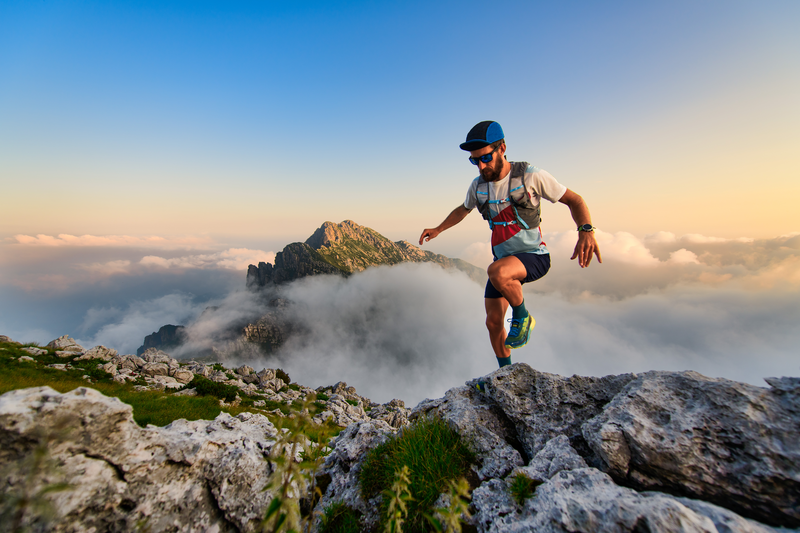
94% of researchers rate our articles as excellent or good
Learn more about the work of our research integrity team to safeguard the quality of each article we publish.
Find out more
ORIGINAL RESEARCH article
Front. Anim. Sci. , 02 November 2023
Sec. Product Quality
Volume 4 - 2023 | https://doi.org/10.3389/fanim.2023.1278495
This article is part of the Research Topic Animal-Derived Foods in Our Diets: Nutrition, Health and Social Implications View all 15 articles
Plant lipids in the diet are known to modify milk fatty acid (FA) composition and mitigate ruminal methane emissions. The objective of the present work was to examine the potential of milled rapeseeds and oats to decrease both milk saturated FAs and ruminal methane emissions in practical farm settings. In the pilot study, six Finnish Ayrshire cows were fed a control diet for 3 weeks, which was then followed by a lipid-rich test diet for 3 weeks. The experimental diets were based on grass silage supplemented with barley and rapeseed meals in the control diet and with oats and milled rapeseeds in the test diet. The lipid inclusion rate was 55 g/kg dry matter (DM). In the main study, the whole Finnish Ayrshire research herd in milk (n = 49–59) was used in a switch-back-designed study. The cows were fed a control diet for 3 weeks, then a test diet for 4 weeks, and, finally, a control diet for 3 weeks. The diets were the same as in the pilot study except for a lower lipid inclusion level of 50 g/kg DM. The test diet decreased DM intake by 15% and energy-corrected milk (ECM) yield by 13% in the pilot study. The adjustment of supplemental lipids from 55 g/kg to 50 g/kg DM was successful, as the DM intake decreased only by 4% relative to the control diet in the main study. Furthermore, the yields of milk, lactose, protein, and fat were also unaffected by dietary lipids in the main study. The milk fat composition was significantly altered in both studies. The milk fat saturated FAs were decreased by 16%–20% in the test diet, mainly due to the de novo FAs of 6- to 16-carbons (a reduction of 22%–48%). Milk fat cis-9 18:1 was increased by 63%–78% in the test diet relative to the control. Dairy products’ (milk, butter, and cheese) organoleptic quality was not compromised by the modified lipid profile. Ruminal methane and hydrogen intensities (n = 23; g or mg/kg ECM) were 20% and 39% lower, respectively, in the test diet than in the control diet. This reduction can be attributed to a lower amount of organic matter fermented in the rumen, as indicated by the lower DM intake and nutrient digestibility.
Ruminants are dependent on the anaerobic microbial ecosystem in the rumen to ferment and transform human-indigestible forages into dairy and meat products of high quality. However, due to the microbial metabolism of carbohydrates, ruminants are also significant producers of enteric methane (CH4). In addition, CH4 formation represents an unproductive loss of dietary energy to the ruminant animal (Min et al., 2022). Adding plant lipids that are not fermentable in the rumen to dairy cow diets suppresses CH4 emission intensity [g CH4/kg energy-corrected milk (ECM)], on average, by 12% (Hristov et al., 2022). Oilseeds have a CH4 mitigation potential similar to that of pure oils, with the advantage that the lipid may be released at a slower rate in the rumen. Therefore, oilseeds may have a less harmful effect on the rumen function (Hristov et al., 2022) and, in turn, allow further lactational performance at high levels of lipid inclusion. However, practical evidence on the feasibility and effectiveness of feeding milled full-fat oilseeds at the whole-herd level to mitigate ruminant methane emissions is lacking.
Cardiovascular disease (CVD) is the leading cause of morbidity and mortality for humans worldwide (Perna and Hewlings, 2023). Compiled evidence suggests that the replacement of saturated fatty acids (SFAs) with unsaturated ones in dairy products may alleviate human CVD risk (Livingstone et al., 2012; Clifton and Keogh, 2017; Vasilopoulou et al., 2020). The research on the effects of individual SFAs is inconclusive, but most studies indicate that SFAs of 12- to 18-carbons may increase the risk for CVD, whereas shorter-chain SFAs may be beneficial or neutral (Perna and Hewlings, 2023). However, some studies suggest that 18:0 stearic acid (SA) does not increase CVD risk (Briggs et al., 2017). Dietary unsaturated fatty acids (FAs) have great potential to modify the FA composition of ruminant milk by decreasing the proportion of SFAs and increasing that of unsaturated FAs inherent to lipid supplements, such as cis-9 18:1 oleic acid (OA) rich in the lipids of rapeseed (Brassica napus) and oats (Avena sativa) (Collomb et al., 2004; Fant et al., 2023). Furthermore, the ability to increase milk fat monounsaturated FAs through dietary inclusion is much greater in magnitude than with polyunsaturated ones (Kliem and Shingfield, 2016). Beyond a certain threshold of dietary lipid supply, both feed intake and milk yield decline significantly (Drackley et al., 2007; Huhtanen et al., 2008; Vanhatalo and Halmemies-Beauchet-Filleau, 2020). Consequently, this threshold, influenced by various factors, especially the basal diet and the characteristics of the lipid supplement (Benchaar et al., 2015; Halmemies-Beauchet-Filleau et al., 2017), should not be exceeded when adjusting milk fat composition in practical farm settings. Moreover, the form in which lipids are included in the ruminant diet significantly affects their bioavailability and the ultimate composition of the final product. Furthermore, rupture of rapeseed seedcoats is necessary to enhance the availability of lipids within the seeds for absorption (Kairenius et al., 2009).
The milk FA composition affects the texture, flavor, and shelf life of dairy products (Kennelly, 1996; Hillbrick and Augustin, 2002). However, monounsaturated FAs are less prone to oxidation than polyunsaturated ones (Kennelly, 1996), which reduces the risk for off-flavors and shorter shelf lives. Furthermore, dairy products with lipids rich in OA have resulted in products with softer textures, but with similar flavors to standard products (Chen et al., 2004; Ryhänen et al., 2005).
The objective of this study was to examine the potential of the lipid in milled rapeseeds and oats to replace a part of the SFAs in milk fat with monounsaturated ones inherent to these lipid supplements and to mitigate ruminal methane emissions in practical farm settings. We hypothesized that replacing rapeseed meal and barley with milled rapeseed and oats in a dairy cow diet will not impair the lactation performance or sensory quality of the dairy products but will soften the milk fat and mitigate rumen methanogenesis.
The experiments were conducted at the University of Helsinki Viikki research farm (60°13′N, 24°02′E) in Finland. The pilot dairy cow study was conducted in the spring of 2018 and the main dairy cow study in the autumn of 2018. Similar dietary ingredients and the same analytical methods were used in both experiments.
The effects of a tailored test diet elevated in lipids were first studied with a limited number of dairy cows. This pilot study was carried out to ensure maximal changes in milk fat composition, without compromising animal health and performance, when later implementing the test diet for a large number of animals. The pilot study was conducted with six multiparous Finnish Ayrshire cows that weighed (mean ± SD) 711 kg ± 35.3 kg, were of parity 3.0 ± 0.63, were 181 ± 32.5 days in milk, and were producing 36.0 kg/d ± 4.77 kg/d of milk pre-trial. All cows were fed a control diet for 3 weeks (period 1), followed by a lipid-rich test diet for another 3 weeks (period 2). The dietary shift was made gradually over 5 days. The dairy cow partial mixed rations (PMRs) were based on grass silage (Table 1). The prewilted grass silage was prepared from a first cut of mixed timothy (Phleum pratense) and meadow fescue (Festuca pratensis) sward, which was ensiled with a formic acid-based additive (4–6 L/t feed; AIV®2 Plus Na; Taminco Finland Ltd, Eastman Chemical Company, Oulu, Finland) in big bales. The concentrates in the PMRs comprised home-grown cereals, rapeseed feeds as a protein supplement, molassed sugar-beet pulp (Opti Leike mure; Lantmännen Feed Ltd, Turku, Finland), and vitamins and minerals (Seleeni-E-Melli TMR; Lantmännen Feed Ltd). The rapeseed protein was isonitrogenously supplied either as a lipid-extracted meal (control diet; Farmarin Rypsi Mixer; Hankkija Ltd, Hyvinkää Suomi) or as domestic full-fat seeds (Hauhon Myllärit Ltd, Hauho, Finland), and was milled using a sieve pore size of 6–8 mm (test diet). The cereal in the control diet was barley (Hordeum vulgare), and in the test diet, oats (Table 1). The amount of additional plant lipids in the test diet from rapeseeds and oats was adjusted to ca. 55 g/kg diet dry matter (DM). The PMRs were distributed three times per day at 09:00, 15:00, and 20:00, fed freely, and supplemented with 3 kg/d of commercial concentrate (Maituri 10 000; Lantmännen Feed Ltd) at milking times. The main ingredients of the commercial concentrate were rapeseed meal, wheat, barley, molassed sugar-beet pulp, sugar-beet molasses, faba beans, and protected fat. The cows had free access to drinking water.
The cows were kept in tie-stalls equipped with PMR feeding troughs (Insentec RIC, Marknesse, the Netherlands) that registered intakes. They were milked twice a day (Delpro; DeLaval, Tumba, Sweden) starting at 06:00 and 17:00. The samples of feed and feces were collected during the last week of both periods. The fecal spot (1-L) sample was taken from the rectum during five consecutive milkings, starting on the morning milking of day 17. Furthermore, all milk from the cows was collected over these milkings to produce around 350 L of control and modified milk. This milk was analyzed for major constituents (using a 15- to 20-ml sample preserved with Bronopol; lactose, crude fat, crude protein, urea), as well as FAs (using an unpreserved milk sample of 100 ml). In addition, ultra-high temperature (UHT) processed milk, cheese, and butter were prepared from raw milk for sensory analyses. After adjusting the milk fat content to 1.5%, UHT milk was produced at Valio R&D (Helsinki, Finland) by heating the milk to 150°C for 3 s. The butter and semi-hard Dutch-type cheese were produced at Häme University of Applied Sciences’ pilot dairy plant (Hämeenlinna, Finland). To produce butter, the cream was pasteurized and churned in two phases at 10°C. Salt was added to achieve a salt content of 1.4%. The test diet butter required a longer churning time than the control diet butter (120 min vs. 240 min). To produce semi-hard Dutch-type cheese, raw milk was standardized to a fat-to-protein ratio of 0.8 and pasteurized (for a minimum of 72°C for 15 s). The DVS CHN-019 starter culture (Chr. Hansen, Hørsholm, Denmark) and the CHY-MAX E (Chr. Hansen) rennet were added. The cheese loaves were ripened for 7 weeks at 11°C. The dry matter and fat contents for the control diet cheese were 53.6% and 19.0%, respectively, and for the test diet cheese 54.0% and 20.0%, respectively. In addition, the samples of milk from individual cows were taken every third day at the morning and evening milking starting from the dietary change. The samples were composited according to milk yield by cow and by day and analyzed in a similar way to the tank milk for the major constituents and FAs.
The whole Finnish Ayrshire research herd in milk (n = 49–59) was used in a switch-back-designed study. The cows were fed a control diet for 3 weeks (period 1) followed by the lipid-rich test diet for 4 weeks (period 2). After this, all cows were switched back to the control diet (3 weeks; period 3). The dietary shifts were made gradually over 5 days. The last week of all periods was the sampling week. The cows were housed in an insulated free-stall barn equipped with a milking robot (Lely Astronaut A3; Lely, Maassluis, the Netherlands). The dairy herd was predominantly autumn calving and the number of cows in milk was 49, 52, 50, and 59 at the beginning of the experiment and during the sampling weeks of periods 1, 2, and 3, respectively. The number of days in milk was, on average, 176, 153, 141, and 117 at the beginning of the experiment and in the sampling weeks of periods 1, 2, and 3, respectively.
The dietary ingredients were the same as in the pilot study. However, based on the observations of the pilot study, the amount of supplemental lipids in the test diet was adjusted from 55 to 50 g/kg DM in order to promote feed intake, and, in turn, higher milk production while on the test diet. The adjustment was carried out by reducing the proportion of milled rapeseed and, correspondingly, increasing that of rapeseed meal in the test diet PMR (Table 1). In addition, propylene glycol was added to the PMR concentrate mixture to prevent concentrate dusting. The chemical composition of the PMR concentrate ingredients is presented in Table 2. The animals had free access to PMRs that were distributed four times per day at 08:00, 12:00, 18:00, and 22:00. When visiting the milking robot, the cows producing less than 30 kg of milk per day, between 30 kg of milk per day and 40 kg of milk per day and over 40 kg of milk per day at the beginning of the trial received 3 kg/d, 4 kg/d or 5 kg/d of commercial concentrate (Maituri 10000, Lantmännen Feed Ltd), respectively, throughout the experiment. The main ingredients of the commercial concentrate were rapeseed meal, maize, barley, sugar-beet molasses, molassed sugar-beet pulp, and protected fat.
Table 2 The chemical composition of the partial mixed rations (PMRs) concentrate ingredients in the main study.
The PMR feeding troughs (Insentec RIC) registered intakes automatically and individually. The milk yield, body weight, and commercial concentrate distribution were individually registered by the milking robot throughout the experiment. The experimental feeds were sampled once a day during the sampling week (n = 7), composited by periods, and stored frozen at −20°C until analysis.
The milk and feces were sampled from 13 multiparous dairy cows that weighed (mean ± SD) 678 kg ± 62.4 kg, were of parity 2.9 ± 1.75, and were producing 32.1 kg/d ± 7.5 kg/d of milk pre-trial. Of these cows, 10 were in late lactation (number of days in milk ranging from 153 to 308 at the beginning of the experiment) and three were in early lactation (number of days in milk ranging from 13 to 27 at the beginning of the experiment). The milk was individually sampled on day 15 of period 1 onwards, via the Lely Shuttle, from the first milking every third day at 09:00 onwards. The milk preserved with Bronopol (15 ml–20 ml) was analyzed for lactose, crude fat, crude protein, and urea, and unpreserved milk (10 ml) for FAs. The milk FA samples were stored frozen at −20°C prior to analysis. In addition, the tank milk was sampled every second day at 09:00 and analyzed for lactose, crude fat, crude protein, and urea throughout the study. The spot fecal samples (1 L) from the rectum were taken every day during the sampling week (n = 7) at 09:00 onwards, composited by cow and period, and frozen at −20°C before the analysis.
All cows freely visited the milking robot equipped with the GreenFeed system (C-Lock Inc., Rapid City, SD, USA) that measures gas exchange (Huhtanen et al., 2015). Automatic gas calibrations using a mixture of nitrogen (N2) and oxygen (O2), and a mixture of CH4, O2, hydrogen (H2), and carbon dioxide (CO2) were performed daily. The CO2 recovery tests were conducted at the beginning of the experiment and every sampling week. Only the records of cows (n = 23) that were in milk during all three experimental periods and, on average, had 10 or more accepted readings from the GreenFeed system (more than 2 min of uninterrupted gas recordings during a visit) in the last week of each experimental period were used in the statistical analysis. These cows weighed 646 kg ± 72.8 kg, were of parity 2.3 ± 1.70, and produced 30.5 kg± 7.74 kg of milk per day pre-trial. Eight cows were in late lactation (number of days in milk ranging from 155 to 332 at the beginning of the experiment) and 15 were in early lactation (number of days in milk ranging from 0 to 36 at the beginning of the experiment). The energy-corrected milk for the gas intensity data was calculated from the tank milk composition of the sampling week and individual milk yields.
The primary DM content of feeds and feces was determined by oven drying at 103°C for 20–24 h. The silage DM content was corrected for volatile losses by Huida et al. (1986). The chemical composition of feeds and feces was analyzed by standard procedures. Prior to the analysis, the dried feed (50°C for 48 h) and fecal (70°C for 48 h) samples were ground to pass through a 1-mm sieve. The ash was determined by ashing at 600°C for 20–24 h (Heraeus Thermicon T; Heraeus, Hanau, Germany). The neutral detergent fiber (NDF) was determined using sodium sulfite (Van Soest et al., 1991) and α-amylase (only concentrates) with an automatic FiberTherm FT12 analyzer (Gerhardt, Königswinter, Germany). The NDF content is reported on an ash-free basis. The crude protein was analyzed, as described by Pitkänen et al. (2023), using undried material for feces. For the analysis of total fat, the samples were hydrolyzed with 800 mL of HCl (4 mol/L) (SoxCap 2047 hydrolysis unit; FOSS Analytical, Hillerød, Denmark) following an extraction with 90 mL of petroleum ether (FOSS Soxtec 8000 extraction unit; FOSS Analytical, Hillerød, Denmark). The starch content was measured by using the amyloglucosidase and α-amylase method with a K-TSTA kit (Megazyme Co., Wickslow, Ireland) and a spectrophotometer (Shimadzu UV-VIS mini1240; Shimadzu Europa GmbH, Duisburg, Germany), according to the manufacturer’s instructions (Pitkänen et al., 2023). The silage fermentation quality was determined from undried samples, as described by Pitkänen et al. (2023). The FA analysis of feeds and milk is described in detail by Lamminen et al. (2019). In brief, the lipids in feeds were extracted with a mixture of hexane and isopropanol (3: 2, vol: vol), and the lipids in milk with a mixture of ammonia, ethanol, diethyl ether, and hexane (0.2: 1.0: 2.5: 2.5, vol: vol). The fatty acid methyl esters were prepared and analyzed using a gas chromatograph (GC2010 Plus; Shimadzu, Kyoto, Japan) equipped with a 100-m fused silica capillary column (CP-SIL 88, Agilent J&W, Santa Clara, CA, USA). The milk lactose, crude fat, crude protein, and urea contents were determined by mid-infrared analysis in a commercial laboratory (MilkoScan FT+, Foss Electric A/S, Hillerød, Denmark; Valio Ltd, Seinäjoki, Finland). A trained sensory panel (n = 10) was used to evaluate the test and control UHT milks. Overall liking was rated, and the sensory profile of the milks was studied using the Check-All-That-Apply (CATA) method. Regarding the test and control butter and cheese, both the concept and sensory properties were evaluated by the respondents (n = 151), who were at least monthly users of butter and at least weekly users of cheese. Interest toward the concept, overall liking, product attributes (CATA), preference (which butter/cheese would you prefer), and reasons for preference were studied.
Energy-corrected milk yield was corrected to an energy content of 3.14 MJ/kg (Luke, 2023). The metabolizable energy (ME), metabolizable protein (MP), and protein balance in the rumen (PBV) were calculated according to the Finnish feed evaluation system (Luke, 2023). The apparent digestibility of nutrients was calculated using acid-insoluble ash as an internal marker in feeds and feces (Van Keulen and Young, 1977).
The data were analyzed using PROC MIXED of the Statistical Analysis System (SAS version 9.4, 2012). In the pilot dairy cow study, the data on nutrient intake and digestibility were analyzed with pairwise t-tests (PDIFF option), with a statistical model containing diet as a fixed effect and cow as a random effect. The time series data on lactational performance and milk composition were analyzed by ANOVA for repeated measures using polynomial contrast (linear, quadratic, cubic), and a model that had the sampling day as the fixed effect with a Satterthwaite correction. The AR(1) covariance structure was applied with a cow as the subject for repeated measures. In the main dairy cow study, only data obtained during sampling weeks were analyzed by ANOVA for linear and quadratic responses. The statistical model contained period as a fixed and cow as a random effect. The RedJade Sensory Software (RedJade Sensory Solutions LLC, Pleasant Hill, CA, USA) was used for the collection and analysis of the sensory data of the UHT milks evaluated by an expert panel. The Z test was used to analyze differences between the milks in overall liking and sensory profile. Data on the sensory evaluation of cheese and butter by a consumer panel was analyzed using Microsoft Excel® (version 2016; Microsoft Corporation, Redmond, WA, USA). The differences in overall liking and product preference were analyzed using the t-test, and the frequencies of the different product attributes and product preferences were calculated. The reasons to prefer a cheese/butter were asked with an open question. In all analyses, the results were considered statistically significant when the p-value was ≤ 0.05. The differences at a p-value > 0.05 to 0.10 were considered as a trend toward significance. The normality of the residuals was tested using a univariate procedure and the Shapiro–Wilk test. If the residuals were not normally distributed, the variables were transformed (log, square, inverse) to obtain a normal distribution of the residuals.
The chemical composition of the experimental feeds is presented in Table 3. The grass silages were of high (main study) or moderate (pilot study) nutritive value in terms of their digestible organic matter contents, which is typical of the early to normal growth stage for silage making. In the main study, the grass silage was restrictively fermented, as indicated by the low levels of fermentation acids and high levels of residual sugars. In the pilot study, the grass silage was more extensively fermented. The forage-to-concentrate ratio of the diets consumed averaged 54: 46 and 51:49 on a DM basis for the pilot and the main dairy cow studies, respectively. The experimental PMR concentrates were isonitrogenous for rapeseed protein, but in the control diet, the concentrate contained more starch and less total fat than that of the test diet. Furthermore, milled rapeseeds contained 10 times more total fat than rapeseed meal (Table 2). The predominant FA in grass silage was cis-9,cis-12,cis-15 18:3 α-linolenic acid (ALA), whereas for the PMR concentrates cis-9,cis-12 18:2 linoleic acid (LA) and OA were the most abundant (Table 3). The FA composition of the PMR concentrate ingredients used in the main dairy cow study is presented in Table 2. Of the total FA in the milled rapeseeds, OA formed 53 g/100 g FA followed by 22 g/100 g FA of LA, and 12 g/100 g FA of ALA. The lipids in all the experimental rapeseed feeds were low in cis-13 22:1 erucic acid. The oats contained 1.7 times more total fat than barley. Compared with barley lipids, oat lipids contained more OA (12 g/100 g FA vs 34 g/100 g FA) and less LA (54 g/100 g FA vs 40 g/100 g FA).
Table 3 The chemical composition of the experimental feeds in the pilot study and in the main study.
In the pilot dairy cow study, milled rapeseeds together with the oats tended to decrease DM intake by 3.3 kg/d (p = 0.072; Table 4) relative to the control diet, but on ME intake the decrease was only numerical (p > 0.10). The test diet increased the intake of total fat by 0.78 kg/d (p < 0.001). The intake of all FA, OA, LA, and ALA, in particular, was increased by the test diet (p < 0.001; Supplemental Table 1). Furthermore, the apparent total tract digestibility of all nutrients was lower for the test than the control diet (p ≤ 0.003; Table 4; Supplemental Table 1).
Table 4 Nutrient intake, apparent total tract digestibility coefficients, and the composition of tank milk used for processing dairy products, and the dairy product sensory quality in the pilot study.
In the main dairy cow study, milled rapeseeds together with oats decreased the DM intake by 0.9 kg/d (p = 0.027 for quadratic response; Table 5) relative to the control diet; the decrease originating mainly from the lower silage intake (p = 0.009). Furthermore, the test diet decreased crude protein and starch intake (p ≤ 0.003), but increased that of energy-rich total fat by 0.98 kg/d (p < 0.001). Of the individual FAs, the consumption of OA, LA, and ALA in particular was increased by the test diet relative to the control (p < 0.001). Lipid supplementation had no effect on ME intake (p > 0.10). For both diets, the PBV was positive and was, on average, 34 g/kg diet DM. The apparent total tract digestibility of all nutrients was lower for the test than for the control diet (p < 0.001).
In the pilot dairy cow study, the milk yield tended to decrease cubically from 26.6 kg/d to 23.3 kg/d (p = 0.053) and the ECM yield decreased linearly from 27.6 kg/d to 23.9 kg/d (p = 0.041) after switching from the control to the test diet (Supplemental Table 2). However, dietary plant lipids had no effect on milk fat, lactose, protein, and urea concentrations (p > 0.10).
In the main dairy cow study, milled rapeseeds together with oats did not affect the yields of milk, ECM, lactose, fat, or protein (p > 0.10 for quadratic response; Table 6). However, the yields of milk, ECM, lactose, and protein decreased linearly (p ≤ 0.025) during the experiment. Dietary plant lipids had no effect on milk fat or lactose concentration (p > 0.10), but there was a subtle quadratic increase (p = 0.010) in the milk protein concentration, with a concomitant linear decrease (p = 0.028) in the milk urea concentration as the experiment progressed.
The milk FA composition was similarly modified by the test diet in both experiments (Table 6; Supplemental Table 2), with the large changes reaching a plateau within 10 days after the dietary changes (Figures 1, 2; Supplemental Table 2). The milled rapeseeds together with oats decreased the total SFA in milk fat by 11.7% to 14.2%-units (p < 0.001 for quadratic response; Table 6; Supplemental Table 2) relative to the control diet. The milk fat concentration of all SFAs of 6- to 16-carbon, 16:0 palmitic acid (PA), in particular, was decreased (p≤ 0.005) by the test diet. By contrast, it almost doubled the milk fat OA and SA concentrations (p < 0.001) relative to the control diet. Furthermore, it resulted in minor decreases in milk fat LA and ALA (p < 0.001) concentrations. The test diet increased milk fat concentration of total trans FAs (p < 0.001), of which trans-11 18:1 predominated. However, the increases in milk fat trans FAs were rather limited in magnitude.
Figure 1 Effect of milled rapeseeds and oats on tank milk saturated fatty acid (SFA), monounsaturated fatty acid (MUFA), and polyunsaturated fatty acid (PUFA) concentrations in the main dairy cow study. Arrows represent the dietary change of the whole herd from control diet in period 1 (P1) to lipid supplemented diet in period 2 (P2), and back to control diet for period 3 (P3).
Figure 2 Effect of milled rapeseeds and oats on tank milk palmitic acid (PA, 16:0), stearic acid (SA, 18:0), and oleic acid (OA, cis-9 18:1) concentrations in the main dairy cow study. Arrows represent the dietary change of the whole herd from control diet in period 1 (P1) to lipid supplemented diet in period 2 (P2), and back to control diet for period 3 (P3).
No significant differences in sensory characteristics were seen in the UHT milk, butter, and cheese produced from the test milk and the control milk (p >0.05; Table 4). In overall ratings, the new products with less saturated fat got very similar ratings to the control products. There was also no major difference in which products, the test or the control, were preferred. The test butter was considered softer and easier to spread, and more than 40% of respondents found nothing to improve in the butter. The saltiness of the test cheese was better and the taste milder than in the control cheese. Both cheeses were soft, and a slightly harder construction had been hoped for. Regarding the concept of reduced saturated fat dairy products, over half of the respondents considered a good FA composition important. Based on the product description, 70% of respondents would probably buy milk products with a modified FA composition.
The dairy cow gas exchange is presented in Table 7. The milled rapeseeds together with the oats decreased ruminal CH4 and H2 total emissions (g/d and mg/d, respectively), yields (g/kg DM intake or mg/kg DM intake), and intensities (g/kg ECM or mg/kg ECM; p < 0.001 for the quadratic response). Depending on the emission unit, the decrease was 16%–20% for CH4 and 36%–39% for H2. However, the effect of plant lipids on CO2 emissions (decrease of 3%–5%; p ≤ 0.084) was limited in magnitude. The plant lipids had no major effect on the O2 consumption of dairy cows (p > 0.10).
Table 7 Gas production or consumption (mass per day), gas yield (mass per dry matter intake), and gas intensity (mass per energy-corrected milk production) in the main study.
The novel feature of this experiment was in assessing the feasibility of simultaneously decreasing both bovine milk fat SFAs and ruminal methane emissions when milled rapeseeds and oats instead of rapeseed meal and barley are fed to animals in practical whole-herd conditions. In addition, the milk was processed into several dairy products (e.g., UHT milk, butter, and cheese), of which the sensory quality was evaluated to confirm the applicability up to the final products.
Given the limited number of animals, the results on feed intake and milk yields obtained in the pilot study should be interpreted with some caution. However, it is noteworthy that the results were highly consistent between the pilot and the main dairy cow studies, except for variations in animal performance. Nevertheless, at high lipid inclusion rates, a significant decrease in feed intake and milk yield, as observed in the pilot study, is expected when a situation-specific threshold in lipid supply is surpassed (Drackley et al., 2007; Benchaar et al., 2015; Halmemies-Beauchet-Filleau et al., 2017). This is discussed in more detail later below.
The main forage component of the diet affects bovine milk FAs (Glasser et al., 2008) and CH4 response to plant lipids (Vanhatalo and Halmemies-Beauchet-Filleau, 2020). Our experimental diets were based on digestible grass silage that is typical in northern latitudes. The grass silage-rich diets together with using oats instead of barley as the cereal for the test diet PMR led to moderate starch contents for the control (123–158 g/kg DM) and test diets (104 g/kg DM). The lipid content and the FA composition of milled rapeseeds, oats, and barley were similar to previous reports (Welch, 1975; Brask et al., 2013b), with OA forming a major part of the lipid for both milled rapeseeds (53 g/100 g FA) and oats (34 g/100 g FA) in the present work.
The milled rapeseeds together with oats decreased DM intake by 15% relative to the control diet in the pilot study. The fluctuation in the daily feed intake indicated a slight excess in lipid supplementation for efficient rumen function. This was reflected also in the standard error of the mean (SEM), which was moderately high for feed intake. To maintain a higher and more regular feed intake, the lipid supplementation rate was decreased from 55 g/kg to 50 g/kg test diet DM for the main study. The lower rate was successful as DM intake was only decreased by 4% relative to the control diet in the main study. At high inclusion rates (i.e., at 40 g/kg or more in DM), lipid supplementation has often suppressed feed intake (Huhtanen et al., 2008; Bayat et al., 2018; Ramin et al., 2021a), with the decrease being generally more pronounced on starch-rich diets (Benchaar et al., 2015; Vanhatalo and Halmemies-Beauchet-Filleau, 2020). The rather low dietary starch content together with the non-excessive lipid inclusion rate probably explains the limited reduction in the feed intake in the main study. In addition to the decreased DM intake, using oats that contain less starch than barley in the test diet relative to the control diet contributed to 0.77 kg and 1. 28 kg lower daily starch intake by the test diet cows in the pilot and main dairy cow studies, respectively. However, the lower starch intake on the test diet was compensated by energy-rich lipids leading to similar ME intakes between diets in both studies.
The milled rapeseeds together with oats substantially increased OA, LA, and ALA intakes relative to the control diet, thus reflecting the FA content and composition of the dietary feed ingredients. The vast majority of the supplemental OA was derived from milled rapeseeds and to a lesser extent from oats. The increase in OA intake was of similar magnitude to the previous studies using high rapeseed oil supplementation (Ferlay et al., 1993; Bayat et al., 2018). It is noteworthy that on the control diet ALA, inherent to the chloroplasts in forage leaves (Glasser et al., 2013), represented the major FA consumed by cows; therefore, highlighting the importance of the basal forage to FA intake. Though forages have rather low lipid concentrations, lipid intake from forage can be substantial because forage intake is typically high in ruminant diets (Glasser et al., 2013).
In the present study, supplying a 50–55 g/kg diet DM of lipids from milled rapeseeds and oats significantly suppressed organic matter and the fiber total tract digestibility. This may also explain, at least in part, the decrease in DM intake of the test diet relative to the control diet. Jenkins (1993) proposed various explanatory mechanisms for this, including the direct adverse effects of unsaturated FAs on ruminal microbial communities, cellulolytic microbes in particular, and free FAs forming a protective lipid layer over feed particles in the rumen. However, several reports have subsequently challenged these theories on fiber-rich diets based on grass or legume silage that indicate little if any effect of plant oils on fiber digestibility, even at rather high inclusion rates (Benchaar et al., 2015; Halmemies-Beauchet-Filleau et al., 2017; Bayat et al., 2018). In addition to lipid supplementation, switching cereal fiber quality from barley in the control diet to oats in the test diet may have contributed to a lowered fiber digestion of the test diet. Furthermore, replacing barley with oats has previously decreased NDF digestibility (Vanhatalo et al., 2006; Ramin et al., 2021b). This can be attributed to the significantly higher indigestible NDF content of oats relative to barley (Ramin et al., 2021b).
Once the seedcoat is ruptured, the lipid digestibility of whole rapeseeds is similar to pure oil (Brask et al., 2013b). The digestibility of lipids is often increased (Benchaar et al., 2015; Halmemies-Beauchet-Filleau et al., 2017) or unaffected (Ferlay et al., 1993; Brask et al., 2013a; Brask et al., 2013b) by plant lipid supplementation. In the present studies, however, the apparent digestibility of total fat was unexpectedly lower for the lipid-supplemented test diet than for the control diet. In part, this may be attributable to the reduced intestinal absorption of SA at high post-ruminal flows (Glasser et al., 2008). Indeed, the intake of 18-carbon dietary unsaturated FAs was many times higher on the test diet relative to the control diet, with SA being the end-product of their ruminal biohydrogenation (Shingfield et al., 2010). Though the milling of rapeseeds was assessed as being visually successful, it can also be speculated that some seeds may have escaped the milling through the 6- to 8-mm sieves intact.
The linear decrease in the ECM yield in the pilot study after dietary change from the control to the test diet is in line with the concomitant large reduction in feed intake, and a numerical 6% decrease in the ME intake. However, due to the experimental design, the effect of time and diet cannot be separated in the pilot study. Therefore, a part of the linear decline in animal performance can be attributed to the natural and gradual decline in the milk yield of late-lactation cows. However, in the main dairy cow study, the ECM yield was unaffected by dietary plant lipids, which is consistent with similar ME intakes across treatments due to a much more limited decrease in feed intake. Previously milled rapeseeds have neither affected the ECM yields when supplementing diets based on grass silage (Kairenius et al., 2009; Mierlita et al., 2023) nor a mixture of grass and maize silages (Brask et al., 2013b). In addition, replacing barley with oats has not affected the ECM (Ramin et al., 2021b) or slightly increased it (Vanhatalo et al., 2006). Similar to the pilot study, the linear decrease in the ECM, and protein and lactose yields during the main study can be attributed to the advances in the lactation stage of animals, as 10 out of 13 were in late lactation at the beginning of the experiment and thus on a descending lactation curve. It is worth noting, however, that the decline in milk yield was almost twice less rapid in the main study than in the pilot study between the periods. This confirms that the advance in the lactation stage was not the only cause of the decline in the milk yield in the pilot study. Overall, milled rapeseeds together with oats had negligible effects on the production of milk and the major constituents of milk in the main dairy cow study.
The milled rapeseeds together with oats significantly modified milk FA composition. Relative to the control diet, the total SFA content of milk fat on the test diet was 14.2%-units lower in the pilot study and 11.7%-units lower in the main study. Plant lipids decreased total SFA by, on average, 0.013%- to 0.015%-units per g of supplemental FA. This decrease was similar in extent to previous reports for milled rapeseeds (0.013%-units per g of supplemental FA; Collomb et al., 2004), and for pure rapeseed oil supplementation (0.015%- to 0.019%-units per g of supplemental FA; Bayat et al., 2018; Razzaghi et al., 2022) the decrease in SFA being generally lower on diets high in fiber and low in starch (Razzaghi et al., 2022; Mierlita et al., 2023). Moreover, the 6- to 16-carbon SFAs, derived entirely or in the case of PA 50%–80% from mammary de novo synthesis (Halmemies-Beauchet-Filleau et al., 2013), were consistently 22% to 48% lower in milk fat from the test diet than from the control diet. This is in good agreement with the increased supply of long-chain FAs known to inhibit mammary de novo synthesis of short- and medium-chain SFAs (Shingfield et al., 2010).
The total monounsaturated FA was 48%–59% higher in milk fat from the test diet than the control diet. This increase principally originated from OA (0.013%- to 0.015%-units per g of supplemental FA) which was the predominant FA in the dietary lipid sources rapeseeds and oats. The increase in milk fat OA was similar to previous studies with rapeseed oil (0.010%- to 0.016%-units per g of supplemental FA; Bayat et al., 2018; Razzaghi et al., 2022) or when replacing barley with oats (0.015%-units per g of supplemental FA; Fant et al., 2023). Milk fat OA has a dual origin. Part of it originates from direct mammary uptake, with circulating OA being derived predominantly from the diet (Shingfield et al., 2010) or during a negative energy balance also from adipose tissue mobilization (Gross et al., 2011; Jorjong et al., 2014). Another part of milk fat OA originates from mammary desaturation of SA, which is the end-product of ruminal biohydrogenation of dietary18-carbon unsaturated FA (Shingfield et al., 2010). Therefore, the significant increase in OA, LA, and ALA intakes for the test diet is directly, and, via SA, also indirectly reflected in the milk OA in the present study. The increase in milk fat SA on the test diet is a typical response to plant lipid supplementation (Bayat et al., 2018; Razzaghi et al., 2022; Fant et al., 2023).
The present increases in milk trans FAs were limited for lipid in milled rapeseeds and oats (0.0011%- to 0.0014%-units per g of supplemental FA) compared with previous studies with pure rapeseed oil (0.0046%- to 0.0062%-units per g of supplemental FA; Bayat et al., 2018; Razzaghi et al., 2022). In addition, the major trans isomers increased in milk fat by the test diet were trans-11 18:1 vaccenic acid and cis-9,trans-11 18:2 rumenic acid, with potentially beneficial effects on human health (Field et al., 2009; Koba and Yanagita, 2014). The moderate increase in milk fat trans FAs was in line with a previous report indicating higher ruminal OA and lower trans-FA outflow when milled rapeseeds were used instead of pure rapeseed oil supplementation (Kairenius et al., 2009). This suggests partial protection from the ruptured rapeseed seedcoat against ruminal lipid metabolism.
Despite the higher LA and ALA intakes, their milk fat concentrations were slightly lower on the test diet relative to the control diet. This is consistent with more extensive biohydrogenation of LA and ALA relative to OA in the rumen (Shingfield et al., 2010) and the limited effects on milk LA and ALA, when these polyunsaturated FAs have been supplemented in the form of plant oils (Rego et al., 2009; Halmemies-Beauchet-Filleau et al., 2017). The increase in ALA intake through forage generally results in a higher transfer efficiency from the diet into milk (Kalač and Samková, 2010; Halmemies-Beauchet-Filleau et al., 2013), probably due to the fact that more microbial digestion of surrounding material is needed before forage lipids are exposed to ruminal metabolism. This is supported by the concomitant decrease in milk fat ALA content and grass silage consumption despite higher general ALA intake on the test diet in the present study.
The sensory characteristics of the UHT milk, butter, and cheese containing less SFAs were similar to those of the control products and were preferred by an equal percentage of consumers as the control products. In general, the test diet butter and cheese were perceived to be of softer texture relative to the control products. Rapeseed lipid inclusion in the diet resulted in softer textures of dairy products, with acceptable organoleptic quality also previously (Ryhänen et al., 2005; Halmemies-Beauchet-Filleau et al., 2011). Furthermore, no change in the milk sensory quality was observed when oats replaced barley as a cereal in the dairy cow diet (Vanhatalo et al., 2006). The concept of reduced-saturated-fat dairy products was received positively by Finnish consumers, and respondents had a positive view of it. Most consumers considered the products suitable for themselves, and they would be ready to buy them if the product quality is the same as with current products. Some consumers did not entirely understand how the change in FA composition was achieved. This should be taken into account when communicating about these types of products. The consumers’ level of acceptance and attitudes toward test butter with low levels of SFAs and a low carbon emission footprint has been reported in a separate paper (Asioli et al., 2023). This complementary study indicated that about one-third of Finnish consumers was willing to pay a premium price for the new type of butter, the consumer attitudes being most promising with young and highly educated consumers.
For a considerable time, many human dietary guidelines recommend that SFA intake should be restricted to reduce the risk of CVD. As dairy foods are often the single greatest dietary source of SFAs, there has been a considerable number of studies examining how dairy cow diets can be modified to reduce the SFA content of milk fat, mainly by replacing them with cis-monounsaturated FAs or ALA. There are, however, few detailed human randomized controlled trials (RCTs) examining the chronic impact of such changes on milk FAs on markers of CVD risk. The review of 10 published RCTs, by Livingstone et al. (2012), indicated a tendency toward a believed beneficial lowering effect on fasting serum total and low-density lipoprotein cholesterol (LDL-C) following chronic consumption of modified milk and dairy foods. The recent detailed RESET RCT (Vasilopoulou et al., 2020) used diets containing milk, cheese, and butter with normal (control) or modified FA composition (Kliem et al., 2019), which was similar to the test diet milk in the current dairy cow studies. The study found that in adults at a moderate CVD risk, the consumption of FA-modified dairy foods for 12 weeks significantly moderated the increase in the levels of serum LDL-C seen on the conventional dairy food diet and improved vascular endothelial function. This provides more confidence that milk FA modification, as in the current studies, can provide health benefits. There is, however, increasing uncertainly that the heavy reliance on serum LDL-C as the key risk factor for CVD is too simplistic, in part because it takes no account of the variation in risk linked to the LDL particle size profile (Givens, 2023).
The effects of plant lipids on ruminal methanogenesis are dependent on the level of supplementation, the FA profile of the supplements, and the composition of the basal diet (Vanhatalo and Halmemies-Beauchet-Filleau, 2020). Lipids in milled rapeseeds and oats significantly decreased ruminal H2 load in the main dairy cow study. In addition, CH4 and H2 intensities (g or mg gas/kg ECM) were 20% and 39% lower, respectively, on the test diet than on the control diet. For each 1% plant lipid added to the diet, CH4 intensity was reduced by 4.6%. This agrees well with previous plant lipid data for rapeseed oil (a reduction of 4.5%–5.2% in CH4 intensity for each additional 1% in plant lipid; Bayat et al., 2018; Razzaghi et al., 2022) and replacing barley with oats (a reduction of 6.0% in CH4 intensity for each additional 1% in plant lipid; Fant et al., 2021; Ramin et al., 2021b) on high-grass silage diets. However, it was less effective compared with milled rapeseeds in a diet based on a mixture of grass and maize silage (a reduction of 8.2% in CH4 intensity for each additional 1% in plant lipid; Brask et al., 2013b).
The reduction of ruminal CH4 and H2 production in the present study can be attributed to the lower amount of organic matter fermented in the rumen, as indicated by the lower DM intake and nutrient whole-tract digestibility. Having more organic matter in the feces could be expected to increase CH4 emissions from manure. However, Ramin et al. (2021a) reported similar total fecal CH4 emissions in vitro (L/d) from feces of cows fed rapeseed lipids compared with unsupplemented ones, despite higher amounts of organic matter being left in the feces. This was due to a significantly lower CH4 yield (L/kg fecal organic matter) from the feces of cows fed rapeseed lipids. Furthermore, it is possible that the ruminal biohydrogenation of dietary unsaturated FAs served as a minor alternative sink for metabolic H2 to mitigate CH4 formation (Beauchemin et al., 2022). Dietary lipid supplementation may also shift rumen fermentation patterns from acetate to propionate (Vanhatalo and Halmemies-Beauchet-Filleau, 2020). However, decreases in methane production due to rapeseed lipids have not been associated with shifts in ruminal fermentation patterns on grass silage-based diets (Brask et al., 2013a; Bayat et al., 2018).
About 155.2 million tons of bovine milk is produced in EU-27 (Eurostat, 2021). If all the dairy cows in EU-27 consumed a diet mitigating CH4 emissions by 3 g of each kg of milk produced, which is comparable to the decrease observed in the present study, then annual CH4 emissions would decrease by 465,600 t in the EU-27 area. This decrease would represent about 8.4% of the annual bovine CH4 emission, 5.6% of the annual agricultural CH4 emission, and 3.2% of the annual total CH4 emission in EU-27 (Eurostat, 2021).
Replacing rapeseed meal and barley with full-fat milled rapeseed and oats in a whole-dairy-herd diet had no adverse effects on ME intake and milk production at a 50 g/kg lipid supplementation rate in the diet DM, but modified milk fat composition as OA inherent to lipid supplements replaced a substantial proportion of the SFAs in the milk fat. This decrease in milk fat SFAs can be attributed to the lower level of mammary de novo synthesis due to the increased supply of OA and its biohydrogenation end-product SA for milk fat synthesis. The dairy products (UHT milk, butter, cheese) with a modified lipid profile were of a similar organoleptic quality to the control products. Further research is needed to assess whether or not the changed milk FA profile has long-lasting health benefits when consumed by humans. The lipids in the milled rapeseeds and oats significantly decreased ruminal H2 load and further CH4 emissions, which is consistent with lower DM intake and nutrient digestibility. Therefore, milled rapeseeds and oats as regular dietary ingredients are an efficient means to soften milk fat and mitigate methane emissions at the whole-herd level.
The original contributions presented in the study are included in the article/Supplementary Material. Further inquiries can be directed to the corresponding author.
Ethical approval was not required for the study involving animals in accordance with the local legislation and institutional requirements because no prior authorization is required for projects that are likely to cause a level of harm lower than that caused by the introduction of a needle. Therefore, milk and fecal sampling carried out in this project did not require ethical approval according to national regulations (https://avi.fi/en/services/individuals/licences-notices-and-applications/animals/laboratory-animals).
AH-B-F: Conceptualization, Supervision, Writing – review & editing, Writing – original draft. SJ: Conceptualization, Writing – review & editing, Supervision. TK: Conceptualization, Supervision, Writing – review & editing. AT: Writing – original draft, Writing – review & editing. DG: Conceptualization, Funding acquisition, Project administration, Writing – review & editing. AV: Conceptualization, Funding acquisition, Project administration, Writing – review & editing.
The author(s) declare financial support was received for the research, authorship, and/or publication of this article. This study was financed in part by EIT Food Project: Dairy products with reduced saturated fatty acids (grant number 18095).
The authors thank the contribution of the staff at the University of Helsinki Viikki research farm and the laboratory of Animal Science. The valued contributions of MSc students Milja Korjus, Kasperi Ojala, Vappu Tauriainen, and Tuire Tapola for practical experimental work at the dairy barn are much appreciated.
Author AT was employed by the company Valio Ltd.
The remaining authors declare that the research was conducted in the absence of any commercial or financial relationships that could be construed as a potential conflict of interest.
All claims expressed in this article are solely those of the authors and do not necessarily represent those of their affiliated organizations, or those of the publisher, the editors and the reviewers. Any product that may be evaluated in this article, or claim that may be made by its manufacturer, is not guaranteed or endorsed by the publisher.
The Supplementary Material for this article can be found online at: https://www.frontiersin.org/articles/10.3389/fanim.2023.1278495/full#supplementary-material
Asioli D., Zhou X., Halmemies-Beauchet-Filleau A., Vanhatalo A., Givens D. I., Rondoni A., et al. (2023). Consumers’ valuation for low-carbon emission and low–saturated fat butter. Food Qual. Pref. 108, 104859. doi: 10.1016/j.foodqual.2023.104859
Bayat A. R., Tapio I., Vilkki J., Shingfield K. J., Leskinen H. (2018). Plant oil supplements reduce methane emissions and improve milk fatty acid composition in dairy cows fed grass silage-based diets without affecting milk yield. J. Dairy Sci. 101, 1136–1151. doi: 10.3168/jds.2017-13545
Beauchemin K. A., Ungerfeld E. M., Abdalla A. L., Alvarez C., Arndt C., Becquet P., et al. (2022). Invited review: Current enteric methane mitigation options. J. Dairy Sci. 105, 9297–9326. doi: 10.3168/jds.2022-22091
Benchaar C., Hassanat F., Martineau R., Gervais R. (2015). Linseed oil supplementation to dairy cows fed diets based on red clover silage or corn silage: Effects on methane production, rumen fermentation, nutrient digestibility, N balance, and milk production. J. Dairy Sci. 98, 7993–8008. doi: 10.3168/jds.2015-9398
Brask M., Lund P., Hellwing A. L. F., Poulsen M., Weisbjerg M. R. (2013a). Enteric methane production, digestibility and rumen fermentation in dairy cows fed different forages with and without rapeseed fat supplementation. Anim. Feed Sci. Technol. 184, 67–79. doi: 10.1016/j.anifeedsci.2013.06.006
Brask M., Lund P., Weisbjerg M. R., Hellwing A. L. F., Poulsen M., Larsen M. K., et al. (2013b). Methane production and digestion of different physical forms of rapeseed as fat supplements in dairy cows. J. Dairy Sci. 96, 2356–2365. doi: 10.3168/jds.2011-5239
Briggs M. A., Petersen K. S., Kris-Etherton. P. M. (2017). Saturated fatty acids and cardiovascular disease: Replacements for saturated fat to reduce cardiovascular risk. Healthc. (Basel) 5, 29. doi: 10.3390/healthcare5020029
Chen S., Bobe G., Zimmerman S., Hammond E. G., Luhman C. M., Boylston T. D., et al. (2004). Physical and sensory properties of dairy products from cows with various milk fatty acid compositions. J. Agric. Food Chem. 52, 3422–3428. doi: 10.1021/jf035193z
Clifton P. M., Keogh J. B. (2017). A systematic review of the effect of dietary saturated and polyunsaturated fat on heart disease. Nutr. Metab. Cardiovasc. Dis. 27, 1060–1080. doi: 10.1016/j.numecd.2017.10.010
Collomb M., Sollberger H., Bütikofer U., Sieber R., Stoll W., Schaeren W. (2004). Impact of a basal diet of hay and fodder beet supplemented with rapeseed, linseed and sunflowerseed on the fatty acid composition of milk fat. Int. Dairy J. 14, 549–559. doi: 10.1016/j.idairyj.2003.11.004
Drackley J. K., Overton T. R., Ortiz-Gonzalez G., Beaulieu A. D., Barbano D. M., Lynch J. M., et al. (2007). Responses to increasing amounts of high-oleic sunflower fatty acids infused into the abomasum of lactating dairy cows. J. Dairy Sci. 90, 5165–5175. doi: 10.3168/jds.2007-0122
Eurostat (2021). Available at: https://ec.europa.eu/eurostat (Accessed June 27, 2023).
Fant P., Leskinen H., Ramin M., Huhtanen P. (2023). Effects of replacement of barley with oats on milk fatty acid composition in dairy cows fed grass silage-based diets. J. Dairy Sci. 106, 2347–2360. doi: 10.3168/jds.2022-22327
Fant P., Ramin M., Huhtanen P. (2021). Replacement of barley with oats and dehulled oats: Effects on milk production, enteric methane emissions, and energy utilization in dairy cows fed a grass silage-based diet. J. Dairy Sci. 104, 12540–12552. doi: 10.3168/jds.2021-20409
Ferlay A., Chabrot J., Elmeddah Y., Doreau M. (1993). Ruminal lipid balance and intestinal digestion by dairy cows fed calcium salts of rapeseed oil fatty acids or rapeseed oil. J. Anim. Sci. 71, 2237–2245. doi: 10.2527/1993.7182237x
Field C. J., Blewett H. H., Proctor S., Vine D. (2009). Human health benefits of vaccenic acid. Appl. Physiol. Nutr. Metab. 34, 979–991. doi: 10.1139/H09-079
Givens D. I. (2023). Dairy foods and cardiometabolic diseases: an update and a reassessment of the impact of saturated fatty acids. Proc. Nutr. Soc 82, 320–345. doi: 10.1017/S0029665123000083
Glasser F., Doreau M., Maxin G., Baumont R. (2013). Fat and fatty acid content and composition of forages: A meta-analysis. Anim. Feed Sci. Technol. 185, 19–34. doi: 10.1016/j.anifeedsci.2013.06.010
Glasser F., Ferlay A., Chilliard Y. (2008). Oilseed lipid supplements and fatty acid composition of cow milk: a meta-analysis. J. Dairy Sci. 91, 4687–4703. doi: 10.3168/jds.2008-0987
Gross J., van Dorland H. A., Bruckmaier R. M., Schwarz F. J. (2011). Milk fatty acid profile related to energy balance in dairy cows. J. Dairy Res. 78, 479–488. doi: 10.1017/S0022029911000550
Halmemies-Beauchet-Filleau A., Kairenius P., Ahvenjärvi S., Toivonen V., Huhtanen P., Vanhatalo A., et al. (2013). Effect of forage conservation method on plasma lipids, mammary lipogenesis, and milk fatty acid composition in lactating cows fed diets containing a 60: 40 forage-to-concentrate ratio. J. Dairy Sci. 96, 5267–5289. doi: 10.3168/jds.2013-6571
Halmemies-Beauchet-Filleau A., Kokkonen T., Lampi A. M., Toivonen V., Shingfield K. J., Vanhatalo A. (2011). Effect of plant oils and camelina expeller on milk fatty acid composition in lactating cows fed diets based on red clover silage. J. Dairy Sci. 94, 4413–4430. doi: 10.3168/jds.2010-3885
Halmemies-Beauchet-Filleau A., Shingfield K. J., Simpura I., Kokkonen T., Jaakkola S., Toivonen V., et al. (2017). Effect of incremental amounts of camelina oil on milk fatty acid composition in lactating cows fed diets based on a mixture of grass and red clover silage and concentrates containing camelina expeller. J. Dairy Sci. 100, 305–324. doi: 10.3168/jds.2016-11438
Hillbrick G., Augustin M. A. (2002). Milkfat characteristics and functionality: opportunities for improvement. Aust. J. Dairy Tech. 57, 45–51.
Hristov A. N., Melgar A., Wasson D., Arndt C. (2022). Symposium review: Effective nutritional strategies to mitigate enteric methane in dairy cattle. J. Dairy Sci. 105, 8543–8557. doi: 10.3168/jds.2021-21398
Huhtanen P., Cabezas-Garcia E. H., Utsumi S., Zimmerman S. (2015). Comparison of methods to determine methane emissions from dairy cows in farm conditions. J. Dairy Sci. 98, 3394–3409. doi: 10.3168/jds.2014-9118
Huhtanen P., Rinne M., Nousiainen J. (2008). Evaluation of concentrate factors affecting silage intake of dairy cows: a development of the relative total diet intake index. Animal 2, 942–953. doi: 10.1017/S1751731108001924
Huida L., Väätäinen H., Lampila M. (1986). Comparison of dry matter contents in grass silages as determined by oven drying and gas chromatographic water analysis. Ann. Agric. Fenn. 25, 215–230. http://urn.fi/URN:NBN:fi-fe2014102145444.
Jenkins T. C. (1993). Lipid metabolism in the rumen. J. Dairy Sci. 76, 3851–3863. doi: 10.3168/jds.S0022-0302(93)77727-9
Jorjong S., Van Knegsel A. T. M., Verwaeren J., Lahoz M. V., Bruckmaier R. M., De Baets B., et al. (2014). Milk fatty acids as possible biomarkers to early diagnose elevated concentrations of blood plasma nonesterified fatty acids in dairy cows. J. Dairy Sci. 97, 7054–7064. doi: 10.3168/jds.2014-8039
Kairenius P., Toivonen V., Ahvenjärvi S., Vanhatalo A., Givens D. I., Shingfield K. J. (2009). “Effects of rapeseed lipids in the diet on ruminal lipid metabolism and milk fatty acid composition in cows fed grass silage-based diets. Abstract,” in Ruminant Physiology: Digestion, Metabolism and Effects of Nutrition on Reproduction and Welfare. Proc. XI Int. Symp. Rum. Phys. (Wageningen, The Netherlands: Wageningen Academic Publishers).
Kalač P., Samková E. (2010). The effects of feeding various forages on fatty acid composition of bovine milk fat: A review. Czech J. Anim. Sci. 55, 521–537. doi: 10.17221/2485-CJAS
Kennelly J. J. (1996). The fatty acid composition of milk fat as influenced by feeding oilseeds. Anim. Feed Sci. Technol. 60, 137–152. doi: 10.1016/0377-8401(96)00973-X
Kliem K., Humphries D., Markey O., Vasilopoulou D., Fagan C., Grandison A., et al. (2019). Food chain approach to lowering the saturated fat of milk and dairy products. Int. J. Dairy Technol. 72, 100–109. doi: 10.1111/1471-0307.12564
Kliem K. E., Shingfield K. J. (2016). Manipulation of milk fatty acid composition in lactating cows: Opportunities and challenges. Eur. J. Lipid Sci. Technol. 118, 1661–1683. doi: 10.1002/ejlt.201400543
Koba K., Yanagita T. (2014). Health benefits of conjugated linoleic acid (CLA). Obes. Res. Clin. Pract. 8, e525–e532. doi: 10.1016/j.orcp.2013.10.001
Lamminen M., Halmemies-Beauchet-Filleau A., Kokkonen T., Jaakkola S., Vanhatalo A. (2019). Different microalgae species as a substitutive protein feed for soya bean meal in grass silage based dairy cow diets. Anim. Feed Sci. Technol. 247, 112–126. doi: 10.1016/j.anifeedsci.2018.11.005
Livingstone K. M., Lovegrove J. A., Givens D. I. (2012). The impact of substituting SFA in dairy products with MUFA or PUFA on CVD risk: evidence from human intervention studies. Nutr. Res. Rev. 25, 193–206. doi: 10.1017/S095442241200011X
Luke (2023) Finnish feed tables and nutrient requirements of farm animals. Available at: https://www.luke.fi/en/luonnonvaratieto/science-and-information/feed-tables-and-nutrient-requirements (Accessed June 27, 2023).
Mierlita D., Santa A., Mierlita S., Daraban S. V., Suteu M., Pop I. M., et al. (2023). The effects of feeding milled rapeseed seeds with different forage: concentrate ratios in Jersey dairy cows on milk production, milk fatty acid composition, and milk antioxidant capacity. Life 13, 46. doi: 10.3390/life13010046
Min B. R., Lee S., Jung H., Miller D. N., Chen R. (2022). Enteric methane emissions and animal performance in dairy and beef cattle production: Strategies, opportunities, and impact of reducing emissions. Animals 12, 948. doi: 10.3390/ani12080948
Perna M., Hewlings S. (2023). Saturated fatty acid chain length and risk of cardiovascular disease: A systematic review. Nutrients 15, 30. doi: 10.3390/nu15010030
Pitkänen O., Halmemies-Beauchet-Filleau A., Räisänen S. E., Jaakkola S., Kokkonen T., Vanhatalo A. (2023). Processed fava bean as a substitute for rapeseed meal with or without rumen-protected methionine supplement in grass silage-based dairy cow diets. J. Dairy Sci. 106, 3217–3232. doi: 10.3168/jds.2022-22897
Ramin M., Chagas J. C., Smidt H., Exposito R. G., Krizsan S. J. (2021a). Enteric and fecal methane emissions from dairy cows fed grass or corn silage diets supplemented with rapeseed oil. Animals 11, 1322. doi: 10.3390/ani11051322
Ramin M., Fant P., Huhtanen P. (2021b). The effects of gradual replacement of barley with oats on enteric methane emissions, rumen fermentation, milk production, and energy utilization in dairy cows. J. Dairy Sci. 104, 5617–5630. doi: 10.3168/jds.2020-19644
Razzaghi A., Leskinen H., Ahvenjärvi S., Aro H., Bayat A. R. (2022). Energy utilization and milk fat responses to rapeseed oil when fed to lactating dairy cows receiving different dietary forage to concentrate ratio. Anim. Feed Sci. Technol. 293, 115454. doi: 10.1016/j.anifeedsci.2022.115454
Rego O. A., Alves S. P., Antunes L. M. S., Rosa H. J. D., Alfaia C. F. M., Prates J. A. M., et al. (2009). Rumen biohydrogenation-derived fatty acids in milk fat from grazing dairy cows supplemented with rapeseed, sunflower, or linseed oils. J. Dairy Sci. 92, 4530–4540. doi: 10.3168/jds.2009-2060
Ryhänen E. L., Tallavaara K., Griinari J. M., Jaakkola S., Mantere-Alhonen S., Shingfield K. J. (2005). Production of conjugated linoleic acid enriched milk and dairy products from cows receiving grass silage supplemented with a cereal-based concentrate containing rapeseed oil. Int. Dairy J. 15, 207–217. doi: 10.1016/j.idairyj.2004.07.003
Shingfield K. J., Bernard L., Leroux C., Chilliard Y. (2010). Role of trans fatty acids in the nutritional regulation of mammary lipogenesis in ruminants. Animal 4, 1140–1166. doi: 10.1017/S1751731110000510
Vanhatalo A., Halmemies-Beauchet-Filleau A. (2020). “Optimising ruminal function: the role of silage and concentrate in dairy cow nutrition to improve feed efficiency and reduce methane and nitrogen emissions,” in Improving rumen function. Eds. McSweeney C. S., Mackie R. I. (Cambridge, United Kingdom: Burleigh Dodds Science Publishing), 651–692.
Vanhatalo A., Gäddnäs T., Heikkilä T. (2006). Microbial protein synthesis, digestion and lactation responses of cows to grass or grass-red clover silage diet supplemented with barley or oats. Agric. Food Sci. 15, 252–267. doi: 10.2137/145960606779216236
Van Keulen J., Young B. A. (1977). Evaluation of insoluble ash as a natural marker in ruminant digestibility studies. J. Anim. Sci. 44, 282–287. doi: 10.2527/jas1977.442282x
Van Soest P. V., Robertson J. B., Lewis B. A. (1991). Methods for dietary fiber, neutral detergent fiber, and nonstarch polysaccharides in relation to animal nutrition. J. Dairy Sci. 74, 3583–3597. doi: 10.3168/jds.S0022-0302(91)78551-2
Vasilopoulou D., Markey O., Kliem K. E., Fagan C. C., Grandison A. S., Humphries D. J., et al. (2020). Reformulation initiative for partial replacement of saturated with unsaturated fats in dairy foods attenuates the increase in low-density lipoprotein cholesterol and improves flow-mediated dilatation compared with conventional dairy: the randomized, controlled RESET study. Am. J. Clin. Nutr. 111, 739–748. doi: 10.1093/ajcn/nqz344
Keywords: plant lipid, grass silage, milk fat, saturated fatty acid, trans fatty acid, organoleptic quality, methane, hydrogen
Citation: Halmemies-Beauchet-Filleau A, Jaakkola S, Kokkonen T, Turpeinen AM, Givens DI and Vanhatalo A (2023) Milled rapeseeds and oats decrease milk saturated fatty acids and ruminal methane emissions in dairy cows without changes in product sensory quality. Front. Anim. Sci. 4:1278495. doi: 10.3389/fanim.2023.1278495
Received: 16 August 2023; Accepted: 16 October 2023;
Published: 02 November 2023.
Edited by:
Virginia C. Resconi, University of Zaragoza, SpainReviewed by:
Paolo Silacci, Agroscope, SwitzerlandCopyright © 2023 Halmemies-Beauchet-Filleau, Jaakkola, Kokkonen, Turpeinen, Givens and Vanhatalo. This is an open-access article distributed under the terms of the Creative Commons Attribution License (CC BY). The use, distribution or reproduction in other forums is permitted, provided the original author(s) and the copyright owner(s) are credited and that the original publication in this journal is cited, in accordance with accepted academic practice. No use, distribution or reproduction is permitted which does not comply with these terms.
*Correspondence: Aila Vanhatalo, YWlsYS52YW5oYXRhbG9AaGVsc2lua2kuZmk=
Disclaimer: All claims expressed in this article are solely those of the authors and do not necessarily represent those of their affiliated organizations, or those of the publisher, the editors and the reviewers. Any product that may be evaluated in this article or claim that may be made by its manufacturer is not guaranteed or endorsed by the publisher.
Research integrity at Frontiers
Learn more about the work of our research integrity team to safeguard the quality of each article we publish.