- 1Department of Livestock and Pasture Science, University of Fort Hare, Alice, South Africa
- 2Risk and Vulnerability Science Center, University of Fort Hare, Alice, South Africa
- 3Department of Rural Development and Agrarian Reform, Grahamstown Veterinary Laboratory, Makhanda, South Africa
Introduction: Feedlots have emerged as an ideal mitigation option to pursue sustainable and efficient livestock production. This paper aims to elucidate how the establishment and widespread adoption of feedlots have provided solutions to complex problems of food security, animal welfare, and environmental sustainability.
Methods: An observational cross-sectional study compared fecal egg count per gram (EPG), weight gain, and body condition score (BCS) in feedlot and non-feedlot cattle at high throughput abattoirs. Cattle (n = 120) of different age and sex groups, farms, and breeds were selected from two commercial abattoirs (EA1 and EA2) in the Eastern Cape Province.
Results: At EA1, non-feedlot cattle exhibited higher EPG values (323.3±28.9) than feedlot cattle (73.3±13.3), indicating a potentially greater susceptibility to internal parasitic infections. The similar weight gains between feedlot (298.1±4.7) and non-feedlot cattle (287.16±7.79) were attributed to the sufficient natural pasture in the communally raised cattle. However, body condition scores were significantly (P< 0.05) better in feedlot than in non-feedlot cattle. Nonetheless, the feedlot farms of origin had a significant effect (P< 0.05) on the EPG and body condition score values in EA1, with no significant effect in EA2.
Discussion: Moreover, the negative correlation between EPG and body condition scores highlights that as parasite load increases, there might be a subtle tendency for body condition to decrease. These results underscore the importance of feedlots as an effective management strategy to improve animal health and productivity. Further investigations into the factors driving the differences in non-feedlot cattle are needed for informed decision-making in livestock management and abattoir operations.
1 Introduction
Feedlots in cattle production have become a prominent practice to raise and finish cattle efficiently for the meat industry (Sifiso et al., 2013). South Africa produces approximately 21.4% of the total meat produced on the continent and 1% of global meat production (Saki and Hoffmann, 2020). In addition, livestock industry contributes 34.1% to the total domestic agricultural production and provides 36% of the population’s protein needs (Rich et al., 2022). This makes livestock production one of the most important farming practices for economic growth and food security. The South African Red Meat sub-sector is divided into commercial and smallholder or emerging sectors (RMRDSA, 2018). In both sectors, cattle are vital in meeting the growing global demand for animal-derived products and economic sustainability.
Cattle are pivotal in meeting the ever-increasing global demand for meat and dairy products. Despite the pivotal role played by the beef sector in South Africa, the agriculture industry is currently the most significant negative contributor (12.3%) to the decrease in the gross domestic product (GDP) (-0.4%), primarily due to a decline in the production of animal products and field crops (Stats SA, 2023). The increase in human population in urban and rural communities resulted in an increased demand for fresh meat products, thus requiring an increase in beef cattle slaughtered at the abattoirs. Formidable challenges such as resource depletion, land scarcity, water constraints, and greenhouse gas emissions further affect beef production. The limitations of extensive grazing systems, which often require vast expanses of land and contribute significantly to deforestation, have led to a quest for more efficient and resource-conserving cattle-rearing methods. Feedlots have emerged as an effective solution to ensure a sustainable future for livestock production due to their reduced land requirements while optimizing feed utilization and improving growth rates and animal health (Nyhodo et al., 2014).
While feedlot prominence is evident, there exist research gaps on the impacts of feedlots on factors such as animal welfare, food safety, and environmental sustainability. Some studies involving dairy and beef cattle described the existence of a genetic antagonism between animal resistance to worms and overall postweaning growth (Jackson, 2013), while other researchers have reported low or no correlation between these variables (Heckler et al., 2016; Zapa et al., 2021). The ongoing impact of parasitic diseases on non-feedlot cattle has intrigued an interest in delving into alternative feedlot production systems (Scholtz et al., 2013). The significant parasites that affect cattle rearing in tropical and subtropical regions are gastrointestinal nematodes (Mertz et al., 2005; Charlier et al., 2009; Zapa et al., 2021). The main gastrointestinal nematodes that parasitize bovines are those of the genera Cooperia, Haemonchus, Trichostrongylus, Nematodirus, and Ostertagia. The genus Cooperia (particularly the species Cooperia punctata) is the most prevalent nematode in cattle in several regions of the globe, such as Africa, South America, the United States, and Asia (Wymann et al., 2007; Rabelo et al., 2010; Ramünke et al., 2018). Cattle are more susceptible to parasitic infections, which lead to decreased feed intake, poor weight gain, and compromised immune function, thereby affecting the overall health and productivity of the animals (Zapa et al., 2021; Trindade et al., 2023).
Cattle feedlot conditions have been a subject of considerable interest due to their potential impact on animal performance. This study aims to investigate the hypothesis that feedlot conditions do not significantly affect body condition scores, weight gain, and fecal egg counts of cattle. This paper, therefore, examines the role of feedlots in cattle production, addressing a pressing need for a balanced understanding of their advantages and drawbacks. By shedding light on the differences in health indicators between feedlot and non-feedlot cattle, this study aims to promote sustainable livestock production and pave the way for making informed decisions about management practices. The state of health of cattle before slaughter is also crucial for ensuring well-being of the animals and maintaining the safety and quality of the final meat products (Jaja et al., 2017a) So, the objective of the present study was to evaluate the body condition scores, parasite egg loads (determined by fecal egg counts; eggs per gram in a mixed infection), and weight gain of feedlot and non-feedlot cattle slaughtered at high throughput abattoirs in the Eastern Cape Province, South Africa.
2 Materials and method
2.1 Ethical clearance
The ethical approval to commence the study was applied to the University of Fort Hare Research Ethics Committee (AREC) (JAJ041SMPO01/22/A). Consent to carry out the study was obtained from the relevant abattoirs.
2.2 Study site
The study was conducted from high through-put commercial abattoirs: EA1 abattoir and EA2 abattoir (Figure 1). The EA1 is at 32.97°S and 27.87°E in the Buffalo City Metropolitan Municipality. The EA2 is at 31.54°S and 26.53°E in the Enoch Mgijima municipality in the Chris Hani district of the Eastern Cape Province, South Africa. These abattoirs have long been at the forefront of the industry, upholding high animal welfare and livestock management standards. The records of slaughter for the previous months showed that each abattoir slaughtered at least 300 cattle daily. Approximately 480–850 mm of rainfall is received in the study area annually, mainly in the summer. The Eastern Cape is approximately 586–2371 m above sea level; this high altitude occasionally causes snow. The Eastern Cape Province has a cold semi-arid climate coupled with a temperate oceanic climate (Kottek et al., 2006). The ambient temperature ranges from 18°C to 39°C with a mean temperature of 20.5°C.
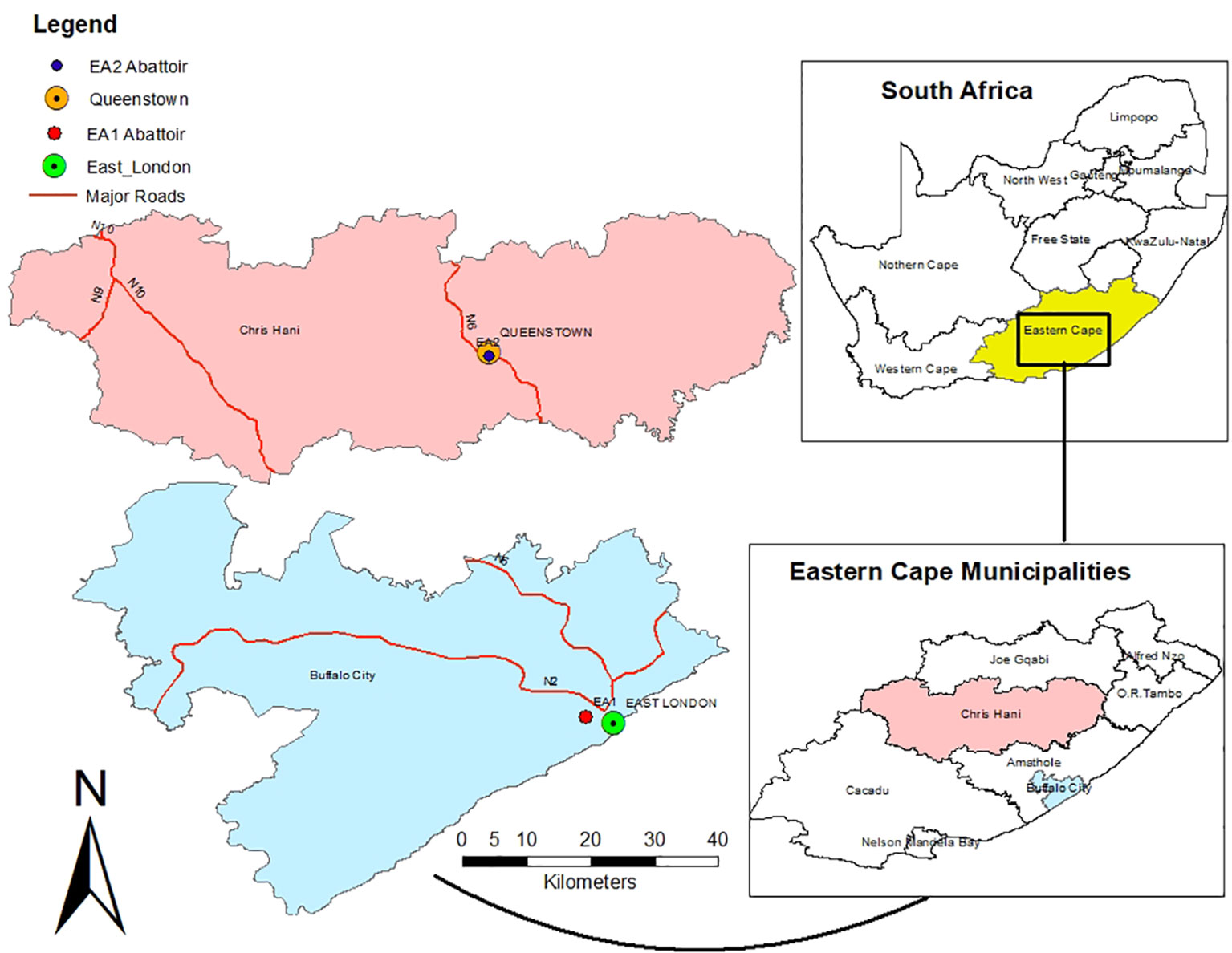
Figure 1 Map showing two abattoirs (EA1 and EA2) in Buffalo City and Chris Hani local municipalities.
The study area vegetation includes a variety of dominant plant species ranging from Acacia karroo, Themeda triandra, and Digitaria eriantha which forms part of grasslands, thicket and forest biomes. The abattoirs’ cattle came from communal and commercial farms all over the province. However, they were either from feedlot or non-feedlot farms. Feedlot animals are usually subjected to bought-in or home-grown feed and kept in pens or large paddocks. While non-feedlot animals mainly depend on grazing on veld or planted pastures, they remain on veld to achieve better growth rates with minimal or no supplementation.
2.3 Study design
An observational cross-sectional study was done in which, prior to slaughter, body condition scores were observed by trained personnel. The areas examined were tail, head, and loin (short ribs, pins, and hooks). A five-point scale description was used to determine the body condition scores, where 1 denoted very poor or emaciated, 2 denoted poor, 3 denoted good, 4 denoted fat, and 5 denoted excessively fat animals (Mpisana et al., 2022). However, it was re-arranged to fit into a 3-point scale of (1–2) thin, (3–4) adequate, and (5) fat (Jaja et al., 2017b). The recorded data on the type of production system, geographical origin, specific farms, sex, age, and weight gain was obtained from the abattoirs. Where such records did not exist, age was calculated through dentition and breed phenotypes were used to determine the breed type (Dupuy et al., 2013; Mpakama et al., 2014; Soji et al., 2015). Animals were grouped into two groups based on sex, breed, and age (young, denoting cattle less than 3 years, and old, meaning those 3 years and older) for easy statistical analysis.
2.4 Sampling procedures
Data were collected from beef cattle slaughtered at the two study abattoirs, encompassing feedlot and non-feedlot animals. Data was collected periodically, once a week, over 30 days. Data was collected from local mixed cattle breeds across the Eastern Cape Province. The sampling procedure employed was systematic random sampling. Sampling units were selected at equal intervals, with the first cattle randomly selected. Cattle were randomly selected from two abattoirs based on their origin, specifically whether they originated from a feedlot or a non-feedlot environment. When all the selected cattle were from feedlots, efforts were made to ensure representation from various farms within the feedlot area. The sample size was calculated by the power analysis method. The sample size was determined at a 5% level of significance and power of study at 80% (β=0.20) (Charan and Kantharia, 2013). With the standard deviation from previous studies, the sample size using power analysis for the experiment was 120 animals (Heckler et al., 2016; Zapa et al., 2021). The power analysis formula used was:
Where:
n=sample size
SD= standard deviation =1.56 (From previous studies).
= 1.96 (From Z table) at 5% level of significance
=0.842 (From Z table) at 80% power
d=effect size =0.8
Therefore
animals per group
Therefore, for two groups (feedlot and non-feedlot), a total of 120 animals was used.
2.5 Fecal sample collection
One hundred and twenty (120) fresh fecal samples were collected from the rectum of beef cattle at the EA1 (n=60) and EA2 (n=60) abattoirs. Of the 60 samples from each abattoir, there was an equal representation of feedlot (n=30) and non-feedlot cattle (n=30). The initial animal was selected randomly as the starting point. Then systematic random sampling was employed where the sampling interval was determined from the desired sample size (30) and the total number of animals to be slaughtered on the day. For instance, in EA2 the population of animals slaughtered on the first day of collection was 301 all from feedlot areas. Hence, the sampling interval was 10, and every 10th animal was selected in the population. The abattoirs separated the slaughter of the two groups of animals which made it easier to identify the starting and ending point of either feedlot or non-feedlot animals. The fecal samples were collected with new surgical gloves for each animal. The collected samples were immediately placed in baggies labeled with a unique animal identification number, place, and collection date and kept in a cooler box with ice packs. The fecal samples were further sent to the veterinary laboratory to analyze the number of eggs per gram (EPG) by sedimentation method using the McMaster technique (Paras et al., 2018). The fecal samples collected from the EA1 abattoir were sent to the Grahamstown veterinary laboratory, and the ones collected from EA2 were sent to the Queenstown veterinary laboratory for analysis. As soon as the fecal samples arrived at the laboratory, they were stored in a refrigerator at 4°C and processed within 3 days.
2.6 Fecal egg count analysis
Before the analysis, the fecal samples were scored for consistency in phases of concentrated (0.5), pellets (1.0), softly formed (1.5), soft doughy (2.0), soft, thin porridge (2.5), and diarrhea (3.0-3.5). The eggs per gram count (EPG) was then done to determine the internal parasite burden from fecal samples. The internal parasites that were examined included long-necked bankrupt worm (Nematodiruss spp), white bankrupt worm (Strongyloides spp), other roundworms, coccidian oocysts (Eimeria bovis), liver fluke (Fasciola spp) and conical fluke (Paramphistomum spp). Tapeworm, wireworm (Haemonchus spp), brown stomach worm (Ostertagia spp), nodular worm (Oesophagostomum spp), and bankrupt worm (Trichostrongylus spp) were also examined.
The number of eggs was determined by the sedimentation method, which uses a specific gravity that allows eggs to float on the slide. A sugar or salt solution with a specific gravity higher than the feces was mixed with the fecal material as it allowed the eggs to float to the slide’s top, making them easily visible. 4g of feces were weighed and added to 56 ml of sugar solution in a cylinder cup. The feces were broken with a tongue depressor to homogenize the mixture. After homogenizing the mixture, the solution was drained through the tea strainer to remove large pieces. Once the mixture was strained, the remaining solution was then stirred back and forth several times. A Pasteur pipette was used to withdraw a sub-sample of the filtrate and fill the first compartment of the McMaster counting chamber. The second chamber was also filled with another sub-sample, and the counting chamber was allowed to stand for five minutes to allow the eggs to float to the surface and the debris to go to the bottom of the chamber. The subsample of the filtrate was examined under a compound microscope at 10 x 10 magnification.
The eggs engraved within the area of both chambers were identified and counted with the help of a parasite guide to internal parasites of ruminants. The number of eggs seen in each chamber was recorded in a spreadsheet for each fecal sample. The number of eggs observed in both chambers was added, and eggs per gram (EPG) was calculated. The eggs per gram (EPG) were obtained by multiplying the egg counts by the constant factor 50. In each sample after the preparation for the sedimentation method, the remaining fecal material was weighed and prepared for centrifugation at 800-1000 rpm for 5 to 7 minutes. Some types of eggs (Fasciola) are heavier than others and may not float well in solutions of lower specific gravity. The intensity of infection was extrapolated using a severity index defined by the Royal Veterinary College London and the Food and Agriculture Organisation index of 2009 (Degefu et al., 2011). The intensity of mixed infections according to egg per gram (EPG) was subdivided into four, namely, 0 (no infection), 50-200 (light infection), 200-800 (moderate infection), and above 800 EPG (heavy infection) (Zapa et al., 2021). The EPG obtained can be used to determine the necessity of deworming individual animals and give the abattoir’s infection level. EPG is an effective management practice that, if utilized correctly, can create a healthier herd.
2.7 Rumen content analysis
The animals sampled for feces were marked and identified at slaughter for evisceration to collect rumen content. Rumen samples collected were placed in labeled baggies denoting each animal. The rumen content samples were analyzed at the University of Fort Hare laboratory using the Diode Array Near Infrared (NIR) Analysis System (DA 7250 GP) (PerkinElmer Inc, USA). The rumen content collected from the abattoirs was analyzed for protein, starch, acid detergent fiber (ADF), neutral detergent fiber (NDF), acid detergent lignin (ADL), moisture, phosphorus, and calcium levels on the same collection day. The sample was placed in 2 small rotating sample dishes on the NIR instrument, one after the other. The results produced by the instrument showed the average of both samples for a more accurate estimate of the composition. The results were then exported into a Microsoft Excel file, and the same analysis procedure was repeated for each rumen sample. The analysis of rumen content was to provide insights into the nutritional status of the cattle. By examining the composition of the rumen contents, such as different feed components, fibrous materials, we could assess the type and quality of the diet between the feedlot and non-feedlot cattle. This also gives insights into the extent which cattle can obtain essential nutrients from their feed as these may affect the weight gain and body condition scores in different feedlot settings.
2.8 Statistical analysis
All the data was analyzed using the John’s Macintosh Project (JMP) version 17 procedure of the Statistical Analysis System (SAS). Before the analysis, normality and variance homogeneity were tested using histograms and probability plots. Body condition score values were square-rooted to confer them to follow the normal distribution. Fecal egg counts per gram (EPG) data were transformed using to stabilize variances between groups before statistical analysis (Dobson et al., 2009; Sweeny et al., 2011). Prevalence of each specific parasite in a mixed infection was calculated as: .
All data was subjected to analysis of variance (ANOVA), where comparisons of the means of continuous variables (weight, fecal egg count, body condition score) between the production system (feedlot vs. non-feedlot) and abattoirs (EA1 and EA2) were made. For all the analyses, treatment means were separated using the Tukey-Kramer HSD significance difference method. A level of P< 0.05 was set as the criterion for statistical significance. Farm, production system (feedlot and non-feedlot), and rumen feed content composition effects were declared significant at the 5% level of probability (P< 0.05). The effect of feedlot condition on weight, body condition score, and fecal egg count was analyzed using the following model:
Where
Response variable (weight, body condition score, fecal egg count)
Overall mean
– Effect of the feedlot condition (feedlot & non-feedlot), age, sex, breed
Random error term
The Chi-Square Test assessed the association between categorical variables (age, gender, breed, body condition score) and the feedlot status. The statistical association between parasite burden (fecal egg count per gram) and production system (feedlot and non-feedlot) was evaluated separately for each abattoir (EA1 and EA2). Pearson correlation analysis was also performed to explore potential associations between weight, fecal egg count, body condition score, and rumen feed content composition.
3 Results
Based on the results, the frequencies of nematode genera identified in feedlot cattle were consistent, with Ostertagia, Haemonchus, and Trichostrongylus each comprising 35% of the total. Trichuris and Paramphistomum accounted for 16.7% and 15.8%, respectively (Table 1). In contrast, among non-feedlot cattle, Ostertagia, Haemonchus, and Trichostrongylus also constituted 42.5% each, with Trichuris and Paramphistomum making up 17.5% and 18.3%, respectively. These differences exhibited statistically significant variations (P< 0.05) between the feedlot and non-feedlot cattle groups. However, it is noteworthy that most parasites examined were absent (0%) in feedlot and non-feedlot cattle groups. These included Nematodirus, Strongyloides, Fasciola, and Eimeira bovis. Notably, the absence of these parasites did not yield statistically significant differences (P > 0.05) between the two production systems.
In Table 2, the fecal egg counts per gram (EPG) observed in abattoir EA1 were notably higher in non-feedlot cattle (EPG=323) in comparison to feedlot cattle (EPG= 73). This difference in EPG also yielded statistical significance (P< 0.05), signifying a meaningful variance in the mean EPG values between the two groups. It is worth mentioning that feedlot cattle demonstrated higher live weights, with an average of 298.1 4.7 kg, in contrast to non-feedlot cattle, which had an average live weight of 287.16 7.79 kg. However, this disparity in live weight did not reach statistical significance (P > 0.05) when comparing the two production systems (feedlot and non-feedlot). Furthermore, an important observation was made regarding body condition scores in the EA1 abattoir. In this context, feedlot cattle exhibited significantly higher body condition scores than their non-feedlot counterparts (P< 0.05). Conversely, in the EA2 abattoir, no significant differences were found in EPG, live weight, or body condition scores when comparing the two production systems. The analysis indicated that non-feedlot cattle were more susceptible to parasitic infections, with a notable portion experiencing high infections (EPG 800+), as depicted in Figure 2. However, when it came to body condition scores, a more significant percentage of non-feedlot cattle demonstrated moderate scores (35%) compared to feedlot cattle (33%). Good body condition scores were primarily prevalent among feedlot cattle (6.7%), while they were less common among non-feedlot cattle (1.7%). Table 3 shows analyses of rumen feed content composition in cattle from both feedlot and non-feedlot production systems. In the EA1 abattoir, several nutritional components, including Protein (21.1% vs. 23.4%), Fiber (8.9% vs. 8.7%), Ash (9.5% vs. 8.3%), Calcium (6.1% vs. 5.7%), and ADF (17.5% vs. 15.2%), exhibited statistically significant differences (P< 0.05) between the feedlot and non-feedlot cattle, respectively. However, in the EA2 abattoir, there were no significant variations (P > 0.05) in rumen feed content composition values between the feedlot and non-feedlot cattle.
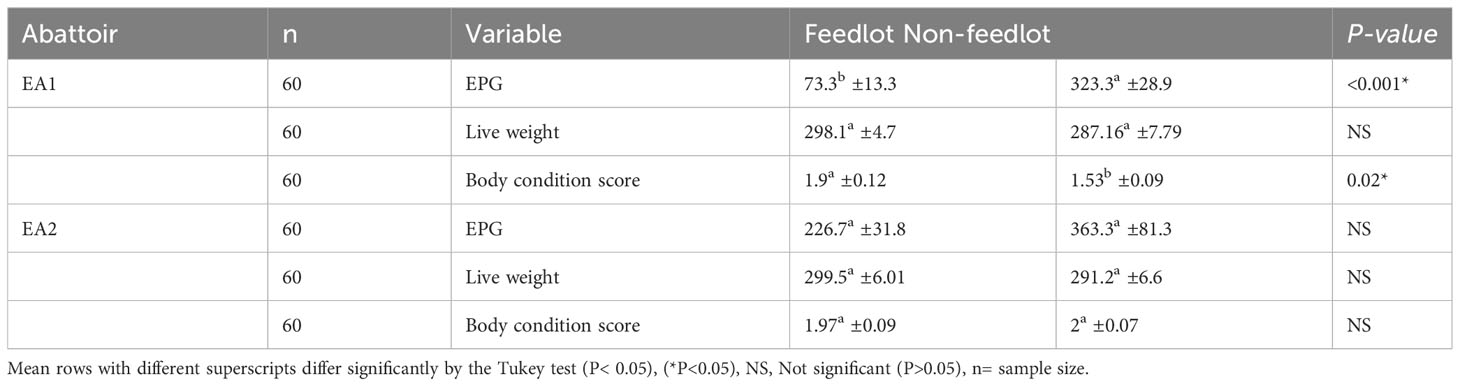
Table 2 Impact of production system on fecal egg per gram (EPG), live weight, and body condition scores.
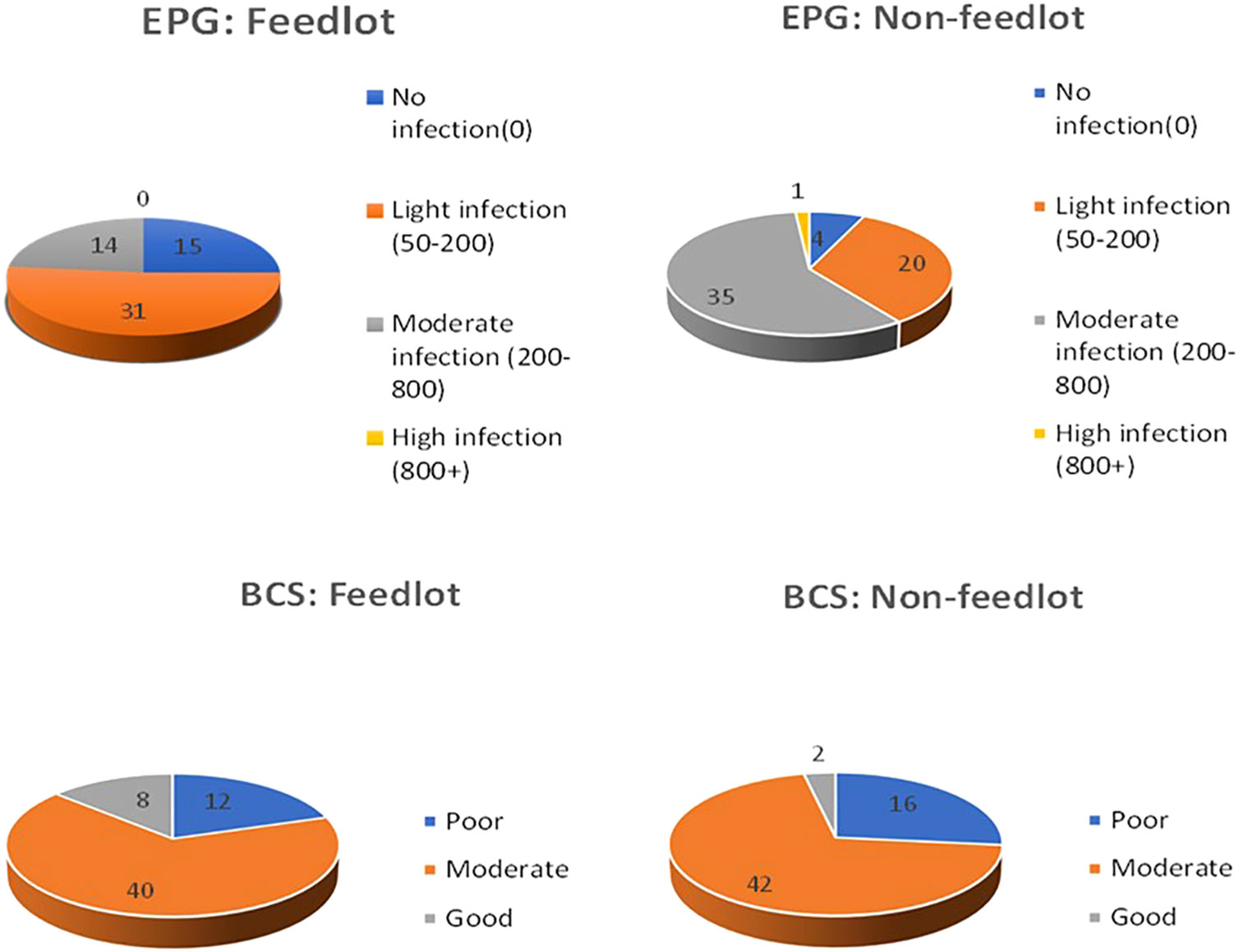
Figure 2 Comparative distribution of fecal egg count (EPG) and body condition score (BCS) in feedlot and non-feedlot cattle.
Table 4 explores the influence of different farms (F) on fecal egg counts, live weight, and body condition scores of the feedlot and non-cattle. In the EA1 abattoir, fecal egg counts per gram (EPG) displayed significant differences (P< 0.05) between farms, with the highest EPG recorded in non-feedlot cattle (F1: 342.3; F2: 306.3) and the least in feedlot cattle (F3: 53.6; F4: 90.6). Body condition scores also exhibited significant variability between farms, with the highest mean scores observed in feedlot cattle (F4: 2.13) and the lowest in (F1: 1.36). However, there were no significant differences (P > 0.05) in live weight between the farms in this abattoir. Similarly, in EA2, there were significant differences (P< 0.05) in body condition scores between farms. However, no significant variations were observed between farms concerning fecal egg counts (EPG) and live weight of feedlot and non-feedlot cattle.
Table 5 explores the relationship between various risk factors, including age, breed, and sex, in conjunction with body condition scores and parasite burden (degree of infection) among feedlot and non-feedlot cattle, with each group analyzed separately in their respective abattoirs. In EA1 Abattoir, a significant association (P< 0.05) was identified between sex and the degree of infection in feedlot cattle. Notably, more females (43.3%) exhibited moderate infections by mixed parasites compared to males (0%). Conversely, similar trends were observed among non-feedlot cattle, with more females showing moderate infections than males. No differences were observed among infections in non-feedlot cattle. Across both abattoirs, age, breed, body condition score, and degree of infection did not exhibit significant differences between feedlot and non-feedlot cattle.
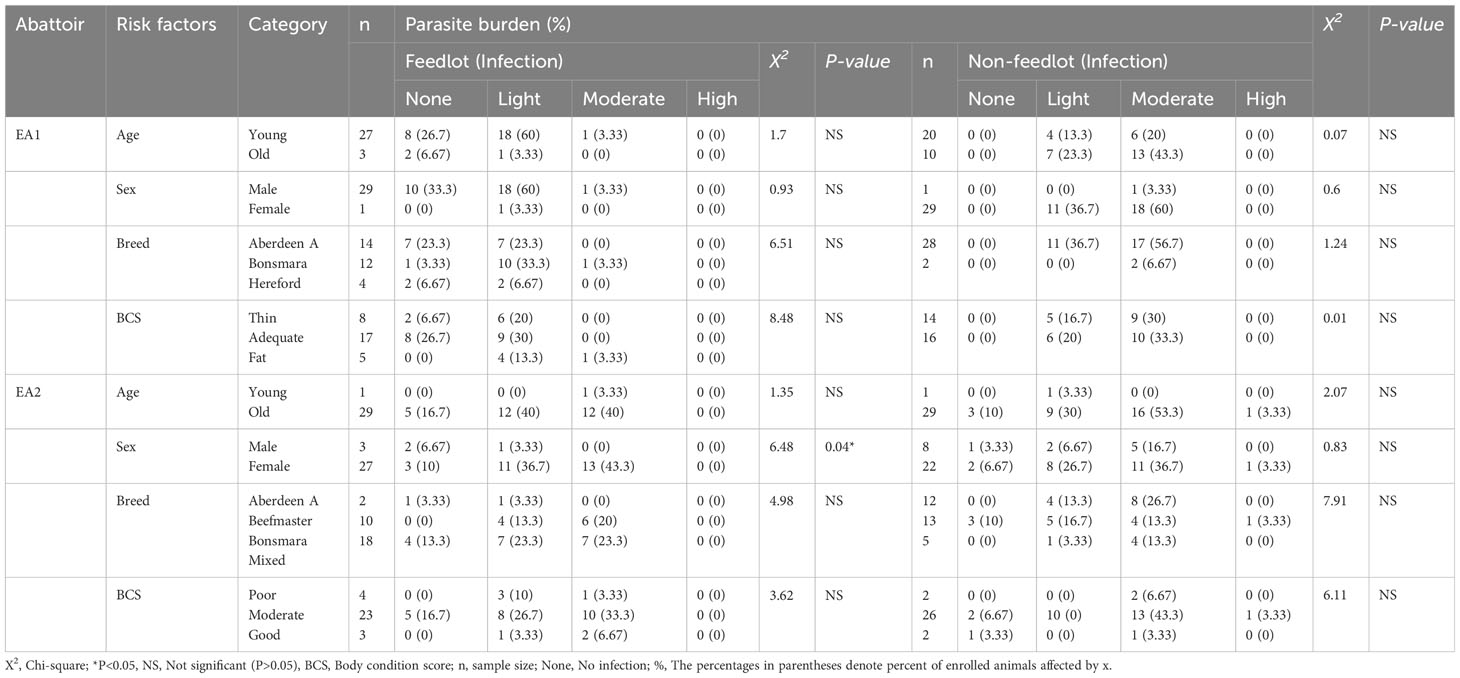
Table 5 Association between various risk factors, body condition score, and parasite burden of feedlot and non-feedlot cattle.
Table 6 reports Pearson correlation coefficients to identify associations among fecal egg counts (EPG), live weight, body condition scores, and the composition of rumen feed content in feedlot cattle. Significant negative correlations (P<0.05) were identified between EPG and live weight (r=-0.02), fiber (r=-0.35), ADL (r=-0.254), and starch (r=-0.414), hinting at a subtle relationship between parasite load and feed nutrient composition. In contrast, a positive correlation was found between EPG and Ash (r = 0.32), implying potential interactions between ash content and parasite presence. Moreover, positive correlations were observed between body condition score and calcium (r = 0.27), highlighting the potential influence of calcium content on cattle body condition. Notably, no significant correlations (P> 0.05) were identified between EPG, body condition score, and weight gain in feedlot cattle, underlining the complexity of these interactions.
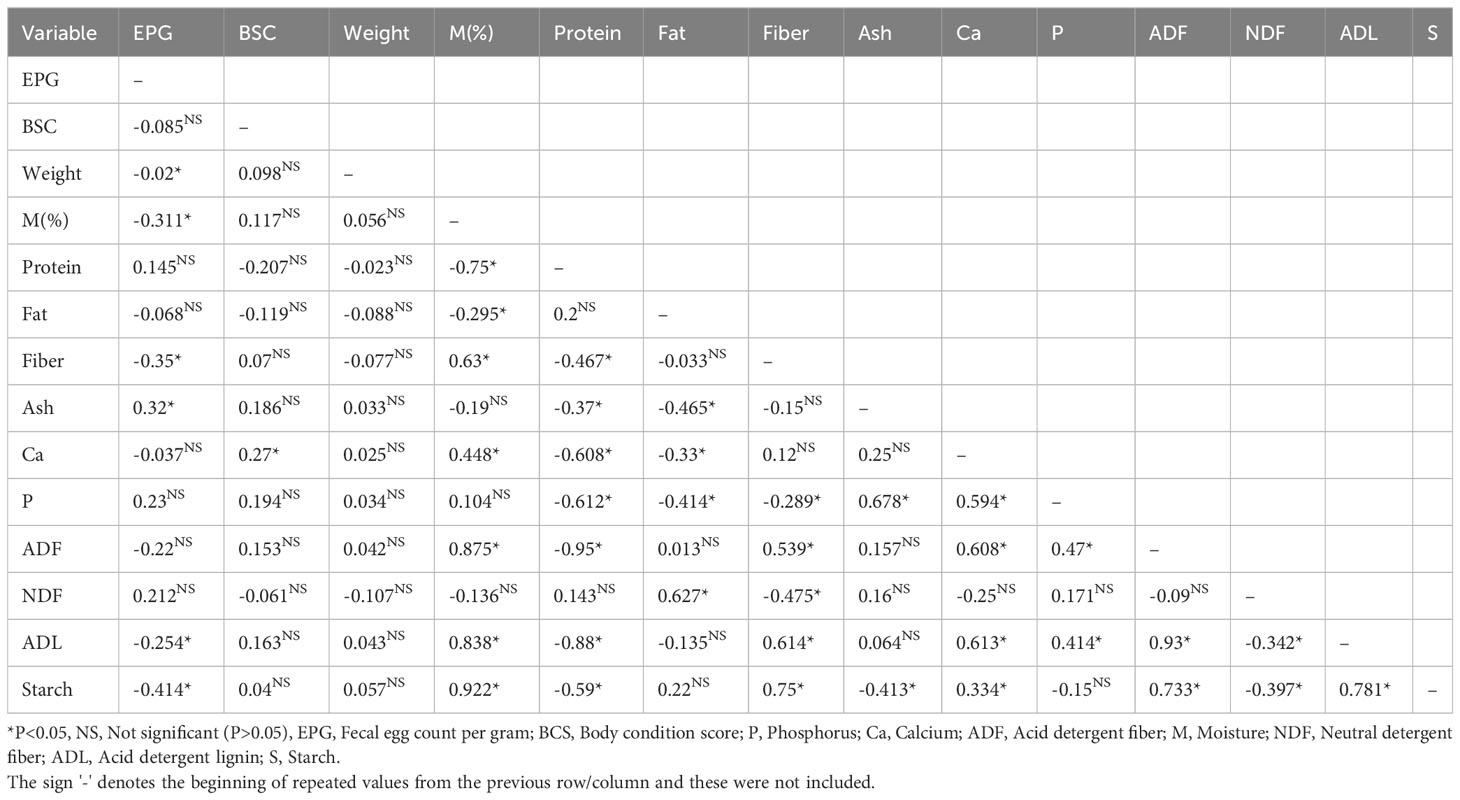
Table 6 Pearson correlation between fecal egg count, live weight, body condition score, and rumen feed content composition in feedlot cattle.
Conversely, significant negative correlations emerged between EPG and body condition score (r=-0.166) and ash (r=-0.34) in non-feedlot cattle (Table 7). Similarly, significant correlations were identified between body condition scores and moisture content (r=-0.257), ash (r=-0.347), and starch (r=-0.368). Body condition score was positively correlated with phosphorus (r=0.326). However, no significant correlations (P > 0.05) were observed between EPG, live weight, and rumen feed content composition.
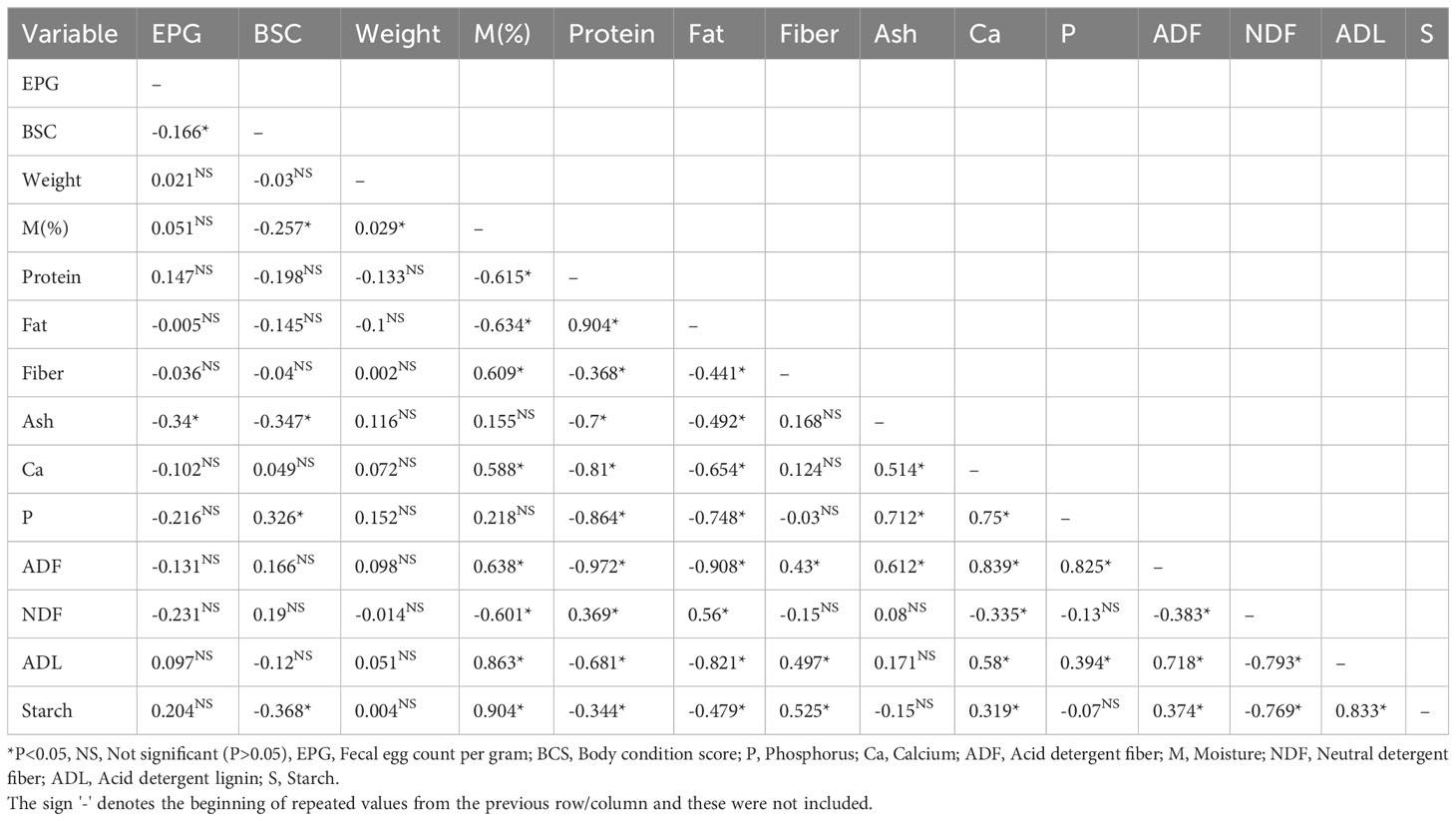
Table 7 Pearson correlation between fecal egg count, live weight, body condition score, and rumen feed content composition in non-feedlot cattle.
4 Discussion
Abattoirs are the ultimate destination for food animals, marking a pivotal juncture in the meat production chain. Abattoir offers invaluable insights into livestock health and the epidemiology of animal diseases (Dupuy et al., 2013; Jaja et al., 2017b). As animals undergo processing within these facilities, they become representative snapshots of the broader livestock population within the Eastern Cape Province. This representation holds particular importance due to its potential to provide a comprehensive cross-section of livestock diversity. Abattoirs, in this sense, act as reservoirs of valuable information that can shed light on animal diseases and their prevalence.
Identifying nematode genera in both feedlot and non-feedlot cattle provides valuable insights into the parasitic landscape within these distinct production systems. The results revealed a diverse range of nematode genera, each potentially playing a role in the health and overall well-being of the animals. Ostertagia, Haemonchus, and Trichostrongylus emerged as the predominant nematode genera in feedlot and non-feedlot cattle. Similarly, studies found Haemonchus and Trichostrongylus to be the most dominant genus (Heckler et al., 2016; Waruiru, 2004). However, the findings also contradict that of most researchers, where Cooperia spp were found to be the most prevalent genera in cattle, followed by Haemonchus, Trichostrongylus, and Oesophagostomum (Zapa et al., 2021; Trindade et al., 2023). The dominant genera in this study may be due to gastrointestinal nematode adaptability and prevalence across diverse feedlot and non-feedlot systems. The significant proportions of these genera between the two production systems suggest that factors beyond mere presence influence their prevalence, potentially relating to the animals’ environment, climate change, feeding practices, and other management aspects. These nematodes are known to impact the gastrointestinal health of cattle, potentially leading to reduced feed efficiency, weight gain, and overall productivity (Charlier et al., 2009; Rashid et al., 2019).
The findings of this study offer valuable insights into the interplay between fecal egg counts (EPG), live weights, and body condition scores in feedlot and non-feedlot cattle within the context of two distinct abattoirs, EA1 and EA2. In abattoir EA1, significantly higher EP observed in non-feedlot cattle than feedlot cattle underscores a distinct difference in parasitic burden between these two groups. These findings align with the research that pasture-based cattle have higher fecal egg counts than feedlot cattle (Merlin et al., 2017; Zapa et al., 2021; Maples et al., 2022). Thus, the current study shows that non-feedlot cattle are more prone to parasitic infections. This discrepancy aligns with the notion that they might be more exposed to parasitic infections due to their grazing habits and environmental exposure.
Furthermore, parasite burdens are associated with changes in cattle behavior, including time spent walking, lying, and feeding that indicate discomfort, with welfare implications, even in subclinically affected cattle (Högberg et al., 2021). This observation highlights the potential impact of management practices on parasitic burden and productivity. The higher EPG values in non-feedlot cattle may be because these abattoirs also receive cattle supply from communal cattle farmers. The communal cattle suppliers may not have adequate medicine and proper disease control infrastructure. Therefore, their animals may be susceptible to disease due to the high costs, absence, or inappropriateness of the available animal health and production inputs (Musemwa et al., 2010; Soji et al., 2015). In the EA2 abattoir, the statistically insignificant differences in EPG values between the feedlot and non-feedlot cattle may be due to anthelmintic resistance, sampling design, and animal origin. Undetected animals carrying anthelmintic-resistant worms entering the feedlot increase the parasite burden and could cause significant productivity losses (Fazzio et al., 2014; Gałązka et al., 2023).
The finding that feedlot cattle exhibited higher live weights than non-feedlot cattle in EA1 aligns with expectations, given the feedlot systems’ optimized feeding practices and controlled environments. These findings align with the research that found that the average weight gain of cattle kept in pastures decreased with the increase in parasite burden (Heckler et al., 2016; Mertz et al., 2005; Jackson, 2013). The reduced live weights in non-feedlot cattle may be due to parasitism affecting productivity, impairing weight gain, reproduction, lactation, and feed use efficiency. However, the lack of significant difference between production systems regarding live weight suggests that while feedlot cattle might be consistently heavier, this difference might not be statistically significant in these abattoirs. This may be because the heavy rainfall experienced in 2023 may have closed the gap in live weight gain between feedlot and non-feedlot animals, as there was sufficient natural pasture in communally raised cattle (Mdoda et al., 2023).
The significantly higher body condition scores in feedlot cattle than non-feedlot cattle in EA1 indicate that the nutritional status of the animals does vary between the two systems. This finding aligns with most research in which body condition scores in feedlot cattle are considerably higher and better (Bohnert et al., 2013; Madziga et al., 2013; Mulliniks et al., 2016). This suggests that the feeding practices and dietary management in feedlot and non-feedlot settings influence the overall body condition of the cattle. Good body condition scores more frequently in feedlot cattle further accentuate the potential advantages of managed feeding practices in supporting optimal body conditions. Animals in good intensive management systems and adequate veterinary care are expected to be in better body condition than cattle extensively managed with little veterinary input. Interestingly, in abattoir EA2, the results showed no significant differences between EPG, live weight, and body condition scores for feedlot and non-feedlot cattle. Factors beyond the immediate production systems might have played a more substantial role in shaping the health and characteristics of the animals in this abattoir. This underscores the influence of location-specific conditions and practices on cattle health.
Rumen content was analyzed to determine if the feed composition significantly influenced this study’s feedlot and non-feedlot results. In the case of the EA1 abattoir, several nutritional components exhibited statistically significant differences between feedlot and non-feedlot cattle. Feedlot cattle had a lower protein content in their rumen feed than non-feedlot cattle. While the difference in fiber content between the two groups was slight, it was statistically significant primarily due to variations in the types or amounts of fiber-rich feed components included in the feedlot and non-feedlot cattle diets. The higher ash content in the rumen feed of feedlot cattle implies a more significant presence of minerals. This could result from the specific mineral supplements or feed additives used in feedlots to meet the nutritional requirements of cattle in intensive production systems (Cornelius Jacobus Lindeque du Toit, 2017).
Feedlot cattle exhibited a significantly higher ADF content. ADF represents the portion of plant material that is less digestible and consists of cellulose, lignin, and other complex compounds. The higher ADF in feedlot cattle’s rumen feed may indicate that their diet contains more fibrous or mature plant materials. The differences in rumen feed content composition between feedlot and non-feedlot cattle may be related to dietary formulations, as feedlot cattle are specifically fed highly nutritious feeds for improved animal health that enhance heavier weights (Nyhodo et al., 2014; Sifiso et al., 2013). In contrast, in the EA2 abattoir, no significant variations were observed in rumen feed content composition values between feedlot and non-feedlot cattle. Therefore, further research may be needed to elucidate the specific dietary and management practices that lead to these differences in rumen feed content composition and to assess their implications for cattle health and production performance.
This study delved into the interplay between farm origin and fecal egg count (EPG), live weight, and body condition scores from EA1 and EA2 abattoirs. The significant differences in EPG and body condition scores between farms provide insights into the relationship between farm practices and cattle health. The observed variations suggest that farm practices positively influence cattle health and well-being. The differential parasitic burdens and body condition scores indicate that targeted management practices, including parasite control and nutritional strategies, are pivotal in shaping animal health within specific farm settings (Zapa et al., 2021). Non-feedlot farms had cattle with significantly higher EPG values compared to the feedlot farms. Climatic conditions, grazing patterns, deworming strategies, feed composition, and animal husbandry practices between farms may drive these differences. Hence, future investigations could delve deeper into the specific management practices of each farm to unravel the underlying factors driving the observed differences. By identifying the specific practices contributing to varying health outcomes, stakeholders can work toward more targeted interventions that optimize cattle health and performance across different farm contexts.
Fecal egg counts are a rapid method of evaluating animal parasite burdens (Nielsen, 2022). Exploring the association between various risk factors, body condition score, and parasite burden in feedlot and non-feedlot cattle within EA1 and EA2 abattoirs has unraveled intriguing insights. The analysis in EA1 abattoir unveiled significant associations between sex and degree of infection, specifically in feedlot cattle. The more moderate infection in females than males in feedlot cattle contradicts the findings of Osorio-Acre and Segura-Correa (2011), who found no significant difference in EPG counts between male and female cattle. The significant association between sex and parasite burden in EA1 feedlot cattle suggests that sex-specific management practices or physiological differences, such as lactation and pregnancy, might contribute to varying infection levels in females (Michael et al., 2019). However, further investigation is needed to discern the underlying factors.
Non-feedlot cattle in EA1 did not show a significantly similar trend, where most females showed moderate infection compared to males. The observation implies that while the impact of sex on infection might be present, other factors might played a role in the non-feedlot settings. In both abattoirs, the analysis revealed that age, breed, body condition score, and degree of infection were not significantly different between feedlot and non-feedlot cattle. This suggests that while specific trends within each setting exist, the overall differences in these factors might not be statistically meaningful in the context of these specific abattoirs (Sweeny et al., 2011).
The observation of a weak negative correlation between EPG and body condition score in non-feedlot cattle highlights a potential but delicate relationship between parasitic burden and the physical state of the animals. This suggests that as parasite load increases, there might be a subtle tendency for body condition to decrease. The results are similar to that of research where a significant negative correlation was found between body condition score and EPG (Larsson et al., 2006; Sweeny et al., 2011; Zapa et al., 2021). However, the non-significant correlation coefficient in feedlot cattle between EPG, live weight, and body condition scores in this study indicates that this association is weak and could be influenced by numerous other factors. Notably, the feedlot and non-feedlot EPG, live weight, and body condition score differences in this study might be affected by farm, geographical location, and cattle production systems.
A weak negative correlation between EPG and live weight in feedlot cattle implies that there might be a subtle link between parasite load and weight gain, suggesting that higher EPG values could be linked to marginally lower weight gains. The findings contradict many researchers in which significantly strong negative correlations were found between EPG and weight gain in feedlot cattle (Vercruysse and Claerebout, 2001; Burggraaf et al., 2007; Merlin et al., 2017; Zapa et al., 2021; Shephard et al., 2022). The observation in this study aligns with the notion that parasitic infections can affect nutrient absorption and utilization, potentially impacting overall growth. Significant correlations were detected between EPG, body condition score, and various rumen feed elements. Negative correlations between body condition score and ash content suggest that an increase in body condition score is linked to a decrease in ash content, and a weakly positive correlation identified between body condition scores and starch hints at the role of carbohydrates in body condition scores.
Current livestock farming is very dynamic, and improving the genetics of animals using reproductive tools (artificial insemination, embryo transfers) may significantly increase the herd’s productivity. This, in turn, may trigger a different parasite x host interaction, which may produce different results from those obtained in the present study. The present work brings information regarding the association between EPG, body condition score, and weight gain in feedlot and non-feedlot cattle, which are important and deserve attention for science, as previously discussed.
The findings of this study emphasize the need for tailored management practices in feedlot and non-feedlot cattle systems. Effective parasite control measures, regular monitoring of EPG, and strategies to improve nutritional status are essential for maximizing growth and maintaining optimal body condition. Future research endeavors could focus on elucidating how parasitic infections impact weight gain and body condition in feedlot cattle and exploring the multifactorial determinants of these correlations in non-feedlot cattle. Furthermore, understanding the interactions between parasitic burden, nutrition, genetics, and environmental factors will inform evidence-based management approaches that promote animal health, welfare, and sustainable livestock production. Continued research in this area has the potential to contribute to the development of targeted interventions, improved grazing management, and optimized feedlot practices, ultimately benefiting both cattle producers and the broader agricultural industry.
Effective control of gastrointestinal parasite burden in feedlot systems provides cattle with growth benefits in the production system. Identifying sustainable and cost-effective parasite controls remains essential for managing and producing cattle globally. This study provides evidence to justify investment in interventions for feedlot and non-feedlot cattle to minimize exposure to the larvae of, as well as treat excessive burdens of, gastrointestinal nematodes and improve both productivity and welfare. It further recommends feedlots as the most desirable production system for reducing cattle’s gastrointestinal parasite burden and improving its health and nutritional status. The study’s findings may be limited to high-throughput abattoirs, and despite this limitation, this study contributes valuable insights to the existing body of knowledge and different throughput levels will be considered for future research endeavors in this field. Moreover, future research may consider extending the study duration and including the impact of seasons as these may provide more comprehensive understanding of how seasonal changes affect cattle health and performance.
5 Conclusion
Non-feedlot cattle exhibited higher EPG values than feedlot cattle, indicating a potentially greater susceptibility to internal parasitic infections. These results underscore the importance of effective parasite management strategies in feedlot operations to mitigate the impact of parasitic infestations on animal health and productivity. Notable differences in body condition scores between feedlot and non-feedlot cattle could be attributed to these systems’ specific nutritional regimens and management practices. The insights gained from this research contribute to developing effective strategies for enhancing cattle health and productivity, ultimately impacting the meat industry’s sustainability and animal welfare. However, further investigations into the underlying factors driving associations between EPG and weight gain in feedlot and non-feedlot cattle. Moreover, these investigations are warranted to guide informed decision-making in livestock management and abattoir operations.
Data availability statement
The original contributions presented in the study are included in the article/supplementary material. Further inquiries can be directed to the corresponding authors.
Ethics statement
The animal study was approved by University of Fort Hare Animal Research Ethics Committee (AREC). The study was conducted in accordance with the local legislation and institutional requiremements.
Author contributions
BM: Conceptualization, Data curation, Methodology, Writing – original draft, Writing – review & editing. MS: Conceptualization, Supervision, Writing – review & editing. GM: Formal analysis, Investigation, Writing – review & editing. SM: Formal analysis, Investigation, Writing – review & editing. IJ: Conceptualization, Supervision, Writing – review & editing.
Funding
The author(s) declare financial support was received for the research, authorship, and/or publication of this article. We are grateful to The National Research Foundation (NRF-PMDS22061422419) for financial assistance and the participating abattoirs for their consent to use their facilities.
Acknowledgments
We are grateful to GM and SM of the Grahamstown Veterinary Laboratory and Mr. Patrick Molefe of the Queenstown Veterinary Laboratory for their technical assistance in the analysis.
Conflict of interest
The authors declare that the research was conducted in the absence of any commercial or financial relationships that could be construed as a potential conflict of interest.
Publisher’s note
All claims expressed in this article are solely those of the authors and do not necessarily represent those of their affiliated organizations, or those of the publisher, the editors and the reviewers. Any product that may be evaluated in this article, or claim that may be made by its manufacturer, is not guaranteed or endorsed by the publisher.
References
Bohnert D., Stalker L., Mills R. R., Nyman A., Falck S. J., Cooke R. F. (2013). Late gestation supplementation of beef cows differing in body condition score: Effects on cow and calf performance. J. Anim. Sci. 91 (11), 1–7. doi: 10.2527/jas.2013-6301
Burggraaf V. T., Brooky A. R., Boom C. J. (2007). “Response of beef calves to different levels of postweaning ingestion of gastrointestinal parasite larvae,”. Aust. J. Exp. Agric. 47 (11), 1297–1303. doi: 10.1071/EA06275
Charan J., Kantharia N. (2013). “How to calculate sample size in animal studies?,”. J. Pharmacol. Pharmacotherapeutics 4 (4), 303–306. doi: 10.4103/0976-500X.119726
Charlier J., Höglund J., von Samson-Himmelstjerna G., Dorny P., Vercruysse J. (2009). “Gastrointestinal nematode infections in adult dairy cattle: Impact on production, diagnosis and control,”. Vet. Parasitol. 164 (1), 70–79. doi: 10.1016/j.vetpar.2009.04.012
Cornelius Jacobus Lindeque du Toit B. (2017). Mitigation of enteric methane emissions from ruminants in sub-tropical production systems (Pretoria, South Africa: University of Pretoria).
Degefu H., Abera C., Yohannes M., Tolosa T. (2011). Gastrointestinal helminth infections in small-scale dairy cattle farms of Jimma town, Ethiopia. Ethiop. J. Appl. Sci. Technol. 2 (1), 31–37.
Dobson R. J., Sangster N. C., Besier R. B., Woodgate R. G. (2009). “Geometric means provide a biased efficacy result when conducting a faecal egg count reduction test (FECRT),”. Vet. Parasitol. 161 (1–2), 162–167. doi: 10.1016/j.vetpar.2008.12.007
Dupuy C., Morignat E., Maugey X., Vinard J., Hendrikx P., Ducrot C., et al. (2013). “Defining syndromes using cattle meat inspection data for syndromic surveillance purposes: A statistical approach with the 2005-2010 data from ten French slaughterhouses,”. BMC Vet. Res. 9, 1–7. doi: 10.1186/1746-6148-9-88
Fazzio L. E., Sánchez R. O., Streitenberger N., Galvan W. R., Giudici C. J., Gimeno E. J. (2014). “The effect of anthelmintic resistance on the productivity in feedlot cattle,”. Vet. Parasitol. 206 (3–4), 240–245. doi: 10.1016/j.vetpar.2014.10.010
Gałązka M., Klich D., Filip-Hutsch K., Olech W., Anusz K., Pyziel A. M. (2023). “Endoparasite loads and the efficacy of conventional anthelmintics against gastrointestinal nematodes in captive European bison,”. Int. J. Parasitol. Parasites Wildl 21, 224–231. doi: 10.1016/j.ijppaw.2023.06.005
Heckler R., Borges D., Vieira M., Conde M., Green M., Amorim M., et al. (2016). “New approach for the strategic control of gastrointestinal nematodes in grazed beef cattle during the growing phase in central Brazil,”. Vet. Parasitol. 221, 123–129. doi: 10.1016/j.vetpar.2016.03.010
Högberg N., Hessle A., Lidfors L., Baltrušis P., Claerebout E., Höglund J. (2021). “Subclinical nematode parasitism affects activity and rumination patterns in first-season grazing cattle,”. Animal 15 (6), 1–8. doi: 10.1016/j.animal.2021.100237
Jackson A. (2013). Parasitic gastroenteritis in calves during their first season at grass: The potential for a performance-based targeted selective anthelmintic treatment programme. (United Kingdom: University of Glasgow, Scottish Centre for Production Animal Health and Food Safety).
Jaja I. F., Mushonga B., Green E., Muchenje V. (2017a). “Financial loss estimation of bovine fasciolosis in slaughtered cattle in South Africa,”. Parasite Epidemiol. Control 2 (4), 27–34. doi: 10.1016/J.PAREPI.2017.10.001
Jaja I. F., Mushonga B., Green E., Muchenje V. (2017b). “Seasonal prevalence, body condition score and risk factors of bovine fasciolosis in South Africa,”. Vet. Anim. Sci. 4, 1–7. doi: 10.1016/j.vas.2017.06.001
Kottek M., Grieser J., Beck C., Rudolf B., Rubel F. (2006). “World map of the Köppen-Geiger climate classification updated,”. Meteorologische Z. 15 (3), 259–263. doi: 10.1127/0941-2948/2006/0130
Larsson A., Dimander S. O., Rydzik A., Uggla A., Waller P. J., Höglund J. (2006). “A 3-year field evaluation of pasture rotation and supplementary feeding to control parasite infection in first-season grazing cattle-Effects on animal performance,”. Vet. Parasitol. 142 (3–4), 197–206. doi: 10.1016/j.vetpar.2006.07.017
Madziga I., Alawa C., Lamidi O. S., Goska D. Y., Adesote A. A. (2013). “Feedlot assessment of four indigenous breeds of cattle in Nigeria,”. Int. J. Of Life Sci. Med. Res. 3 (1), 35–38. doi: 10.5963/LSMR0301006
Maples W. E., Brorsen B. W., Peel D., Hicks B. (2022). Observational study of the effect of metaphylaxis treatment on feedlot cattle productivity and health. Front. Vet. Sci. 9, 1–10. doi: 10.3389/fvets.2022.947585
Mdoda L., Christian M., Agbugba I. (2023). “Use of Information systems (Mobile phone app) for enhancing smallholder farmers’ Productivity in Eastern Cape Province, South Africa: implications on food security,”. J. Knowledge Economy 14, 1–17. doi: 10.1007/s13132-023-01212-0
Merlin A., Chauvin A., Lehebel A., Brisseau N., Froger S., Bareille N., et al. (2017). “End-season daily weight gains as rationale for targeted selective treatment against gastrointestinal nematodes in highly exposed first-grazing season cattle,”. Prev. Vet. Med. 138, 104–112. doi: 10.1016/j.prevetmed.2017.01.011
Mertz K. J., Hildreth M. B., Epperson W. B. (2005). “Assessment of the effect of gastrointestinal nematode infestation on weight gain in grazing beef cattle,”. J. Am. Vet. Med. Assoc. 226 (5), 779–783. doi: 10.2460/javma.2005.226.779
Michael J., Baruselli P., Campanile G. (2019). Influence of nutrition, body condition, and metabolic status on reproduction in female beef cattle: A review. Theriogenology Journal (Elsevier), 125. doi: 10.1016/j.theriogenology.2018.11.010
Mpakama T., Chulayo A. Y., Muchenje V. (2014). “Bruising in slaughter cattle and its relationship with creatine kinase levels and beef quality as affected by animal related factors,”. Asian-Australas J. Anim. Sci. 27 (5), 717–725. doi: 10.5713/ajas.2013.13483
Mpisana Z., Jaja I. F., Byaruhanga C., Marufu M. C. (2022). “Body condition scores, fluke intensity, liver pathology, and carcass quality of different dairy cattle genotypes infected with Fasciola species at high throughput abattoirs in South Africa,”. Parasitol. Res. 121 (6), 1671–1682. doi: 10.1007/S00436-022-07504-9/FIGURES/3
Mulliniks J. T., Sawyer J., Harrelson F., Mathis C., Cox S., Loest C., et al. (2016). “Effect of late gestation bodyweight change and condition score on progeny feedlot performance,”. Anim. Prod Sci. 56 (12), 1998–2003. doi: 10.1071/AN15025
Musemwa L., Mushunje A., Chimonyo M., Mapiye C. (2010). Low cattle market off-take rates in communal production systems of South Africa: Causes and mitigation strategies. J. Sustain. Dev. Afr. 12 (5), 209–226.
Nielsen M. K. (2022). “Parasite faecal egg counts in equine veterinary practice,”. Equine Veterinary Educ. 34 (11), 584–591. doi: 10.1111/eve.13548
Nyhodo B., Mmbengwa V. M., Balarane A., Ngetu X. (2014). “Formulating the least cost feeding strategy of a custom feeding programme: A linear programming.,”. Int. J. Sustain. Dev. 7, 85–92.
Paras K. L., George M. M., Vidyashankar A. N., Kaplan R. M. (2018). “Comparison of fecal egg counting methods in four livestock species,”. Vet. Parasitol. 257, 21–27. doi: 10.1016/j.vetpar.2018.05.015
Rabelo T., Welber S., Zanetti Lopes D., Buzulini C., Fernando De Almeida Borges I., Claudio I., et al. (2010). Helminth fauna of bovines from the Central-Western region, Minas Gerais State, Brazil Fauna helmintológica de bovinos da região Centro-oeste do Estado de Minas Gerais, Brasil. (Brazil: SciELO Ciência Rural), 934–938.
Ramünke S., De Almeida Borges F., Von Son-De Fernex E., Von Samson-Himmelstjerna G., Krücken J. (2018). “Molecular marker sequences of cattle Cooperia species identify Cooperia spatulata as a morphotype of Cooperia punctata,”. PloS One 13 (7), 1–21. doi: 10.1371/journal.pone.0200390
Rashid M. H., Beveridge I., Vaughan J. L., Jabbar A. (2019). “Worm burdens and associated histopathological changes caused by gastrointestinal nematodes in alpacas from Australia,”. Parasitol. Res. 118 (3), 1031–1038. doi: 10.1007/s00436-019-06237-6
Rich K. M., Schaefer K. A., Thapa B., Hagerman A. D., Shear H. E. (2022). 2022 ReSAKSS Annual Trends and Outlook Report 33 An Overview of Meat Processing in Africa Introduction and Scope of Chapter. Kigali, Rwanda Washington, DC: AKADEMIYA2063 International Food Policy Research Institute (IFPRI).
RMRDSA (2018). Red Meat Research and Development (RMRD), THE SOUTH AFRICAN RED MEAT INDUSTRY. (South Africa: Red Meat Producers Organisation).
Saki A., Hoffmann W. H. (2020) Economic Sustainability of Extensive Beef Production in South Africa. Available at: https://scholar.sun.ac.za.
Scholtz M., Mpayipheli M., Frylinck L., Theunissen A., Strydom P., Jonker T., et al. (2013). Beef-Cattle-management-and-systems-development (Pretoria: Department of Animal Production Institute (ARC)).
Shephard R. W., Hancock A. S., Playford M., Oswin S. (2022). “A systematic review and meta-analysis of impact of strongyle parasitism on growth rates in young cattle,”. Veterinary Parasitol. 309, 1–9. doi: 10.1016/j.vetpar.2022.109760
Sifiso N., Lindikaya M., Bonani N. (2013) Mainstreaming subsistence farmers through communal feedlot: Case of Umzimvubu Custom Feeding Program in Mount Frere. Available at: https://www.researchgate.net/profile/Myeki-Lindikaya/publication/312554593 (Accessed 13, 2022).
Soji Z., Mabusela S. P., Muchenje V. (2015). “Associations between animal traits, carcass traits and carcass classification in a selected abattoir in the Eastern Cape Province, South Africa,”. S Afr J. Anim. Sci. 45 (3), 278–288. doi: 10.4314/sajas.v45i3.6
Stats SA (2023). STATISTICAL RELEASE P0441 Gross domestic product (Pretoria, South Africa: Department of Statistics South Africa). Available at: www.statssa.gov.za,info@statssa.gov.za,Tel+27123108911.
Sweeny J. P. A., Gardner G. E., Dobson R. J., Jacobson C., Bell K. (2011). “Associations between trichostrongylid worm egg count and productivity measures in Dorper lambs,”. Vet. Parasitol. 180 (3–4), 307–314. doi: 10.1016/j.vetpar.2011.03.015
Trindade A., Couto L., Heller L., Zapa D., de Aquino L., Ferreira L., et al. (2023). “Cattle tick and gastrointestinal nematodes strategic control in dairy 31/32 Gyr x Holstein and beef ½ Brangus: is the same way?,”. Livest Sci. 268, 1–12. doi: 10.1016/j.livsci.2023.105154
Vercruysse J., Claerebout E. (2001). Treatment vs non-treatment of helminth infections in cattle: defining the threshold. Vet. Parasitol. 98, 195–214. doi: 10.1016/S0304-4017(01)00431-9
Waruiru R. M. (2004). The In£uence of Supplementation with Urea^Molasses Blocks on Weight Gain and Nematode Parasitism of Dairy Calves in Central Kenya In£uence of supplementation with urea^molasses blocks on weight gain and nematode parasitism of dairy calves in central Kenya. Vet. Res. Commun. 28 (4), 307–315. doi: 10.1023/B:VERC.0000026670.64936.18
Wymann M. N., Bonfoh B., Traore K., Tembely S., Zinsstag J. (2007). “Species diversity and acquisition of gastrointestinal parasites in calves aged 0-13 months in periurban livestock production in Mali,”. Vet. Parasitol. 143 (1), 67–73. doi: 10.1016/j.vetpar.2006.07.025
Keywords: abattoir, body condition score, fecal egg count, weight, feedlot cattle, high throughput
Citation: Mpofu BI, Slayi M, Mutero G, Mlahlwa S and Jaja IF (2023) Assessing body condition scores, weight gain dynamics, and fecal egg counts in feedlot and non-feedlot cattle within high throughput abattoirs of the Eastern Cape Province. Front. Anim. Sci. 4:1302320. doi: 10.3389/fanim.2023.1302320
Received: 27 September 2023; Accepted: 04 December 2023;
Published: 15 December 2023.
Edited by:
Peter Erickson, University of New Hampshire, United StatesReviewed by:
Eric Hatungimana, University of Rwanda, RwandaTess Stahl, The State University of New Jersey, United States
Copyright © 2023 Mpofu, Slayi, Mutero, Mlahlwa and Jaja. This is an open-access article distributed under the terms of the Creative Commons Attribution License (CC BY). The use, distribution or reproduction in other forums is permitted, provided the original author(s) and the copyright owner(s) are credited and that the original publication in this journal is cited, in accordance with accepted academic practice. No use, distribution or reproduction is permitted which does not comply with these terms.
*Correspondence: Beautiful Isabel Mpofu, isabelmpofu24@gmail.com; Ishmael Festus Jaja, ijaja@ufh.ac.za