- 1Centre de recherche et développement de Sherbrooke, Agriculture & Agri-Food Canada, Sherbrooke, QC, Canada
- 2Centre de recherche et développement de Québec, Agriculture & Agri-Food Canada, Québec, QC, Canada
This mini-review was undertaken to demonstrate the impact of trace mineral feeding management of dairy cows on the ecosystem by discussing their role in the animal metabolism, dietary recommendations, current feeding practices, and their excretion in manure pertaining to five trace minerals, i.e., cobalt, copper, iron, manganese, and zinc. The first part of this mini-review relates the importance of trace minerals in dairy cow metabolism, and how recommendations are obtained. The following section showed that the transition period from the dry to the lactating phase is challenging for dairy cattle, and current trace mineral recommendations have been questioned for this period due to the role of some trace minerals in immunity and oxidative metabolism. Furthermore, trace mineral overfeeding is a common practice in intensive dairy production system in Canada, the USA, and the UK, which is far from precision nutrition. Trace minerals in excess of requirements are directly excreted into the manure. The practice of trace mineral overfeeding could have detrimental effects on the ecosystem when manure with high trace mineral concentrations is repeatedly spread on fields. In conclusion, an integrative approach assessing the impact of trace mineral overfeeding in cow diets on the ecosystem is needed.
1 Introduction
Worldwide, agriculture is facing growing challenges. Indeed, there is a societal pressure for producing more food products in a sustainable manner while using less inputs. Achieving this will require a holistic approach that connects all systems together. It has been known for many years that excesses of phosphorus (P) and protein in cow rations augment P and nitrogen (N) excreted in manure, respectively (Wattiaux and Karg, 2004; Senaratne and Plaizier, 2020), that could in turn impact water and air quality (Powell and Broderick, 2011). The concept of precision feeding has emerged to mitigate P and N excretion into the environment by insuring an adequacy between dietary supply and cow requirements (Cerosaletti et al., 2004). While the impact of P and N excretion in the environment has been widely studied in dairy cows, the effect of excessive dietary trace minerals (TM) in cow rations on the ecosystem has received little attention. Nevertheless, due to the use of high doses of copper (Cu) and zinc (Zn) in pig feed for their antimicrobial properties, the impact of pig slurry spreading with high concentrations of Cu and Zn on the water-soil-plant continuum has been assessed (Jondreville et al., 2002; Legros et al., 2013; De Conti et al., 2016). Qian et al. (2018) concluded that pig manure application with high concentrations of Cu and Zn will cause an environmental risk in surface soil within the next 10-50 years if high supplementation of Cu and Zn in pig feed and additives continues. Although such high amounts of Cu and Zn are not used in dairy production, previous surveys have shown that cows receive rations containing higher TM levels than the recommendations in many countries (Li et al., 2005; Sinclair and Atkins, 2015; Duplessis et al., 2021).
The objective of this mini-review is to demonstrate the importance of considering the impact of cow TM feeding management on the ecosystem by discussing TM role in the animal metabolism, dietary recommendations, current feeding practices, and TM excretion in manure pertaining to five TM, i.e., cobalt (Co), Cu, iron (Fe), manganese (Mn), and Zn.
2 Importance of trace minerals for dairy cows and dietary recommendations
It has been well recognized that some TM have essential roles in cow metabolism (National Academies of Sciences, Engineering, Medicine (NASEM, 2021). A complete review has been published on functions and absorption mechanisms of TM in dairy cows (Goff, 2018). This is beyond the scope of this mini-review to describe in details the role of studied TM. Briefly, cows do not have Co requirements per se, but Co is needed by ruminal bacteria to synthesize vitamin B12 (Martens et al., 2002), which is necessary for cow metabolism. Copper is an essential component of many enzymes, such as cytochrome oxidase, required for electron transport during aerobic respiration, superoxide dismutase, for cell protection of adverse effects of oxygen metabolites (Goff, 2018). It is also related to carbohydrate and lipid metabolism and bone formation (Suttle, 2022). Iron has a role of coenzymes amongst others in the electron transport chain, but its primary function is being a component of heme in hemoglobin and myoglobin (Goff, 2018). Manganese and Zn are also a component of superoxide dismutase for cell protection. Manganese is a component of several enzymes involved, for instance, in carbohydrate and lipid metabolism and reproduction (Suttle, 2022). Zinc is a component of over 200 enzymes related to carbohydrate, protein, and lipid metabolism (NASEM, 2021), keratin generation, and many more. Copper, Fe, and Zn also have a role in immune function, as their deficiency increases cow susceptibility to disease, such as mastitis (Yang and Li, 2015). In the same line, being involved in keratin generation, Zn has a role in teat keratin formation, which is a natural and physical barrier against bacteria to enter into the mammary gland and cause mastitis (Wilde, 2006). It is worth noting that Cu, Fe, Mn, and Zn could all act as pro- and antioxidants (Goff, 2018).
Cow requirements for Co, Cu, Fe, Mn, and Zn were established about 50 years ago by the National Research Council (NRC) in the USA and have been recently updated (NASEM, 2021). For selected TM, except Co, a factorial approach is used to determine requirements (NASEM, 2021), meaning that cow TM requirements are based on the summation of the nutrients needed for maintenance, milk production, pregnancy, and growth, if applicable. Regarding Co, a fixed dietary concentration regardless of the lactation stage or pregnancy is used. It should be noted that other requirement references are available, such as those from the Institut National de la Recherche Agronomique (INRA, 2018) in France and the European Federation of Animal Science (EAAP Scientific Series, 2011) in The Netherlands. These last 2 references use static dietary requirements for all selected TM, regardless of the status of the animal. It is worth mentioning that there is no dietary Fe recommendation in INRA (2018), because it is considered that the adult cow diet with typical ingredients provides greater Fe than the animal requirement. Unfortunately, agreements in TM recommendations are rare among the different sources (Suttle, 2022), and recommendations have changed over the years with novel knowledge (Table 1). Comparisons of recommendations from previous NRC (2001) and current NASEM (2021) showed that Co, Mn, and Zn recommendations have increased, Cu decreased, and no change for Fe. It should also be noted that maintenance requirement has increased due to a revision of the literature, and absorption coefficients have been revised for most studied TM. For each essential mineral, there is a range of dietary supply that optimizes animal health; above that, the maximum tolerable level and even toxicity might be reached, and below that, the animal can be deficient. Either situation (i.e., deficiency and toxicity) might be detrimental for animals and should be avoided (NRC, 2005). The maximum tolerable level is defined as “the dietary level that, when fed for a defined period of time, will not impair animal health or performance” (NRC, 2005). In the NASEM (2021), Co, Fe, and Mn recommendations are based on adequate intake and Cu and Zn recommendations based on estimated average requirements. Adequate intake is defined as “the daily nutrient intake to meet or exceed the requirements based on limited experimental data” and estimated average requirements as “average daily nutrient intake estimated to meet the requirements of half of the healthy individuals” (Institude of Medicine, 2006).
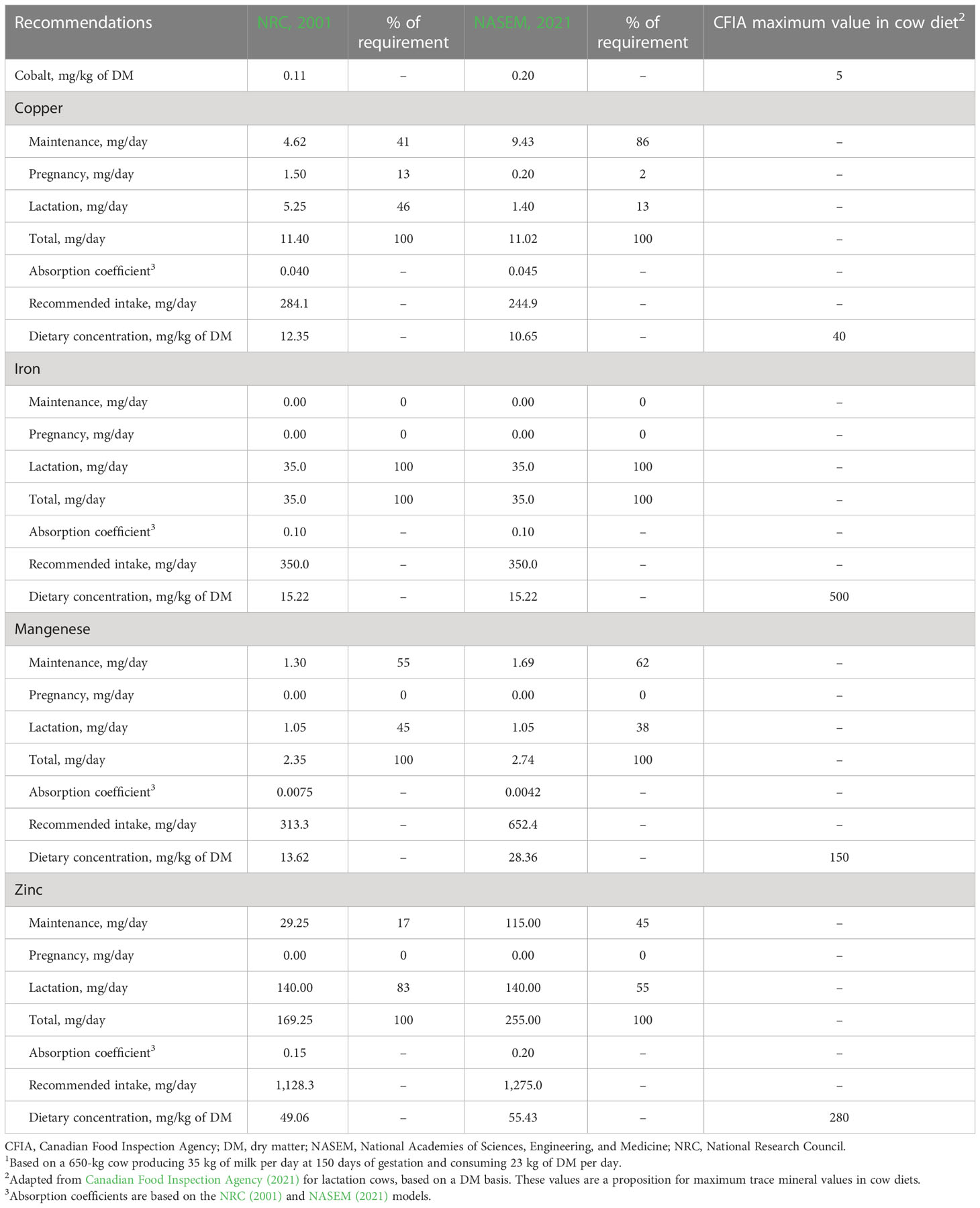
Table 1 Comparison between previous dietary recommendations of cobalt, copper, iron, manganese, and zinc in dairy cows from National Research Council and current from National Academies of Sciences, Engineering, and Medicine1.
3 Current feeding practices regarding selected trace minerals in dairy cows
The previous section stressed that some TM are essentials for dairy cows, and this is why they should be found in animal diets. It has been reported that bioavailability of some TM in home-grown feedstuffs is relatively low (NRC, 2001). Moreover, it is well documented that TM interactions occur in the rumen through TM binding with fiber fractions or the formation of insoluble metal complexes, such as with Cu, molybdenum, and sulfur (Spears, 2003), which also decrease their bioavailability. Hence, it is a common practice to supplement cow diets with TM, either with inorganic or organic forms, or a blend of both (Nocek et al., 2006). To cope with the low bioavailability of ingredients, one can formulate rations having higher TM concentrations than needed as an insurance to fulfill cow requirements (López-Alonso, 2012). A survey has reported that 66% of Canadian nutritionists had intentionally formulated a ration above their software criteria for some TM (Duplessis et al., 2023). In a review, López-Alonso (2012) stated that there are 2 situations regarding TM feeding strategy: the case of intensive and extensive farming. The former relies on high producing animals receiving commercial TM supplement inputs which are often given in amounts greater than the animal needs. The latter aims to limit farm inputs including TM supplementation, and relies on homegrown crop to fulfill TM requirements, which could lead to deficiency depending on soil composition (Grace and Knowles, 2012). According to Suttle (2022), in some situations, cow TM requirements can mostly be fulfilled by TM naturally present in feeds and forages. In the same line, Duplessis et al. (2021) reported that forages included in the diet were apparently sufficient in most herds to fulfill Co, Fe, and Mn requirements of lactating cows.
Cross-sectional studies have been conducted in some countries to evaluate TM dietary supply in accordance to the recommendations (Li et al., 2005; Hristov et al., 2007; Castillo et al., 2013; Sinclair and Atkins, 2015; Duplessis et al., 2021). A survey conducted in 54 Wisconsin dairy herds revealed that 50 and 94% of farms feeding total mixed ration had Zn and Cu concentrations exceeding NRC recommendations, respectively (Li et al., 2005). They concluded, however, that this oversupplying was unlikely to reach toxicity leading to animal health issues. Castillo et al. (2013) also found that diets from 39 Californian herds provided TM greater than the NRC recommendations; centile 10th and 90th ranging between 16 and 196, 1,940 and 3,924, 281 and 601, and 34 and 105% over the recommendations for Cu, Fe, Mn, and Zn, respectively. Except for Fe, dietary Cu, Mn, and Zn did not exceed the maximum tolerable levels for dairy cows. Hristov et al. (2007) found that diets of lactating cow on 6 Idaho farms had Cu and Zn concentrations exceeding NRC recommendations. In central and northern England dairy farms, Sinclair and Atkins (2015) observed that both early and late lactation rations had Cu, Fe, Mn, and Zn concentrations well above NRC requirements; with early lactation diets containing more TM per kg of dry matter (DM) than late lactation diets. The average dietary Cu, Fe, Mn, and Zn concentrations exceeded the NRC recommendations by respectively 87, 3040, 654, and 106% in early lactation (Sinclair and Atkins, 2015). In 100 Canadian dairy herds, Duplessis et al. (2021) concluded that dietary supplies of Co, Cu, Fe, Mn, and Zn were higher than the NRC recommendations, but the adequacy between supply and requirement was better for cows having less than 21 days in milk than thereafter in the lactation. In that cross-sectional study, the median percentages of supply above the recommendations for Co, Cu, Fe, Mn, and Zn were 405, 52, 1,460, 372, and 65%, respectively (Duplessis et al., 2021). The current management of overfeeding TM can cause TM accumulation in cow body and have detrimental health impact (Daniel et al., 2023). A case of chronic Cu poisoning in one UK herd feeding a ration containing 50 mg of Cu/kg of DM intake was reported (Bidewell et al., 2012). Moreover, Kendall et al. (2015) found that 40% of dairy cows in UK had a liver Cu concentration above healthy range, making them more susceptible to chronic Cu toxicity. Hence, dietary Cu concentration should be closely monitored to avoid toxicity (Grace and Knowles, 2015) and even recommendations should be stricter (Suttle, 2016). In this regard, the Canadian Food Inspection Agency (CFIA) has proposed maximum TM concentrations allowed in dairy cattle diets (Table 1), which are well above the NASEM recommendations (CFIA, 2021). For Cu and Fe, the proposed maximum concentrations equal the maximum tolerable levels whereas for Co, Mn, and Zn the values proposed by CFIA are lower by 5, 13, and 1.8 folds than the maximum tolerable levels reported in NRC (2005). In comparison, European regulations are stricter (Hejna et al., 2018). According to Suttle (2022), maximum TM tolerable levels are often considered as optimal TM levels, which amplifies TM overfeeding.
In the past years, NRC (2001) recommendations for TM have been criticized (Overton and Yasui, 2014). Indeed, these authors mentioned that the evaluation of cow TM requirements based on the classical factorial approach is not perfect because quantities needed to optimize functions in which TM are related, such as immunity, oxidative metabolism, and other biological functions are very difficult to measure. Given the role of some TM in immunity and oxidative metabolism, cows in transition and early lactation might have greater TM requirements than cows in mid- or late-lactation. Castillo et al. (2013) stated that this might support a modest TM overfeeding during the transition period. The majority of Canadian dairy nutritionists also responded that the transition period is the most critical period regarding TM (Duplessis et al., 2023).
Early lactation cows overfed Zn had greater metalloenzyme activity known for their antioxidant effects in their blood compared with cows receiving the basal diet without Zn supplementation (Sobhanirad and Naserian, 2012). In their experiment, Weiss and Socha (2005) concluded that Mn requirements for lactating and dry cows in the NRC (2001) reference were not sufficient to meet animal needs. In the same line, Nocek et al. (2006) observed an improved reproductive performance, such as earlier days in milk at first estrus, when cows received a mineral supplement containing 9.1 and 3.3 times NRC (2001) requirements for Co and Mn, respectively, from 55 days before calving until almost 2 lactations. However, in mid-lactation cows, supplying a mineral supplement providing 2 times the NRC requirements for Cu, Mn, and Zn failed to have positive impacts on oxidative metabolism and the immune system (Yasui et al., 2019). Moreover, Marchand et al. (2022) did not observe any positive effects on major ruminal fermentation characteristics and fiber and starch digestibility to increase the levels of TM above requirements in mid-lactation cows. In a report, Goselink and Jongbloed (2012) concluded that results of studies evaluating benefits of feeding Cu and Zn above the requirements to dairy cows were variable and inconclusive, although some suggested a decrease of somatic cell count in milk when feeding Zn in excess of requirements (Sobhanirad et al., 2010). In a review that compiled 100 years of mineral research in dairy cattle, Weiss (2017) stated that TM deficiency symptoms have been well studied, but impacts of TM overfeeding on animal responses have not been well covered.
Numerous recent studies have been conducted to compare performance between animals receiving either inorganic or organic sources of TM (Rabiee et al., 2010; Akins et al., 2013; Faulkner et al., 2017; Mion et al., 2023). Although it is beyond the scope of this review to discuss the difference between these 2 sources of TM, organic TM supplement are claimed to be absorbed more efficiently than their inorganic form, although conflictual results exist in the literature in which no absorption improvement was noted (Faulkner et al., 2017; Goff, 2018). A better absorption coefficient of organic sources could probably be associated with lower TM excretion in manure along with lesser dietary quantity needs of TM supplements to obtain the same animal performance compared with the use of inorganic source (Nocek et al., 2006), although this statement was criticized by Suttle (2022).
4 Impact of trace mineral feeding on trace mineral concentrations in manure
As previously mentioned, for various reasons, there is a current tendency to formulate cow diets above TM recommendations. The major concern about feeding TM above the recommendations is the increase TM excretion in manure which could have deleterious impact on the ecosystem (Brugger and Windisch, 2015). Indeed, TM concentrations of manure is a mirror of TM concentrations in the diet (Hejna et al., 2019), in which dietary TM concentrations are positively correlated with manure TM concentrations (Wang et al., 2013). Faulkner et al. (2017) found that a diet providing greater Zn ended with greater manure Zn concentration. Nevertheless, the higher Mn concentration in a by-product-fiber based diet compared to a forage-based diet did not result in a higher manure Mn concentration, but in a higher apparent Mn absorption [i.e. (Mn intake – Mn in feces)/Mn intake] (Faulkner et al., 2017). In the trial of Weiss and Socha (2005), supplying more Mn in the ration led to greater Mn excretion in manure and also to greater apparent Mn absorption. Wang et al. (2013) compared Cu and Zn concentrations in cow manure in China at 3 different time points and found that Cu and Zn concentrations in manure increased by 212 and 95%, respectively, from 1990 to 2008. They mentioned that this could probably be explained by the increase TM supplementation in cow diets. Marchand et al. (2022) reported that when ingestion of Co, Mn, and Zn was higher by 206, 229 and 93%, respectively, fecal excretion in these minerals was higher by 221, 202 and 73%, respectively.
Table 2 summarizes TM concentrations of dairy cow manure, feces, and/or urine from several studies conducted in different regions of the world. Copper and Zn are the 2 most studied TM. Trace mineral concentrations of urine are lower than in feces (Safley et al., 1984; Weiss and Socha, 2005; Hristov et al., 2007). Hence, feces are the major contributor of TM concentrations in manure. Wide variations among studies and among animals within the same study exist. Manure Cu and Zn concentrations varied from 24 to 409 and from 127 to 239 mg/kg of DM, respectively (Table 2). Different feeding management among regions could partially explain the variability among trials. For cows receiving a corn and alfalfa silage-based diet, percentages of Cu, Mn, and Zn found in feces from the dietary intake averaged about 97, 89, and 76%, respectively (Faulkner et al., 2017). These data stressed that apparent TM absorption is low.
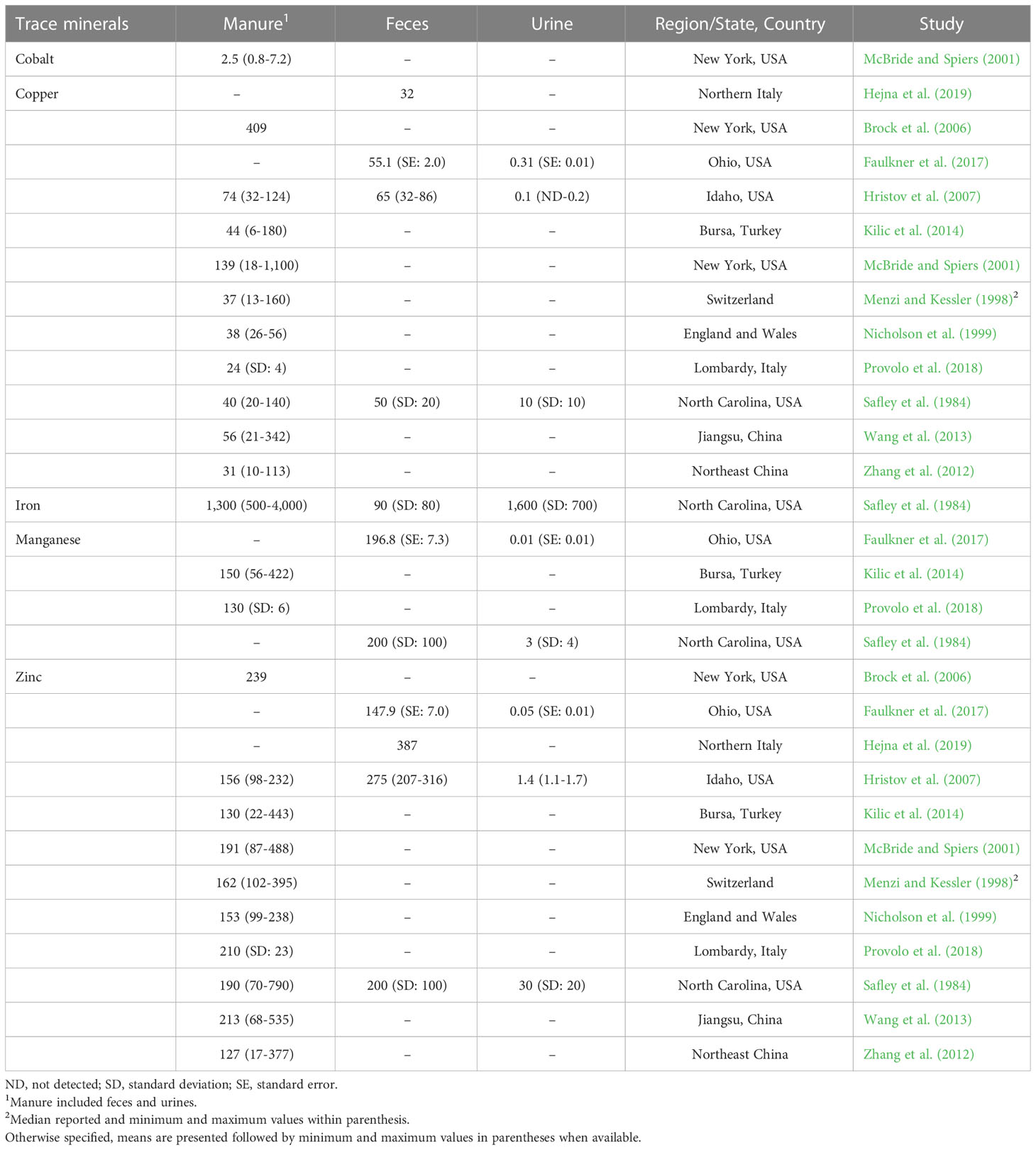
Table 2 Manure, feces, and/or urine concentrations (mg/kg of dry matter) of cobalt, copper, iron, manganese, and zinc of dairy cows from different studies.
5 Discussion
Previous sections have shown that TM have important roles to play in cattle metabolism, but have also demonstrated that, worldwide, cows are generally fed a ration containing higher TM than the animal requirements. Excess dietary TM ends up in the manure and manure spreading can cause a long-term TM build up in soils. Moreover, some TM, such as Cu and Zn, are also used in footbaths for digital dermatitis control. Bolan et al. (2003) observed that dairy herds using Cu footbath had greater Cu concentration in manure. Using large quantities of TM in herd management can have harmful impact on the environment if we continue to ignore it and work independently rather than in an integrated manner. According to Hristov et al. (2007), the best way to reduce TM concentrations in manure is by achieving TM precision nutrition. However, there are several bottlenecks for TM precision nutrition. For instance, most nutritionists use their software references for TM concentrations of ingredients to formulate cow diets (Duplessis et al., 2023). Hence, the actual TM provided by the diet might greatly differ from the predicted TM supply by the software. Weiss (2017) stated that: “A more holistic, whole-farm approach to mineral supplementation is needed.” Indeed, since dietary TM concentrations reflect manure TM concentrations, there is an urgent need to assess the impact of TM feeding management on the ecosystem equilibrium. In their review, Powell and Broderick (2011) stressed the fundamental role of transdisciplinary dairy nutrition-soil science research to assess environmental challenge. Future research can take this concept even further by extending the integration of knowledge to dairy nutrition, soil and plant science, water quality, and human and animal health using the One Health approach. Furthermore, it has been shown that cyanobacteria have TM requirements to grow, especially Fe, and cause bloom in freshwater that could be detrimental to human and animal health (Facey et al., 2019). It is not known whether high concentrations of TM from cattle overfeeding are responsible for impaired water quality. However, TM in excess of requirements are directly excreted into the manure. When manure with high concentrations of TM is repeatedly applied to fields, it can be hypothesized that many different environmental problems can emerge such as eutrophication and potentially cyanobacterial growth in freshwater bodies. On the other hand, at high concentrations, Cu and Zn can be toxic to cyanobacteria (Facey et al., 2019).
Unlike P and N, TM are overlooked when it comes to manure spreading rate calculations as current fertilisation recommendations are solely based on N, P, and potassium (K), and crop requirements for TM are not well known (CRAAQ, 2010). In addition, TM are not included in eutrophication management strategies, due to a lack of knowledge about their environmental effects. Given the wide variability of TM concentrations in manure, future holistic studies should aim at assessing the role of TM in cow manure on the ecosystem. Hence, an integrated approach studying the impact of cow feeding management with respect to TM on the animal-soil-plant-water system is needed.
Author contributions
MD: Conceptualization, writing of the original draft, and revision. IR: Revision of the original draft. All authors contributed to the article and approved the submitted version.
Conflict of interest
The authors declare that the research was conducted in the absence of any commercial or financial relationships that could be construed as a potential conflict of interest.
Publisher’s note
All claims expressed in this article are solely those of the authors and do not necessarily represent those of their affiliated organizations, or those of the publisher, the editors and the reviewers. Any product that may be evaluated in this article, or claim that may be made by its manufacturer, is not guaranteed or endorsed by the publisher.
References
Akins M. S., Bertics S. J., Socha M. T., Shaver R. D. (2013). Effects of cobalt supplementation and vitamin B12 injections on lactation performance and metabolism of Holstein dairy cows. J. Dairy Sci. 96, 1755–1768. doi: 10.3168/jds.2012-5979
Bidewell C. A., Drew J. R., Payne J. H., Sayers A. R., Higgins R. J., Livesey C. T. (2012). Case study of copper poisoning in a British dairy herd. Veterinary Rec. 170, 464. doi: 10.1136/vr.100267
Bolan N. S., Khan M. A., Donaldson J., Adriano D. C., Matthew C. (2003). Distribution and bioavailability of copper in farm effluent. Sci. Total Environ. 309, 225–236. doi: 10.1016/S0048-9697(03)00052-4
Brock E. H., Ketterings Q. M., McBride M. (2006). Copper and zinc accumulation in poultry and dairy manure-amended fields. Soil Sci. 171, 388–399. doi: 10.1097/01.ss.0000209360.62945.95
Brugger D., Windisch W. M. (2015). Environmental responsibilities of livestock feeding using trace mineral supplements. Anim. Nutr. 1, 113–118. doi: 10.1016/j.aninu.2015.08.005
Canadian Food Inspection Agency. (2021). Proposed document to be incorporated by reference - tables of maximum nutrient values for feeds [Online]. Available at: https://inspection.canada.ca/animal-health/livestock-feeds/regulatory-modernization/tables-of-maximum-nutrient-values-for-feeds/eng/1615479105920/1615479106259#t5 (Accessed April 13, 2022).
Castillo A. R., St-Pierre N. R., Silva del Rio N., Weiss W. P. (2013). Mineral concentrations in diets, water, and milk and their value in estimating on-farm excretion of manure minerals in lactating dairy cows. J. Dairy Sci. 96, 3388–3398. doi: 10.3168/jds.2012-6121
Centre de référence en agriculture et agroalimentaire du Québec (CRAAQ). (2010). Guide de référence en fertilisation (Québec, QC, Canada: CRAAQ).
Cerosaletti P. E., Fox D. G., Chase L. E. (2004). Phosphorus reduction through precision feeding of dairy cattle. J. Dairy Sci. 87, 2314–2323. doi: 10.3168/jds.S0022-0302(04)70053-3
Daniel J.-B., Brugger D., van der Drift S., van der Merwe D., Kendall N. R., Windisch W. M., et al. (2023). Zinc, copper, and manganese homeostasis and potential trace metal accumulation in dairy cows: Longitudinal study from late lactation to subsequent mid-lactation. J. Nutr. doi: 10.1016/j.tjnut.2023.02.022
De Conti L., Ceretta C. A., Ferreira P. A. A., Lourenzi C. R., Girotto E., Lorensini F., et al. (2016). Soil solution concentrations and chemical species of copper and zinc in a soil with a history of pig slurry application and plant cultivation. Agricult. Ecosyst. Environ. 216, 374–386. doi: 10.1016/j.agee.2015.09.040
Duplessis M., Fadul-Pacheco L., Santschi D. E., Pellerin D. (2021). Toward precision feeding regarding minerals: What is the current practice in commercial dairy herds in québec, Canada? Animals 11, 1320. doi: 10.3390/ani11051320
Duplessis M., Wright T. C., Bejaei M. (2023). A survey of Canadian dairy nutritionists to assess current trace element formulation practices. J. Dairy Sci. 106. doi: 10.3168/jds.2022-22943
EAAP Scientific Series (2011). NorFor Nordic feed evaluation system (Wageningen, The Netherlands: Wageningen Academic Publishers).
Facey J. A., Apte S. C., Mitrovic S. M. (2019). A review of the effect of trace metals on freshwater cyanobacterial growth and toxin production. Toxins 11, 643. doi: 10.3390/toxins11110643
Faulkner M. J., St-Pierre N. R., Weiss W. P. (2017). Effect of source of trace minerals in either forage- or by-product–based diets fed to dairy cows: 2. apparent absorption and retention of minerals. J. Dairy Sci. 100, 5368–5377. doi: 10.3168/jds.2016-12096
Goff J. P. (2018). Invited review: Mineral absorption mechanisms, mineral interactions that affect acid-base and antioxidant status, and diet considerations to improve mineral status. J. Dairy Sci. 101, 2763–2813. doi: 10.3168/jds.2017-13112
Goselink R. M. A., Jongbloed A. W. (2012). Zinc and copper in dairy cattle feeding. report 519. ISSN 1570-8616 (Wageningen, The Netherlands:Wageningen UR Livestock Research).
Grace N. D., Knowles S. O. (2012). Trace element supplementation of livestock in new Zealand: Meeting the challenges of free-range grazing systems. Veterinary Med. Int. 2012, 639472. doi: 10.1155/2012/639472
Grace N. D., Knowles S. O. (2015). Taking action to reduce the risk of copper toxicity in cattle. Veterinary Rec. 177, 490–491. doi: 10.1136/vr.h5977
Hejna M., Gottardo D., Baldi A., Dell’Orto V., Cheli F., Zaninelli M., et al. (2018). Review: Nutritional ecology of heavy metals. Animal 12, 2156–2170. doi: 10.1017/S175173111700355X
Hejna M., Moscatelli A., Onelli E., Baldi A., Pilu S., Rossi L. (2019). Evaluation of concentration of heavy metals in animal rearing system. Ital. J. Anim. Sci. 18, 1372–1384. doi: 10.1080/1828051X.2019.1642806
Hristov A. N., Hazen W., Ellsworth J. W. (2007). Efficiency of use of imported magnesium, sulfur, copper, and zinc on Idaho dairy farms. J. Dairy Sci. 90, 3034–3043. doi: 10.3168/jds.2007-0013
INRA. (2018). INRA feeding system for ruminants (Wageningen, The Netherlands: Wageningen Academic Publishers).
Institude of Medicine. (2006). Dietary reference intakes: The essential guide to nutrient requirements (Washington, DC: The National Academies Press).
Jondreville C., Revy P. S., Jaffrezic A., Dourmad J. Y. (2002). Copper in pig nutrition: Essential trace element, growth promoter, and its potential adverse effects on human nutrition and environment [Le cuivre dans l’alimentation du porc: Oligo-élément essentiel, facteur de croissance et risque potentiel pour l’Homme et l’environnement]. Productions Animales 15, 247–265. doi: 10.20870/productions-animales.2002.15.4.3706
Kendall N. R., Holmes-Pavord H. R., Bone P. A., Ander E. L., Young S. D. (2015). Liver copper concentrations in cull cattle in the UK: Are cattle being copper loaded? Veterinary Rec. 177, 493. doi: 10.1136/vr.103078
Kilic I., Uguz S., Asik B. B. (2014). Soil pollution by trace metals derived from animal feed and manure in the bursa region of Turkey. Toxicol. Environ. Chem. 96, 1476–1488. doi: 10.1080/02772248.2015.1029927
Legros S., Doelsch E., Feder F., Moussard G., Sansoulet J., Gaudet J. P., et al. (2013). Fate and behaviour of Cu and zn from pig slurry spreading in a tropical water–soil–plant system. Agricult. Ecosyst. Environ. 164, 70–79. doi: 10.1016/j.agee.2012.09.008
Li Y., McCrory D. F., Powell J. M., Saam H., Jackson-Smith D. (2005). A survey of selected heavy metal concentrations in Wisconsin dairy feeds. J. Dairy Sci. 88, 2911–2922. doi: 10.3168/jds.S0022-0302(05)72972-6
López-Alonso M. (2012). Trace minerals and livestock: not too much not too little. ISRN Veterinary Sci. 2012, 704825. doi: 10.5402/2012/704825
Marchand C., Royer I., Gervais R., Girard C. L., Benchaar C., Hassanat F., et al. (2022). Effects of two levels of dietary trace minerals on ruminal fermentation, digestibility, trace mineral excretion and lactation performance in Holstein cows. J. Dairy Sci. 105 E-Suppl. 1, 402.
Martens J. H., Barg H., Warren M., Jahn D. (2002). Microbial production of vitamin B12. Appl. Microbiol. Biotechnol. 58, 275–285. doi: 10.1007/s00253-001-0902-7
McBride M. B., Spiers G. (2001). Trace element content of selected fertilizers and dairy manures as determined by ICP-MS. Commun. Soil Sci. Plant Anal. 32, 139–156. doi: 10.1081/CSS-100102999
Menzi H., Kessler J. (1998). “Heavy metal content of manures in Switzerland,” in Proc 8th international conference on the FAO ESCORENA network on recycling of agricultural, municipal and industrial residues in agriculture. Eds. Martinez J., Maudet M. N. (Rennes, France: RAMIRAN 98).
Mion B., Ogilvie L., Van Winters B., Spricigo J. F. W., Anan S., Duplessis M., et al. (2023). Effects of replacing inorganic salts of trace minerals with organic trace minerals in the pre- and postpartum diets on mineral status, antioxidant biomarkers, and health of dairy cows. J. Anim. Sci. doi: 10.1093/jas/skad041
National Academies of Sciences Engineering and Medicine (NASEM). (2021). Nutrient requirements of dairy cattle (Washington, DC: The National Academies Press).
National Research Council. (2001). Nutrient requirements of dairy cattle (Washington, DC, USA: National Academy Press).
National Research Council. (2005). Mineral tolerance of animals (Washington DC, USA: National academies press).
Nicholson F. A., Chambers B. J., Williams J. R., Unwin R. J. (1999). Heavy metal contents of livestock feeds and animal manures in England and Wales. Bioresource Technol. 70, 23–31. doi: 10.1016/S0960-8524(99)00017-6
Nocek J. E., Socha M. T., Tomlinson D. J. (2006). The effect of trace mineral fortification level and source on performance of dairy cattle. J. Dairy Sci. 89, 2679–2693. doi: 10.3168/jds.S0022-0302(06)72344-X
Overton T. R., Yasui T. (2014). Practical applications of trace minerals for dairy cattle. J. Anim. Sci. 92, 416–426. doi: 10.2527/jas.2013-7145
Powell J. M., Broderick G. A. (2011). Transdisciplinary soil science re impacts of dairy nutrition on manure chemistry and the environment. Soil Sci. Soc. America J. 75, 2071–2078. doi: 10.2136/sssaj2011.0226
Provolo G., Manuli G., Finzi A., Lucchini G., Riva E., Sacchi G. A. (2018). Effect of pig and cattle slurry application on heavy metal composition of maize grown on different soils. Sustainability 10, 2684. doi: 10.3390/su10082684
Qian X., Wang Z., Shen G., Chen X., Tang Z., Guo C., et al. (2018). Heavy metals accumulation in soil after 4 years of continuous land application of swine manure: A field-scale monitoring and modeling estimation. Chemosphere 210, 1029–1034. doi: 10.1016/j.chemosphere.2018.07.107
Rabiee A. R., Lean I. J., Stevenson M. A., Socha M. T. (2010). Effects of feeding organic trace minerals on milk production and reproductive performance in lactating dairy cows: A meta-analysis. J. Dairy Sci. 93, 4239–4251. doi: 10.3168/jds.2010-3058
Safley L. M. Jr., Barker J. C., Westerman P. W. (1984). Characteristics of fresh dairy manure. Trans. Am. Soc. Agric. Engineers 27, 1150–1153, 1162.
Senaratne V., Plaizier J. C. (2020). Relationships between dietary and cow factors with the fecal phosphorus contents of dairy cows in Manitoba. Can. J. Anim. Sci. 100, 210–213. doi: 10.1139/cjas-2019-0040
Sinclair L. A., Atkins N. E. (2015). Intake of selected minerals on commercial dairy herds in central and northern England in comparison with requirements. J. Agric. Sci. 153, 743–752. doi: 10.1017/S0021859614001026
Sobhanirad S., Carlson D., Bahari Kashani R. (2010). Effect of zinc methionine or zinc sulfate supplementation on milk production and composition of milk in lactating dairy cows. Biol. Trace Element Res. 136, 48–54. doi: 10.1007/s12011-009-8526-3
Sobhanirad S., Naserian A. A. (2012). Effects of high dietary zinc concentration and zinc sources on hematology and biochemistry of blood serum in Holstein dairy cows. Anim. Feed Sci. Technol. 177, 242–246. doi: 10.1016/j.anifeedsci.2012.06.007
Spears J. W. (2003). Trace mineral bioavailability in ruminants. J. Nutr. 133, 1506S–1509S. doi: 10.1093/jn/133.5.1506S
Suttle N. F. (2016). Reducing the risk of copper toxicity in dairy cattle. Veterinary Rec. 178, 196–196. doi: 10.1136/vr.i793
Wang H., Dong Y., Yang Y., Toor G. S., Zhang X. (2013). Changes in heavy metal contents in animal feeds and manures in an intensive animal production region of China. J. Environ. Sci. 25, 2435–2442. doi: 10.1016/S1001-0742(13)60473-8
Wattiaux M. A., Karg K. L. (2004). Protein level for alfalfa and corn silage-based diets: II. nitrogen balance and manure characteristics. J. Dairy Sci. 87, 3492–3502. doi: 10.3168/jds.S0022-0302(04)73484-0
Weiss W. P. (2017). A 100-year review: From ascorbic acid to zinc - mineral and vitamin nutrition of dairy cows. J. Dairy Sci. 100, 10045–10060. doi: 10.3168/jds.2017-12935
Weiss W. P., Socha M. T. (2005). Dietary manganese for dry and lactating Holstein cows. J. Dairy Sci. 88, 2517–2523. doi: 10.3168/jds.S0022-0302(05)72929-5
Wilde D. (2006). Influence of macro and micro minerals in the peri-parturient period on fertility in dairy cattle. Anim. Reprod. Sci. 96, 240–249. doi: 10.1016/j.anireprosci.2006.08.004
Yang F. L., Li X. S. (2015). Role of antioxidant vitamins and trace elements in mastitis in dairy cows. J. Advanced Veterinary Anim. Res. 2, 1–9. doi: 10.5455/javar.2015.b48
Yasui T., Ehrhardt R. M., Bowman G. R., Vázquez-Añon M., Richards J. D., Atwell C. A., et al. (2019). Effects of trace mineral amount and source on aspects of oxidative metabolism and responses to intramammary lipopolysaccharide challenge in midlactation dairy cows. Animal 13, 1000–1008. doi: 10.1017/S1751731118002525
Keywords: trace element, multidisciplinary, metal, cattle, one health
Citation: Duplessis M and Royer I (2023) Mini-Review: The importance of an integrated approach to assess trace mineral feeding practices in dairy cows. Front. Anim. Sci. 4:1155361. doi: 10.3389/fanim.2023.1155361
Received: 31 January 2023; Accepted: 27 February 2023;
Published: 28 March 2023.
Edited by:
Kristen A. Johnson, Washington State University, United StatesReviewed by:
Terry Engle, Colorado State University, United StatesJesse Goff, Iowa State University, United States
Neville Suttle, Moredun Foundation, United Kingdom
Copyright © 2023 His Majesty the King in Right of Canada, as represented by the Minister of Agriculture and Agri-Food Canada for the contribution of Mélissa Duplessis and Isabelle Royer. This is an open-access article distributed under the terms of the Creative Commons Attribution License (CC BY). The use, distribution or reproduction in other forums is permitted, provided the original author(s) and the copyright owner(s) are credited and that the original publication in this journal is cited, in accordance with accepted academic practice. No use, distribution or reproduction is permitted which does not comply with these terms.
*Correspondence: Mélissa Duplessis, bWVsaXNzYS5kdXBsZXNzaXNAYWdyLmdjLmNh