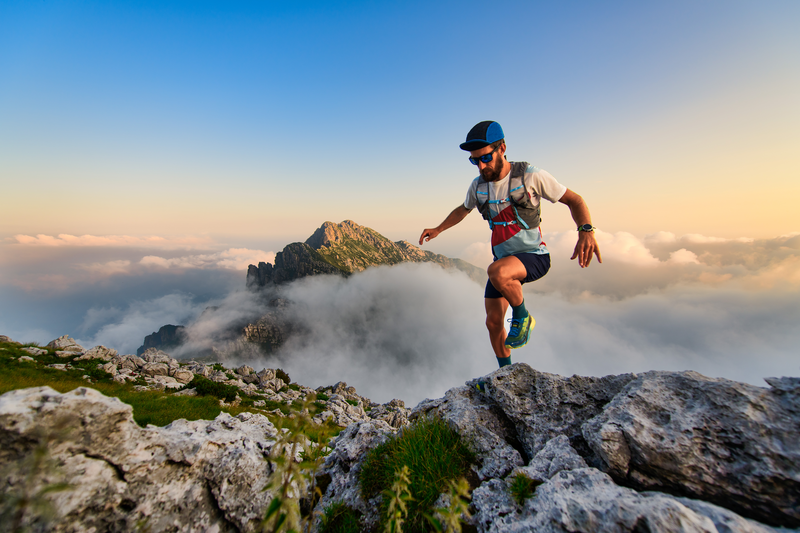
94% of researchers rate our articles as excellent or good
Learn more about the work of our research integrity team to safeguard the quality of each article we publish.
Find out more
REVIEW article
Front. Anim. Sci. , 19 July 2023
Sec. Animal Nutrition
Volume 4 - 2023 | https://doi.org/10.3389/fanim.2023.1204863
Pork is one of the most consumed animal-derived protein sources around the world, but less than 45% of dietary nitrogen (N) is converted into edible lean meat. This inefficiency contributes to the 80% of human-induced N emissions that have resulted in exceeding the planetary boundaries for N. Reducing this inefficiency requires using a multifaceted approach. Swine genetic selection programs have improved the rate of lean growth in recent decades, but further improvements may be difficult because of lack of genetic variation within the global pig population. Breeding programs to increase litter size have led to intrauterine growth restriction and an increased proportion of low-birth-weight piglets which do not utilize dietary protein as efficiently as normal birth weight pigs. Intact males have greater lean growth rate and N efficiency than castrates and gilts, and the use of immunocastration technology has been implemented in some countries to capture this benefit while minimizing boar taint in pig meat. Use of other metabolic modifiers including porcine somatotropin and ractopamine, alone or in combination with immunocastration, can further enhance lean growth and dietary N efficiency, but a general lack of consumer acceptance and potential food safety concerns have led to regulatory restrictions in many countries. Because feed production contributes about 70% of N emissions in pig production systems, use of precision feed formulation and feeding practices, selected dietary feed additives, feed processing, and minimizing feed wastage appear to offer the greatest opportunity to reduce N waste and environmental footprint to improve the sustainability of pork production.
Globally, only 20% of nitrogen (N) is retained in useful products with 80% of various N forms lost to the environment (Sutton et al., 2019). This inefficiency in N utilization contributes to the human-induced N emissions that have resulted in exceeding the planetary boundaries for N (Rockström et al., 2009) and led the United Nations to launch a global initiative on Sustainable Nitrogen Management (UNEP, 2019). This initiative involves efforts to mitigate N pollution by focusing on strategies that enhance the efficiency of N utilization in agricultural production (Liu et al., 2020). Although animal agriculture has been criticized as being a major contributor to many environmental problems including climate change, air pollution, land degradation, water shortage and pollution, and loss of biodiversity, it is also an indispensable component of our global food system, economies, and society (Steinfeld et al., 2006). Food producing farm animals contribute 40% of the total agricultural gross domestic product, employ 1.3 billion people, create livelihoods for 1 billion poor people, provide 33% of dietary protein intake, and contribute essential nutrients for overcoming undernourishment (Steinfeld et al., 2006). However, the global livestock industry also contributes about one-third of human-induced N emissions (i.e., nitrates, ammonia, nitrous oxide, and other N oxides; Uwizeye et al., 2020). Although methane (CH4) and carbon dioxide (CO2) are the major greenhouse gas (GHG) emissions from ruminants, nitrous oxide (N2O) emissions are the predominant GHG produced from pig manure, which is a much more potent contributor to increased global warming potential compared with CO2 and CH4. Although N2O is present in the atmosphere at a lower concentration (6%) compared with CH4 (16%) and CO2 (76%), its global warming potential is nearly 10 times greater than CH4 and nearly 300 times greater than CO2 (U.S. EPA https://www.epa.gov/ghgemissions/understanding-global-warming-potentials). In addition, ammonia and N oxides contribute to air pollution, cause acidification and eutrophication, and pose risks to human health (Galloway et al., 2008). Nitrates and organic-N also contribute to water pollution and biodiversity loss (Erisman et al., 2013).
Historically, pork was the most produced and consumed animal-derived protein source in the world until recently, but now follows poultry (FAO, 2022). Future consumer demand for all animal protein sources, including pork, is expected to increase in the coming decades (MacLeod et al., 2013). From a N utilization efficiency (NUE) perspective, pigs (10 to 44%) have an advantage over beef cattle (4 to 8%), are comparable to dairy cattle (15 to 35%), but are less efficient than poultry (25 to 62%) for their ability to convert dietary N into edible food products (Gerber et al., 2014). Pork supply chains are complex systems beginning with the production of fertilizers and chemicals to produce feed crops, production of by-products used as feed ingredients, feed processing, animal housing and production, transportation of feed and animals, water use for drinking and cleaning, energy use for light, heat, and ventilation, and manure management (McAuliffe et al., 2016). Uwizeye et al. (2020) reported that poultry and pork supply chains contribute 29% of the total N emissions from food animal production, with 68% of these emissions being associated with feed production. Therefore, feed formulation, selected feed additives, feed manufacturing processes, and precision feeding strategies that minimize N waste appear to offer the greatest opportunity for improvement in NUE. However, it is also important to consider the feasibility and potential magnitude for improvement in NUE through genetic selection and use of metabolic modifiers.
Improving NUE in pork production is only possible by defining the criteria for measurement and establishing baselines to evaluate progress over time. The ultimate goal of feeding pigs is to produce safe, wholesome, lean, and nutritious pork with the fewest resources and least environmental impact. To accomplish this goal, NUE needs to be quantified at multiple levels of scale beginning at the individual pig level. Whole body N efficiency is commonly used as the measure for expressing NUE and is calculated as the amount of N retained in the body divided by the amount of N consumed by the animal. Millet et al. (2018) proposed using standardized ileal digestible (SID) lysine (Lys) per kg lean meat as a more specific functional measure of NUE because dietary protein requirements are expressed relative to SID Lys and the ultimate goal of pig production is to produce meat. These are reasonable measures to use at the individual animal level but nutrient requirements and subsequent performance varies among individual pigs, and pigs are housed and raised in groups in pens and barns on farms. Therefore, it is difficult to measure and manage individual differences in NUE between pigs under commercial conditions, which is essential if measurable improvements are to be made. Gerber et al. (2014) suggested that new indicators and approaches are required to assess nutrient use in longer, more complex livestock supply chains beyond individual animals or production units. From a pork production systems perspective, it is also useful to use a mass balance approach to calculate NUE at the farm level using nutrients and pigs as inputs and kg live weight, kg carcass weight, kg lean meat, or kg N in manure as outputs to optimize economic and environmental efficiency. Therefore, NUE should be assessed at multiple levels of scale using multiple criteria to reduce N waste from pork production systems to meet the global goals of Sustainable Nitrogen Management (UNEP, 2019).
Any factor (e.g., genetic changes, sex, feeding programs, feed ingredients, feed additives) that affects the rate of whole-body protein gain, and/or the proportion of muscle accretion relative to non-muscle gain, ultimately affects the amino acid (AA) needs of an animal (i.e., maintenance versus productive needs). This is primarily due to the differences in AA composition of different tissues (Beach et al., 1943; Lloyd et al., 1978; Bikker et al., 1994; Conde-Aguilera et al., 2014; Dai et al., 2014), differential rates of tissue growth over time, and turnover rates (i.e., fractional rate of synthesis versus fractional rate of degradation) of AA within tissues. Lysine (Lys) is used as a standard reference for AA utilization efficiency because of its central role in the ideal AA concept and it being the most studied AA in swine nutrition (Kim et al., 2009a; NRC, 2012; van Milgen and Dourmad, 2015). Digestibility of AA are expressed on an apparent ileal digestibility (AID) or standardized ileal digestibility basis. Use of SID is preferred over AID because it accounts for basal endogenous losses, which vary among individual AA, can represent 4% of N intake in 50 kg growing pigs (Lautrou et al., 2022), is independent of dietary AA and nutrient composition, and values are additive among feedstuffs used in diet formulations (Stein et al., 2007).
The potential for improving the efficiency of AA utilization requires understanding marginal efficiencies of using SID Lys for maintenance and retention in addition to maintenance needs. Modeling of published empirical data reported in the NRC (2012) resulted in using estimates of the marginal efficiency of SID Lys for protein deposition of 0.682 at 20 kg body weight (BW) and 0.586 at 120 kg BW, indicating that the marginal efficiency of using SID Lys for protein deposition declines with increasing BW. This reduction in efficiency also accounts for between-animal variability (NRC, 2012) where the coefficient of variation for BW gain among pigs of similar BW in commercial production facilities is often greater than 10% (de Lange et al., 2012). In addition, these efficiency estimates of using SID Lys for protein deposition are less than the SID Lys estimate of 0.75 for maintenance (NRC, 2012). This difference in Lys efficiency is somewhat reflected by examining the empirical Lys data reported in NRC (2012), where approximately 19.3 g SID Lys/kg BW gain is required at 20 kg BW, but 20.0 g SID Lys/kg BW gain is needed at 120 kg BW. On a whole-body protein deposition basis, using 7.1 g of Lys per 100 g whole-body protein deposition is equivalent to 10.4 and 12.5 g SID Lys per 100 g whole-body protein deposition at 20 and 120 kg BW, respectively (NRC, 2012). Thus, as pigs are marketed at increasing BW, with increased rates of lean tissue deposition per day as well as increased rates of lean tissue deposition relative to rates of deposition of other non-lean tissues (Strathe et al., 2009; Danfær and Strathe, 2012), the increases in growth and protein deposition may be occurring during a period of growth where marginal efficiencies are decreasing (at least for Lys). Similar to Lys, there are maintenance and retention efficiency values for all AA (NRC, 2012; van Milgen and Dourmad, 2015), but often there is a lack of empirical data to confirm these values. For example, although there are 42 data points available for Lys requirement estimates, only 19 data estimates are available for tryptophan, 13 for threonine, 11 for isoleucine, 7 for valine, and only 1 data point is available for each of several other indispensable AA (i.e., arginine, histidine, isoleucine, and leucine) as reported in NRC (2012). Therefore, more research is needed to improve our understanding of the nutritional needs for each of these indispensable AA as well as potential opportunities for improving AA utilization efficiencies for metabolic purposes.
The fate of undigested and unabsorbed AA and N entering the large intestine has generally been considered to have minimal nutritional value in pigs because several studies have shown limited or no benefit of infusing individual or protein bound AA into the large intestine (Fuller and Reeds, 1998). This assumption has been based on studies that have shown no improvements in whole-body N balance when pigs fed Lys or sulfur AA limiting diets were infused with Lys (Wünsche et al., 1982) or methionine (Darragh et al., 1994) in the large intestine. However, other studies (James and Smith, 1976; Smith and James, 1976; Gargallo and Zimmerman, 1981; Blachier et al., 2007) have suggested that AA uptake occurs in colonocytes through AA transporters. In addition, N absorption from the large intestine is predominantly in the form of ammonia produced from microbial fermentation of undigested protein (Fuller and Reeds, 1998). A large proportion of absorbed ammonia is detoxified to urea in the liver and excreted in the urine (Canh et al., 1997), but a significant portion of urea is also recycled into the gastrointestinal tract (Jackson et al., 1984; Mosenthin et al., 1992). Most of the recycled urea enters the small intestine of pigs (Mosenthin et al., 1992) where urea is hydrolyzed to provide N for microbial AA synthesis which can be absorbed and utilized as an endogenous AA source (Torrallardona et al., 2003). Columbus et al. (2014) fed a valine-limiting diet to growing pigs and infused casein or urea into the large intestine and observed that 80% of infused N was absorbed and whole-body N retention improved regardless of N source. Results from this study suggest that absorption of N from the large intestine appears to be in the form of nonprotein N, which is returned to the small intestine in the form of urea and subsequently used for microbial AA production in the cecum and large intestine. Therefore, N metabolism in the large intestine should be considered when determining N and AA requirements and dietary supply (Columbus et al., 2014).
Kornegay and Harper (1997) summarized results from studies showing that nursery pigs retain a greater percentage of N consumed (40 to 50%) than finishing pigs (30 to 50%), gestating sows (35 to 45%), and lactating sows (20 to 40%). These inefficiencies of N utilization are relatively low and indicate that there are tremendous opportunities for improvement through dietary interventions. Efficiencies of dietary SID AA utilization for maintenance, protein accretion, and milk protein production for growing-finishing pigs and gestating and lactating sows have been summarized (NRC, 2012). In general, AA utilization efficiency for maintenance functions range from 60% (methionine + cysteine) to 147% (arginine) among growth, gestation, and lactation phases, but AA retention efficiencies are greater for growing-finishing pigs (52 to 127%) than for gestating sows (40 to 96%) and lactating sows (58 to 82%). The lower AA utilization for sows is due to greater mobilization of AA from body protein compared with growing-finishing pigs. In gestating sows, protein retention is a function of developing fetuses, mammary tissue, placenta and chorioallantoic fluid, uterus, and maternal body protein deposition (NRC, 2012). During lactation, energy intake determines the extent of maternal body protein utilization for milk protein synthesis based on adequacy of dietary AA intake above maintenance requirements. Improving our understanding of potential improvements in AA utilization efficiency in each stage of swine production requires addition research to obtain more information on:
● Estimates of minimal catabolism of individual AA for maintenance.
● Estimates of intestinal endogenous ileal AA losses for gestating and lactating sows.
● Estimates of intestinal endogenous losses of AA based on effects of dietary fiber and antinutritional factors.
● Amino acid composition of mammary tissue, placenta, and uterus tissue at various stages of gestation.
A major contributor to inefficiency of N and nutrient utilization in swine production is intrauterine growth restriction or retardation (IUGR). Intrauterine growth restriction has been defined as impaired growth and development of mammalian embryos and fetuses and their organs during pregnancy (Romanelli et al., 2019) resulting from insufficient uptake of nutrients, especially AA, from the placenta (Lin et al., 2014). Genetic selection for increased litter size is the primary factor contributing to IUGR because of uterine crowding and increased nutrient demand during gestation (Town et al., 2004; Foxcroft et al., 2009). Estimates for the incidence of IUGR piglets born in litters produced on commercial swine farms range from 15 to 20% (Wu et al., 2006) to as much as 30% (Amdi et al., 2013; Hales et al., 2013). As a result, IUGR pigs contributes to significant economic losses associated with low birth weight which is associated with increased mortality and morbidity, impaired gastrointestinal tract development, a permanent reduction in growth rate, feed utilization, and a malfunctioning immune system throughout the entire life cycle (Wang et al., 2005; Dong et al., 2014; Zhang et al., 2015; Che et al., 2017). Resistance of IUGR piglets to a pathogen challenge post-weaning is compromised due to a moderate suppression of the immune response (Amdi et al., 2020). In addition, IUGR piglets have altered plasma hormone concentrations, differences in alpha diversity and metabolic capabilities of small intestine microbial communities (Xiong et al., 2020), as well as heavier brain, liver, lungs, and adrenal gland weight, and reduced blood glucose levels at birth (Lynegaard et al., 2020). Wang et al. (2013) showed direct linkages between maternal nutrition and epigenetic modifications of fetal muscle leading to impaired metabolism of nutrients and reduced growth and development of muscle in IUGR fetuses. Specifically, the total number of muscle fibers were less on day 90 and 100 of gestation in IUGR fetal piglets compared with normal birth weight piglets, and 37 proteins involved with energy and protein metabolism, structure and type of muscle fibers, nutrient transport, intracellular environment, and tissue integrity were differentially expressed between normal and IUGR piglets. Interestingly, although there were no differences in chemical composition of meat between IUGR and normal pigs, meat from IUGR pigs had greater pH, lower electrical conductivity and drip loss, was more tender, and had greater consumer sensory scores for taste, smell, and overall desirability (Matyba et al., 2021).
Genetic selection against IUGR appears possible without affecting current litter size levels if selection criteria includes both litter size and within-litter proportion of IUGR piglets in the breeding program (Matheson et al., 2018). Wang et al. (2017) suggested that targeting maternal nutrition during late gestation should reduce the incidence of IUGR. Because of the angiogenic effects of arginine, glutamine, and glutamate, it may be possible to increase placental weight, embryonic and fetal survival, growth, and development (Romanelli et al., 2019). However, our current understanding of the cellular and metabolic mechanisms of amino acid regulation of metabolic pathways, embryonic and fetal growth, and nutrient and oxygen flows through the placenta and fetuses is poor and requires additional research.
Several researchers have modeled dietary NUE in swine by estimating the amount of N retained in the body, N excreted in feces and urine, and N emissions from manure as a percentage of total dietary N (Millet et al., 2018). Results from these studies have shown low NUE (30 to 43%) based on the amount of N retained as a percentage of total dietary N consumed. These N-flow values are supported by data conducted using an animal metabolism crate manure storage system designed to simulate manure storage conditions in typical swine facilities (Trabue and Kerr, 2014; Kerr et al., 2018; Trabue et al., 2019a; Trabue et al., 2019b; Kerr et al., 2020; Trabue et al., 2021a; Trabue et al., 2021b; Trabue et al., 2022). In these studies, calculated mass balance of N did not, however, account for all of the consumed N, with these researchers speculating that the difference may be due to ammonia losses from metabolism crates and flooring surfaces during the fecal and urine collection process which has been shown to represent 19 to 25% of the N consumed (Burton and Beauchamp, 1986; van Kempen et al., 2003; Li et al., 2011; Costa, 2017).
In growing pigs, AA and N requirements for maintenance functions are determined by basal endogenous losses during digestion related to feed intake and diet composition; skin and hair losses; and minimal AA catabolism associated with basal turnover of body proteins, synthesis of essential nitrogenous compounds, and basal urinary urea excretion (Moughan, 1999). Body protein deposition up to maximal genetic potential occurs once AA needs for maintenance functions are met and adequate energy is available. Body protein deposition is determined by the difference between protein synthesis and degradation based on pig body weight, sex, and the use of various metabolic modifiers such as porcine somatotropin, immunocastration, and feed additives such as ractopamine. Whole body and lean growth rates, feed efficiency, dressing percentage, and retail meat yields of pigs have dramatically increased over the last several decades (Oksbjerg et al., 2000; Chen et al., 2002; Fix et al., 2010). Fix et al. (2010) concluded that the 45% improvement in lean efficiency, as measured by lean gain per unit of feed intake, was equally attributable to both genetics and feeding programs. Calculations to estimate efficiency of N utilization using the data reported by Fix et al. (2010) suggest a 20% improvement in N use for lean deposition (0.78 g carcass fat free lean per kg crude protein (CP) intake for 1980 pigs versus 0.93 kg carcass fat free lean per kg CP intake for 2005 pigs when all pigs consumed a more nutrient dense diet in 2005. However, feeding the more nutrient dense 2005 diet to the 1980 pig would have greatly exceeded their energy and nutrient requirements resulting in the 1980 pig being highly inefficient in dietary N utilization.
Nitrogen excretion and emissions are a function of both N intake and retention. Numerous studies have shown that reducing dietary CP concentrations and supplementing the diet with adequate amounts of crystalline AA results in a reduction in N excretion in manure due to a reduction in excess dietary N from AA and other nonprotein N sources. Kerr (2003) conducted a comprehensive review of studies published between 1967 and 1995 on the effects of feeding low CP-AA supplemented diets on N excretion in pigs ranging from 11 to 85 kg BW and concluded that for each one percentage reduction in dietary CP, N excretion was reduced by approximately 8%, with the reduction in N excretion being consistent across all body weights. This reduction in N concentration in manure also has an impact on aerial ammonia emissions (Latimier et al., 1993; Pfeiffer, 1993) which indicates a direct linkage between nutrient inputs into the animal and subsequent environmental impact. Additional studies conducted since the Kerr (2003) review consistently show that N excretion is reduced by 6 to 10% for each one percentage reduction in dietary CP (Canh et al., 1998c; Noblet et al., 2001; Shriver et al., 2003; Portejoie et al., 2004; Leek et al., 2005; Kerr et al., 2006; Leek et al., 2007; Lynch et al., 2007; Powers et al., 2007; Le et al., 2009; Jarret et al., 2011b; Osada et al., 2011; Li et al., 2015; Monteiro et al., 2017; Trabue et al., 2021a; Ball et al., 2022; Vonderohe et al., 2022), with concomitant reductions in ammonia emissions (Canh et al., 1998c; Hayes et al., 2004; Portejoie et al., 2004; Leek et al., 2005; Philippe et al., 2006; Leek et al., 2007; Lynch et al., 2007; Le et al., 2009; Li et al., 2015; Liu et al., 2017). As a result, replacing dietary CP concentrations with crystalline AA to meet estimated requirements can reduce N excretion up to 50% depending upon the magnitude of decreased dietary CP and the availability of crystalline AA.
Although a reduction in dietary CP concentrations can have a dramatic effect on reducing N excretion, increased dietary fiber concentrations have generally shown a minimal impact on total N excretion. However, feeding high fiber diets increases the partitioning of N excretion from urine to feces (Canh et al., 1997; Younes et al., 1997; Mroz et al., 2000; Zervas and Zijlstra, 2002a; Zervas and Zijlstra, 2002b; Jarret et al., 2011a; Jha and Leterme, 2012; Jha and Berrocoso, 2016), which has a positive effect on increasing the amount of N retained in the manure. In contrast to the increase in manure N concentrations resulting in increased N emissions observed in pigs fed high concentrations of dietary CP, manure-N emissions are generally reduced by feeding pigs elevated levels of dietary fiber (Canh et al., 1998a, Canh et al., 1998b; Mroz et al., 2000; Kerr et al., 2006; Le et al., 2008; Galassi et al., 2010; Jarret et al., 2011a; Trabue and Kerr, 2014; Philippe et al., 2015; Kerr et al., 2020; Trabue et al., 2022). The lower emissions of ammonia from manure from pigs fed high fiber diets is partially due to a shift in N excretion from the urea-N in the urine to the microbial-N in the feces (Canh et al., 1997; Zervas and Zijlstra, 2002a; Zervas and Zijlstra, 2002b), lower manure pH (Canh et al., 1998a; Canh et al., 1998b; van der Peet-Schwering et al., 1999; Kai et al., 2008; Ye et al., 2008; Trabue et al., 2022) and manure crusting (Trabue and Kerr, 2014; Kupper et al., 2020).
Despite the well-known beneficial impacts of feeding low CP and high dietary fiber diets on reducing manure N concentrations and subsequent N-based emissions, the impact of nutrient composition of swine diets on GHG emissions within the barn or manure storage system is less understood. In general, feeding pigs diets lower dietary CP has no effect on CO2, CH4, or N2O (Velthof et al., 2005; Le et al., 2009; Osada et al., 2011; Li et al., 2015; Trabue et al., 2021a; Trabue et al., 2021b), although there are some exceptions, but with contradictory results. Feeding pigs reduced CP diets has resulted in increased emissions of CO2 and CH4, but no effect on N2O (Clark et al., 2005); increased emissions of N2O, but no effect on CH4 (Kerr et al., 2006); no effect on CO2, decreased CH4, but increased N2O emissions (Philippe et al., 2006); and may result in manure with a reduced potential for CH4 production (Jarret et al., 2011a). In a similar and inconsistent manner, pigs fed diets containing increased dietary fiber have shown an increase (Velthof et al., 2005; Jarret et al., 2011a; Jarret et al., 2011b; Li et al., 2011) or no change (Kerr et al., 2006) in CH4 emissions; decreased (Kerr et al., 2006) or no effect (Li et al., 2011) on N2O emissions; and no effect on CO2 emissions (Li et al., 2011). Lastly, Trabue and Kerr (2014); Kerr et al. (2020), and Trabue et al. (2022) reported no effect of feeding high dietary fiber diets on CO2, CH4, or N2O emissions. Therefore, within the production facility, diet composition differences have dramatic effects on N excretion and NH3 emissions, but limited and inconsistent effects on GHG emissions. Overall, results from several studies clearly show that by reducing dietary CP levels while meeting dietary AA requirements for whole body protein needs, the proportion of dietary N excreted in manure or being emitted from manure is decreased, and the proportion of dietary N retained in the body is increased, thereby improving efficiency of dietary N utilization.
Life Cycle Assessment (LCA) of environmental impacts of swine diets, feeding programs, pig farms, and pork supply chains is an emerging approach to reduce the carbon, N, and environmental footprint of pork production (Shurson et al., 2022; Shurson and Urriola, 2022). This can be accomplished by using multi-objective feed formulation that includes LCA data for ingredients, based on geographic origin and calculated using standardized methods (LEAP, 2015), as constraints when formulating low environmental impact diets used in precision pig feeding programs (Mackenzie et al., 2016; Garcia-Launay et al., 2018; de Quelen et al., 2021). Environmental impact data of feed ingredients are determined based on types and amounts of production inputs and processes, which are indirectly linked to nutritional composition and digestibility of ingredients that affect carbon, N, and phosphorus utilization efficiency and emissions. Monteiro et al. (2021) used a modeling approach to determine correlations between pig production traits and LCA impacts for 1 kg of body weight gain at the farm gate. Results from this study showed that N excretion was positively correlated with feed conversion ratio (r = 0.96), and several LCA measures including climate change (r = 0.96), acidification potential (r = 0.97), eutrophication potential (r = 0.97), and land occupation (r = 0.96) regardless of type of feeding program used.
Measurement of NUE should involve multiple criteria at multiple scales beginning with whole body N efficiency or SID Lys per kg lean meat at the metabolic level of individual pigs. Although it may not be intuitively apparent, the high mortality and reduced lean growth rate and efficiency resulting from multiple systemic developmental deficiencies of low-birth-weight pigs caused by IUGR are significant contributors to suboptimal NUE on commercial swine farms. Mass balance of N intake, retention, excretion, and emissions are also important measures of NUE that can be applied at the individual pig, group, farm, and supply chain levels. Reducing dietary CP concentrations while providing adequate supplementation of crystalline AA is an effective approach for reducing N excretion, and the benefits of increasing dietary fiber on increasing N retention in manure to minimize emissions are important contributors to NUE. The high correlation of N excretion with several LCA measures indicates that multiobjective feed formulation can be useful for reducing N footprint at the pig farm level.
Pig genotype is an important component that affects nutrient utilization efficiency. Kyriazakis (2011) evaluated the potential for including 1) feed wastage associated with feeding behavior traits, 2) digestion efficiency, 3) maintenance requirements, 4) net efficiency of energy and nutrient utilization, and 5) partitioning of limiting nutrient within productive and between productive and immune functions as selection criteria in swine breeding programs to improve nutrient utilization efficiency. Of these factors, only improving digestion efficiency and reducing maintenance requirements provide some opportunity for increasing nutritional efficiency. Although there appears to be significant genetic variation in feeding behavior among pig genotypes, the opportunity to reduce nutrient losses from feed wastage associated with this trait appears to be minimal. There also appears to be minimal variation in net efficiency of nutrient utilization among pig genotypes, and no evidence of measurable variation in nutrient partitioning within and between productive and immune functions. While there appears to be limited variation in nutrient utilization efficiency associated with genetics, genetic selection has resulted in a tremendous increase in lean deposition rates. When increased lean deposition is combined with slaughtering leaner pigs at heavier weights, the proportion of nutrients retained in consumer-edible lean tissue is increased, which increases the efficiency of dietary nutrients when expressed on an edible lean pork product basis.
As previously described, Monteiro et al. (2021) used a modeling approach to show that the use of feed conversion as selection criteria in pig breeding programs is a promising holistic approach for improving nutrient utilization efficiency and reducing the environmental impact of pig production. However, an increased gain-to-feed ratio is not necessarily equivalent to an improvement in feed efficiency (Crews, 2005) because different sources of variability in feed intake must be considered, including nutrient requirements for maintenance and growth. As a result, residual feed intake, defined as the difference between observed average daily feed intake (ADFI) and the ADFI predicted from production and maintenance requirements of pigs has been proposed to potentially improve indices of nutrient utilization efficiency. Saintilan et al. (2013) evaluated the use of residual feed intake as a selection criterion to improve feed efficiency and potential to reduce N and P excretion in four pig genotypes. Heritability estimates for N and P excretion traits were low to moderate, ranging from 0.29 to 0.40, with greater correlations between feed conversion ratio and N and P excretion traits in sire breeds (0.57 to 0.86) than dam breeds (0.38 to 0.53), indicating that residual feed intake could be used successfully in genetic selection programs to reduce N and P excretion. Shirali et al. (2013) also identified quantitative trait loci for feed efficiency measured by residual energy intake and its association with production, feed conversion ratio, and N excretion traits at different stages of growth of a commercial genotype. They reported that regulation of feed efficiency is partially independent of production traits, where changes in efficiency of feed utilization are due to underlying contributors to residual energy intake including metabolism, digestion, and protein turnover, and different genes were responsible for feed utilization efficiency at different stages of growth. Despite considerable interest in residual feed intake as a selection criterion in swine breeding programs, it has not been widely adopted.
Lean tissue growth rates for intact males (boars) are up to 30% greater compared with physical castrates (barrows) and immature females (gilts), which results in a greater proportion of N retained in the carcass of the N consumed, and thereby improves NUE caused by reduced demand of AA needed for maintenance (Knudson et al., 1985; Campbell and Taverner, 1988; Hansen and Lewis, 1993; Quiniou and Noblet, 1995; Xue et al., 1997; van Lunen and Cole, 1998). While rates of lean tissue deposition are greater in boars compared with gilts or barrows, little is known about differences in marginal efficiency of AA utilization. However, the percentage of protein in the muscle, and muscle fiber diameter are similar between boars and barrows (Knudson et al., 1985) with some data (Hansen and Lewis, 1993) suggesting that efficiency of protein (Lys) intake used for growth may be greater for boars compared with barrows or gilts. This observation is supported by Moehn et al. (2004) who observed that the rate of Lys catabolism decreases with increasing growth potential, thereby suggesting that boars have a higher marginal efficiency of Lys utilization compared to barrows. However, the presence of boar taint in pork products is considered unacceptable among most consumers (Xue and Dial, 1997).
Boar taint is caused by androstenone, which is produced in the testes and is a pheromone that serves as a sexual attractant to sows, along with skatole (3-methylindole), and other indoles which are produced in the intestine from microbial degradation of tryptophan (Claus et al., 1994) and found in intact male pigs at increased amounts upon reaching puberty (Babol and Squires, 1995). These compounds accumulate in adipose tissue and their concentrations are affected by age at sexual maturity, BW, genotype, and nutrition (Squires et al., 1993; Xue and Dial, 1997; Dunshea et al., 2001; Aldal et al., 2005; Squires et al., 2020). These compounds are markedly reduced in adipose tissue of physically castrated pigs (Bonneau, 1982; Wesoly and Weiler, 2012). Short-term strategies to control boar taint have been ineffective due to high genetic variation among individuals and populations. Although selective breeding, genome editing, and metabolic profiling may be effective approaches for managing this problem, they are long-term potential solutions (Squires et al., 2020). Therefore, the use of physical castration or immunological castration to prevent boar taint and provide similar pork quality characteristics are the only current solutions (Elsbernd et al., 2016).
Barrows have greater feed intake and growth rate, and reduced gain efficiency and carcass lean percentage than gilts (Ekstrom, 1991). As a result, gilts require greater concentrations of dietary AA to maximize rate and efficiency of gain than barrows (Cromwell et al., 1993). Furthermore, the dietary AA concentrations needed to maximize feed efficiency and carcass lean percentage are greater than those required for maximum growth rate (Cromwell et al., 1993). As a result of differences in AA requirements and feed intake between barrows and gilts, penning barrows and gilts separately and feeding diets that more closely match their respective nutrient requirements can reduce feed cost, increase revenue from improved carcass lean, and improve NUE of the swine production system. In addition, penning barrows and gilts separately may improve growth performance of barrows due to overcoming behavior differences associated with social rank and aggression between barrows and gilts in mixed sex pens (Mikesell and Kephart, 1999). However, economic, growth performance, carcass composition, and nutritional efficiency advantages of split-sex feeding apply only to the growing-finishing period (35 to 105 kg) because there are minimal if any differences in growth rate, feed intake, gain efficiency, and lysine requirements of weaned pigs between 19 to 54 days of age (Hill et al., 2007). Therefore, in contrast to the limited potential for using genetic selection to improve marginal AA efficiency, there is significant opportunity for improving NUE by using separate sex feeding programs. Unfortunately, split-sex feeding is underutilized in commercial pig productions systems around the world, but could be widely implemented to obtain economic and NUE advantages compared with mixed sex feeding systems.
As previously discussed, pigs marketed at increasing BW have increased rates of lean tissue deposition per day, and greater deposition relative to rates of deposition of other non-skeletal muscle tissues (Strathe et al., 2009; Danfær and Strathe, 2012), but may undergo decreasing marginal efficiencies (at least for Lys). Diets for growing pigs tend to be greater in energy density and digestible AA concentrations than gestation and lactation diets to meet nutritional needs. Feed efficiency (kg body weight gain/kg feed) is a crude measure for N utilization but is greatest in early growth (1.0 to 2.0 kg feed/kg body weight gain) and declines to 3 to 4 kg feed/kg body weight gain in the late finishing phase depending on genotype, sex, and market weight (Villamar and Sardá, 2019). Therefore, increasing market weight of pigs generally reduces NUE.
The endocrine system is an important component in the complex process of partitioning energy and nutrients between muscles and adipose tissue during animal growth and provides a viable opportunity to improve nutrient utilization efficiency.
Administration of pST (growth hormone) to growing pigs has been shown to consistently increase growth rate 10 to 20%, decrease feed intake 10 to 15%, increase gain efficiency 15 to 30%, decrease lipid accretion 20 to 80%, and increase protein deposition 20 to 50% (Etherton and Smith, 1991; Mikel et al., 1993; Etherton, 2000; Oliver et al., 2003). While boars do respond to somatotropin treatment, growth performance responses to somatotropin appear to be greater in gilts and barrows compared with boars (Krick et al., 1992; McCauley et al., 2003). This growth improvement is due to altering the partitioning of absorbed energy and nutrients rather than improving nutrient digestibility or changing muscle fiber type (Beerman et al., 1990; Etherton and Smith, 1991; Krick et al., 1992; Mikel et al., 1993). The decrease in adipose tissue from pST administration is largely due to a reduction in lipogenesis (Etherton, 2000), while the increase in protein deposition is mainly due to a decrease in protein degradation and AA catabolism (Vann et al., 2000). Unfortunately, despite the potential for improving NUE in growing pigs, this technology has not been adopted for several reasons including negative consumer attitudes toward hormones used in food production, delivery system challenges (Foster, 1999), regulatory approval, and the increased level of management required.
As previously discussed, intact male pigs have greater growth rate, feed efficiency, and carcass lean than physically castrated male pigs, but pork derived from boar may contain “boar taint” which is an undesirable off-flavor sensory characteristic that many consumers find objectionable (Meier-Dinkel et al., 2013). However, the practice of surgical castration of male pigs to prevent boar taint in pig meat has been considered undesirable, especially without the use of anesthesia, from an animal welfare (McGlone and Hellman, 1988; McGlone et al., 1993) and from a consumer perspective (Aaslyng et al., 2016). As a result, pig producers in some countries such as the United Kingdom and Ireland have not been allowed to physically castrate male pigs since 2003 (Rault et al., 2011). Consequently, alternatives to physical castration have been explored, such as genetic selection to reduce boar taint (Grindflek et al., 2011), using sexed semen to produce only females (Roca et al., 2011), slaughtering male pigs at lower body weights (Dunshea et al., 2001), and feeding diets containing indigestible fiber and improving facility sanitation (Wesoly and Weiler, 2012). To date, however, all of these approaches require overcoming significant barriers before they can be effectively implemented. Therefore, there has been tremendous interest, and implementation in some countries, in the use of GnRF in commercial pig production systems to capture the advantages of greater growth performance and carcass lean deposition of intact males (Dunshea et al., 2013; Asmus et al., 2014), while avoiding the negative effects of surgical castration on pig welfare and undesirable boar taint in pork products which are important for consumer acceptance (Rickard-Bell et al., 2009). The challenges and opportunities of using immunocastration for sustainable pork production in Europe were reviewed by Kress et al. (2019), who suggested that while the balance between the environmental and economic benefits of raising boars, and the reduced welfare concerns and the risk of boar taint in pork is attractive, effective communication of the synergistic and conflicting relationships between the pillars of sustainability of using this technology throughout the supply chain is needed for widespread acceptance.
The impacts of using immunocastration in male pigs has been extensively studied and a meta-analysis of numerous published studies has been conducted to quantitatively summarize effects on growth, carcass, meat quality, reproductive organs, and boar taint compounds (Batorek et al., 2012). Entire males and immunocastrates have greater growth rate, feed efficiency, and similar N retention compared with physical castrates and gilts (Elsbernd et al., 2015). Male pigs immunologically castrated with GnRF have less carcass fat and improved lean gain efficiency than physically castrated pigs (Pauly et al., 2009). Immunologically castrated pigs can be slaughtered between 3 and 10 weeks after the second GnRF dose in administered, but increasing this interval results in increases carcass backfat and belly thickness, while reducing carcass lean percentage (Lealiifano et al., 2011; Harris et al., 2017; Harris et al., 2018). Significant environmental benefits resulting from the magnitude of improvement in growth performance and carcass lean achieved by using immunocastration have been estimated to be 3.8% less (carcass weight basis) than physical castrates (de Moraes et al., 2013). In addition, the improvement in feed efficiency also results in reduced manure production and a reduction of land use devoted to crop production of 31 m2 per pig for immunocastrates compared with physical castrates.
The use of GnRF in gilts has been shown to improve feed intake and weight gain by pausing estrus activity. Oliver et al. (2003) showed that the effects of sex, pST, and GnRF on growth performance are additive in group housed boars and gilts. Lean tissue deposition rate of gilts treated with GnRF was greater than untreated gilts, but less than those treated with pST, and the combination of GnRF and pST. Compared with gilts, intact males had greater lean deposition rates, but only the combination treatments improved lean deposition rate while individual treatments had no effect.
Short-term opportunities for non-dietary interventions to improve NUE appear to be limited to split sex feeding, reducing market weight, and the use of GnRF for intact males and gilts in countries where government regulations allow its use. The potential for additional long-term improvement in NUE may be possible if residual feed intake is widely incorporated into swine genetic selection programs. However, the tradeoffs between improved animal welfare by avoiding physical castration with consumer concerns regarding food safety associated with use of GnRF, along with the risk of broken needles from product administration, and the need for a higher level of management at the farm level will determine long-term use of immunocastration and contributions toward improving NUE.
Perhaps the greatest opportunity to improve NUE in pig production systems is by using state-of-the-art diet formulation practices and strategic use of various types of feed additives such as ractopamine, antibiotics, L-carnitine, conjugated linoleic acid, and feed enzymes.
Although reducing dietary CP concentrations by five percentage units in combination with AA supplementation can reduce N excretion up to 50%, achieving this magnitude of NUE requires understanding the benefits and limitations of using different methodologies to determine digestibility of AA in feed ingredients (Kong and Adeola, 2014; Stein, 2017; Zhang and Adeola, 2017). Accurate estimates of SID of AA are dependent on accurate estimates of basal endogenous losses. Bloxham et al. (2022) compared total AA content and SID values of ingredients in two databases and reported that SID values were primarily affected by the total AA concentrations in feed ingredients, which may have resulted from inadequate methods to estimate basal endogenous losses of AA and statistical analysis approaches used. Park et al. (2013) developed prediction equations to estimate basal endogenous losses of protein and AA in pigs fed N-free diets using experimental data from several published studies and reported that initial pig BW and the ratio of feed intake per maintenance feed intake were two main factors that reduced endogenous N losses.
Efficiency of dietary N utilization is also affected by AA imbalance, excesses relative to requirements, inadequate energy intake, digestibility of feedstuffs, fiber concentration and type, phytate content, and non-protein N concentrations (NRC, 2012). Furthermore, not all digested and absorbed AA are biologically available and vary among protein sources. Although AA bioavailability provides a more accurate assessment of their utilization than digestibility, bioavailability estimates are variable, are difficult and costly to obtain, and may not be additive in mixtures of feed ingredients (NRC, 2012). There are instances when absorbed AA may not be completely bioavailable because derivatives of heat damaged Lys can be absorbed but not utilized, and infrequent feeding can cause rapid absorption but incomplete utilization of crystalline AA (NRC, 2012).
Historically, evaluation systems used to determine nutritional value of feed ingredients have been based on dietary inclusion rates determined by constraints of nutrient concentrations and digestibility, but have not accounted for differences in the rate, extent, and timing of nutrient digestion and fermentation in the gastrointestinal tract (Heyer et al., 2022). Therefore, an emerging approach for evaluating the nutritional value of feed ingredients involves measuring the digestion kinetics of dietary starch, fiber, and protein in pigs (Gerrits et al., 2021). Chen et al. (2019) showed substantial differences in in vitro digestion kinetics among protein sources based on N solubilization and the release of free AA and low molecular weight peptides. As a result, these researchers suggested that the kinetics of release of low molecular weight peptides may be useful in predicting in vivo protein digestion kinetics in pigs. Ye et al. (2022) determined protein digestion kinetics in sows fed diets containing low, medium, and highly degradable protein and observed no effect on litter weight gain, milk composition, and N excretion due to different protein digestion kinetics but showed that feeding protein sources with a high proportion of slowly degradable protein reduced body weight loss and protein mobilization of maternal body tissue during lactation. As more is learned about nutrient digestion kinetics in various feed ingredients used in swine diets, more strategic diet formulation strategies will become possible for improving nutritional efficiency, minimizing the incidence of diarrhea in weaned pigs, and reducing the amount of nutrients excreted in manure.
An imbalance of dietary AA relative to pig requirements reduces NUE and can lead to AA deficiency, toxicity, and antagonism (Harper et al., 1970; D'Mello, 2003). Amino acid deficiencies can reduce feed intake, growth rate, and contribute to feed wastage (NRC, 2012). Protein and AA toxicity rarely occurs but can lead to diarrhea in young pigs, and toxic levels of some AA can reduce feed intake, growth rate, and cause abnormal behavior (NRC, 2012). Antagonistic effects among certain types of AA involves an excess of one AA interfering with the utilization of another structurally of chemically related AA which inhibits their use in protein synthesis and increases their requirement (NRC, 2012). Antagonism among neutral and branched chain AA (leucine, isoleucine, and valine) is commonly observed in swine diets containing high amounts of corn protein (Salyer et al., 2013; Gonçalves et al., 2015; Cemin et al., 2019; Kwon et al., 2019; Kwon et al., 2020, Kwon et al., 2021, Kwon et al., 2022).
The concept of ideal protein AA profiles originated from the observation that the AA composition of high-quality dietary protein was similar to the AA composition of animal tissues (ARC, 1981; van Milgen and Dourmad, 2015). This led to the approach of expressing AA requirements based on an ideal AA profile that provides an optimal balance of AA for maintenance and productive functions for growth, reproduction, or lactation (NRC, 2012). Therefore, achieving optimal dietary NUE requires the use of the ideal protein AA profile estimates to ensure an optimal AA balance when formulating swine diets.
The benefits of feeding low CP-AA supplemented diets on N excretion in manure were previously described in Section 2.4. However, growth performance and carcass composition responses must be maintained. Results from several studies have shown that dietary CP can be reduced by up to 4 percentage units, if adequate amounts of crystalline AA are supplemented to meet estimated requirements and reduce N excretion and emissions in manure (Cappelaere et al., 2021) without compromising growth performance (Kerr et al., 2003a; Kerr et al., 2003b; Nyachoti et al., 2006; Yue and Qiao, 2008). However, inconsistent results have been reported in other studies regarding the effects of reducing dietary CP level by more than 3 percentage units on growth performance of pigs (Wang et al., 2018). Reduced growth performance in pigs fed low CP diets observed in some studies has been attributed to a lack of intact protein and hydrolyzed peptides which are associated with maintaining N retention and whole-body homeostasis (Guay et al., 2006), improving activities of digestive enzymes (Shimizu, 2004), and reducing excessive oxidation of AA (Yen et al., 2004). In addition, depending on the frequency of meals, studies have shown that digestion and absorption of AA occurs more rapidly in diets containing high amounts of crystalline AA than in diets comprised primarily of intact protein sources such as soybean meal (Yen et al., 2004). Amino acid utilization efficiency may be different in low CP diets if the dietary ideal protein AA profiles do not match the changing requirements of pigs during each growth stage (van Milgen and Dourmad, 2015).
Ractopamine is a β-agonist that is added to swine feed in the U.S. to increase growth rate and efficiency (Puls et al., 2014) by increasing muscle protein synthesis (Ulrey et al., 2013) and reducing lipid content (Moore et al., 2009) without affecting pork meat quality (Elsbernd et al., 2015). Results from a meta-analysis conducted by Apple et al. (2007) supported the conclusion that ractopamine can be used to improve body weight gain, feed efficiency, and carcass leanness when added to diets containing a minimum of 16% CP and fed to pigs with minimum body weight of 68 kg during the last 20 to 40 kg of body weight gain before slaughter (Bohrer et al., 2013). Feeding 10 mg/kg diet of ractopamine has been shown to increase whole body protein deposition, and skeletal muscle protein deposition relative to whole body protein is greater (81%) compared than in pigs fed diets without ractopamine (54%; Schinckel et al., 2003; Webster et al., 2007). This improvement in skeletal muscle protein deposition results in a 1.08 percentage unit improvement in boneless meat yield compared with pigs fed control diets (Bohrer et al., 2013). Studies evaluating the use of ractopamine in diets for intact male pigs and immunological castrates have also shown improvements in growth performance and carcass lean deposition (Moore et al., 2009; Lowe et al., 2014; Puls et al., 2014; Costa et al., 2018).
Despite the multiple benefits of using ractopamine in finishing pig diets, including improving NUE, ractopamine is one of the most controversial feed additives in the world (Abbas et al., 2022). Ractopamine is not allowed to be used in EU pork production, and pork produced using ractopamine is not allowed to be imported into the European Union because the European Food Safety Authority determined that there is insufficient safety data and may be detrimental to animal well-being (Balcombe et al., 2021). In contrast, in the U.S. and several other countries, ractopamine is approved for use as a livestock feed additive to increase growth rate and muscle mass while reducing feed intake, carcass fat deposition, and N excretion in manure, and that meat products from animals fed ractopamine are safe for human consumption (Abbas et al., 2022). However, because the U.S. is a major exporter of pork to countries such as China, Russia, and Taiwan which have banned the importation of pork produced using ractopamine, many U.S. meat packers have stopped accepting pigs that were fed ractopamine to maintain the ability to export pork (Abbas et al., 2022). As a result, there is limited use of ractopamine in finishing pig diets in the U.S. and the future use of this technology is uncertain but dependent on scientific, medical, and toxicology evidence determined by standardized analytical methodology, and applying science to trade policies for imports and exports of meat products produced with ractopamine (Abbas et al., 2022).
Antibiotics have been used at therapeutic levels to prevent, control, and treat disease, and for growth promotion when fed at subtherapeutic levels in swine diets for many years. Several of these antibiotics are also used in human medicine and their widespread use in animal production has been attributed to the development of antimicrobial resistant bacteria which has created significant global public health concerns (Van Boeckel et al., 2015). As a result, the European Union has banned the use of antibiotics for growth promotion of farm animals while other countries such as the United States, Canada, and Australia have implemented regulations that restrict antibiotic use by requiring prescriptions from licensed veterinarians (Lekagul et al., 2019). However, other major pig production countries including China and Brazil have no restrictions on using antibiotics for growth promotion (Maron et al., 2013). Despite the contributions of subtherapeutic use of antimicrobials to the development of antimicrobial resistance and the serious global consequences on human and animal health, Van Boeckel et al. (2015) projected a 67% increase in antimicrobial use in animal production by 2030 due to increasing consumer demand for animal derived food products in middle income countries and a shift toward more intensive, large-scale farms where antimicrobials have historically been routinely used.
Numerous studies have shown that feeding growth promoting antibiotics improve BW gain and feed efficiency in starter, grower, and finisher pigs, with the greatest responses occurring at young age and under disease challenge conditions (Gaskins et al., 2002; Lekagul et al., 2019). Several physiological, nutritional, and metabolic effects have been reported and attributed to the mechanism of action of the growth performance improvements, including a 3.0% increase in apparent N digestibility, 5.8% increased N retention, and a 10% reduction in N excretion relative to N retained when tylosin was fed to growing pigs (Gaskins et al., 2002). Similarly, Han et al. (2001) reported that N excretion may be reduced by 5.1 to 18.8% when 11 to 23 kg pigs are fed selected antibiotics as growth promotants. Pi et al. (2019) fed a diet containing a mixture of ampicillin, gentamycin, and metronidazole to growing pigs for 2 weeks to evaluate NUE and AA digestibility and observed improved apparent ileal digestibility of CP and total AA, alterations in the microbiome and N metabolism in the intestinal tract.
Yu et al. (2017) fed creep feed without or with a mixture of antibiotics (olaquindox, oxytetracycline calcium, and kitasamycin) to litters of pigs from 7 to 42 days of age (early antibiotic intervention), and then subsequently fed normal or low CP diets without or with the antibiotic mixture from 42 to 185 days of age on NUE. They observed an increase in growth rate of pigs fed the low CP diets with antibiotics from day 42 to 185, which was not observed when feeding normal CP diets. Early antibiotic intervention increased CP digestibility, and altered blood parameters, fecal microbial fermentation profile, and glucose and putrescine concentrations. However, Kiarie et al. (2018) fed a corn-soybean meal diet containing antibiotics (chlortetracycline hydrochloride and tiamulin) or benzoic acid and observed an improvement in apparent total tract digestibility of CP from benzoic acid but not antibiotics. Puiman et al. (2013) provided antibiotics intravenously to neonatal pigs suggested that changes in AA and N metabolism are caused by changes in the microbiome in the gastrointestinal tract and liver and have limited impact of whole-body growth on neonatal pigs.
Unfortunately, limited research has been conducted to determine the effects of antibiotics or antibiotic-like compounds on N emissions. A few previous studies showed that in-feed antibiotics improve N digestibility and retention (Roth and Kirchgessner, 1993; Gaskins et al., 2002), but this effect is not always consistent (Kerr et al., 2022). A recent study by Ni et al. (2019) reported no effect of feeding diets containing antibiotics compared to pigs fed no antibiotics on ammonia emissions in wean-to-finish pigs. Because of antimicrobial resistance concerns, however, continued use of growth promoting antibiotics in animal feeds is not an acceptable strategy for improving nutrient utilization and sustainability of food animal production.
Carnitine is a naturally occurring compound, found in relatively high concentrations in animal by-products but in low concentrations in plant-based feed ingredients (Owen et al., 1997). Its primary function is to facilitate the transport of long chain fatty acids into the mitochondria for energy production (Fritz and Yue, 1963). Because of its role in energy metabolism and utilization, numerous studies have evaluated the use of L-carnitine as a feed additive to improve reproductive, lactation, and growth performance of pigs (Eder, 2009; Ringseis et al., 2018). Limited studies have shown that supplementation of L-carnitine in gestating sow diets is effective for improving reproductive performance in grain-soybean meal-based diets, no effect of oral supplementation of L-carnitine or addition to milk replacer has been observed for suckling piglets, but inconsistent growth performance responses have been observed in weaned pigs (Ringseis et al., 2018). A few studies have reported improvements in growth performance from dietary L-carnitine supplementation have been reported (Owen et al., 1996; Heo et al., 2000; Rinker et al., 2003; Birkenfeld et al., 2005), but most studies have shown no beneficial effects on growth performance (Hoffman et al., 1993; Cho et al., 1999; Owen et al., 2001a; Owen et al., 2001b; Han and Thacker, 2006; Pietruszka et al., 2009; Keller et al., 2011). Reasons for the inconsistent growth performance and carcass composition responses of pigs post-weaning and during the grower-finisher period are unclear, but differences in dietary concentrations of micronutrients that serve as co-factors for enzymes involved in L-carnitine biosynthesis may be contributing factors (Ringseis et al., 2018). Keller et al. (2011) provided evidence that carnitine may increase muscle mass by stimulating the anabolic IGF-1 pathway and suppressing the pro-apoptotic and atrophy-related genes associated with apoptosis of muscle fibers and proteolysis of muscle proteins. As a result, studies have shown improvements in protein accretion rate, N retention, and reduced urinary N excretion (Heo et al., 2000), and increases in carcass lean and muscle (Owen et al., 2001a; Owen et al., 2001b) when L-carnitine was fed to growing-finishing pigs. Results from these studies suggest that adding L-carnitine to growing pig diets increases utilization of fat for energy and spares AA for protein synthesis but warrant more investigation.
Conjugated linoleic acid (CLA) is produced from positional and geometric isomers of linoleic acid, which is an essential omega-6 fatty acid (Schmid et al., 2006). Several mechanisms of dietary CLA responses have been identified including a reduction in body fat deposition and increased synthesis of lean tissue in mice (Park et al., 1997; Park et al., 1999), increased energy expenditure, regulation of adipocyte metabolism, adipokines and cytokines, increased β-oxidation in skeletal muscle (Park and Pariza, 2007), and reduced catabolic effects of immune function in muscle (Pariza et al., 2000). Müller et al. (2001) showed that feeding isoenergetic diets containing CLA to growing pigs resulted in a positive energy balance with no appreciable effect on overall metabolism except for a slight improvement in protein deposition. Results from several studies have shown that addition of CLA to growing pig diets improve growth performance and carcass lean (Dugan et al., 1997; Ostrowska et al., 1999; Dugan et al., 2001; Thiel-Cooper et al., 2001; Wiegand et al., 2001; Weber et al., 2006), and increase CLA concentrations in meat (Wiegand et al., 2002; Lauridsen et al., 2005; Schmid et al., 2006), which have been shown to provide several human nutrition and health benefits (Roche et al., 2001). However, other studies have shown no effects on growth rate or feed conversion when CLA was added to weaned pig diets (Weber et al., 2001), when added at high concentrations to growing-finishing diets (Morel et al., 2008; Tous et al., 2013; Ivanovis et al., 2015), or when added to low protein grower-finisher diets (Martínez-Aispuro et al., 2014). Differences in responses among studies may be due to several factors including age and body weight of pigs, sex, genotype, dietary CLA concentration, type and proportion of CLA isomers fed, and feeding duration.
Several studies have shown that feeding diets containing CLA to growing-finishing pigs can provide comparable or improved growth performance and meat quality responses (Weber et al., 2006; Pompeu et al., 2013; Pinelli-Saavedra et al., 2019) compared to feeding ractopamine, which as previously described, has been restricted or banned from use in several countries. Additional studies have shown that diets supplemented with betaine in combination with CLA improve N utilization (Fernández-Fígares et al., 2012), increased growth rate and carcass protein deposition (Fernández-Fígares et al., 2008), and increased yield of lean cuts (Rojas-Cano et al., 2011) in obese Iberian pigs. Synergistic effects of feeding diets containing betaine and CLA have also been reported for reducing CO2, NH3, and NO2 emissions from pigs under commercial conditions (Lachica et al., 2019). Unfortunately, cost of CLA and need for regulatory approval in various countries has prevented its use in swine diets.
Phytate has a significant effect on protein utilization in monogastric by affecting the solubility of dietary protein, activity of endogenous enzymes, and endogenous protein flow (Selle et al., 2012). Cowieson et al. (2009) suggested that phytate crosslinks with proteins in the stomach resulting in an increased secretion of pepsin, hydrochloric acid, and mucin, which subsequently cause a cascade effect involving sodium bicarbonate, and endogenous AA secretion and recovery of dietary and endogenous proteins in the small intestine. Phytate has been shown to increase endogenous protein flow that affects its digestion and availability of protein as a substrate for fermentation in the lower portion of the gastrointestinal tract (Cowieson et al., 2004; Cowieson and Ravindran, 2007; Onyango et al., 2009). As a result of these significant phytate-protein interactions, Cowieson et al. (2016) suggested that the ratio of phytate to protein in feed ingredients should be considered when formulating swine and poultry diets.
Several studies have shown improvements in growth performance of pigs when phytase was added to diets considered nutritionally adequate in P, which has led to the possibility that phytase improves protein and AA digestibility in addition to its effects on improving P utilization (Selle and Ravindran, 2008). Cowieson et al. (2017) conducted a systematic review and meta-analysis of amino acid digestibility responses from feeding microbial phytase to pigs from 6 to 70 kg in body weight, and reported an overall improvement of 2.8% on apparent ileal AA digestibility coefficients, with a range from +0.9% for methionine and +4.1% for proline. However, because of these small and variable improvements in AA digestibility from microbial phytase use, it is imprudent to make feed formulation adjustments to account for the release of AA.
Exogenous carbohydrase and protease enzymes are commonly added to swine diets but their effects for improving protein and AA digestibility are inconsistent depending on the enzyme and composition of ingredients (Rojas and Stein, 2017). Thermal stability of exogenous enzymes is essential for achieving the desired response. Kerr et al. (2013) evaluated the addition of several commercially available enzyme products containing various carbohydrases (endo-1, 4-β-xylanase, hemicellulose, xylanase, β-glucanase, endo-1, 3(4)-β-glucanase), a protease, or phytase in corn-based starter and finisher swine diets containing 30% corn dried distiller’s grain solubles (DDGS) on growth performance and nutrient digestibility. Interestingly, several of these enzyme products resulted in small reductions in N digestibility but had no effect of growth performance of pigs. In contrast, improvements in CP digestibility have been observed when β-glucanases were added to barley-soybean meal diets but not for wheat-, corn-, or rye-soybean meal diets (Li et al., 1996). Addition of β-mannanase to a corn-soybean meal-based diets improved feed efficiency of pigs less than 15 kg BW but had no effect on N digestibility in 96 kg barrows (Pettey et al., 2002). Pig growth performance responses have also varied when various carbohydrases (e.g., β-glucanase, α-galactosidase, galactomannanase, β-mannanase, xylanase) have been added to diets containing corn DDGS ranging from no effects (Jacela et al., 2010; Jones et al., 2010) to improvements (Yoon et al., 2010). However, Jang et al. (2021) conducted a meta-analysis of data from 34 studies on growth performance responses from various types of enzymes and reported that although feeding diets containing corn DDGS slightly reduced growth performance of growing pigs, the addition of exogenous enzymes to these diets resulted in slight improvements (approximately 2%) in growth rate and gain efficiency in corn DDGS diets and in corn-soybean meal diets compared with unsupplemented diets.
No improvements in feed conversion (Rooke et al., 1998) or protein and AA digestibility (Caine et al., 1997) were observed when feeding diets containing soybean meal pre-treated with a protease enzyme compared with untreated soybean meal. Adding a cellulase enzyme to sorghum-soybean meal diets had no effect on N digestibility (Kim et al., 1998; Park et al., 2003), but adding a mixture of α-galactosidase, β-mannanase, and β-mannosidase to corn-soybean meal diets improved AA digestibility (Kim et al., 2003). Similarly, addition of a mixture of enzymes (cellulase, galactanase, mannanase, and pectidase) improved protein digestibility in diets containing corn, soybean meal, canola meal, barley, peas, wheat and wheat by-products (Omogbenigun et al., 2004). Although it is common practice to use mixtures of enzymes (i.e., carbohydrases, proteases, phytase) in swine diets, antagonistic, synergistic, additive effects can occur (Cowieson and Bedford, 2009; Cowieson, 2010).
Feeding a diet containing high concentrations of n-3 polyunsaturated fatty acids from linseed and walnut meal to growing pigs increased N retention by more than 8%, biological value of protein by 14.8%, and reduced the amount of N excreted resulting in greater than 40% of N retained (Hăbeanu et al., 2019). In general, any feed additive that improves growth performance and AA digestibility can contribute to greater NUE of swine diets.
Improvement in NUE through dietary interventions is only possible by using accurate estimates of SID of AA and ideal protein amino acid profiles in precision feed formulation, and characterizing feed ingredients based on their CP, fiber, and starch digestion kinetics may provide further improvements in NUE in the future. Despite their effectiveness for improving NUE, ractopamine and antibiotics are declining in their use because increased regulations associated with human health concerns. Strategic use of other types of feed additives such as L-carnitine, CLA, and exogenous enzymes may provide improvements in NUE if the conditions for optimizing their effectiveness are identified to produce more consistent responses.
Precision feed formulation and feeding programs are essential and are beginning to be implemented on commercial swine farms to optimize nutritional efficiency and productivity while minimizing the environmental footprint in pig production systems (Andretta et al., 2018; Gaillard et al., 2020; Pomar et al., 2021). Precision feeding is not possible without dynamic and accurate estimates of net energy and nutrient digestibility and bioavailability data in specific batches of each feed ingredient used to formulated diets fed to pigs (Shurson et al., 2021). Precision feeding of pigs (Pomar and Remus, 2019) has been shown to reduce feed costs by more than 8%, N and P excretion by about 40% (Andretta et al., 2014), and GHG emissions by 6% (Andretta et al., 2018). The challenges of precision feed formulation have also been described by de Lange et al. (2012): “The use of dietary amino acids for protein deposition involve digestion, absorption, and post-absorptive metabolism; biological processes which are all influenced by factors associated with the animal (pig genotype, physiological state, and health status) and the environment (diet composition, thermal and physical environment) and should be considered when establishing optimum dietary amino acid levels for groups of pigs”. Working backwards from this statement can help improve the ability to provide optimal nutrition to achieve greater environmental sustainability of pork production.
Accurate prediction of the lean growth rate of pigs allows for more accurate diet formulation and feeding management to ensure that the diets being fed meet, but do not exceed, their nutritional needs, which has a direct impact on the amount of excess nutrients excreted in manure and their subsequent environmental consequences. Because pigs are fed in groups, greater nutritional efficiency can be achieved if the BW and rate of lean growth of pigs within each group is similar so that the diets being fed better match the requirements of the entire group. However, under commercial conditions, there can be large differences in animal-to-animal lean growth rates in addition to differences in gender, genetic line, and nutrition (Schinckel and de Lange, 1996; Wiseman et al., 2007a; Wiseman et al., 2007b; Schinckel et al., 2008; Fix et al., 2010; Strathe et al., 2010; Schinckel et al., 2012; Strathe et al., 2015), which requires increasing margins of safety for AA in feed formulations to ensure that the nutrient requirements of a high proportion of pigs in a heterogenous group are met. As a result, overfeeding nutrients often occurs under these conditions. However, as previously discussed in section 3.2, penning barrows and gilts separately and feeding diets that more closely match their respective nutrient requirements, due to sex differences in AA requirements and feed intake, can improve NUE of swine production systems. An alternative approach that can overcome this challenge is to provide group housing of pigs of varying BW but use computerized individual feeding stalls capable of delivering customized diets (de Quelen et al., 2021).
Assessing the nutritional value of feed ingredients used in swine diets has evolved beyond determining metabolizable and net energy and digestible AA concentrations in feed ingredients. Although it is well accepted that SID AA digestibility must be used instead of total tract AA digestibility when formulating precision swine diets (Stein et al., 2007), obtaining SID values for AA in feed ingredients is a tedious task that requires time, money, and use of animals, and is also an imperfect science (Shurson et al., 2021). Estimates of endogenous AA losses obtained in an experiment can vary substantially from published values in the scientific literature due to differences in experimental conditions used in individual studies such as pig genotype, gastrointestinal health of the pigs, method of digesta sampling, and analytical procedures used (Boisen and Moughan, 1996; Jansman et al., 2002; Adeola et al., 2016). Therefore, endogenous AA losses must be determined under the experimental conditions used to determine SID values for AA of feed ingredients to provide accurate estimates and to compare with data from comparable studies. The nutritional value of feed ingredients must also include considerations of the role of functional nutrients and anti-nutritional compounds that affect health, efficiency, and productivity of pigs. Use of near infrared spectroscopy (Zhou et al., 2012; Noel et al., 2021) and validated prediction equations and models (Messad et al., 2016; Zeng et al., 2017) can be used to estimate SID AA concentrations of several types of feed ingredients but more efforts are needed for inclusion of additional ingredients. These approaches are essential for dynamically estimating nutrient content and digestibility of actual feed ingredients being fed to improve precision in feed formulation compared with using average, static published values. The National Animal Nutrition Program (NANP, 2023) has developed a large database of nutrient profiles for numerous ingredients with mean nutrient values and statistical variation estimates which can be useful in stochastic feed formulation. However, more efforts are needed for determining concentrations of anti-nutritional factors such as trypsin inhibitors, biogenic amines, and masked and emerging mycotoxins in feeding ingredients because of their detrimental effects on energy and nutrient utilization efficiency and animal health.
Multi-phase feeding is an essential feeding management practice that improves nutrient utilization efficiency by more closely matching nutrient composition of diets with the changing requirements of group-housed pigs. Feed budgets are an important part of multi-phase feeding because they provide management guidance of when the transition from one diet to the next in the sequence should occur based on actual feed consumption of the group of pigs being fed. Compared with a 2-phase grower-finisher feeding program, precision feeding (blending of two feeds to match requirements) has been shown to increase NUE by up to 40% (Monteiro et al., 2016; Andretta et al., 2018; Monteiro et al., 2021). Mixing high and low nutrient content feeds according to requirements of individual pigs and using individual feeding has been shown to reduce N excretion from 42 g/d with multi-phase group feeding to 38 g/d for individual customized feeding (Andretta et al., 2014). Similarly, a study to compare using a 3-phase grower-finisher group feeding strategy with daily adjustments of feed composition based on the growth performance of the average pig in the group and showed a 12% reduction in N excretion and no effects on growth when adjusting diet composition daily (Gaillard et al., 2020). For gestating sows, using a multi-phase feeding strategy reduced N excretion by 19% and feed cost by 8% (Dourmad et al., 2015).
The goal of feed processing is to improve the nutrient utilization of ingredients used in complete feeds by 1) producing a homogeneous mixture of dietary ingredients, 2) decrease antinutritional factors, 3) increase feed safety, 4) increase palatability and feed intake, and 5) achieve a particle size that improves feed efficiency while minimizing gut health problems (van der Poel et al., 2020). Optimal and efficient feed processing is dependent on a detailed understanding of molecular structures and functional properties of feed ingredients. Processes such as grinding, conditioning, roasting, pelleting, and extrusion change the three-dimensional structure, functional properties, and digestibility of Lys, protein, starch, and dietary fiber (van der Poel et al., 2020). Therefore, the molecular structure of feeds (Yu and Prates, 2017) and effects of various types of feed processing (de Vries et al., 2012; Rojas and Stein, 2015; Salazar-Villanea et al., 2016) on protein and AA digestibility must be considered when attempting to improve NUE in swine diets.
Reducing particle size of feed ingredients by grinding is a common practice and effective way of improving energy and nutrient digestibility of swine diets (Wondra et al., 1995a). Several studies have shown that reducing particles size improves digestibility of protein and AA in corn (Wondra et al., 1995b), barley-field pea diets (Oryschak et al., 2002), lupins (Kim et al., 2009b), and wheat (Mavromichalis et al., 2000). One study showed an improvement in energy digestibility but not protein and AA digestibility when particle size of DDGS is reduced (Liu et al., 2012). Other studies showed no effect of particle size reduction on AA digestibility of corn (Rojas and Stein, 2015), barley (Medel et al., 2000), and soybean meal (Fastinger and Mahan, 2003) except for an increase in four indispensable AA. However, in nearly all types of feed ingredients and diets, dry matter, digestible energy, and metabolizable energy concentrations increase as particle size is reduced, resulting in improvements in feed conversion (Rojas and Stein, 2017). However, diet particle size is one of several factors associated with an increase in gastric ulcers in pigs, which is a common problem in commercial swine farms worldwide which leads to economic losses from decreased growth rate and feed intake, increased mortality and morbidity (De Witte et al., 2017).
Extrusion has a greater effect on the physicochemical characteristics of feed ingredients than pelleting (Zijlstra et al., 2009) due to changes in temperature, pressure, friction, and attrition during the process, where it has been shown to improve feed conversion by 8% and N digestibility by 6% for complete feeds (Sauer et al., 1990). Extruding feed ingredients containing high concentrations of anti-nutritional factors has been shown to improve ileal digestibility of AA in pigs (Owusu-Asiedu et al., 2002; Sun et al., 2006; Stein and Bohlke, 2007; Rojas et al., 2016). Hydrothermal processing, such as extrusion and expansion, reduces heat-labile anti-nutritional factors and subsequently improves protein and AA digestibility in swine diets (Zentek et al., 2020). However, inconsistent responses for improvements in protein and AA digestibility have been reported among various studies. Extrusion had no effect on improving protein and AA digestibility of corn (Muley et al., 2007) and a flaxseed-field pea mixture, but protein and AA digestibility of soybean meal (Chae et al., 1997) and field peas (Stein and Bohlke, 2007; Htoo et al., 2008) have been shown to be improved. Extrusion of diets containing rapeseed meal either not toasted or toasted for 60 or 120 minutes resulted in improvements of 3.4%, 4.3%, and 9.3% in apparent ileal CP digestibility, respectively compared with feeding meal diets (Salazar-Villanea et al., 2018). Extrusion, with or without pelleting, of diets containing corn, soybean meal, DDGS, and soybean hulls has also been shown to improve digestibility of protein and most indispensable AA (Rojas et al., 2016). However, the reasons for inconsistent digestibility responses among studies and ingredients are unclear.
Pelleting involves steam and pressure as feed is forced through a pellet die which changes the physicochemical properties of ingredients (Zijlstra et al., 2009). Nitrogen digestibility of corn-soybean meal diets has been shown to be improved by 5 to 8% when pelleted compared with feeding meal diets to pigs (Wondra et al., 1995a). However, thickness of the pellet die had no effect on SID of AA in corn and wheat fed to pigs (Lahaye et al., 2007). Several other studies have shown that CP and AA digestibility is improved in wheat-canola meal diets (Lahaye et al., 2008), field peas (Stein and Bohlke, 2007), and wheat-soybean meal diets (Ginste and de Schrijver, 1998). Improvements in AA digestibility from conditioning and pelleting processes have been attributed to increased starch gelatinization and changes in protein conformation which improve the efficiency of endogenous enzyme to diets these substrates (Rojas and Stein, 2017). As a result, pelleting swine diets has been shown in numerous studies to improve feed conversion by 4 to 12 percentage units (Walker, 1989; Steidinger et al., 2000; Xing et al., 2004; Lewis et al., 2015; Paulk and Hancock, 2016).
Feed wastage contributes to N and other nutrient inefficiencies in pig production systems but there are no simple or practical methods for measuring it in commercial pig facilities (Skerman et al., 2016). A practical minimum feed wastage is 5% but can be as much as 15% or more depending on types of feeders and feeder management (Skerman et al., 2016). Skerman et al. (2016) conducted a simulation experiment to determine the effect of 0%, 4.2%, 9.4% and 15.2% feed wastage on ammonium nitrogen (NH4-N) and total Kjeldahl nitrogen (TKN) of pig manure in facilities. As expected, NH4-N and TKN significantly increased in manure between 0 and 4% feed wastage, due to N concentrations in unconsumed feed being greater than digested and excreted feed. However, further increases in feed wastage from 4% to 9% and from 9% to 15% did not result in the same magnitude increase in NH4-N and TKN, which the researchers suggested was due to decay products being produced.
Managing variability in energy and digestible nutrient composition of feed ingredients, along with variability in nutrient requirements and feed intake of groups of pigs under commercial conditions may be the greatest challenge for improving NUE. Dynamically estimating nutrient content and digestibility of actual feed ingredients being fed is necessary for improving precision in feed formulation. Separate sex, multi-phase feeding of group housed pigs, and the potential widespread use of computerized individual feeding stalls capable of delivering customized diets are practical solutions for substantially reduce overfeeding and underfeeding of nutrients and improve NUE. Feed processing methods that consistently improve nutrient digestibility and feeder design and adjustments also offer significant opportunities to reduce N waste on pig farms.
Increasing the amount of N retained in the pig, especially in edible lean meat, while also reducing N excretion and emissions are needed in order for swine production to become more environmentally sustainable. However, there are many challenges for improving NUE on a molecular or biochemical basis in pigs. Improving marginal efficiencies of AA use for whole body protein accretion using genetic selection in breeding programs appears be limited because such improvements have low heritability and there are 20 indispensable and dispensable AA efficiencies to target. Furthermore, even if transgenic techniques can be used to alter such efficiencies (e.g., growth hormone insertion ‘Beltsville pig’, Thompson, 1997; phytase enzyme gene insertion ‘Enviropig™’, Forsberg et al., 2013), the commercial adoption of genetically modified lines in the swine industry have many ethical and regulatory challenges (Thompson, 1997; Bovenkerk, 2020). Although NUE can also be improved through sperm sexing for gender selection, immunocastration, as well as use of exogenous growth hormone, ractopamine, and other metabolism modifiers; they also create implementation and management challenges, can be negatively perceived by consumers, involve national regulatory restrictions, and affect international trade policies.
Paramount to improving NUE, is the need for greater understanding of nutrient needs for lean growth, prediction of feed intake, and causes of variation of these two factors within specific populations of pigs being fed. Unfortunately, research studies to identify and quantify external factors affecting short- and long-term rates of lean growth and feed intake have been limited. These effects include but are not limited to, environmental (e.g., heat, cold, building gases), social (e.g., group size, animal mixing, social structure), managerial (feed and water space needs, out-of-feed events, feed wastage), and disease (disease-specific effects, post-infection lean growth, and compensatory feed intake). The challenge of determining whether to formulate a common diet for the highest lean growth potential individuals or the average lean growth potential of a population of pigs in group-feeding programs inherently results in suboptimal N and nutrient utilization efficiency. If diets are formulated to meet the nutrient needs of the animals with highest lean growth potential, then a substantial proportion of animals within the contemporary group are consuming nutrients in excess of their nutrient requirements resulting in suboptimal nutritional efficiency and excess excretion in manure. This excess nutrient is further compounded by the magnitude and intentional use of a margin of safety commonly assigned to AA concentrations and digestibility of major feed ingredients due to uncertainty of actual values of the specific sources being fed. This diet feed formulation practice ensures that all nutrient requirements are met or exceeded so that the genetic potential for lean growth is achieved. In contrast, formulating diets for the average lean growth potential in a group result in underfeeding as well as overfeeding nutrients to the majority of pigs within a group which also reduces nutritional efficiency. Given that excess nutrient intake does not increase lean tissue deposition, NUE is consequently abysmal in both of these scenarios. There is increasing interest in using individual feeding stations in group-housing systems that allow custom blending of different diets to more accurately deliver accurate nutrition to individual pigs in precision feeding systems. Multi-phase feeding strategies are an essential management practice to improve nutrient utilization efficiency, which allows more closely matching nutrient content of diets with constantly changing energy and digestible nutrient requirements during growth, reproduction, and lactation phases. Regardless of the feeding scenario used, a greater understanding of these factors is needed so that modeling efforts can be developed to accurately describe rates of feed intake and lean tissue accretion in order to optimize precision feeding of an entire group of animals.
If nutrient requirements and feed intake of pigs are more predictable, then feed formulation can be improved to match the unique nutritional needs more accurately under various commercial production scenarios. The use of the ideal AA concept clearly provides an avenue to prevent AA limitations and inefficiencies in feed formulations, but better information is needed on the impact of excess AA on lean growth and overall nutrient utilization; especially because excess AA are deaminated and their N is subsequently excreted from the body. In addition, methods for managing variation in energy and nutrient composition and digestibility/bioavailability of feed ingredients is crucial. Although animal protein by-products and grain milling co-products are considered to have high variation in nutritional composition, it is also known that corn and soybean meal vary in their composition and digestibility. Therefore, because corn and soybean meal are often the two major energy and protein sources used in swine diets, accurately predicting their energy and digestive AA concentrations of specific sources being fed would improve overall dietary NUE. Use of NIRS and validated prediction equations based on chemical composition of ingredients are promising approaches to provide accurate, dynamic estimates for precision diet formulation. Strategic use of selected feed additives that have consistently been shown to improve nutrient digestibility and subsequent feed conversion can provide additional benefits for improving dietary N utilization if they are cost effective. Unfortunately, more research to determine the mechanisms of action to improve the predictability of positive responses of feed additives under various commercial production scenarios is needed. Feed manufacturing processes such as grinding, conditioning, roasting, pelleting, and extrusion can change the three-dimensional molecular structure of feed ingredient matrices to improve their digestibility and potentially, their nutrient bioavailability. However, the economic value of improved energy and nutrient digestibility resulting from these processes must greater than the cost of performing such energy-intensive processes.
Overall, it is clear that accurate feed formulation and feeding closer to actual nutrient needs of pigs has a profound effect on reducing excretion of nutrients, especially N-containing compounds, and ultimately the environmental footprint of pork production. Therefore, ultimate goal for improving NUE in pork production systems must involve the 4 R’s of getting the “right amount of energy and nutrients in the right feed fed to the right pigs at the right time”.
Both GS and BK contributed to the conceptualization, data curation, analysis, writing, reviewing, and editing of this manuscript. All authors contributed to the article and approved the submitted version.
The authors declare that the research was conducted in the absence of any commercial or financial relationships that could be construed as a potential conflict of interest.
All claims expressed in this article are solely those of the authors and do not necessarily represent those of their affiliated organizations, or those of the publisher, the editors and the reviewers. Any product that may be evaluated in this article, or claim that may be made by its manufacturer, is not guaranteed or endorsed by the publisher.
Aaslyng M. D., Broge E. H. D. L., Brockhoff P. B., Christensen R. H. B. (2016). The effect of skatole and androstenone on consumer response towards fresh pork from m. longissimus thoracis et lumborum and m. semimembranosus. Meat Sci. 116, 174–185. doi: 10.1016/j.meatsci.2016.01.013
Abbas K., Raza A., Vasquez R. D., Roldan M. J. M., Malhotra N., Huang J.-C., et al. (2022). Ractopamine at the center of decades-long scientific and legal disputes: a lesson on benefits, safety issues, and conflicts. Biomolecules 12, 1342. doi: 10.3390/biom12101342
Adeola O., Xue P. C., Cowieson A. J., Ajuwon K. M. (2016). Basal endogenous losses of amino acids in protein nutrition research for swine and poultry. Anim. Feed Sci. Tech 221, 274–283. doi: 10.1016/j.anifeedsci.2016.06.004
Aldal I., Andresen O., Egeli A. K., Haugen J. E., Grodum A., Fjetland O., et al. (2005). Levels of androstenone and skatole and the occurrence of boar taint in fat from young boars. Livest. Prod. Sci. 95, 121–129. doi: 10.1016/j.livprodsci.2004.12.010
Amdi C., Krogh U., Flummer C., Oksbjerg N., Hansen C. F., Theil P. K. (2013). Intrauterine growth restricted piglets defined by their head shape ingest insufficient amounts of colostrum. J. Anim. Sci. 91, 5605–5613. doi: 10.2527/jas.2013-6824
Amdi C., Lynegaard J. C., Thymann T., Williams A. R. (2020). Intrauterine growth restriction in piglets alters blood cell counts and impairs cytokine responses in peripheral mononuclear cells 24 days post-partum. Sci. Rep. 10, 4683. doi: 10.1038/s41598-020-61623-w
Andretta I., Hauschild L., Kipper M., Pires P. G. S., Pomar C. (2018). Environmental impacts of precision feeding programs applied in pig production. Animal 12, 1990–1998. doi: 10.1017/S1751731117003159
Andretta I., Pomar C., Rivest J., Pomar J., Lovatto P. A., Radünz Neto J. (2014). The impact of feeding growing-finishing pigs with daily tailored diets using precision feeding techniques on animal performance, nutrient utilization, and body and carcass composition. J. Anim. Sci. 92, 3925–3936. doi: 10.2527/jas.2014-7643
Apple J. K., Rincker P. J., McKeith F. K., Carr S. N., Armstrong T. A., Matzat P. D. (2007). Review: meta-analysis of the ractopamine response in finishing swine. Prof. Anim. Sci. 23, 179–196. doi: 10.15232/S1080-7446(15)30964-5
ARC (1981). The nutrient requirements of pigs. agricultural research council working party. Commonwealth Agric. Bureaux (Slough, England).
Asmus M. D., Tavarez M. A., Tokach M. D., Dritz S. S., Schroeder A. L., Nelssen J. L., et al. (2014). The effects of immunological castration and corn dried distillers grains with solules withdrawal on growth performance, carcass characteristics, fatty acid analysis, and iodine value of pork fat depots. J. Anim. Sci. 92, 2116–2132. doi: 10.2527/jas2013-6910
Babol J., Squires J. (1995). Quality of meat from entire male pigs. Food Res. Int. 28, 201–212. doi: 10.1016/0963-9969(95)93528-3
Balcombe K., Bradley D., Fraser I. (2021). Do consumers really care? an economic analysis of consumer attitudes towards food produced using prohibited production methods. J. Agric. Econ. 72, 452–469. doi: 10.1111/1477-9552.12410
Ball M. E. E., Smyth S., Beattie V. E., McCracken K. J., McCormack U., Muns R., et al. (2022). The environmental impact of lowering dietary crude protein in finishing pig diets-the effect on ammonia, odour and slurry production. Sustainability 14, 12016. doi: 10.3390/su141912016
Batorek N., Čandek-Potokar M., Bonneau M., Van Milgen J. (2012). Meta-analysis of the effect of immunocastration on production performance, reproductive organs and boar taint compounds. Animal 8, 1330–1338. doi: 10.1017/S1751731112000146
Beach E. F., Munks B., Robinson A. (1943). The amino acid composition of animal tissue protein. J. Biol. Chem. 148, 431–439. doi: 10.1016/S0021-9258(18)72300-4
Beerman D. H., Fishell V. K., Roneker K., Boyd R. D., Armbruster G., Souza L. (1990). Dose-response relationships between porcine somatropin, muscle composition, muscle fiber characteristics and pork quality. J. Anim. Sci. 68, 2690–2697. doi: 10.2527/1990.6892690x
Bikker P., Verstegan M. W. A., Bosch M. W. (1994). Amino acid composition of growing pigs is affected by protein and energy intake. J. Nutr. 124, 1961–1969. doi: 10.1093/jn/124.10.1961
Birkenfeld C., Ramanau A., Kluge H., Spilke J., Eder K. (2005). Effects of dietary l-carnitine supplementation on growth performance of piglets from control sows or sows treated with l-carnitine during pregnancy and lactation. J. Anim. Physiol. Anim. Nutr. 89, 277–283. doi: 10.1111/j.1439-0396.2005.00517x
Blachier F., Mariotti F., Huneau J. F., Tome D. (2007). Effects of amino acid-derived luminal metabolites on the colonic epithelium and physiopathological consequences. Amino Acids 33, 547–562. doi: 10.1007/s00726-006-0477-9
Bloxham D. J., Azain M., Pesti G. M., Wu S.-B. (2022). Total amino acid level affects the results of standardized ileal digestibility assays for feed ingredients for swine. Anim. Nutr. 13, 19–30. doi: 10.1016/j.aninu.2022.08.018
Bohrer B. M., Kyle J. M., Boler D. D., Rincker P. J., Ritter M. J., Carr S. N. (2013). Meta-analysis of the effects of ractopamine hydrochloride on carcass cutability and primal yields of finishing pigs. J. Anim. Sci. 91, 1015–1023. doi: 10.2527/jas2012-5647
Boisen S., Moughan P. J. (1996). Dietary influences on endogenous ileal protein and amino acid loss in the pig-a review. Acta Agric. Scand. Sect. A. Anim. Sci. 46, 154–164. doi: 10.1080/09064709609415866
Bonneau M. (1982). Compounds responsible for boar taint, with special emphasis on androstenone: a review. Livest. Prod. Sci. 9, 687–705. doi: 10.1016/0301-6226(82)90017-3
Bovenkerk B. (2020). Ethical perspectives on modifying animals: beyond welfare arguments. Anim. Front. 10, 45–50. doi: 10.1093/af/vfz055
Burton D. L., Beauchamp E. G. (1986). Nitrogen losses from swine housings. Agric. Wastes 15, 59–74. doi: 10.1016/0141-4607(86)90126-5
Caine W. R., Sauer W. C., Tamminga S., Verstegen M. W. A., Schulze H. (1997). Apparent ileal digestibilities of amino acids in newly weaned pigs fed diets with protease-treated soybean meal. J. Anim. Sci. 75, 2962–2969. doi: 10.2527/1997.75112962x
Campbell R. G., Taverner M. R. (1988). Genotype and sex effects on the relationships between energy-intake and protein deposition in growing pigs. J. Anim. Sci. 66, 676–686. doi: 10.2527/jas1988.663676x
Canh T. T., Aarnink A. J. A., Schutte J. B., Sutton A., Langhout D. J., Langhout D. D. J., et al. (1998c). Dietary protein affects nitrogen excretion and ammonia emissions from slurry of growing finishing pigs. Livest. Prod. Sci. 56, 181–191. doi: 10.1016/S0301-6226(98)00156-0
Canh T. T., Aarnink A. J. A., Verstegen M. W. A., Schrama J. W. (1998a). Influence of dietary factors on the pH and ammonia emission of slurry from growing-finishing pigs. J. Anim. Sci. 76, 1123–1130. doi: 10.2527/1998.7641123x
Canh T. T., Sutton A. L., Aarnink A. J. A., Verstegen M. W. A., Schrama J. W., Bakker G. C. M. (1998b). Dietary carbohydrates alter the fecal composition and pH and the ammonia emission from slurry of growing pigs. J. Anim. Sci. 76, 1887–1895. doi: 10.2527/1998.7671887x
Canh T. T., Verstegen M. W. A., Aarnink A. J. A., Schrama J. W. (1997). Influence of dietary factors on nitrogen partitioning and composition of urine and feces of fattening pigs. J. Anim. Sci. 75, 700–706. doi: 10.2527/1997.753700x
Cappelaere L., Grandmaison J. L. C., Martin N., Lambert W. (2021). Amino acid supplementation to reduce environmental impacts of broiler and pig production: a review. Front. Vet. Sci. 8. doi: 10.3389/fvets.2021.689259
Cemin H. S., Tokach M. D., Dritz S. S., Woodworth J. C., DeRouchey J. M., Goodband R. D. (2019). Meta-regression analysis to predict the influence of branched-chain and large neutral amino acids on growth performance of pigs. J. Anim. Sci. 97, 2505–2514. doi: 10.1093/jas/skz118
Chae B. J., Han I. K., Kim J. H., Yang C. J., Chung Y. K., Rhee Y. C., et al. (1997). Effects of extrusion conditions of corn and soybean meal on the physico-chemical properties, ileal digestibility and growth of weaned pig. Asian Australas. J. Anim. Sci. 10, 170–177. doi: 10.5713/ajas.1997.170
Che L., Yang Z., Xu M., Xu S., Che L., Lin Y., et al. (2017). Maternal nutrition modulates fetal development by inducing placental efficiency changes in gilts. BMC Genomics 18, 213. doi: 10.1186/s12864-017-3601-1
Chen P., Baas T. J., Mabry J. W., Dekkers J. C. M., Koehler K. J. (2002). Genetic parameters and trends for lean growth rate and its components in U.S. Yorkshire, duroc, Hampshire, and landrace pigs. J. Anim. Sci. 80, 2062–2070. doi: 10.1093/ansci/80.8.2062
Chen H., Wierenga P. A., Hendriks W. H., Jansman A. J. M. (2019). In vitro protein digestion kinetics of protein sources for pigs. Animal 13, 1154–1164. doi: 10.1017/S1751731118002811
Cho W. Y., Kim J. H., Be S. H., Han I. K., Han Y. K., Heo K. N., et al. (1999). Effects of l-carnitine with different lysine levels on growth and nutrient digestibility in pigs weaned at 21 days of age. Asian-Australas. J. Anim. Sci. 12, 799–805. doi: 10.5713/ajas.1999.799
Clark O. G., Moehn S., Edeogu I., Price J., Lenard J. (2005). Manipulation of dietary protein and nonstarch polysaccharide to control swine manure emissions. J. Environ. Qual. 34, 1461–1466. doi: 10.2134/jeq2004.0434
Claus R., Weiler U., Herzog A. (1994). Physiological aspects of androstenone and skatole formation in the boar - a review with experimental data. Meat Sci. 38, 289–305. doi: 10.1016/0309-1740(94)90118-X
Columbus D. A., Lapierre H., Htoo J. K., de Lange C. F. M. (2014). Nonprotein nitrogen is absorbed from the large intestine and increases nitrogen balance in growing pigs fed a valine-limiting diet. J. Nutr. 144, 614–620. doi: 10.3945/jn.113.187070
Conde-Aguilera J. A., Cobo-Ortega C., Mercier Y., Tesseraud S., van Milgen J. (2014). The amino acid composition of tissue protein is affected by the total sulfur amino acid supply in growing pigs. Animal 8, 401–409. doi: 10.1017/S1751731113002425
Costa A. (2017). Ammonia concentrations and emissions from finishing pigs reared in different growing rooms. J. Environ. Qual. 46, 255–260. doi: 10.2134/jeq2016.04.0134
Costa O. A. D., Feddern V., Athayde N. B., Manzke N. E., de Oliveira Roça R., dos Santos Lopes L., et al. (2018). Ractopamine supplementation improves leanness and carcass yield, minimally affecting pork quality in immunocastrated pigs. Sci. Agric. 75, 197–207. doi: 10.1590/1678-992X-2016-0321
Cowieson A. J. (2010). Strategic selection of exogenous enzymes for corn/soy-based poultry diets. J. Poult. Sci. 47, 1–7. doi: 10.2141/jpsa.009045
Cowieson A. J., Acamovic T., Bedford M. R. (2004). The effects of phytase and phytic acid on the loss of endogenous amino acids and minerals from broiler chickens. Br. Poult. Sci. 45, 101–108. doi: 10.1080/00071660410001668923
Cowieson A. J., Bedford M. R. (2009). The effect of phytase and carbohydrase on ileal amino acid digestibility in monogastric diets: complementary mode of action? World’s Poult. Sci. J. 65, 609–624. doi: 10.1017/S0043933909000427
Cowieson A. J., Bedford M. R., Selle P. H., Ravindran V. (2009). Phytate and microbial phytase: implications for endogenous nitrogen losses and nutrient availability. World’s Poult. Sci. J. 65, 401–418. doi: 10.1017/S0043933909000294
Cowieson A. J., Ravindran V. (2007). Effect of phytic acid and microbial phytase on the flow and amino acid composition of endogenous protein at the terminal ileum of growing broiler chickens. Br. J. Nutr. 98, 745–752. doi: 10.1017/S0007114507750894
Cowieson A. J., Ruckebusch J. P., Knap I., Guggenbuhl P., Fru-Nji F. (2016). Phytate-free nutrition: a new paradigm in monogastric animal production. Anim. Feed Sci. Technol. 222, 180–189. doi: 10.1016/j.anifeedsci.2016.10.016
Cowieson A. J., Ruckebusch J.-P., Sorbara J. O. B., Wilson J. W., Guggenbuhl P., Tanadini L., et al. (2017). A systematic view on the effect of microbial phytase on ileal amino acid digestibility in pigs. Anim. Feed Sci. Technol. 231, 138–149. doi: 10.1016/janifeedsci.2017.07.007
Crews D. H. Jr. (2005). Genetics of efficient feed utilization and national cattle evaluation: a review. Genet. Mol. Res. 4, 152–165.
Cromwell G. L., Cline T. R., Crenshaw J. D., Crenshaw T. D., Ewan R. C., Hamilton C. R., et al. (1993). The dietary protein and(or) lysine requirements of barrows and gilts. J. Anim. Sci. 71, 1510–1519. doi: 10.2527/1993.7161510x
D'Mello J. P. F. (2003). “Adverse effects of amino acids,” in Amino acids in animal nutrition. Ed. D’Mello J. P. F. (Wallingford, UK: CABI), 125–142.
Dai Z., Wu Z., Jia S., Wu G. (2014). Analysis of amino acid composition in proteins of animal tissues and foods as pre-column o-phthaldialdehyde derivatives by HPLC with fluorescence detection. J. Chrom. B. 964, 116–127. doi: 10.1016/j.jchromb.2014.03.025
Danfær A. C., Strathe A. B. (2012). “Quantitative and physiological aspects of pig growth,” in Nutritional physiology of pigs. Eds. Knudsen K. E. B., Kjeldsen N. J., Poulsen H. D., Jensen B. B. (Copenhagen (Denmark: SEGES Danish Pig Research Centre).
Darragh A. J., Cranwell P. D., Moughan P. J. (1994). Absorption of lysine and methionine from the proximal colon of the piglet. Br. J. Nutr. 71, 739–752. doi: 10.1079/bjn19940191
de Lange C. F. M., Levesque C. L., Kerr B. J. (2012). “Amino acid nutrition and feed efficiency,” in Feed efficiency in swine. Ed. Patience J. F. (Wageningen: Wageningen Academic Publishers), 81–100. doi: 10.3920/978-90-8686-756-1_4
de Moraes P., Allison J., Robinson J. A., Baldo G. L., Boeri F., Borla P. (2013). Life cycle assessment (LCA) and environmental product declaration (EPD) of an immunological product for boar taint control in male pigs. J. Environ. Assess. Policy Manage. 15, 1–26. doi: 10.1142/S1464333213500014
de Quelen F., Brossard L., Wilfart A., Dourmad J.-Y., Garcia-Launay F. (2021). Eco-friendly feed formulation and on-farm feed production as ways to reduce the environmental impacts of pig production without consequences on animal performance. Front. Vet. Sci. 8. doi: 10.3389/fvets.2021.689012
de Vries S., Pustjens A. M., Schols H. A., Hendriks W. H., Gerrits W. J. J. (2012). Improving digestive utilization of fiber-rich feedstuffs in pigs and poultry by processing and enzyme technologies: a review. Anim. Feed Sci. Technol. 178, 123–138. doi: 10.1016/j.anifeedsci.2012.10.004
De Witte C., Devriendt B., Flahou B., Bosschem I., Ducatelle R., Smet A., et al. (2017). Helicobacter suis induces changes in gastric inflammation and acid secretion markers in pigs of different ages. Vet. Res. 48, 34. doi: 10.1186/s13567-017-0441-6
Dong L., Zhong X., Ahmad H., Li W., Wang Y., Zhang L., et al. (2014). Intrauterine growth restriction impairs small intestinal mucosal immunity in neonatal piglets. J. Histochem. Cytochem. 62, 510–518. doi: 10.1369/0022155414532655
Dourmad J. Y., van Milgen J., Valancogne A., Dubois D., Brossard L., Noblet J. (2015). “Chapter 4 – modeling nutrient utilization in sows: a way towards the optimization of nutritional supplies,” in Nutritional modelling for pigs and poultry. Eds. Sakomura N. K., Gous R. M., Kyriazakis I., Hauschild L. (Wallingford, UK: CABI Publishing), 50–61.
Dugan M. E. R., Aalhus J. L., Lien K. A., Schaefer A. L., Kramer J. K. G. (2001). Effects of feeding different levels of conjugated linoleic acid and total oil to pigs on live animal performance and carcass composition. Can. J. Anim. Sci. 81, 505–510. doi: 10.4141/A00-101
Dugan M. E. R., Aalhus J. L., Schaefer A. L., Kramer J. K. G. (1997). The effect of conjugated linoleic acid on fat to lean repartitioning and feed conversion in pigs. Can. J. Anim. Sci. 77, 723–725. doi: 10.4141/A97-084
Dunshea F. R., Allison J. R. D., Bertram M., Boler D. D., Brossard L., Campbell R., et al. (2013). The effect of immunization against GnRF on nutrient requirements of male pigs: a review. Animal 7, 1769–1778. doi: 10.1017/S1751731113001407
Dunshea F., Colantoni C., Howard K., McCauley I., Jackson P., Long K. A., et al. (2001). Vaccination of boars with a GnRH vaccine (Improvac) eliminates boar taint and increases growth performance. J. Anim. Sci. 79, 2524–2535. doi: 10.2527/2001.79102524x
Eder K. (2009). Influence of l-carnitine on metabolism and performance of sows. Br. J. Nutr. 102, 645–654. doi: 10.1017/S0007114509990778
Ekstrom K. E. (1991). “Genetic and sex considerations in swine nutrition,” in Swine nutrition. Eds. Miller E. R., Ullrey D. E., Lewis A. J. (Stoneham, MA: Butterworth-Heinemann), 415–424.
Elsbernd A. J., Patience J. F., Prusa K. J. (2016). A comparison of the quality of fresh and frozen pork from immunologically castrated males versus gilts, physical castrates, and entire males. Meat Sci. 111, 110–115. doi: 10.1016/j.meatsci.2015.07.003
Elsbernd A. J., Stalder K. J., Karriker L. A., Patience J. F. (2015). Comparison among gilts, physical castrates, entire males, and immunological castrates in terms of growth performance, nitrogen and phosphorus retention, and carcass iodine value. J. Anim. Sci. 93, 5702–5710. doi: 10.2527/jas.2015-9295
Erisman J. W., Galloway J. N., Seitzinger S., Bleeker A., Dise N. B., Petrescu A. M. R., et al. (2013). Consequences of human modification of the global nitrogen cycle. Phil. Trans. R. Soc B 368, 20130166. doi: 10.1098/rstb.2013.0116
Etherton T. D. (2000). The biology of somatotropin in adipose tissue growth and nutrient partitioning. J. Nutr. 130, 2623–2625. doi: 10.1093/jn/130.11.2623
Etherton T. D., Smith S. B. (1991). Somatotropin and β-adernergic agonists: their efficacy and mechanisms of action. J. Anim. Sci. 69 (Suppl. 2), 2–26. doi: 10.2527/1991.69SUPPL22X
FAO (2022). Meat market review: emerging trends and outlook (Rome, Italy: Food and Agriculture Organization of the United Nations). Available at: https://www.fao.org/3/cc3164en/cc3164en.pdf.
Fastinger N. D., Mahan D. C. (2003). Effect of soybean meal particle size on amino acid and energy digestibility in grower-finisher swine. J. Anim. Sci. 81, 697–704. doi: 10.2527/2003.813697x
Fernández-Fígares I., Conde-Aguilera J. A., Nieto R., Lachica M., Aguilera J. F. (2008). Synergistic effects of betaine and conjugated linoleic acid on the growth and carcass composition of growing Iberian pigs. J. Anim. Sci. 86, 102–111. doi: 10.2527/jas.2006-0230
Fernández-Fígares I., Lachica M., Martín A., Nieto R., González-Valero L., Rodríguez-López J. M., et al. (2012). Impact of dietary betaine and conjugated linoleic acid on insulin sensitivity, protein and fat metabolism of obese pigs. Animal 6, 1058–1067. doi: 10.1017/S1751731111002308
Fix J. S., Cassady J. P., van Heugten E., Hanson D. J., See M. T. (2010). Differences in lean growth performance of pigs sampled from 1980 and 2005 commercial swine fed 1980 and 2005 representative feeding programs. Livest. Sci. 128, 108–114. doi: 10.1016/j.livsci.2009.11.006
Forsberg C. W., Meidinger R. G., Liu M., Gottrill M., Golovan S., Phillips J. P. (2013). Integration, stability and expression of the e. coli phytase transgene in the cassie line of Yorkshire EnviropigTM. Transgenic Res. 22, 379–389. doi: 10.1007/s11248-012-9646-7
Foster T. P. (1999). Somatotropin delivery to farmed animals. Adv. Drug Del. Rev. 38, 151–165. doi: 10.1016/s0169-409x(99)00014-9
Foxcroft G. R., Dixon W. T., Dyck M. K., Novak S., Harding J. C. S., Almeida F. C. R. L. (2009). Prenatal programming of postnatal development in the pig. Soc Reprod. Fert. Suppl. 66, 213–231.
Fritz I. B., Yue K. T. N. (1963). Long-chain carnitine acyltransferase and the role of acylcarnitine derivatives in the catalytic increase of fatty acid oxidation induced by carnitine. J. Lipid Res. 4, 279–288. doi: 10.1016/S0022-2275(20)40302-5
Fuller M. F., Reeds P. J. (1998). Nitrogen cycling in the gut. Annu. Rev. Nutr. 18, 385–411. doi: 10.1146/annurev.nutr.18.1.385
Gaillard C., Brossard L., Dourmad J.-Y. (2020). Improvement of feed and nutrient efficiency in pig production through precision feeding. Anim. Feed Sci. Technol. 268, 114611. doi: 10.1016/j.anifeedsci.2020.114611
Galassi G., Colombini S., Malagutti L., Crovetto G. M., Rapetti L. (2010). Effects of high fibre and low protein diets on performance, digestibility nitrogen excretion and ammonia emission in the heavy pig. Anim. Feed Sci. Technol. 161, 140–148. doi: 10.1016/j.anifeedsci.2010.08.009
Galloway J. N., Townsend A. R., Erisman J. W., Bekunda M., Cai Z., Freney J. R., et al. (2008). Transformation of the nitrogen cycle: recent trends, questions and potential solutions. Science 320, 889–892. doi: 10.1126/science.1136674
Garcia-Launay F., Dusart L., Espagnol S., Laisse-Redoux S., Gaudré D., Méda B., et al. (2018). Multiobjective formulation is an effective method to reduce environmental impacts of livestock feeds, br. J. Nutr. 120, 1298–1309. doi: 10.1017/S0007114518002672
Gargallo J., Zimmerman D. (1981). Effect of casein and starch infusion in the large intestine on nitrogen metabolism of growing swine. J. Nutr. 111, 1390–1396. doi: 10.1093/jn/111.8.1390
Gaskins H. R., Collier C. T., Anderson D. B. (2002). Antibiotics as growth promotants: mode of action. Anim. Biotech. 13, 29–42. doi: 10.1081/ABIO-120005768
Gerber P., Uwizeye A., Schulte R., Opio C., de Boer I. (2014). Nutrient use efficiency: a valuable approach to benchmark the sustainability of nutrient use in global livestock production? Curr. Opin. Environ. Sustain. 9–10, 122–130. doi: 10.1016/j.cosust.2014.09.007
Gerrits W. J. J., Schop M. T. A., de Vries S., Dijkstra J. (2021). ASAS-NANP symposium: digestion kinetics in pigs: the next step in feed evaluation and a ready-to-use modeling exercise. J. Anim. Sci. 99, 1–8. doi: 10.1093/jas/skab020
Ginste J. V., de Schrijver R. (1998). Expansion and pelleting of starter, grower and finisher diets for pigs: effects on nitrogen retention, ileal and total tract digestibility of protein, phosphorus and calcium and in vitro protein quality. Anim. Feed Sci. Technol. 72, 303–314. doi: 10.1016/S0377-8401(97)00192-2
Gonçalves M. A. D., Nitikanchana S., Tokach M. D., Dritz S. S., Bello N. M., Goodband R. D., et al. (2015). Effects of standardized ileal digestible tryptophan: lysine ratio on growth performance of nursery pigs. J. Anim. Sci. 93, 3909–3918. doi: 10.2527/jas.2015-9083
Grindflek E., Meuwissen T. H., Aasmundstad T., Hamland H., Hansen M. H., Nome T., et al. (2011). Revealing genetic relationships between compounds affecting boar taint and reproduction in pigs. J. Anim. Sci. 89, 680–692. doi: 10.2527/jas.2010-3290
Guay F., Donovan S. M., Trottier N. L. (2006). Biochemical and morphological developments are partially impaired in intestinal mucosa from growing pigs fed reduced-protein diets supplemented with crystalline amino acids. J. Anim. Sci. 84, 1749–1760. doi: 10.2527/jas.2005-558
Hăbeanu M., Lefter N. A., Gheorghe A., Untea A., Grigore D.-M., Varzaru I., et al. (2019). Evaluation of performance, nitrogen metabolism and tissue composition in barrows fed an n-3 PUFA-rich diet. Animals 9, 234. doi: 10.3390/ani9050234
Hales J., Moustsen V. A., Nielsen M. B. F., Hansen C. F. (2013). Individual physical characteristics of neonatal piglets affect preweaning survival of piglets born in a noncrated system. J. Anim. Sci. 91, 4991–5003. doi: 10.2527/jas.2012-5740
Han I. K., Lee J. H., Piao X. S., Li D. (2001). Feeding and management system to reduce environmental pollution in swine production – review. Asian-Aust. J. Anim. Sci. 14, 432–444. doi: 10.5713/ajas.2001.432
Han Y.-K., Thacker P. A. (2006). Effects of l-carnitine, selenium-enriched yeast, jujube fruit and hwangto (red clay) supplementation on performance and carcass measurements of finishing pigs. Asian-Australs. J. Anim. Sci. 2, 217–223. doi: 10.5713/ajas.2006.217
Hansen B. C., Lewis A. J. (1993). Effects of dietary protein concentration (corn:soybean meal ratio) on the performance and carcass characteristics of growing boars, barrows, and gilts: mathematical descriptions. J. Anim. Sci. 71, 2122–2132. doi: 10.2527/1993.7182122x
Harper A. E., Benevenga N. J., Wohlheuter R. M. (1970). Effects of ingestion of disproportionate amounts of amino acids. Physiol. Rev. 50, 428–558. doi: 10.1152/physrev.1970.50.3.428
Harris E. K., Mellencamp M. A., Johnston L. J., Cox R. B., Shurson G. C. (2017). Effect of time interval between the second improvest® dose and slaughter and corn dried distillers grains with solubles feeding strategies on carcass composition, primal cutout, and pork quality of immunologically castrated pigs. Meat Sci. 127, 13–20. doi: 10.1016/j.meatsci.2017.01.003
Harris E. K., Mellencamp M. A., Johnston L. J., Cox R. B., Shurson G. C. (2018). Effectiveness of different corn dried distillers grains with solubles feeding strategies and increasing the time intervals between the second improvest dose and slaughter of immunologically castrated pigs on belly and pork fat quality. Meat Sci. 135, 62–73. doi: 10.1016/j.meatsci.2017.08.025
Hayes E. T., Leek A. B. G., Curran T. P., Dodd V. A., Carton O. T., Beattie V. E., et al. (2004). The influence of diet crude protein level on odour and ammonia emissions from finishing pig houses. Bioresour. Technol. 91, 309–315. doi: 10.1016/S0960-8524(03)00184-6
Heo K., Odle J., Han I. K., Cho W., Seo S., van Heugten E., et al. (2000). Dietary l-carnitine improves nitrogen utilization in growing pigs fed low energy, fat containing diets. J. Nutr. 130, 1809–1814. doi: 10.1093/jn/130.7.1809
Heyer C. M. E., Jaworski N. W., Page G. I., Zijlstra R. T. (2022). Effect of fiber fermentation and protein digestion kinetics on mineral digestion in pigs. Animals 12, 2053. doi: 10.3390/ani12162053
Hill G. M., Baido S. K., Cromwell G. L., Mahan D. C., Nelssen J. L., Stein H. H. (2007). Evaluation of sex and lysine during the nursery period. J. Anim. Sci. 85, 1453–1458. doi: 10.2527/jas.2006-402
Hoffman L. A., Ivers D. J., Ellersieck M. R., Veum T. L. (1993). The effect of l-carnitine and soybean oil on performance and nitrogen and energy utilization by neonatal and young pigs. J. Anim. Sci. 71, 132–138. doi: 10.2527/1993.711132x
Htoo J. K., Meng X., Patience J. F., Dugan M. E. R., Zijlstra R. T. (2008). Effects of coextrusion of flaxseed and field pea on the digestibility of energy, ether extract, fatty acids, protein, and amino acids in grower-finisher pigs. J. Anim. Sci. 86, 2942–2951. doi: 10.2527/jas.2007-0313
Ivanovis J., Pantic S., Dokmanovic M., Glamoclija N., Markovic R., Janjic J., et al. (2015). Effect of conjugated linoleic acids in pig nutrition on quality of meat. Proc. Food Sci. 5, 105–108. doi: 10.1016/j.profoo.2015.09.029
Jacela J. Y., Dritz S. S., DeRouchey J. M., Tokach M. D., Goodband R. D., Nelssen J. L. (2010). Effects of supplemental enzymes in diets containing distillers dried grains with solubles on finishing pig performance. Prof. Anim. Sci. 26, 412–424. doi: 10.15232/S1080-7446(15)30623-9
Jackson A. A., Picou D., Landman J. (1984). The non-invasive measurement of urea kinetics in normal man by constant infusion of 15N15N-urea. Hum. Nutr. Clin. Nutr. 38, 339–354. doi: 10.1093/jn/123.12.2129
James P. S., Smith M. W. (1976). Methionine transport by pig colonic mucosa measured during early post-natal development. J. Physiol. 262, 151–168. doi: 10.1113/jphysiol.1976.sp011590
Jang J.-C., Zeng Z., Urriola P. E., Shurson G. C. (2021). Effects of feeding corn distillers dried grains with solubles diets with and without supplemental enzymes on growth performance of pigs: a meta-analysis. Transl. Anim. Sci. 5, 1–15. doi: 10.1093/tas/txab029
Jansman A., Smink W., Van Leeuwen P., Rademacher M. (2002). Evaluation through literature data of the amount and amino acid composition of basal endogenous crude protein at the terminal ileum of pigs. Anim. Feed Sci. Tech 98, 49–60. doi: 10.1016/S0377-8401(02)00015-9
Jarret G., Cozannet P., Martinez J., Dourmad J. Y. (2011a). Effect of different quality wheat dried distiller's grain solubles (DDGS) in pig diets on composition of excreta and methane production from faeces and slurry. Livest. Sci. 140, 275–282. doi: 10.1016/j.livsci.2011.04.006
Jarret G., Martinez J., Dourmad J. Y. (2011b). Effect of biofuel co-products in pig diets on the excretory patterns of n and c and on the subsequent ammonia and methane emissions from pig effluent. Animal 5, 622–631. doi: 10.1017/S1751731110002041
Jha R., Berrocoso J. F. D. (2016). Dietary fiber and protein fermentation in the intestine of swine and their interactive effects on gut health and on the environment: a review. Anim. Feed Sci. Technol. 212, 18–26. doi: 10.1016/j.anifeedsci.2015.12.002
Jha R., Leterme P. (2012). Feed ingredients differing in fermentable fibre and indigestible protein content affect fermentation metabolites and faecal nitrogen excretion in growing pigs. Animal 6, 603–611. doi: 10.1017/S1751731111001844
Jones C. K., Bergstrom J. R., Tokach M. D., DeRouchey J. M., Goodband R. D., Nelssen J. L., et al. (2010). Efficacy of commercial enzymes in diets containing various concentrations and sources of dried distillers grains with solubles for nursery pigs. J. Anim. Sci. 88, 2084–2091. doi: 10.2527/jas.2009-2109
Kai P., Pedersen P., Jensen J., Hansen M., Sommer S. (2008). A whole-farm assessment of the efficacy of slurry acidification in reducing ammonia emissions. Europ. J. Agron. 28, 148–154. doi: 10.1016/j.eja.2007.06.004
Keller J., Ringseis R., Priebe S., Guthke R., Kluge H., Eder K. (2011). Dietary l-carnitine alters gene expression in skeletal muscle of piglets. Molecul. Nutr. Food Res. 55, 419–429. doi: 10.1002/mnfr.201000293
Kerr B. J., Southern L. L., Bidner T. D., Friesen K. G., Easter R. A. (2003b). Influence of dietary protein level, amino acid supplementation, and dietary energy levels on growing-finishing pigs performance and carcass composition. J. Anim. Sci. 81:3075–3087. doi: 10.2527/2003.81123075x
Kerr B. J., Trabue S. L., Andersen D. S., Van Weelden M. B., Pepple L. M. (2020). Dietary composition and particle size effect on swine manure characteristics and gas emissions. J. Environ. Qual. 49, 1384–1395. doi: 10.1002/jeq2.20112
Kerr B. J., Trabue S. L., van Weelden M. B., Andersen D. S., Pepple L. M. (2018). Impact of narasin on manure composition, microbial ecology, and gas emissions from finishing pigs fed either a corn-soybean meal or a corn-soybean meal-dried distillers grains with solubles diets. J. Anim. Sci. 95, 4030–4036. doi: 10.1093/jas/sky053
Kerr B. J., Trachsel J. M., Bearson B. L., Loving C. L., Bearson S. M. D., Byrne K. A., et al. (2022). Evaluation of digestively resistant or soluble fibers, short- and medium-chain fatty acids, trace minerals, and antibiotics in nonchallenged nursery pigs on performance, digestibility, and intestinal integrity. J. Anim. Sci. 100, 1–19. doi: 10.1093/jas/skac282
Kerr B. J., Weber T. E., Shurson G. C. (2013). Critical evaluation of commercially available enzymes, probiotics, and yeast on apparent nutrient digestion and growth in nursery and finishing pigs fed diets containing 30% dried distillers grains with solubles. Prof. An. Sci. 29, 508–517. doi: 10.15232/S1080-7446(15)30272-2
Kerr B. J., Ziemer C. J., Trabue S. L., Crouse J. D., Parkin T. B. (2006). Manure composition of swine as affected by dietary protein and cellulose concentrations. J. Anim. Sci. 84, 1584–1592. doi: 10.2527/2006.8461584x
Kiarie E., Voth C., Wey D., Zhu C., Vingerhoeds P., Borucki S., et al. (2018). Comparative efficacy of antibiotic growth promoter and benzoic acid on growth performance, nutrient utilization, and indices of gut health in nursery pigs fed corn-soybean meal diet. Can. J. Anim. Sci. 98, 868–874. doi: 10.1139/cjas-2018-0056
Kim I. H., Hancock J. D., Hines R. H., Kim C. S. (1998). Effects of cellulase enzymes and bacterial feed additives on nutritional value of sorghum grain for finishing pigs. Asian Australas. J. Anim. Sci. 11, 538–544. doi: 10.5713/ajas.1998.538
Kim S. W., Hurley W., Wu G., Ji F. (2009a). Ideal amino acid balance for sows during gestation and lactation. J. Anim. Sci. 87, E123–E132. doi: 10.2527/jas.2008-1452
Kim S. W., Knabe D. A., Hong K. J., Easter R. A. (2003). Use of carbohydrases in corn-soybean meal-based nursery diets. J. Anim. Sci. 81, 2496–2504. doi: 10.2527/2003.81102496x
Kim J. C., Mullan B. P., Heo J. M., Hansen C. F., Pluske J. R. (2009b). Decreasing dietary particle size of lupins increases apparent ileal amino acid digestibility and alters fermentation characteristics in the gastrointestinal tract of pigs. Br. J. Nutr. 102, 350–360. doi: 10.1017/S0007114508191231
Knudson B. K., Hogberg M. G., Merkel R. A., Allen R. E., Magee W. T. (1985). Developmental comparisons of boars and barrows: i. growth rate, carcass and muscle characteristics. J. Anim. Sci. 61, 789–796. doi: 10.2527/jas1985.614789x
Kong C., Adeola O. (2014). Invited review – evaluation of amino acid and energy utilization in feedstuff for swine and poultry diets. Asian australas. J. Anim. Sci. 27, 917–925. doi: 10.5713/ajas.2014.r.02
Kornegay E. T., Harper A. F. (1997). Environmental nutrition: nutrient management strategies to reduce nutrient excretion of swine. Prof. Anim. Sci. 13, 99–111. doi: 10.15232/S1080-7446(15)31861-1
Kress K., Millet S., Labussière É., Weiler U., Stefanski V. (2019). Sustainability of pork production with immunocastration in Europe. Sustainability 11, 3335. doi: 10.3390/su11123335
Krick B. J., Roneker K. R., Boyd R. D., Beermann D. H., David P. J., Meisinger D. J. (1992). Influence of genotype and sex on the response of growing pigs to recombinant porcine somatotropin. J. Anim. Sci. 70, 3024–3034. doi: 10.2527/1992.70103024x
Kupper T., Hani C., Neftel A., Kincaid C., Buhler M., Amon B., et al. (2020). Ammonia and greenhouse gas emissions from slurry storage-a review. Agric. Eco. Sys. Environ. 300, 106963. doi: 10.1016/j.agee.2020.106963
Kwon W. B., Soto J. A., Stein H. H. (2020). Effects on nitrogen balance and metabolism of branched-chain amino acids by growing pigs of supplementing isoleucine and valine to diets with adequate or excess concentrations of dietary leucine. J. Anim. Sci. 98, 1–10. doi: 10.1093/jas/skaa346
Kwon W. B., Soto J. A., Stein H. H. (2021). Effects of dietary valine, isoleucine, and tryptophan supplementations to diets containing excess leucine from corn protein on growth performance of growing pigs. J. Anim. Sci. 99, 61–62. doi: 10.1093/jas/skab054.103
Kwon W. B., Soto J. A., Stein H. H. (2022). Effects of dietary leucine and tryptophan on serotonin metabolism and growth performance of growing pigs. J. Anim. Sci. 100, 1–10. doi: 10.1093/jas/skab356
Kwon W. B., Touchette K. J., Simongiovanni A., Syriopoulos K., Wessels A., Stein H. H. (2019). Excess dietary leucine in diets for growing pigs reduces growth performance, biological value of protein, protein retention, and serotonin synthesis. J. Anim. Sci. 97, 4282–4292. doi: 10.1093/jas/skz259
Kyriazakis I. (2011). Opportunities to improve nutrient efficiency in pigs and poultry through breeding. Animal 5, 821–832. doi: 10.1017/S1751731110002545
Lachica M., Montalvo G., Morales J., Fernández-Fígares I. (2019). Effects of betaine and CLA on gas emission of pigs in farm conditions. EAAP Sci. Ser. 138, 165–166. doi: 10.3920/978-90-8686-891-9_23
Lahaye L., Ganier P., Thibault J. N., Riou Y., Sève B. (2008). Impact of wheat grinding and pelleting in a wheat-rapeseed meal diet on amino acid ileal digestibility and endogenous losses in pigs. Anim. Feed Sci. Technol. 141, 287–305. doi: 10.1016/j.anifeedsci.2007.06.016
Lahaye L., Riou Y., Sève B. (2007). The effect of grinding and pelleting of wheat and maize on amino acids true ileal digestibility and endogenous losses in growing pigs. Livest. Sci. 109, 138–140. doi: 10.1016/j.livsci.2007.01.123
Latimier P., Dourmad J. Y., Corlouer A., Chauvel J., le Pan J., Gautier M., et al. (1993). Effect of three protein feeding strategies, for growing-finishing pigs, on growth performance and nitrogen output in the slurry. J. Rech. Porcine en France 25, 295–300.
Lauridsen C., Mu H., Henckel P. (2005). Influence of dietary conjugated linoleic acid (CLA) and age at slaughtering on performance, slaughter- and meat quality, lipoproteins, and tissue deposition of CLA in barrows. Meat Sci. 69, 393–399. doi: 10.1016/j.meatsci.2004.08.009
Lautrou M., Cappelaere L., Montminy M.-P. L. (2022). Phosphorus and nitrogen nutrition in swine production. Anim. Front. 12, 23–29. doi: 10.1093/af/vfac068
Le P. D., Aarnink A. J. A., Jongbloed A. W. (2009). Odour and ammonia emission from pig manure as affected by dietary crude protein level. Livest. Sci. 121, 267–274. doi: 10.1016/j.livsci.2008.06.021
Le P. D., Aarnink A. J. A., Jongbloed A. W., van der Peet-Schwering C. M. C., Ogink N. W. M., Verstegen M. W. A. (2008). Interactive effects of dietary crude protein and fermentable carbohydrate levels on odour from pig manure. Livest. Sci. 114, 48–61. doi: 10.1016/j.livsci.2007.04.009
Lealiifano A. K., Pluske J. R., Nicholls R. R., Dunshea F. R., Campbell R. G., Hennessy D. P., et al. (2011). Reducing the length of time between slaughter and the secondary gonadotropin-releasing factor immunization improves growth performance and clears boar taint compounds in male finishing pigs. J. Anim. Sci. 89, 2782–2792. doi: 10.2527/jas.2010-3267
LEAP (2015). Environmental performance of animal feed supply chains: guidelines for assessment (Rome, Italy: Livest. Environ. Assess. Perform. Partnership, Food and Agriculture Organization).
Leek A. B. G., Callan J. J., Henry R. W., O’Doherty J. V. (2005). The application of low crude protein wheat-soyabean diets to growing and finishing pigs: 2. the effects on nutrient digestibility, nitrogen excretion, faecal volatile fatty acid concentration and ammonia emission from boars. Ir. J. Agric. Food Res. 44, 247–260. Available at: http://www.jstor.org/stable/25562551.
Leek A. B. G., Hayes E. T., Curran T. P., Callan J. J., Beattie V. E., Dodd V. A., et al. (2007). The influence of manure composition on emissions of odour and ammonia from finishing pigs fed different concentrations of dietary crude protein. Bioresour. Technol. 98, 3431–3439. doi: 10.1016/j.biortech.2006.11.003
Lekagul A., Tangcharoensathien V., Yeung. S. (2019). Patterns of antibiotic use in global pig production: a systematic review. Vet. Anim. Sci. 7, 100058. doi: 10.1016/j.vas.2019.100058
Lewis L. L., Stark C. R., Fahrenholz A. C., Goncalves M. A. D., DeRouchey J. M., Jones C. K. (2015). Effects of pelleting conditioner retention time on nursery pig growth performance. J. Anim. Sci. 93, 1098–1102. doi: 10.2527/jas.2014-8072
Li W., Powers W., Hill G. M. (2011). Feeding distillers dried grains with solubles and organic trace mineral sources to swine and the resulting effect on gaseous emissions. J. Anim. Sci. 89, 3286–3299. doi: 10.2527/jas.2010-3611
Li S., Sauer W. C., Mosenthin R., Kerr B. (1996). Effect of beta-glucanase supplementation of cereal-based diets for starter pigs on the apparent digestibilities of dry matter, crude protein and energy. Anim. Feed Sci. Technol. 59, 223–231. doi: 10.1016/0377-8401(95)00909-4
Li Q. F., Trottier N., Powers W. (2015). Feeding reduced crude protein diets with crystalline amino acids supplementation reduce air gas emissions from housing. J. Anim. Sci. 93, 721–730. doi: 10.2527/jas.2014-7746
Lin G., Wang X., Wu G., Feng C., Zhou H., Li D., et al. (2014). Improving amino acid nutrition to prevent intrauterine growth restriction in mammals. Amino Acids 46, 1605–1623. doi: 10.1007/s00726-014-1725-z
Liu S., Ni J. Q., Radcliffe J. S., Vonderohe C. E. (2017). Mitigation of ammonia emission from pig production using reduced dietary crude protein with amino acid supplementation. Bioresour. Technol. 233, 200–208. doi: 10.1016/j.biortech.2017.02.082
Liu P., Souza L. W. O., Baidoo S. K., Shurson G. C. (2012). Impact of distillers dried grains with solubles particle size on nutrient digestibility, DE and ME content, and flowability in diets for growing pigs. J. Anim. Sci. 90, 4925–4932. doi: 10.2527/jas.2011-4604
Liu L., Zhang X., Xu W., Liu X., Li Y., Wei J., et al. (2020). Challenges for global sustainable nitrogen management in agricultural systems. J. Agricult. Food Chem. 68, 3354–3361. doi: 10.1021/acs.jafc.0c00273
Lloyd L. E., McDonald B. E., Crampton E. W. (1978). Fundamentals of nutrition. 2nd Ed (San Francisco, CA: W.H. Freeman and Company).
Lowe B. K., Gerlemann G. D., Carr S. N., Rincker P. J., Schroeder A. L., Petry D. B., et al. (2014). Effects of feeding ractopamine hydrochloride (Paylean) to physical and immunological castrates (Improvest) in a commercial setting on carcass cutting yields and loin quality. J. Anim. Sci. 92, 3715–3726. doi: 10.2527/jas.2013-7515
Lynch M. B., Sweeney T., Callan J. J., Flynn B., O’Doherty (2007). The effect of high and low dietary crude protein and inulin supplementation on nutrient digestibility, nitrogen excretion, intestinal microflora and manure ammonia emissions from finisher pigs. Animal 1, 1112–1121. doi: 10.1017/S1751731107000407
Lynegaard J. C., Hansen C. F., Kristensen A. R., Amdi C. (2020). Body composition and organ development of intra-uterine growth restricted pigs at weaning. Animal 14, 2. doi: 10.1017/S175173111900171X
Mackenzie S. G., Leinonen I., Ferguson N., Kyriazakis I. (2016). Towards a methodology to formulate sustainable diets for livestock: accounting for environmental impact in diet formulation. Br. J. Nutr. 115, 1860–1874. doi: 10.1017/S0007114516000763
MacLeod M., Gerber P., Mottet A., Tempio G., Falcucci A., Opio C., et al. (2013). Greenhouse gas emissions from pig and chicken supply chains – a global life cycle assessment (Rome: Food and Agriculture Organization of the United Nations (FAO).
Maron D. F., Smith T. J., Nachman K. E. (2013). Restrictions on antimicrobial use in food animal production: an international regulatory and economic survey. Globalization Health 9, 48. doi: 10.1186/1744-8603-9-48
Martínez-Aispuro M., Figueroa-Velasco J. L., Zamora-Zamora V., Cordero-Mora J. L., Narciso-Gaytán, Sánchez-Torres M. T., et al. (2014). Effect of CLA supplementation to low-protein diets on the growth performance, carcass characteristics, plasma urea nitrogen concentration, and fatty acid profile in the meat of pigs. Braz. Arch. Biol. Technol. 57, 742–754. doi: 10.1590/S1516-8913201401407
Matheson S. M., Walling G. A., Edwards S. A. (2018). Genetic selection against intrauterine growth retardation in piglets: a problem at the piglet level with a solution at the sow level. Genet. Sel. Evol. 50, 46. doi: 10.1186/s12711-018-0417-1
Matyba P., Florowski T., Dasiewicz K., Ferenc K., Olszewski J., Trela M., et al. (2021). Performance and meat quality of intrauterine growth restricted pigs. Animals 11, 254. doi: 10.3390/ani11020254
Mavromichalis I., Hancock J. D., Senne B. W., Gugle T. L., Kennedy G. A., Hines R. H., et al. (2000). Enzyme supplementation and article size of wheat in diets for nursery and finishing pigs. J. Anim. Sci. 78, 3086–3095. doi: 10.2527/2000.78123086x
McAuliffe G. A., Chapman D. V., Sage C. L. (2016). A thematic review of life cycle assessment (LCA) applied to pig production. Environ. Impact Assess. Rev. 56, 12–22. doi: 10.1016/j.eiar.2015.08.008
McCauley I., Watt M., Suster D., Kerton D. J., Oliver W. T., Harrell R. J., et al. (2003). A GnRF vaccine (Improvac®) and porcine somatotropin (Reporcin®) have synergistic effects upon growth performance in both boars and gilts. Aust. J. Agric. Res. 54, 11–20. doi: 10.1071/AR02037
McGlone J. J., Hellman J. M. (1988). Local and general anesthetic effects on behavior and performance of two- and seven-week-old castrated and uncastrated piglets. J. Anim. Sci. 66, 3049–3058. doi: 10.2527/jas1988.66123049x
McGlone J. J., Nicholson R. I., Hellman J. M., Herzog D. N. (1993). The development of pain in young pigs associated with castration and attempts to prevent castration -induced behavioral changes. J. Anim. Sci. 71, 1441–1446. doi: 10.2527/1993.7161441x
Medel P., Garcia M., Lazaro R., de Blas C., Mateos G. G. (2000). Particle size and heat treatment of barley in diets for early-weaned piglets. Anim. Feed Sci. Technol. 84, 13–21. doi: 10.1016/S0377-8401(00)00111-5
Meier-Dinkel L., Trautmann J., Frieden L., Tholen E., Knorr C., Sharifi A. R., et al. (2013). Consumer perception of boar meat as affected by labelling information, malodorous compounds and sensitivity to androstenone. Meat Sci. 93, 248–256. doi: 10.1016/jmeatsci.2012.09.002
Messad F., Letourneau-Montminy M. P., Charbonnear E., Sauvant D., Guay F. (2016). Meta-analysis of the amino acid digestibility of oilseed meal in growing pigs. Animal 10, 1635–1644. doi: 10.1017/S1751731116000732
Mikel W. B., Althen T. G., Rogers R. W., Moore A. B., Miller H. W., Miller L. F. (1993). Effects of exogenous porcine somatotropin on the carcass composition, hormonal and metabolic profiles, lipogenic capacity, and binding of insulin to erythrocyte receptors of fast- versus slow-growing swine. J. Anim. Sci. 71, 1786–1795. doi: 10.2527/1993.7171786x
Mikesell R. E., Kephart K. B. (1999). Effect of grouping arrangement on behavior and performance of finishing pigs. Livest. Prod. Sci. 57, 291–294. doi: 10.1016/S0301-6626(98)00179-1
Millet S., Aluwé M., van den Broeke A., Leen F., De Boever J., De Campeneere S. (2018). Review: pork production with maximal nitrogen efficiency. Animal 12, 1060–1067. doi: 10.1017/S1751731117002610
Moehn S., Ball R. O., Fuller M. F., Gillis A. M., de Lange C. F. M. (2004). Growth potential, but not body weight or moderate limitation of lysine intake, affects inevitable lysine catabolism in growing pigs. J. Nutr. 134, 2287–2292. doi: 10.1093/jn/134.9.2287
Monteiro A. N. T. R., Bertol T. M., de Oliveria P. A. V., Dourmad J. Y., Coldebella A., Kessler A. M. (2017). The impact of feeding growing-finishing pigs with reduced dietary protein levels on performance, carcass traits, meat quality and environmental impacts. Livest. Sci. 198, 162–169. doi: 10.1016/j.livsci.2017.02.014
Monteiro A. N. T. R., Brossard L., Gilbert H., Dourmad J.-Y. (2021). Environmental impacts and their association with performance and excretion traits in growing pigs. Front. Vet. Sci. 8. doi: 10.3389/fvets.2021.677857
Monteiro A. N. T. R., Garcia-Launay F., Brossard L., Wilfart A., Dourmad J.-Y. (2016). Effect of feeding strategy on environmental impacts of pig fattening in different contexts of production: evaluation through life cycle assessment. J. Anim. Sci. 94, 4832–4837. doi: 10.2527/jas.2016-0529
Moore K. L., Dunshea F. R., Mullan B. P., Hennessy D. P., D’Souza D. N. (2009). Ractopamine supplementation increases lean deposition in entire and immunocastrated male pigs. Anim. Prod. Sci. 49, 1113–1119. doi: 10.1071/AN09076
Morel P. C., Janz J. A., Zou M., Purchas R. W., Hendriks W. H., Wilkinson B. H. (2008). The influence of diets supplemented with conjugated linoleic acid, selenium, and vitamin e, with or without animal protein, on the composition of pork from female pigs. J. Anim. Sci. 86, 1145–1155. doi: 10.2527/jas.2007-0358
Mosenthin R., Sauer W. C., de Lange C. F. M. (1992). Trace studies of urea kinetics in growing pigs: i. the effect of intravenous infusion of urea on urea recycling and the site of urea secretion into the gastrointestinal tract. J. Anim. Sci. 70, 3458–3466. doi: 10.2527/1992.70113458x
Moughan P. J. (1999). “Protein metabolism in the growing pig,” in Quantitative biology of the pig. Ed. Kyriazakis I. (Wallingford, UK: CABI), 299–331.
Mroz Z., Moeser A. J., Vreman K., van Diepen J. T. M., van Kempen T., Canh T. T., et al. (2000). Effects of dietary carbohydrates and buffering capacity on nutrient digestibility and manure characteristics in finishing pigs. J. Anim. Sci. 78, 3096–3106. doi: 10.2527/2000.78123096x
Muley N. S., van Heugten E., Moeser A. J., Rausch K. D., van Kempen T. A. T. G. (2007). Nutritional value for swine of extruded corn and corn fractions obtained after dry milling. J. Anim. Sci. 85, 1695–1701. doi: 10.2527/jas.2006-127
Müller H. L., Kirchgessner M., Roth F. X., Stangl G. I. (2001). Effect of conjugated linoleic acid on energy metabolism in growing-finsihing pigs. Anim. Physiol. Anim. Nutr. 83, 85–94. doi: 10.1046/j.1439-0396.2000.00253.x
NANP (2023) National animal nutrition program. Available at: https://animalnutrition.org/.
Ni J. Q., Shi C., Liu S., Richert B. T., Vonderohe C. E., Radcliffe J. S. (2019). Effects of antibiotic-free pig rearing on ammonia emissions from five pairs of swine rooms in a wean-to-finish experiment. Environ. Int. 131, 104931. doi: 10.1016/j.envint.2019.104931
Noblet J., Le Bellego L., van Milgen J., Dubois S. (2001). Effects of reduced dietary protein level and fat addition on heat production and nitrogen and energy balance in growing pigs. Anim. Res. 50, 227–238. doi: 10.1051/ANIMRES:2001129
Noel S. J., Jørgensen H. J. H., Bach Knudsen K. E. (2021). Prediction of protein and amino acid composition and digestibility in individual feedstuffs and mixed diets for pigs using near-infrared spectroscopy. Anim. Nutr. 7, 1242–1252. doi: 10.1016/j.aninu.2021.07.004
NRC (2012). Nutrient requirements of swine. 11th rev (Washington, DC, (USA: National Academy Press).
Nyachoti C. M., Omogbenigun F. O., Rademacher M., Blank G. (2006). Performance responses and indicators of gastrointestinal health in early-weaned pigs fed low-protein amino acid-supplemented diets. J. Anim. Sci. 84, 125–134. doi: 10.2527/2006.841125x
Oksbjerg N., Petersen J. S., Sorensen I. L., Henckel P., Vestergaard M., Ertbjerg P., et al. (2000). Long-term changes in performance and meat quality of Danish landrace pigs: a study on a current compared with an unimproved genotype. Anim. Sci. 71, 81–92. doi: 10.1017/S1357729800054916
Oliver W. T., McCauley I., Harrell R. J., Suster D., Kerton D. J., Dunshea F. R. (2003). A gonadotropin-releasing factor vaccine (Improvac) and porcine somatotropin have synergistic and additive effects on growth performance in group-housed boars and gilts. J. Anim. Sci. 81, 1959–1966. doi: 10.2527/2003.8181959x
Omogbenigun F. O., Nyachoti C. M., Slominski B. A. (2004). Dietary supplementation with multienzyme preparations improves nutrient utilization and growth performance in weaned pigs. J. Anim. Sci. 82, 1053–1061. doi: 10.2527/2004.8241053x
Onyango E. M., Asem E. K., Adeola O. (2009). Phytic acid increases mucin and endogenous amino acid losses from the gastrointestinal tract of chickens. Br. J. Nutr. 101, 836–842. doi: 10.1017/S0007114508047740
Oryschak M. A., Simmins P. H., Zijlstra R. T. (2002). Effect of dietary particle size and carbohydrase and/or phytase supplementation on nitrogen and phosphorus excretion of grower pigs. Can. J. Anim. Sci. 82, 533–540. doi: 10.4141/A02-016
Osada T., Takada R., Shinzato I. (2011). Potential reduction of greenhouse gas emission from swine manure by using a low-protein diet supplemented with synthetic amino acids. Anim. Feed Sci. Technol. 168-167, 562–574. doi: 10.1016/j.anifeedsci.2011.04.079
Ostrowska E., Muralitharan M., Cross R. F., Bauman D. E., Dunshea F. R. (1999). Dietary conjugated linoleic acids increase lean tissue and decrease fat deposition in growing pigs. J. Nutr. 129, 2037–2042. doi: 10.1093/jn/129.11.2037
Owen K. Q., Jit H., Maxwell C. V., Nelssen J. L., Goodband R. D., Tokach M. D., et al. (2001a). Dietary l-carnitine suppresses mitochondrial branched keto acid dehydrogenase activity and enhances protein accretion and carcass characteristics of swine. J. Anim. Sci. 79, 3104–3112. doi: 10.2527/2001.79123104x
Owen K. Q., Kim I. H., Kim C. S. (1997). The role of l-carnitine in swine nutrition and metabolism. Kor. J. Anim. Nutr. Feed. 21, 41–58.
Owen K. Q., Nelssen J. L., Goodband R. D., Tokach M. D., Friesen K. G. (2001b). Effect of l-carnitine on growth performance and body composition in nursery and growing finishing pigs. J. Anim. Sci. 79, 1509–1515. doi: 10.2527/2001.7961509x
Owen K. Q., Nelssen J. L., Goodband R. D., Weeden T. L., Blum S. A. (1996). Effect of l-carnitine and soybean oil on growth performance and body composition of early-weaned pigs. J. Anim. Sci. 74, 1612–1619. doi: 10.2527/1996.7471612x
Owusu-Asiedu A., Baidoo S., Nyachoti C. (2002). Effect of heat processing on nutrient digestibility in pea and supplementing amylase and xylanase to raw, extruded or micronized pea-based diets on performance of early-weaned pigs. Can. J. Anim. Sci. 82, 367–374. doi: 10.4141/A01-051
Pariza M. W., Park Y., Cook E. M. (2000). Mechanisms of action of conjugated linoleic acid: evidence and speculation. Proc. Soc Exp. Biol. Med. 223, 8–13. doi: 10.1046/j.1525-1373.2000.22302.x
Park Y., Albright K. J., Storkson J. M., Cook M. E., Liu W., Pariza M. W. (1999). Changes in body composition in mice during feeding and withdrawal of conjugated linoleic acid. Lipids 34, 243–248. doi: 10.1007/s11745-999-0359-7
Park Y., Albright K. J., Storkson J. M., Cook M. E., Pariza M. W. (1997). Effects of conjugated linoleic acid on body composition in mice. Lipids 32, 853–858. doi: 10.1007/s11745-997-0109-x
Park J. S., Kim I. H., Hancock J. D., Hines R. H., Cobb C., Cao H., et al. (2003). Effects of amylase and cellulase supplementation in sorghum-based diets for finishing pigs. J. Anim. Sci. 16, 70–76. doi: 10.5713/ajas.2003.70
Park C. S., Oh S. I., Kim B. G. (2013). Prediction of basal endogenous losses of amino acids based on body weight and feed intake in pigs fed nitrogen-free diets. Rev. Colomb. Cienc. Pecu. 26, 186–192. Available at: http://www.scielo.org.co/scielo.php?script=sci_arttext&pid=S0120-06902013000300005&lng=en&nrm=iso.
Park Y., Pariza M. W. (2007). Mechanisms of body fat modulation by conjugated linoleic acid (CLA). Food Res. Int. 40, 311–323. doi: 10.1016/j.foodres.2006.11.002
Paulk C. B., Hancock J. D. (2016). Effects of an abrupt change between diet form on growth performance of finishing pigs. Anim. Feed Sci. Technol. 211, 132–136. doi: 10.1016/j.anifeedsci.2015.10.017
Pauly C., Spring P., O’Doherty J. V., Ampuero Kragten S., Bee G. (2009). Growth performance, carcass characteristics and meat quality of group-penned surgically castrated, immunocastrated (Improvac®) and entire male pigs and individually penned entire male pigs. Animal 3, 1057–1066. doi: 10.1017/S1751731109004418
Pettey L. A., Carter S. D., Senne B. W., Shriver J. A. (2002). Effects of beta-mannanase addition to corn-soybean meal diets on growth performance, carcass traits, and nutrient digestibility of weanling and growing-finishing pigs. J. Anim. Sci. 80, 1012–1019. doi: 10.2527/2002.8041012x
Pfeiffer A. (1993). Protein reduced feeding concepts, a contribution to reduced ammonia emissions in pig fattening. Zuchtungskunde 65, 431–443.
Philippe F. X., Laitat M., Canart B., Farnir F., Massart L., Vandenheede M., et al. (2006). Effects of a reduction of diet crude protein content on gaseous emissions from deep-litter pens for fattening pigs. Anim. Res. 55, 397–407. doi: 10.1051/animres:2006029
Philippe F. X., Laitat M., Wavreille J., Nicks B., Cabaraux J. F. (2015). Effects of high-fibre diet on ammonia and greenhouse gas emissions from gestating sows and fattening pigs. Atmos. Environ. 109, 197–204. doi: 10.1016/j.atmosenv.2015.03.025
Pi Y., Gao K., Peng Y., Mu C. L., Zhu W. Y. (2019). Antibiotic-induced alterations of the gut microbiota and microbial fermentation in protein parallel the changes in host nitrogen metabolism of growing pigs. Animal 13, 2. doi: 10.1017/S1751731118001416
Pietruszka A., Jacyno E., Kolodziej A., Kawecka M., Elzanowski C., Matysiak B. (2009). Effects of l-carnitine and iron diet supplementation on growth performance, carcass characteristics and blood metabolites in fattening pigs. Agric. Food Sci. 18, 27–34. doi: 10.2137/145960609788066816
Pinelli-Saavedra A., González-Ríos H., Dávila-Ramírez J. L., Islava-Lagarda T. Y., Esquerra-Brauer I. R. (2019). Dietary conjugated linoleic acid (CLA) has comparable effects to ractopamine on growth performance, meat quality and fatty acid profiles of loin muscles of finishing pigs under commercial husbandry. Ital. J. Anim. Sci. 18, 713–722. doi: 10.1080/1828051X.2019.1568839
Pomar C., Andretta I., Remus A. (2021). Feeding strategies to reduce nutrient losses and improve sustainability of growing pigs. Front. Vet. Sci. 8. doi: 10.3389/fvets.2021.742220
Pomar C., Remus A. (2019). Precision pig feeding: a breakthrough toward sustainability. Anim. Front. 9, 52–59. doi: 10.1093/af/vfz006
Pompeu D., Wiegand B. R., Evans H. L., Rickard J. W., Gerlemann G. D., Hinson R. B., et al. (2013). Effect of corn dried distillers grains with solubles, conjugated linoleic acid, and ractopamine (paylean) on growth performance and fat characteristics of late finishing pigs. J. Anim. Sci. 91, 793–803. doi: 10.2527/jas.2012-5257
Portejoie S., Dourmad J. Y., Martinez J., Lebreton Y. (2004). Effect of lowering dietary crude protein on nitrogen excretion, manure composition and ammonia emission from fattening pigs. Livest. Prod. Sci. 91, 45–55. doi: 10.1016/j.livprodsci.2004.06.013
Powers W. J., Zamzow S. B., Kerr B. J. (2007). Reduced crude protein effects on aerial emissions from swine. App. Eng. Agric. 23, 539–546. doi: 10.13031/2013.23487
Puiman P., Stoll B., Mølbak L., de Bruijn A., Schierbeek H., Boye M., et al. (2013). Modulation of the gut microbiota with antibiotic treatment suppresses whole body urea production in neonatal pigs. Am. J. Physiol. Gastrointest. Liver Physiol. 304, G300–G310. doi: 10.1152/ajpgi.00229.2011
Puls C. L., Rojo A., Ellis M., Boler D. D., McKeith F. K., Killefer J., et al. (2014). Growth performance of immunologically castrated (with improvest) barrows (with or without ractopamine) compared to gilt, physically castrated barrow, and intact male pigs. J. Anim. Sci. 92, 2289–2295. doi: 10.2527/jas.2013-6861
Quiniou N., Noblet J. (1995). Prediction of tissular body-composition from protein and lipid deposition in growing pigs. J. Anim. Sci. 73, 1567–1575. doi: 10.2527/1995.7361567x
Rault J. L., Lay D. C. Jr., Marchant-Forde J. N. (2011). Castration-induced pain in pigs and other livestock. Appl. Anim. Behav. Sci. 135, 214–225. doi: 10.1016/j.applanim.2011.10.017
Rickard-Bell C., Curtis M. A., van Barneveld R. J., Mullan B. P., Edwards A. C., Gannon N. J., et al. (2009). Ractopamine hydrochloride improves growth performance and carcass composition in immunocastrated boars, intact boars, and gilts. J. Anim. Sci. 87, 3536–3543. doi: 10.2527/jas.2009-2002
Ringseis R., Keller J., Eder K. (2018). Basic mechanisms of the regulation of l-carnitine status in monogastrics and efficacy of l-carnitine as a feed additive in pigs and poultry. J. Anim. Physiol. Anim. Nutr. 102, 1686–1719. doi: 10.1111/jpn.12959
Rinker M. J., Carter S. D., Real D. E., Nelssen J. L., Tokach M. D., Goodband R. D., et al. (2003). Effects of increasing dietary l-carnitine on growth performance of weanling pigs. J. Anim. Sci. 81, 2259–2269. doi: 10.2527/2003.8192259x
Roca J., Parrilla I., Rodriguez-Martinez H., Gil M. A., Cuello C., Vazquez J. M., et al. (2011). Approaches towards efficient use of boar semen in the pig industry. Reprod. Domest. Anim. 46 (Suppl. 2), 79–83. doi: 10.1111/j.1439-0531.2011.01828.x
Roche H. M., Noone E., Nugent A., Gibney M. J. (2001). Conjugated linoleic acid: a novel therapeutic nutrient? Nutr. Res. Rev. 14, 173–187. doi: 10.1079/NRR200122
Rockström J., Steffen W., Noone K., Persson Å., Chapin F. S., Lambin E. F., et al. (2009). A safe operating space for humanity. Nature 461, 472–475. doi: 10.1038/461472a
Rojas O. J., Stein H. H. (2015). Effects of reducing the particle size of corn grain on the concentration of digestible and metabolizable energy and on the digestibility of energy and nutrients in corn grain fed to growing pigs. Livest. Sci. 181, 187–193. doi: 10.1016/j.livsci.2015.09.013
Rojas O. J., Stein H. H. (2017). Processing of ingredients and diets and effects on nutritional value for pigs. J. Anim. Sci. Biotechnol. 8, 48. doi: 10.1186/s40104-017-0177-1
Rojas O. J., Vinyeta E., Stein H. H. (2016). Effects of pelleting, extrusion, or extrusion and pelleting on energy and nutrient digestibility of diets containing different levels of fiber and fed to growing pigs. J. Anim. Sci. 94, 1951–1960. doi: 10.2527/jas.2015-0137
Rojas-Cano M., Lara L., Lachia M., Aguilera J. F., Fernández-Fígares I. (2011). Influence of betaine and conjugated linoleic acid on development of carcass cuts of Iberian pigs growing from 20 to 50 kg body weight. Meat Sci. 88, 525–530. doi: 10.1016/j.meatsci.2011.02.004
Romanelli A., Dieguez S. N., Soraci A. L. (2019). Dietary amino acid supplementation and its relationship with placental and fetal growth in pigs. J. Anim. Vet. Adv. 18, 215–226. doi: 10.36478/java.2019.215.226
Rooke J. A., Slessor M., Fraser H., Thomson J. R. (1998). Growth performance and gut function of piglets weaned at four weeks of age and fed protease-treated soya-bean meal. Anim. Feed Sci. Technol. 70, 175–190. doi: 10.1016/S0377-8401(97)00083-7
Roth F. X., Kirchgessner M. (1993). Influence of avilamycin and tylosin on retention and excretion of nitrogen in growing pigs. J. Anim. Physiol. Anim. Nutr. 69, 175–185. doi: 10.1111/j.1439-0396.1993.tb00803.x
Saintilan R., Mérour I., Brossard L., Tribout T., Dourmad J. Y., Sellier P., et al. (2013). Genetics of residual feed intake in growing pigs: relationships with production traits, and nitrogen and phosphorus excretion traits. J. Anim. Sci. 91, 2542–2554. doi: 10.2527/jas2012-5687
Salazar-Villanea S., Hendriks W. H., Bruininx E. M. A. M., Gruppen H., van der Poel A. F. B. (2016). Protein structural changes during processing of vegetable feed ingredients used in swine diets: implications for nutritional value. Nutr. Res. Rev. 29, 126–141. doi: 10.1017/S0954422416000056
Salazar-Villanea S., Bruininx E. M. A. M., Gruppen H., Hendriks W. H., Carré P., Quinsac A., et al. (2018). Pelleting and extrusion can ameliorate negative effects of toasting of rapeseed meal on protein digestibility in growing pigs. Animal 12, 950–958. doi: 10.1017/S1751731117002476
Salyer J. A., Tokach M. D., DeRouchey J. M., Dritz S. S., Goodband R. D., Nelssen J. L. (2013). Effects of standardized ileal digestible tryptophan:lysine in diets containing 30% dried distillers grains with solubles on finishing pig performance and carcass traits. J. Anim. Sci. 91, 3244–3252. doi: 10.2527/jas.2012-5502
Sauer W. C., Mosenthin R., Pierce A. B. (1990). The utilization of pelleted, extruded, and extruded and repelleted diets by early weaned pigs. Anim. Feed Sci. Technol. 31, 269–275. doi: 10.1016/0377-8401(90)90131-Q
Schinckel A. P., de Lange C. F. M. (1996). Characterization of growth parameters needed as inputs for pig growth models. J. Anim. Sci. 74, 2021–2036. doi: 10.2527/1996.7482021x
Schinckel A. P., Einstein M. E., Jungst S., Matthews J. O., Booher C., Dreadin T., et al. (2012). Daily feed intake, energy intake, growth rate and measures of dietary energy efficiency of pigs from four sire lines fed diets with high or low metabolizable and net energy concentrations. Asian-Aust. J. Anim. Sci. 25, 410–420. doi: 10.5713/ajas.2011.11212
Schinckel A. P., Li N., Richert B. T., Preckel P. V., Einstein M. E. (2003). Development of a model to describe the compositional growth and dietary lysine requirements of pigs fed ractopamine. J. Anim. Sci. 81, 1106–1119. doi: 10.2527/2003.8151106x
Schinckel A. P., Mahan D. C., Wiseman T. G., Einstein M. E. (2008). Growth of protein, moisture, lipid, and ash of two genetic lines of barrows and gilts from twenty to one hundred twenty-five kilograms of body weight. J. Anim. Sci. 86, 460–471. doi: 10.2527/jas.2007-0625
Schmid A., Collomb M., Sicher R., Bee G. (2006). Conjugated linoleic acid in meat and meat products: a review. Meat Sci. 73, 29–41. doi: 10.1016/j.meatsci.2005.10.010
Selle P. H., Ravindran V. (2008). Phytate-degrading enzymes in pig nutrition. Livest. Sci. 113, 99–122. doi: 10.1016/j.livsci.2007.05.014
Selle P. H., Cowieson A. J., Cowieson N. P., Ravindran V. (2012). Protein-phytate interactions in pig and poultry nutrition-a reappraisal. Nutr. Res. Rev. 25, 1–17. doi: 10.1017/S0954422411000151
Shimizu M. (2004). Food-derived peptides and intestinal functions. Biofactors 21, 43–47. doi: 10.1002/biof.552210109
Shirali M., Duthie C.-A., Doeschl-Wilson A., Knap P. W., Kanis E., Arendonk J. A. M., et al. (2013). Novel insight into the genomic architecture of feed and nitrogen efficiency measured by residual energy intake and nitrogen excretion in growing pigs. BMC Genet. 14, 121. doi: 10.1186/1471-2156-14-121
Shriver J. A., Carter S. D., Sutton A. L., Richert B. T., Senne B. W., Pettey L. A. (2003). Effects of adding fiber sources to reduced crude protein, amino acid-supplemented diets on nitrogen excretion, growth performance, and carcass traits of finishing pigs. J. Anim. Sci. 81, 492–502. doi: 10.2527/2003.812492x
Shurson G. C., Hung Y.-T., Jang J. C., Urriola P. E. (2021). Measures matter – determining the true nutri-physiological value of feed ingredients for swine. Animals 11, 1259. doi: 10.3390/ani11051259
Shurson G. C., Pelton R. E. O., Yang Z., Urriola P. E., Schmitt J. (2022). Environmental impacts of eco-nutrition swine feeding programs in spatially explicit geographic regions of the united states. J. Anim. Sci. 100, 1–7. doi: 10.1093/jas/skac356
Shurson G. C., Urriola P. E. (2022). Sustainable swine feeding programs require the convergence of multiple dimensions of circular agriculture and food systems with one health. Anim. Front. 12, 30–40. doi: 10.1093/af/vfac077
Skerman A., Willis S., Tait S., Yap S. D. (2016). “Effect of feed wastage on piggery manure characteristics and methane potential,” in Australian Pork limited (St. Lucia, Queensland, Australia: Department of Agriculture, and Advanced Water Management Centre at the University of Queensland). 51 pp.
Smith M. W., James P. S. (1976). Amino acid transport by the helicoidal colon of the new-born pig. Biochim. Biophys. Acta 419, 391–394. doi: 10.1016/0005-2736(76)90366-7
Squires E. J., Adeola O., Young L. G., Hacker R. R. (1993). The role of growth hormones, b-adrenergic agents and intact males in pork production: a review. Can. J. Anim. Sci. 73, 1–23. doi: 10.4141/cjas93-001
Squires E. J., Bone C., Cameron J. (2020). Pork production with entire males: directions for control of boar taint. Animals 10, 1665. doi: 10.3390/ani10091665
Steidinger M. U., Goodband R. D., Tokach M. D., Dritz S. S., Nelssen J. L., McKinney L. J., et al. (2000). Effects of pelleting and pellet conditioning temperatures on weanling pig performance. J. Anim. Sci. 78, 3014–3018. doi: 10.2527/2000.78123014x
Stein H. H. (2017). Procedures for determining digestibility of amino acids, lipids, starch, fibre, phosphorus, and calcium in feed ingredients fed to pigs. Anim. Prod. Sci. 57, 2317–2324. doi: 10.1071/AN17343
Stein H. H., Bohlke R. A. (2007). The effects of thermal treatment of field peas (Pisum sativum l.) on nutrient and energy digestibility of growing pigs. J. Anim. Sci. 85, 1424–1431. doi: 10.2527/jas.2006-712
Stein H. H., Seve B., Fuller M. F., Moughan P. J., de Lange C. F. M. (2007). Amino acid bioavailability and digestibility in pig feed ingredients: terminology and application. J. Anim. Sci. 85, 172–180. doi: 10.2527/jas.2005-742
Steinfeld H., Gerber P., Wassenaar T., Castel V., Rosales M., de Haan C. (2006). “Livestock’s long shadow,” in The livestock (Rome: Environment and Development Initiative (LEAD). Food and Agricultural Organization of the United Nations). Available at: http://www.fao.org/docrep/010/a0701e/a0701e00.HTM.
Strathe A. B., Danfaer A., Jorgensen H., Kebreab E. (2015). A dynamic growth model for prediction of nutrient partitioning and manure production in growing–finishing pigs: model development and evaluation. J. Anim. Sci. 93, 1061–1073. doi: 10.2527/jas2014-8262
Strathe A. B., Danfaer A., Sorensen H., Kebreab E. (2010). A multilevel nonlinear mixed-effects approach to model growth in pigs. J. Anim. Sci. 88, 638–649. doi: 10.2527/jas.2009-1822
Strathe A. B., Sorensen H., Danfaer A. (2009). A new mathematical model for combining growth and energy intake in animals: the case of the growing pig. J. Theor. Biol. 261, 165–175. doi: 10.1016/j.jtbi.2009.07.039
Sun T., Lærke H. N., Jørgensen H., Knudsen K. E. B. (2006). The effect of extrusion cooking of different starch sources on the in vitro and in vivo digestibility in growing pigs. Anim. Feed Sci. Technol. 131, 67–86. doi: 10.1016/j.anifeedsci.2006.02.009
Sutton M. A., Howard C. M., Adhya T. K., Baker E., Baron J., Basir A., et al. (2019). “Nitrogen-grasping the challenge,” in A manifesto for science-in-Action through the international nitrogen management system. summary report (Edinburgh, UK: Center for Ecology and Hydrology).
Thiel-Cooper R. L., Parrish F. C. Jr., Sparks J. C. (2001). Conjugated linoleic acid changes swine performance and carcass composition. J. Anim. Sci. 79, 1821–1828. doi: 10.2527/2001.7971821x
Thompson P. B. (1997). Ethics and the genetic engineering of food animals. J. Agricul. Environ. Ethics. 10, 1–23. doi: 10.1023/A:1007758700818
Torrallardona D., Harris C. I., Fuller M. F. (2003). Pigs’ gastrointestinal microflora provide them with essential amino acids. J. Nutr. 133, 1127–1131. doi: 10.1093/jn/133.4.1127
Tous N., Lizardo R., Vilà B., Gispert M., Font-l-Furnols M., Esteve-Garcia E. (2013). Effect of a high dose of CLA in finishing pig diets on fat deposition and fatty acid composition in intramuscular fat and other fat depots. Meat Sci. 93, 517–524. doi: 10.1016/j.meatsci.2012.10.005
Town S. C., Putman C. T., Turchinsky N. J., Dixon W. T., Foxcroft G. R. (2004). Number of conceptuses in utero affects porcine fetal muscle development. Reproduction 128, 443–454. doi: 10.1530/rep.1.00069
Trabue S., Kerr B. (2014). Emissions of greenhouse gases, ammonia, and hydrogen sulfide from pigs fed standard diets and diets supplemented with dried distillers grains with solubles. J. Environ. Qual. 43, 1176–1186. doi: 10.2134/jeq2013.05.0207
Trabue S. L., Kerr B. J., Scoggin K. D. (2019a). Swine diets impact manure characteristics and gas emissions: part II sulfur level. Sci. Tot. Environ. 689, 800–807. doi: 10.1016/j.scitotenv.2019.06.130
Trabue S. L., Kerr B. J., Scoggin K. D. (2019b). Swine diets impact manure characteristics and gas emissions: part II sulfur source. Sci. Tot. Environ. 689, 1115–1124. doi: 10.1016/j.scitotenv.2019.06.272
Trabue S. L., Kerr B. J., Scoggin K. D., Andersen D., van Weelden M. (2021a). Swine diets impact manure characteristics and gas emissions: part I protein level. Sci. Tot. Environ. 755, 142528. doi: 10.1016/j.scitotenv.2020.142528
Trabue S. L., Kerr B. J., Scoggin K. D., Andersen D., van Weelden M. (2021b). Swine diets impact manure characteristics and gas emissions: part II protein source. Sci. Tot. Environ. 763, 144207. doi: 10.1016/j.scitotenv.2020.144207
Trabue S. L., Kerr B. J., Scoggin K. D., Andersen D. S., van Weelden M. (2022). Swine diets: impact of carbohydrate sources on manure characteristics and gas emissions. Sci. Tot. Environ. 825, 153911. doi: 10.1016/j.scitotenv.2022.153911
Ulrey W. D., Nurnett T. J., Brunelle S. L., Lombardi K. R., Coleman M. R. (2013). Determination and confirmation of parent and total ractopamine in bovine, swine, and turkey tissues by liquid chromatography with tandem mass spectrometry: final action 2011.23. J. AOAC Int. 96, 917–924. doi: 10.5740/jaoacint.12-395
UNEP (2019). “Sustainable nitrogen management resolution,” in UNEP/EA.4/Res (Nairobi, Kenya: United Nations Environmental Programme), 14. Available at: https://wedocs.unep.org/bitstream/handle/20.500.11822/37074/UNEA%20resolution%20on%20SNM%20-%20final%20text%20transmitted%20to%20UNEP%20secretariat.pdf?sequence=1&isAllowed=y#:~:text=resolution%20UNEP%2F%20EA.-,4%2F%20Res.,quality%20and%20greenhouse%20gas%20emissions.
Uwizeye A., de Boer I. J. M., Opio C. I., Schulte R. P. O., Falcucci A., Tempio G., et al. (2020). Nitrogen emissions along the global livestock supply chains. Nat. Food 1, 437–446. doi: 10.1038/s43016-020-0113-y
Van Boeckel T. P., Brower C., Gilbert M., Grenfell B. T., Levin S. A., Robinson T. P., et al. (2015). Global trends in antimicrobial use in food animals. PNAS 112, 5649–5654. doi: 10.1073/pnas.1503141112
van der Peet-Schwering C. C. M., Aarnink A. J. A., Rom H. B., Dourmad J. Y. (1999). Ammonia emissions from pig houses in the Netherlands, Denmark and France. Livest. Prod. Sci. 58, 265–269. doi: 10.1016/S0301-6226(99)00017-2
van der Poel A. F. B., Abdollahi M. R., Cheng H., Colovic R., den Hartog L. A., Miladinovic D., et al. (2020). Future directions of animal feed technology research to meet the challenges of a changing world, anim. Feed Sci. Technol. 270, 114692. doi: 10.1016/j.anifeedsci.2020.114692
van Kempen T. A. A. G., Baker D. H., van Heugten E. (2003). Nitrogen losses in metabolism trials. J. Anim. Sci. 81, 2649–2650. doi: 10.2527/2003.81102649x
van Lunen T. A., Cole D. J. A. (1998). Growth and body composition of highly selected boars and gilts. Anim. Sci. 67, 107–116. doi: 10.1017/S135772980000984X
van Milgen J., Dourmad J. Y. (2015). Concept and application of ideal protein for pigs. J. Anim. Sci. Biotech. 6, 15. doi: 10.1186/s40104-015-0016-1
Vann R. C., Nguyen H. V., Reeds P. J., Burrin D. G., Fiorotto M. L., Steele N. C., et al. (2000). Somatotropin increases protein balance by lowering body protein degradation in fed, growing pigs. Am. J. Physiol. Endocrinol. Metab. 278, E477–E483. doi: 10.1152/ajpendo.2000.278.3.E477
Velthof G. L., Nelemans J. A., Oenema O., Kuikman P. J. (2005). Gaseous nitrogen and carbon losses from pig manure derived from different diets. J. Environ. Qual. 34, 698–706. doi: 10.2134/jeq2005.0698
Villamar C. A., Sardá C. (2020). Nutrients cycle within swine production: generation, characteristics, treatment and revaluation. Livestock Health Farming. doi: 10.5772/intechopen.89733
Vonderohe C. E., Mills K. M., Liu S., Asmus M. D., Otto-Tice E. R., Richert B. T., et al. (2022). The effect of reduced CP, synthetic amino acid supplemented diets on growth performance and nutrient excretion in wean to finish swine. J. Anim. Sci. 100, 1–15. doi: 10.1093/jas/skac075
Walker N. (1989). A comparison of wheat-based or barley-based diets given as-libitum as meal or pellets to finishing pigs. Anim. Feed Sci. Technol. 22, 263–267. doi: 10.1016/0377-8401(89)90068-0
Wang J., Feng C., Liu T., Shi M., Wu G., Bazer F. (2017). Physiological alterations associated with intrauterine growth restriction in fetal pigs: causes and insights for nutritional optimization. Mol. Reprod. Dev. 84, 897–904. doi: 10.1002/mrd.22842
Wang T., Huo Y., Shi F., Xu R., Hutz R. J. (2005). Effects of intrauterine growth retardation on development of the gastrointestinal tracts in neonatal pigs. Biol. Neonate 88, 66–72. doi: 10.1159/000084645
Wang T., Liu C., Feng C., Wang X., Lin G., Zhu Y., et al. (2013). IUGR alters muscle fiber development and proteome in fetal pigs. Front. Biosci. (Landmark Ed.) 18, 598–607. doi: 10.2741/4123
Wang Y., Zhou J., Wang G., Cai S., Zeng X., Qiao S. (2018). Advances in low-protein diets for swine. J. Anim. Sci. Biotechnol. 9, 60. doi: 10.1186/s40104-018-0276-7
Weber T. E., Richert B. T., Belury M. A., Gu Y., Enright K., Schinckel A. P. (2006). Evaluation of the effects of dietary fat, conjugated linoleic acid, and ractopamine on growth performance, pork quality, and fatty acid profiles in genetically lean gilts. J. Anim. Sci. 84, 720–732. doi: 10.2527/2006.843720x
Weber T. E., Schinckel A. P., Houseknecht K. L., Richert B. T. (2001). Evaluation of conjugated linoleic acid and dietary antibiotics as growth promotants in weanling pigs. J. Anim. Sci. 79, 2542–2549. doi: 10.2527/2001.79102542x
Webster M. J., Goodband R. D., Tokach M. D., Nelssen J. L., Dritz S. S., Unruh J. A., et al. (2007). Interactive effects between ractopamine hydrochloride and dietary lysine on finishing pig growth performance, carcass characteristics, pork quality, and tissue accretion. Prof. Anim. Scientist 23, 597–611. doi: 10.15232/S1080-7446(15)31029-9
Wesoly R., Weiler U. (2012). Nutritional influences on skatole formation and skatole metabolism in the pig. Animals 2, 221–242. doi: 10.3390/ani2020221
Wiegand B. R., Parrish F. C. Jr., Swan J. E., Larsen S. T., Baas T. J. (2001). Conjugated linoleic acid improves feed efficiency, decreases subcutaneous fat, and improves certain aspects of meat quality in stress-genotype pigs. J. Anim. Sci. 79, 2187–2195. doi: 10.2527/2001.7982187x
Wiegand B. R., Sparks J. C., Parrish F. C. Jr., Zimmerman D. R. (2002). Duration of feeding conjugated linoleic acid influences growth performance, carcass traits, and meat quality of finishing barrows. J. Anim. Sci. 80, 637–643. doi: 10.2527/2002.803637x
Wiseman T. G., Mahan D. C., Moeller S. J., Peters J. C., Fastinger N. D., Ching S., et al. (2007a). Phenotypic measurements and various indices of lean and fat tissue development in barrows and gilts of two genetic lines from twenty to one hundred twenty-five kilograms of body weight. J. Anim. Sci. 85, 1816–1824. doi: 10.2527/jas.2006-406
Wiseman T. G., Mahan D. C., Peters J. C., Fastinger N. D., Ching S., Kim Y. Y. (2007b). Tissue weights and body composition of two genetic lines of barrows and gilts from twenty to one hundred twenty-five kilograms of body weight. J. Anim. Sci. 85, 1825–1835. doi: 10.2527/jas.2006-407
Wondra K. J., Hancock J. D., Behnke K. C., Hines R. H., Stark C. R. (1995a). Effects of particle size and pelleting on growth performance, nutrient digestibility, and stomach morphology in finishing pigs. J. Anim. Sci. 73, 757–763. doi: 10.2527/1995.733757x
Wondra K. J., Hancock J. D., Kennedy G. A., Hines R. H., Behnke K. C. (1995b). Reducing particle size of corn in lactation diets from 1, 200 to 400 micrometers improves sow and litter performance. J. Anim. Sci. 73, 421–426. doi: 10.2527/1995.732421x
Wu G., Bazer F. W., Wallace J. M., Spencer T. E. (2006). Board-invited review: intrauterine growth retardation: implications for the animal sciences. J. Anim. Sci. 84, 2316–2337. doi: 10.2527/jas.2006-156
Wünsche J., Hennig U., Meinl M., Kreienbring F., Bock H. D. (1982). Absorption and utilization of amino acids infused into the cecum of growing pigs. 1. measurement of n-balance for utilization of lysine and isoleucine; isoleucine requirement of growing pigs. Arch. Tierernahr. 32, 337–348. doi: 10.1080/17450398209421023
Xing J. J., van Heugten E., Li D. F., Touchette K. J., Coalson J. A., Odgaard R. L., et al. (2004). Effects of emulsification, fat encapsulation, and pelleting on weanling pig performance and nutrient digestibility. J. Anim. Sci. 82, 2601–2609. doi: 10.2527/2004.8292601x
Xiong L., You J., Zhang W., Zhu Q., Blachier F., Yin Y., et al. (2020). Intrauterine growth restriction alters growth performance, plasma hormones, and small intestinal microbial communities in growing-finishing pigs. J. Anim. Sci. Biotechnol. 11, 86. doi: 10.1186/s40104-020-00490-x
Xue J., Dial G. D. (1997). Raising intact male pigs for meat: detecting and preventing boar taint. Swine Health Prod. 5, 151–158.
Xue J., Dial G. D., Pettigrew J. E. (1997). Performance, carcass, and meat quality advantages of boars over barrows: a literature review. Swine Health Prod. 5, 21–28.
Ye H., Langendijk P., Jaworski N. W., Wu Y., Bai Y., Lu D., et al. (2022). Protein digestion kinetics influence maternal protein loss, litter growth, and nitrogen utilization in lactating sows. Front. Nutr. 9. doi: 10.3389/fnut.2022.862823
Ye Z., Zhang G., Li B., Strom J. S., Tong G., Dahl P. J. (2008). Influence of airflow and liquid properties on the mass transfer coefficient of ammonia in aqueous solutions. Biosyst. Eng. 100, 422–434. doi: 10.1016/j.biosystemseng.2008.04.016
Yen J. T., Kerr B. J., Easter R. A., Parkhurst A. M. (2004). Difference in rates of net portal absorption between crystalline and protein-bound lysine and threonine in growing pigs fed once daily. J. Anim. Sci. 82, 1079–1090. doi: 10.2527/2004.8241079x
Yoon S. Y., Yang Y. X., Shinde P. L., Choi J. Y., Kim J. S., Kim Y. W., et al. (2010). Effects of mannanase and distillers dried grain with solubles on growth performance, nutrient digestibility, and carcass characteristics of grower-finisher pigs. J. Anim. Sci. 88, 181–191. doi: 10.2527/jas.2008-1741
Younes H., Remesy C., Behr S., Demigne C. (1997). Fermentable carbohydrate exerts a urea-lowering effect in normal and nephrectomized rats. Am. J. Physiol. 272, G515–G521. doi: 10.1152/ajpgi.1997.272.3.G515
Yu P., Prates L. L. (2017). Molecular structure of feeds in relation to nutrient utilization and availability in animals: a novel approach. Engineering 3, 726–730. doi: 10.1016/J.ENG.2017.03.007
Yu M., Zhang C., Yang Y., Mu C., Su Y., Yu K., et al. (2017). Long-term effects of early antibiotic intervention on blood parameters, apparent nutrient digestibility, and fecal microbial fermentation profile in pigs with different dietary protein levels. J. Anim. Sci. Biotechnol. 8, 60. doi: 10.1186/s40104-017-0192-2
Yue L. Y., Qiao S. Y. (2008). Effects of low-protein diets supplemented with crystalline amino acids on performance and intestinal development in piglets over the first 2 weeks after weaning. Livest. Sci. 115, 144–152. doi: 10.1016/j.livsci.2007.06.018
Zeng Z. K., Shurson G. C., Urriola P. E. (2017). Prediction of the concentration of standardized ileal digestible amino acids and safety margins among sources of distillers dried grains with solubles for growing pigs: a meta-analysis approach. Anim. Feed Sci. Tech. 231, 150–159. doi: 10.1016/j.anifeedsci.2017.07.010
Zentek J., Boroojeni F. G. (2020). (Bio)Technological processing of poultry and pig feed: impact on the composition, digestibility, anti-nutritional factors and hygiene. Anim. Feed Sci. Technol. 268, 114576. doi: 10.1016/j.anifeedsci.2020.114576
Zervas S., Zijlstra R. T. (2002a). Effects of dietary protein and oathull fiber on nitrogen excretion patterns and postprandial plasma urea profiles in grower pigs. J. Anim. Sci. 80, 3238–3246. doi: 10.2527/2002.80123238x
Zervas S., Zijlstra R. T. (2002b). Effects of dietary protein and fermentable fiber on nitrogen excretion patterns and plasma urea in grower pigs. J. Anim. Sci. 80, 3247–3256. doi: 10.2527/2002.80123247x
Zhang F., Adeola O. (2017). Techniques for evaluating digestibility of energy, amino acids, phosphorus, and calcium in feed ingredients for pigs. Anim. Nutr. 3, 344–352. doi: 10.1016/j.aninu.2017.06.008
Zhang S., Regnault T. R., Barker P. L., Botting K. J., McMillen I. C., McMillan C. M., et al. (2015). Placental adaptations in growth restriction. Nutrients 7, 360–389. doi: 10.3390/nu7010360
Zhou L. J., Zhang L. Y., Zhang E. X., Li. J. T., Yang W. J., Wang Z. Y. (2012). Rapid determination of swine available energy and amino acids in corn distillers dried grains with solubles by near-infrared reflectance spectroscopy. Anim. Feed Sci. Technol. 175, 198–202. doi: 10.1016/j.anifeedsci.2012.06.001
Keywords: amino acids, environmental impacts, metabolic modifiers, lean tissue growth, nitrogen balance, nutrition, swine
Citation: Shurson GC and Kerr BJ (2023) Challenges and opportunities for improving nitrogen utilization efficiency for more sustainable pork production. Front. Anim. Sci. 4:1204863. doi: 10.3389/fanim.2023.1204863
Received: 12 April 2023; Accepted: 27 June 2023;
Published: 19 July 2023.
Edited by:
Irida Palamidi, Agricultural University of Athens, GreeceReviewed by:
Markus Rodehutscord, University of Hohenheim, GermanyCopyright © 2023 Shurson and Kerr. This is an open-access article distributed under the terms of the Creative Commons Attribution License (CC BY). The use, distribution or reproduction in other forums is permitted, provided the original author(s) and the copyright owner(s) are credited and that the original publication in this journal is cited, in accordance with accepted academic practice. No use, distribution or reproduction is permitted which does not comply with these terms.
*Correspondence: Gerald C. Shurson, c2h1cnMwMDFAdW1uLmVkdQ==
Disclaimer: All claims expressed in this article are solely those of the authors and do not necessarily represent those of their affiliated organizations, or those of the publisher, the editors and the reviewers. Any product that may be evaluated in this article or claim that may be made by its manufacturer is not guaranteed or endorsed by the publisher.
Research integrity at Frontiers
Learn more about the work of our research integrity team to safeguard the quality of each article we publish.