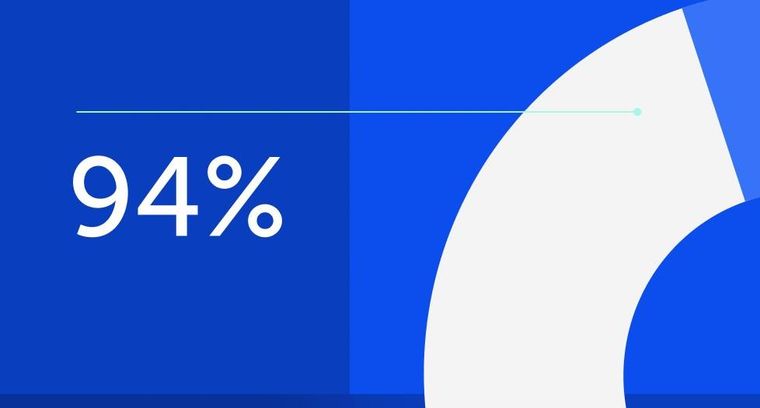
94% of researchers rate our articles as excellent or good
Learn more about the work of our research integrity team to safeguard the quality of each article we publish.
Find out more
ORIGINAL RESEARCH article
Front. Anim. Sci., 11 December 2023
Sec. Animal Welfare and Policy
Volume 4 - 2023 | https://doi.org/10.3389/fanim.2023.1258935
The widespread adoption of advanced technology, like automatic milking systems in the United States, allows cows to establish individual milking, feeding, and resting schedules, setting them apart from others. However, it is unknown how cow parity affects cow behavior, stress, and system efficiency. We hypothesized primiparous (PR) cows would spend more time in and around the milking robot (AMS), receive greater agonistic interactions, and show elevated physiological stress behaviors compared to multiparous (MU) cows. The study aimed to evaluate the impact of cow parity on behavior and welfare near and inside the AMS. Twenty-four lactating-Holstein dairy cows [12 primiparous (3.0 ± 0.2 yr) and 12 multiparous (6.1 ± 1.9 yr)] housed in the same pen at a guided traffic AMS facility were marked and observed for 6-consecutive days (91 to 102 of lactation). Study cows were identified by specific colored-paint markings, their milk yield and visits, their behavior, Heart Rate-Variability (HRV), and activity were recorded inside the commitment pen (CP) and the AMS. Statistical calculations were performed using JMP Pro 16.1.0, and P ≤ 0.05 was considered significant. Multiparous cows produced more daily milk yield than PP cows (47.30 Vs 33.79 kg), while parity showed no effect on daily milking frequency or milk yield per visit. Primiparous cows spent significantly more time inside the CP than MP cows (68.87 vs 24.38 m), while MP cows approached the AMS entry more often than the PR cows (4.83 vs 2.03), MU cows displaced other cows more inside the CP than PR cows (6.90 vs 2.59). PR cows showed lower HRV (RMSSD: 9.23 vs 17.58 ms) and (SDRR: 19.58 vs 33.64 ms) values than MU cows, whereas MU cows showed a lower Low-Frequency to High-Frequency Ratio (4.39 vs 8.65) than PR cows inside the CP. MU cows spent more time (m) lying (698.06), particularly at night (396.57), than PP cows (556.96, 286.68), while MP cows exhibited more prolonged total lying bouts than PP cows (93.06 vs 71.32 m). Overall, cow parity influenced behavior, activity, and stress indicators of primiparous more than multiparous cows and impacted the overall efficiency and success of the AMS
In recent years, the dairy industry has experienced an increase in the implementation of automated technologies (Cogato et al., 2021). Automatic milking systems (AMS) are one of the most significant technological advances in the current dairy industry. AMS relies on cows voluntarily entering the milking stalls as individuals apart from the herd without the guidance of farm staff (Salfer et al., 2018). As a result, it is critical to understand the interactions between cows and their environment that influence behavior and movement through the AMS facility to achieve system success (Jacobs et al., 2012: Siewert et al., 2019). Motivation to enter the milking stall and negative social interactions can hinder cow welfare and the efficiency of AMS farms (Jacobs and Siegford, 2012). Negative interactions in AMS facilities can increase standing time, decrease lying time, decrease rumination rate, and increase agonistic behaviors (Fadul-Pacheco et al., 2021), thus potentially causing milk production complications resulting from competition for food, lying space, and access to AMS milking robots (Bøe and Færevik, 2003; von Keyserlingk et al., 2008; Fadul-Pacheco et al., 2021).
Facility design dictates cow traffic throughout the AMS facility and can influence cows’ daily milking visits, resting, and feeding schedules (Jacobs and Siegford, 2012). Some researchers have identified the importance of waiting areas outside AMS stalls to reduce social competition to enter the milking system (Hermans et al., 2003; Melin et al., 2006; Rodenburg and House, 2007; Jacobs et al., 2012). Commitment pens (CP) are gated areas in front of milking stalls designed for cows to wait to enter the milking stalls on their own accord but cannot leave until milking is complete (Jacobs et al., 2012; Rodenburg, 2017).
Farmers with AMS have expressed that parity has an impact on AMS efficiency (Siewert et al., 2019). Research has shown differences in trends between primiparous and multiparous cows across different traffic flow systems, which subsequently impact milk production. For example, in guided-flow systems, primiparous cows yielded less milk compared to their multiparous counterparts (Spolders et al., 2004; Siewert et al., 2019). Cow parity can also influence the expression of agonistic behaviors, like displacement, blocking, and hesitation, causing stress prior to entering the milking robots (Jacobs et al., 2012; Lobeck-Luchterhand et al., 2014). Based on these findings, behaviors expressed inside the CP may differ across parity, potentially impacting long-term behavior, welfare, and milk production.
Thus, the objective of this study was to evaluate the impact of cow parity on behavior and welfare near and inside the AMS. We hypothesized primiparous (PR) cows would spend more time in and around the AMS, receive greater agonistic interactions, and show elevated stress behaviors compared to multiparous (MU) cows around and inside the AMS in a guided traffic barn.
Prior to the start of the study, all protocols were submitted to and approved by the Clemson University Institutional Animal Care and Use Committee (AUP#2021-0064).
Twenty-four lactating Holstein dairy cows were selected for the current study from a larger herd (120 lactating dairy cows) housed in the LaMaster Dairy Farm AMS facility at Clemson, SC, USA. Cows were enrolled in the study when they reached day 97 of lactation and continued for 6 consecutive days until day 102 of lactation. Cows were divided into 2 groups (n = 12/group) balanced for the stage in lactation, with each group having access to two AMSs. Group A consisted of 12 primiparous (PR) cows (mean age 3.0 ± 0.2 yr), and group B contained 12 multiparous (MU) cows (mean age 6.1 ± 1.9 yr and parity 4.1 ± 1.9). The cows were selected based on the expectation that they would be lactating for the full duration of the trial, and were allowed 6 milking permissions per day.
The AMS entrance and exit gate design for cow traffic flow was the same between the groups. Both AMS were left-handed milking robots, forcing clockwise cow traffic. The Holding area (HA) had an automatic selection gate that enabled access to the CP of the AMS. Gates only opened to a commitment pen (CP) if cows were granted permission based on their last milking time, and exit gates only opened toward the feed alley if the milking was complete. There were two selection gates, one granting access to the holding area (the area next to the CP) and one for exiting the CP post milking. All gates and alleys were of equal lengths for both groups.
The AMS (Delaval VMS V300), feeding alley, stall layouts, and management practices were identical for both groups. The AMS was operational 24/7 except for the extent of 3 20-minute robot cleaning cycles (0400, 1230, and 2000 h). Feeding of a PMR occurred twice daily (0730 and 1530 h) and diet feed and chemical composition and feeding schedule were identical for both groups. Cows were offered a concentrate pellet during the milking process; the amount of concentrate was dependent upon the individual projected milk yield. Footbaths were present in the CP exit alley containing a 5% copper sulfate solution.
Six Wyze Cam v3 cameras (Wyze labs, Inc, Seattle, USA) recorded behavior for each group 24 h/d for 6 consecutive days. Cameras were focused on the CP in front of the AMS, CP entry gate, the AMS itself, and the AMS exit and entrance gates. Focal cows were identified on video by specific colored paint markings using livestock paint (LA-CO Industries, Inc, IL, USA) across their back. Parity was identified by colored livestock paint down the spine from the shoulder to the tail head. Primiparous cows were marked with red livestock spray paint, and multiparous cows were marked with blue livestock paint. Focal cows were equipped with a Polar H10 heart rate sensor (Polar Electro Europe BV, Fleurier, Switzerland), a data transmission watch, and a triaxial accelerometer (Onset HOBO PendantG acceleration data loggers) to record Heart Rate Variability HRV (Hopster and Blokhuis, 1994) and activity data (Wolfger et al., 2015). The number of daily milking visits, total milk per visit, and daily milk yield (kg) were recorded for each of the focal cows.
Individual cows were identified on video by the specific paint-colored markings and cow behavior within the commitment pen, and AMS was recorded.
In the Commitment pen, the time taken for focal cows to enter the CP from the holding area (i.e., Entry duration; duration of time from the start of selection gate opening to the time of gate closing behind the focal cow) was recorded for each milking visit. Behavioral observations were recorded throughout the duration of time spent inside the CP before entering the milking robot. The observation began after the entry selection gate closed behind the cow, and the time of entry was recorded. All behaviors (i.e., exploration, displacement, idling, tail swishing, etc.) were recorded for each cow, and the quantity and duration of each behavior was noted. The number of displacement events inside the CP was recorded for focal cows. Displacement is defined as an interaction between 2 cows when 1 cow initiates physical contact (actor), causing another cow (reactor) to back out or leave the AMS entry alley, therefore, losing their place in line to get milked (Witaifi et al., 2018). Once cows entered the milking robot, the total time inside the CP was recorded. All behaviors observed and recorded are described in an ethogram of CP behaviors (Table 1.1).
In the AMS, cameras were located outside each milking stall, focused on the cow’s hindlegs. Behavioral observations while inside the milking stall (i.e., stepping, kicking, hoof lifting, idling, etc.) were recorded for focal cows. The duration of each successful milking event, frequency of each behavior, and total milk yield per visit were recorded. Durations of each event were summed to create the total duration for each event type for each 24-h period for 6d. The time taken for the cow to exit the AMS milking stall [i.e., duration of time from the start of AMS exit gate opening to the time of exit gate closing behind the focal cow (Jacobs and Siegford, 2012)] was also recorded. All behaviors observed and recorded are described in an ethogram of AMS behaviors (Table 1.2).
A Polar heart rate monitor was used for recording heart rate (HR) and HRV parameters. The monitor consisted of an electrode chest belt with a built-in Polar H10 HR sensor secured around the thorax of focal cows. The belt was fixed with the HR sensor located proximal to the elbow on the left side of the body. Electrodes were moistened with a non-spermicidal, multi-purpose lubricant to augment contact between electrode and skin to increase conductivity and enhance data transmission of the device. A wristwatch receiver was linked to individual HR sensors and attached to the cow’s collar. Belts and watches were fixed on focal cows 12 hours prior to observations to allow for habituation. HRV data collection started at 0000 h on the second day and through the 6th day of observation.
The HRV monitor recorded changes in electrical potential to detect maximum, minimum, and average HR, and beat-to-beat (RR) intervals. Data were transmitted from HR sensor transmitter to wristwatch receivers that received and sorted the data. This data were downloaded onto a personal computer via a polar interface (Polar ProTrainer Equine edition) for statistical analysis. Kubios HRV software, version 2.2 (Biomedical Signal Analysis Group, Department of Applied Physics, University of Kuopio, Finland, 2014), was used to analyze HRV data. Data were detrended to remove long-term trend components, and artifact correction was made following procedures established by Schmidt et al. (2010). The most informative time and frequency domain measures were obtained for the analysis of HRV in the current study.
HR measured in beats per minute (BPM) represents the average heart rate per minute throughout the recorded time period. Heart rate variability is defined as irregular time intervals between successive heart beats (Moss, 1995). The variation between heart beats is a result of rhythmic oscillation of the components that regulates cardiac activity. Akselrod (1995) and Cerutti et al. (1995) reported the function of rhythmic oscillation is to maintain cardiovascular homeostasis within a defined range and coordinate responses to challenges. HRV describes the antagonistic influences of both the sympathetic and vagal branches of the Autonomic Nervous System (ANS) on the sinus node of the heart. In the current study, HRV variables, such as the root mean square of successive beat-to-beat intervals (RMSSD) and the standard deviation of the beat-to-beat interval (SDRR), were calculated by determining the difference between consecutive inter-beat intervals before squaring and summing them, and these values were averaged, and square root was obtained (Von Borell et al., 2007; Ali et al., 2017). Therefore, a decrease in HRV values indicates a shift toward sympathetic dominance, indicating a stress response, whereas increased HRV values indicate parasympathetic dominance or a resting state (Mestivier et al., 1997).
Moreover, HRV data were also analyzed using the autoregressive model of power spectral analysis (as described by Bernasconi et al., 1998), estimating the spectral distribution of intervals between heartbeats. Generally, there are two major peaks: the first one is the low frequency (LF) component attributed to the sympathetic activity, and the other one is known as the high frequency (HF) component that reflects the vagal activity (Pagani et al., 1986; Akselrod, 1995; Bernasconi et al., 1998). Therefore, the LF/HF ratio resembles the sympathovagal balance (SVB) (Pagani et al., 1986; Akselrod, 1995; Bernasconi et al., 1998). In the current study, HR and HRV data were labeled and averaged for each cow based on location (inside AMS and inside the CP).
An acceleration data logger (Onset HOBO PendantG acceleration data loggers, Onset Computer Corporation, Bourne, MA) was firmly attached to the HRV chest belt on the right dorsal side near the withers to prevent changes in logger orientation. Activity data were recorded starting at 2400 h on 1d of observation through the 6d of observation. The loggers were attached at the same time as the HRV chest belts were fixed on the focal cows. The accelerators were oriented so that the Y-axis captured sideways movement, the X-axis captured forward and the backward movement, and Z-axis captured vertical movement. Various daily activity variables were recorded and split by daytime hours (0630-1830 h), nighttime hours (1831-0629 h), and total activity/day. We documented cow lying time (min), lying frequency, duration of lying bouts (min), idle time (min), and acceleration activity (g) for each cow.
The raw accelerometer data, consisting of the date, time, and the related impulse in the X, Y, and Z dimensions, were downloaded from the devices (HOBOware Graphing & Analysis Software, Bourne, MA) at the end of each 6-day observation period. Data on cows’ vertical (az: dorsoventral movement across vertical levels), horizontal (ax: craniocaudal movement within the same vertical level), and lateral movement (ay: mediolateral movement within the same vertical level), during light hours were obtained directly from loggers. Cows’ total-triaxial activity (As) was calculated by summing and averaging raw movement data using the following formula.
Acceleration data were post-processed using MATLAB (MATLAB and Statistics Toolbox Release 2012, The MathWorks, Inc., Natick, MA). To accurately calculate lying time (min), lying frequency, duration of lying bouts (min), idle time (min); the data (az: dorsoventral movement across vertical levels) from the observational period were smoothed from noisy components by removing all minor acceleration fluctuations using a loop function. Data smoothing included passing of the raw acceleration values (Aj) through an asymmetrical 3 point-moving average low-pass filter (i = the middle point in the 3 point-moving average low pass filter) and through a step function to define thresholds used to remove minor fluctuations (t = threshold values of minor fluctuations, i.e., between 0.001 and 0.043 g). After processing the data, lying events were recognized by detecting massive shifts in acceleration in the z-axis of activity. In order to precisely detect acceleration shifts due to lying and define thresholds for minor fluctuations in the z axis, timestamped videos of cows while lying were obtained and compared with the corresponding activity data. Using this approach enabled us to define shifts in z axis acceleration caused by lying down, and remaining non-lying fluctuations were used to define threshold levels for data smoothing as shown in the following equations.
All statistical calculations for milk yield, AMS behavior, CP behavior, and cow activity and HRV were preformed using JMP Pro 16.1.0, and P ≤ 0.05 was considered evidence of statistical significance. Evaluation of data with a Shapiro-Wilk’s test (P>0.05) and a visual inspection of histograms revealed that data from all measurements were normally distributed. To describe the influence of parity on the response variables of interest (milk production, AMS behaviors, CP behaviors, HRV, and activity) during the six days of observations, general linear mixed models were developed that related the response variables (i.e., daily milk yield, idle, laying time, etc.), to the fixed effect of parity and the random effects of day of observation and individual cow. If parity was found to have significant effects on the response variables, the nature of the effects was further studied using T-tests (i.e., Fisher’s Protected Least Significant Difference Test). Since more than one observer contributed to data collection, inter-observer reliability with the primary observer was calculated using Cohen’s kappa Agreement coefficient (K), following Landis and Koch (1977). A power analysis was executed, employing CP time as a determinant factor to identify a medium effect size (Cohen’s d = 0.5) with a two-tailed alpha level set at 0.05 and a desired power of 0.80. The analysis revealed that to attain adequate statistical power for the variable of interest in our study, a minimum sample size of 10 cows would be necessary. In anticipation of potential attrition or data loss, we opted to enlist 12 cows for each group, thereby ensuring that our study maintains a robust sample size capable of detecting meaningful effects. Inter-observer reliability was measured during the observer-training period before data collection occurred when trainees simultaneously observed the same videos. Inter-observer agreement was considered good [Kappa = 0.96 (P< 0.001), CI (0.90, 0.99)].
Multiparous cows produced more daily milk yield than PP cows (P = 0.021), while parity showed no effect on daily milking frequency or milk yield per visit (Table 2).
Primiparous cows spent significantly more time inside the CP than MP cows (P = 0.001; Table 3), while MP cows approached the AMS milking robot more often than the PP cows (P = 0.030). Tail-swishing was observed more frequently in MP cows than PP cows (P = 0.040), while PP cows idled more inside AMS and CP than MP cows (P = 0.001). MP cows displaced other cows (Displacement-actor; DP-A) more inside the CP than PP cows (P = 0.032; Table 3). However, parity did not significantly influence CP entry duration or exploratory behaviors.
Multiparous cows spent more time inside the milking robot (P = 0.023; Table 4) and stepped more often during the milking process (P = 0.013) than PP cows. On the contrary, PP cows took significantly more time to leave the milking stall after milking than MP cows (P = 0.002). However, parity did not show a significant influence on kick frequency or idle time inside the AMS milking robot (Table 4).
PP cows showed lower RMSSD (P = 0.023) and SDRR (P = 0.012; Table 5) values than MP cows, whereas MP cows showed a lower LF/HF ratio than PP cows inside the CP (P = 0.003). However, there was no significant difference in heart rate between PP and MP inside the CP (Table 5).
Table 5 Comparison of primiparous and multiparous cow HR and HRV parameters in the AMS commitment pen.
Table 6 showed that PP cows had lower RMSSD (P = 0.025) and SDRR (P = 0.019) values inside the AMS milking robot than MP cows. Parity also significantly influenced the LF/HF ratio, as PP cows had higher LF/HF ratios than MP cows (P = 0.018). However, parity did not impact HR values in cows inside the AMS milking robot (Table 6).
Table 6 Comparison of primiparous and multiparous cow HR and HRV parameters in the AMS milking robot.
Parity had a significant impact on total and nighttime lying time (Table 7.1), as MP cows spent more time lying (P = 0.003), particularly at night (P = 0.030) than PP cows. However, parity had no impact on lying time during daytime hours. PP cows laid down more frequently in total (P = 0.020) and during daytime hours (P = 0.040) than MP cows (Table 7.2). However, there was no parity impact on nighttime lying frequency (Table 7.2). MP cows exhibited longer lying bouts than PP cows (P = 0.010). However, parity showed no influence on the duration of lying bouts during daytime or nighttime (Table 7.3).
Parity significantly affected total and daytime idle time. PP cows were observed idling for longer durations than MP cows in both totals (P = 0.021) and daytime hours (P = 0.032; Table 8.1); however, no differences were recorded during the nighttime period. PP cows exhibited greater total (P = 0.031) and daytime (P = 0.041) acceleration activity than MP cows (Table 8.2). However, parity had no significant effect on cow nighttime acceleration activity.
The potential for increased milk production exists when using Automatic Milking Systems (AMS) compared to traditional parlor systems that milk cows twice daily (De Koning et al., 2002; Rotz et al., 2003; Wade et al., 2004). The number of milking events per cow in an AMS can vary, depending on the cow’s parity and, subsequently, its set milking intervals, motivation to access the milking stall, cow traffic, and the frequency of fetching overdue cows by farm staff. Efficient operation of the AMS is crucial to ensure that each cow achieves its designated number of daily milking events, maximizing milk production. It is important to consider cow’s parity, welfare, and behavioral needs, as factors that result in cows spending more time in or near the AMS may reduce their time at the feed bunk or lying in stalls (Uetake et al., 1997). Therefore, the aim of this study was to assess how cow parity affects behavior and welfare in the vicinity and within the Automatic Milking System.
It is well established that cows higher in parity produce more milk than cows of lower parity, but this can vary based on many factors, including but not limited to; facility traffic flow, type of milking system, and feed provided (Beauchemin and Rode, 1994; Deming et al., 2013; Neave et al., 2017; Siewert et al., 2019). In the current study, parity influenced daily milk yield, as MP cows produced more milk than PP cows during the observation period. Adrien et al. (2012) reported that total milk production at 60 DIM was greater in multiparous cows than primiparous cows. Siewert et al. (2019) also found that multiparous cows produced greater daily milk yield than primiparous cows within a similar DIM range (89-118 DIM) to the current study.
The current study showed that parity significantly impacted time spent inside the CP, AMS approach attempts, tail swishing, idle time, and displacement behavior. PP cows spent greater durations inside the CP prior to milking, and PP also stood idle significantly longer than MP cows in the CP. This is consistent with Solano et al. (2022), who reported that PP cows spent longer waiting times than MP cows before entering the milking stalls in early and mid-lactation and attributed these findings to the mixed grouping of PP and MP cows inside the CP, competing for AMS entry.
Similarly, Dijkstra et al. (2012) stated that submissive cows, like first lactation cows, are forced to spend greater time waiting to milk due to the dominant structure dairy cows have been known to express. Another study reported that lower-ranking cows generally had to wait longer in the CP than dominant cows before accessing the AMS (Halachmi, 2009). Primiparous cows are lower in social ranking than MP cows, which prevents them from entering the milking robot as quickly as other cows inside the CP. This is likely a stressful situation for PP cows as they are unable to feed, drink, or lie down in this area (Dijkstra et al., 2012; Norring et al., 2012). The lack of locomotion activity permitted in the restricted space of CPs accounts for our findings of longer idle times in PP cows inside the CP than MP cows. However, no previous research has investigated the influence of parity on cow idle time, specifically inside the CP. However, some studies have observed an effect of parity on idle time within other areas of the barn. Gomez and Cook (2010) reported reduced idle time in PP cows within free stalls, possibly related to social competition and increased feeding time. Thus, the influence of parity on idle time cows inside the CP waiting to milk should be further investigated in the future as it can influence daily feeding and resting activity and negatively impact milk production.
Parity also significantly influenced the number of AMS approach attempts, tail swishing, and displacement behavior in MP more than PP cows in the CP. The number of times the cows approached the AMS milking robot was recorded while confined in the CP, waiting to access the milking robot. This behavior was evaluated to assess the cow’s motivation to enter the milking robot. MP cows made more AMS approach attempts and entered through the gates faster than PP cows in this study, suggesting MP cows were more motivated to milk. If cows are highly motivated to approach the AMS, waiting times inside the CP could be reduced, thus preventing prolonged stress (Dijkstra et al., 2012; Norring et al., 2012) and potentially increasing milk production. However, various social interactions can also interfere with cow motivation to approach AMS milking stalls, like head-butting and displacement agonistic behaviors.
Time spent inside the CP can be influenced by factors like competition to enter milking stalls, leading to agonistic behaviors. Halachmi (2009) reported dominant cows would not wait in the pre-established milking queue, instead would displace lower-ranking cows until they reached the head of the line to milk. However, most research investigating parity influence on agonistic behavior has focused on displacement in feed bunk or free stall areas. Val-Laillet et al. (2009) reported restricted feed space resulted in significantly greater DP-A behavior in MP cows. Whereas Westin et al. (2016) discussed how social competition could also impact lying behavior, with lower-ranking cows receiving more displacements from lying stalls. Primiparous cows are typically socially inferior to MP dairy cows due to being smaller in size, younger, and unfamiliar with preestablished social groups in the facility (Nogues et al., 2020; Fadul-Pacheco et al., 2021). Thus, these reports support the current findings of MP cows displaying greater DP-A behavior in the AMS CP. The area outside AMS robots can cause substantial social competition as cows are competing to enter the milking stalls. Similarly, in the current study, we hypothesized to see MP cows displace lower-ranking cows, like PP cows, more frequently in the CP. This behavior was attributed to the higher dominance status of MP cows and their better familiarity with the milking process in the AMS robots.
Tail swishing is a natural cow behavior performed in situations that irritate the cows (Weary et al., 2011). In the current study, we observed tail swishing inside the CP as a sign of annoyance or irritation to other cows inside the CP while waiting to enter the milking stalls. However, previous research generally evaluated tail swishing as an annoyance to flies (Wehrend et al., 2006; Weary et al., 2011; Perttu et al., 2020). We found MP cows tail-swished more frequently than PP cows inside the CP, thus, suggesting MP cows were more irritated while waiting or competing to enter milking stalls. Although there is no previous research with similar findings as the current study, other studies have found increased tail-swishing could be a sign of cow agitation or a response to fear, stress, or painful stimuli (Eicher and Dailey, 2002; Willson et al., 2021).
MP cows were observed stepping more frequently and spending greater time milking inside AMS milking stalls than PP cows in the current study. We also found PP cows took significantly longer to exit the milking stalls post-milking than MP cows. Castro et al. (2012) reported the average time spent in the AMS was approximately 7 min per cow. Similar to current findings, Sitkowska et al. (2016) found MP cows spent greater time (383 s) than PP cows (361 s) in the milking robot. They also reported the average milking time accounted for 70% of MP cows and 66% PP cows (Sitkowska et al., 2016). Considering MP cows typically produce higher milk yield per visit than PP cows (Johnson et al., 2003), we would expect longer AMS times in MP cows (Kliś et al., 2021). We also observed greater stepping frequency in MP cows in the present study, which agrees with Gygax et al. (2008), who reported cows stepping in the AMS >95% of all milking events, and that the stepping rate increased in cows of higher parity. Although the milking process can be stressful, stepping behavior is considered a minor stress response in cows (Lupoli et al., 2001; Gygax et al., 2008), indicating that MP cows were more stressed than PP while milking in AMS. In the present study, PP cows took significantly longer to exit the milking robot than MP cows once the milking process was complete. This could be due to a multitude of reasons, including crowding at AMS exit gate, desire to receive more feed concentrate, or lack of understanding when milking in the novel AMS environment. While little research has been conducted investigating the effect of parity on AMS exit duration, Jacobs and Siegford (2012) reported cows were more likely to hesitate when other cows were near the exit gate. Conversely, Jacobs et al. (2012) found parity did not influence exit hesitation in their study. However, investigating possible influences of factors such as parity on AMS exit duration would be beneficial as occupying AMS for longer durations creates longer waiting times for other cows and can hinder maximum milk production, system efficiency, and cow welfare (Rodenburg, 2013).
The findings of the present study suggest that PP cows were more stressed while waiting to be milked in the CP. PP cows exhibited lower RMSSD and SDRR values and higher LF/HF ratios than MP cows. RMSSD accounts for short-term, high-frequency components of HRV, strongly reflects the vagal tone, and is highly correlated to LF/HF ratio (Kleiger et al., 1992; Hagen et al., 2005; Gygax et al., 2008). Lower RMSSD values indicate increased cow stress levels (Hagen et al., 2005; Von Borell et al., 2007; Buck et al., 2013). Although previous research has not investigated HRV parameters while cows were explicitly waiting in the CP, other studies have reported similar parity effects on HRV values. Kovács et al. (2013) reported that MP cows showed lower levels of stress than PP cows. Another study reported that SDRR tends to be lower in PP cows than in MP cows in all behavioral patterns (Buck et al., 2013). Such a lower HRV in PP cows suggests that these cows might not have enough training time or sufficient experience milking in AMS, resulting in greater stress responses while waiting in AMS CPs (Szentléleki et al., 2015). Although previous studies reported significant differences in HRV parameters when cows wait to milk and inside the AMS, for instance, Jurkovich et al. (2017) reported a higher sympathetic tone during periods before milking in AMS, suggesting cows were more stressed.
Parity significantly impacted multiple cow HRV parameters while milking in AMS. The current study found PP cows had lower RMSSD and SDRR values and higher LF/HF ratios, which suggests that PP cows were more stressed than MP cows inside the AMS. This is consistent with other research findings of PP cows being more susceptible to AMS technology than MP cows. For instance, Kovács et al. (2013) reported MP cows had higher SDRR values than PP cows, thus suggesting PP cows were more stressed while milking in AMS. Whereas Hagen and colleagues (2005) found the only factor that influenced HRV parameters while milking was cow body weight, thus, suggesting PP and MP cows would likely differ in stress during the milking process solely due to MP cows being larger in size. Moreover, mechanisms that cause such physiological stress should be further examined to control for cow-level effects of stress when milking in AMS (Frei et al., 2022).
Increased cow daily lying time is often associated with parity and stage in lactation (Vasseur et al., 2012; Westin et al., 2016). Westin et al. (2016) reported higher parity cows spent 0.5 h/d more lying time than PP cows. Norring and colleagues (2012) also found that daily lying time increased with cow age and parity. However, other researchers reported a weak correlation between parity and daily lying time (Charlton et al., 2016). Our results partially agree with the previous research findings, as MP cows spent more time lying down in total, particularly during nighttime hours (1831 – 0629h), than PP cows. A possible explanation could be that cows in AMS farms spend considerable amounts of time waiting in the CP to milk, thus, limiting the available time to lay down, especially for PP or lower-ranking cows (Melin et al., 2006; Halachmi, 2009; Westin et al., 2016). Moreover, MP cows in the current study spent less time waiting to enter the milking stalls, which may allow them more time to rest longer than PP cows.
In the present study, PP cows performed higher daily and daytime lying frequencies than MP cows. Charlton et al. (2016) reported moderate correlations between parity and lying bout frequency, and Westin et al. (2016) found lying frequency was influenced by parity with higher frequencies in younger cows. Other researchers also observed a complex interaction between parity and lying bouts, where PP cows performed higher lying bout frequencies than older cows (Vasseur et al., 2012). It is plausible PP cows laid down more frequently than MP cows because of a lack of understanding of AMS procedures, as PP cows are fetched to milk more regularly than MP cows (Gednalske, 2021).
MP cows in the current study showed longer daily lying bouts than PP cows, which agrees with previous research. Charlton and colleagues (2016) also found a moderate correlation between parity and mean lying bout duration. Similarly, Vasseur et al. (2012) reported cow parity being moderately positively correlated with mean lying bout duration. At the same time, other researchers observed no effect of parity on daily lying bouts (Hart et al., 2013). Nonetheless, shorter and more frequent lying bouts may indicate increased cow discomfort (Bell et al., 2009; Dippel et al., 2009; Charlton et al., 2016).
Inactive standing or idle time was considered when cows were resting or standing without engaging in any other activity or interaction (Hagen et al., 2005). The majority of previous research investigating inactive standing was conducted in terms of feeding space/activity or stall flooring type effect on cow idle time. For instance, DeVries and Von Keyserlingk (2006) found feeding competition had no influence on PP cow standing time, and Rushen et al. (2007) reported cows standing idle longer in facilities with concrete flooring. No research has found a parity effect on daily idle time. However, in the present study, we found a parity influence on daily and daytime inactive standing, as PP cows spent greater time standing idle than MP cows. The elevated idle times reported in PP cows might suggest overcrowding or social competition in the AMS CP, as primiparous cows are typically lower ranking than MP cows (Olofsson, 1999; DeVries et al., 2004; Huzzey et al., 2006; Proudfoot et al., 2009).
While acceleration activity has yet to be extensively evaluated in dairy cows in AMS farms, one study found acceleration (g) activity varied greatly among individual cows (Yin et al., 2013). The accelerometers used in this study recorded three-dimensional movement used to monitor cow behavior within the AMS facility remotely. In the current study, we found PP cows showed greater daily and daytime acceleration (g) activity than MP cows. No previous research has investigated the impact of parity on cow acceleration. However, activity data are consistent with the reported lying and idling durations from the present study, indicating that PP cows were more active and performed more locomotory behaviors than MU. These findings could be attributed to the fact that primiparous cows are smaller in size and produce less milk than MP cows (Beauchemin and Rode, 1994; Neave et al., 2017), and spend less time milking (Castro et al., 2012), lying (Lobeck-Luchterhand et al., 2014), and standing (Gomez and Cook, 2010), enabling them to perform more activity throughout the day.
In the current study, primiparous cows spent more time idling in the commitment pen, waiting to enter the milking robot, unlike multiparous cows, while the latter performed more displacement agonistic behaviors and attempted to approach the milking robot gate more frequently. Moreover, primiparous cows showed increased HRV-related stress levels in the commitment pen and milking robot than multiparous cows, spent less total time lying, and performed more frequent and shorter lying bouts compared to the multiparous cows. Overall, findings from the current study suggest that cow parity influenced behavior, activity, and stress indicators of primiparous more than multiparous cows and impacted the overall efficiency and success of the AMS. However, it was also noted that multiparous cows exhibited greater stepping than primiparous cows inside the milking robots which indicates higher levels of stress. Separate grouping or advanced training of primiparous cows may be possible interventions to lessen the social competition when milking and reduce possible impacts on their behavior and welfare. However, it should be noted that only one experimental herd and a single barn design were included in the current study, thus, providing a very specific description of events. In the future, the inclusion of numerous experimental herds and differing group sizes may help lead the industry to an ideal solution to promote optimum group mixing and cow behavior within the AMS. Since this study was part of a more significant trial running on the farm, cows were assigned for testing on 97 DIM; further investigation is still needed to test the influence of parity on cow behavior in AMS in different stages of the lactation cycle.
The raw data supporting the conclusions of this article will be made available by the authors, without undue reservation.
Prior to the start of the study, all protocols were submitted to and approved by the Clemson University Institutional Animal Care and Use Committee (AUP#2021-0064). Animals, housing, and AMS. The study was conducted in accordance with the local legislation and institutional requirements.
LD: Writing – original draft, Writing – review & editing, Data curation, Methodology. EF: Conceptualization, methodology, Writing- review & editing. MA: Conceptualization, Writing – original draft, Writing – review & editing. AA: Conceptualization, Formal Analysis, Supervision, Writing – original draft, Writing – review & editing.
The author(s) declare financial support was received for the research, authorship, and/or publication of this article. Approved as Technical Contribution No. 7205 of the Clemson University Experiment Station. This project was partially funded by the South Carolina Dairy Association Endow. This material is based upon work supported by NIFA/USDA, under projects number SC-1700551 and SC-1700608.
We would like to thank all the staff and student workers at the LaMaster Dairy Farm at Clemson University for their time and effort on this project.
The authors declare that the research was conducted in the absence of any commercial or financial relationships that could be construed as a potential conflict of interest.
All claims expressed in this article are solely those of the authors and do not necessarily represent those of their affiliated organizations, or those of the publisher, the editors and the reviewers. Any product that may be evaluated in this article, or claim that may be made by its manufacturer, is not guaranteed or endorsed by the publisher.
Adrien M. L., Mattiauda D. A., Artegoitia V., Carriquiry M., Motta G., Bentancur O., et al. (2012). Nutritional regulation of body condition score at the initiation of the transition period in primiparous and multiparous dairy cows under grazing conditions: milk production, resumption of post-partum ovarian cyclicity and metabolic parameters. Anim. (Cambridge England); Anim. 6, 292–299. doi: 10.1017/S175173111100142X
Akselrod S. (1995). “Components of heart rate variability: basic studies,” in Heart Rate Variability. Eds. Malik M., Camm A. J. (Armonk, NY: Futura Publishing Comp. Inc), 147–163.
Ali A. B., Gutwein K. L., Heleski C. R. (2017). Assessing the influence of upper lip twitching in naive horses during an aversive husbandry procedure (ear clipping). J. Vet. Behav. 21, 20–25. doi: 10.1016/j.jveb.2017.07.001
Beauchemin K. A., Rode L. M. (1994). Compressed baled alfalfa hay for primiparous and multiparous dairy cows. J. Dairy Sci. 77, 1003–1012. doi: 10.3168/jds.S0022-0302(94)77036-3
Bell N. J., Bell M. J., Knowles T. G., Whay H. R., Main D. J., Webster A. (2009). The development, implementation and testing of a lameness control programme based on HACCP principles and designed for heifers on dairy farms. Veterinary J. 180, 178–188. doi: 10.1016/j.tvjl.2008.05.020
Bernasconi P., Messmer E., Bernasconi A., Tholen A. (1998). Assessment of the sympatho-vagal interaction in central serous chorioretinopathy measured by power spectral analysis of heart rate variability. Graefes Arch. Clin. Exp. Ophthalmol. 236, 571e576. doi: 10.1007/s004170050123
Bøe K. E., Færevik G. (2003). Grouping and social preferences in calves, heifers and cows. Appl. Anim. Behav. Sci. 80, 175–190. doi: 10.1016/S0168-1591(02)00217-4
Buck M., Friedli K., Steiner B., Gygax L., Wechsler B., Steiner A. (2013). Influence of manure scrapers on dairy cows in cubicle housing systems. Livestock Sci. 158, 129–137. doi: 10.1016/j.livsci.2013.10.011
Castro A., Pereira J. M., Amiama C., Bueno J. (2012). Estimating efficiency in automatic milking systems. J. Dairy Sci. 95, 929–936. doi: 10.3168/jds.2010-3912
Cerutti S., Bianchi A. M., Mainardi L. T. (1995). “Spectral analysis of the heart rate variability signal,” in Heart Rate Variability. Eds. Malik M., Camm A. J. (Armonk, NY: Futura Publishing Comp. Inc), 63–74.
Charlton G. L., Bouffard V., Gibbons J., Vasseur E., Haley D. B., Pellerin D., et al. (2016). Can automated measures of lying time help assess lameness and leg lesions on tie-stall dairy farms? Appl. Anim. Behav. Sci. 175, 14–22. doi: 10.1016/j.applanim.2015.02.011
Cogato A., Brščić M., Guo H., Marinello F., Pezzuolo A. (2021). Challenges and tendencies of automatic milking systems (AMS): A 20-years systematic review of literature and patents. Anim. (Basel); Anim. (Basel) 11, 356. doi: 10.3390/ani11020356
De Koning K., van der Vorst Y., Meijering A. (2002). “Automatic milking experience and development in Europe,” in The First North Am. Conf. Robotic Milking (Wageningen, the Netherlands: Wageningen Academic Press), I-1–I-11.
Deming J. A., Bergeron R., Leslie K. E., DeVries T. J. (2013). Associations of housing, management, milking activity, and standing and lying behavior of dairy cows milked in automatic systems. J. Dairy Sci. 96, 344–351. doi: 10.3168/jds.2012-5985
DeVries T. J., Von Keyserlingk M. (2006). Feed stalls affect the social and feeding behavior of lactating dairy cows. J. Dairy Sci. 89, 3522–3531. doi: 10.3168/jds.S0022-0302(06)72392-X
DeVries T. J., Von Keyserlingk M., Weary D. M. (2004). Effect of feeding space on the inter-cow distance, aggression, and feeding behavior of free-stall housed lactating dairy cows. J. Dairy Sci. 87, 1432–1438. doi: 10.3168/jds.S0022-0302(04)73293-2
Dijkstra C., Veermäe I., Praks J., Poikalainen V., Arney D. R. (2012). Dairy cow behavior and welfare implications of time waiting before entry into the milking parlor. J. App. Anim. Welf. Sci. 15, 329–345. doi: 10.1080/10888705.2012.709137
Dippel S., Dolezal M., Brenninkmeyer C., Brinkmann J., March S., Knierim U., et al. (2009). Risk factors for lameness in cubicle housed Austrian Simmental dairy cows. Pre. Vet. Med. 90, 102–112. doi: 10.1016/j.prevetmed.2009.03.014
Eicher S. D., Dailey J. W. (2002). Indicators of acute pain and fly avoidance behaviors in Holstein calves following tail-docking. J. Dairy Sci. 85, 2850–2858. doi: 10.3168/jds.S0022-0302(02)74372-5
Fadul-Pacheco L., Liou M., Reinemann D. J., Cabrera V. E. (2021). A preliminary investigation of social network analysis applied to dairy cow behavior in automatic milking system environments. Anim. (Basel) 11, 1229. doi: 10.3390/ani11051229
Fogsgaard K. K., Røntved C. M., Sørensen P., Herskin M. S. (2012). Sickness behavior in dairy cows during Escherichia coli mastitis. J. Dairy Sci. 95, 630–638. doi: 10.3168/jds.2011-4350
Frei A., Evans N. P., King G., McAloon C. G., Viora L. (2022). Associations between cow-level parameters and heart rate variability as a marker of the physiological stress response in dairy cows. J. Dairy Res. 3, 1–6. doi: 10.1017/S0022029922000565
Gednalske B. L. (2021). Factors Associated with Milk Production on Automatic Milking System Farms (Minnesota, USA: Retrieved from the University of Minnesota Digital Conservancy). Available at: https://hdl.handle.net/11299/225093.
Gomez A., Cook N. B. (2010). Time budgets of lactating dairy cattle in commercial freestall herds. J. Dairy Sci. 93, 5772–5781. doi: 10.3168/jds.2010-3436
Gygax L., Neuffer I., Kaufmann C., Hauser R., Wechsler B. (2008). Restlessness behaviour, heart rate and heart-rate variability of dairy cows milked in two types of automatic milking systems and auto-tandem milking parlours. Appl. Anim. Behav. Sci. 109, 167–179. doi: 10.1016/j.applanim.2007.03.010
Hagen K., Langbein J., Schmied C., Lexer D., Waiblinger S. (2005). Heart rate variability in dairy cows—influences of breed and milking system. Physiol. Behav. 85, 195–204. doi: 10.1016/j.physbeh.2005.03.019
Halachmi I. (2009). Simulating the hierarchical order and cow queue length in an automatic milking system. Biosyst. Eng. 102, 453–460. doi: 10.1016/j.biosystemseng.2009.01.010
Hart K. D., McBride B. W., Duffield T. F., DeVries T. J. (2013). Effect of milking frequency on the behavior and productivity of lactating dairy cows. J. Dairy Sci. 96, 6973–6985. doi: 10.3168/jds.2013-6764
Hermans G., Ipema A. H., Stefanowska J., Metz J. (2003). The effect of two traffic situations on the behavior and performance of cows in an automatic milking system. J. Dairy Sci. 86, 1997–2004. doi: 10.3168/jds.S0022-0302(03)73788-6
Herskin M. S., Kristensen A.-M., Munksgaard L. (2004). Behavioural responses of dairy cows toward novel stimuli presented in the home environment. Appl. Anim. Behav. Sci. 89, 27–40. doi: 10.1016/j.applanim.2004.06.006
Hopster H., Blokhuis H. J. (1994). Validation of a heart-rate monitor for measuring a stress response in dairy cows. Canad. J. Anim. Sci. 74 (3), 465–474. doi: 10.4141/cjas94-066
Huzzey J. M., DeVries T. J., Valois P., von Keyserlingk M. A. G. (2006). Stocking density and feed barrier design affect the feeding and social behavior of dairy cattle. J. Dairy Sci. 89, 126–133. doi: 10.3168/jds.S0022-0302(06)72075-6
Jacobs J. A., Ananyeva K., Siegford J. M. (2012). Dairy cow behavior affects the availability of an automatic milking system. J. Dairy Sci. 95, 2186–2194. doi: 10.3168/jds.2011-4749
Jacobs J. A., Siegford J. M. (2012). Invited review: The impact of automatic milking systems on dairy cow management, behavior, health, and welfare. J. Dairy Sci. 95, 2227–2247. doi: 10.3168/jds.2011-4943
Johnson C. R., Lalman D. L., Brown M. A., Appeddu L. A., Buchanan D. S., Wettemann R. P. (2003). Influence of milk production potential on forage dry matter intake by multiparous and primiparous Brangus females. J. Anim. Sci. 81, 1837–1846. doi: 10.2527/2003.8171837x
Jurkovich V., Kezer F. L., Ruff F., Bakony M., Kulcsar M., Kovács L. (2017). Heart rate, heart rate variability, faecal glucocorticoid metabolites and avoidance response of dairy cows before and after changeover to an automatic milking system. Acta Veterinaria Hungarica 65, 301–313. doi: 10.1556/004.2017.029
Kleiger R. E., Stein P. K., Bosner M. S., Rottman J. N. (1992). Time domain measurements of heart rate variability. Cardiol. Clinics 10, 487–498. doi: 10.1016/S0733-8651(18)30230-3
Kliś P., Piwczyński D., Sawa A., Sitkowska B. (2021). Prediction of lactational milk yield of cows based on data recorded by AMS during the periparturient period. Animals 11, 383. doi: 10.3390/ani11020383
Kovács L., Kézér L., Tőzsér J. (2013). “Measuring stress level of dairy cows during milking using by geometric indices of heart rate variability,” in Scientific Papers: Animal Science & Biotechnologies/Lucrari Stiintifice: Zootehnie si Biotehnologii. Facultatea de Zootehnie si Biotehnologii, Timisoara, Animal Science & Biotechnologies / Lucrari Stiintifice, 46.
Krohn C. C. (1994). Behaviour of dairy cows kept in extensive (loose housing/pasture) or intensive (tie stall) environments. III. Grooming, exploration and abnormal behaviour. Appl. Anim. Behav. Sci. 42, 73–86. doi: 10.1016/0168-1591(94)90148-1
Landis J. R., Koch G. G. (1977). The measurement of observer agreement for categorical data. Biometrics, 159–174. doi: 10.2307/2529310
Lobeck-Luchterhand K., Silva P. R. B., Chebel R. C., Endres M. I. (2014). Effect of prepartum grouping strategy on displacements from the feed bunk and feeding behavior of dairy cows. J. Dairy Sci. 97, 2800–2807. doi: 10.3168/jds.2013-7401
Lupoli B., Johansson B., Uvnäs-Moberg K., Svennersten-Sjaunja K. (2001). Effect of suckling on the release of oxytocin, prolactin, cortisol, gastrin, cholecystokinin, somatostatin and insulin in dairy cows and their calves. J. Dairy Res. 68, 175–187. doi: 10.1017/S0022029901004721
Melin M., Hermans G. G. N., Pettersson G., Wiktorsson H. (2006). Cow traffic in relation to social rank and motivation of cows in an automatic milking system with control gates and an open waiting area. Appl. Anim. Behav. Sci. 96, 201–214. doi: 10.1016/j.applanim.2005.06.013
Mestivier D., Chau N. P., Chanudet X., Bauduceau B., Larroque P. (1997). Relationship between diabetic autonomic dysfunction and heart rate variability assessed by recurrence plot. Am. J. Physiol. Heart Circ. Physiol. 272, 1094–11099. doi: 10.1152/ajpheart.1997.272.3.H1094
Moss A. J. (1995). Heart Rate Variability. Eds. Malik M., Camm A. J. (Armonk, NY: Futura Publishing Comp. Inc), iii–iiv.
Neave H. W., Lomb J., von Keyserlingk M. A. G., Behnam-Shabahang A., Weary D. M. (2017). Parity differences in the behavior of transition dairy cows. J. Dairy Sci. 100, 548–561. doi: 10.3168/jds.2016-10987
Nogues E., Lecorps B., Weary D. M., von Keyserlingk M. A. (2020). Individual variability in response to social stress in dairy heifers. Animals 10, 1440. doi: 10.3390/ani10081440
Norring M., Valros A., Munksgaard L. (2012). Milk yield affects time budget of dairy cows in tie-stalls. J. Dairy Sci. 95, 102–108. doi: 10.3168/jds.2010-3458
Olofsson J. (1999). Competition for total mixed diets fed for ad libitum intake using one or four cows per feeding station. J. Dairy Sci. 82, 69–79. doi: 10.3168/jds.S0022-0302(99)75210-0
Pagani M., Lombardi F., Guzzetti S., Rimoldi O., Furlan R., Pizzinelli P., et al. (1986). Power spectral analysis of heart rate and arterial pressure variabilities as a marker of sympatho-vagal interaction in man and conscious dog. Circ. Res. 59, 178–1193. doi: 10.1161/01.RES.59.2.178
Perttu R. K., Heins B. J., Phillips H. N., Endres M. I., Moon R. D., Sorge U. S. (2020). Effects of mesh leggings on fly pressure and fly avoidance behaviors of pastured dairy cows. J. Dairy Sci. 103, 846–851. doi: 10.3168/jds.2019-17267
Proudfoot K. L., Veira D. M., Weary D. M., von Keyserlingk M. A. G. (2009). Competition at the feed bunk changes the feeding, standing, and social behavior of transition dairy cows. J. Dairy Sci. 92, 3116–3123. doi: 10.3168/jds.2008-1718
Rodenburg J. (2013). “Success factors for automatic milking,” in Precision Dairy Conference, Mayo Civic Center, Rochester, Minnesota. 22–34.
Rodenburg J. (2017). Robotic milking: Technology, farm design, and effects on work flow. J. Dairy Sci. 100, 7729–7738. doi: 10.3168/jds.2016-11715
Rodenburg J., House H. K. (2007). “Field observations on barn layout and design for robotic milking,” in Sixth International Dairy Housing Conference Proceeding, American Society of Agricultural and Biological Engineers, Rochester, Minnesota: Proceedings of the precision dairy conference and expo, Mayo Civic Center) 16-18 June 2007. 21.
Rotz C. A., Coiner C. U., Soder K. J. (2003). Automatic milking systems, farm size, and milk production. J. Dairy Sci. 86, 4167–4177. doi: 10.3168/jds.S0022-0302(03)74032-6
Rushen J., Haley D., de Passillé A. M. (2007). Effect of softer flooring in tie stalls on resting behavior and leg injuries of lactating cows. J. Dairy Sci. 90, 3647–3651. doi: 10.3168/jds.2006-463
Salfer J. A., Siewert J. M., Endres M. I. (2018). Housing, management characteristics, and factors associated with lameness, hock lesion, and hygiene of lactating dairy cattle on Upper Midwest United States dairy farms using automatic milking systems. J. Dairy Sci. 101, 8586–8594. doi: 10.3168/jds.2017-13925
Schmidt A., Möstl E., Wehnert C., Aurich J., Müller J., Aurich C. (2010). Cortisol release and heart rate variability in horses during road transport. Hormones Behav. 57 (2), 209–215. doi: 10.1016/j.yhbeh.2009.11.003
Schwanke A. J., Dancy K. M., Neave H. W., Penner G. B., Bergeron R., DeVries T. J. (2022). Effects of concentrate allowance and individual dairy cow personality traits on behavior and production of dairy cows milked in a free-traffic automated milking system. J. Dairy Sci. 105 (7), 6290–6306. doi: 10.3168/jds.2021-21657
Siewert J., Salfer J., Endres M. (2019). Milk yield and milking station visits of primiparous versus multiparous cows on automatic milking system farms in the Upper Midwest United States. J. Dairy Sci. 102, 3523–3530. doi: 10.3168/jds.2018-15382
Sitkowska B., Piwczyński D., Brzozowski M., Aerts J. (2016). Quarter milking in primiparous and multiparous cows. Rocz.Nauk.Polskiego Towar.Zootech 12, 35–48. doi: 10.5604/01.3001.0013.5397
Solano L., Halbach C., Bennett T. B., Cook N. B. (2022). Milking time behavior of dairy cows in a free-flow automated milking system (JDS Communications).
Spolders M., Meyer U., Flachowsky G., Coenen M. (2004). Differences between primiparous and multiparous cows in voluntary milking frequency in an automatic milking system. Ital. J. Anim. Sci. 3, 167–175. doi: 10.4081/ijas.2004.167
Szentléleki A., Nagy K., Széplaki K., Kékesi K., Tozsér J. (2015). Behavioural responses of primiparous and multiparous dairy cows to the milking process over an entire lactation. Ann. Anim. Sci. 15, 185. doi: 10.2478/aoas-2014-0064
Uetake K., Hurnik J. F., Johnson L. (1997). Effect of music on voluntary approach of dairy cows to an automatic milking system. Appl. Anim. Behav. Sci. 53, 175–182. doi: 10.1016/S0168-1591(96)01159-8
Val-Laillet D., Guesdon V., von Keyserlingk M. A. G., de Passillé A. M., Rushen J. (2009). Allogrooming in cattle: Relationships between social preferences, feeding displacements and social dominance. Appl. Anim. Behav. Sci. 116, 141–149. doi: 10.1016/j.applanim.2008.08.005
Vasseur E., Rushen J., Haley D. B., de Passillé A. M. (2012). Sampling cows to assess lying time for on-farm animal welfare assessment. J. Dairy Sci. 95, 4968–4977. doi: 10.3168/jds.2011-5176
Von Borell E., Langbein J., Després G., Hansen S., Leterrier C., Marchant-Forde J., et al. (2007). Heart rate variability as a measure of autonomic regulation of cardiac activity for assessing stress and welfare in farm animals—A review. Physiol. Behav. 92, 293–316. doi: 10.1016/j.physbeh.2007.01.007
von Keyserlingk M., Olenick D., Weary D. M. (2008). Acute behavioral effects of regrouping dairy cows. J. Dairy Sci. 91, 1011–1016. doi: 10.3168/jds.2007-0532
Wade K. M., van Asseldonk M. A. P. M., Berentsen P. B. M., Ouweltjes W., Hogeveen H. (2004). “Economic efficiency of automatic milking systems with specific emphasis on increases in milk production,” in Automatic Milking—A Better Understanding. Eds. Meijering A., Hogeveen H., de Koning C. J. A. M. (Wageningen, the Netherlands: Wageningen Academic Publishers), 62–67.
Weary D. M., Schuppli C. A., Von Keyserlingk M. (2011). Tail docking dairy cattle: Responses from an online engagement. J.Anim. Sci. 89, 3831–3837. doi: 10.2527/jas.2011-3858
Wehrend A., Hofmann E., Failing K., Bostedt H. (2006). Behaviour during the first stage of labour in cattle: Influence of parity and dystocia. Appl. Anim. Behav. Sci. 100, 164–170. doi: 10.1016/j.applanim.2005.11.008
Westin R., Vaughan A., de Passillé A. M., Devries T. J., Pajor E. A., Pellerin D., et al. (2016). Lying times of lactating cows on dairy farms with automatic milking systems and the relation to lameness, leg lesions, and body condition score. J. Dairy Sci. 99, 551–561. doi: 10.3168/jds.2015-9737
Willson D. W., Baier F. S., Grandin T. (2021). An observational field study on the effects of changes in shadow contrasts and noise on cattle movement in a small abattoir. Meat Sci. 179, 108539. doi: 10.1016/j.meatsci.2021.108539
Witaifi A. A., Ali A. B., Siegford J. M. (2018). Stall and feed bunk stocking rates impact cows’ diurnal behavior and activity in automatic milking system farms. J. Vet. Beh. 24, 48–55. doi: 10.1016/j.jveb.2018.01.004
Wolfger B., Mang A. V., Cook N., Orsel K., Timsit E. (2015). Evaluation of a system for monitoring individual feeding behavior and activity in beef cattle. J. Anim. Sci. 93 (8), 4110–4114. doi: 10.2527/jas.2015-8947
Keywords: dairy cows, behavior, parity, automatic milking system, heart rate variability, commitment pen, milking robot
Citation: Davis L, French EA, Aguerre MJ and Ali A (2023) Impact of parity on cow stress, behavior, and production at a farm with guided traffic automatic milking system. Front. Anim. Sci. 4:1258935. doi: 10.3389/fanim.2023.1258935
Received: 14 July 2023; Accepted: 24 November 2023;
Published: 11 December 2023.
Edited by:
Marcia Endres, University of Minnesota Twin Cities, United StatesReviewed by:
Meagan King, University of Manitoba, CanadaCopyright © 2023 Davis, French, Aguerre and Ali. This is an open-access article distributed under the terms of the Creative Commons Attribution License (CC BY). The use, distribution or reproduction in other forums is permitted, provided the original author(s) and the copyright owner(s) are credited and that the original publication in this journal is cited, in accordance with accepted academic practice. No use, distribution or reproduction is permitted which does not comply with these terms.
*Correspondence: Matias J. Aguerre, bWFndWVyckBjbGVtc29uLmVkdQ==
†These authors have contributed equally to this work
Disclaimer: All claims expressed in this article are solely those of the authors and do not necessarily represent those of their affiliated organizations, or those of the publisher, the editors and the reviewers. Any product that may be evaluated in this article or claim that may be made by its manufacturer is not guaranteed or endorsed by the publisher.
Research integrity at Frontiers
Learn more about the work of our research integrity team to safeguard the quality of each article we publish.