- 1Department of Preventive Veterinary Medicine and Animal Health, School of Veterinary Medicine and Animal Science, University of São Paulo, Pirassununga, SP, Brazil
- 2Facoltà di Medicina Veterinaria, Università degli Studi di Teramo, Teramo, Italy
- 3Graduation Program in One Health, University Santo Amaro, São Paulo, SP, Brazil
- 4Department of Agricultural Biotechnology, Animal Biotechnology Section, Faculty of Agriculture, Van Yüzüncü Yıl University, Van, Türkiye
- 5Federal Institute of Education, Science, and Technology of Rio Grande do Sul, Sertão, RS, Brazil
Introduction: The welfare of breeding boars is often overlooked, resulting in limited scientific data to foster discussion of the topic. We aimed to investigate the effect of different boar housing conditions on their offspring’s emotionality.
Methods: Eighteen boars were housed in three different conditions: crates (C; n = 6), pens (P; n = 6), or enriched pens (E; n = 6). Boars were distributed by semen quality (SQ; high, medium, or low). Three semen pools were used to inseminate 13 gilts housed in outdoor paddocks. At 25 days of age, 138 suckling piglets were subjected to open field (OF), novel object (NO), and elevated plus-maze (EPM) tests. Saliva was collected before and after the OF and NO tests to measure cortisol concentrations. At the end of the experiment, hair samples were collected for DNA paternity tests. Piglets were classified based on their behavioral responses using hierarchical cluster analysis of the principal components extracted from factor analysis of mixed data. The variables were reduced to seven principal components (dimensions, Dims), which explained 73% of the total variation, and were analyzed using linear mixed models. The models included each Dim as a dependent variable, paternal treatment and body weight (BW) as fixed effects, and paternal SQ as a random effect. Kruskal−Wallis and Wilcoxon rank-sum tests were used to compare the cortisol concentration ratios (before and after the OF and NO tests) between groups.
Results: There was an effect of treatment on Dim 3 (EPM; activity/fear), with higher values in C piglets than E piglets (p = 0.047). Although C piglets had significantly higher values than P piglets in Dim 4 (EPM; anxiety; p = 0.029) and Dim 6 (NO; inactivity far from the object/exploration; p < 0.0001), the effect of the paternal treatment × BW interaction was significant in both dimensions (p < 0.05). The cortisol ratio in E piglets was greater than that in P and C piglets (p < 0.05).
Discussion and conclusion: Our findings indicate that boar breeding environments affect the stress response and emotionality (anxiety, fear, and exploration) of their offspring.
1 Introduction
The transmission of male experiences to their offspring involves a complex interplay with the contribution of females. Male health, lifestyle, physiology, behavior, and fertility interact with female life experiences, including age, environmental resources, mating, and opportunities at fertilization (Champagne, 2020). Males show individual variability in the contribution of environmental effects (Soubry et al., 2014), including stress (Lacal and Ventura, 2018), to epigenetic markers in sperm cells (Rodgers and Bale, 2015), which is influenced by the different stages of spermatogenesis in which challenges occur (Donkin and Barrès, 2018). Most likely, changes are caused by epigenetic mechanisms that play an important role in neurologic reprogramming and developmental effects (Bale, 2015; Morgan et al., 2019), and can affect animal behavior (Guerrero-Bosagna et al., 2020). Stressful conditions experienced by the parents can influence the offspring’s health, including disruptions in stress response mechanisms (Lacal and Ventura, 2018) and, consequently, alterations in neonatal neuroplasticity (Bale, 2015; Morgan et al., 2019).
Confined commercial pig housing systems expose boars to challenges that often exceed their coping abilities, altering their species-specific behavior (Broom et al., 1995; Hötzel and Machado Filho, 2004). Behavioral and emotional disruptions have been linked to poor welfare in animals (Tatemoto et al., 2020). In addition, when animals experience challenges in an attempt to maintain homeostasis, a coordinated response of the central nervous system (CNS) (Johnson et al., 1992) activates the hypothalamic−pituitary−adrenal (HPA) axis (Muráni et al., 2010), which plays a fundamental role by secreting molecules such as neurotransmitters and glucocorticoids (Sorrells et al., 2009). Piglets subjected to neonatal stress presented downregulation of hippocampal receptors responsible for providing negative feedback to the HPA axis (Poletto et al., 2006). Glucocorticoids play an essential role in the CNS (Johnson et al., 1992), modulating a variety of functions, such as memory, attention, sleep, and emotional states (Saraiva et al., 2005). These physiological changes can be quantified to determine animal stress levels and an animal’s ability to cope with its environment (Siegford et al., 2008). Keeping pigs in poor and barren environments for their species-specific needs can affect their behaviors and emotions (Kanitz et al., 2016); moreover, it can affect future generations (Rutherford et al., 2014). Thus, improving welfare is usually linked with an increase in an animal’s opportunity to express typical behaviors and avoid undesirable behaviors (Tarou and Bashaw, 2007; Temple et al., 2011; Tatemoto et al., 2020).
When an individual is exposed to challenging environmental events, its adaptive system is activated, and gamete alterations and phenotype modifications may be heritable (Donkin and Barrès, 2018). These adaptive mechanisms are regulated throughout their lives (Meaney and Szyf, 2005; Skinner and Guerrero-Bosagna, 2009; Bale, 2015; Chan et al., 2020) and by variations in the phenotype of individuals (Otten et al., 2015). Male gametes transmit important information related to cardiometabolic diseases (Temple et al., 2011), changes in glucose and cholesterol metabolism (Zhao et al., 2020), embryonic development (Alves et al., 2019), stress responses (Rodgers et al., 2013; Nestler, 2016), and anxiety disorders (Schiele and Domschke, 2018), among others. Events in humans, such as exposure to cigarettes (Marczylo et al., 2012; Siddeek et al., 2018), toxicants, poor nutrition, drugs, stress, and exercise routine, affect epigenetic markers in sperm cells (Marcho et al., 2020) and influence their progeny (Nilsson et al., 2018). Such epigenetic markers can permanently modify emotionality in animals, with consequences for their welfare (Schiele and Domschke, 2018; Guerrero-Bosagna et al., 2020).
Animal behavior is one of the most important indicators of the conditions to which an individual has been exposed and therefore it must be evaluated to understand how to meet the biological demands of species (Bateson and Martin, 2021). Emotional states in animals, such as fear, anger, and panic, provide an adaptive and fundamental function for survival, and are processed in specific areas of the brain (Paul et al., 2005; Panksepp, 2007; Tatemoto et al., 2023). Because the emotional state of animals is one of the fundamental conditions for animal welfare (Fraser, 2008; Dawkins, 2017), tests have been developed to assess emotionality through behavioral responses to the environment. Assessing offspring fear and anxiety through open field (OF), novel object (NO) (Puppe et al., 1999; Tatemoto et al., 2020), and elevated plus-maze (EPM) (Rodgers and Johnson, 1995; Rodgers and Dalvi, 1997; Andersen et al., 2000a; Andersen et al., 2000b; Janczak et al., 2000; Carobrez and Bertoglio, 2005) tests may provide an integrative approach to determining adaptive behavioral mechanisms in response to boar life experiences.
Understanding the impact of the parental environment on offspring behavior and emotionality can help evaluate a species resilience. The objective of our research was to investigate the effect of boar housing conditions on their offspring’s emotionality.
2 Materials and methods
This research was performed at the University of São Paulo (USP) Campus Fernando Costa, Pirassununga—São Paulo, Brazil. The Ethics Committee on Animal Use approved this study (protocol number CEUA 6555081018). In addition, the study was carried out in accordance with the ARRIVE guidelines (https://arriveguidelines.org/). An experimental timeline is presented in Figure 1.
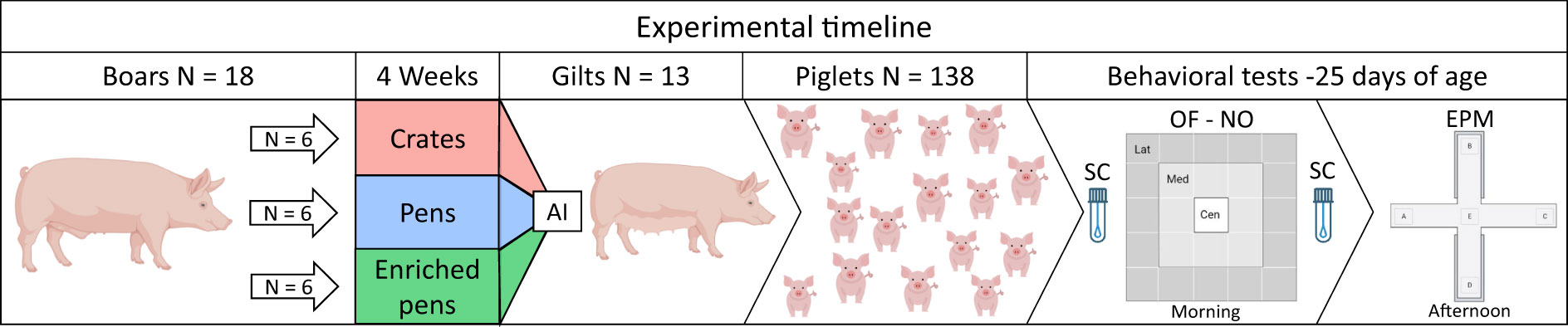
Figure 1 Experimental timeline. Groups on the left (n = 6) each represent a boar housing condition: in crates, pens, or enriched pens. The semen from boars was used to inseminate gilts. The offspring were evaluated on physiological and behavioral tests in the following order: 1—saliva sample, 2—open field (OF) and novel object (NO) tests, 3—saliva sample, and 4—elevated plus-maze (EPM) test. SC, salivary cortisol concentration. All evaluations occurred during the nursing period.
2.1 Boar housing treatments
A total of 18 12-month-old boars (Landrace × Large White, Topgen) were used. Semen quality (motility features assessed by computer-assisted sperm analysis and sperm cell morphology) was used to evenly distribute the animals among housing treatments. The boars were allocated to three different experimental housing treatment systems: crates (C; n =6), individual pens (P; n = 6), and individual pens with environmental enrichment (E; n = 6). A nipple drinker supplied water ad libitum, and animals were fed in troughs daily at 0700 h and 1300 h, with 2.8 kg of gestational diet per day. Details about housing conditions were previously reported by Bernardino (2021) and Sabei et al. (2023).
Semen collection was performed weekly, and semen collected in the fourth week was used for insemination. The semen was kept in commercial diluent (Androstar® Plus—Minitube, Germany) for 5 days at 17°C until insemination.
2.2 Gilt housing, handling, and insemination
For this study, 13 gilts (Afrodite®, Topgen) were group housed in paddocks (400 m2 per gilt). Each paddock had a fence, a mud pool, shade, and an individual shelter. The gilts were fed a gestational diet on the ground, with an average of 2.5 kg per day per gilt; the feed was divided into two meals, at 0700 h and 1300 h. Water was supplied ad libitum by two nipple drinkers.
The gilts were synchronized with altrenogest (Regumate®, MSD Animal Health) in accordance with the manufacturer’s recommendation (5 mL/day/animal for 18 days). The gilts were artificially inseminated with a semen pool from six boars, including two boars from each of the housing treatments matched in semen quality. Three types of semen pools (high, medium, and low quality) were obtained, all of which included samples from boars in all three housing treatments. Four gilts were inseminated with high semen quality, four with medium semen quality, and five with low semen quality. Details about housing conditions, handling, and piglet management were previously reported by Sabei et al. (2023).
2.3 Management from farrowing to weaning
All farrowing was monitored, piglets were guided to their first colostrum intake, and their umbilical cords were cut and disinfected. On the third day of life, piglets were ear-tagged for identification. No piglet underwent management practices such as iron dextran administration, tooth clipping or grinding, tail docking, or surgical castration. All piglets had access to creep feeding and were weighed at 25 days of age. A postpartum lactation diet was provided ad libitum to the sows at 0700 h and 1600 h on rubber mats. Piglets and sows had ad libitum access to water from nipple drinkers.
2.4 Assessment of piglet emotionality
One hour before testing, each sow and its litter were moved to a pen (9 m2) near the test room. Each piglet was identified with a non-toxic animal marker and gently carried in a random order from its pen to the test arena. Three behavioral tests were performed to assess piglet emotionality at 25 days of age (n = 138). Piglets underwent a combination of an OF test and a NO test (Puppe et al., 2007; Bernardino et al., 2016; Zupan and Zanella, 2017; Tatemoto et al., 2020; Farias et al., 2023) in the morning. Five hours later, piglets were subjected to an EPM test (Rodgers and Dalvi, 1997; Andersen et al., 2000a; Andersen et al., 2000b; Farias et al., 2023) in the same order as the OF and NO tests. Each piglet was returned to its sow after each test. After each piglet was tested, urine and feces were removed from the test arenas, and the arenas were cleaned with alcohol to reduce possible chemical clues and avoid interference in the outcomes of the following test.
Open field and novel object tests: The test arena was 2.75 m × 2.75 m and was divided into 25 drawn white squares. All walls were 2.2 m high and had a trapdoor-shaped window to gently place the piglet into the test arena. The squares along the walls were named the lateral (Lat), the central squares was named the center area (Cen), and the squares between Lat and Cen were named the medium areas (Med); for details, see Figure 2A. A traffic cone (as a novel object) was introduced by a pulley system into the arena center immediately after the open field test. Each test lasted 5 min, for a total of 10 min in the combined test.
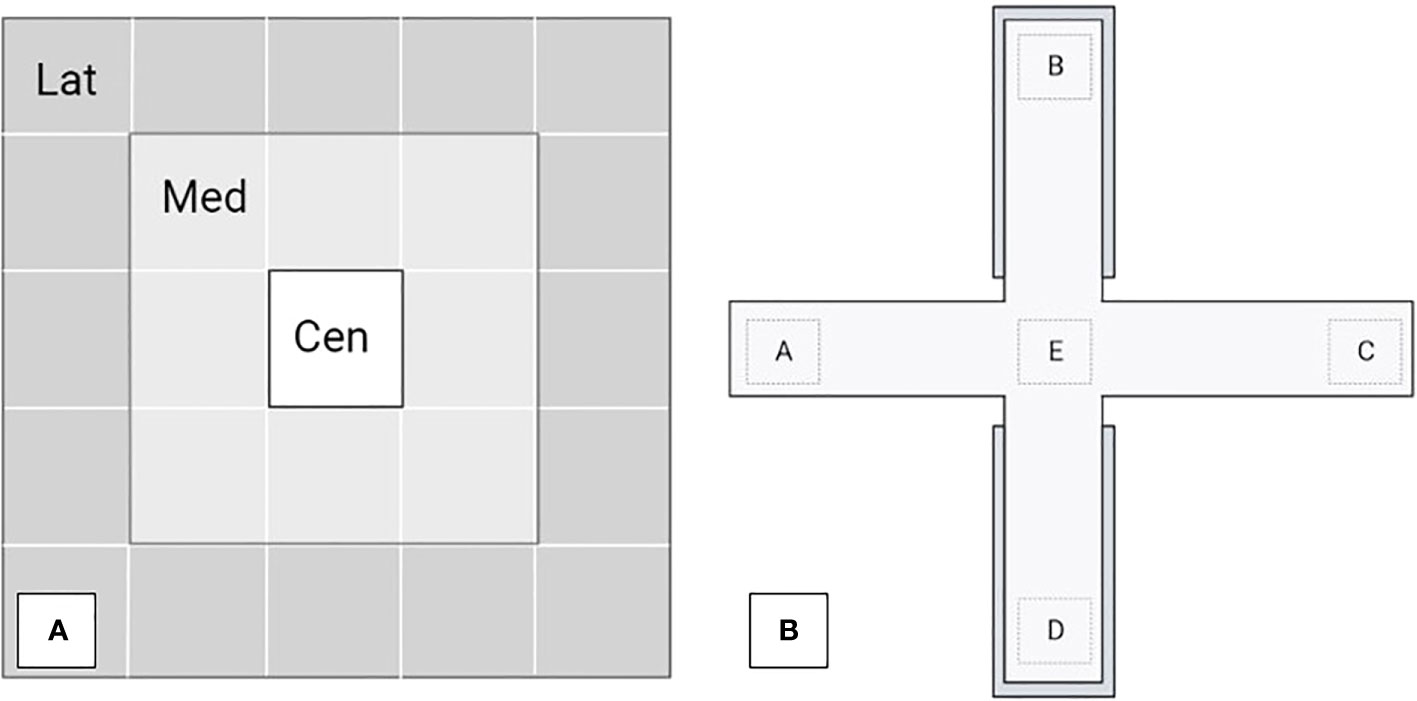
Figure 2 (A) The open field and novel object arena was divided into three areas: lateral (Lat), medium (Med), and center (Cen). (B) The elevated plus-maze test was divided into five areas: open arms (A, C), closed arms (B, D), and center (E). Each test lasted 5 min.
Elevated plus-maze test: The maze was set 1 meter above the floor in an experimental room and consisted of four arms of equal length (1.2 m) and width (0.6 m). Two of these arms had solid walls (0.45 m in height); these arms were opposite each other. The test was divided into five areas for behavioral assessment: the open arms (A and C), closed arms (B and D), and center (E); see Figure 2B. Rubber mats were placed on the floor around the arena. The test lasted for 5 min per piglet.
All tests were video-recorded (Intelbras Bullet Vhd 1220b G4 Ir 3,6 mm, 20 m, 1080p Full, Intelbras®, Brazil) for subsequent analysis. A single trained observer assessed the behaviors listed in Table 1 using Boris software (Friard and Gamba, 2016). Observer training involved memorizing the predetermined commands inserted in the software ethogram.
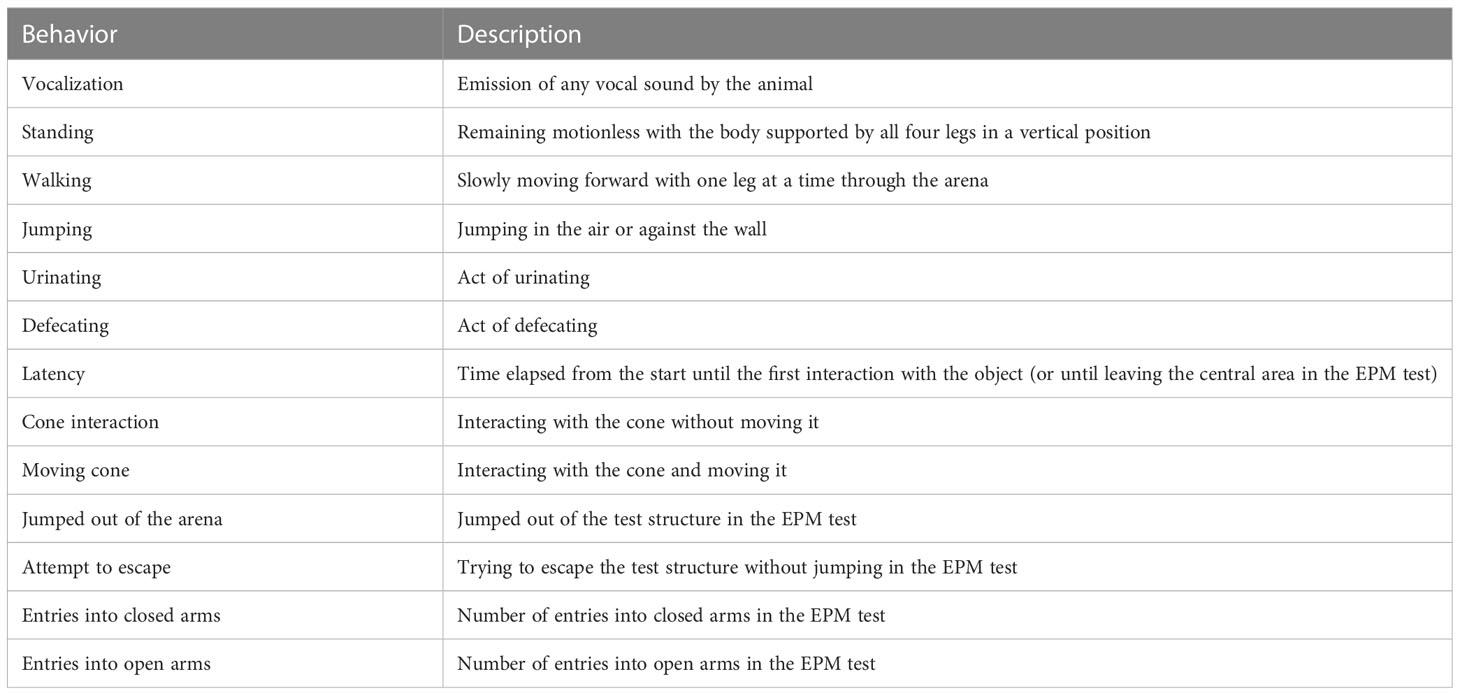
Table 1 Ethogram used for assessing the behavior of piglets during each 5-min test: open field, novel object, and elevated plus-maze (EPM) (Andersen et al., 2000a; Janczak et al., 2002; Carobrez and Bertoglio, 2005; Colonnello et al., 2010; Donald et al., 2011; Reimert et al., 2013; Bernardino et al., 2016; Tatemoto et al., 2019b; Haigh et al., 2020; Sarmiento et al., 2021).
Table 2 shows the behavior assessed in the tests by specific arena area and its corresponding interpretation based on the literature (Andersen et al., 2000a; Janczak et al., 2002; Carobrez and Bertoglio, 2005; Colonnello et al., 2010; Donald et al., 2011; Reimert et al., 2013; Bernardino et al., 2016; Tatemoto et al., 2019b; Haigh et al., 2020; Sarmiento et al., 2021; Farias et al., 2023).
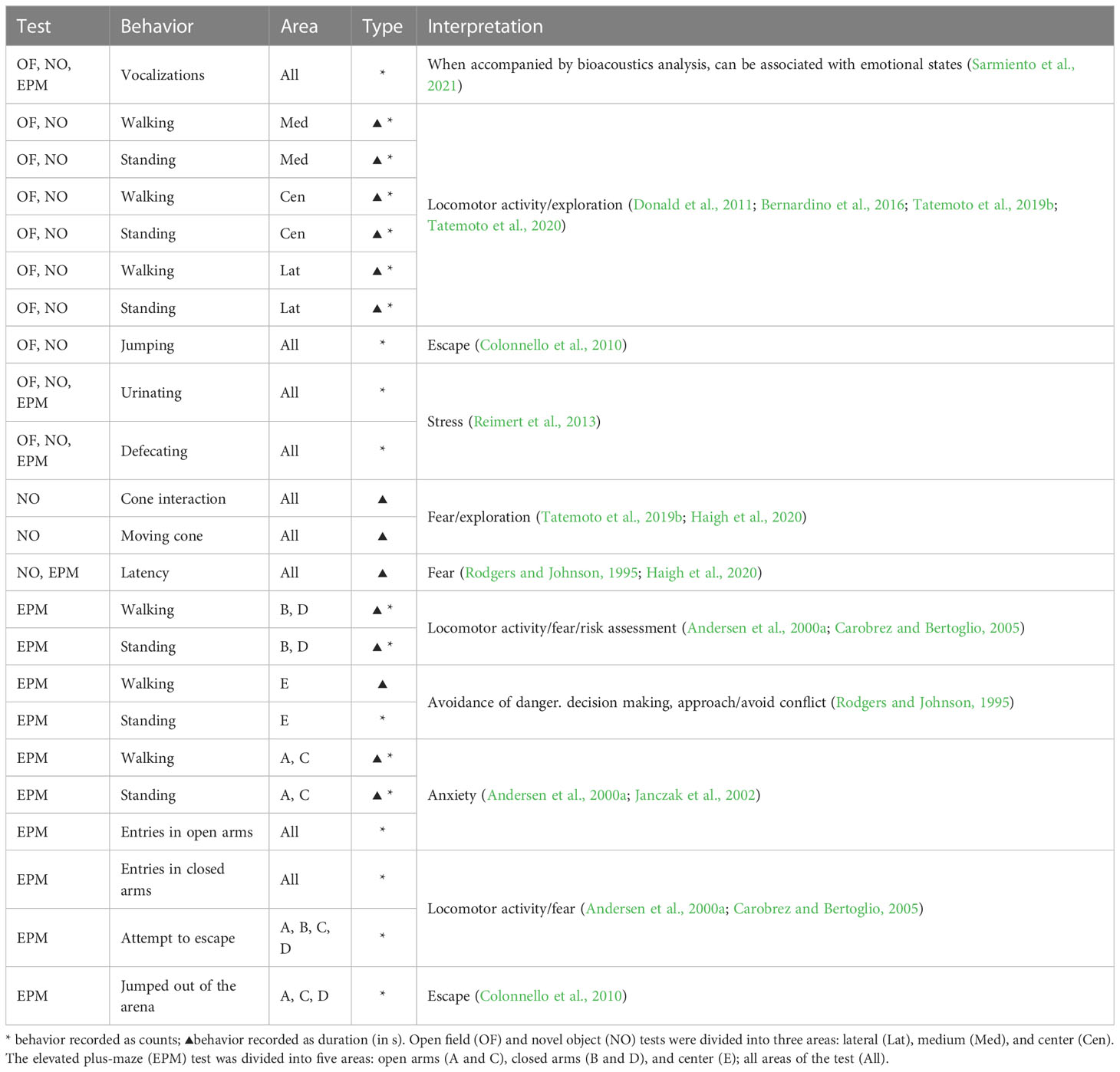
Table 2 Interpretations of each type of pig behavior observed in specific areas of the test arena according to the literature.
2.5 Salivary cortisol concentration and paternity test
Saliva samples were collected from each piglet using cotton swabs (Johnson & Johnson) immediately before and 20 min after the OF and NO tests in the morning. Salivary cortisol concentrations were measured using a validated cortisol enzyme immunoassay (Palme and Möstl, 1997; Siegford et al., 2008; Palme, 2019; Tatemoto et al., 2019a; Farias et al., 2021; Sarmiento et al., 2021; Sabei et al., 2023).
At the end of the experiment, hair samples from boars and piglets were collected from the lumbar region and sent to the NEOGEN® Laboratory (Indaiatuba, São Paulo) for a paternity test. The technique used was a single nucleotide polymorphism analysis. The results reported in Sabei et al. (2023) were used to determine the paternal (boar) treatment associated with each piglet for subsequent data analysis.
2.6 Data analysis
2.6.1 Descriptive analysis
The dataset included behavior from 138 animals and information on the animals’ sires. The 138 piglets were evaluated in three different behavioral tests. Sixty-eight behavioral variables were recorded during the tests (Table 2). Piglet data were classified based on their behavioral responses using the methodology described by Çakmakçı (2022). In accordance with this methodology, principal component analysis (PCA) and hierarchical cluster analysis (HCA) were used to classify the piglets based on their coping styles. PCA is a factorial method applied to datasets in which a group of individuals is defined by a collection of quantitative variables (Pagès, 2014).
Hierarchical clustering is an additional approach used to study the similarities among individuals with respect to all characteristics (Husson et al., 2010). This method allowed the inclusion of categorical and continuous variables, which represents a modification of the previous approach that included only numerical variables for PCA and HCA. A factor analysis of mixed data (FAMD) was used to reduce the dimensionality of the dataset before applying HCA. FAMD, a multivariate statistical technique that can handle datasets with a combination of categorical and continuous variables (Pagès, 2015), was used to reduce the dimensionality of the dataset. The number of dimensions retained after FAMD was estimated using k-fold cross-validation with the missMDA R package (Josse and Husson, 2016).
In the initial stage, animals were classified based on their behavior to validate the dataset for classification purposes. The R package psych (Revelle, 2022) was used to conduct Bartlett’s test of sphericity to determine whether or not there was an adequate correlation between behavioral variables and to perform the Kaiser−Meyer−Olkin (KMO) test to assess sample adequacy. Initially, 68 variables were tested with the KMO test. Variables with KMO values below 0.5 were excluded from the dataset, as any value below 0.5 is considered “unacceptable” by Kaiser and Rice (2016). The second stage was to examine commonalities, which represent the proportion of variation in a given variable that can be explained by the selected components. Variables with a low commonality value (below 0.6) were excluded in the second stage. After these stages, 25 variables matching the validity criteria were retained for FAMD. The final FAMD analysis was thus performed on 138 individuals, described by 25 behavioral variables and treatments. Piglets were then clustered based on seven retained principal components scores. Hopkins’ H statistic was computed on scores of retained principal component using the R package clustertend to determine if the dataset is clusterable (Hopkins and Skellam, 1954). Hopkins’ H statistic values greater than 0.5 suggest that the dataset is clusterable (Lawson and Jurs, 1990). The R package FactoMineR (Le et al., 2008) was used to perform FAMD and HCA. The results were visualized using the R package factoextra (Kassambara and Mundt, 2020).
2.6.2 Analysis of behavioral variables
The dependent variables were behavioral variables, which were reduced to seven principal components, and were modeled using a linear mixed model in the R package glmmTMB (Brooks et al., 2017). The models included covariates (paternal treatment and piglet body weight) and their interactions as fixed effects. If interactions were not significant, they were excluded from the model. The model also included seminal quality as a random effect. The model parameters were generated using the R package car, and the results were plotted using the R package effects (Fox and Weisberg, 2019). Seven models, each representing a component, were constructed. The residual distributions of the models were checked by the R package performance (Lüdecke et al., 2021). Estimated marginal means were obtained using the R package emmeans (Searle et al., 2022). Post hoc pairwise comparisons were performed to compare classes of independent variables using p-values adjusted by the Tukey method in the R package multcomp (Hothorn et al., 2008). The differences were considered significant at p < 0.05. Using the R package ggstatsplot (Patil, 2021), a chi-squared test of independence was performed to examine whether or not there is a significant association between the clusters and paternal treatment. Analyses were performed using R version 4.1.2 (Team R Core, 2022).
2.6.3 Salivary cortisol concentrations
Salivary cortisol concentrations (pg/50 µl) were analyzed by calculating the ratio between the values obtained before and after the test (ratio = before/after). For some animals, the saliva sample volume was insufficient for analysis, resulting in the following sample sizes per treatment: C, n = 16; P, n = 7; and E, n = 17. The Grubbs’ test was used to remove outliers, according to a p-value < 0.05. Subsequently, Kruskal−Wallis and Wilcoxon rank-sum tests were used to compare the ratio between treatments. The differences were considered significant at p < 0.05. Analysis was performed using R version 4.1.2 (Team R Core, 2022).
3 Results
3.1 Factor analysis of mixed data
Bartlett’s test of sphericity [chi-square (X2 (300)) = 2749.479, p < 0.001] and KMO measure of sample adequacy (0.71; p < 0.05) suggested that the data met the assumptions for PCA. The findings of k-fold cross-validation indicated that the analysis should be restricted to the first seven components. Consequently, the description was restricted to these components, called dimensions (Dim), which explained 73.03% of the total variance (Figure 3).
The correlation coefficient between a component and a variable was used to select the variables to describe the components (Table 3). The correlations of the variables with the dimensions are presented in descending order.
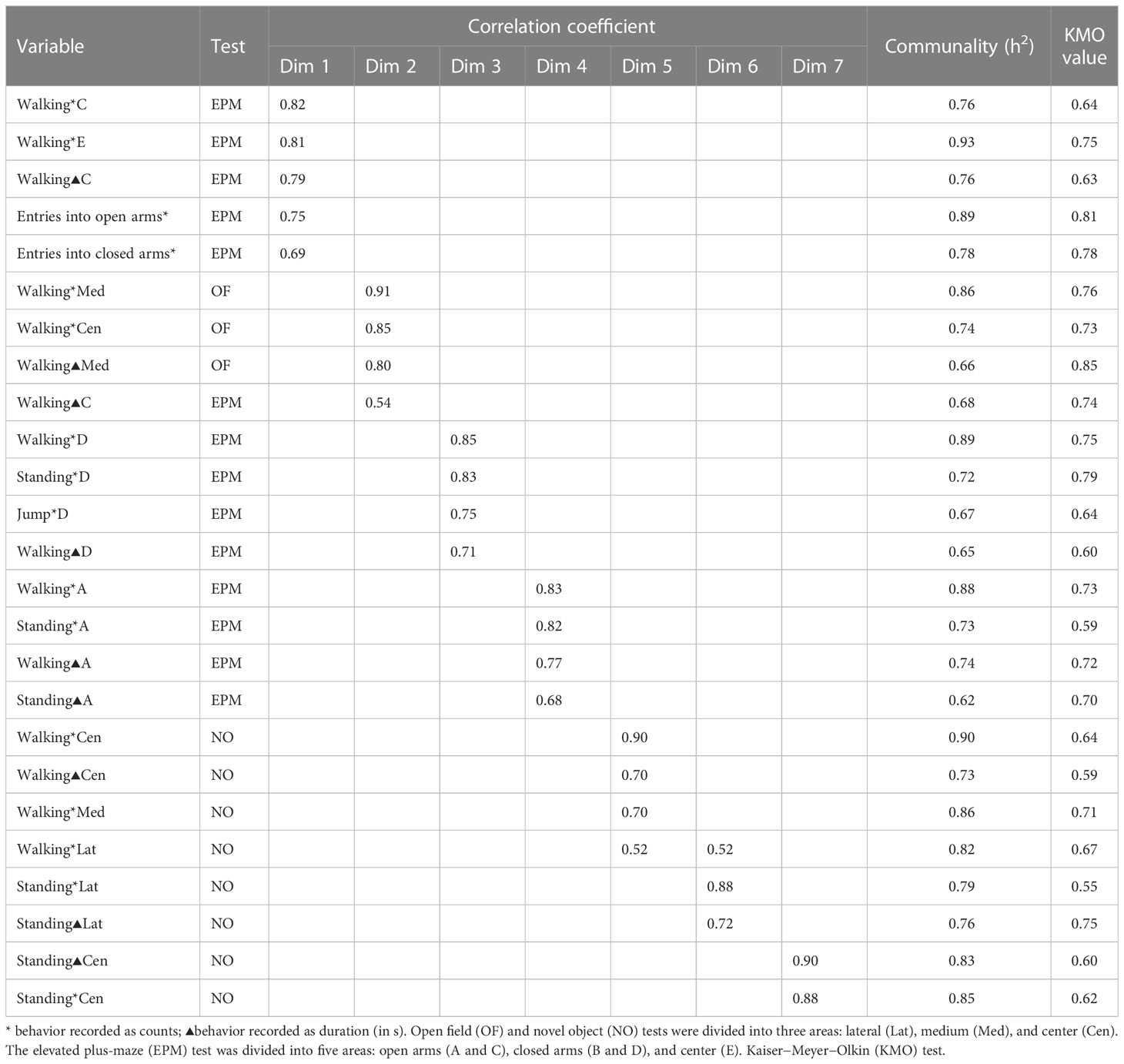
Table 3 Description of dimensions (Dims) by quantitative variables according to the correlation coefficients (in descending order).
3.2 Piglet behaviors
In Figure 4, paternal treatment is indicated by the color to display dispersion around centroids associated with specific categories [paternity test results are described in the previous work by Sabei et al. (2023) regarding determination of paternal treatment of the piglets]. Colors reflect the categories of categorical variables. Confidence ellipses are drawn around the categories of treatment to facilitate plane representation and enable visualization of whether or not two categories differed significantly. Groups with no overlapping confidence ellipses differed significantly.
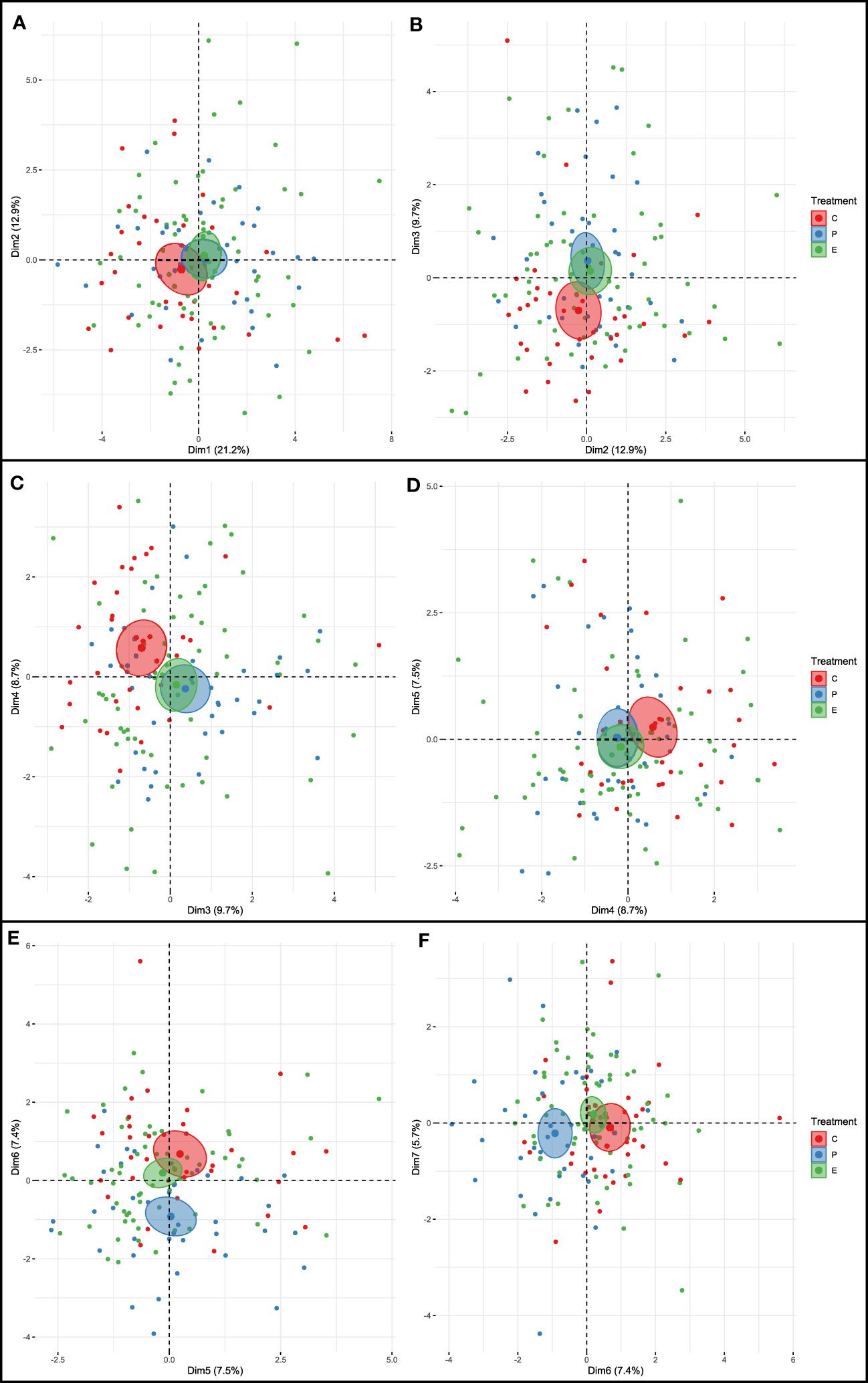
Figure 4 Principal component analysis of the seven dimensions (Dims). (A) Dims 1 and 2; (B) Dims 2 and 3; (C) Dims 3 and 4; (D) Dims 4 and 5; (E) Dims 5 and 6; and (F) Dims 6 and 7. The individuals are shown in colors according to the paternal treatment: crates (C), pens (P), and enriched pens (E).
The effects of treatment on behavioral responses were assessed using the seven dimensions generated by FAMD to represent these behaviors. There was a significant effect of treatment on Dims 3, 4, and 6 (p < 0.05). Furthermore, the effect of the treatment by body weight interaction on Dim 4 (EPM; anxiety) was significant (Figure 5). The Dim 3 (EPM; activity/fear) values of C piglets (i.e., piglets fathered by C boars) were significantly lower than those of P piglets. The Dim 4 values of C piglets were significantly higher than those of P and E piglets. The behaviors in Dim 4 (EPM; anxiety) decreased with increased body weight in C and P piglets but increased with increased body weight in E piglets. The P piglets had lower values of Dim 6 (NO; inactivity far from the object/exploration) than the E and C piglets.
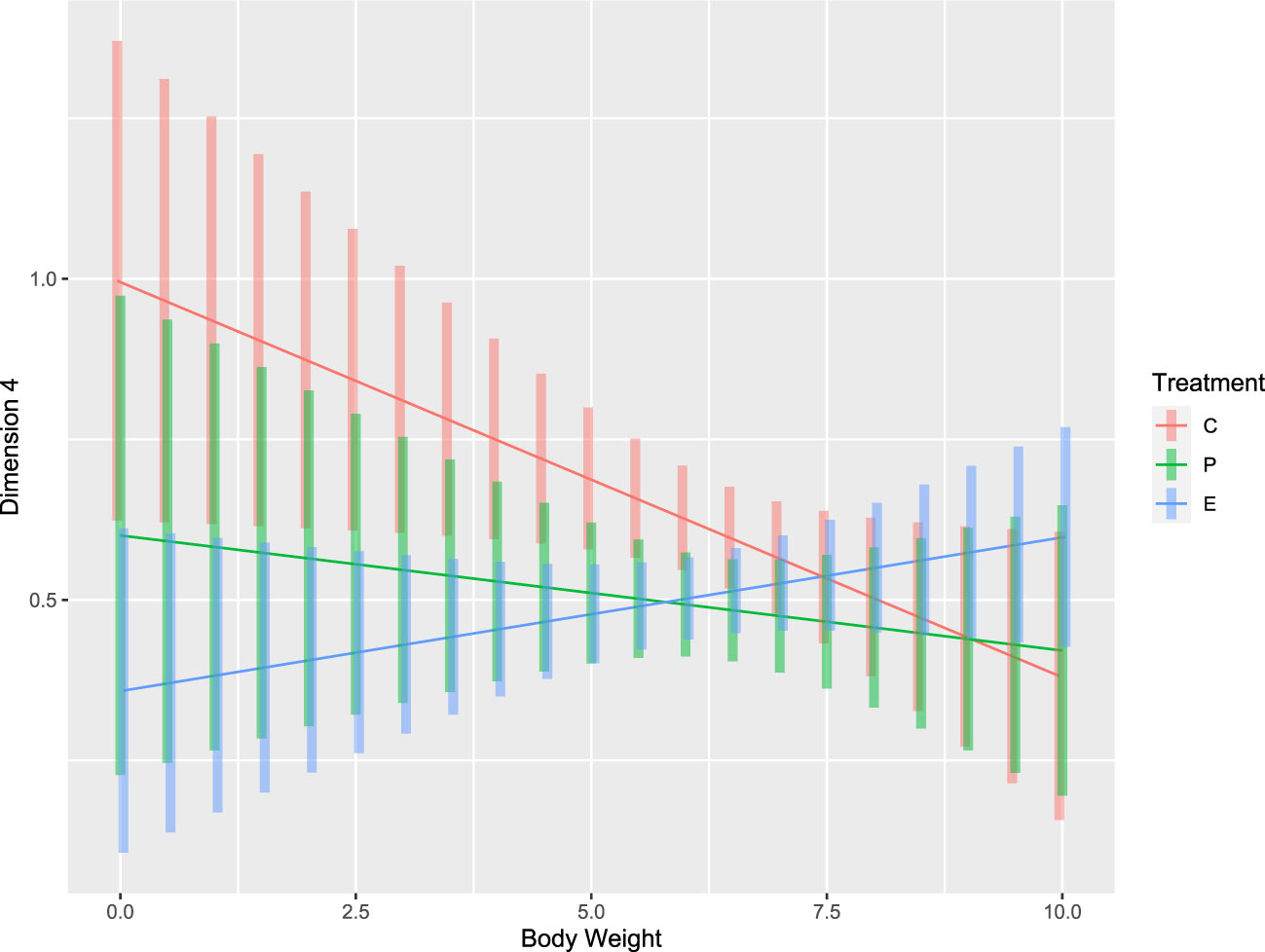
Figure 5 Body weight distributions for dimensions with significant interactions with treatment (p < 0.05).
Figure 4 shows two-dimensional scatterplots of treatment groups and behavioral dimensions. When Dims 3 and 4 were evaluated together (Figure 4C), P and E piglets were similar, whereas C piglets were significantly different (p < 0.05). In contrast, when Dims 5 and 6 were evaluated together (Figure 4E), C and E piglets were similar, and P piglets were significantly different (p < 0.05). A similar scenario was also observed when Dims 6 and 7 were assessed together (Figure 4F), with similarity between C and E piglets, and significant difference with P piglets (p < 0.05).
Figure 6A shows two-dimensional scatterplots of piglets in the clusters (cluster 1, n = 91; cluster 2, n = 21; cluster 3, n = 26), in which individuals are colored according to their cluster. Supplementary Table 1 provides quantitative variables, eta-squared values, and p-values. Eta-squared values indicate the proportion of variability in the quantitative variable explained by a categorical variable. Descriptions of each cluster based on the quantitative variables are provided in Supplementary Table 2. Overall, the results suggest a strong association between the cluster variable and the quantitative variables.
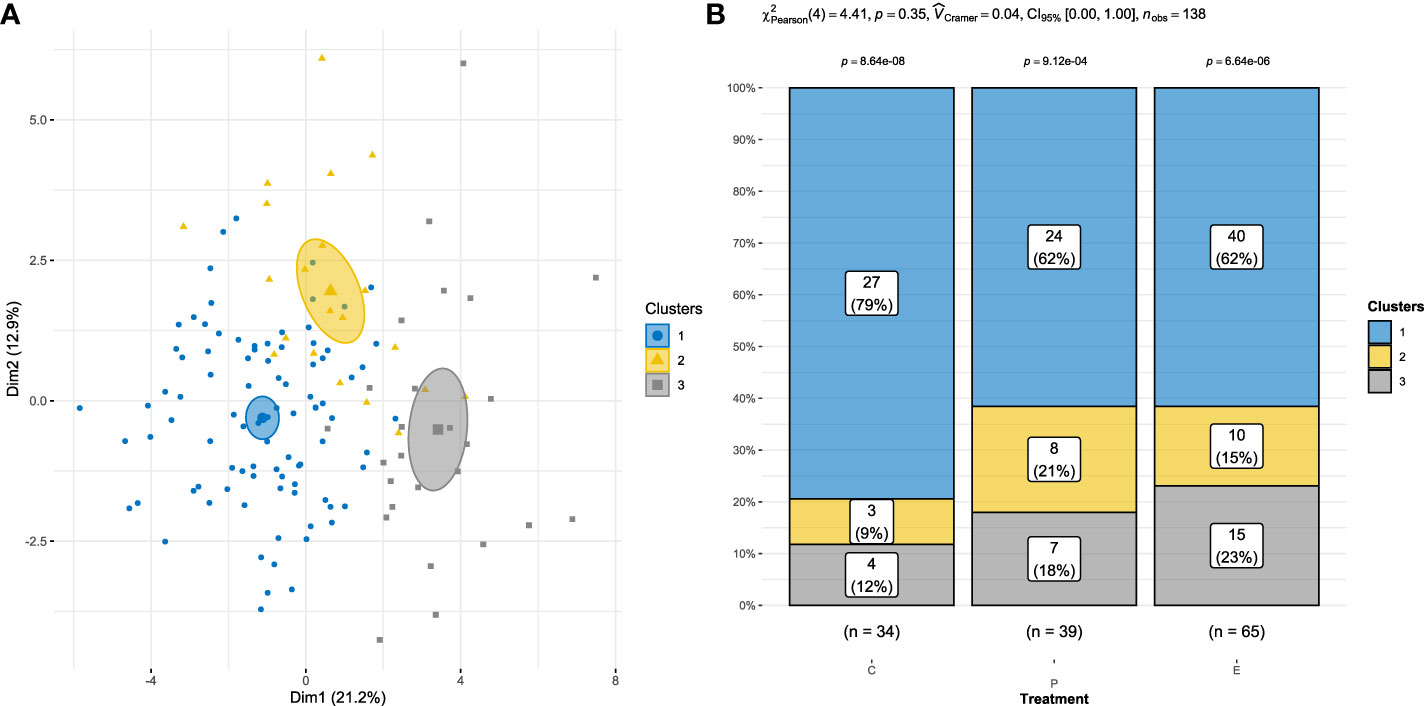
Figure 6 (A) Individuals are shown in colors according to the cluster to which they belong. (B) The association between paternal treatment [crates (C), pens (P), and enriched pens (E)] and cluster (chi-squared test) was not significant.
The chi-squared test results indicated that there was no statistically significant relationship between treatment and clusters (p = 0.350), indicating that these coping styles were independent of treatment (Figure 6B).
3.3 Salivary cortisol concentration
Figure 7A shows salivary cortisol concentrations before and after the OF and ON tests, and the comparisons are shown in Figure 7B. E piglets had a higher cortisol ratio than P and C piglets (p < 0.05); this value was close to 1, indicating that the salivary cortisol concentrations remained steady/did not vary much before and after the OF and NO tests. Conversely, P and C piglets had a cortisol ratio of less than 1, meaning that the salivary cortisol concentrations increased/were higher after the OF and N tests than before.
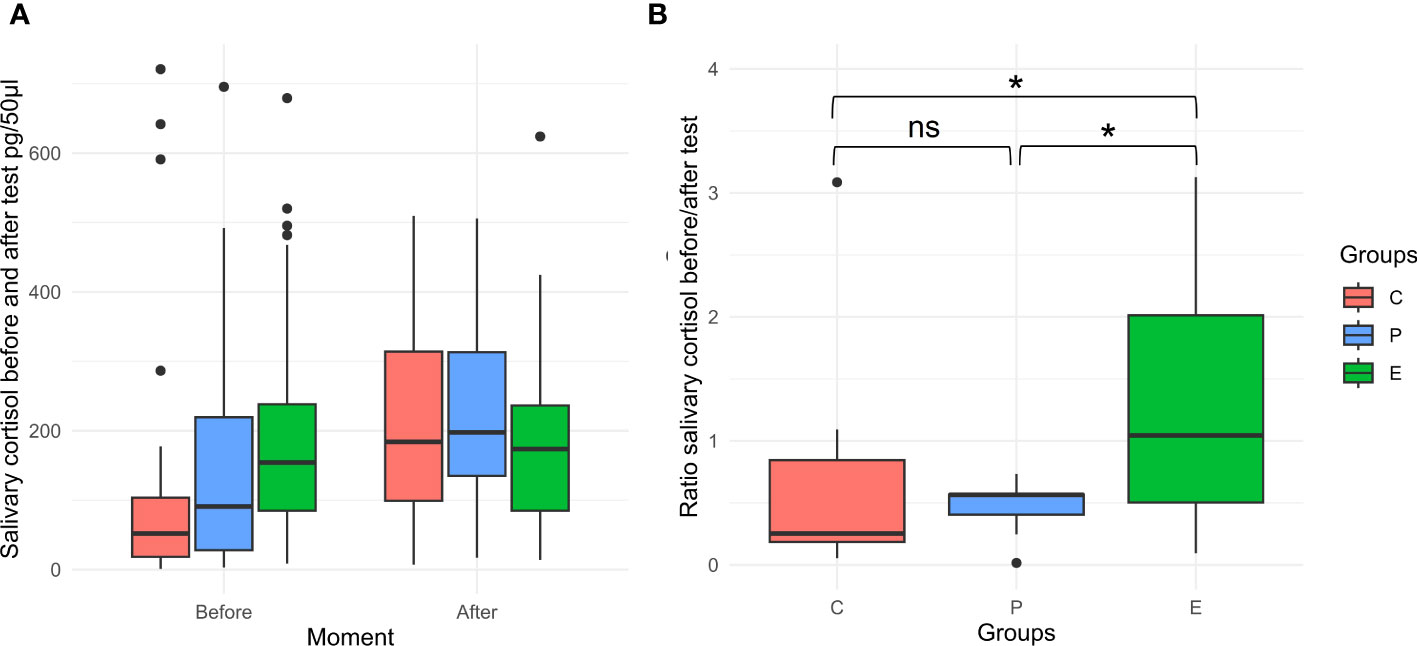
Figure 7 (A) Salivary cortisol concentrations before and after the open field (OF) and novel object (NO) tests according to paternal treatment: crates (C), pens (P), and enriched pens (E). (B) Salivary cortisol ratio (before/after the OF and NO tests). ns, not significant, p > 0.05; *, significant, p < 0.05.
4 Discussion
The present study demonstrated that the housing conditions of boars influenced their offspring’s behaviors in the OF, NO, and EPM tests, and their stress response during the tests. The results showed that most of the observed behaviors of C piglets were associated with higher outcomes of anxiety, fear, and locomotor activity. In addition, the results indicated that the body weight of piglets affected their performance during the behavioral tests. The behavioral modifications observed in our study are consistent with the literature in which parental environment modulated the development of their offspring (Braun and Champagne, 2014; Chan et al., 2020).
During the EPM test, E piglets exhibited more behaviors in the closed arms than C piglets. Avoiding open arms represents fear of novelty, avoidance (Andersen et al., 2000a), or even risk assessment behaviors (Carobrez and Bertoglio, 2005). This indicates that E piglets may be more fearful or presented more risk assessment behavior. P and E piglets had less anxiety related to locomotor activity in the EPM test than C piglets. Spending more time walking in the open arms or entering any arm more frequently is associated with locomotion activity and anxiety (Andersen et al., 2000a; Janczak et al., 2000; Janczak et al., 2002). Previous studies have suggested that such behaviors are heritable through sperm epigenetic factors, including those related to anxiety disorders (Babenko et al., 2015; Schiele and Domschke, 2018). Similar results were found in chronically stressed rodents, whose offspring showed greater locomotor activity associated with anxiety in the EPM test (Zheng et al., 2021). Semen (sperm and seminal fluid) seems to play a fundamental role in the observed changes (Chan et al., 2020). In chronically stressed mice, environment affected semen quality in the father and in his offspring, showing lower concentration and motility (Zheng et al., 2021). We previously reported the impact of housing stress on semen parameters in the same boars used in the current study (Bernardino, 2021; Bernardino et al., 2022). Epigenetic sperm alterations and poor semen quality in mice were associated with increased methylation and decreased sperm motility and fertility (Rodgers et al., 2015; Zheng et al., 2021). We anticipate that our results are possibly linked to epigenetic mechanisms in boar semen that have not yet been elucidated (Bernardino, 2021; Nery da Silva et al., 2021). Extracellular vesicles, histone modification, and microRNA in the sperm and seminal fluid were related to generational stress transmission and neurodevelopmental programming in mice (Babenko et al., 2015; Morgan et al., 2019; Chan et al., 2020). Other possible candidates for transmitting generational exposures are the extracellular vesicles secreted by the annexes glands of the male reproductive system, such as epididymosomes, proteasomes, and liposomes (Sullivan and Saez, 2013; Bernardino, 2021). In addition, an interaction was observed between paternal treatment and offspring body weight in the present study, indicating that body weight can also influence behavior and environmental perception, specifically play behavior (Weller et al., 2019), social relationships linked to aggressiveness (Andersen et al., 2000), and nociception (Janczak et al., 2012; Sabei et al., 2023).
A treatment effect was also observed during the NO test; P piglets spent less time standing far away from the novel object than C and E piglets, indicating that these piglets exhibited less exploration or greater inactivity far from the object. These variables in the NO test are rarely reported in the scientific literature because the latency toward the NO is the primary variable studied (Schulz et al., 2022). In addition, the behavior showed is not clearly associated with animal affective states (Forkman et al., 2007). Another topic that could be related to our findings is the buffering effect of the gilts housing conditions. In a commercial scenario, gilts and sows do not have access to an environment like paddocks, which could lead to more discrepant outcomes than we found with the piglets. Likewise, animal ecological conditions have a potential role in changing digestive microbiota in the individual (Mitrea et al., 2022), which could modify social interactions, species-specific behaviors, and stress in their offspring (Janczak et al., 2012; Rodgers et al., 2013). Finally, the piglets' behavioural observation during the tests without the birth parents’ knowledge gives robustness to the collected data. However, increasing the number of evaluators could improve the reliability of the data.
We observed three clear piglet coping styles in the clustering analysis. The first was reduced exploration activity, fear, and anxiety. The second was increased exploration activity unrelated to fear or anxiety. The third was increased exploration activity, fear, and anxiety. However, there was no significant association between paternal treatment and piglet cluster. The majority of piglets in all three clusters were fathered by C boars (79%), and the distribution of piglets in the other two paternal treatments varied across clusters. Although there were no significant associations between piglet coping styles and paternal treatment, it would be interesting to include more animals in future studies, as this approach could provide us with an integrative understanding of coping styles in relation to parental experiences.
There is clear evidence that parental stress can be transmitted to offspring through epigenetic mechanisms, modifying the response of the HPA axis to challenges in the offspring (Rodgers et al., 2013; Nestler, 2016; Chan et al., 2020). The disorganization of the HPA axis due to maladaptive paternal stress can modulate the neuronal development of offspring, reducing their resilience in the face of possible challenges (Nestler, 2016; Chan et al., 2020). According to the literature, the HPA axis associated with the circadian rhythm of piglets at early age is not well organized (Evans et al., 1988; Poletto et al., 2006). We found a possible association between paternal housing conditions and the cortisol levels of their offspring when facing challenges of social isolation and novelty. The piglets exhibited different cortisol levels before and after the OF and NO tests. P and C piglets had a greater physiological stress-related response to OF and NO tests than E piglets. Thus, enrichment of boar housing conditions altered the HPA axis response of offspring during behavioral tests. In addition to behavioral changes, such evidence suggests that E piglets may have a better ability to cope with challenges, thereby increasing their resilience and improving their welfare. However, it is worth noting that our study utilized a single observer, and we acknowledge the potential for this approach to introduce limitations. In an ideal scenario, incorporating multiple observers would have further enhanced the reliability and validity of our observations. Nevertheless, given the available resources and practical constraints, we ensured rigorous training for our observer to mitigate potential biases. While evaluating intraobserver reliability would have been valuable, it is an aspect we plan to explore in future studies to strengthen the robustness of our findings.
5 Conclusions
The boar housing condition modulated the stress response and emotionality (anxiety, fear, and exploration) of their offspring according to behavioral tests, even though the duration of boar exposure to these housing conditions was short (only 4 weeks). Environments with fewer resources can modulate the vulnerability of male animal offspring or impose an adaptive disadvantage when offspring are exposed to challenges (Chan et al., 2020), as often observed in commercial pig housing systems. Given the effects of paternal housing environment on the emotionality and stress physiology of offspring, we propose that future studies investigate other possible impacts of the paternal environment, such as those related to offspring physical health. The mechanisms involved in the emotional changes reported in offspring in the current study seem to depend not only on the present characteristics (behavior and physiology), but also on epigenetic markers in the semen (Bernardino, 2021). We suggest that future studies consider these factors to clarify the mechanisms by which paternal environments can alter offspring emotionality.
Data availability statement
The raw data supporting the conclusions of this article will be made available by the authors, without undue reservation.
Ethics statement
The animal study was reviewed and approved by The Ethics Committee on Animal Use approved this study, protocol number CEUA 6555081018, University of São Paulo (USP) Campus Fernando Costa, Pirassununga—São Paulo, Brazil.
Author contributions
LS and MPS designed the study; acquired, analyzed, and interpreted the data; wrote the original draft; and prepared figures. TB designed the study, and acquired and interpreted the data. CÇ developed the statistical approach for data analysis, interpreted the data, and prepared figures. SF, MZ, and DS acquired data. RP interpreted the data. AZ generated the original concept, designed the study, obtained funding, and interpreted the data. All authors contributed to the revision of the final version. All authors contributed to the article and approved the submitted version.
Funding
This project was sponsored with a grant awarded to AZ from the Fundação de Amparo à Pesquisa do Estado de São Paulo (FAPESP), process number 2018/01082-04. LS received a scholarship from the Coordenação de Aperfeiçoamento de Pessoal de Nível Superior—Brazil (CAPES)—(CAPES/PROEX Funding Code 1841/2016 and 88887.626403/2021-00). TB received a scholarship from the FAPESP under process numbers 2017/05602-4 and 2018/01082-4. MP was awarded a scholarship from the University of Teramo, Italy.
Acknowledgments
We are thankful to the Brazilian farm Topgen that granted access to the animals used in the experiment. We are grateful to Certified Humane, which provided partial funds for developing the research project. Vedovati Pisos is acknowledged for donating the rubber mats used in the experimental work. Professor Cesar Augusto Pospissil Garbossa and Professor Andre Furugen Cesar de Andrade from the School of Veterinary and Animal Science, University of São Paulo (USP), provided access to facilities and laboratories to carry out the work. We are grateful to Dr. Harriet Bartlett for reviewing the manuscript. Biorender software was used to create drawings. Finally, we thank all students, professors, and staff involved in this research at the USP.
Conflict of interest
The authors declare that the research was conducted without any commercial or financial relationships that could be construed as a potential conflict of interest.
Publisher’s note
All claims expressed in this article are solely those of the authors and do not necessarily represent those of their affiliated organizations, or those of the publisher, the editors and the reviewers. Any product that may be evaluated in this article, or claim that may be made by its manufacturer, is not guaranteed or endorsed by the publisher.
Supplementary material
The Supplementary Material for this article can be found online at: https://www.frontiersin.org/articles/10.3389/fanim.2023.1208768/full#supplementary-material
References
Alves M. B. R., de Arruda R. P., De Bem T. H. C., Florez-Rodriguez S. A., Sá Filho M. F. de, Belleannée C., et al. (2019). Sperm-borne miR-216b modulates cell proliferation during early embryo development via K-RAS. Sci. Rep. 9, 1–14. doi: 10.1038/s41598-019-46775-8
Andersen L. I., Andenæs H., Bøe K. E., Jensen P., Bakken M. (2000). The effects of weight asymmetry and resource distribution on aggression in groups of unacquainted pigs. Appl. Anim. Behav. Sci. 68, 107–120. doi: 10.1016/S0168-1591(00)00092-7
Andersen I. L., Bøe K. E., Fœrevik G., Janczak A. M., Bakken M. (2000a). Behavioural evaluation of methods for assessing fear responses in weaned pigs. Appl. Anim. Behav. Sci. 69, 227–240. doi: 10.1016/S0168-1591(00)00133-7
Andersen I. L., Færevik G., Bøe K. E., Janczak A. M., Bakken M. (2000b). Effects of diazepam on the behaviour of weaned pigs in three putative models of anxiety. Appl. Anim. Behav. Sci. 68, 121–130. doi: 10.1016/S0168-1591(00)00098-8
Babenko O., Kovalchuk I., Metz G. A. S. (2015). Stress-induced perinatal and transgenerational epigenetic programming of brain development and mental health. Neurosci. Biobehav. Rev. 48, 70–91. doi: 10.1016/j.neubiorev.2014.11.013
Bale T. L. (2015). Epigenetic and transgenerational reprogramming of brain development. Nat. Publ Gr 16, 332–344. doi: 10.1038/nrn3818
Bateson M., Martin P. (2007). Measuring Behaviour: An Introductory Guide (4th ed.) (Cambridge: Cambridge University Press), 1–246. doi: 10.1017/9781108776462
Bernardino T. (2021). Boar welfare influence the quality of the gametes. [Ph.D.thesis]. (São Paulo (SP), Brazil: University of São Paulo) 1–109. doi: 10.11606/T.10.2021.tde-15032022-145834
Bernardino T., Tatemoto P., Morrone B., Mazza Rodrigues P. H., Zanella A. J. (2016). Piglets born from sows fed high fibre diets during pregnancy are less aggressive prior to weaning. PloS One 11, e0167363. doi: 10.1371/journal.pone.0167363
Bernardino T., Teodoro P. C. C., Batissaco L., Celeghini E. C. C., Zanella A. J. (2022). Poor welfare compromises testicle physiology in breeding boars. PloS One 17, e0268944. doi: 10.1371/journal.pone.0268944
Braun K., Champagne F. A. (2014). Paternal influences on offspring development: behavioural and epigenetic pathways neuroendocrinology. J. Neuroendocrinol 26, 697–706. doi: 10.1111/jne.12174
Brooks M., Elizabeth, Kristensen K., Van Benthem K. J., Magnusson A., Berg C., et al. (2017). glmmTMB balances speed and flexibility among packages for zero-inflated generalized linear mixed modeling. R J. 9, 378–400. doi: 10.32614/RJ-2017-066
Broom D. M., Mendl M. T., Zanella A. J. (1995). A comparison of the welfare of sows in different housing conditions. Anim. Sci. 61, 369–385. doi: 10.1017/S1357729800013928
Çakmakçı C. (2022). Sheep’s coping style can be identified by unsupervised machine learning from unlabeled data. Behav. Processes 194, 104559. doi: 10.1016/J.BEPROC.2021.104559
Carobrez A. P., Bertoglio L. J. (2005). Ethological and temporal analyses of anxiety-like behavior: the elevated plus-maze model 20 years on. Neurosci. Biobehav. Rev. 29, 1193–1205. doi: 10.1016/j.neubiorev.2005.04.017
Champagne F. A. (2020). Interplay between paternal germline and maternal effects in shaping development: the overlooked importance of behavioural ecology. Funct. Ecol. 34, 401–413. doi: 10.1111/1365-2435.13411
Chan J. C., Morgan C. P., Adrian Leu N., Shetty A., Cisse Y. M., Nugent B. M., et al. (2020). Reproductive tract extracellular vesicles are sufficient to transmit intergenerational stress and program neurodevelopment. Nat. Commun. 11, 1499. doi: 10.1038/s41467-020-15305-w
Colonnello V., Iacobucci P., Newberry R. C. (2010). Vocal and locomotor responses of piglets to social isolation and reunion. Dev. Psychobiol 52, 1–12. doi: 10.1002/dev.20406
Dawkins M. S. (2017). Animal welfare and efficient farming: is conflict inevitable? Anim. Prod Sci. 57, 201–208. doi: 10.1071/AN15383
Donald R. D., Healy S. D., Lawrence A. B., Rutherford K. M. D. (2011). Emotionality in growing pigs: is the open field a valid test? Physiol. Behav. 104, 906–913. doi: 10.1016/j.physbeh.2011.05.031
Donkin I., Barrès R. (2018). Sperm epigenetics and influence of environmental factors. Mol. Metab. 14, 1–11. doi: 10.1016/j.molmet.2018.02.006
Evans F. D., Christopherson R. J., Aherne F. X. (1988). Development of the circadian rhythm of cortisol in the gilt from weaning until puberty. Can. J. Anim. Sci. 68, 1105–1111. doi: 10.4141/cjas88-126
Farias S. S., Montechese A. C. D., Bernardino T., Rodrigues P. H. M., de Araujo Oliveira C. A., Zanella A. J. (2021). Two hours of separation prior to milking: is this strategy stressful for jennies and their foals? Animals 11, 1–17. doi: 10.3390/ani11010178
Farias S. S., Dierings A. C., Mufalo V. C., Sabei L., Parada Sarmiento M., Silva A. N. da, et al. (2023). Asinine milk mitigates stress-mediated immune, cortisol and behavioral responses of piglets to weaning: a study to foster future interventions in humans. Front. Immunol. 14. doi: 10.3389/fimmu.2023.1139249
Forkman B., Boissy A., Meunier-Salaün M.-C., Canali E., Jones R. B. (2007). A critical review of fear tests used on cattle, pigs, sheep, poultry and horses. Physiol. Behav. 92, 340–374. doi: 10.1016/j.physbeh.2007.03.016
Fox J., Weisberg S. (2019) An r companion to applied regression. Available at: https://socialsciences.mcmaster.ca/jfox/Books/Companion/ (Accessed 23, 2022).
Fraser D. (2008). Understanding animal welfare. Acta Vet. Scand. 50, S1. doi: 10.1186/1751-0147-50-S1-S1
Friard O., Gamba M. (2016). BORIS: a free, versatile open-source event-logging software for video/audio coding and live observations. Methods Ecol. Evol. 7, 1325–1330. doi: 10.1111/2041-210X.12584
Guerrero-Bosagna C., Pértille F., Gomez Y., Rezaei S., Gebhardt-Henrich S. G., Vögeli S., et al. (2020). DNA Methylation variation in the brain of laying hens in relation to differential behavioral patterns. Comp. Biochem. Physiol. - Part D Genomics Proteomics 35, 100700. doi: 10.1016/j.cbd.2020.100700
Haigh A., Chou J. Y., O’Driscoll K. (2020). Variations in the behavior of pigs during an open field and novel object test. Front. Vet. Sci. 7. doi: 10.3389/fvets.2020.00607
Hopkins B., Skellam J. G. (1954). A new method for determining the type of distribution of plant individuals. Ann Bot 18, 213–227. doi: 10.1093/oxfordjournals.aob.a083391
Hothorn T., Bretz F., Westfall P. (2008). Simultaneous inference in general parametric models. Biom J. 50, 346–363. doi: 10.1002/BIMJ.200810425
Hötzel M. J., Machado Filho L. C. P. (2004). Bem-estar animal na agricultura do século XXI. Rev. Etol 6, 3–15.
Husson F., Josse J., Pagès J. (2010). Principal component methods - hierarchical clustering - partitional clustering: why would we need to choose for visualizing data? Formaliz Math 18, 17–26. doi: 10.2478/v10037-010-0003-0
Janczak A. M., Andersen I. L., Bøe K. E., Færevik G., Bakken M. (2002). Factor analysis of behaviour in the porcine and murine elevated plus-maze models of anxiety. Appl. Anim. Behav. Sci. 77, 155–166. doi: 10.1016/S0168-1591(02)00030-8
Janczak A. M., Pedersen L. J., Jensen K. H., Andersen I. L., Bøe K. E., Bakken M. (2000). No effect of variation in handling on behaviour in a porcine elevated plus-maze- a brief report. Appl. Anim. Behav. Sci. 69, 169–173. doi: 10.1016/S0168-1591(00)00111-8
Janczak A. M., Ranheim B., Fosse T. K., Hild S., Nordgreen J., Moe R. O., et al. (2012). Factors affecting mechanical (nociceptive) thresholds in piglets. Vet. Anaesth Analg 39, 628–635. doi: 10.1111/j.1467-2995.2012.00737.x
Johnson E. O., Kamilaris T. C., Chrousos G. P., Gold P. W. (1992). Mechanisms of stress: a dynamic overview of hormonal and behavioral homeostasis. Neurosci. Biobehav. Rev. 16, 115–130. doi: 10.1016/S0149-7634(05)80175-7
Josse J., Husson F. (2016). missMDA: a package for handling missing values in multivariate data analysis. J. Stat. Softw 70, 1–31. doi: 10.18637/JSS.V070.I01
Kaiser H. F., Rice J. (2016). Little jiffy, mark iv. Educ. Psychol. Meas. 34, 111–117. doi: 10.1177/001316447403400115
Kanitz E., Hameister T., Tuchscherer A., Tuchscherer M., Puppe B. (2016). Social support modulates stress-related gene expression in various brain regions of piglets. Front. Behav. Neurosci. 10. doi: 10.3389/fnbeh.2016.00227
Kassambara A., Mundt F. (2020) Factoextra extract and visualize the results of multivariate data analyses. Available at: https://scirp.org/reference/referencespapers.aspx?referenceid=3067217 (Accessed 14, 2023).
Lacal I., Ventura R. (2018). Epigenetic inheritance: concepts, mechanisms and perspectives. Front. Mol. Neurosci. 11. doi: 10.3389/fnmol.2018.00292
Lawson R. G., Jurs P. C. (1990). New index for clustering tendency and its application to chemical problems. J. Chem. Inf Comput. Sci. 30, 36–41. doi: 10.1021/CI00065A010/ASSET/CI00065A010.FP.PNG_V03
Le S., Josse J., Husson F. (2008). FactoMineR: An R package for multivariate analysis. J. Stat. Software 25, 1–18. doi: 10.18637/jss.v025.i01
Lüdecke D., Ben-Shachar M. S., Patil I., Waggoner P., Makowski D. (2021). Performance: an r package for assessment, comparison and testing of statistical models. J. Open Source Softw 6, 3139. doi: 10.21105/JOSS.03139
Marcho C., Oluwayiose O. A., Pilsner J. R. (2020). The preconception environment and sperm epigenetics. Andrology 8, 924–942. doi: 10.1111/andr.12753
Marczylo E. L., Amoako A. A., Konje J. C., Gant T. W., Marczylo T. H. (2012). Smoking induces differential miRNA expression in human spermatozoa: a potential transgenerational epigenetic concern? Epigenetics 7, 432–439. doi: 10.4161/epi.19794
Meaney M. J., Szyf M. (2005). Maternal care as a model for experience-dependent chromatin plasticity? Trends Neurosci. 28, 456–463. doi: 10.1016/j.tins.2005.07.006
Mitrea L., Nemeş S. A., Szabo K., Teleky B. E., Vodnar D. C. (2022). Guts imbalance imbalances the brain: a review of gut microbiota association with neurological and psychiatric disorders. Front. Med. 9. doi: 10.3389/fmed.2022.813204
Morgan C. P., Chan J. C., Bale T. L., Baxter E. M., Jarvis S., Palarea-Albaladejo J., et al. (2019). Driving the next generation: paternal lifetime experiences transmitted via extracellular vesicles and their small RNA cargo. Biol. Psychiatry 85, 164–171. doi: 10.1016/j.biopsych.2018.09.007
Muráni E., Ponsuksili S., D’Eath R. B., Turner S. P., Kurt E., Evans G., et al. (2010). Association of HPA axis-related genetic variation with stress reactivity and aggressive behaviour in pigs. BMC Genet. 11, 74. doi: 10.1186/1471-2156-11-74
Nery da Silva A., Silva Araujo M., Pértille F., Zanella A. J. (2021). How epigenetics can enhance pig welfare? Animals 12, 32. doi: 10.3390/ani12010032
Nestler E. J. (2016). Transgenerational epigenetic contributions to stress Responses: fact or fiction. PloS Biol. 14, e1002426. doi: 10.1371/journal.pbio.1002426
Nilsson E. E., Sadler-Riggleman I., Skinner M. K. (2018). Environmentally induced epigenetic transgenerational inheritance of disease. Environ. Epigenet. 4, 1–13. doi: 10.1093/eep/dvy016
Otten W., Kanitz E., Tuchsherer M. (2015). The impact of pre-natal stress on offspring development in pigs. J. Agric. Sci. 153, 907–919. doi: 10.1017/S0021859614001361
Pagès J. (2014). Multiple factor analysis by example using r. Mult Factor Anal. by Ex Using R, 1–253. doi: 10.1201/B17700/MULTIPLE-FACTOR-ANALYSIS-EXAMPLE-USING-J
Pagès J. (2015) Multiple factor analysis by example using r (CRC Press Taylor & Francis Group). Available at: https://books.google.com/books?hl=pt-BR&lr=&id=BR5jDAAAQBAJ&oi=fnd&pg=PP1&dq=Pag%C3%A8s,+J.+2015.+Multiple+Factor+Analysis+by+Example+Using+R.+CRC+Press&ots=irRzNSavt7&sig=mEkvWRlhD232UlzPUgjxDFbabw0#v=onepage&q&f=false (Accessed 14, 2023).
Palme R. (2019). Non-invasive measurement of glucocorticoids: advances and problems. Physiol. Behav. 199, 229–243. doi: 10.1016/j.physbeh.2018.11.021
Palme R., Möstl E. (1997). Measurement of cortisol metabolites in faeces of sheep as a parameter of cortisol concentration in blood. Int. J. Mamm Biol. 62, 192–197.
Panksepp J. (2007). Neurologizing the psychology of affects: how appraisal-based constructivism and basic emotion theory can coexist. Perspect. Psychol. Sci. 2, 281–296. doi: 10.1111/j.1745-6916.2007.00045.x
Patil I. (2021). Visualizations with statistical details: the “ggstatsplot” approach. J. Open Source Softw 6, 3167. doi: 10.21105/JOSS.03167
Paul E. S., Harding E. J., Mendl M. (2005). Measuring emotional processes in animals: the utility of a cognitive approach. Neurosci. Biobehav. Rev. 29, 469–491. doi: 10.1016/j.neubiorev.2005.01.002
Poletto R., Steibel J. P., Siegford J. M., Zanella A. J. (2006). Effects of early weaning and social isolation on the expression of glucocorticoid and mineralocorticoid receptor and 11β-hydroxysteroid dehydrogenase 1 and 2 mRNAs in the frontal cortex and hippocampus of piglets. Brain Res. 1067, 36–42. doi: 10.1016/j.brainres.2005.10.001
Puppe B., Ernst K., Schön P. C., Manteuffel G. (2007). Cognitive enrichment affects behavioural reactivity in domestic pigs. Appl. Anim. Behav. Sci. 105, 75–86. doi: 10.1016/j.applanim.2006.05.016
Puppe B., Schön P. C., Wendland K. (1999). Monitoring of piglets’ open field activity and choice behaviour during the replay of maternal vocalization: a comparison between observer and PID technique. Lab. Anim. 33, 215–220. doi: 10.1258/002367799780578110
Reimert I., Bolhuis J. E., Kemp B., Rodenburg T. B. (2013). Indicators of positive and negative emotions and emotional contagion in pigs. Physiol. Behav. 109, 42–50. doi: 10.1016/j.physbeh.2012.11.002
Revelle W. (2022) Procedures for psychological, psychometric, and personality research [R package psych version 2.2.9]. Available at: https://cran.r-project.org/package=psych (Accessed 23, 2022).
Rodgers A. B., Bale T. L. (2015). Germ cell origins of posttraumatic stress disorder risk: the transgenerational impact of parental stress experience. Biol. Psychiatry 78, 307–314. doi: 10.1016/j.biopsych.2015.03.018
Rodgers R. J., Dalvi A. (1997). Anxiety, defence and the elevated plus-maze. Neurosci. Biobehav. Rev. 21, 801–810. doi: 10.1016/S0149-7634(96)00058-9
Rodgers R. J., Johnson N. J. T. (1995). Factor analysis of spatiotemporal and ethological measures in the murine elevated plus-maze test of anxiety. Pharmacol. Biochem. Behav. 52, 297–303. doi: 10.1016/0091-3057(95)00138-M
Rodgers A. B., Morgan C. P., Bronson S. L., Revello S., Bale T. L. (2013). Paternal stress exposure alters sperm microRNA content and reprograms offspring HPA stress axis regulation. J. Neurosci. 33, 9003–9012. doi: 10.1523/JNEUROSCI.0914-13.2013
Rodgers A. B., Morgan C. P., Leu N. A., Bale T. L. (2015). Transgenerational epigenetic programming via sperm microRNA recapitulates effects of paternal stress. Proc. Natl. Acad. Sci. 112, 13699–13704. doi: 10.1073/pnas.1508347112
Rutherford K. M. D., Piastowska-ciesielska A., Donald R. D., Robson S. K., Ison S. H., Jarvis S., et al. (2014). Prenatal stress produces anxiety prone female offspring and impaired maternal behaviour in the domestic pig. Physiol. Behav. 129, 255–264. doi: 10.1016/j.physbeh.2014.02.052
Sabei L., Bernardino T., Parada Sarmiento M., Barbosa B. S., Farias S de S., Ghantous G. F., et al. (2023). Life experiences of boars can shape the survival, aggression, and nociception responses of their offspring. Front. Anim. Sci. 4. doi: 10.3389/fanim.2023.1142628
Saraiva E. M., Furtunato J. M. S., Gavina C. (2005). Osilações do cortisol na depressão e no sono/vigília. Rev. Port Psicossomática 7, 89100.
Sarmiento M. P., Bernardino T., Tatemoto P., Polo G., Zanella A. J. (2021). The in-utero experience of piglets born from sows with lameness shapes their life trajectory. Sci. Rep. 11, 1–11. doi: 10.1038/s41598-021-92507-2
Schiele M. A., Domschke K. (2018). Epigenetics at the crossroads between genes, environment and resilience in anxiety disorders. Genes Brain Behav. 17, 1–15. doi: 10.1111/gbb.12423
Schulz M., Zieglowski L., Kopaczka M., Tolba R. H. (2022). The open field test as a tool for behaviour analysis in pigs – recommendations for set-up standardisation. A systematic review. Eur. Surg. Res. 64, 726. doi: 10.1159/000525680
Searle S. R., Speed F. M., Milliken G. A. (2022). Population marginal means in the linear model: an alternative to least squares means. Am Stat 34, 216–241. doi: 10.1080/00031305.1980.10483031
Siddeek B., Mauduit C., Simeoni U., Benahmed M. (2018). Sperm epigenome as a marker of environmental exposure and lifestyle, at the origin of diseases inheritance. Mutat. Res. - Rev. Mutat. Res. 778, 38–44. doi: 10.1016/j.mrrev.2018.09.001
Siegford J. M., Rucker G., Zanella A. J. (2008). Effects of pre-weaning exposure to a maze on stress responses in pigs at weaning and on subsequent performance in spatial and fear-related tests. Appl. Anim. Behav. Sci. 110, 189–202. doi: 10.1016/j.applanim.2007.03.022
Skinner M. K., Guerrero-Bosagna C. (2009). Environmental signals and transgenerational epigenetics. Epigenomics 1, 111–117. doi: 10.2217/epi.09.11
Sorrells S. F., Caso J. R., Munhoz C. D., Sapolsky R. M. (2009). The stressed CNS: when glucocorticoids aggravate inflammation. Neuron 64, 33–39. doi: 10.1016/j.neuron.2009.09.032
Soubry A., Hoyo C., Jirtle R. L., Murphy S. K. (2014). A paternal environmental legacy: evidence for epigenetic inheritance through the male germ line. BioEssays 36, 359–371. doi: 10.1002/bies.201300113
Sullivan R., Saez F. (2013). Epididymosomes, prostasomes, and liposomes: their roles in mammalian male reproductive physiology. REPRODUCTION 146, R21–R35. doi: 10.1530/REP-13-0058
Tarou L. R., Bashaw M. J. (2007). Maximizing the effectiveness of environmental enrichment: suggestions from the experimental analysis of behavior. Appl. Anim. Behav. Sci. 102, 189–204. doi: 10.1016/j.applanim.2006.05.026
Tatemoto P., Bernardino T., Alves L., Cristina de Oliveira Souza A., Palme R., José Zanella A. (2019a). Environmental enrichment for pregnant sows modulates HPA-axis and behavior in the offspring. Appl. Anim. Behav. Sci. 220, 104854. doi: 10.1016/j.applanim.2019.104854
Tatemoto P., Bernardino T., Alves L., Zanella A. J. (2019b). Sham-chewing in sows is associated with decreased fear responses in their offspring. Front. Vet. Sci. 6. doi: 10.3389/fvets.2019.00390
Tatemoto P., Bernardino T., Morrone B., Queiroz M. R., Zanella A. J. (2020). Stereotypic behavior in sows is related to emotionality changes in the offspring. Front. Vet. Sci. 7. doi: 10.3389/fvets.2020.00079
Tatemoto P., Pértille F., Bernardino T., Zanella R., Zanella A. J., Tatemoto P., et al. (2023). An enriched maternal environment and stereotypies of sows differentially affect the neuro-epigenome of brain regions related to emotionality in their piglets. Epigenetics 18, 127. doi: 10.1080/15592294.2023.2196656
Team R Core (2022) R: a language and environment for statistical computing. Available at: https://www.r-project.org/.
Temple D., Manteca X., Velarde A., Dalmau A. (2011). Assessment of animal welfare through behavioural parameters in Iberian pigs in intensive and extensive conditions. Appl. Anim. Behav. Sci. 131, 29–39. doi: 10.1016/j.applanim.2011.01.013
Weller J. E., Camerlink I., Turner S. P., Farish M., Arnott G. (2019). Playful pigs: early life play-fighting experience influences later life contest dynamics. Anim. Behav. 158, 269–279. doi: 10.1016/j.anbehav.2019.09.019
Zhao W. L., Gu N. H., Li Z. Z., Wang G. S., Cheng C. Y., Sun F. (2020). Autism-like behaviors and abnormality of glucose metabolism in offspring derived from aging males with epigenetically modified sperm. Aging (Albany NY) 12, 19766–19784. doi: 10.18632/AGING.104061
Zheng X., Li Z., Wang G., Wang H., Zhou Y., Zhao X., et al. (2021). Sperm epigenetic alterations contribute to inter- and transgenerational effects of paternal exposure to long-term psychological stress via evading offspring embryonic reprogramming. Cell Discovery 7, 101. doi: 10.1038/s41421-021-00343-5
Keywords: behavioral test, fetal programming, paternal stress, pig, piglets, stress, swine, welfare
Citation: Sabei L, Parada Sarmiento M, Bernardino T, Çakmakçı C, Farias SS, Sato D, Zanella MIG, Poletto R and Zanella AJ (2023) Inheriting the sins of their fathers: boar life experiences can shape the emotional responses of their offspring. Front. Anim. Sci. 4:1208768. doi: 10.3389/fanim.2023.1208768
Received: 19 April 2023; Accepted: 20 June 2023;
Published: 10 July 2023.
Edited by:
Kyle McLean, The University of Tennessee, Knoxville, United StatesReviewed by:
Cécile Bienboire-Frosini, Institut de Recherche en Sémiochimie et Ethologie Appliquée (IRSEA), FranceAbdelkareem Ahmed, Botswana University of Agriculture and Natural Resources, Botswana
Copyright © 2023 Sabei, Parada Sarmiento, Bernardino, Çakmakçı, Farias, Sato, Zanella, Poletto and Zanella. This is an open-access article distributed under the terms of the Creative Commons Attribution License (CC BY). The use, distribution or reproduction in other forums is permitted, provided the original author(s) and the copyright owner(s) are credited and that the original publication in this journal is cited, in accordance with accepted academic practice. No use, distribution or reproduction is permitted which does not comply with these terms.
*Correspondence: Adroaldo José Zanella, YWRyb2FsZG8uemFuZWxsYUB1c3AuYnI=; Leandro Sabei, c2FiZWlfbGVAYWx1bW5pLnVzcC5icg==
†These authors have contributed equally to this work and share first authorship