha (5% lower than FFP), and this was further reduced to 54 kg/ha by using NI in FFPSNI (10% lower than FFPS). The average N leaching from the crop block was 26 kg N/ha (range 8 to 62 kg N/ha), which was skewed by the relatively high leaching in the above-average drainage year of 2017/18 (717 mm versus an average of 423 mm drainage). However, leaching from the crop block was lower than from the pasture block due to the stable state of the soil with very little extra N mineralization because the crop block was dedicated to one location on-farm and not migrated across the farm, which increases the soil mineral N pool post cultivation of pasture. Furthermore, the deep rooting nature of maize (leaching only recorded below 162 cm), winter growth of the catch-crop (annual ryegrass), and the absence of grazing animals (urine patches) on the block reduced N leaching. The dilution effect of the crop block on the overall farm leaching (weighted mean) meant that the FFP had a N leaching loss of 57 kg N/ha compared with the 49 kg N/ha for the FF and the 70 kg N/ha for the CF. The N leaching was further reduced to 55 kg N/ha in FFPS, and 50 kg N/ha in FFPSNI (Table 2).
Compared with the CF as a baseline, the FFP scenario achieved an average N leaching reduction of 22% while the FF achieved 31%. The reductions for FFPS and FFPSNI were 25 and 33%, respectively, although reductions showed large variability across the modelled years (Figure 1A).
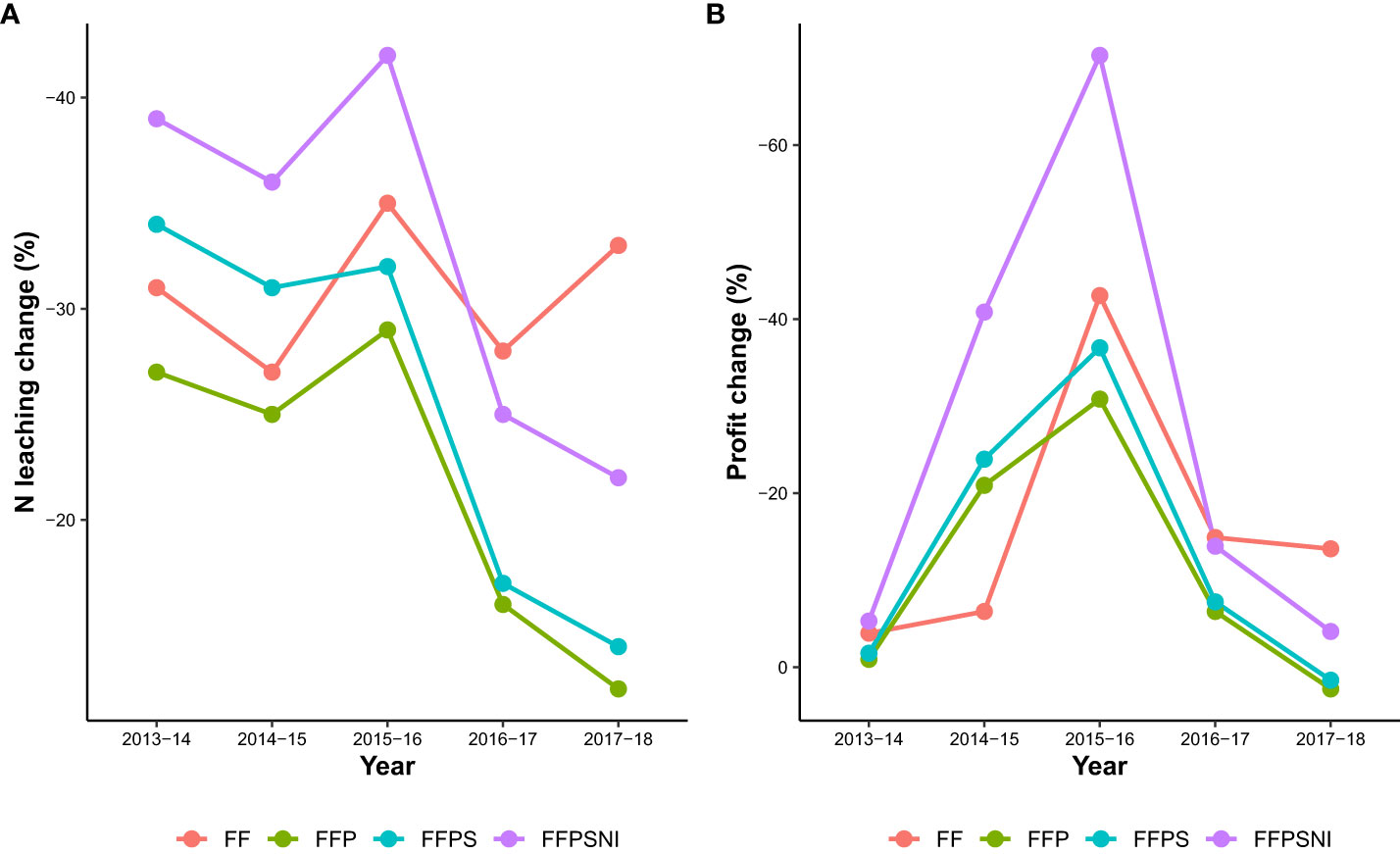
Figure 1 Predicted percentage change in (A) nitrogen (N) leaching and (B) operating profit for the Future Farm (FF; standoff pad), Future Farm plus maize crop (FFP; feed pad), FFP plus salt (FFPS; feed pad), and FFP plus salt and nitrification inhibitors (FFPSNI; feed pad) relative to the Current Farm (CF) over five simulated years.
FFP had an operating profit reduction of 11% compared with 16% in the FF, resulting in the FFP having a cost of mitigating of $10/kg N compared with $16/kg N mitigated in the FF. The addition of salt and NI resulted in profit reductions of 14% in FFPS and 27% in FFPSNI, with large variability across modelled years (Figure 1B). Although leaching reduction was greatest in FFPSNI, it was also the most expensive at $20/kg N mitigated (Table 2).
4 Discussion
Observed results from the P21 trial (2011-16), using actual milk prices (average of $6.08/kg MS), showed average operating profit of $2,086 for the CF and $1,807 for the FF, a reduction of $279/ha (Clark et al., 2019). On-farm measured leaching reduction in the FF compared with the CF was on average 43% over the five years. Our modelling study was based on the P21 trial design but differed from the actual trial by running simulations over a different set of five years (2013-18) presenting a different set of climatic drivers. Despite this difference, our predicted reductions in the FF compared with the CF of $328/ha for operating profit (average milk price of $6.06/kg MS) and 31% for N leaching indicate that our models captured the principles of the stacked N mitigations in FF and the effect of these on N leaching and operating profit in this comparison. Although comparing model output with observed data was not the objective here, it provides confidence that the models can be used to extrapolate the P21 results, including the alternative FFP, FFPS and FFPSNI systems, into untested climate and price scenarios and that the right trends would emerge.
The variation in N leaching reductions for the alternative systems over the five modelled years (Figure 1A) is largely due to variation in annual drainage (228 to 717 mm), which resulted in N leaching from CF varying from 35 to 106 kg N/ha over the five-year period. The most stacked system (FFPSNI) achieved the greatest N leaching reduction in three of the five years, but the least stacked system (FF) achieved the greatest reduction in the two high-drainage years (2016-17 and 2017-18). The year 2017-18 had the highest drainage (717 mm). In that year, the FF had a reduction in N leaching of 33% compared with 12% in the FFP, 14% in FFPS and 22% in FFPSNI (Figure 1A). The moderate leaching reduction in these systems in this high-drainage year is due to the high leaching (62 kg N/ha) predicted for the maize block. Considering that the maize yield in this year was average, it is possible that the large drainage volume removed the soil mineral N to below the root zone thus reducing the N nutrient pool available for maize uptake (lowering yield) in this high rainfall year (1,465 mm compared to an average of 1,187 mm). The result that leaching reduction was greatest in FF in these high-drainage years can be explained by the absence of a crop block in this system and the lower N leaching rate from the permanent pasture cover aided by the lower stocking rate and duration-controlled grazing (standoff) to reduce urinary N deposition onto paddocks when plant uptake is low.
Figure 1B shows that profit changes for the alternative systems compared with the CF varied considerably over the five years. The highest milk price ($8.68/kg MS) was in 2013-14 and the lowest ($4.06/kg MS) in 2015-16. Profit changes for these years show that the mitigation costs in the stacked systems result in more profit squeezed in years with a low milk price compared with years with a high milk price. In four of the five years, FFP and FFPS had the smallest profit reduction which was driven by higher milk production and revenue per hectare for these systems. Although production and revenue for the FFPSNI system matched this, it does not follow the same trend because of the relatively high cost of the NI. The year 2014-15 had a relatively low milk price ($4.58/kg MS) favoring the FF system which showed the lowest profit change. This system had a lower stocking rate, less imported feed and no cropping costs. It is known that systems with lower input costs generally show more of an advantage when the milk price is low, but also have less agility for profit advantage when the milk price is above average (Neal and Roche, 2019).
One of the main goals of this study was to evaluate alternatives to the FF system that would achieve worthwhile N leaching reductions while simultaneously eliminating or reducing the negative impact on farm profitability. Our modelling indicated that none of the systems could eliminate the negative impact on profitability; however, two systems, FFP and FFPS, reduced the adverse impacts on profitability. Although both achieved worthwhile leaching reductions, they could not match FF. On the other hand, the FFPSNI system matched the leaching reduction of FF, but at a prohibitive cost to profitability (-27%). There are compromises between leaching reduction and reducing the negative impact on profitability and farmers will have to determine where the best compromise lies for their situation. This decision will largely be affected by their N leaching targets and financial situation, but also by other risk factors not considered in this modelling exercise e.g., debt levels affecting appetite for off-paddock infrastructure, their region and potential for crop failure, complexity of the system, skill levels and extra labor requirements. An example would be the FF versus the FFP systems where farmers will have to decide if reducing a profit loss by 5% is worth giving up 9% on leaching reduction, and more importantly, is it worth changing to a more complex system with cropping and feeding maize silage on a feed pad. This trade-off example can be taken further by weighing up a reduction in profit loss of 2% against giving up 6% in leaching reduction (compared with FF, Table 2), but then facing the added complexity of feeding salt to cows in summer-autumn in the FFPS system.
The mitigation costs of $16/per kg N for the FF and $10/per kg N mitigated for the FFP are relatively low compared with abatement costs in the literature of $50 per kg N for NI and $32 - 37 per kg N achieved with standoff (duration-controlled grazing) (Romera et al., 2017). This is probably because in both FF and FFP, the focus was not on a single technology but rather a combination of system changes (N fertilizer, stocking rate, cow genetic merit, low-protein supplements, dedicated crop block) and off-paddock infrastructure. Combinations of mitigation options, where the focus is on increasing N use efficiency so less N is available for loss to the environment appear to be more cost-effective than single mitigation options where an expensive additive (NI) or infrastructure (off-paddock facilities) are used. Compared to $50/kg N from Romera et al. (2017) our estimate for the cost of NI alone came to $44/kg N. This is a relatively expensive strategy and contributed to the high cost of mitigation for the FFPSNI system of $20/kg N mitigated.
A typical temperate, pasture-based dairy farm in the Waikato region of New Zealand can achieve N leaching reductions of 31% by stacking N mitigations including off-paddock infrastructure (standoff), but the associated profit reduction can be substantial (16%). Adding a dedicated maize block to this system and changing the standoff to a lower-cost feed pad, can achieve worthwhile leaching reductions of 22%, while softening the negative impact on profitability (11%). A dedicated crop block as part of the mitigation approach can be favorable when leaching targets are moderate and when there are reasons to maintain stock numbers and milk production. Caveats are that the crop block should not be migrated across the farm property, but rather maintained in the same location, to reduce the risk of N mineralization when perennial pasture is tilled into a crop block, and the maize should be followed by a catch-crop (e.g., annual ryegrass). Maize is established with minimal cultivation, only effluent N is recycled onto the crop, yields are above average, and the catch-crop is cut-and-carry harvested and not grazed in-situ during winter (Williams et al., 2019). A feed pad is also required to increase feed utilization through reduced wastage and capture effluent for reuse as an organic fertilizer source. Furthermore, the home-grown maize crop is used to reduce the need for imported feed-N. It should be noted that there are questions on the practicality and desirability of continuous maize cropping on the same block, especially in terms of disease pressure, and soil carbon stocks (Wall et al., 2020).
Stacking five of the eight top-rated N mitigation strategies has demonstrated the potential to achieve substantial N leaching reductions at farm-scale. The choice of stacked options will depend on the leaching target and if and how much farmers are prepared to sacrifice profitability to future-proof their businesses.
Data availability statement
The original contributions presented in the study are included in the article/supplementary material. Further inquiries can be directed to the corresponding author.
Author contributions
PB: Writing – original draft, Writing – review & editing. CD: Writing – review & editing. KM: Writing – review & editing. DS-V: Conceptualization, Project administration, Writing – review & editing.
Funding
The author(s) declare financial support was received for the research, authorship, and/or publication of this article. This work was funded by New Zealand dairy farmers through DairyNZ Inc as part of Solutions at Scale -Farm Systems Modelling (2002) and Catchment Level Solutions (8075).
Acknowledgments
We acknowledge internal reviews by our colleagues Chris Glassey, Paul Edwards and Claire Phyn, who contributed to improving the manuscript.
Conflict of interest
Authors PB, CD, KM, and DS-V were employed by the company DairyNZ Ltd.
Publisher’s note
All claims expressed in this article are solely those of the authors and do not necessarily represent those of their affiliated organizations, or those of the publisher, the editors and the reviewers. Any product that may be evaluated in this article, or claim that may be made by its manufacturer, is not guaranteed or endorsed by the publisher.
References
Beukes P. C., Romera A. J., Gregorini P., Clark D. A., Chapman D. F. (2011). Using a whole farm model linked to the APSIM suite to predict production, profit, and N leaching for next generation dairy systems in the Canterbury region of New Zealand. In: Proceedings of the 19th International Congress on Modelling and Simulation, Perth, Australia, 12-16 December 2011. Available at: http://mssanz.org.au/modsim2011.
Beukes P. C., Romera A. J., Gregorini P., Macdonald K. A., Glassey C. B., Shepherd M. A. (2017). The performance of an efficient dairy system using a combination of nitrogen leaching mitigation strategies in a variable climate. Sci. Total Environ. 599-600, 1791–1801. doi: 10.1016/j.scitotenv.2017.05.104
Clark D. A., Macdonald K. A., Glassey C. B., Roach C. G., Woodward S. L., Griffiths W. M., et al. (2019). Production and profit of current and future dairy systems using differing nitrogen leaching mitigation methods; the Pastoral 21 experience in Waikato. New Zeal. J. Agr. Res. 63 (4), 505–528. doi: 10.1080/00288233.2019.1577276
Densley R. J., Austin G. M., Williams I. D., Tsimba R., Edmeades G. O. (2006). Maize silage and winter crop options to maximise drymatter and energy for NZ dairy systems. Pr. N. Z. Grassl. Assoc. 68, 193–197. doi: 10.33584/jnzg.2006.68.2647
Di H. J., Cameron K. C. (2002). The use of a nitrification inhibitor, dicyandiamide (DCD), to decrease nitrate leaching and nitrous oxide emissions in a simulated grazed and irrigated grassland. Soil Use Manage. 18, 395–403. doi: 10.1111/j.1475-2743.2002.tb00258.x
Holzworth D. P., Huth N. I., deVoil P. G., Zurcher E. J., Herrmann N. I., McLean G., et al. (2014). Evolution towards a new generation of agricultural systems simulation. Environ. Modell. Software 62, 327–350. doi: 10.1016/j.envsoft.2014.07.009
Kovács G. J., Németh T., Ritchie J. T. (1995). Testing simulation models for the assessment of crop production and nitrate leaching in Hungary. Agr. Syst. 49, 385–397. doi: 10.1016/0308-521X(95)00032-Z
Kristensen H. L., Thorup-Kristensen K. (2004). Root growth and nitrate uptake of three different catch crops in deep soil layers. Soil Sci. Soc America J. 68, 529–537. doi: 10.2136/sssaj2004.5290
Ledgard S. F., Welton B., Betteridge K. (2015). Salt as a mitigation option for decreasing nitrogen leaching losses from grazed pastures. J. Sci. Food Agric. 95, 3033–3040. doi: 10.1002/jsfa.7179
Lee J. M., Donaghy D. J., Roche J. R. (2008). Effect of defoliation severity on regrowth and nutritive value of perennial ryegrass (Lolium perenne L.) dominant swards. Agron. J. 100, 308–314. doi: 10.2134/agronj.2007-0099
McDowell R. W., Monaghan R. M., Smith C., Manderson A., Basher L., Burger D. F., et al. (2021). Quantifying contaminant losses to water from pastoral land uses in New Zealand III. What could be achieved by 2035? NZ J. Agric. Res. 64 (3), 390–410. doi: 10.1080/00288233.2020.1844763
Monaghan R. M., de Klein C. A. M. (2014). Integration of measures to mitigate reactive nitrogen losses to the environment from grazed pastoral dairy systems. J. Agric. Sci. 152, S45–S56. doi: 10.1017/S0021859613000956
Neal M., Roche J. R. (2019). Profitable and resilient pasture-based dairy farm businesses in New Zealand. Anim. Prod. Sci. 60, 169–174. doi: 10.1071/AN18572
New Zealand Agricultural Greenhouse Gas Research Centre (NZAGRC) (2022) 2020-2021 Annual Highlights. Available at: www.nzagrc.org.nz (Accessed June 19, 2023).
Romera A. J., Cichota R., Beukes P. C., Gregorini P., Snow V., Vogeler I. (2017). Combining restricted grazing and nitrification inhibitors to reduce nitrogen leaching on New Zealand dairy farms. J. Environ. Qual. 46, 72–79. doi: 10.2134/jeq2016.08.0325
Romera A. J., Palliser C. C., Beukes P. C. (2007). “Reducing nitrogen leaching in a pastoral dairy farm by replacing nitrogen fertiliser with maize silage. Pp 600-604,” in Meeting the Challenges for Pasture-Based Dairying. Proceedings of the 3rd Dairy Science Symposium, University of Melbourne, Victoria, Australia, 18-20 September 2007.
Scharf P. C., Wiebold W. J., Lory J. A. (2002). Corn yield response to nitrogen fertiliser timing and deficiency level. Agron. J. 94, 435–441. doi: 10.2134/agronj2002.4350
Shepherd M., Lucy G., Vogeler I., Balvert S. (2018). The effect of drought and nitrogen fertiliser addition on nitrate leaching risk from a pasture soil; an assessment from a field experiment and modelling. J. Sci. Food Agric. 98, 3795–3805. doi: 10.1002/jsfa.8893
Steinfeld H., Gerber P., Wassenaar T. D., Castel V., Rosales M., de Haan C. (2006). Livestock’s Long Shadow: Environmental Issues and Options (Rome: FAO).
Tanner C. C., Depree C. V., Sukias J. P. S., Wright-Stow A. E., Burger D. F., Goeller B. C. (2022) Constructed Wetland Practitioners Guide: Design and Performance Estimates (Hamilton, New Zealand: DairyNZ/NIWA) (Accessed June 22, 2023).
Wall A. M., Campbell D. I., Morcom C. P., Mudge P. L., Schipper L. A. (2020). Quantifying carbon losses from periodic maize silage cropping of permanent temperate pastures. Agric. Ecosys. Environ. 301, 107048. doi: 10.1016/j.agee.2020.107048
Williams I., Beukes P., Densley R. (2019). “Dedicated cropping blocks within Dairy Farms: Using known mitigation strategies at a farm systems level,” in Nutrient Loss Mitigations for Compliance in Agriculture. Eds. Currie L. D., Christensen C. L. (Palmerston North, New Zealand: Fertilizer and Lime Research Centre, Massey University), 5. Available at: http://flrc.massey.ac.nz/publications.html.
Keywords: modelling, N input, off-paddock infrastructure, recycling N, maize block, salt, nitrification inhibitor, operating profit
Citation: Beukes PC, Depree C, Macintosh KA and Silva-Villacorta D (2024) Stacking nitrogen mitigation strategies for future pasture-based dairy farms: impacts on leaching and profit. Front. Anim. Sci. 5:1277131. doi: 10.3389/fanim.2024.1277131
Received: 14 August 2023; Accepted: 25 January 2024;
Published: 09 February 2024.
Edited by:
Innocent Rugoho, Independent Researcher, Melbourne, VIC, AustraliaReviewed by:
Anita Fleming, Lincoln University, New ZealandRachelle Meyer, The University of Melbourne, Australia
Copyright © 2024 Beukes, Depree, Macintosh and Silva-Villacorta. This is an open-access article distributed under the terms of the Creative Commons Attribution License (CC BY). The use, distribution or reproduction in other forums is permitted, provided the original author(s) and the copyright owner(s) are credited and that the original publication in this journal is cited, in accordance with accepted academic practice. No use, distribution or reproduction is permitted which does not comply with these terms.
*Correspondence: Pierre C. Beukes, pierre.beukes@dairynz.co.nz