- 1Animal Behavior and Cognition Lab, Department of Animal Science, University of California, Davis, Davis, CA, United States
- 2School of Environmental and Rural Science, University of New England, Armidale, NSW, Australia
- 3Animal Behaviour and Welfare Team, Agriculture and Food, Commonwealth Scientific and Industrial Research Organisation (CSIRO), Armidale, NSW, Australia
Immune competence in domestic sheep is gaining more attention in genetic selection programs that seek to enhance flock immunity and animal welfare. A goal of many programs is to identify behavioral phenotypes that indicate stress-coping strength, as well as disease resilience. The current study set out to explore the relationship between immune responsiveness, physiological stress, and behavioral response among sheep that had been selected for ‘low’ (LR) or ‘high’ (HR) response to cell-mediated and antibody-mediate reactivity to a clostridial vaccine. Multiparous ewes were placed in four experiments which exposed the animals to various threats including, dog presence, human proximity, visual isolation from flock, and physical restraint. To evaluate the consistency of behavioral phenotypes, all ewes were placed in the test circuit one year later. Basal body temperature (via iButton) and serum cortisol concentrations were collected prior to and after the circuit each year. Immune group (HR vs. LR) was not found to be related to behavioral performance during the dog, human, isolation, or restraint challenge. Immune group categorization was also unrelated to pre- and post-cortisol concentrations, and the change in cortisol concentrations during testing. There was a negative relationship identified between response to visual isolation and change in cortisol response during testing, indicating that sheep which were more active or agitated during visual isolation from the flock experienced less of an increase in serum cortisol levels and were perhaps experiencing a negative, high arousal state compared to less reactive sheep (e.g., freeze behavior) (χ2(4, N=99) = 42.72, P <0.0001). There was also a post hoc, positive relationship identified between weight measurements and immune group, such that individuals with greater body weight were more likely to be in the high immune responsiveness group (P= 0.01). Specifically, for every unit increase in weight, there was a ~49% chance of being categorized in the HR group. This is relevant for selection programs because producers that seek to enhance immune responsiveness and performance may be able to select sheep that carry a greater body mass, and while not found in the present study, could lead to greater flock immunity.
1 Introduction
Selective breeding for disease resilience in livestock has been of increased interest for reasons of animal and environmental health, animal welfare, and consumer pressure. Programs that prioritize health and welfare of the animal should be streamlined, since factors that compromise one often also compromise the other (Roger, 2008). Traditionally, selective breeding programs have been focused on enhancing performance traits such as body mass, average daily gain, net feed intake, and certain carcass traits. Selective breeding of livestock for production traits, however, carries the risk of a decline in fitness and an increase in susceptibility to environmental stressors and infectious diseases (Rauw et al., 1998). Drawing on early work on immune responsiveness in mice and chickens (reviewed by van der Zijpp, 1983) and pigs (Mallard et al., 1992), Wilkie and Mallard (1999) proposed genetic selection for high immune responsiveness or against low immune responsiveness, as a strategy to help counter this trend. Genetic selection for immune responsiveness has been explored in numerous studies in dairy (Mallard et al., 2015) and beef cattle (Hine et al., 2019), pigs (Mallard et al., 1992), sheep (Hine et al., 2022), fish (Wiegertjes et al., 1996) and chickens (Pinard et al., 1992). The general approach has been to combine measures of the strength of antibody and cellular immune responses following vaccination to generate an index of combined immune responsiveness (CIR, e.g. Reverter et al., 2021). The CIR trait has low to moderate heritability and is favorably associated with a diversity of health and production outcomes that develop over the lifetime of the animal (e.g. Mallard et al., 2015; Hine et al., 2021; Hine et al., 2022; Cartwright et al., 2023).
To acknowledge the increased need for a tool to select animals for improved immune function, a multitude of authors have released reports on the potentiality of using behavioral activity under familiar settings (Burgunder et al., 2018; Högberg et al., 2021) and reactivity during challenging or stress-inducing events (Caroprese et al., 2010) as a method for identifying individuals with greater immune responsiveness. Observing behavior as a tool for selection is desirable, as it is non-invasive, non-time consuming and inexpensive. When combined with other factors that promote proper health and welfare in sheep (e.g., proper housing, handling, and hygiene), there is an opportunity to optimize animal livelihood on farms with more concentrated focus on minimizing disease prevalence. Most attention thus far has been oriented towards reducing the seroprevalence of intestinal parasites (Grant et al., 2020) in sheep since this can negatively impact flock performance by altering appetite and durations of grazing, in addition treatments (i.e., anthelmintic) for parasites can lead to parasitic resistance causing further issues. Severity of infection with gastrointestinal nematodes affects demeanor as assessed by qualitative behavioral assessment (Grant et al., 2020). Lying time and motion index were both decreased by exposure to gastrointestinal parasites in lambs (Högberg et al., 2021), however, these effects could be due to either or a combination of pathological consequences of infection and activity of the immune system. A clearer link between immune function and behavior is evident in the study of Adams and Fell (1997) which found that approach distance to a human was reduced in an arena test in parasite-immune but not naïve sheep when challenged with the abomasal parasite, Haemonchus contortus. The interplay between parasitic load, behavior and energy expenditure is more logically explained, since parasite burden influences nutrient absorption, however, the mechanistic role of the central nervous system in modulating HPA-axis activation, immune responsiveness, and behavior in sheep is less understood. With considerable question of how HPA-axis activation, immune responsiveness and behavior interact in sheep, it is difficult to know the physiological consequences of behavioral selection in this species (Ciliberti et al., 2017).
According to current scientific understanding, the immune system and CNS respond rapidly to environmental and endogenous challenges and are connected through the ANS via molecular signaling (neuropeptides, neurohormones) (Borhetti et al., 2009; Caroprese et al., 2010; Ciliberti et al., 2017) such that efficient crosstalk between the immune system and the neuroendocrine system will result in an adaptive response to pathogens (Borhetti et al., 2009). Further, communication between the brain and the immune system is facilitated by the sympathetic branch of the ANS and the HPA axis (Sternberg, 2006; Koolhaas, 2008). Sapolsky et al. (2000) suggests that the role of glucocorticoids in acute phase response of the immune system are both stimulating (promote disease prevention) and inhibitory (to prevent autoimmune disease), yet the comprehensive role of glucocorticoids in the immune system is largely an area of confusion. The general stress adaptation syndrome (Selye, 1946) describes the physiological outcomes during acute and chronic stressors such that acute stressors will adaptively activate the sympathetic nervous system and HPA-axis to protect the animal from immediate harm or injury, while chronic stress and prolonged HPA-axis activation can have detrimental effects on bodily tissues and may lead to negative inflammatory responses (McEwen et al., 1997) caused by insufficient cell reception and feedback loops.
Chronic stress in farmed species, exceedingly sheep, can be a significant issue leading to psychological stress, damage to reproductive organs, and hypothetically compromised immune function resulting in greater risk of disease. Depending on the physiological marker being observed, the impacts of long-term stress can be indicated through immune responsiveness. Destrez et al. (2012) found that lambs subjected to chronic unpredictable and uncontrollable events had lower granulocyte (a white blood cell responsible for releasing an enzyme when the immune system is under attack) counts compared to control lambs. On the other hand, Caroprese et al. (2006) reported that positive interactions with a human handler can also alter immune responsiveness, with artificially reared lambs having an enhanced humoral response to antigens after receiving prolonged gentling. Though Destrez et al. (2012) and Caroprese et al. (2006) highlight the impact of psychological state on immune responsiveness, they both reported that cortisol concentrations did not differ between treatments after a certain amount of time. To date, most studies looking at the interplay of stress, behavior and immune function assess immune responsiveness after an acute stressor (Sutherland et al., 2019; Shamsi et al., 2023). Further, understanding immune responsiveness, stress and behavior is complicated by differential findings dependent on time of day (Destrez et al., 2012), type, intensity and duration of stimulus applied Sutherland et al., 2019). The role of temperament and coping styles in immune responsiveness and stress is also relatively unknown in sheep (Koolhaas, 2008).
A consistent association between behavioral reactivity and immune responsiveness in sheep is not evident from the above studies. Identifying a coherent pattern of response between the immune system, HPA-axis activity and behavior would ease selection processes targeted at identifying sheep that are immune and stress resilient. Observing behavioral performance to infer improved immune resilience therein improved health and welfare is possible, however, tricky when the association between behavior, stress and immunity is under-reported in this species. To further confuse the matter, the relationship between HPA-axis activation and behavioral reactivity in sheep is somewhat inconsistent. While some authors report a positive relationship between HPA-axis activation and withdrawal or freeze behavior in the presence of a human (Caroprese et al., 2010; Yu et al., 2021), others have reported no differences between activity level and cortisol response in sheep (Beausoleil et al., 2012; Cakmakci et al., 2021). Nonetheless, behavioral phenotypes are recognized as strong determinants of disease susceptibility in evolutionary biology (Barber and Dingemanse, 2010). Sheep phenotyped for CIR provide a valuable resource for examining these associations.
For this study, ewes were classified as high immune responsiveness (HR) or low immune responsiveness (LR) based on their cell-mediated immune responses to a multivalent clostridial vaccine and a Johne’s disease vaccine and antibody-mediated responses to the clostridial vaccine. A year later, sheep were subjected to a battery of challenges including a dog, human, isolation, and restraint challenge in a circuit test to assess their behavioral reactivity, which was repeated another year after that. The sheep were not actively mounting a response to the vaccine at the time of circuit testing. The objectives of the current study were to 1.) explore the relationship between immune responsiveness and behavioral reactivity to a human, dog, isolation, and restraint challenge in a circuit test; 2.) explore the relationship between behavioral responses to the circuit test and the change in cortisol concentrations collected before and after testing; 3.) identify the relationship between immune responsiveness and change in cortisol concentrations prior to and after testing. The authors hypothesize that 1.) sheep that belong to the high immune responsiveness group will display increased levels of activity during circuit testing relative to sheep that belong to the low immune responsiveness group. This hypothesis is informed by previous reports that pigs that were more active in an open environment had higher immune responsiveness (circulating pro-inflammatory cytokines) and decreased levels of cortisol relative to less active pigs. Secondly, the authors hypothesize that 2.) behavioral responses of sheep that are more active during the circuit test will have a negative relationship with change in cortisol concentrations relative to sheep that are less active. This hypothesis is informed by the assumption that sheep that are more resilient to acute and chronic stressors are those that have decreased levels of stress when presented with a challenge and will be more willing to explore their environment compared to animals that are more likely to perform vigilance/immobility behaviors associated with higher HPA-axis activity (Caroprese et al., 2010; Pajor et al., 2013; Sutherland et al., 2019; Aydogdu and Karaca, 2021). Lastly, the authors hypothesize that 3.) animals that belong to the high immune responsiveness group will have an increased change in cortisol concentrations relative to animals that belong in the high immune responsiveness group. This third hypothesis is informed by the assumption that animals that are more susceptible to acute and chronic stress may experience suppressive effects of cortisol on the immune system and enhanced inflammatory responses (McEwen et al.,1997).
2 Methods
2.1 Ethical statement
The protocol and conduct of the study were approved by the University of New England Animal Ethics Committee, under the New South Wales Animal Research Act 1985 (Animal Research Authority numbers 16-003 and 17-015).
2.2 Animals
One hundred adult ewes from the Sheep CRC Information Nucleus Flock in Armidale, NSW, Australia were used in this study. The sheep were 3 or 4 years old (depending on year of birth) at the beginning of the study and were a mixture of Merino (n=73) and Maternal (Merino x Border Leicester or similar, n=27) breeds. Sheep weighed an average of 52.5 ± 6.4 kg at the beginning of the experiment and 57.1 ± 6.6 kg at the time of testing one year later. The sheep were selected from a larger population of 472 ewes that had been immune competence phenotyped as described by Hine et al. (2022), based on both their cell-mediated and antibody-mediated immune responses to vaccines. Sheep were then ranked on immune competence and the top 50 (High) and bottom 50 (Low) ranked ewes that were available for further testing were selected for the current study.
The sheep used in this study were raised in the same flock under extensive grazing conditions and were housed at pasture during periods of behavioral testing. Sheep underwent routine husbandry procedures involving mustering with dogs for monitoring and maintenance of health. All sheep had previous exposure to the isolation box test and flight speed assessment. Sheep had no experience with attention bias testing, the arena, maze testing or food competition testing.
2.3 Experimental design
The ewes underwent a series of behavioral tests and physiological measures over a period of 3 weeks, which were repeated on the same animals one year later. At the beginning of the experiment, the sheep were weighed, had numbers painted (non-toxic livestock marker) on their rump for individual identification during testing and were drafted into four cohorts of approximately 25 animals, balancing for breed and bodyweight. Sheep were assigned the same identification number and cohort in the second year. Between the years, sheep were returned to the farm. Only 83 of the ewes were available for re-testing during the second year.
Each year, testing occurred in two phases; a circuit in which each sheep underwent consecutive testing. The two additional tests are not further reported on in this paper. The cohorts were returned to four different paddocks with access to pasture and a small amount of supplementary lucerne hay while not being tested. During the circuit, all sheep individually underwent the following consecutive procedures over a period of approximately 14 min: blood sampling, dog threat test, human proximity test, isolation box test, restraint test and a second blood sampling. Prior to their allocated circuit testing day, all the sheep within each testing group were walked to the yards by humans on foot and had ibuttons inserted for assessment of internal body temperature during the circuit. Temperature loggers were programmed to begin recording on the morning of testing. After circuit testing was completed for all cohorts, they were combined and all sheep were kept together for further testing. The details for each of these procedures are given below.
2.4 Dog threat test
An attention bias test set-up was used to conduct the dog threat test for the purposes of this study. This test is used to observe the relative attention paid towards a predator threat and food, as a measure of anxious states in sheep (Lee et al., 2016). This study used the attention bias test arena and testing procedure described by Monk et al. (2018). The test arena was 4.2 m x 4 m in size, enclosed with 1.8 m high opaque walls. Lucerne hay was placed in the center of the test arena. When a sheep entered, a dog standing outside the arena was visible through a window on the side. After 3 s, the window was closed and the dog was removed to a waiting area. The same dog was used each year (kelpie cross border collie). After the window had closed, the sheep remained in the arena for 3 min while behaviors were recorded using a video camera. Zones were overlaid onto video footage and were not physically delineated in the arena. The ethogram is provided in Table 1.
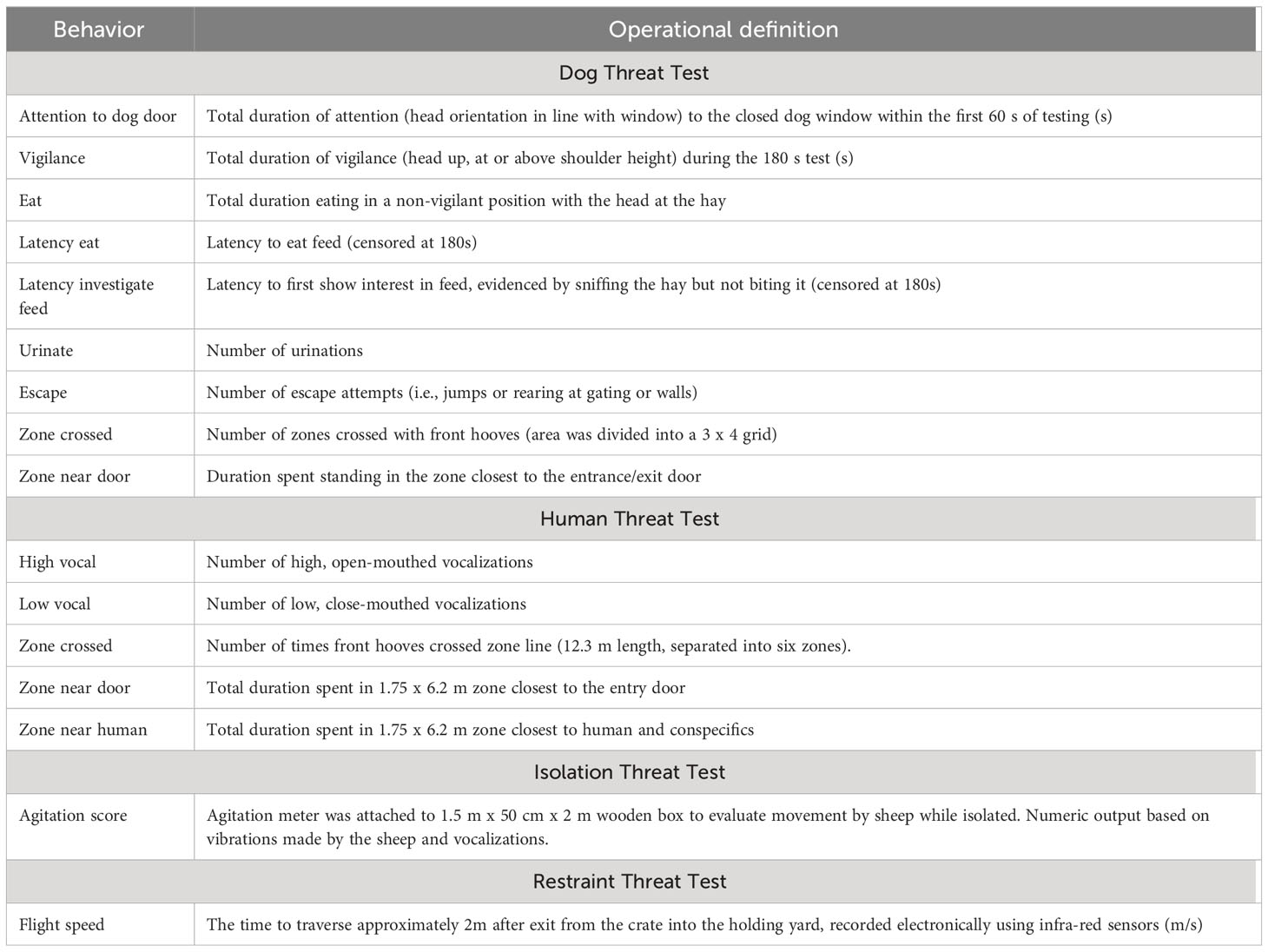
Table 1 Operational definitions of behaviors recorded in the dog threat test, human threat test, flisolation threat test and restraint threat test
2.5 Human threat test
A traditional arena test was used to conduct the human threat test for the purposes of this study. The arena test measures conflict between aversion of a human and motivation to reunite with conspecifics (Murphy et al., 1994). The methodology used was similar to that described by Murphy et al. (1994). The arena used in this study was 12 m x 6 m in size, with approximately 1.2 m high walls covered with shade cloth. At one end of the arena, 3 conspecifics were kept in a small pen with access to water. The conspecifics were not part of the tested cohort. A stationary human sat on a stool positioned directly in front of the pen. The human differed between the two years; both were adult males. Test sheep entered the arena from the end furthest from the human and remained in the arena for 3 min. Their behaviors were recorded using a video camera. The arena was divided into 6 zones, which were overlaid onto video footage. The durations spent in each zone were scaled by a factor of 0.1 times the zone number (i.e. 0.1 x duration in zone 1, 0.2 x duration in zone 2 and so on). The scaled durations were combined to produce a zone score between 18 and 108, where a higher score indicated the sheep spent a higher proportion of time in the zone closest to the human and sheep.
During the first year of testing, some sheep escaped from the circuit during or after the arena test by jumping over a fence. These sheep were mustered back into the yards to continue the circuit as quickly as possible but may have undergone additional stress due to this process.
2.6 Isolation box test
The isolation box test measures the level of agitation exhibited by a sheep during restraint in a wooden box (Bickell et al., 2009; Murphy et al., 1994). The isolation box was 1.5 m x 50 cm x 2 m with an open roof covered by shade-cloth, that was set on rubber tires. Sheep were moved into the box for a total of 30 s. Agitation was recorded using an agitation meter, which was calibrated at the beginning and middle of each test day (School of Animal Biology, University of Western Australia).
2.7 Restraint test
Sheep were restrained in a shaded roll-over sheep handling crate, designed at CSIRO (Armidale, Australia), which was kept upright, to allow excess heat to dissipate from the wool around the eye. Sheep were restrained for up to 5 min, however the time of restraint was reduced for some animals that took longer to move through the previous behavioral tests, as to maintain the correct timing for blood sampling.
2.8 Flight speed
Flight time was measured as the time to traverse 1.7m after exiting a weigh crate into an open holding yard, as a measure of agitation or aversion to handling (Blache and Ferguson, 2005). Time was recorded electronically using infra-red sensors (Ruddweigh Australia Pty Ltd., Guyra, Australia), then speed was calculated as meters per second.
2.9 Blood sampling and analysis
Prior to commencing the circuit of tests, and at the end of testing (14 min later), a 10ml blood sample was collected via jugular venipuncture for cortisol (CORT) analysis using a plastic serum vacutainer (red-top, Becton Dickinson, Macquarie Park, NSW, Australia). The blood samples were centrifuged at 2000 × g for 15 min at 5°C, then the serum was distributed into 2 ml aliquots that were stored at −20°C until analysis for CORT concentration. Serum CORT concentrations were determined using a commercial radioimmunoassay (CORT RIA, MP Biomedicals, Solon, Ohio, USA). The average intra-assay coefficients of variance (CV) for quality controls containing 34.2, 86.9 and 182.6 nmol/L of CORT were 12.0, 9.8 and 4.8% respectively. The inter-assay coefficients of variance were 17.5, 8.9 and 11.0% respectively.
2.10 Body temperature
Body temperature was recorded during the circuit testing using Thermochron iButtons® (Model number DS1922L-F5, accuracy 0.5°C, resolution 0.063°C, weight 3.3 g; Embedded Data Systems, Lawrenceburg, United States), which were attached to blank, progesterone-free Controlled Internal Drug Release devices (CIDR®, Zoetis, Melbourne, Australia) as described by Lea et al. (2008). The CIDRs were inserted into the vagina of the sheep at least one day prior to testing, and were removed immediately following the final blood sample collection at the end of the circuit. Temperature loggers were programmed to log at 1 min intervals, beginning at 07:00 on the morning of testing. Body temperatures were collated for each animal 5 min prior to blood sampling and at time 13 min, just prior to removal of the CIDR at the end of the testing circuit. Temperatures were extracted using the program eTemperature version 8.32 (OnSolution, Castle Hill, Australia).
2.11 Immune responsiveness
Ewes from two cohorts of lambs born in 2012 and 2013 were phenotyped for immune responsiveness in 2015 at 2 or 3 years of age. Ewes were vaccinated with commercial multivalent clostridial vaccine (Ultravac 5in1, Zoetis, Rhodes, NSW, Australia) and Johne’s disease vaccine (Gudair, Zoetis) according to the manufactures’ instructions. Ewes were then moved onto a livestock road transport vehicle and transported for 2 hours. Ewes had received routine clostridial vaccination as lambs prior to immunophenotyping hence clostridial vaccination was a booster and responses to clostridial antigens were secondary (recall) responses of the adaptive immune system. Gudair is a single shot vaccine containing inactivated (killed) Mycobacterium paratuberculosis strain 316F. Fourteen days later, blood was collected for determining antibody concentrations to tetanus antigen. Cell mediated responses were assessed by injecting 0.05 ml of each vaccine intradermally at separate sites on the medial thigh. Control sites received an equal volume of sterile saline for injection (Baxter, Toongabbie, NSW, Australia). Skin thickness was assessed 2 days later. CIR was estimated by combining (with equal weighting) measures of antibody mediated immune responsiveness to tetanus antigen and cell mediated immune responsiveness to the clostridial and Gudair vaccines. Standardization of residual values was undertaken to ensure equal weighting was given to both antibody and cell mediated immune responses in estimation of CIR (Hine et al., 2022). Fifty ewes with the highest and fifty ewes with the lowest CIR were enrolled in the study.
2.12 Statistical analysis
Preliminary assessments using Spearman’s rank order correlations (alpha = 0.05) were used evaluate individual consistency over the years as well as to investigate for possible relationships between change in cortisol concentrations, behavioral responses from the circuit test, and immune group. Visualizations using the ggplot package in R studio version 4.2.1 (R Development Core Team, 2018) were used with either year or breed as a factor on the x-axis and behavioral and cortisol responses on the y-axis, colored by immune group. Using the glmmTMB package, data were fit to linear mixed effects models for repeated measures with the behavioral variable as the response, year as a fixed factor, immune group as a factor term, individual ID as a random effect and restricted effects maximum likelihood (REML). The models also included a weight x breed interaction if the estimate appeared to be significant. These models were then compared to null models without immune group or change in cortisol concentrations as predictor terms to investigate if there was improvement of fit over the null according to AIC values from a chi square test. The glmmTMB package was used for this study since this package allows for negative binomial, Poisson, compois or truncated Poisson error distributions on zero-inflated data. Post hoc analysis was also performed using spearman’s rank order correlations to look at the relationships between cortisol concentrations before and after the circuit test and various behavioral responses. Non-parametric methods were used for this as both the cortisol responses and behavioral responses were abnormally distributed. Data was controlled for year, breed, weigh 1 and weigh 2 prior to being assessed using Spearman’s rank order correlations. Weight measurements were normally distributed and so a logistic regression was fit to the data with a logit link, using the polr utility in the MASS package (Venables and Ripley, 2002; Schiller et al., 2020) with a binary immune group response to explore the relationship between weight and immunity.
3 Results
3.1 Temporal consistency of behavioral and physiological measures across years
Behaviors collected during the dog threat test that produced positive and significant rank order correlations across both years were duration of vigilance (rs=-0.61, P<0.001), frequency of eating (rs=0.45, P=0.01), frequency of zone crossing (rs=0.43, P<0.001) and duration near the dog door (rs=0.49, P=<0.001) (Table 2). Duration of eating in the dog threat test was nearly significant across years (rs=0.40, P=0.09). Behaviors from the arena test that produced positive and significant rank order correlations across both years were low-pitched vocalizations (rs=-0.28, P=0.01) and duration of time spent in zone closest to conspecifics and human (rs=0.48, P<0.01). Cortisol measures taken before testing (rs=0.27, P<0.05), at the end of the circuit testing (rs=0.48, P<0.01), flight speed (rs=0.44, P<0.001) and behavior within the isolation box test (rs=0.31, P<0.001) were significantly correlated between the years. These behaviors were considered for analysis against other immune and stress response measures as they would be more indicative of a behavioral phenotype.
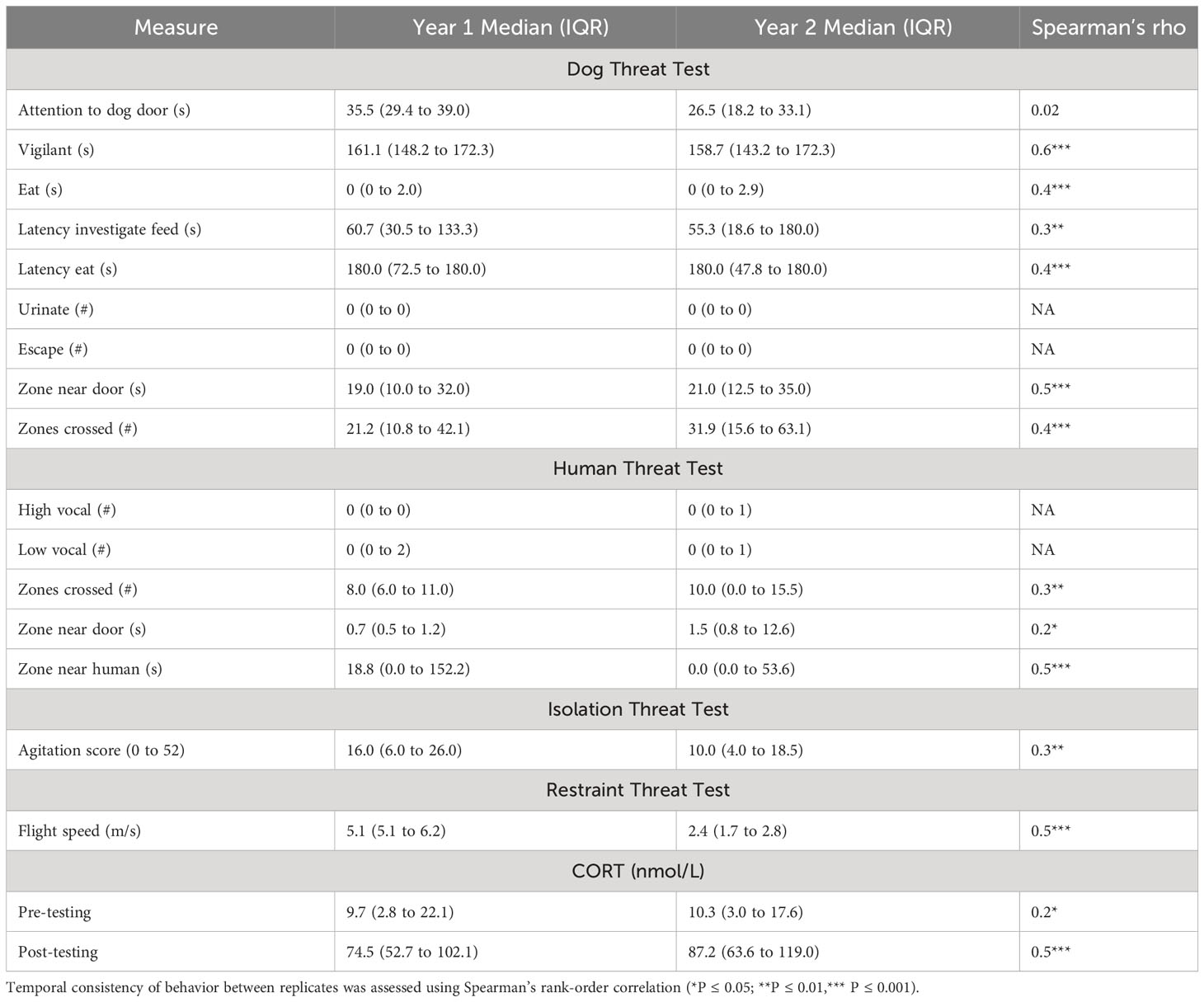
Table 2 Median (IQR) frequency and duration of behaviors measured in dog threat test, human threat test, isolation threat test, and restraint threat test, and serum cortisol concentrations before and after circuit of tests, across two consecutive years (n = 81).
3.2 Immune responsiveness and behavioral activity
According to preliminary visualizations, there appeared to be no relationships between immune group and behavioral responses in ewes (Figure 1). Further assessment using general linear mixed effects modeling for repeated measures confirmed that there was no relationship present between immune group and behavioral responses from the circuit test. Variables that were consistent between years 1 and 2 from the dog and human challenge included duration of ‘vigilance’, ‘attention to dog door’, duration of time in the zone nearest the dog door, zone score, and latency to sniff and investigate feed. As an example, adding immune group to the model with duration of ‘vigilance’ as a response resulted in no improvement of fit over the null model without the immune group term (χ2(4, N=99) = 0.22, P=0.64) (Figure 2). Likewise, there was no improvement of fit in the ‘attention to dog door’ model when immune group was included as a predictor term (χ2(4, N=99) = 0.33, P=0.56). These results were consistent with results from the isolation and restraint test. Immune group was not a significant predictor in the human, dog, visual isolation and restraint test models.
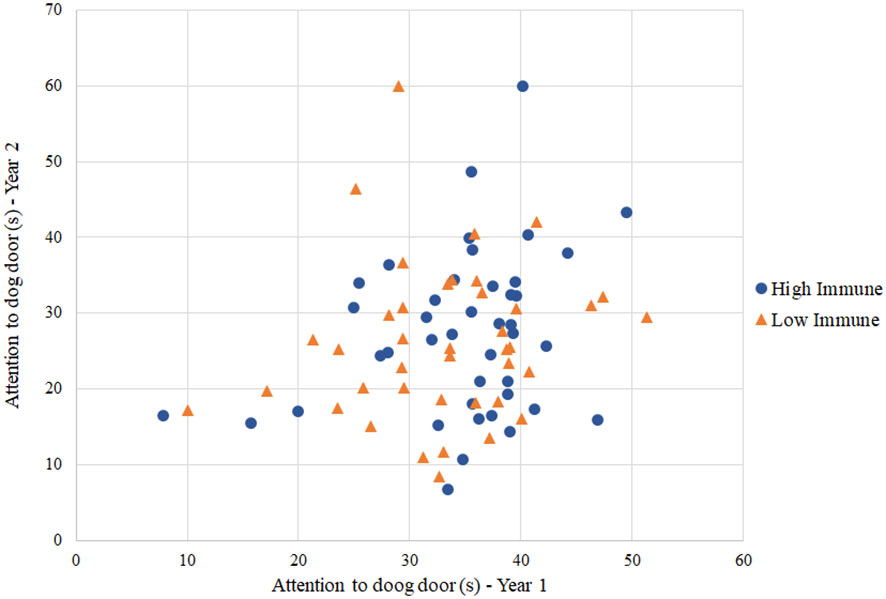
Figure 1 Scatterplot of duration of ‘attention to dog door’ per year, colored by group. Immune group status was not a significant predictor term in the ‘attention to dog door’ model (χ2(4, N=99) = 0.33, P=0.56).
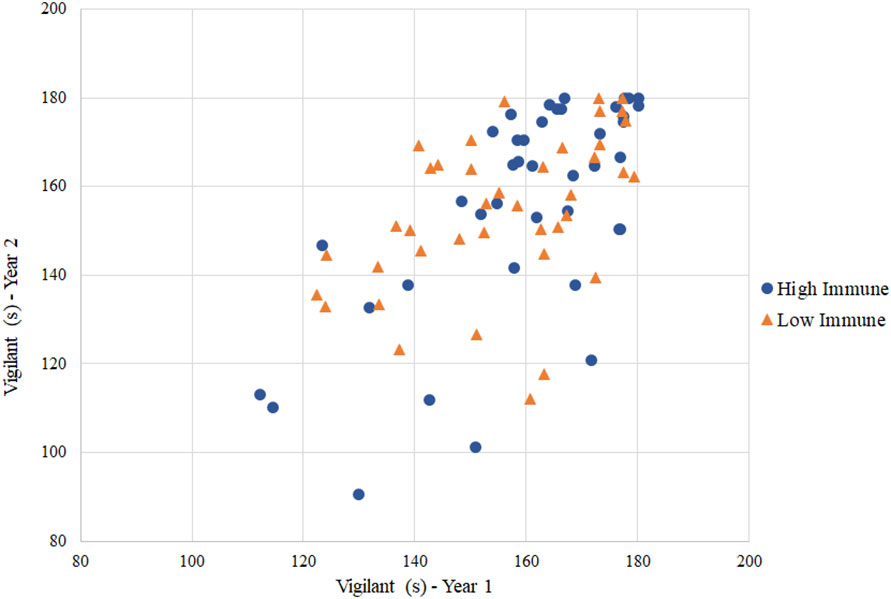
Figure 2 Scatterplot of duration of ‘vigilance’ in the dog test per year, colored by immune group. Immune group status was not a significant predictor term in the ‘vigilance’ model (χ2(4, N=99) = 0.22, P=0.64).
3.3 Behavioral activity and HPA-axis activity
According to repeated measures analysis, there were no obvious relationships between change in cortisol concentrations and durations of behavior from the dog and human challenge tests. There did appear, however, to be a relationship between change in cortisol concentration and reactivity in isolation (Figure 3). Adding change in cortisol concentrations as a linear predictor showed improvement of fit over the null model for the visual isolation model (χ2(4, N=99) = 42.72, P<0.001). When looking at relationships between variables within year using residual data, there was a significant positive relationship identified between post-test cortisol concentrations and duration of vigilance (rs=0.21, P=0.04) and a significant negative relationship with duration of time near the dog door (rs=-0.29, P=0.01) in year 1, within the dog challenge test. Like what was found with parametric analysis, there was a significant negative relationship identified between post-test cortisol concentrations and visual isolation scores in the second year (rs= -0.28, P=0.01).
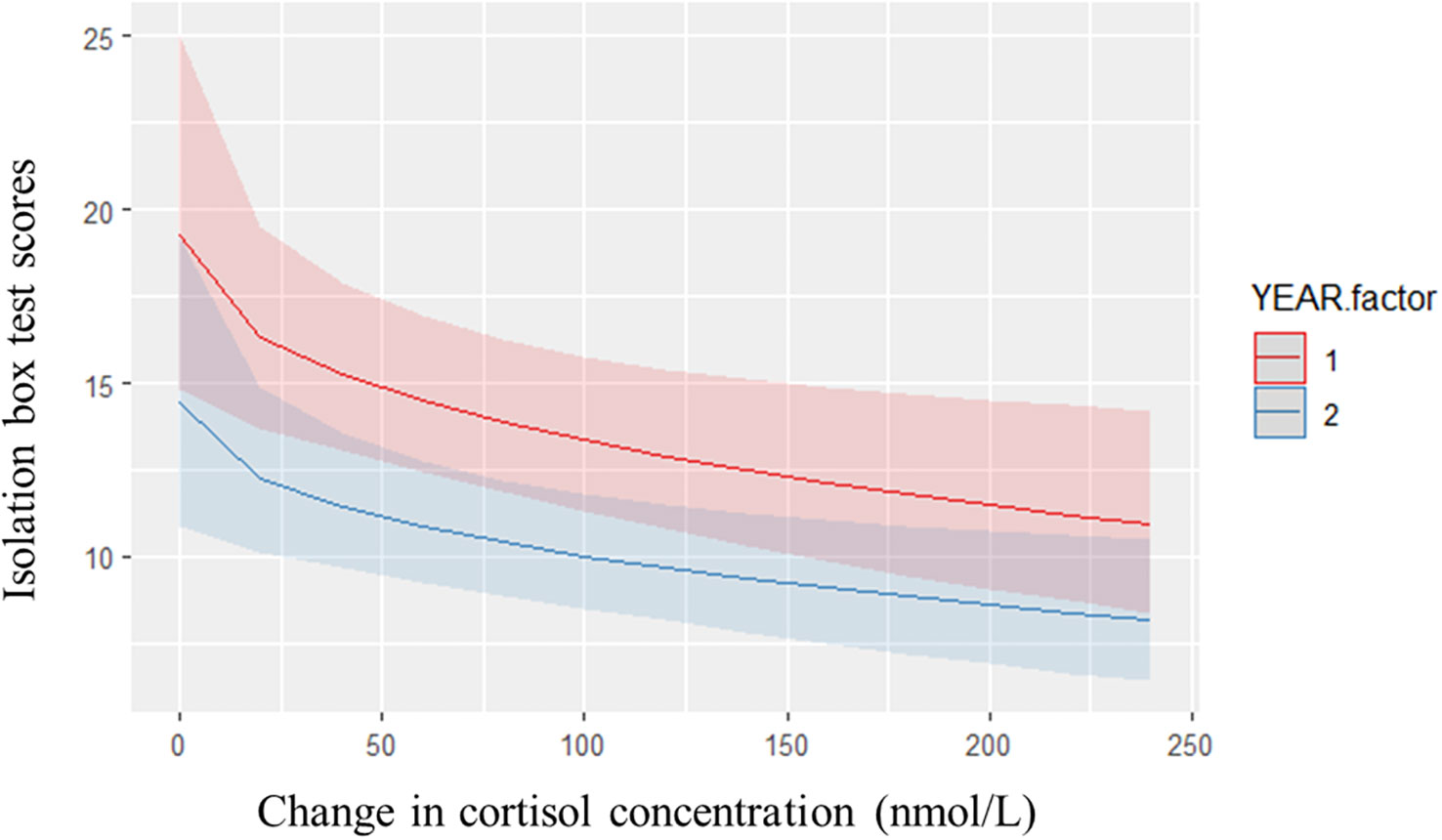
Figure 3 Predicted counts for isolation box test scores against change in cortisol concentrations, colored by year. Changes is cortisol concentrations significantly impacted changes in behavioral reactivity in the isolation box test (χ2(4, N=99) = 42.72, P < 0.001).
3.4 Immune responsiveness and HPA-axis activity
Addition of immune group to the pre-test, post-test and change in cortisol concentration models did not result in improvement of fit over the null model [pre-test (χ2(7, N=99) = 0.41, P= 0.52); post-test (χ2(7, N=99) = 0.33, P= 0.57); and change in cortisol (χ2(7, N=99) = 0.40, P= 0.53)].
3.5 Immune responsiveness and other biological indicators
Post hoc analysis of other biological indicators including weight and body temperature using logistic regression analysis indicated a relationship between weigh 1 and 2 and immune group. According to logistic regression, a one unit increase in ‘weigh1’ increased the odds of high immune responsiveness by 0.84 units and a one unit increase in ‘weigh 2’ increased the odds of high immune responsiveness by 0.86 units (Figures 4, 5). Holding all other variables constant, it was found that the odds of being categorized in the HR group increased by 0.51% (95% CL [0.16, 1.38]) for every one unit increase in weigh 1 and the odds of being categorized in the HR group increased by 43% (95% CL [1.54, 1.31]) for every one unit increase in weigh 2. There was also a breed by weight interaction detected for the immune group models.
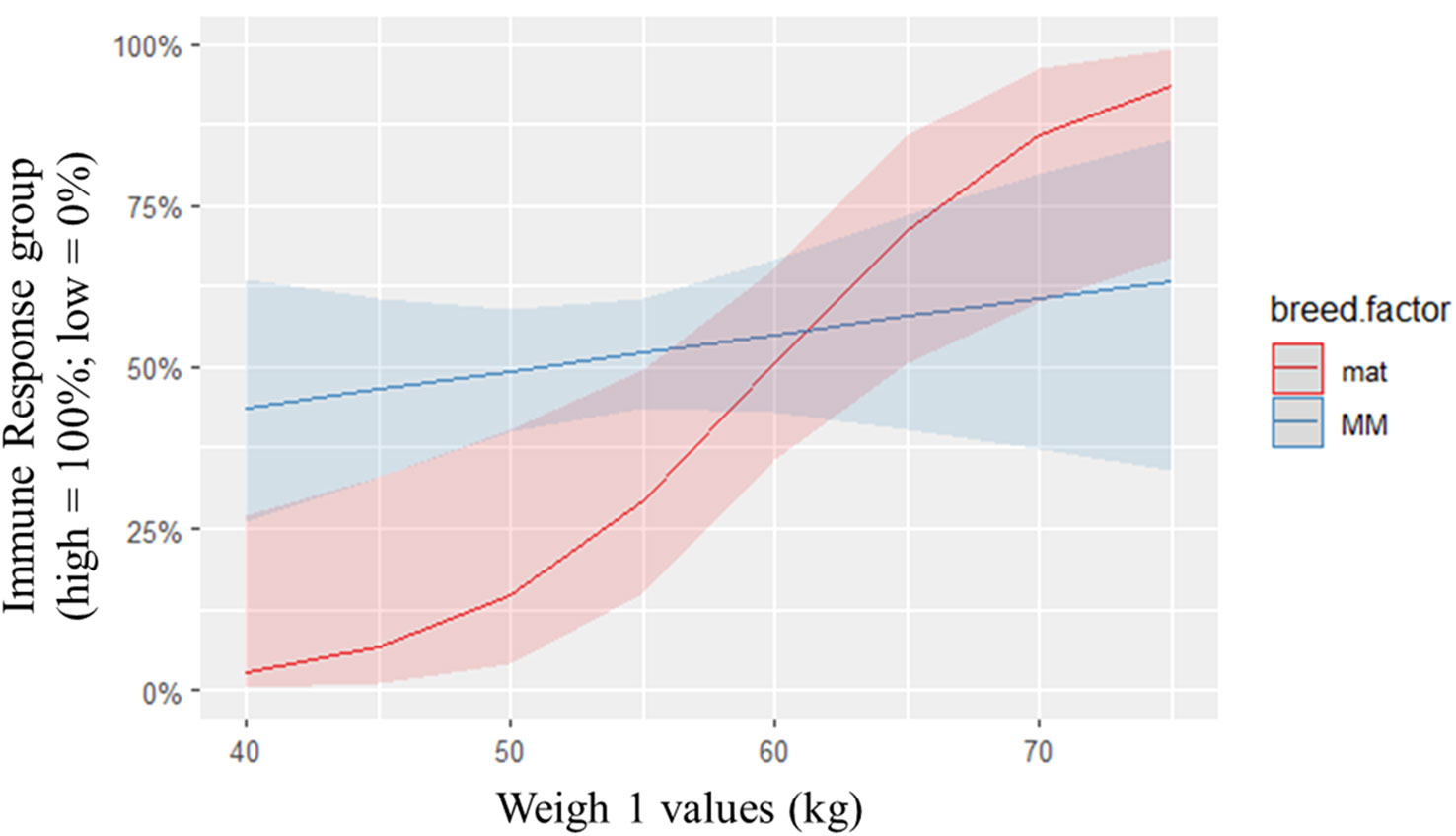
Figure 4 Predicted probabilities of immune response group against ‘weigh 1’, colored by breed. The odds of being categorized in the HR group increased by 0.51% (95% CL [0.16, 1.38]) for every one unit increase in weigh 1. There was a significant breed x weight interaction detected. As weight increased, the odds of being categorized in the HR group increased for maternal (mat) sheep over Merino (MM) sheep.
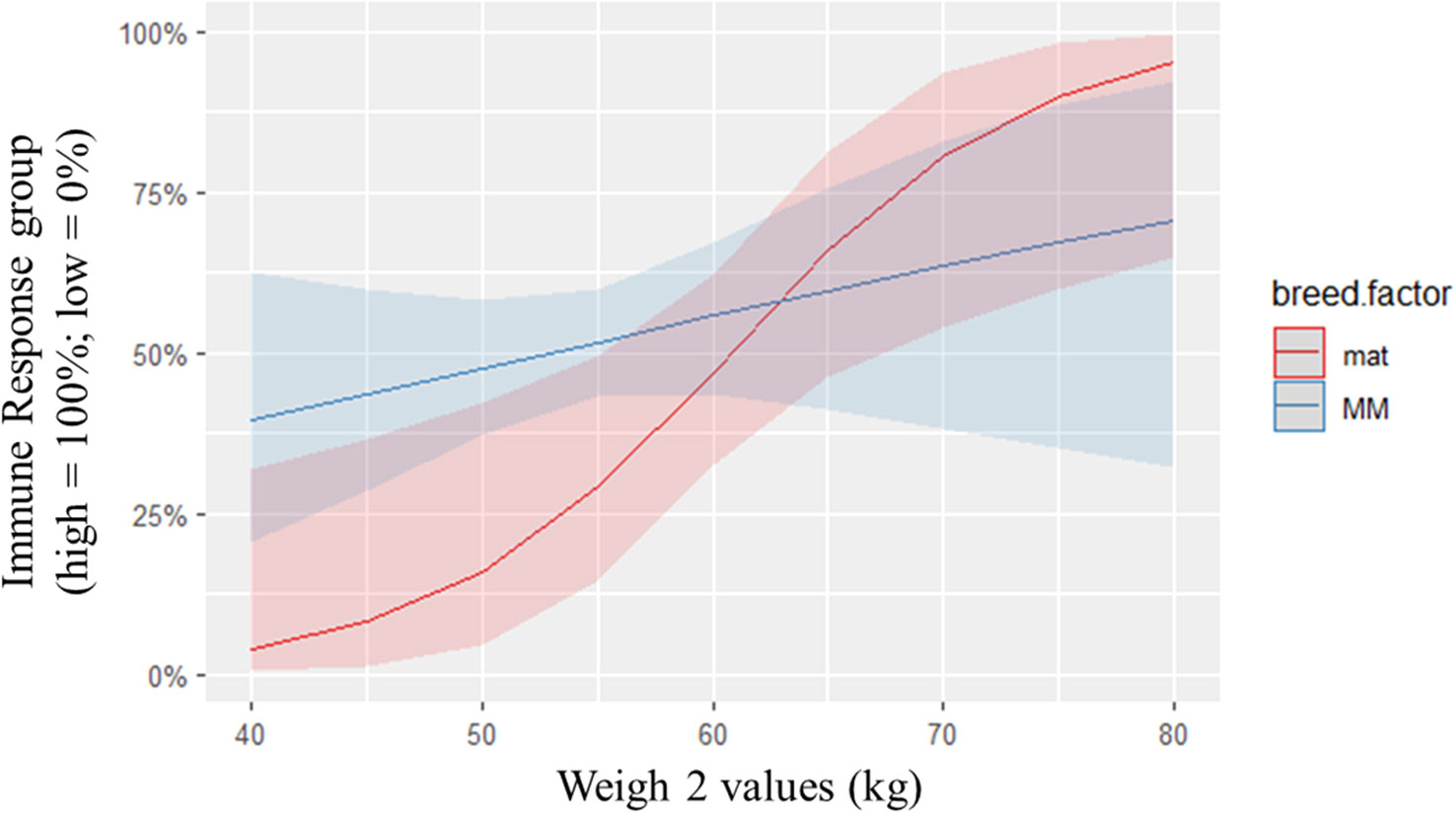
Figure 5 Predicted probabilities of immune response group against ‘weigh 2’, colored by breed. The odds of being categorized in the HR group increased by 43% (95% CL[1.54, 1.31]) for every one unit increase in weigh 2. There was a significant breed x weight interaction detected. As weight increased, the odds of being categorized in the HR group increased for maternal (mat) sheep over Merino (MM) sheep.
4 Discussion
The current study found no evidence of a relationship between immune responsiveness and behavioral reactivity to back-to-back threat tests, nor was there a relationship observed between immune responsiveness and cortisol concentrations. Previous studies have found mixed evidence regarding the relationship between immune responsiveness, stress and behavior in sheep. Generally, the results seem dependent on the specific immunological indicators being measured and the duration, type and intensity of stimulus being used. Importantly, in the current study, antibody and cell mediated immune responsiveness were combined into a single measure of CIR. This statistical procedure combines complex and potentially antagonistic pathways underpinning antibody and cell mediated mechanisms that may obscure more proximate relationships between immune function and specific behaviors. Similar to findings from Shamsi et al. (2023), there was no relationship identified between immune reactivity and behavioral responses observed in this study. Shamsi et al. (2023) also used a dog and human stimulus during novel object testing and reported that sheep infected with TG (Toxoplasma gondii) did not show altered behavior compared to uninfected sheep. Likewise, Destrez et al. (2012) found that most physiological indicators of chronic stress including heart rate, immune response and cortisol concentrations were not different between lambs that underwent a chronic stress treatment and controls. Delayed Hypersensitivity (DTH) responses to the clostridial vaccine were measured in the current study to reflect cell-mediated immune responses, and this response was calculated into total immune responsiveness to categorize sheep as high or low responders. Dharbhar and McEwen (1996) reported that DTH responses are enhanced during acute, non-invasive stressful experiences, and were further enhanced with increasing intensity. Chronic stress, on the other hand, may suppress DTH responses (Dharbhar and McEwen, 1996). Production of antibody (anti-tetanus toxoid serum IgG1) was used to reflect the antibody-mediated immune response, which was also included in the calculation to get overall immune responsiveness in sheep. Sutherland et al. (2019) found no relationship between behaviors observed in an open field test, stress (visual isolation) and total salivary immunoglobulin (Ig) A, however, there was a decrease in salivary IgA to the parasite antigen. Different from previous studies, this study did not challenge ewes that were actively mounting an immune response at the time of the test. It is possible that if ewes were tested shortly after receiving the vaccine (within 2 weeks), there may have been associations observed between serum IgG1 concentrations, DTH responses and behavioral and cortisol concentrations.
4.1 Individual consistency in behavioral and physiological response to threat
The current study identified context-specific repeatability in response to threat in relation to certain behaviors. Duration of vigilance, latency to investigate feed, zones crossed and frequency of pawing in response to a dog threat were all found to be consistent across years 1 and 2 of the study. In response to a human threat, high pitched vocalizations, low pitched vocalizations and duration of time spent in the zone closest to the human and conspecifics were also found to be consistent between the years. Responses to human threat in the arena test have been previously identified as a reliable indicator of individual differences in reactivity among sheep, and according to a review by Dodd et al. (2012), demonstrate moderate to high repeatability.
In the current study, flight speed following restraint and behavior within the isolation box test were repeatable over time. According to the review by Dodd et al. (2012), behaviors measured in restraint tests are often more repeatable and can be used as indicators of maternal behavior in sheep, suggesting that restraint tests may be more valuable when developing selection criterion in a flock. Flight speed post restraint has debatable value in terms of selection, and has weak association with carcass traits in lambs. Interestingly, response to restraint reflected in flight speed was found to be inconsistent across time by Blache and Ferguson (2005), and response to visual isolation as reflected by agitation in the isolation box test was identified to be repeatable across varying timelines (Blache and Ferguson, 2005; Murphy et al., 1994; Plush et al., 2011; Dodd et al., 2012). The discrepancy in the literature may be due to differences in time intervals between threat tests, as sheep in this study were run through a circuit of other tests prior to entering the isolation and restraint tests, as opposed to exposing animals to only one test in a single day.
Individual cortisol concentrations collected at the end of the test circuit, a reflection of general HPA-axis activation due to the cumulative challenge of the test circuit, were found to be consistent across years. The circuit of threat testing was intended to induce maximal cortisol responses in the sheep by exposing them to a range of stressors in order to determine low and high cortisol responders. Previous work has used a pharmacological induction of maximal cortisol response (i.e., ACTH) in sheep, rather than an environmental induction like the current study, to determine low and high cortisol responders (Lee et al., 2014). Lee et al. (2014) found that low cortisol responders showed more activity and were closer to the human in the arena test as compared to high cortisol responders. In the current study, a relationship between activity and proximity to human in the arena test was also found, however, sheep with lower cortisol levels were not found to be more active or closer to the human as Lee et al. (2014) reported. It can be projected that internally versus externally induced stress can manifest alternative behavioral strategies given the absence or presence of live feedback from threatening stimuli. Kilgour and Szantar-Coddington (1997) found no differences in adrenocortical responses within the arena test between two flocks selected for differences in fertility and Beausoleil et al. (2012) reported no consistent difference in cortisol concentrations between sheep selected based on activity level in the arena. The utility of cortisol reactivity as a selection trait is questionable (Kilgour and Szantar-Coddington, 1997), however, it may be relevant when considering stress levels experienced after intense handling and moving interventions (i.e., post-transport and during pre-slaughter) (Hemsworth et al., 2011). Identifying individuals that have consistently lower cortisol concentrations after handling may be valuable in terms of improving animal welfare (i.e., selecting individuals with higher stress resiliency).
4.2 Immune responsiveness and behavioral activity
As previously mentioned, there are mixed findings pertaining to the relationship between immune responsiveness and behavioral activity. Most studies in sheep that observe this relationship look at the dynamic between parasite burden and behavior. Grant et al. (2020) used a QBA (Qualitative Behavioral Assessment) to score sheep based on behavioral performance, to be compared against FEC (Fecal Egg Count) values and found that there was a tendency for observers to score sheep with high FECs as more docile, likely due to increased fatigue and reduced energy levels. Contrary to these results, Högberg et al. (2021) found that lambs with lower parasite loads had shorter daily lying times than lambs with higher parasite loads, which may have resulted from discomfort due to infection. Adams and Fell (1997) also found that abomasal nematodes influenced movement behavior of sheep in response to a human stimulus. Specifically, they observed that uninfected sheep made more moves during the test compared to infected sheep, and even showed smaller distances to approach the human. These results could be due to fatigue experienced in infected sheep, or even differences in emotional response to the human stimulus during testing, however this needs more investigation. Additionally, Gonzalez-Garduno et al. (2018) reported that behavior in sheep infected with gastrointestinal nematode is gestational state (pregnant vs. non-pregnant) and age dependent in that lactating ewes and lambs were more susceptible to gastrointestinal nematode compared to pregnant ewes, and lambs older than 300 days of age showed higher IgA relative to younger lambs.
The authors previously hypothesized that sheep belonging to the high immune responsiveness group would display increased levels of activity during circuit testing relative to sheep that belonged to the low immune responsiveness group. This hypothesis was informed by previous reports that pigs that were more active in an open environment had higher immune responsiveness (circulating pro-inflammatory cytokines) and decreased levels of cortisol relative to less active pigs. Other studies have found that acutely stress-inducing events can cause suppression (Sevi et al., 2001; Caroprese et al., 2010), enhancement (Dharbhar and McEwen, 1996) or have minimal to no effect (Destrez et al., 2012; Shamsi et al., 2023) on the immune response. Tuchscherer et al. (2009) found that pigs challenged with isolation for 4h caused a decrease in pro-inflammatory cytokines TNF-α, which could be maladaptive for the host that may need to prepare for a stressor or potential disease. Similarly, Caroprese et al. (2010) found that ewes that were more active during a 10 min isolation test had lower levels of TNF-α compared to more active sheep. On the other hand, Zhang et al. (2021) found that TNF-α concentrations were lower in “calm” animals, or those that were less active during an open field test. These authors inferred that this meant less active or more calm sheep have lower baseline levels of inflammatory signaling which may be advantageous due to reduced likelihood of oxidative stress. Sevi et al. (2001) found that sheep that were not challenged with re-grouping and relocation showed higher cell-mediated immune responses compared to sheep that were challenged. It is logical that sheep that are currently fighting an infection would have altered physiological activity that would consequentially affect behavioral responses. The results of the current study are most aligned with the recent report by Shamsi et al. (2023) finding no relationship between the response to a novel object or unfamiliar human and seroprevalence of TG. Authors also found that TG infection did not alter vocalization behavior. Immune responsiveness, as assessed in our study population, is expected to persist across the lifetime of the animal. However, in the current study, ewes were not actively mounting an immune response at the time of behavioral testing. Potential relationships between immune function and behavior may be more evident at a time when ewes are actively mounting an immune response or fighting an infection.
4.3 Behavioral activity and HPA-axis activity
Behavioral activity in response to a challenge and HPA-axis activation is a much more researched area in sheep, yet there is still disagreement in the literature on what the role is of serum cortisol concentrations when sheep are managing a stressor. In the current study, a negative relationship was found between change in cortisol concentrations and reactivity during visual isolation. In other words, sheep that were more active or agitated during visual isolation from the flock experienced less of an increase in serum cortisol levels and were perhaps experiencing a reduced negative, high arousal state compared to less active sheep. This is in line with our prediction that sheep which are less active in restraint would have increased cortisol concentrations relative to their more active counterparts. Caroprese et al. (2010) observed that shorter durations of bleating and movement (withdrawal behavior) during isolation were associated with higher cortisol concentrations. Additionally, Aydogdu and Karaca (2021) observed that increased cortisol concentrations were associated with decreased incidences of bleating and sniffing a human stimulus, which is also supported by Pajor et al. (2013). This indicates that sheep experiencing visual isolation and a negative state may be more likely to perform freeze or withdrawal behavior. Isolating sheep from the flock can be an incredibly stress or anxiety inducing event, and one strategy to cope with this challenge could be to become more immobile.
Some further explanations for this outcome could be due to variation in perception and previous experience with stress-inducing (Destrez et al., 2012) or positive events (Caroprese et al., 2006) altering perception of threat, and/or that HPA-axis activation is also dependent on type, duration, and intensity of stressor. Taken together, this could indicate that HPA-axis activation is affected by perception of the stressor, which is modulated by previous experience. Destrez et al. (2012) found that chronic exposure to aversive events actually decreased cortisol levels in lambs that were challenged with an unpredictable and uncontrollable circumstance. It is likely that these previous events altered the lamb’s perception and ability to cope with stressor and led them to developing to a mechanism for managing further aversive events. Caroprese et al. (2006) found that repeated gentling of sheep, assumed to be a pleasure-inducing event, also altered responses to visual isolation from the flock. Artificially reared lambs that had received gentling had reduced cortisol levels post-isolation compared to artificially reared lambs that did not receive gentling. Other studies have reported no such relationship between behavioral activity levels and cortisol concentrations following arena and open field testing (Beausoleil et al., 2012; Cakmakci et al., 2021).
Other relationships were identified through non-parametric analysis, between pre-test and post-test cortisol concentrations and behavioral responses to the dog and human challenge. In the first year of the study, there was a positive relationship identified between post-test cortisol levels and duration of vigilance in the dog test, and a negative relationship between post-test cortisol levels and duration of time near the dog door. This indicates that sheep that were more vigilant and less likely to stand near the zone where the dog was presented had increased cortisol concentrations. This finding aligns with previous studies, for example, Hemsworth et al. (2011) found increased dog use while moving sheep to cause increased levels of cortisol concentrations compared to no dog use. Additionally, sheep that were given an anxiogenic treatment prior to attention bias testing showed increased levels of vigilance towards the dog stimulus and increased levels of general vigilance in their environment (Lee et al., 2016). Thus, it is possible that sheep that had higher cortisol levels and exhibited increased vigilance and decreased locomotion (potentially freeze behavior) in the presence of the dog were experiencing a more negative arousal state compared to animals that had lower cortisol levels. This finding does, however, contrast with some previous work that found environmental induction of a chronic stress state caused decreased vigilance during attention bias testing (Verbeek et al., 2019), while exogenous stimulation of cortisol using ACTH had no impact on attention bias in sheep (Monk et al., 2019). The relationship between cortisol levels and these behaviors in the dog challenge were apparently not stable into the second year of testing. In the second year there was a nearly significant negative relationship identified between post-test cortisol concentrations and attention to the dog door and a nearly significant relationship between pre-test (baseline) cortisol concentrations and latency to sniff food. These results indicate that sheep that had higher cortisol levels post-testing paid less attention to the dog door and sheep that had higher pre-test cortisol concentrations had a longer latency to sniff food in the dog test. These findings are somewhat in conflict with the first-year evidence that sheep that were more vigilant or paid more attention to the dog door were perhaps in a state of greater stress or anxiety compared to individuals that were less vigilant. High latencies to sniff the food associated with high cortisol concentrations are in line with year 1 results and could be influenced by anxiety as well (Salvin et al., 2020). It should be stated, however, that the role of serum cortisol concentrations as they relate to anxiety levels in sheep is under reported. This supports the notion that the role of cortisol in behavioral expression is complex and still an area of confusion.
4.4 Immune responsiveness and HPA-axis activity
The current study hypothesized that animals that belong to the high immune responsiveness group would have a greater change in cortisol concentrations during testing relative to animals that belonged in the low immune responsiveness group. This third hypothesis was informed by the assumption that animals that are more susceptible to acute and chronic stress may experience suppressive effects of cortisol on the immune system and enhanced inflammatory responses (McEwen et al.,1997). This hypothesis was not supported by results of the current study, suggesting that there was no relationship between change in cortisol concentrations and inherent potential to mount specific cell mediated- and antigen-mediated responses in sheep. Caroprese et al. (2010) found that sheep with increased plasma cortisol concentrations (hyperactivity of the HPA-axis) had high immunological reactivity that could potentially increase inflammatory responses. These authors also found a lower CD4+/CD8+ (T-cell imbalance) ratio in sheep that exhibited higher cortisol concentrations compared to sheep exhibiting lower cortisol concentrations. Sheep that had lower immune responsiveness associated with high cortisol concentrations were perhaps more susceptible to on-coming disease. In the study of Lee et al. (2014), sheep with high and low reactivity to ACTH challenge did not differ in production of pro-inflammatory cytokines in response to subsequent intravenous challenge with the potent activator of the innate immune system, lipopolysaccharide. Tuchscherer et al. (2009) found that 4 h of isolation in piglets suppressed TNF-α concentration in vivo and pro-inflammatory cytokine production in vitro. Cytokine concentrations after isolation had a negative correlation with cortisol, suggesting that cortisol had a suppressive effect on the immune system. The contemporaneous associations Caroprese et al. (2010) and Tuchscerer et al. (2009) identified are interesting and holds implications for developing our understanding of the role of the HPA-axis and the immune system. In the current study, a link between immune responsiveness and subsequent HPA-axis function during stress was not observed.
4.5 Immune responsiveness and other biological indicators
Analysis outside of the three hypotheses listed was done to explore the relationship between immune responsiveness and other biomarkers such as weight and body temperature. The body temperature variables were unrelated to immune responsiveness, while weight measurements had a very strong relationship with immune profiles such that as weight increased so did the probability of being categorized in the high immune responsiveness group. Inherent immune responsiveness was related to weight, meaning that ewes with improved cell-mediated and antibody-mediated responses have greater body mass compared to animals that have reduced immune responsiveness. This contrasts with the associations seen between CIR and body weight in younger sheep (Hine et al., 2022). In these previous studies, phenotypic correlations between CIR and bodyweight up to 12 months of age were not significant. A possible explanation for these findings is that ewes that had more efficient immune responsiveness after a challenge were subsequently able to effectively fight infection prior to meeting any biological consequences related to anemia, anorexia, diarrhea- all of which can result in weight loss. Alternatively, it is possible that larger animals are more likely to have better immune responsiveness, however, the mechanism here is harder to theorize. Perhaps individuals with larger body mass have more resources and energy to mount an appropriate adaptive immune response to challenge. In either case, this relationship is preferred in the context of selection programs that seek to enhance productivity and immunity in sheep. Due to the significant breed x immune group interaction observed in this study, certain breeds and weights classes may also harness considerable benefit over others in terms of immune responsiveness.
5 Limitations
During the first year of testing, some sheep escaped from the circuit during or after the human threat test by jumping over a fence. These sheep were encouraged back into the yards to continue the circuit as quickly as possible but may have undergone additional stress due to this process. Additionally, to standardize timing for post-circuit blood sampling collection, sheep that took longer to get through the circuit were restrained for less than 5-minute duration. Multiple comparisons were conducted to assess the relationships between traits from the dog and human threat test with other variables, however, these analyzes were not subject to a Bonferroni adjustment, which may be considered a more conservative approach. The advantages of circuit testing include maximizing the number of experiments that can be completed in a shorter duration of time, however, performing a single test per day is recommended for future work as to avoid the confound of order effects. It is possible that sheep went through a sensitive age transition between years that influenced results in the second year. For example, Yu et al. (2021) reported time sensitive changes in relationships between observed behaviors in the arena test among juvenile Tibetan sheep, with some correlations emerging only after reaching sexual maturity. In the current study, however, ewes were mature animals. Immune responses were only measured once in the ewes, a year before behavioral testing, and not concurrently with the circuit assay.
6 Conclusion
The current study set out to investigate the relationships between immune responsiveness, behavior and the stress response. Findings suggest that there was no relationship to be observed between immune responsiveness and behavioral reactivity, nor immune responsiveness and stress response. This finding is important as it implies that the potential to mount a high or low immune response to vaccination may not be related to behavioral or HPA-axis outcomes during later stressful challenges. The combination of antibody-mediated and cell-mediated immune responses into a single score may obscure associations between CIR and individual behaviors that are themselves more proximally linked to individual (neuro)physiological pathways. Though behaviors during isolation may not indicate immune responsiveness, they may be used to identify animals that are more likely to enter a negative, high arousal state when separated from the flock during management procedures. Visually separating sheep from the flock is known to be highly stress-inducing and should be avoided when unnecessary. Perhaps the most relevant results in terms of selection programs would be the positive relationship between weight measurements and immune response as well as the individual consistency in stress response regardless of immune group. There appears to be the presence of context-dependent responses to a dog or human stimuli, with overlap in response to isolation and physical restraint. However, before we can draw strong conclusions about domain-general responses (i.e., consistent individual differences in threat response), it is suggested that future authors conduct additional longitudinal studies with more frequent replicates.
Data availability statement
The datasets presented in this study can be found in online repositories. The names of the repository/repositories and accession number(s) can be found below: The dataset generated in this experiment can be accessed through the CSIRO Data Access Portal [URL: https://doi.org/10.25919/zrsf-2s57].
Ethics statement
The protocol and conduct of the study were approved by the University of New England Animal Ethics Committee, under the New South Wales Animal Research Act 1985 (Animal Research Authority numbers 16-003 and 17-015).
Author contributions
Conceptualization, all authors. Methodology, JM, and CL. Formal analysis, KS and KH. Resources, CL. Data curation, JM. Writing—original draft preparation, KS and KH. Writing—review and editing, all authors. Visualization, KS and KH. Supervision, CL and KH. Project administration, JM. Funding acquisition, CL. All authors contributed to the article and approved the submitted version.
Funding
This work was supported by the Australian Commonwealth Scientific and Industrial Research Organisation (CSIRO) and the University of New England School of Environmental and Rural Science project expense support. JM was supported by the Sheep Cooperative Research Centre (Sheep CRC) and the Australian Commonwealth Government. Testing immune responsiveness of sheep was funded by Meat & Livestock Australia (Project B.LSM.0069). The funders had no role in study design, data collection and analysis, decision to publish, or preparation of the manuscript.
Acknowledgments
Thank you to the staff and students at CSIRO for their assistance during the experiments: Jim Lea, Sue Belson, Tim Dyall, Troy Kalinowski, Grant Uphill, Brian Dennison, Jody McNally, Jess Burton and Koli the dog. We thank Brad Hine, Ian Colditz, Geoff Hinch and Sam Clark for their contributions to experimental design and supervision during the experiments. We also thank Brad Hine for immuno-phenotyping the sheep and Ian Colditz for advice on the manuscript.
Conflict of interest
The authors declare that the research was conducted in the absence of any commercial or financial relationships that could be construed as a potential conflict of interest.
Publisher’s note
All claims expressed in this article are solely those of the authors and do not necessarily represent those of their affiliated organizations, or those of the publisher, the editors and the reviewers. Any product that may be evaluated in this article, or claim that may be made by its manufacturer, is not guaranteed or endorsed by the publisher.
References
Adams D. B., Fell L. R. (1997). The effect of infection with the abomasal nematode, Haemonchus contortus, on the Avoidance Behavior of sheep in a motivation-choice test. Intl. J. Parasitol. 27 (6), 665–673. doi: 10.1016/S0020-7519(97)00021-0
Aydogdu N., Karaca S. (2021). The effect of behavioral reactivity on maternal behavior and offspring growth performance in Norduz ewes. Appl. Anim. Behav. Sci. 242, 1–8. doi: 10.1016/j.applanim.2021.105419
Barber I., Dingemanse N. J. (2010). Parasitism and the evolutionary ecology of animal personality. Philosophical Transactions of the Royal Society B. Biol. Sci. 365, 4077–4088. doi: 10.1098/rstb.2010.0182
Beausoleil N. J., Blacke D., Stafford K. J., Mellor D. J., Noble A. D. L. (2012). Selection for temperament in sheep: Domain-general and context specific traits. Appl. Anim. Behav. 139, 74–85. doi: 10.1016/j.applanim.2012.02.020
Bickell S., Poindron P., Nowak R., Chadwick A., Ferguson D., Blache D. (2009). Genotype rather than non-genetic behavioural transmission determines the temperament of merino lambs. Anim. Welfare 18 (4), 459–466.
Blache D., Ferguson D. (2005). Genetic estimates for temperament traits in sheep breeds. Meat Livestock Australia Sydney.
Borhetti P., Saleri R., Mocchegiani E., Corradi A., Martelli P. (2009). Infection, immunity and the neuroendocrine response. Vet. Immuno. And Immunopath. 130, 141–162. doi: 10.1016/j.vetimm.2009.01.013
Burgunder J., Petrezelkova K. J., Modry D., Kato A., MacIntosh A. J. J. (2018). Fractal measures in activity patterns: Do gastrointestinal parasites affect the complexity of sheep behavior? Appl. Anim. Behav. Sci. 205, 44–53. doi: 10.1016/j.applanim.2018.05.014
Cakmakci C., Karaca S., Maria G. A. (2021). Does coping style affect behavioral responses and growth performance of lambs weaned at different ages? J. @ Vet. Behav. 42, 64–74. doi: 10.1016/j.jveb.2020.10.009
Caroprese M., Albenzio M., Marzano A., Schena L., Annicchiarico G., Sevi A. (2010). Relationship between cortisol response to stress and behavior, immune profile, and production performance of dairy ewes. J. Dairy Sci. 93, 2395–2403. doi: 10.3168/jds.2009-2604
Caroprese M., Napolitano F., Albenzio M., Annicchiarico G., Musto M., Sevi A. (2006). Influence of gentling on lamb immune response and human-lamb interactions. Appl. Anim. Behav. Sci. 99, 118–131. doi: 10.1016/j.applanim.2005.08.023
Cartwright S. L., Schmied J., Karrow N., Mallard B. A. (2023). Impact of heat stress on dairy cattle and selection strategies for thermotolerance: a review. Front. veterinary Sci. 10. doi: 10.3389/fvets.2023.1198697
Ciliberti M. G., Albenzio M., Ighese C., Santillo A., Marino R., Sevi A., et al. (2017). Peripheral blood mononuclear cell proliferation and cytokine production in sheep. J. Dairy Sci. 100, 750–756. doi: 10.3168/jds.2016-11688
Destrez A., Deiss V., Leterrier C., Boivin X., Boissy A. (2012). Long-term exposure to unpredictable and uncontrollable aversive events alters fearfulness in sheep. Animal. 7 (3), 476–484. doi: 10.1017/S1751731112001796
Dharbhar F. S., McEwen B. S. (1996). Stress-induced enhancement of antigen-specific cell mediated immunity. J. Immunol 156 (7), 2608–2614. doi: 10.4049/jimmunol.156.7.2608
Dodd C. L., Pitchford W. S., Hocking Edwards J. E., Hazel S. J. (2012). Measures of behavioral reactivity and their relationships with production traits in sheep: A review. Appl. Anim. Behav. Sci. 140, 1–15. doi: 10.1016/j.applanim.2012.03.018
Gonzalez-Garduno R., Mendoza-de Gives P., Lopenz-Arellano M. E., Aguilar-Marcelino I., Torres-Hernandez G., Ojeda-Robertos N. G., et al. (2018). Influence of the physiological stage of Blackbelly sheep on immunological behavior against gastrointestinal nematodes. Exp. Parasitol. 193, 20–26. doi: 10.1016/j.exppara.2018.08.003
Grant E. P., Wickham S. I., Anderson F., Barnes A. L., Flemin P. A., Miller D. W. (2020). Behavioral assessment of sheep is sensitive to level of gastrointestinal parasite infection. Appl. Anim. Behav. Sci. 223, 1–9. doi: 10.1016/j.applanim.2019.104920
Hemsworth P. H., Rice M., Karlen M. G., Calleja L., Barnett J. L., Nash J., et al. (2011). Human-animal interactions at abattoirs: Relationships between handling and animal stress in sheep and cattle. Appl. Anim. Behav. Sci. 135, 1–2, 24–33. doi: 10.1016/j.applanim.2011.09.007
Hine B. C., Acton G. A., Elks D. J., Niemeyer D. D. O., Bell A. M., Colditz I. G., et al. (2022). Targeting improved resilience in Merino sheep - Correlations between immune competence and health and fitness trait. Animal 16, 100544. doi: 10.1016/j.animal.2022.100544
Hine B. C., Bell A. M., Niemeyer D. D. O., Duff C. J., Butcher N. M., Dominik S., et al. (2019). Immune competence traits assessed during the stress of weaning are heritable and favourably genetically correlated with temperament traits in Angus cattle. Journal of. Anim. Sci. 97, 4053–4065. doi: 10.1093/jas/skz260
Hine B. C., Bell A. M., Niemeyer D. D. O., Duff C. J., Butcher N. M., Dominik S., et al. (2021). Associations between immune competence phenotype and feedlot health and productivity in Angus cattle. J. Anim. Sci. 99, skab016. doi: 10.1093/jas/skab016
Högberg N., Hessle A., Lidfors L., Enweji N., Hoglund J. (2021). Nematode parasitism affects lying time and overall activity patterns in lambs following pasture exposure around weaning. Vet. Parasitol. 296, 1–6. doi: 10.1016/j.vetpar.2021.109500
Kilgour R. J., Szantar-Coddington M. R. (1997). The arena test and cortisol response of sheep as indirect selection criteria for the improvement of lamb survival. Anim. Repro. Sci. 46, 97–108. doi: 10.1016/S0378-4320(96)01591-6
Koolhaas J. M. (2008). Coping style and immunity in animals: Making sense of individual variation. Brain Behav. Immun. 22, , 662–, 667. doi: 10.1016/j.bbi.2007.11.006
Lea J. M., Niemeyer D. D. O., Reed M. T., Fisher A. D., Ferguson D. M. (2008). Development and validation of a simple technique for logging body temperature in free-ranging cattle. Aust. J. Exp. Agric. 48 (7), 741–745.
Lee T. K., Lee C., Bischof R., Lambert G. W., Clarke I. J., Henry B. A. (2014). Stress-induced behavioral and metabolic adaptations lead to an obesity-prone phenotype in ewes with elevated cortisol responses. Psychoneuroendocrinology. 47, 166–1177. doi: 10.1016/j.psyneuen.2014.05.015
Lee C., Verbeek E., Doyle R., Bateson M. (2016). Attention bias to threat indicates anxiety differences in sheep. Biol. Lett. 12, 20150977. doi: 10.1098/rsbl.2015.0977
McEwen B. S., Biron C. A., Brunson K. W., Bulloch K., Chambers W. H., Dhabhar F. S., et al. (1997). The role of adrenocorticoids as modulators of immune function in health and disease: neural, endocrine and immune interactions. Brain Res. Rev. 23 (1-2), 79–133.
Mallard B. A., Emam M., Paibomesai M., Thompson-Crispi K., Wagter-Lesperance L. (2015). Genetic selection of cattle for improved immunity and health. Japanese J. Veterinary Res. 63, S37–S44. doi: 10.14943/jjvr.63.suppl.s37
Mallard B. A., Wilkie B. N., Kennedy B. W., Quinton M. (1992). Use of estimated breeding values in a selection index to breed Yorkshire pigs for high and low immune and innate resistance factors. Anim. Biotechnol. 3, 257–280. doi: 10.1080/10495399209525776
Monk J. E., Belson S., Lee C. (2019). Pharmacologically-induced stress has minimal impact on judgement and attention biases in sheep. Sci. Rep. 9 (1), 11446. doi: 10.1038/s41598-019-47691-7
Monk J. E., Doyle R. E., Colditz I. G., Belson S., Cronin G. M., Lee C. (2018). Towards a more practical attention bias test to assess affective state in sheep. PLoS One 13 (1), e0190404. doi: 10.1371/journal.pone.0190404
Murphy P. M., Purvis I. W., Lindsay D. R., Le Neindre P., Orgeur P., Poindron P. (1994). “Measures of temperament are highly repeatable in merino sheep and some are related to maternal behaviour,” in Proceedings of the Australian Society of Animal Production, Vol. 20. 247–250.
Pajor F., Kovacs A., Tozser J., Poti P. (2013). The influence of temperament on cortisol concentrations and metabolic profile in Tsigai lambs. Archives Anim. Breed. 53 (4), 465–474. doi: 10.7482/0003-9438-56-056
Pinard M.-H., van Arendonk J. A. M., Nieuwland M. G. B., van der Zijpp A. J. (1992). Divergent selection for immune responsiveness in chickens: estimation of realized heritability with an animal model1. J. Anim. Sci. 70, 2986–2993. doi: 10.2527/1992.70102986x
Plush K. J., Hebart M. L., Brien F. D., Hynd P. I. (2011). The genetics of temperament in Merino sheep and relationships with lamb survival. Appl. Anim. Behav. Sci. 134, 130–135. doi: 10.1016/j.applanim.2011.07.009
R Development Core Team (2018). R: a language and environment for statistical computing (Vienna: R Foundation for Statistical Computing).
Rauw W. M., Kanis E., Noordhuizen-Stassen E. N., Grommers F. J. (1998). Undesirable side effects of selection for high production efficiency in farm animals, a review. Livestock. Production Sci. 56, 15–33. doi: 10.1016/S0301-6226(98)00147-X
Reverter A., Hine B. C., Porto-Neto L., Li Y., Duff C. J., Dominik S., et al. (2021). ImmuneDEX: a strategy for the genetic improvement of immune competence in Australian Angus cattle. J. Anim. Sci. 99, skaa384. doi: 10.1093/jas/skaa384
Roger P. A. (2008). The impact of disease and disease prevention on sheep welfare. Small Rumin. Res. 76, 104–111. doi: 10.1016/j.smallrumres.2007.12.005
Salvin H., Café L., Lees A., Morris S., Lee C. (2020). A novel protocol to measure startle magnitude in sheep. Appl. Anim. Behav. Sci. 228, 1–9. doi: 10.1016/j.applanim.2020.104996
Sapolsky R. M., Romero L. M., Munch A. U. (2000). How do glucocorticoids influence stress responses? Integrating permissive, suppressive, stimulatory, and preparative actions. Endo. Rev. 21 (1), 55–89. doi: 10.1210/edrv.21.1.0389
Schiller K., McVey C., Doyle S., Horback K. (2020). Chute scoring as a potential method for assessing individual differences in arousal among ewes. Appl. Anim. Behav. Sci. 230, 1–9. doi: 10.1016/j.applanim.2020.105073
Selye H. (1946). The general adaptation syndrome and the diseases of adaptation. J. Clin. Endocrin. Metab. 6 (2), 117–230. doi: 10.1210/jcem-6-2-117
Sevi A., Taibi L., Albenzio M., Muscio A., Dell’Aquila S. D., Napolitano F. (2001). Behavioral, adrenal, immune and productive responses of lactating ewes to regrouping and relocation. J. Anim. Sci. 79 (6), 1457–1465. doi: 10.2527/2001.7961457x
Shamsi S., Fahey H., Rast L., Freire R. (2023). Is Toxoplamsa gondii infection related to spatial problem solving and fear response in sheep? Appl. Anim. Behav. Sci. 263, 1–6. doi: 10.1016/j.applanim.2023.105933
Sternberg E. M. (2006). Neural regulation of innate immunity: a coordinated nonspecific host response to pathogens. Nat. Revs. Immuno. 6, 318–328. doi: 10.1038/nri1810
Sutherland M., Dowling S., Shaw R., Hickey J., Fraser D., Cameron C., et al. (2019). Stress-induced immunomodulation in low and high reactive sheep. Animals. 9 (3), 1–13. doi: 10.3390/ani9030104
Tuchscherer M., Kanitz E., Puppe B., Tuchscherer A., Viergutz T. (2009). Changes in endocrine and immune responses of neonatal pigs exposed to a psychosocial stressor. Res. Vet. Sci., 87 (3), 380–388.
van der Zijpp A. J. (1983). Breeding for immune responsiveness and disease resistance. World's Poultry Sci. J. 39, 118–131. doi: 10.1079/WPS19830012
Verbeek E., Colditz I., Blache D., Lee C. (2019). Chronic stress influences attentional and judgement bias and the activity of the HPA axis in sheep. PLoS One 14 (1), e0211363. doi: 10.1371/journal.pone.0211363
Wiegertjes G. F., Stet R. M., Parmentier H. K., van Muiswinkel W. B. (1996). Immunogenetics of disease resistance in fish: a comparative approach. Dev. Comp. Immunol. 20, 365–381. doi: 10.1016/S0145-305X(96)00032-8
Wilkie B., Mallard B. (1999). Selection for high immune response: an alternative approach to animal health maintenance? Veterinary Immunol. Immunopathology 72, 231–235. doi: 10.1016/S0165-2427(99)00136-1
Yu Y., Wang Y., Zhong L., Zhu H., Qu J. (2021). Behavioral and physiological traits in yearling Tibetan sheep (Ovis aries). Anim 11, 1–10. doi: 10.3390/ani11061676
Keywords: behavior, stress, immune responsiveness, sheep, immunity
Citation: Schiller K, Monk JE, Lee C and Horback K (2023) Associations between immune competence phenotype and stress response in sheep. Front. Anim. Sci. 4:1160202. doi: 10.3389/fanim.2023.1160202
Received: 06 February 2023; Accepted: 23 August 2023;
Published: 19 September 2023.
Edited by:
Pasquale De Palo, University of Bari Aldo Moro, ItalyReviewed by:
Edna Hillmann, Humboldt University of Berlin, GermanyTakashi Bungo, Okayama University of Science, Japan
Copyright © 2023 Schiller, Monk, Lee and Horback. This is an open-access article distributed under the terms of the Creative Commons Attribution License (CC BY). The use, distribution or reproduction in other forums is permitted, provided the original author(s) and the copyright owner(s) are credited and that the original publication in this journal is cited, in accordance with accepted academic practice. No use, distribution or reproduction is permitted which does not comply with these terms.
*Correspondence: Kristina Horback, a21ob3JiYWNrQHVjZGF2aXMuZWR1