- 1School of Agriculture and Food Sustainability, Faculty of Science, The University of Queensland, St Lucia, QLD, Australia
- 2Centre for Animal Science, Queensland Alliance for Agriculture and Food Innovation, The University of Queensland, St Lucia, QLD, Australia
Introduction: Australia is the world’s largest producer and exporter of fine wool. External factors (i.e., non-genetic) that affect wool phenotype need to be identified and managed to minimize any negative effects on wool quality. Climatic stress is one external event that can affect wool phenotype, other stressors include shearing, nutrition, disease, pregnancy, and lambing. This study focuses on the relationship between Saxon Merino ram wool phenotype and wool cortisol. The hypotheses had two parts (i) wool cortisol levels would vary seasonally and variations would correlate with wool phenotype (ii) that these seasonal variations would also correlate with thermal indices.
Methods: Cortisol levels in Saxon Merino ram wool samples were determined using a cortisol enzyme-immunoassay (EIA) using polyclonal cortisol antibody. The wool cortisol results were correlated for significance with thermal indices including Temperature Humidity Index [THI] and Chill Index [CI] obtained from the Australian Bureau of Meteorology.
Results: Part 1 of hypothesis one was supported with wool cortisol showing significant seasonal variation. Part 2 of hypothesis 1 was not supported, with no statistically significant causality present between total wool cortisol levels and the price index of Saxon Merino ram wool. However, there was significant causality between wool cortisol and coarse edge measurement (CEM) of wool. Hypothesis 2, the proposal that thermal indices would impact on wool cortisol was also not supported.
Discussion: It can be concluded that although conclusive causality between total wool cortisol and wool phenotype quality could not be ascertained, there were indirect effects of cortisol levels on wool phenotypes with significant effects on the CEM. The CEM of Merino Saxon ram wool can be manipulated by assessing and managing cortisol levels during the growth cycles of Merino wool, through appropriate nutrition and husbandry procedures stress could be managed.
Introduction
Merino sheep grow fine wool that is regarded amongst the finest in the world (Rogers, 2006). Merino breeds were the main contributor to Australia becoming the largest producer and exporter of fine quality wool globally (Rogers, 2006). Up to 80% of the wool Australia exports to the apparel industry has high-quality wool demands, which can be met by Merino wool (Sawyer and Narayan, 2019). The Merino sheep breed originated in Spain during the Middle Ages and was imported to Australia in the early 19th century (Macpherson, 2008; Ciani et al., 2015). Merino sheep breed in Australia include Peppin, Saxon (hereditary and homogeneity features), South Australian, and Spanish Merino (Carter and Clarke, 1957). One hundred of the Merino sheep imported to Tasmania in the 19th century were Saxon Merino (Ciani et al., 2015), and this breed will be the focus of this study. Merino sheep features include (1) large thermal adapt- able zone; 12°C to +32°C (Srikandakumar et al., 2003), (2) Merino sheep wool grows year-round, all over the body (Rogers, 2006) and (3) up to one hundred million wool follicles per sheep, with follicle efficiency inducing fine wool production (Rogers, 2006).
Defining fine wool phenotype quality requires a combination of traits including fibre diameter (FD), staple strength (SS), staple break region (SBR), staple length (SL), comfort factor (COMF), and spinning fineness (SF). There is also coarse edge measurement (CEM) or prickle factor (PF), curvature (CURV), and greasy fleece weight (GFW) (Rogers, 2006; Mcgregor et al., 2011; Holman and Malau-Aduli, 2012). Saxon Merino ram wool phenotypic quality is affected by extrinsic factors, including nutrition, disease, and climatic variations (Black et al., 2008; Marai et al., 2008). Other examples of on-farm factors that may become stressors include acute (short term), including transportation and relocation (Narayan and Parisella, 2017; Lees et al., 2020), or chronic (long term) stressors, including climatic extremes and breeding (Tilbrook et al., 1999; Narayan and Parisella, 2017). Stressors alter homeostasis of animals (Silmen et al., 2016; Tilbrook and Ralph, 2016) and influence wool phenotype traits including FD, SL, SS, and GFW (Kumar et al., 2010; Weaver et al., 2021). In addition to impacts on wool quality, stressors will also have negative impacts on animal fertility (Tilbrook et al., 1999; Breen et al., 2007; McManus et al., 2020).
Despite Merino breeds demonstrating good environmental adaptability they are nevertheless susceptible to physical and emotional stressors of shearing; with fear of herding by dogs, isolation from other sheep, heavy manhandling during shearing process, and thermal stressors (Pluske et al., 2010; Tattersall et al., 2012; Knight, 2020). Australian environments are subject to wet and dry extremes (Islam et al., 2015; Aryal et al., 2019; Mckeon et al., 2021), which can exacerbate the effects of thermal stressors (Marai et al., 2008; Gaughan et al., 2017). As the mating cycles of Merino sheep are photoperiod (season) dependent, extrinsic changes, particularly in feed availability and early occurrence of high temperature coupled with increasing daylight hours can have a negative impact on fertility due to environmentally induced stress (Fabre-Nys et al., 2015). Rams begin the mating cycle nearly 1.5 months before females to ensure readiness for the onset of oestrus (Rosa and Bryant, 2003). All stressors induce physical (e.g., behavioural) and physiological (e.g., hormonal) responses in animals as they attempt to restore homeostasis (Killen et al., 2013; Rashamol et al., 2018; Tilbrook and Ralph, 2016; Zoratti et al., 2023). Biological responses invoke fight or flight reactions to the stressor. The intensity of these reactions is dependent on the duration and intensity of the stressor and distance from the homeostatic balance that the sheep are experiencing (Von Borell, 2001; Carcangiu et al., 2008; Tilbrook and Ralph, 2016). Sheep implement physical responses to restore the body to homeostatic state. The responses include seeking shelter from sun, wind, or rain and variations in feed consumption (Haidary, 2004; Nienaber and Hahn, 2007; Tilbrook and Ralph, 2016). Responses can vary dependent on sexuality of the sheep, which can yield different physiological responses and variations in hormone production; meaning that the stressor effects will differ between males and females resulting in differing impacts on Merino wool phenotypes (Tilbrook and Clarke, 2006).
A typical physiological response to stressors is activation of the hypothalamo-pituitary adrenal (HPA) axis and production of glucocorticoids such as cortisol, a well-established biomarker of physiological stress in sheep (Killen et al., 2013; Sawyer and Narayan, 2019; Weaver et al., 2021). The endocrine signals required for cortisol production originates in the hypothalamus. Cortisol is synthesised by cholesterol and is stored in the adrenal cortex being passively released in response to stimulation by the adrenocorticotropic hormone (ACTH), which originates in the pituitary gland (Narayan et al., 2018). Cortisol flows from the adrenal cortex into the bloodstream. It is then circulated throughout the body and in Merino sheep are absorbed by wool fibres (Sawyer and Narayan, 2019; Hantzopoulou et al., 2022). The presence of free-cortisol in-vivo impedes the immune system (McManus et al., 2020), which can lead to negative welfare outcomes. Strategies will then need to be implemented to restore sheep welfare (Nienaber and Hahn, 2007; Gaughan et al., 2017).
Cortisol can be detected in sheep wool thereby preventing possible bias invasive methods (e.g., blood collection) can produce (Nejad et al., 2014). Cortisol retrieval via intravenous extractions from the blood can elevate cortisol levels which are detectable minutes to hours after the extraction, but not instantaneously. Pretesting activities where microclimates change can induce a stress response leading to an increase in circulating cortisol levels (Adenkola and Ayo, 2010; Verbeek et al., 2012; Lees et al., 2020). Cold stress generates cortisol pathways the same as other stress related cortisol production, except cold stress generates insulin production decreasing glycogen levels causing hypoglycaemia in sheep (Bhimte et al., 2018).
The primary aim of the current study was to determine correlations between wool cortisol as an index of physiological stress and Saxon Merino ram wool quality phenotypes. The first part of this study measured total cortisol levels in Saxon Merino ram wool and correlated cortisol with wool phenotypes that contribute to price index. It was hypothesised that cortisol levels will influence the wool phenotypes. The second part of this study was to determine if there were correlations between cortisol levels that vary seasonally, and hot-cold thermal indices. The presence of heat and cold with their impacts on Merino sheep and wool production. It is hypothesised that hot and cold extreme weather and corresponding thermal indices, will impact cortisol production, and affect wool phenotype quality.
Methods
This study was conducted with the approval of The University of Queensland (UQ) Animal Care and Ethics Committee (ANRFA: 2021/AE000485), in accordance with the guidelines described by the Australian code for the care and use of animals for scientific purposes (2013) and Queensland Department of Agriculture and Fisheries (2009). Animal data (mid-side wool) was collected by a stud operator in Tasmania, the authors did not undertake sample collection. The day-to-day operations of the stud and reproductive/breeding practices were commercial in confidence. Without knowing the daily routines of the rams from the stud, only conclusions that align with the data measured and the limited information supplied can be made.
Field methods: sheep welfare, origin, and wool samples
The body condition and overall health status of the sheep from which the wool samples were obtained was unknown, as husbandry procedures, and health data were not included in supplied data. The sheep used were from a large Merino stud located in the Tasmanian Midlands, an area that is well known for Merino production. This region has a cool temperate climate classification, where summer is mild to warm, and winter is cold with an average daily temperature range of +3°C to +23°C. The annual rainfall range is between 450 to 500 mm on average (Bureau of Meteorology, 2022a; Bureau of Meteorology, 2022b). Twenty Saxon Merino ram weaners that had not yet been used for breeding were selected, and not castrated. The rams were born in 2020, and the wool samples (n = 20) were obtained from the mid-side region of the body in 2021 (at age; 12 to 18 months), by the stud manager from the second shearing event wool clips and sent to the Stress Laboratory at The University of Queensland, Gatton Campus for cortisol analysis.
Laboratory methods: wool processing and hormone testing
Each wool clip sample (n(sheep)=20) was removed from storage in the fridge and then was cut using scissors into 10 mm sub-samples (Figure 1). As there was variation in the staple length between sheep, the total number of sub samples per sheep varied from 5 to 7, thus a total of 125 sub samples were available for cortisol assay. Each 10 mm section accounted for approximately 1 month’s wool growth (Downes and Sharry, 1971). The 10 mm sub samples were placed in 3 cm x 3 cm weighing trays and weighed (average weight was 45 mg). The wool samples were washed in 3 mL of 100% isopropanol to remove contaminants. Samples were then dried overnight in a desiccator. The dried wool samples were then removed from the desiccator, weighed (to obtain clean sample weight), finely chopped to<0.5 (mm) pieces using a scissors, placed into 1.5mL Eppendorf tubes, and treated with 1000 µL of alcohol (100% analytical grade methanol), then refrigerated overnight at 4°C.
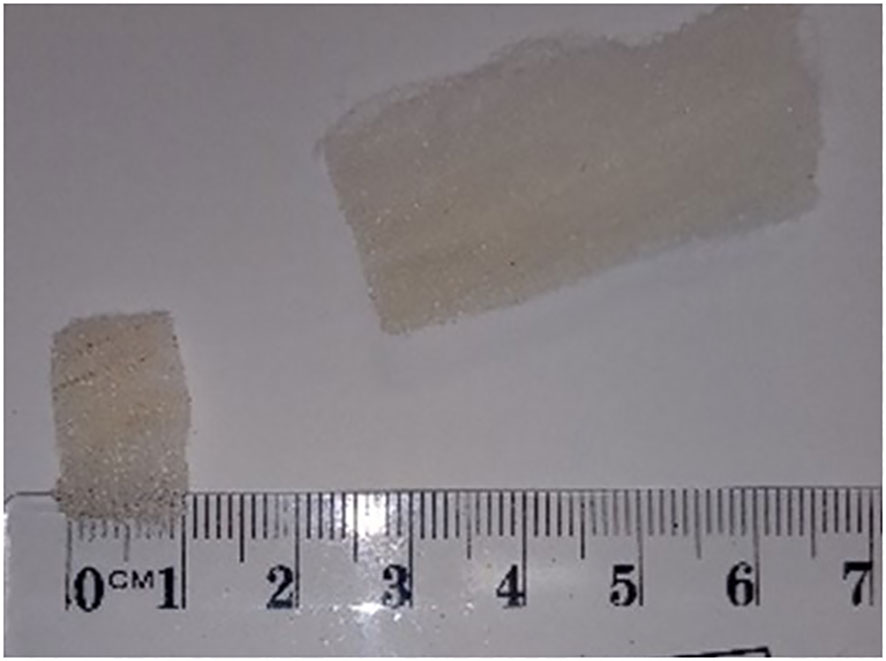
Figure 1 Sample of Saxon Merino ram wool shorn from the mid-side region of the rams being cut into 10 mm sections for cortisol analysis which is representative of monthly wool growth.
After the removal of the sample extracts from the fridge, 500 µL of methanol was aliquoted from each of the original tube into new 1.5mL Eppendorf tubes and left to dry overnight in the fume hood. This Eppendorf tube with dried methanol extract were then reconstituted with 25 µL of alcohol (100% methanol) and 475 µL of enzyme-immunoassay assay buffer added, and then vortexed individually (see for buffer details; Sawyer et al., 2021). The laboratory analysis of wool cortisol in the samples were performed using an enzyme-immunoassay established methods described earlier (Sawyer et al., 2021 and used most recently by Hantzopoulou et al., 2022). Briefly, samples were assayed in duplicate and the wool cortisol levels were expressed as ng/g.
Attributes of Saxon Merino ram wool sample phenotypes
Analysis of the wool phenotype were conducted using Laser-scan. Saxon Merino ram wool quality values evaluated were the FD, SF, COMF, CEM, SL, CURV, and GFW. The descriptions of these traits are as follows; FD average in microns recorded, the FD standard deviation (SD) were values enveloping 68% of FD values. The coefficient of variation (CV) measures the variation of the FD in the samples, where 15% is uniform, and 30% is highly variable. Course Edge Management (CEM) or prickle factor (PF) are related to the number of fibres thicker than 30 µm. Comfort Factor (COMF) is measured as the number of wool fibres protruding from the primary fibre below 30.5 µm and measured as a percentage of fleece. Spin Fineness (SF) determined from the FD and CV defining the minimum fineness of processing performance. CURV/crimp is measured by degrees/mm of fibre. Greasy fleece weight (GFW) is the weight of the Saxon Merino ram wool before removing contaminants, including wax, suint, vegetable matter, and dust (Rogers, 2006; Macpherson, 2008; Mcgregor et al., 2011; Holman and Malau-Aduli, 2012).
Climatic data
Climate data for Midlands Tasmania was obtained from the Bureau of Meteorology website (Bureau of Meteorology, 2022c; Bureau of Meteorology, 2022d; Bureau of Meteorology, 2022e; Bureau of Meteorology, 2022f; Bureau of Meteorology, 2022g; Bureau of Meteorology, 2022h; Bureau of Meteorology, 2022i; Bureau of Meteorology, 2022j; Bureau of Meteorology, 2022k; Bureau of Meteorology, 2022l; Bureau of Meteorology, 2022m; Bureau of Meteorology, 2022n). When calculating the average THI, the average monthly data was used as source for temperature and relative humidity (RH) measure. Values from the 0900 h and 1500 h RH readings were used to represent average daily RH values. The 1500 h relative humidity values were calculated from the yearly averages (Bureau of Meteorology, 2022o), as a decrease of 20% from 0900 h to 1500 h. The temperature was taken as the average monthly temperature, and the following method was applied in calculating THI values in THI units.
Where T = ambient temperature or dry bulb temperature in °C, RH = relative humidity in %, adapted from Thom (1959).
BOM wind values taken at the 0900 h readings for the Ross, The Boulevards site were used in the CI calculations. The temperature was the average temperature for each month and the rainfall was also the average value for each month. The CI is measured in kJ/m2/hour, which is heat lost to the environment in Kilojoules per metre squared (estimated whole sheep) per hour (Donnelly, 1984).
(Ugurlu et al., 2014; Nel et al., 2021).
A study by Weaver et al. (2021) indicated that at day 0-14 (pre-treatment phase), cortisol levels present in wool samples taken from dry ewe sheep were<5 ng/g, high levels were recorded > 20 ng/g (end of the treatment phase >56 days). Due to a lack of prior original data on Merino ram wool cortisol, for the current study, low cortisol levels were set at 5 ng/g cortisol presence, equating to the pre-treatment phase of the study by Weaver et al. (2021), since we do not have equivalent baseline wool cortisol data for Saxon rams. Chronic stress level was defined as > 20 ng/g based on concentrations at post treatment compared to treatment period in the study by Weaver et al. (2021).
Statistical analysis
Modelling the data in this current study required the use of parametric statistical analysis for the data sets. This was conducted using R programming language for statistical computing, for thermal indices and wool phenotypes including descriptive statistics of cortisol and wool phenotypes. Particularly phenotypes that represent ease of use for the end products that are available with the wool staples such as: prickle factor (PF) or comfort factor (CF) and coarse edge measurement (CEM). One-way ANOVA (single factor) and multiple linear regression were used to compare the changes in wool cortisol and wool phenotype across seasons. All wool phenotype measures (SS, SL, CEM, CF, SF and CURV) were within expected range of values. Bureau of Meteorology (BOM) data was used to determine Temperature Humidity Index (THI) and Chill Index (CI) values, then correlations to cortisol levels were calculated.
The wool cortisol data was calculated by dividing 12 months of the year by number of samples per staple length of the individual sheep wool samples, to evaluate cortisol levels during months of the year 2021, enabling comparison to monthly climate measurements. Sizes of the staple lengths of wool samples varied, producing 5-, 6-, 7- sub samples for 10-mm samples per staple length; hence each 10 mm sample represented different amounts of time growth. Adjustments allowed regression analysis between cortisol and monthly thermal stress indices climate is independent and cortisol dependent variable, which were measured with Pearson R-values for linear correlation, p-values (p< 0.05) for probability and t-test for significance.
Wool cortisol data was plotted with mean, median, and range to gain an overall perspective of the cortisol levels in the wool samples. One-way ANOVA was conducted on the 20 samples to analyse for variations in cortisol levels. Regression analysis was conducted between total and mean cortisol data and price index (represents overall wool quality), and with selected important phenotype qualities. The price index supplied by stud operator, based on sale price. Pearson R-values for linear correlation, p-values for probability and t-test for significance were all recorded. For statistical significance, the p-values were set at 0.05 (5%), and t-critical values were set at 19 degrees of freedom (df), 2.093.
Results
Hypothesis one: correlations between wool cortisol and wool phenotypes
Descriptive statistics for cortisol showed a min=0.85 ng/g-max=277.70 ng/g with range of 276.84 ng/g, median of 14.19 ng/g, mean of 28.59 ng/g. Hypothesis 1, was not supported with regression analysis for wool phenotypes (price index) and cortisol levels revealing (= 0.191, adjusted =0.036, P=0.42), i.e., there is no linear correlation between price index and cortisol levels, and no causal connection. Apart from coarse edge measurement with positive R-value (=0.493, adjusted =0.243, P=0.027), there was no correlation between any other of the measured individual wool phenotype qualities and cortisol level (Table 1). Although we found no statistical significance between price and cortisol, the cortisol levels did vary over the course of the year with statistically significant P-value, (Fstat = 4.754, df = 19, P<0.0001).

Table 1 Saxon Merino ram wool phenotype descriptive statistics and total cortisol with wool phenotype Regression analysis statistics.
Figure 2 is representative of the variation of the cortisol in all the 20 Saxon Merino sheep wool samples, indicating whether there is variation in wool cortisol levels for the year 2021. This will indicate whether correlations could be expected between these cortisol levels and different qualities of the Saxon Merino sheep wool.
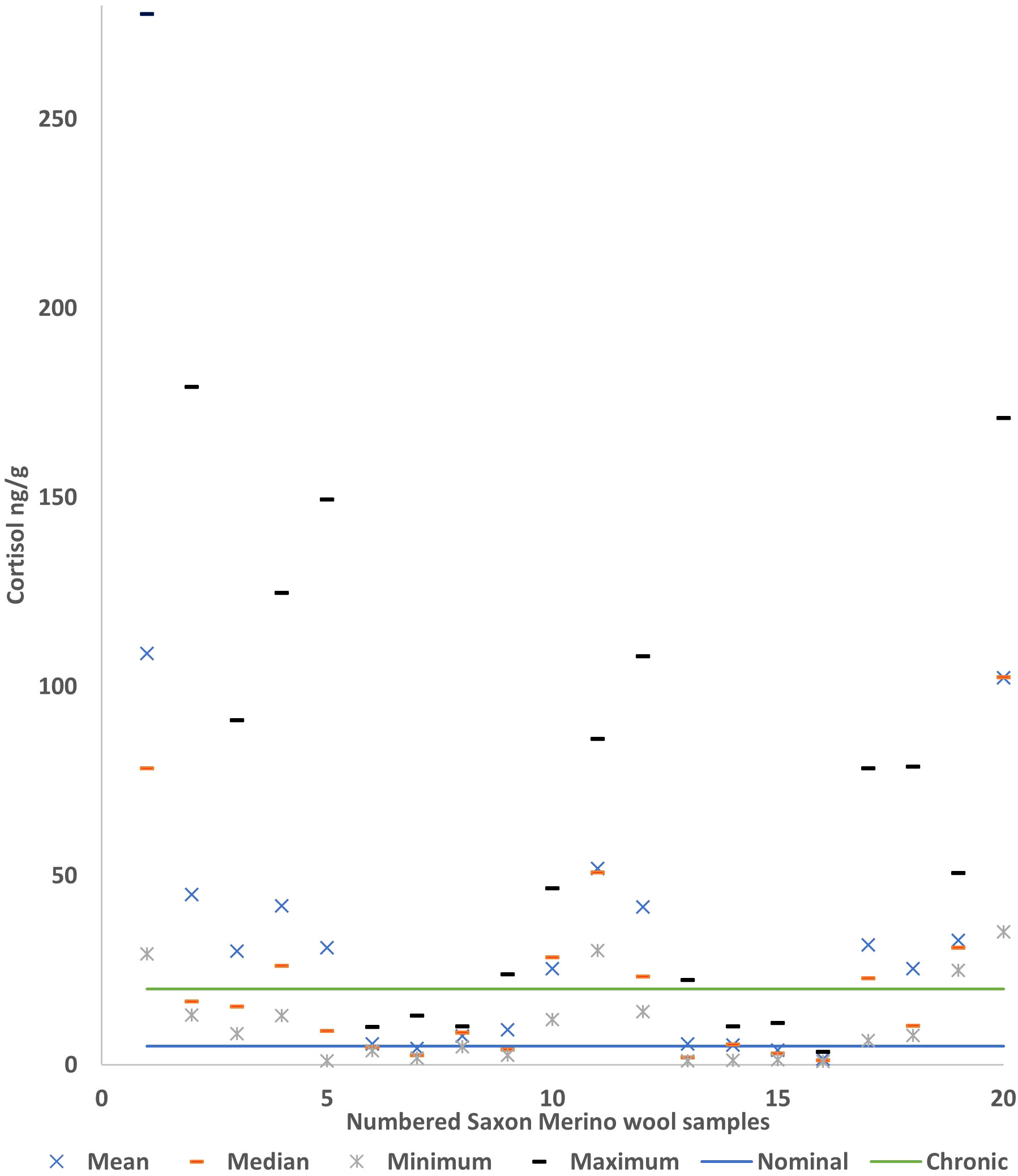
Figure 2 The range of the Saxon Merino ram wool cortisol levels, wool samples consistently below the nominal level in the chart are from sheep 7, 14, 15, and 16, indicating that minimal stress conditions were experienced by these sheep. Wool samples from sheep 5, 6, 7, 8, 9 and sheep 13, indicated much lower averages and range indicative of mid-range stress levels. Samples 1 to 5, 10 to 12, and 17 to 20 displayed levels of cortisol above that of the chronic stress baseline level set by Weaver et al., 2021, indicating Saxon Rams can express higher stress threshold.
The regression analysis for cortisol and thermal indices did not support hypothesis 2 (correlation between climate indices and wool cortisol), the THI and cortisol linear correlations produce P-value > 0.05 (= 0.95, adjusted =0.744, P=0.193), and CI and cortisol linear correlations produce P-value>0.05 (=0.983, adjusted =0.907, P=0.074). The THI and CI data are presented in Table 2 and displayed in Figure 3. The THI level is consistently below the THI threshold of 72 (no heat stress). The CI levels are consistently above CI 2, and chronic stress occurs for nine months above the CI 3 threshold.

Table 2 Mean monthly temperature - humidity index (THI) and chill index (CI) for the year 2021 in the Tasmanian Midlands region using BOM data and equations 1 and 2.
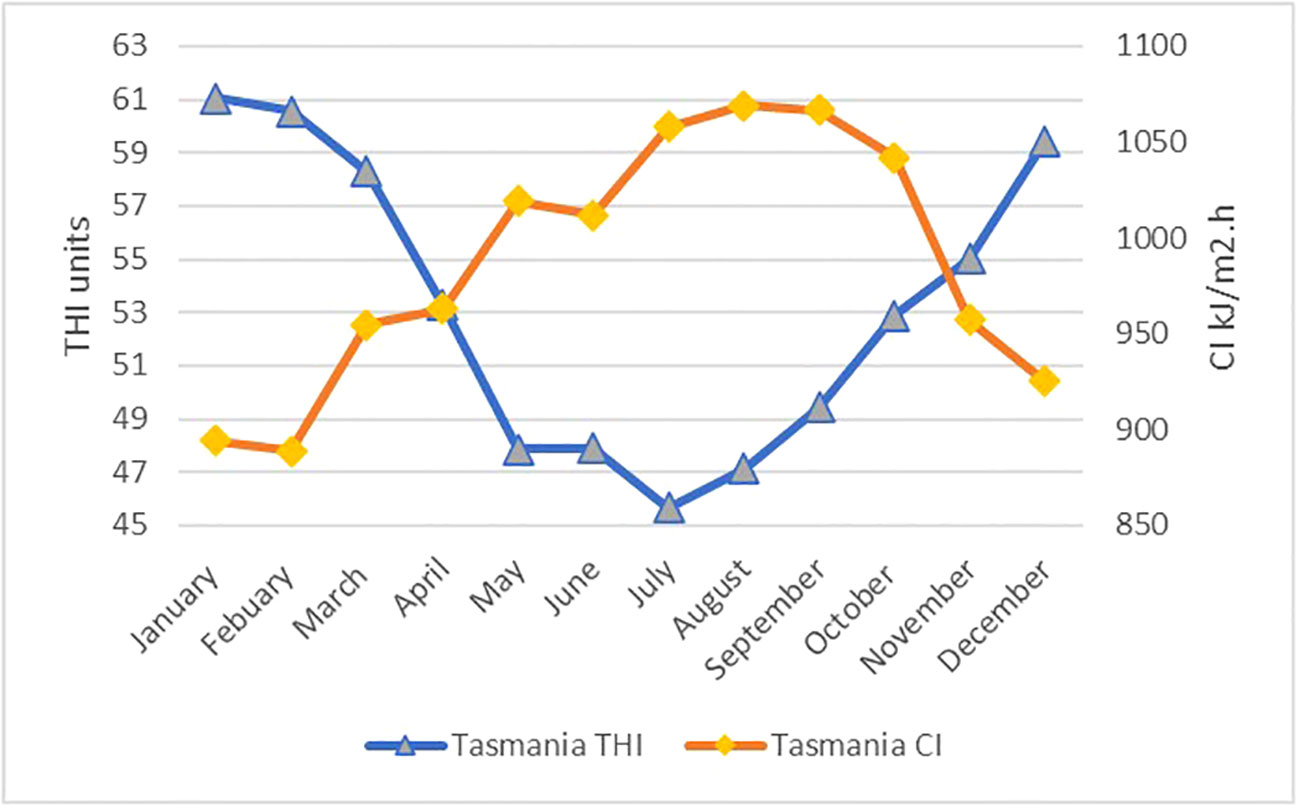
Figure 3 A comparison of the mean monthly temperature - humidity index (THI) and chill index (CI) for the year 2021 in the Tasmanian Midlands region, indicating that the sheep are under moderate to severe CI all year round.
Both thermal indices underwent regression analysis with 12 thermal indices (each) to 125 sub-samples, Table 3 presents calculations from the multiple regression analysis between the thermal indices and total cortisol levels. Linear correlation and p-value were obtained for THI levels with total cortisol levels from the n=20 Saxon merino sheep, and CI levels with the total cortisol levels of the n=20 Saxon Merino sheep.
CI = {[11.7 + (3.1 x ave wind speed/2)](40 – ave temperature) +481+(481(1- X ave rain))} ().
THI = (0.8 ∗ T) + ∗ (T − 14.4) + 46.4 (THI units).
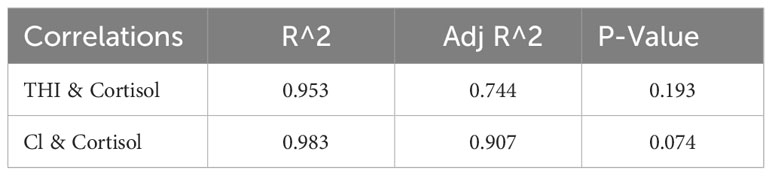
Table 3 Regression analysis statistics between thermal indices THI, CI, and total cortisol Saxon Merino ram wool samples.
Figure 4 represents both thermal indices and their variations throughout the year, these variations are plotted on same axis against the varying cortisol levels in the Merino sheep cortisol. Similarities in patterns of either plot is expected to be representative of causation between thermal indices and cortisol levels in Merino sheep wool.
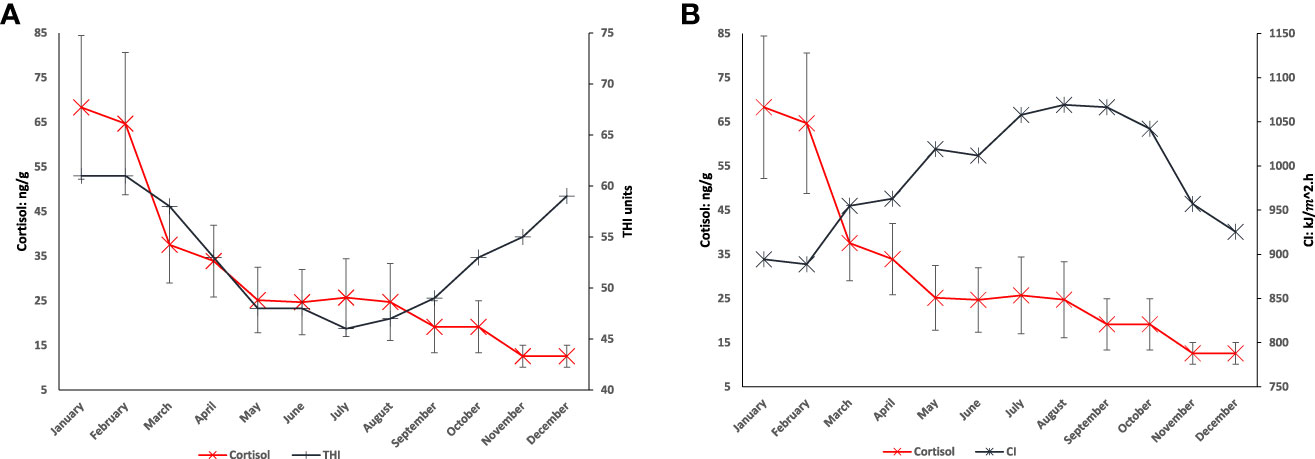
Figure 4 (A) displays THI (THI units) levels throughout the year, in contrast to cortisol levels (ng/g, with error bars) for the 20 sheep throughout the year. Similarity in patterns at start of year until May with both THI (THI units) levels and cortisol levels (ng/g) decreasing rapidly, and then different patterns until December. (B) displays CI (kj/m2.h) levels throughout the year in contrast to 20 sheep cortisol levels (ng/g) (with error bars) throughout the year. No obvious pattern similarities January to May, patterns are similar from May until December.
Discussion
The focus of this study was to determine the relationship between wool phenotype quality, and changes in wool cortisol as an index of physiological stress and climatic indices, using data from Saxon Merino Rams in Tasmanian highlands. Results do not indicate any direct causality between cortisol levels present in the Merino wool samples, although there was evidence to support that there was some impact on wool phenotype quality. When assessing the wool phenotype there are mitigating factors to be discussed that can affect linear correlation calculations and effects on wool phenotype quality.
Saxon Merino ram wool cortisol
Analysis of the cortisol levels in the Saxon Merino ram wool samples displayed significant variations in the wool cortisol levels, as was expected for the data set (Dunicic et al., 2019). These results were expected due to factors attributed to social status (dominant rams) and mating seasons. Dominant rams in the flock experience less stress due to elevated testosterone levels; typically rams have lower cortisol levels than females due to testosterone presence (Carcangiu et al., 2008). The presence of active gonads can alter the way the hypothalamic pituitary adrenal axis responds and produces less cortisol and bypasses stress initiate procedures (Van Lier et al., 2003). No castration recorded and natural processes assumed, with this consideration, testosterone presence can aid in restoring homeostasis or establishing allostasis in sheep undergoing stress, further studies are required to understand this relationship.
Moderate cortisol levels may be due to existing stress associated with pre-shearing activities, including microclimate changes before shearing. This leads to the initiation of acute stressors caused by slight variations in ambient temperature or environmental changes that further stressors can exacerbate (Verbeek et al., 2012; Bhimte et al., 2018; Lees et al., 2020). Cold stress following shearing can increase metabolism by up to 30% for up to five months (Mcgregor et al., 2011). Increased feed intake during cold weather generates an increase in endogenous heat production during the digestion process, accommodating an allostatic condition (McManus et al., 2020).
Price and wool cortisol
There was no correlation between wool cortisol due to stress and the price index of the Saxon Merino ram wool samples. However, there was a relationship between stress cortisol levels and coarse edge measurement (CEM) values, the only recorded causal relationship in this study. Indicating a causal relationship between wool cortisol and wool phenotypes exists. Although cortisol and CEM causality exists there may be other factors contributing to the effect on wool phenotype quality, which are beyond the focus of this study. There are other phenotypes that more substantially affect the wool phenotype quality, including fibre diameter (FD) and greasy fleece weight (GFW) (Mcgregor et al., 2011). These features can be influenced indirectly by both cortisol and weather, although the interaction between them is not clear.
In the elevated regions of New Zealand where the climate is characterised by low rainfall, cold temperatures, and harsh winters with poor foraging availabilities the average FD is 16 microns (Gubbins, 2014). These conditions are comparable to Tasmania Midlands with low rainfall, elevated landscapes, cold temperatures, and harsh winters that are indicated by CI 3 level for five months above 1000 kJ/m2/hour, but with better foraging than New Zealand (Gubbins, 2014). Fibre diameter can be affected by the physiological responses that accompanies cold stress, such as increased metabolism, and feed consumption. Although the average FD in this study from Tasmania midlands was 18.63 µm, ± 0.28 µm (Table 1), which is still in the fine wool range, it is thicker than wool under comparable conditions of elevated regions in New Zealand (Gubbins, 2014). Increase in feeding, to produce endothermic activity in the digestive process, increases energy intake that can expand the sheep-skin area with no new follicle formations. The existing follicles are spread further apart, and follicle efficiency succeeds in wool development with thicker FD, a feature common in older sheep (Rogers, 2006; Mcgregor et al., 2011). This effect can contribute to the Merino wool phenotype quality and affect correlation results. There is an average of 4.23 kg GFW for the Saxon Merino rams in the current study, and this implies there are contaminants in the Saxon Merino ram wool (Southcott and Royal, 1971; Fabre-Nys et al., 2015). Southcott and Royal (1971) state there is an average increase of 0.25 kg GFW and 0.13 kg CFW following the administration of 2 x 70 mg testosterone treatments, in Merino wethers. However, this induces higher testosterone levels than during mating. Dominant rams, that are mating could produce testosterone levels that surpass homeostatic ram testosterone levels (Rosa and Bryant, 2003; Van Lier et al., 2003; Carcangiu et al., 2008).
Thermal indices and cortisol
The THI and cortisol display no probability with the p-value and no direct causation with the chart alignment comparisons, indicating no causality between THI and cortisol levels in Saxon Merino sheep in this study. This is supported where the THI levels are consistently below the minimal THI impact threshold, thus no effect expected or observed by the THI on cortisol levels. The CI and cortisol display seasonal cortisol reduction aligned with the mating season in (Figure 4A), where mating season increases testosterone production and reduction of cortisol production in the endocrine system (Van Lier et al., 2003). In both plots in Figure 4, the cortisol level is higher at the start of the year, this may be due to shearing or other husbandry events producing stress that were not in effect at other times of the year. Following the mating season in both 4A and 4B the cortisol level significantly reduces; the THI and cortisol plot displays no similarities after that. However, the CI and cortisol plot displays similarities in the fluctuations of cortisol levels to CI levels (Figure 4B). Pattern behaviour following mating season suggests no acclimatisation process occurred in the sheep (Collier et al., 2019).
The p-value >0.05 for the CI and cortisol causality regression analysis, can be explained by the high variations within and between individual variations in cortisol profiles of the Saxon Merino rams. Adaptation and acclimatisation features of the individual rams can also affect regression calculations (Purvis and Franklin, 2004; Nienaber and Hahn, 2007; Gaughan et al., 2017). Adaptive or acclimatisation mechanisms such as dominant rams with high testosterone levels, or where a sheep eats excessively to overcome the cold, samples of cortisol, with average cortisol below 5 ng/g for the year (Carcangiu et al., 2008; Weaver et al., 2021). The biological clock mechanism frequently resets to maintain synchrony for changes in day length and temperature, which assists with allostasis (Silva and Minomo, 1995). Testosterone’s presence may contribute to the adaptation and promotes development as an adaptation mechanism, which can restore homeostasis with allostatic methods as the biological clock resets homeostasis (Silva and Minomo, 1995). Biological clock activities would affect the stress cortisol and thermal index correlations that are not defined.
The average CI level was above CI 3 for 9 months during 2021, this is indicative that the sheep experienced chronic stress and elevated cortisol levels at the Tasmanian Midlands site. This supports the observation by Weaver et al. (2021) of a 25% reduction in the growth of sheep wool with elevated cortisol levels in sheep. These were observed with an SL range in this study from 70 to 90 mm compared to the expected 120 mm growth as stated by Downes and Sharry (1971). This study indicates much higher levels of stress experienced by Saxon Merino Rams than the Merino ewes in the study by Weaver et al. (2021). Despite no causality between cortisol and wool phenotypes or thermal indices, weather can still affect the quality of Saxon Merino sheep wool. This suggests further study into seasonal variation in wool cortisol and Saxon Merino ram wool growth reduction.
Conclusion
This study investigated the relationship(s) between wool cortisol, wool phenotype, and climate indices. The results indicate that cortisol levels in the Saxon Merino wool samples vary seasonally. Regression analysis indicates that wool cortisol and price are not causally related, which is logical given that other post-harvest factors such as global market status influences price index. However, there are significant results in cortisol and CEM regression analysis, suggesting potential effects of on-farm stress on the overall quality and price. Thermal indices and cortisol regressions did not indicate significant cause and effect exists between the two variables. Regression analysis suggests that the Saxon Merino rams’ seasonal activities and the stud’s routines provide mitigating factors against the effects of stress with limited results. Direct causality was not detected between environmental conditions and cortisol, indirect effects of stress cortisol presence and climate conditions produced effects on phenotypes that can alter wool phenotype quality. If this study was repeated, more data from the Merino stud operator would be required on husbandry procedures. The recommendation for further research is to acclimate Merino sheep to changing conditions, which is aimed at developing the adaptation of Merino sheep to climatic variables.
Data availability statement
The original contributions presented in the study are included in the article/supplementary material. Further inquiries can be directed to the corresponding author.
Ethics statement
The animal studies were approved by The University of Queensland (UQ) Animal Care and Ethics Committee. The studies were conducted in accordance with the local legislation and institutional requirements. Written informed consent was obtained from the owners for the participation of their animals in this study.
Author contributions
EN conceptualised this study and supervised TC. G-CH conducted most of the lab work. All authors contributed to the article and approved the submitted version.
Funding
The author(s) declare financial support was received for the research, authorship, and/or publication of this article. The Australian Wool Education Trust awarded scholarship to Troy Cobb to engage in sheep, wool and related research, in 2022.
Acknowledgments
We would like to acknowledge the contributions made by The Australian Wool Education Trust (AWET) for the scholarship and partial funding for this project. This research was conducted as part of Masters of Animal Science research project for Troy Cobb and supervised by Dr Edward Narayan with support provided by Professor John Gaughan during manuscript draft stages. Georgia Constantina Hantzopoulou conducted the majority of the laboratory analysis of the wool samples. We would like to acknowledge the assistance of Harsh Pahuja, Gregory Sawyer, Janet Bell (Australian Alpaca Fibre Testing) and NSW Stud Merino Breeders Association, Australia.
Conflict of interest
The authors declare that the research was conducted in the absence of any commercial or financial relationships that could be construed as a potential conflict of interest.
Publisher’s note
All claims expressed in this article are solely those of the authors and do not necessarily represent those of their affiliated organizations, or those of the publisher, the editors and the reviewers. Any product that may be evaluated in this article, or claim that may be made by its manufacturer, is not guaranteed or endorsed by the publisher.
References
Adenkola A. Y., Ayo J. O. (2010). Physiological and behavioural responses of live- stock to road transportation stress: a review. Afr. J. Biotechnol. 9 (31), 4845–4856.
Aryal J. P., Saptoka T. B., Khurana R., Khatri-Chhetri A., Rahat D. B., Jat M. L. (2019). Climate change and agriculture in South Asia: adaptation options in smallholder production systems. Environment Dev. Sustainability 22, 5045–5075. doi: 10.1007/s10668-019-00414-4
Bhimte A., Thakar N., Yadav V., Khore A., Lakhani P. (2018). Endocrine changes in livestock during heat and cold stress. J. Pharmacognosy Phytochem. 7 (4), 127–132.
Black P. F., Murray J. G., Nunn M. (2008). Managing animal disease risk in Australia: the impact of climate change. Rev. Scientifique Technique (International Office Epizootics) 27 (20), 563–580. doi: 10.20506/rst.27.2.1815
Breen K. M., Oakley A. E., Pytiak A. V., Tilbrook A. J., Wagenmaker E. R., Karsch F. J. (2007). Does cortisol acting via the type II glucocorticoid receptor me- diate suppression of pulsatile luteinizing hormone secretion in response to psychosocial stress? Endocrinology 148 (4), 1882–1890. doi: 10.1210/en.2006-0973
Bureau of Meteorology (2022a) Annual mean maximum temperature, Ross (The Boulevards). Available at: http://www.bom.gov.au/jsp/ncc/cdio/weatherData/av?p_display_type=dataGraph.
Bureau of Meteorology (2022b) Annual Rainfall, Ross (The Boulevards). Available at: http://www.bom.gov.au/jsp/ncc/cdio/weatherData/av?p_display_type=dataGraph.
Bureau of Meteorology (2022c) Ross, Tasmania, January 2021 Daily Weather Observations. Available at: https://webarchive.nla.gov.au/awa/20211210042524/http://www.bom.gov.au/climate/dwo/202101/html/IDCJDW7042.202101.shtml.
Bureau of Meteorology (2022d) Ross, Tasmania, February 2021 Daily Weather Observations. Available at: http://www.bom.gov.au/climate/dwo/202102/pdf/IDCJDW7042.202102.pdf.
Bureau of Meteorology (2022e) Ross, Tasmania, March 2021 Daily Weather Observations. Available at: http://www.bom.gov.au/climate/dwo/202103/pdf/IDCJDW7042.202103.pdf.
Bureau of Meteorology (2022f) Ross, Tasmania, April 2021 Daily Weather Observations. Available at: http://www.bom.gov.au/climate/dwo/202104/html/IDCJDW7042.202104.shtml.
Bureau of Meteorology (2022g) Ross, Tasmania, May 2021 Daily Weather Observations. Available at: http://www.bom.gov.au/climate/dwo/202105/html/IDCJDW7042.202105.shtml.
Bureau of Meteorology (2022h) Ross, Tasmania, June 2021 Daily Weather Observations. Available at: http://www.bom.gov.au/climate/dwo/202106/html/IDCJDW7042.202106.shtml.
Bureau of Meteorology (2022i) Ross, Tasmania, July 2021 Daily Weather Observations. Available at: http://www.bom.gov.au/climate/dwo/202107/html/IDCJDW7042.202107.shtml.
Bureau of Meteorology (2022j) Ross, Tasmania, August 2021 Daily Weather Observations. Available at: http://www.bom.gov.au/climate/dwo/202108/html/IDCJDW7042.202108.shtml.
Bureau of Meteorology (2022k) Ross, Tasmania, September 2021 Daily Weather Observations. Available at: http://www.bom.gov.au/climate/dwo/202109/html/IDCJDW7042.202109.shtml.
Bureau of Meteorology (2022l) Ross, Tasmania, October 2021 Daily Weather Observations. Available at: http://www.bom.gov.au/climate/dwo/202110/html/IDCJDW7042.202110.shtml.
Bureau of Meteorology (2022m) Ross, Tasmania, November 2021 Daily Weather Observations. Available at: http://www.bom.gov.au/climate/dwo/202111/html/IDCJDW7042.202111.shtml.
Bureau of Meteorology (2022n) Ross, Tasmania, December 2021 Daily Weather Observations. Available at: http://www.bom.gov.au/climate/dwo/202112/html/IDCJDW7042.202112.shtml.
Bureau of Meteorology (2022o) Ross, Tasmania, yearly climate averages for 2021. Available at: http://www.bom.gov.au/climate/averages/tables/cw_093053.shtml.
Carcangiu V., Vacca G. M., Parmeggiani A., Mura M. C., Pazzola M., Dettori M. L., et al. (2008). The effect of shearing procedures on blood levels of growth hormone, cortisol and other stress haematochemical parameters in Sarda sheep. Animal 2 (4), 1–7. doi: 10.1017/S1751731108001651
Carter H. B., Clarke W. H. (1957). Hair follicle group and skin follicle population of Australian Merino sheep. Aust. J. Agric. Res. 8, 91–108. doi: 10.1071/AR9570091
Ciani E., Lasagna E., Andrea M. (2015). Merino and Merino-derived sheep breeds: a genome-wide intercontinental study. Genet. Selection Evol. 64), 47–47. doi: 10.1186/s12711-015-0139-z
Collier R. J., Baumgard L. H., Zimbelman R. B., Xiao Y. (2019). Heat stress: physiology of acclimation and adaptation. Anim. Front. 9 (1), 12–19. doi: 10.1093/af/vfy031
Donnelly J. R. (1984). The productivity of breeding ewes grazing on lucerne or grass and clover pastures on the tablelands of Southern Australia. III. Lamb mortality and weaning percentage. Aust. J. Agric. Res. 35, 709–721. doi: 10.1071/AR9840709
Downes A. M., Sharry L. F. (1971). Measurement of wool growth and its response to nutritional changes. Aust. J. Biol. Sci. 24, 117–130. doi: 10.1071/BI9710117
Dunicic D., Benic M., Zaga I. Z., Valpotic H., Samardzija M. (2019). Influence of season rainfall and air temperature on the reproductive efficiency in Romanov sheep in Croatia. Int. J. Biometeorology 63, 817–824. doi: 10.1007/s00484-019-01696-z
Fabre-Nys C., Kendrick K. M., Scaramuzzi R. J. (2015). The "ram effect": new insights into neural modulation of the gonadotropic axis by male odors and socio-sexual interactions. Front. Neurosci. 9 (111). doi: 10.3389/fnins.2015.00111
Gaughan J. B., Lees A. M., Sejian V. (2017). Sixty years of animal biometeorology. Int. J. Biometeorology 64, 157–163. doi: 10.1007/s00484-017-1459-1
Gubbins T. (2014). Maximising the Reproductive Potential of the Merino: From conception to weaning. Nuffield Aust. Project No 1419., 1–36.
Haidary A. (2004). (PDF) Physiological responses of Naimey Sheep to heat stress challenge under semi-arid environments. Int. J. Agric. Biol. 8530 (2), 6–8.
Hantzopoulou G. C., Sawyer G., Tilbrook A., Narayan E. (2022). Intra-and Inter-sample variation in wool cortisol concentrations of Australian Merino lambs between twice or single shorn ewes. Front. Anim. Sci. 37. doi: 10.3389/fanim.2022.890914
Holman B. W. B., Malau-Aduli A. E. O. (2012). A review of sheep wool quality trait. Annu. Rev. Res. Biol. 2 (1), 1–14.
Islam R., Biswas J. H., Akanda G. R., Amin R., Hasan I. M., Al-Asif A., et al. (2015). Altitude of farmers towards climate change effect on agriculture. Asian J. Med. Biol. Res. 1 (2), 367–379. doi: 10.3329/ajmbr.v1i2.25631
Killen S. S., Mara S., Metcalfe N. B., Mckenzie D. J., Domenici P. (2013). Environmental stressors alter relationships between physiology and behaviour. Trends Ecol. Evol. 28 (11), 651–665. doi: 10.1016/j.tree.2013.05.005
Knight A. (2020). Should NEW ZEALAND do more to uphold animal welfare? Anim. Stud. J. 9 (1), 114–149.
Kumar S., Kumar B. V., Meena A., Meena K. (2010). Effect of heat stress in tropical livestock and different strategies for its amelioration. J. Stress Physiol. Biochem. 7 (1), 45–54.
Lees A. M., Sullivan M. L., Olm J. C. W., Cawdell-Smith A. J., Gaughan J. B. (2020). The influence of heat load on Merino sheep. 1. Growth, performance, behaviour and climate. Anim. Production Sci. 60, 1925–1931. doi: 10.1071/AN19687
Macpherson S. (2008). Wool classing. Wool marketing & Clip preparation, Melbourne, Australia: Woolwise, Australian Wool Education Trust. Viewed on 28th May 2022.
Marai I. F. M., El-Darawany A. A., Fadiel A., Hafez M. A. M. (2008). Reproductive performance traits as affected by heat stress and its alleviation in sheep. Trop. Subtropical Agroecosystems 8 (3), 209–234. doi: 10.1016/j.smallrumres.2006.10.003
Mcgregor B. A., Graaf S. P. D., Hatcher S. (2011). On farm factors affecting physical quality of Merino wool. 1. Nutrition, reproduction, health and management. Small Ruminant Res. 137, 138–150. doi: 10.1016/j.smallrumres.2016.03.019
Mckeon G., Stone G., Ahrens D., Carter J., Chen D., Irvine S., et al. (2021). Queenslands multi-year Wet and Dry period: implications for grazing enterprises and pasture resources. Rangeland J. 43, 121–142. doi: 10.1071/RJ20089
McManus C. M., Faria D. A., Lucci C. M., Louvandini H., Pereira S. A., Paiva S. R. (2020). Heat stress effects on sheep: Are hair sheep more heat resistant? Theriogenology 155, 157–167. doi: 10.1016/j.theriogenology.2020.05.047
Narayan E., Parisella S. (2017). Influences of the stress endocrine system on the reproductive endocrine axis in sheep (Ovis aries). Ital. J. Anim. Sci. 16 (4), 640–651. doi: 10.1080/1828051X.2017.1321972
Narayan E., Sawyer G., Parisella S. (2018). Faecal glucocorticoid metabolites and body temperature in Australian Merino ewes (Ovis aries) during summer artificial insemination (A.I.) program. PloS One 13 (1), 191961–191961. doi: 10.1371/journal.pone.0191961
Nejad J. G., Lohakare J. D., Son J. K., Kwon E. G., West J. W., Sung K. I. (2014). Wool cortisol is a better indicator of stress than blood cortisol in ewes exposed to heat stress and water restriction. Animal 8 (1), 128–132. doi: 10.1017/S1751731113001870
Nel C. L., Cloete S. W. P., Kruger A. G. M., Dzama K. (2021). Long-term genetic selection for reproductive success affects neonatal lamb vitality across cold stress conditions. J. Thermal Biol. 98, 1–9. doi: 10.1016/j.jtherbio.2021.102908
Nienaber J. A., Hahn G. L. (2007). Livestock production system management responses to thermal challenges. Int. J. Biometeorology 52, 149–157. doi: 10.1007/s00484-007-0103-x
Pluske J. M., Slade A. M., Vercoe P. E. (2010). Weather and Wethers: effects of wind temperature and rain on sheep feedlot production. Australas. Agribusiness Rev, 193–214. doi: 10.22004/AG.ECON.114334
Purvis I. W., Franklin I. R. (2004). Major genes and QTL influencing wool production and quality: a review. Genet. Selection Evol. 37 (1), 97–107. doi: 10.1186/1297-9686-37-S1-S97
Rashamol V. P., Sejian V., Bagath M., Krishnan G., Archana P. R., Bhatta R. (2018). Physiological adaptability of livestock to heat stress: an updated review. J. Anim. Biometeorology 6, 62–71. doi: 10.31893/2318-1265jabb.v6n3p62-71
Rogers G. E. (2006). Biology of the wool follicle, an excursion into a unique tissue interaction system waiting to be re-discovered. Experi- Ment. Dermatol. 15 (12), 931–949. doi: 10.1111/j.1600-0625.2006.00512.x
Rosa H. J. D., Bryant M. J. (2003). Seasonality of reproduction in sheep. Small Ruminant Res. 48 (3), 155–171. doi: 10.1016/S0921-4488(03)00038-5
Sawyer G., Fox D. R., Narayan E. (2021). Pre and post partum variation in wool cortisol and wool micron in Australian Merino sheep (ovis aries). PeerJ 9, 1–17. doi: 10.7717/peerj.11288
Sawyer G., Narayan E. (2019). “A review on the influence of climate change on sheep production,” in Comparative Endocrinology of Animals. Ed. Narayan E. (Books on Demand, British library cataloging in publication data). 2019
Silmen I. B., Najar J., Ghram A., Abdrrabba M. (2016). Heat stress effects on livestock molecular, cellular and metabolic aspects, a review. J. Anim. Physiol. Anim. Nutr. 100, 401–412. doi: 10.1111/jpn.12379
Silva D., Minomo RG R. F. (1995). Circadian and seasonal variation of the body temperature of sheep in a tropical environment. Int. J. Biometeorology 39, 69–73. doi: 10.1007/BF01212583
Southcott W. H., Royal W. M. (1971). Effect of implanted testosterone propionate on the growth and wool production of Merino wethers. Aust. J. Aust. Res. 22 (2), 271–282. doi: 10.1071/AR9710271
Srikandakumar A., Johnson E. H., Mahgoub O. (2003). Effect of heat stress on respiratory rate, rectal temperature and blood chemistry in Omani and Australian Merino sheep. Small Ruminant Res. 49, 193–198. doi: 10.1016/S0921-4488(03)00097-X
Tattersall G. J., Sinclair B. J., Withers P. C., Fields P. A., Seebacher F., Cooper C. E., et al. (2012). Coping with thermal challenges: Physiological adaptations to environmental temperatures. Compr. Physiol. 2 (3). doi: 10.1002/cphy.c110055
Thom E. C. (1959). The discomfort index. Weatherwise 12 (2), 57–61. doi: 10.1080/00431672.1959.9926960
Tilbrook A. J., Canny B. J., Serapiglia M. D., Ambrose T. J., Clarke I. J. (1999). Suppression of the secretion of luteinizing hormone due to isolation/restraint stress in gonadectomised rams and ewes is influenced by sex steroids. J. Endocrinol. 160 (3), 469–481. doi: 10.1677/joe.0.1600469
Tilbrook A. J., Clarke I. J. (2006). Neuroendocrine mechanisms of innate states of attenuated responsiveness of the hypothalamo-pituitary adrenal axis to stress. Front. Neuroendocrinol. 27 (3), 285–307. doi: 10.1016/j.yfrne.2006.06.002
Tilbrook A. J., Ralph C. R. (2016). Hormones, stress and the welfare of animals. Anim. Production Sci. 58 (3), 408–415. doi: 10.1071/AN16808
Ugurlu M., Teke B., Akdag F., Arslen (2014). Effect of temperature humidiy index, cold stress index and dry period length on birthweight of Jersey calf. Bulgarian J. Agric. Sci. 20 (5), 1227–1232.
Van Lier E., Perez-Clariget R., Forsberg M. (2003). Sex differences in cortisol secretion after administration of an ACTH analogue in sheep during the breeding season. Anim. Reprod. Sci. 79, 83–89. doi: 10.1016/S0378-4320(03)00083-6
Verbeek E., Oliver M. H., Waas J. R., Mcleay L. M., Blache D., Matthews L. R. (2012). Reduced cortisol and metabolic responses of thin ewes to an acute cold challenge in mid-pregnancy: implications for animal physiology and welfare. PLoS One 7 (5), 37315–37315. doi: 10.1371/journal.pone.0037315
Von Borell E. (2001). The biology of stress and its application to livestock housing and transportation. J. Anim. Sci. 79, 260–267. doi: 10.2527/jas2001.79E-SupplE260x
Weaver S. J., Hynd P. I., Ralph C. R., Hocking-Edwards J. E., Burnard C. L., Narayan E., et al. (2021). Chronic elevation of plasma cortisol causes differential expression of predominating glucocorticoid in plasma, saliva, fecal, and wool matrices in sheep. Domest. Anim. Endocrinol. 74, 106503–106503. doi: 10.1016/j.domaniend.2020.106503
Keywords: merino sheep, climatic stress, cortisol, wool phenotype, merino sheep stress, merino sheep stress mitigations
Citation: Cobb T, Hantzopoulou G-C and Narayan E (2023) Relationship between wool cortisol, wool quality indices of Australian Merino rams and climatic variables in Tasmania. Front. Anim. Sci. 4:1234343. doi: 10.3389/fanim.2023.1234343
Received: 04 June 2023; Accepted: 16 October 2023;
Published: 03 November 2023.
Edited by:
Mohan Mondal, ICAR-National Dairy Research Institute, IndiaReviewed by:
Severiano Silva, Universidade de Trás-os-Montes e Alto, PortugalAloma Zoratti, University of Udine, Italy
Claudia Giannetto, University of Messina, Italy
Copyright © 2023 Cobb, Hantzopoulou and Narayan. This is an open-access article distributed under the terms of the Creative Commons Attribution License (CC BY). The use, distribution or reproduction in other forums is permitted, provided the original author(s) and the copyright owner(s) are credited and that the original publication in this journal is cited, in accordance with accepted academic practice. No use, distribution or reproduction is permitted which does not comply with these terms.
*Correspondence: Edward Narayan, e.narayan@uq.edu.au; Troy Cobb, troy.cobb@uq.net.au
‡ORCID: Edward Narayan, orcid.org/0000-0003-2719-0900