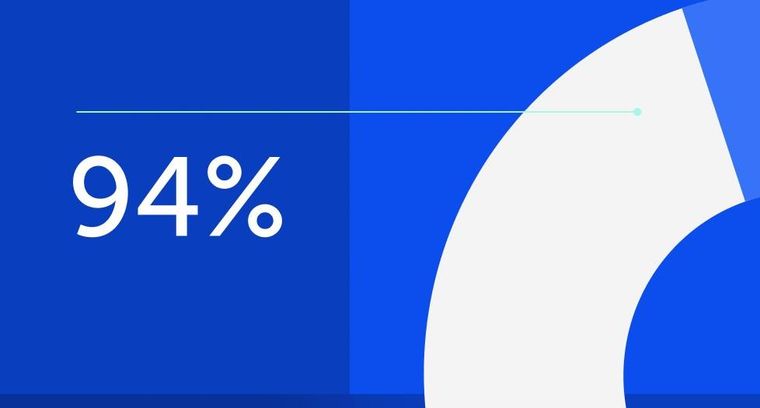
94% of researchers rate our articles as excellent or good
Learn more about the work of our research integrity team to safeguard the quality of each article we publish.
Find out more
BRIEF RESEARCH REPORT article
Front. Anim. Sci., 24 July 2023
Sec. Animal Nutrition
Volume 4 - 2023 | https://doi.org/10.3389/fanim.2023.1214076
In broilers, the effects of crude protein (CP) reduction on animal performance are heterogeneous. This could limit the use of this strategy in commercial farms despite its potential to improve the sustainability of production. The objective of this meta-analysis was to study the effect of lowering dietary CP in fast-growing broilers with a focus on growth performance. A database was built from 29 papers published after 2016, for a total of 106 trials and 268 treatments. To be included in the database, trials had to be iso-energy and iso-lysine. Trials in which the growth rate for the control treatment was below 90% of the genetic potential of the birds were not included. The effect of the CP level was analyzed by multiple linear regression, with the trial as a random effect. A subsample of 33 trials (AACON) met the recommended amino acid (AA)-to-lysine ratios for indispensable AAs. In this subdatabase, average daily gain and average daily feed intake were maintained when dietary CP was reduced. The feed conversion ratio increased by 1.3% when CP was reduced by a one percentage point. The same CP reduction decreased daily nitrogen (N) excretion by 10.4%, whereas N retention was not affected by CP. In conclusion, respecting the ideal protein concept with the use of feed-grade AA allows for a decrease in dietary CP and N excretion with a limited impact on growth performance.
The world population is growing, with a projected 9.5 billion inhabitants by 2050 (UN, 2019). The demand for chicken meat, which is the most consumed meat in the world (FAO, 2020), is expected to increase by 13% by 2031 (OCDE, 2020). At the same time, humanity is facing an unprecedented climatic and environmental crisis. For example, the Earth’s surface temperature has risen faster in the last 50 years than in the previous 2,000 years, mainly as a result of the increased production of anthropogenic greenhouse gases (GHGs; IPCC, 2021). Although poultry production accounts for only 8.7% of GHGs produced by the livestock sector, which in turn accounts for 14.5% of global human-induced GHG emissions, the poultry sector must implement new strategies to continue to meet the demand for animal protein without exacerbating the environmental crisis (Gerber et al., 2013). Environmental impacts of the broiler production are mainly related to the feed production stage (67%–78% of climate change, 55%–69% of energy use, 52%–70% of eutrophication, and 25%–55% of acidification, (Leinonen et al., 2012; da Silva et al., 2014; Méda et al., 2021). Nutrient losses to the environment from manure management also cause significant environmental impacts (Gerber et al., 2013). Broiler chickens excrete approximately 40%–60% of the nitrogen (N) they ingest, and up to 50% of this excreted N can be lost through volatilization (Guiziou and Béline, 2005; Wiedemann et al., 2016; Belloir et al., 2017).
Therefore, lowering the dietary crude protein (CP) content could be a potential lever to reduce environmental impacts (Méda et al., 2011; Gerber et al., 2013; Greenhalgh et al., 2020). In many countries, lowering the dietary CP content results in reduced use of soybean meal (SBM) from South America, which is associated with high environmental impacts (Dalgaard et al., 2008). Decreasing CP also reduces N losses through volatilization, reducing the impacts associated with ammonia (NH3) and nitrous oxide (N2O) emissions (Méda et al., 2017; Van Emous et al., 2019). A decrease in NH3 emissions also improves the respiratory health of birds and workers (Naseem and King, 2018). Finally, reducing dietary CP content can also improve gut health (Qaisrani et al., 2015; Luise et al., 2021) and decrease the occurrence of pododermatitis (Minussi et al., 2021). However, growth performance in response to CP is heterogeneous: there is no unequivocal decrease or increase (Alfonso-Avila et al., 2022; Fraysse et al., 2015). This is likely a result of the amino acids (AAs) that are not always controlled when reducing CP and become limiting when CP content decreases. These include AAs that are less studied such as glycine, histidine, or phenylalanine (Kidd et al., 2021). A meta-analysis was carried out, based on recent studies in fast-growing broilers, to test the low-CP strategy while controlling essential AAs with the addition of free (crystalline or synthetic) AAs.
A literature search was conducted on the public databases Web of Science™ (Clarivate™, Philadelphia, PA, USA) and PubMed® (National Library of Medicine, Bethesda, MD, USA). The following keywords were used to search titles and abstracts: chicken(s), broiler(s), Ross, Cobb, reduce(d) protein diets, reduce(d) CP, low protein, low CP, levels of CP, and protein dilution. To be included in the data set, the trials had to use diets formulated with the same levels of digestible lysine (digLys) and apparent metabolizable energy (AME) or nitrogen-corrected AME (AMEn) within each trial. Trials were removed if the daily Lys intake of the control broilers deviated by more than 20% from the recommended Lys intake, which is derived from Hauschild et al. (2015). Only trials using fast-growing strains (Ross 308 or Cobb 500) were considered, and the bird performance in the control treatment was required to be at least 90% of the genetic potential of the breeder’s guidelines. In addition, feed composition, experimental conditions (bird variety, sex, age, ambient temperature, etc.), and growth performance had to be detailed. Using these selection criteria, a general database (GEN-database) was built with 29 publications from 2016 to 2021, regrouping 106 trials and 268 treatments (see Supplementary Material). The database contained qualitative data such as strain and sex, and qualitative data such as CP content and average daily gain (ADG). The nutrient composition of each diet was recalculated using INRAE feedstuff tables (INRAE-CIRAD-AFZ, 2018) to limit biases related to the use of different formulation matrices in the papers. A general description of the GEN database is provided in Table 1. A subdatabase of treatment where AAs were controlled (AACON-database) was also created, including 33 trials (79 treatments) of the original database (31%) where the levels of Met + Cys/Lys, Thr/Lys, Trp/Lys, Arg/Lys, Val/Lys, Ile/Lys, His/Lys, Gly + Ser/Lys, and Phe + Tyr/Lys were at 95% or above the reference requirements (Table 1). These requirements were calculated by considering digAA-to-digLys ratios taken from Rostagno et al. (2017). To recalculate the N balance (i.e., N intake, N retention, and N excretion), an average value of 29 g N retained/kg body weight gained (BWG) was considered (Belloir et al., 2017). As abdominal fat deposition was measured with different units (e.g., percentage of the live weight, percentage of the carcass), a fat deposition variable was created by aggregating the different fat deposition data expressed as a ratio of the control.
The effect of CP on the variables of interest was studied in both databases using Minitab® (2021) to conduct a multivariate analysis with the trial as a random effect:
where Yij is the value of the studied variable in trial i for diet j, µ is the global intercept, ai is the trial effect, b1 and b2 are the linear and quadratic coefficients of the CP effect, respectively, and Eij is the residual error. The linear model was used when the quadratic effect was not significant. The effect of CP was studied for several datasets. Finally, to ensure the quality of the CP response models, the normality of residuals, the independence of the error, and the homogeneity of the variance were tested. Effects were considered significant at a P-value< 0.05 and trends at a P-value< 0.10.
As stated previously, a fixed coefficient for N retention was used to calculate N balance, except in the 30 trials (81 treatments) that measured N retention. To ensure the accuracy of using a fixed coefficient, these 30 trials were used to compare the measured versus calculated N retention based on the intercept, the slope, and the R2 obtained between observed and predicted values. The mean square prediction error (MSPE) was broken down into error in central tendency (ECT), error due to regression (ER), and error due to disturbance (ED), as suggested by Pomar and Marcoux (2005); Theil (1966), and Bibby and Toutenburg (1977). The ECT indicates the extent to which the average of the predicted dependent variables deviates from the average of the observed values. The ER measures the deviation of the least squares regression coefficient from 1, which is the value obtained if the observed and simulated results were in complete agreement. The ED is the variation in the observed values that are not accounted for by least squares regression of the observed on predicted values. This error is the unexplained variance, and it represents the portion of MSPE that cannot be eliminated by linear transformation (Theil, 1966). In addition, relative MSPE (rMSPE), expressed as a percentage of the observed mean, was used as a measure of the prediction error.
All the birds in the selected publications were male. The origin of the articles in order of importance is Australia (34%, 42%), Europe (24%, 17%), Asia (21%, 17%), North America (14%, 17%), South America (3%, 0%), and the Middle East (3%, 8%) in the GEN-database and AACON-database, respectively. The differences between the two databases were less than 5% for average age, final age, CP content of control feed, AMEn content, and standardized ileal digestible (SID) Lys level (Table 1). The maximum CP reduction reached was 4.9% in the GEN-database and 3.2% in the AACON-database. The levels of AA : Lys were in average 5% lower in the GEN-database than in the AACON-database, with the largest differences being 9% for Gly + Ser : Lys, His : Lys, and Phe + Tyr : Lys (Supplementary Table 1).
In the GEN-database, the decrease in CP content was systematically achieved by a decrease in dietary SBM content (g/kg; SBM = −487.0 + 38.5 × CP; P< 0.001; R2 = 95%; rMSE = 23.9). Decreasing dietary CP content by one percentage point led to a decrease in SBM content of 39 g/kg (i.e., a decrease of 19%). As a consequence, because SBM was approximately 6.6 times richer in potassium (K) than corn (INRAE-CIRAD-AFZ, 2018), the decrease in SBM content led to a decrease in dietary K content (K = 4.53 + 0.013 × SBM; P< 0.001; R2 = 87%; rMSE = 0.587; i.e., a 7% decrease for a one percentage point CP reduction in feed). This resulted in the decrease of dietary electrolyte balance (DEB’ calculated using K+, Na+, and Cl− contents; Mongin, 1981) by 19.5 mEq/kg for the same CP reduction. Simultaneously, the cereal content increased by 32 g/kg in response to the reduction of SBM incorporation. Dietary starch content (g/kg) was correlated with cereal content (R2 = 63%; P< 0.001). Therefore, starch content increased by 5% for a dietary CP decrease of one percentage point (starch = 81.49 − 2.09 × CP; P< 0.001; R2 = 93%; rMSE = 1.67). Corn was the most used cereal, but 24 trials were conducted on a wheat basis without corn. For a one percentage point CP decrease, the synthetic AA content of the feed was increased by 5.2 g/kg (i.e., an increase of 28%).
In the GEN-database, the decrease in dietary CP content led to a linear (P< 0.001) and quadratic (P< 0.001) decrease in average daily feed intake (ADFI) and ADG (Figure 1; Table 2). In contrast, in the AACON-database, the dietary CP content did not affect ADFI and ADG.
Figure 1 Broiler within experiment responses of average daily feed intake, average daily gain, and feed conversion ratio using the general database (A–C) and the AACON-database where amino acid levels are controlled (D–F).
Table 2 Effect of crude protein (CP, in %) on animal performance, fat deposition, and nitrogen balance in the general database (GEN-database) and in the AACON-database where amino acid levels are controlled.
These changes in animal performance in the GEN-database led to a linear increase (P< 0.001) in the feed conversion ratio (FCR) (g/g) by 1.9% for a CP decrease of one percentage point. However, when the levels of digestible AA were controlled (AACON-database), this increase in FCR was lower (+1.2%). Lowering dietary CP content led to a linear increase in fat deposition in the form of abdominal deposition (Table 2) of 8.8% for a CP decrease of one percentage point in the GEN-database; in the AACON-database, based on 12 trials, lowering the CP content did not affect fat deposition (P = 0.152).
The daily N retained was predicted with good accuracy with the rMSPE (4%), mostly because of the disturbance (86%), followed by a central tendency error of 12% and a regression error of 2% (Figure 2). For a one percentage point reduction of dietary CP, the daily recalculated values for N intake, N retention, and N excretion were decreased by 4%, 2%, and 11%, respectively, in the GEN-database (P< 0.001; Table 2). In the AACON-database, a similar decrease in CP content decreased daily N intake by 6% (P< 0.001; Table 2), did not affect N retention, and decreased daily N excretion by 14% (P< 0.001). In both databases, N retention efficiency was increased by approximately 4% (P< 0.001).
Figure 2 The relationship between observed and predicted daily N retained, no. of observations = 81, relative mean square prediction error = 4%, error in central tendency = 12%, error due to regression = 2%, and error due to disturbance = 86%, x = y line (—) and regression line (–).
The effect of reducing CP on diet composition agreed with the results of the meta-analysis by Alfonso-Avila et al. (2022) focus on the N utilization, litter quality, and water consumption, which also showed that a CP reduction of 1% reduced SBM by 25 g/kg, dietary K content by 0.47 g/kg, and the DEB by 19.3 mEq/kg. In comparison, in the GEN-database, the SBM, dietary K, and DEB were reduced by 39 g/kg, 0.50 g/kg, and 19.5 mEq/kg, respectively. The higher decrease in SBM could be explained by a higher usage of free AAs. Despite a more rapid SBM reduction, the equivalent decrease in K and DEB can be explained by the use of K carbonate in some trials. The lowest K value was 3.3 g/kg, while requirements range from 6.3 g/kg to 7.1 g/kg (Oliveira et al., 2005). Very few trials have tested the impact of restoring K content in low-CP diets, except Lambert et al. (2023), who showed that K supplementation to restore DEB in low-CP diets improved growth performance. The fact that decreasing K decreases water consumption is an opportunity to improve litter quality. However, little research is available on the K requirements of chickens. It will therefore be important to determine the level of K to adopt in the LCP strategy, which will maximize litter quality without degrading growth performance.
In the GEN-database, decreasing CP induced a slight increase in ADFI and ADG until they reached a maximum (CP = 20.4% and 21.0%, respectively), after which performance decreased with decreasing CP. For example, reducing CP from 24% to 23% increased ADFI by 1.4%, whereas reducing CP from 19% to 18% reduced ADFI by 0.8%. This quadratic response is consistent with Alfonso-Avila et al. (2022), which did not use selection rules based on the AA level. It is interesting to note that approximately 56% of the trials showed an increase in ADFI, while 26% of the trials showed a concomitant increase in ADG, and 14% showed a positive effect on all growth parameters. In the AACON-database, ADFI and ADG were not impacted by CP levels, indicating that the effect observed in the GEN-database can be linked to a deficiency in AAs. According to the most accepted hypotheses on feed intake regulation, chickens consume to meet their most limiting nutrient (Emmans, 1987) but, in practice, multiple factors may limit the animal from reaching their desired feed intake, such as the bulkiness of the feed (Taylor and Kyriazakis, 2021). The decrease in CP caused a reduction in crude fiber content (Alfonso-Avila et al., 2022), and, therefore, in water-holding capacity, so bulk capacity does not seem to explain the decrease in feed intake in this case. The energy balance, in particular fat deposition, is also reported to drive feed intake (Gous et al., 1990). Trials were all formulated to be iso-AMEn; however, recent results have shown that energy digestibility increased with decreasing CP (Chrystal et al., 2020a; Maynard et al., 2021). It is therefore possible that this increase in AMEn coupled with the increase in fat deposition caused the decreased ADFI. Moreover, intake is regulated both in the short term (e.g., hormonal regulation) and long term (e.g., energetic balance; Bouvarel et al., 2010). For example, as a long-term regulation, the decreased intake observed in the GEN-database could be partly linked to the reduced growth performance, because feed intake is lower in smaller birds.
The current results show that maintaining the levels of Met + Cys, Thr, Trp, Arg, Val, Ile, His, Gly + Ser, and Phe + Tyr in the right ratio to Lys allowed a decrease in CP content without impairing animal performance. Therefore, in the AACON-database, dietary CP could be decreased to 16% without degrading growth performance. In addition, in some trials not included in the AACON-database because they did not control all of the essential AAs, a decrease in CP did not decrease growth performance (Figure 1). This shows that there is still work to be done to fully understand the success factors of CP reduction. The decrease in CP content also leads to the emergence of interest in Gly, His, and Phe + Tyr requirements that are usually not considered in feed formulation for broilers. For example, out of the 166 low-protein diets in the GEN-database, supplementation of Gly was done in 81 treatments, while 16 were supplemented with L-His and 34 with L-Phe. An increase in the FCR was observed in both databases when the CP was decreased. Degradation of the FCR was 37% lower in the CON-AA than in the GEN-database. Therefore, controlling AAs is crucial for successful results with decreased CP.
The data in this meta-analysis confirmed that abdominal fat is increased when CP content is decreased, as previously observed (Belloir et al., 2017; Chrystal et al., 2020b; Cowieson et al., 2020). Abdominal fat represents approximately 20% of total fat (Tůmová and Teimouri, 2010) and is correlated with carcass fat (Hood, 1982; Namroud et al., 2008). Because chicken fat has a low economic value, this fat deposition should be avoided (Fouad and El-Senousey, 2014). Our data indicate that fat deposition is lower when AAs are controlled (Supplementary Figure 1). Therefore, using AA-to-Lys ratios corresponding to the ideal protein profile limits fat deposition; however, this phenomenon still occurs, indicating that the effect of CP on fat deposition is not solely related to AA limitations. This is consistent with the results from Chendrimada et al. (2007), which showed the inhibitory effect of protein with non-essential AA (i.e., Ala and Glu) and mineral N, on the malic enzyme involved in fat deposition.
Our results confirmed that an average of 29 g N/kg BW gain can be used to recalculate the N balance. A single value for the N content of the gain can be used because the water and fat contents are negatively correlated (Liu et al., 2017; Caldas et al., 2019). The decrease in the N content of the gain that should have been caused by the increase in fat is compensated by a decrease in water content. When all essential AAs are controlled, lowering dietary CP content reduces daily N excretion by 14% and increases N retention efficiency by four percentage points for a CP decrease of between 190 g/kg and 180 g/kg (P< 0.001; Table 2). This decreased excretion is consistent with the 10% value reported by Méda et al. (2011). The combination of reduced N excretion and decreased volatility of the N excreted has been shown to lead to a synergetic decrease in total N volatilization in a broiler house (Belloir et al., 2017). To further assess the environmental effects, emissions of nitrogenous gases, such as NH3 and N2O, should be studied to determine how they are specifically impacted by the reduction in dietary CP.
In conclusion, a decrease in dietary CP content, without impairing animal performance, is possible when the levels of indispensable AA are precisely controlled. This strategy can also reduce the need for imported SBM and limit N emissions.
The raw data supporting the conclusions of this article will be made available, on demand, by the authors, without undue reservation.
The animal study was reviewed and approved by the Comité de protection des animaux de l”Université Laval (CPAUL) and the Comité de protection des animaux du Center de recherche en sciences animales de Deschambault (CPA-CRSAD).
TR built the database, performed the graphical and statistical analysis, and wrote the first draft of the manuscript. M-PL-M was the PI of the project. All authors contributed to the article and approved the submitted version.
This study was carried out with the financial support of Agriscience (grant number ASP-136) and Metex animal nutrition. The funding sources had no involvement in study design, collection, analysis, interpretation of data, report writing, or in the decision to submit this article for publication.
Authors SF and WL were employed by company METEX Animal Nutrition.
The remaining authors declare that the research was conducted in the absence of any commercial or financial relationships that could be construed as a potential conflict of interest.
All claims expressed in this article are solely those of the authors and do not necessarily represent those of their affiliated organizations, or those of the publisher, the editors and the reviewers. Any product that may be evaluated in this article, or claim that may be made by its manufacturer, is not guaranteed or endorsed by the publisher.
The Supplementary Material for this article can be found online at: https://www.frontiersin.org/articles/10.3389/fanim.2023.1214076/full#supplementary-material
Alfonso-Avila A. R., Lambert Q. C. W., Letourneau-Montminy M. P. (2022). Effect of low-protein diets on nitrogen utilization and litter quality in broiler chicken production: insight from meta-analysis. Animal 16 (3), 100458. doi: 10.1016/j.animal.2022.100458
Belloir P., Méda B., Lambert W., Corrent E., Juin H., Lessire M., et al. (2017). Reducing the CP content in broiler feeds: impact on animal performance, meat quality and nitrogen utilization. Animal 11 (11), 1881–1889. doi: 10.1017/S1751731117000660
Bibby J., Toutenburg H. (1977). Prediction and improved estimation in linear models (New York, NY: J. Wiley & Sons). Available at: https://lccn.loc.gov/76007533.
Bouvarel I., Tesseraud S., Leterrier C. (2010). L'ingestion chez le poulet de chair: n'oublions pas les régulations à court terme. INRA Productions Animales 23 (5), 391–404. doi: 10.20870/productions-animales.2010.23.5.3317
Caldas J. V., Boonsinchai N., Wang J., England J. A., Coon C. N. (2019). The dynamics of body composition and body energy content in broilers. Poult. Sci. 98 (2), 866–877. doi: 10.3382/ps/pey422
Chendrimada T. P., Freeman M. E., Davis A. J. (2007). Dietary nitrogen intake regulates hepatic malic enzyme messenger ribonucleic acid expression. Poult. Sci. 86 (9), 1980–1987. doi: 10.1093/ps/86.9.1980
Chrystal P. V., Moss A. F., Khoddami A., Naranjo V. D., Selle P. H., Liu S. Y. (2020a). Impacts of reduced-crude protein diets on key parameters in male broiler chickens offered maize-based diets. Poult. Sci. 99 (1), 505–516. doi: 10.3382/ps/pez573
Chrystal P. V., Moss A. F., Yin D., Khoddami A., Naranjo V. D., Selle P. H., et al. (2020b). Glycine equivalent and threonine inclusions in reduced-crude protein, maize-based diets impact on growth performance, fat deposition, starch-protein digestive dynamics and amino acid metabolism in broiler chickens. Anim. Feed Sci. Technol. 261, 114387. doi: 10.1016/j.anifeedsci.2019.114387
Cowieson A. J., Perez-Maldonado R., Kumar A., Toghyani M. (2020). Possible role of available phosphorus in potentiating the use of low-protein diets for broiler chicken production. Poult. Sci. 99 (12), 6954–6963. doi: 10.1016/j.psj.2020.09.045
Dalgaard R., Schmidt J., Halberg N., Christensen P., Thrane M., Pengue W. A. (2008). LCA of soybean meal. Int. J. Life Cycle Assess. 13 (3), 240–254. doi: 10.1065/lca2007.06.342
da Silva V. P., van der Werf H. M., Soares S. R., Corson M. S. (2014). Environmental impacts of French and Brazilian broiler chicken production scenarios: an LCA approach. J. Environ. Manage. 133, 222–231. doi: 10.1016/j.jenvman.2013.12.011
Duraffourd C., De Vadder F., Goncalves D., Delaere F., Penhoat A., Brusset B., et al. (2012). Mu-opioid receptors and dietary protein stimulate a gut-brain neural circuitry limiting food intake. Cell 150 (2), 377–388. doi: 10.1016/j.cell.2012.05.039
Emmans G. C. (1987). Growth, body composition and feed intake1. World's Poultry Sci. J. 43 (3), 208–227. doi: 10.1079/WPS19870014
FAO statistique. (2020). Food and Agriculture Organization of the United Nations, en ligne. Available at: https://www.fao.org/faostat/fr/#home.
Fouad A. M., El-Senousey H. K. (2014). Nutritional factors affecting abdominal fat deposition in poultry: a review. Asian-Australasian J. Anim. Sci. 27 (7), 1057–1068. doi: 10.5713/ajas.2013.13702
Fraysse P., Lessire M., Van Milgen J., Schmidely P., Corrent E., Tesseraud S. (2015). Effet de la réduction de la teneur en protéines des aliments de poulets de chair : approche par méta-analyse. 11èmes journées de la recherche avicole et palmipèdes à foie gras. 11. Journées la Recherche Avicole Palmipèdes à Foie Gras, 539–544.
Gerber P. J., Steinfeld H., Henderson B., Mottet A., Opio C., Dijkman J., et al. (2013). Tackling climate change through livestock: a global assessment of emissions and mitigation opportunities (Rome: Food and Agriculture Organization of the United Nations (FAO).
Greenhalgh S., Chrystal P. V., Selle P. H., Liu S. Y. (2020). Reduced-crude protein diets in chicken-meat production: justification for an imperative. World's Poult. Sci. J. 76 (3), 537–548. doi: 10.1080/00439339.2020.1789024
Guiziou F., Béline F. (2005). In situ measurement of ammonia and greenhouse gas emissions from broiler houses in France. Bioresource Technol. 96 (2), 203–207. doi: 10.1016/j.biortech.2004.05.009
Gous R. M., Emmans G. C., Broadbent L. A., Fisher C. (1990). Nutritional effects on the growth and fatness of broilers. Br. Poult. Sci. 31 (3), 495–505.
Hauschild L., Sakomura N. K., da Silva E. (2015). “AvinespModel: predicting poultry growth, energy and amino acid requirements,” in Nutritional modelling for pigs and poultry. Eds. Sakomura N. K., Gous R. M., Kyriazakis I., Hauschild L. (Wallingford, UK: CAB International), 188–207.
Hood R. L. (1982). The cellular basis for growth of the abdominal fat pad in broiler-type chickens. Poult. Sci. 61 (1), 117–121. doi: 10.3382/ps.0610117
INRAE-CIRAD-AFZ tables. (2018). Tables of composition and nutritional values of feed material, en ligne. Available at: https://feedtables.com.
IPCC. (2021). “Summary for policymakers,” in Climate change 2021: the physical science basis. contribution of working group I to the sixth assessment report of the intergovernmental panel on climate change. Eds. V. Masson-Delmotte, P. Zhai, A. Pirani, S.L. Connors, C. Péan, S. Berger, et al. In Press.
Kidd M. T., Maynard C. W., Mullenix G. J. (2021). Progress of amino acid nutrition for diet protein reduction in poultry. J. Anim. Sci. Biotechnol. 12 (1), 1–9. doi: 10.1186/s40104-021-00568-0
Lambert W., Berrocoso J. D., Swart B., van Tol M., Bruininx E., Willems E. (2023). Reducing dietary crude protein in broiler diets positively affects litter quality without compromising growth performance whereas a reduction in dietary electrolyte balance further improves litter quality but worsens feed efficiency. Anim. Feed Sci. Technol. 297, 115571. doi: 10.1016/j.anifeedsci.2023.115571
Leinonen I., Williams A. G., Wiseman J., Guy J., Kyriazakis I. (2012). Predicting the environmental impacts of chicken systems in the United Kingdom through a life cycle assessment: broiler production systems. Poult. Sci. 91 (1), 8–25. doi: 10.3382/ps.2011-01634
Liu S. Y., Selle P. H., Raubenheimer D., Gous R. M., Chrystal P. V., Cadogan D. J., et al. (2017). Growth performance, nutrient utilisation and carcass composition respond to dietary protein concentrations in broiler chickens but responses are modified by dietary lipid levels. Br. J. Nutr. 118 (4), 250–262. doi: 10.1017/S0007114517002070
Luise D., Bosi P., Corrent E., Simongiovanni A., Lambert W., Trevisi P., et al. (2021). Effets de la baisse de protéine sur la santé intestinale du porcelet: une méta-analyse. Journées Rech. Porcine 53, 393–398.
Maynard C. W., Ghane A., Chrystal P. V., Selle P. H., Liu S. Y. (2021). Sustaining live performance in broilers offered reduced crude protein diets based on corn and wheat blend. Anim. Feed Sci. Technol. 276, 114928. doi: 10.1016/j.anifeedsci.2021.114928
Méda B., Belloir P., Lessire M., Juin H., Corrent E., Lambert W., et al. (2017). Réduction du taux protéique dans l’aliment finition chez le poulet de chair: conséquences environnementales - Archive ouverte HAL, (Journées de la recherche avicole et palmipèdes à foie gras).
Méda B., Garcia-Launay F., Dusart L., Ponchant P., Espagnol S., Wilfart A. (2021). Reducing environmental impacts of feed using multiobjective formulation: what benefits at the farm gate for pig and broiler production? Animal 15 (1), 100024. doi: 10.1016/j.animal.2020.100024
Méda B., Hassouna M., Aubert C., Robin P., Dourmad J. Y. (2011). Influence of rearing conditions and manure management practices on ammonia and greenhouse gas emissions from poultry houses. World's Poultry Sci. J. 67 (3), 441–456. doi: 10.1017/S0043933911000493
Minussi I., Lambert W., Létourneau-Montminy. M. P. (2021). Quantification of the benefits of low protein diets on foot pad dermatitis incidence and severity in broiler chickens. Proc.World. Poult. Cong. (Abstr.) 1, 540.
Mongin P. (1981). Recent advances in dietary anion-cation balance: applications in poultry. Proc. Nutr. Soc. 40 (3), 285–294. doi: 10.1079/PNS19810045
Namroud N. F., Shivazad M., Zaghari M. (2008). Effects of fortifying low crude protein diet with crystalline amino acids on performance, blood ammonia level, and excreta characteristics of broiler chicks. Poult. Sci. 87(11), 2250–2258.
Naseem S., King A. J. (2018). Ammonia production in poultry houses can affect health of humans, birds, and the environment–techniques for its reduction during poultry production. Environ. Sci. Pollut. Res. 25 (16), 15269–15293. doi: 10.1007/s11356-018-2018-y
OCDE statistique. (2022). Perspectives agricoles de l’OCDE et de la FAO 2022-2031. Available at: https://stats.oecd.org/index.aspx?lang=fr.
Oliveira J. E.D., Albino L. F.T., Rostagno H. S., Paez L. E., Carvalho D. C. D. O. (2005). Dietary levels of potassium for broiler chickens. Braz. J. Poult. Sci. 7, 33–37.
Pomar C., Marcoux M. (2005). The accuracy of measuring backfat and loin muscle thicknesses on pork carcasses by the hennessy HGP2, destron PG-100, CGM and ultrasound CVT grading probes. Can. J. Anim. Sci. 85 (4), 481–492. doi: 10.4141/A05-041
Qaisrani S. N., Van Krimpen M. M., Kwakkel R. P., Verstegen M. W. A., Hendriks W. H. (2015). Dietary factors affecting hindgut protein fermentation in broilers: a review. World's Poultry Sci. J. 71 (1), 139–160. doi: 10.1017/S0043933915000124
Rostagno H. S., Albino L. F., Hannas M. I., Donzele J. L., Sakomura N. K., Perazzo F. G., et al. (2017). Brazilian Tables for poultry and swine: composition of foods and nutritional requirements. 4th ed (UFV, Viçosa: Department of Animal Science).
Taylor J., Kyriazakis I. (2021). Towards the prediction of feed intake capacity of modern broilers on bulky feeds. Poult. Sci. 100 (12), 101501. doi: 10.1016/j.psj.2021.101501
Theil H. (1966). Applied economic forecasting (Amsterdam, the Netherlands: North-Holland Publishing Company).
Tůmová E., Teimouri A. (2010). Fat deposition in the broiler chicken: a review. Scientia Agriculturae Bohemica 41 (2), 121–128.
UN. (2019). World population prospects 2019, population division, en ligne. Available at: https://population.un.org/wpp/Graphs/Probabilistic/POP/TOT/900.
Van Emous R. A., Winkel A., Aarnink A. J. A. (2019). Effects of dietary crude protein levels on ammonia emission, litter and manure composition, n losses, and water intake in broiler breeders. Poult. Sci. 98 (12), 6618–6625. doi: 10.3382/ps/pez508
Wiedemann S. G., Phillips F. A., Naylor T. A., McGahan E. J., Keane O. B., Warren B. R., et al. (2016). Nitrous oxide, ammonia and methane from Australian meat chicken houses measured under commercial operating conditions and with mitigation strategies applied. Anim. Production Sci. 56 (9), 1404–1417. doi: 10.1071/AN15561
Keywords: amino acid, sustainability, chicken, crude protein, nitrogen balance, abdominal fat pad, intake, efficiency
Citation: de Rauglaudre T, Méda B, Fontaine S, Lambert W, Fournel S and Létourneau-Montminy M-P (2023) Meta-analysis of the effect of low-protein diets on the growth performance, nitrogen excretion, and fat deposition in broilers. Front. Anim. Sci. 4:1214076. doi: 10.3389/fanim.2023.1214076
Received: 28 April 2023; Accepted: 15 June 2023;
Published: 24 July 2023.
Edited by:
Michael D. Flythe, United States Department of Agriculture, United StatesReviewed by:
Sarah C. Pearce, United States Department of Agriculture, United StatesCopyright © 2023 de Rauglaudre, Méda, Fontaine, Lambert, Fournel and Létourneau-Montminy. This is an open-access article distributed under the terms of the Creative Commons Attribution License (CC BY). The use, distribution or reproduction in other forums is permitted, provided the original author(s) and the copyright owner(s) are credited and that the original publication in this journal is cited, in accordance with accepted academic practice. No use, distribution or reproduction is permitted which does not comply with these terms.
*Correspondence: Marie-Pierre Létourneau-Montminy, bWFyaWUtcGllcnJlLmxldG91cm5lYXUtbW9udG1pbnlAdWxhdmFsLmNh
Disclaimer: All claims expressed in this article are solely those of the authors and do not necessarily represent those of their affiliated organizations, or those of the publisher, the editors and the reviewers. Any product that may be evaluated in this article or claim that may be made by its manufacturer is not guaranteed or endorsed by the publisher.
Research integrity at Frontiers
Learn more about the work of our research integrity team to safeguard the quality of each article we publish.