- Department of Animal Sciences, North Dakota State University, Fargo, ND, United States
This study evaluated whether leukocyte coping capacity (LCC) could be an effective method for detecting physiological stress in growing pigs who are exposed to a mild cycling heating period. We hypothesized that whole blood samples collected from pigs exposed to high environmental temperatures would exhibit a reduced respiratory burst response [measured in relative light units (RLU) using chemiluminescence, where reduced RLU levels indicate a smaller respiratory burst size] compared to pigs that were only exposed to thermoneutral temperatures. A secondary objective was to determine the time required for LCC whole blood samples to reach their maximal RLU value following in vitro challenge using phorbol-12-myristate 13-acetate (PMA) to induce a respiratory burst. Forty growing pigs (mean 66.7 kg; range: 54.9 – 87.5 kg) were assigned to 1 of 2 treatments: 1) heat stress (HS; n = 20), or 2) thermoneutral (TN; n = 20). The HS pigs experienced a cycling mild heat stress period over 48 h (range: 19.5-28.6°C), while TN pigs were exposed to thermoneutral temperatures (range: 18.9-23.7°C). Whole-blood samples were collected via jugular venipuncture at 0, + 4, +8, +24, and +48 h relative to the start of the cycling heating period and were artificially challenged using PMA. Samples were measured at 0, + 15, and +30 min relative to in vitro challenge. No differences were observed between HS and TN treatments (P = 0.67) and no treatment by hour interaction was detected (P = 0.48). Maximal RLU values were obtained at 15 min post-PMA challenge (P < 0.05).
1 Introduction
Effective measurement of the physiological stress response in pigs continues to be a challenge for scientists with an interest in improving on-farm pig welfare. Factors, such as sex (Cooper et al., 2009), human handling (Hemsworth et al., 1987), circadian rhythm (Ruis, 1997), and individual animal variability (Larzul et al., 2015; Kanitz et al., 2019) can heavily influence an animal’s physiology, ultimately leading to spurious or inconclusive results across ages and stages of production. Many common measures, like behavioral evaluation (Smulders et al., 2006), stress hormone measurement (e.g. cortisol; Candiani et al., 2008), heart rate variability (Byrd et al., 2022), growth measures, and immune parameters (Salak-Johnson and Webb, 2018), are also time-intensive to carry out. Accordingly, there is a continued need for relatively rapid methods that can effectively measure the physiological stress response in swine.
Leukocyte coping capacity (LCC) is a particularly interesting measure of physiological stress that has not been previously evaluated in swine. The LCC method is based upon the mammalian polymorphonuclear leukocyte (PMNL; primarily neutrophils) response to a pathogenic challenge, where PMNL produce a respiratory burst of reactive oxygen species (ROS) to inhibit pathogen colonization within the host animal’s tissues (Amulic et al., 2012). However, if an animal is experiencing acute or chronic stress due to some stressor, the animal’s capacity to mount a secondary respiratory burst response is reduced (McLaren et al., 2003), resulting in the release of fewer ROS. Leukocyte coping capacity harnesses this concept for use as an indirect measure of stress. Specifically, whole blood samples collected from animals previously exposed to a stressor are challenged with phorbol-12-myristate 13-acetate (PMA) in vitro and the resulting size of the artificially induced respiratory burst is measured in relative light units (RLU) using chemiluminescence (McLaren et al., 2003). A larger respiratory burst results in greater RLU. Previous studies investigating LCC for detection of stress in wildlife species (McLaren et al., 2003; Huber et al., 2017a) and bull calves (Gaudio et al., 2018) have indicated that LCC is capable of detecting both acute and chronic physiological stress.
A major benefit of the LCC methodology is that it can be evaluated rapidly using a portable luminometer. Previous studies (McLaren et al., 2003; Esteruelas et al., 2016) indicate that maximal LCC levels are achieved at 15 min post-PMA whole blood challenge. This is in stark contrast to measures like animal behavior or hormone analysis, which often require extended periods of video analysis and assay preparation. Therefore, LCC may be useful for rapid detection of physiological stress in swine.
The primary objective of this study was to determine whether LCC could be utilized to detect an acute mild cycling heat stress in growing pigs. We hypothesized that whole blood samples collected from pigs exposed to high environmental temperatures would exhibit a reduced respiratory burst response. Accordingly, we predicted that pigs exposed to the cycling heating period would have reduced RLU levels compared to pigs that were only exposed to thermoneutral temperatures throughout the experimental period. A secondary objective was to determine the amount of time required for the LCC whole blood samples to reach their maximal RLU value following in vitro challenge with PMA. Based on previously published studies in other species, we predicted that maximal RLU values would be reached by 15-min post-challenge.
2 Materials and methods
All experimental procedures described in this study were approved by the Institutional Animal Care and Use Committee at North Dakota State University (protocol # A20090).
2.1 Animals and housing
Forty growing pigs (mean 66.7 kg; range: 54.9 – 87.5 kg) matched for sex (n = 20 gilts; n = 20 barrows) and weight were allocated to 1 of 2 treatments, heat stress (HS; n = 20) or thermoneutral (TN; n = 20), and housed in separate rooms according to their treatment at North Dakota State University’s Animal Nutrition and Physiology Center. The two rooms utilized for the study were located immediately adjacent to one another and were environmentally controlled in a similar manner. All pigs were housed individually on slatted flooring in individual pens (Room 1: 1.83 x 1.52; Room 2: 1.52 x 1.21 m) that provided visual, olfactory, and some physical contact (through adjoining pen bars) with their conspecifics. Feed and water were provided ad libitum within each pen. Feed was formulated according to the pigs’ stage of production. Artificial lighting was provided from 0800h to 1800h while windows spanning the length of both rooms allowed for additional natural lighting. To account for any effects pen size may have on the study results, the experimental procedure was conducted over 2 repetitions (Repetition 1: October 2021, n = 10 pigs per treatment; Repetition 2: January 2022, n = 10 pigs per treatment), where the acute heating period occurred in Room 1 during repetition 1 and Room 2 during repetition 2.
2.2 Cycling heat stress period and blood collection
Pigs in the HS treatment were exposed to a mild cycling heating period that attempted to mimic a warm summer day in North Dakota (range: 19.5-28.6°C). On d 1 of the experimental period, the cycling heating period began at h 0. Temperatures gradually increased from thermoneutral temperatures to maximal temperatures by h 8 on d 1 of the experimental period (Figure 1). The room then gradually returned to thermoneutral temperatures by h 12 (Figure 1). The cycling heating period was repeated on d 2 of the experimental procedure (Figure 1). Pigs in the TN treatment remained in thermoneutral temperatures (range: 18.9-23.7°C) throughout the entire experimental period (Figure 1). Relative humidity (RH) within the rooms during repetition 1 was 33.7% ± 0.3 SE and 39.9% ± 0.2 SE for TN and HS treatments, respectively. During repetition 2, RH within the rooms was 15.2% ± 0.1 SE and 16.3% ± 0.1 SE for TN and HS treatments, respectively.
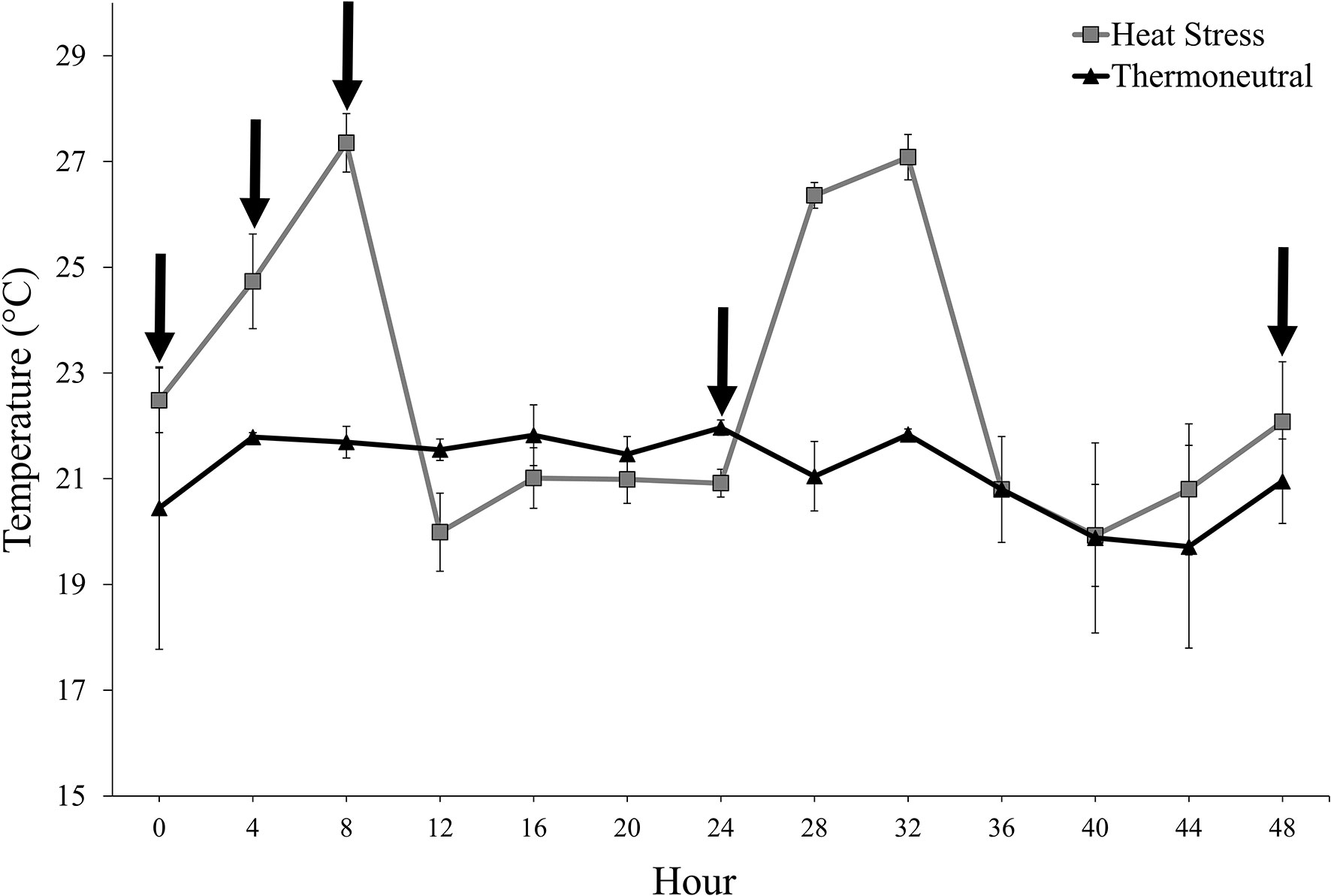
Figure 1 Room temperature (LS means ± SE) according to experimental treatment (heat stress, thermoneutral) throughout the experimental period. The arrows located along the x-axis indicate the blood collection timepoints (0, + 4, +8, +24, and +48 h relative to the start of the experiment).
Blood was collected via jugular venipuncture at 0, + 4, +8, +24, and +48 h relative to the start of the experimental period on d 1. These timepoints were selected strategically to reduce the number of blood draws per pig while still being able to determine whether LCC was altered during periods of high temperature (h 8 on d 1; Figure 1) and remained altered once the temperature within the room returned to thermoneutral conditions (h 24 and 48; Figure 1) At each time point, one experimental personnel entered each individual pen and snared the individually-housed pig using a cotton rope snare. A second experimental personnel then entered the pen and collected approximately 3 mL of blood from the pig’s jugular vein into a blood collection tube containing K3EDTA (BD Vacutainer Plastic Blood Collection Tubes with K3EDTA; Becton Dickinson Inc., Franklin Lakes, NJ, USA). This procedure was repeated for each pig on study at each time point. The order of blood collection was altered according to treatment (Room 1 and Room 2) and individually-housed pig (Pigs 1-10, Room 1; Pigs 11-20, Room 2) to reduce the likelihood of an order effect on the LCC results.
2.3 Leukocyte coping capacity measurement
Immediately after blood collection at each time point, 20 μL of whole blood was divided equally into two 1.5 mL clear microcentrifuge tubes containing 90 μL of luminol (10-4 mol L-1; A5301; Tokyo Chemical Industry Co., Tokyo, Japan) dissolved in phosphate buffered saline (PBS; BP2944-100; Fisher Scientific, Fair Lawn, NJ, USA) and 10 μL of phosphate buffered saline. One tube containing the whole blood-luminol combination served as a control tube (no respiratory burst) and the second tube containing the whole blood-luminol combination served as the challenged tube (respiratory burst induction). To induce a respiratory burst in the second tube, 10 μL of phorbol-12-myristate 13-acetate (PMA; 10-6 mol L-1; AAJ63916MCR; Thermo Scientific, Waltham, MA, USA) dissolved in dimethyl sulfoxide (D128-1; Fisher Scientific, Fair Lawn, NJ, USA) was added. Following preparation of the samples, chemiluminescence (i.e. RLU) was measured using a portable luminometer (Junior LB9509; Berthold Technologies USA LLC., Oak Ridge, TN, USA) at 0, + 15, and +30 min relative to PMA challenge.
2.4 Statistical analysis
Data were analyzed using the mixed procedure in SAS (v. 9.4; SAS Institute, Inc., Cary, NC). The model included treatment, experimental hour, PMA challenge time, repetition, and all 2- and 3-way interactions as fixed effects. The control value was used as a covariate for the challenge value. Because the data collection included two repeated measures (experimental hour within pig and PMA challenge time within hour) and two repeated statements causes issues with model convergence in SAS, a repeated measure of RLU time with hour nested within pig was used, while pig was fit as a random effect. Different covariance structures were tested and the best fit based on Aikaike’s Criterion was selected. The least square means statement with the DIFF option was used to estimate the differences between fixed effects. The Tukey adjustment was used for the P-values. A P-value less than 0.05 was considered a significant result. A P-value between 0.05 and 0.1 was considered a tendency.
3 Results
Heat stressed pigs did not differ from TN pigs in regard to overall mean RLU (55.4 ± 4.1 vs. 57.9 ± 4.1 RLU; P = 0.67) and no treatment by hour interaction was observed (P = 0.48). An hour by repetition interaction was observed (P < 0.0001), where RLU values during repetition 2 gradually increased throughout the experimental period while repetition 1 RLU values remained at basal levels (Figure 2). Specifically, RLU values collected during repetition 2 at h 0 were lower than RLU values collected at all other timepoints (P < 0.006; Figure 2). Repetition 2 RLU values at h 4 were lower than repetition 2 RLU values at h 24 (P = 0.05; Figure 2) and h 48 (P < 0.0001: Figure 2). Additionally, repetition 2 values at h 48 were greater than repetition 2 values at h 8 (P < 0.0001; Figure 2) and h 24 (P = 0.01; Figure 2). Interestingly, there tended (P = 0.054) to be a three-way repetition by treatment by experimental hour interaction (Figure 2), where repetition 2 TN pigs exhibited numerically greater RLU values at h 8 of the experiment compared to repetition 2 HS pigs and all repetition 1 pigs at h 8 (Figure 2).
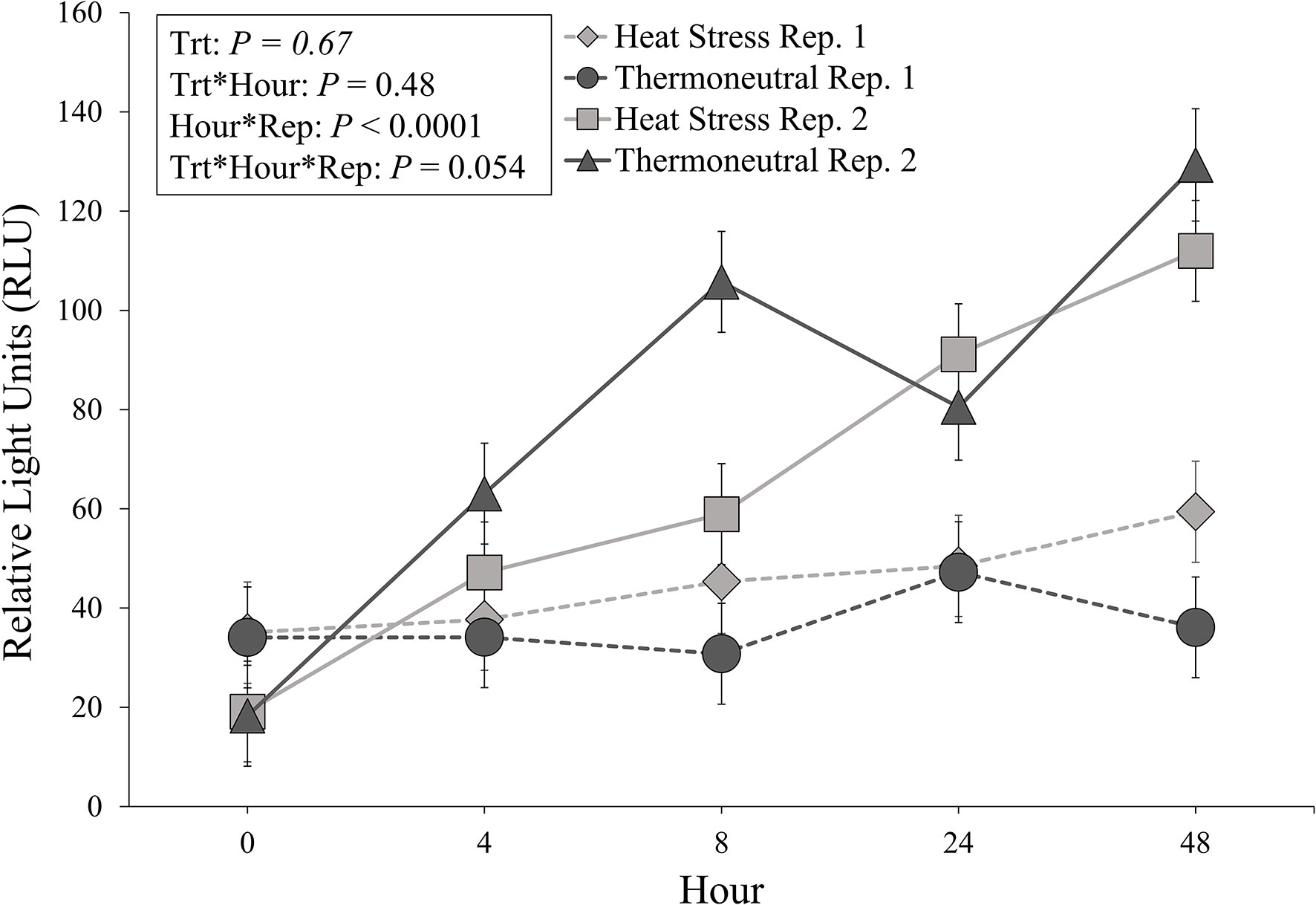
Figure 2 Relative light units (RLU; LS means ± SE) organized by experimental treatment (heat stress, thermoneutral), hour (0, + 4, +8, +24, +48), and experimental repetition (Rep. 1, Rep. 2).
After PMA challenge, RLU levels were measured for each sample at three time points (0, 15, and 30 min) to determine the amount of time required to reach maximal RLU levels. There was an interaction between repetition and post-PMA challenge time (P < 0.0001), where repetition 1 RLU values remained lower than repetition 2 RLU values at 15- and 30-min post-PMA challenge (P < 0.03; data not shown). Regardless, for both repetitions, maximal RLU values were obtained at 15 min post-PMA challenge. During repetition 1, RLU values at 15 min post-PMA challenge were greater than RLU values at 0 min (54.9 ± 5.1 vs. 5.4 ± 5.1; P < 0.0001) but were not different from 30 min post-PMA challenge (54.9 ± 5.1 vs. 62.3 ± 5.6; P = 0.71). Repetition 1 RLU values at 30 min post-PMA challenge were greater than RLU values at 0 min (P < 0.0001). During repetition 2, RLU values at 15 min post-PMA challenge were greater than the 0 min (120.3 ± 5.2 vs. 5.4 ± 5.1; P < 0.0001) and 30 min post-PMA challenge (120.3 ± 5.2 vs. 91.9 ± 5.2; P < 0.0001). Additionally, repetition 2 RLU values at 30 min post-PMA challenge timepoint were greater than RLU values at 0 min (P < 0.0001).
4 Discussion
Previous studies investigating the effectiveness of LCC for detecting stress in mammalian and avian species indicate that the method is a relatively rapid and effective stress measure (McLaren et al., 2003; Huber et al., 2017a). For example, whole blood samples collected from badgers transported for 10 min following capture exhibit a marked decrease in LCC compared to badgers who were not transported (McLaren et al., 2003). Additionally, water voles re-housed to laboratory cages exhibited lower LCC for 6 weeks compared to their conspecifics who were housed in outdoor enclosures (Moorhouse et al., 2007). These data, together, indicate that LCC may be a promising measure of both acute and chronic stress in multiple species.
In response to a pathogenic challenge or stressor, an animal’s PMNLs are activated by pathogen-associated molecular patterns, opsonized bacterial particles, cytokines (Graham et al., 2007), or by stress-induced stimulation of their own α- and β-adreno- and glucocorticoid receptors (Brown et al., 2008; Huber et al., 2019). Once activated, PMNLs produce a respiratory burst of ROS (Amulic et al., 2012) that act to inhibit the colonization of invading pathogens within the host animal’s tissues.
In the short term, the respiratory burst response to a pathogen or stressor, while initially large, is curtailed to reduce the likelihood of tissue damage to the host (Huber et al., 2019). Excessive stimulation of ROS within the body has negative effects on animal and human health (Zeng et al., 2019), and as a result, ROS production in response to an acute stressor is quickly reduced. In the long term, as the time of pathogen or stressor exposure increases, the host’s immune response is greatly diminished and the respiratory burst size remains small (Amer and Fibach, 2005; Dhabhar, 2014). Therefore, both acute and chronic stressors result in limited respiratory burst responses.
This study attempted to evaluate whether LCC could be used as an effective measure of acute swine stress for on-farm research use in response to a mild cycling heating period. No overall treatment differences or treatment by experimental hour interactions were observed during the study. Given the numerical differences observed between treatments during repetition 2 at h 8 of the experimental period, the lack of a treatment by experimental hour interaction could have been driven by the sizeable differences in overall RLU values during repetitions 1 and 2. Specifically, RLU values during repetition 1 remained low for both treatments throughout the experimental period compared to repetition 2, which exhibited a substantial increase in RLU values throughout the experimental period. A potential reason for low RLU values during repetition 1 is unclear since the experimental protocol did not differ between repetitions, other than the time of year.
Repetition 1 occurred in October 2021 and repetition 2 occurred in January 2022, which coincided with a major difference in outdoor temperatures that could have had an effect on the temperature within the experimental rooms. For example, mild October 2021 outdoor temperatures could have resulted in greater room temperatures compared to the much lower January 2022 outdoor temperatures. However, major differences in room temperature between repetitions 1 and 2 were not observed. We would also still expect to see differences between repetition 1 HS and TN pigs during periods of greater environmental room temperatures. However, both repetition 1 treatments exhibited a low LCC response throughout the experimental period.
It is possible that the use of a rope snare throughout the experimental period contributed to the low LCC response throughout repetition 1 and during the beginning stages of repetition 2. We did not expect low LCC values at h 0 of the experimental period for either treatment. The substantial increase observed during repetition 2 may indicate a gradual acclimation to the rope snare that did not occur during repetition 1. Future work is needed to confirm this result. If the use of a rope snare has major effects on LCC results, the method is likely not practical for on-farm research use unless a minimally invasive catheterization procedure can be utilized. Given the role leukocytes play in wound healing (Singer and Clark, 1999), jugular catheterization requiring a cut down procedure would have an effect on the LCC results unless the catheter can remain patent for an extended period of time to allow for healing before beginning the experimental procedure.
The numerical results observed during repetition 2 are similar to our hypothesis and predictions for the primary study objective. Specifically, we hypothesized that heat exposure would decrease LCC (i.e. lower RLU) throughout the experimental period. The large numerical differences between TN and HS treatments at h 8 of the experimental period provide some evidence that LCC could be a potentially useful stress measure since h 8 occurred during a period of maximal temperature. However, these same numerical differences were not observed at 24 or 48 h. This may be due to the relatively mild maximum environmental temperatures reached during the experiment, which were similar to- or just above the upper critical temperature for similar-sized pigs (Huynh et al., 2005). However, the maximal temperatures may not have been great enough to cause lasting observable changes to LCC once the room temperature returned to thermoneutral conditions (h 24 and 48).
Similar to traditional stress measures, sex, body condition, season, and circadian rhythm may have an effect on LCC results depending on the species being evaluated (Esteruelas et al., 2016; Sin et al., 2016). The purpose of the present study was not to evaluate these potential effects. Instead, we controlled for some of these variables as much as possible (i.e. sex, weight, time of day, environment). Additionally, the relationship between LCC and other stress measures (e.g. cortisol concentration and immune measures; Pohlin et al., 2020) was not evaluated. Although other studies have not identified a clear relationship between LCC and commonly measured stress parameters in non-swine species (Huber et al., 2017b), a thorough investigation of LCC at basal levels and the relationship between LCC and other common stress measures is needed to fully determine whether the method can be utilized effectively with swine.
The major benefit of the LCC methodology is that it can be evaluated rapidly using a portable luminometer. Published data from others (McLaren et al., 2003; Esteruelas et al., 2016) and our data indicate that maximal LCC levels are achieved at 15 min post-PMA whole blood challenge. This is in stark contrast to measures like behavioral or cortisol analysis, which often require extended periods of video analysis and assay preparation. Therefore, if total RLU levels are to be used for measuring LCC in the future (as opposed to area under the curve measurements; AUC), it is likely that RLU measurement 15 min post-PMA challenge is sufficient. If AUC is desired, repeated measurement of PMA-challenged samples is likely needed for greater than 30 min.
In conclusion, leukocyte coping capacity is a relatively rapid measure that has been used to detect stress in wildlife species and cattle. However, a mild cycling heating period did not lead to overall changes in growing pig LCC during the present study. Future studies should determine whether LCC is altered in response to a more severe heat stress or other common swine stressors. A lack of LCC differences between treatments may also be due to differences in the LCC response that occurred between study repetitions 1 and 2. Factors, such as the use of a rope snare for jugular blood collection and basal variables that commonly affect physiological stress measures, need to be investigated thoroughly to determine whether LCC is an effective on-farm research tool for measuring stress.
Data availability statement
The raw data supporting the conclusions of this article will be made available by the authors, without undue reservation.
Ethics statement
The animal study was reviewed and approved by North Dakota State University Institutional Animal Care and Use Committee (protocol #A20090).
Author contributions
CB and JY contributed to conception and design of the study. CB and JY contributed to data acquisition. JY performed the statistical analysis. CB interpreted the data. CB drafted the manuscript. CB and JY contributed to manuscript revision, read, and approved the submitted version.
Funding
This research was supported by the North Dakota State Board of Agricultural Research and Education’s Agriculture Research Fund.
Acknowledgments
The authors would like to thank Dominique Sommer, Mary Lisa Kasakamu, Terry Skunberg, Justin Gilbertson, Gregg Baumann, and the undergraduate animal caretakers at NDSU’s Animal Nutrition and Physiology Center. The authors declare no conflicts of interest.
Conflict of interest
The authors declare that the research was conducted in the absence of any commercial or financial relationships that could be construed as a potential conflict of interest.
Publisher’s note
All claims expressed in this article are solely those of the authors and do not necessarily represent those of their affiliated organizations, or those of the publisher, the editors and the reviewers. Any product that may be evaluated in this article, or claim that may be made by its manufacturer, is not guaranteed or endorsed by the publisher.
References
Amer J., Fibach E. (2005). Chronic oxidative stress reduces the respiratory burst response of neutrophils from beta-thalassaemia patients. Br. J. Haematol. 129, 435–441. doi: 10.1111/j.1365-2141.2005.05463.x
Amulic B., Cazalet C., Hayes G. L., Metzler K. D., Zychlinsky A. (2012). Neutrophil function: from mechanisms to disease. Annu. Rev. Immunol. 30 (1), 459–489. doi: 10.1146/annurev-immunol-020711-074942
Brown A. S., Levine J. D., Green P. G. (2008). Sexual dimorphism in the effect of sound stress on neutrophil function. J. Neuroimmunol. 205, 25–31. doi: 10.1016/j.jneuroim.2008.08.005
Byrd C. J., McConn B. R., Gaskill B. N., Schinckel A. P., Green-Miller A. R., Lay D. C., et al. (2022). Characterizing the effect of incrementally increasing dry bulb temperature on linear and nonlinear measures of heart rate variability in nonpregnant, mid-gestation, and late-gestation sows. J. Anim. Sci. 100 (1), 1–9. doi: 10.1093/jas/skac004
Candiani D., Salamano G., Mellia E., Doglione L., Bruno R., Toussaint M., et al. (2008). A combination of behavioral and physiological indicators for assessing pig welfare on the farm. J. Appl. Anim. Welf. Sci. 11 (1), 1–13. doi: 10.1080/10888700701729080
Cooper T. A., Roberts M. P., Kattesh H. G., Kojima C. J. (2009). Effects of transport stress, sex, and weaning weight on postweaning performance in pigs. Prof. Anim. Sci. 25 (2), 189–194. doi: 10.15232/S1080-7446(15)30700-2
Dhabhar F. S. (2014). Effects of stress on immune function: the good, the bad, and the beautiful. Immunol. Res. 58, 193–210. doi: 10.1007/s12026-014-8517-0
Esteruelas N. F., Huber N., Evans A. L., Zedrosser A., Cattet M., Palomares F., et al. (2016). Leukocyte coping capacity as a tool to assess capture- and handling-induced stress in Scandinavian brown bears (ursus arctos). J. Wildl. Dis. 52 (2 Supplement), S40–S53. doi: 10.7589/52.2S.S40
Gaudio E., Bordin S., Lora I., Lora M., Massignani M., De Benedictis G. M. (2018). Leukocyte coping capacity chemiluminescence as an innovative tool for stress and pain assessment in calves undergoing ring castration. J. Anim. Sci. 96 (11), 4579–4589. doi: 10.1093/jas/sky342
Graham D. B., Robertson C. M., Bautista J., Mascarenhas F., Diacovo M. J., Montgrain V., et al. (2007). Neutrophil-mediated oxidative burst and host defense are controlled by a vav-PLCγ2 signaling axis in mice. J. Clin. Invest. 117, 3445–3452. doi: 10.1172/JCI32729
Hemsworth P. H., Barnett J. L., Hansen C. (1987). The influence of inconsistent handling by humans on the behaviour, growth and corticosteroids of young pigs. Appl. Anim. Behav. Sci. 17 (3), 245–252. doi: 10.1016/0168-1591(87)90149-3
Huber N., Fusani L., Ferretti A., Mahr K., Canoine V. (2017a). Measuring short-term stress in birds: comparing different endpoints of the endocrine-immune interface. Phys. Behav. 182, 46–53. doi: 10.1016/j.physbeh.2017.09.017
Huber N., Marasco V., Painer J., Vetter S. G., Göritz F., Kaczensky P., et al. (2019). Leukocyte coping capacity: an integrative parameter for wildlife welfare within conservation interventions. Front. Vet. Sci. 6. doi: 10.3389/fvets.2019.00105
Huber N., Vetter S. G., Evans A. L., Kjellander P., Küker S., Bergvall U. A., et al. (2017b). Quantifying capture stress in free ranging European roe deer (Capreolus capreolus). BMC Vet. Res. 13, 127. doi: 10.1186/s12917-017-1045-0
Huynh T. T. T., Aarnink A. J. A., Verstegen M. W. A., Gerrits W. J. J., Heetkamp M. J. W., Kemp B., et al. (2005). Effects of increasing temperatures on physiological changes in pigs at different relative humidities. J. Anim. Sci. 83 (6), 1385–1396. doi: 10.2527/2005.8361385x
Kanitz E., Tuchscherer M., Otten W., Tuchscherer A., Zebunke M., Puppe B. (2019). Coping style of pigs is associated with different behavioral, neurobiological and immune responses to stressful challenges. Front. Behav. Neurosci. 13. doi: 10.3389/fnbeh.2019.00173
Larzul C., Terenina E., Foury A., Billon Y., Louveau I., Merlot E., et al. (2015). The cortisol response to ACTH in pigs, heritability and influence of corticosteroid-binding globulin. Animal 9 (12), 1929–1934. doi: 10.1017/S1751731115001767
McLaren G. W., Macdonald D. W., Georgiou C., Mathews F., Newman C., Mian R. (2003). Leukocyte coping capacity: a novel technique for measuring the stress response in vertebrates. Exp. Physiol. 88 (4), 541–546. doi: 10.1113/eph8802571
Moorhouse T. P., Gelling M., McLaren G. W., Mian R., Macdonald D. W. (2007). Physiological consequences of captive conditions in water voles (Arvicola terrestris). J. Zool. 271 (1), 19–26. doi: 10.1111/j.1469-7998.2006.00175.x
Pohlin F., Hooijberg E. H., Buss P., Huber N., Viljoen F. P., Blackhurst D., et al. (2020). A comparison of hematological, immunological, and stress responses to capture and transport in wild white rhinoceros bulls (Ceratotherium simum simum) supplemented with azaperone or midazolam. Front. Vet. Sci. 7. doi: 10.3389/fvets.2020.569576
Ruis M. (1997). The circadian rhythm of salivary cortisol in growing pigs: effects of age, gender, and stress. Physiol. Behav. 62 (3), 623–630. doi: 10.1016/S0031-9384(97)00177-7
Salak-Johnson J. L., Webb S. R. (2018). Short- and long-term effects of weaning age on pig innate immune status. Open J. Anim. Sci. 8 (2), 137–150. doi: 10.4236/ojas.2018.82010
Sin Y. W., Newman C., Dugdale H. L., Buesching C., Mannarelli M.-E., Annavi G., et al. (2016). No compensatory relationship between the innate and adaptive immune system in wild-living European badgers. PloS One 11 (10), e0163773. doi: 10.1371/journal.pone.0163773
Singer A. J., Clark R. A. F. (1999). Cutaneous wound healing. N. Engl. J. Med. 341 (10), 738–746. doi: 10.1056/NEJM199909023411006
Smulders D., Verbeke G., Mormède P., Geers R. (2006). Validation of a behavioral observation tool to assess pig welfare. Physiol. Behav. 89 (3), 438–447. doi: 10.1016/j.physbeh.2006.07.002
Keywords: growing pigs, leukocyte coping capacity, heat stress, swine, welfare, stress physiology
Citation: Byrd CJ and Young JM (2023) Evaluating the effect of a mild cycling heating period on leukocyte coping capacity in growing pigs. Front. Anim. Sci. 4:1148218. doi: 10.3389/fanim.2023.1148218
Received: 19 January 2023; Accepted: 11 April 2023;
Published: 21 April 2023.
Edited by:
E. Tobias Krause, Friedrich-Loeffler-Institute, GermanyReviewed by:
Ulrike Gimsa, Research Institute for Farm Animal Biology, GermanySam Ho, Gift of Hope Organ and Tissue Donor Network, United States
Copyright © 2023 Byrd and Young. This is an open-access article distributed under the terms of the Creative Commons Attribution License (CC BY). The use, distribution or reproduction in other forums is permitted, provided the original author(s) and the copyright owner(s) are credited and that the original publication in this journal is cited, in accordance with accepted academic practice. No use, distribution or reproduction is permitted which does not comply with these terms.
*Correspondence: Christopher J. Byrd, christopher.byrd@ndsu.edu