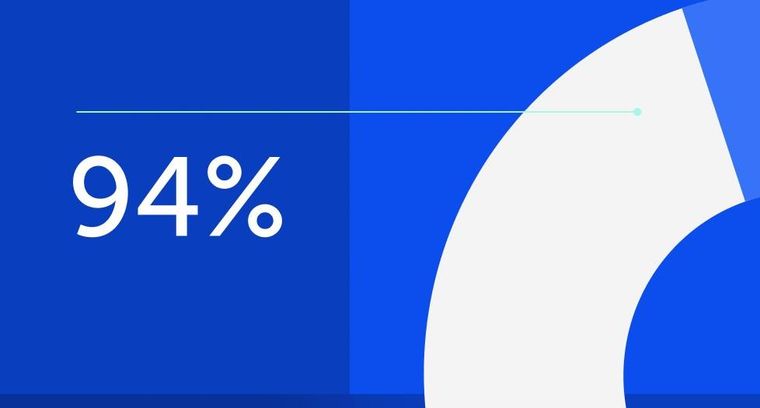
94% of researchers rate our articles as excellent or good
Learn more about the work of our research integrity team to safeguard the quality of each article we publish.
Find out more
PERSPECTIVE article
Front. Anim. Sci., 07 March 2023
Sec. Animal Nutrition
Volume 4 - 2023 | https://doi.org/10.3389/fanim.2023.1135381
This article is part of the Research TopicMitigating the Impact of Animal Production on the Environment: The Ecosystem IntegrationView all 8 articles
In this paper, we propose to view the sustainability of dairy farming as nested within the sustainability of agriculture, a subset of the sustainability of food systems, which in turn could be construed as a subset of the national commitments of a country to achieve the Sustainable Development Goals (SDGs). Disciplinary, multidisciplinary, and interdisciplinary research are essential to study bio-physical system components and their interactions. However, when dairy farming is viewed as nested within broader societal systems, the inclusion of human elements calls for transdisciplinary research. Few of the 17 SDGs are left untouched by the livestock sector. Research should aim at identifying relevant farm-level metrics that are in alignment with any of the 231 indicators supporting the SDGs. We used two examples to illustrate the approach. In the first, SDG 13 (Climate Action) is used as a reminder that despite the current emphasis on reducing milk carbon footprint (kg CO2-e/kg milk), the contribution of the sector to Climate Action depends on reducing its annual emission (kg CO2-e/year; indicator 13.2.2). In the second example, indicator 2.4.1 (land use for sustainable agriculture) of SDG 2 (Zero Hunger) is used to illustrate the potential tradeoffs between Milk N/Intake N as a metric of nitrogen use efficiency at the cow level and metrics such as the input:output ratio of human-edible protein (Milk N/Intake of human-edible N) that prioritize the use of human-inedible feed in dairy rations as a way to enhance efficiency and circularity at the food system level.
Dairy farm sustainability issues are often categorized as either economic, environmental or social (von Keyserlingk et al., 2013), but few sustainability-related publications deal with the three dimensions simultaneously (Segerkvist et al., 2020), let alone with their interactions. The dimensions are however rarely independent of each other and a systems approach is necessary for a full accounting of the interconnections between economic performance, environmental protection, and societal welfare (Glavič and Lukman, 2007). Tradeoffs and synergies must be identified and understood for a full assessment of sustainability. Thus, our objectives were, first to explore definitions of sustainability-related terms to highlight the complex conceptual framework they oftentimes embody. Second, to analyze various perspectives and research approaches purported to strengthen the sustainability of agricultural and dairy systems. Third, to illustrate with two examples the need to consider metrics of dairy systems sustainability that are in alignment with the Sustainable Development Goals (SDGs).
As an adjective, sustainable refers to techniques or methods of harvesting or using a resource without depleting it or damaging it permanently. As a verb, to sustain means to support from below, to maintain the existence, to nurture, to prolong into the future. The ability to be sustained indefinitely or “sustain-ability” as a concept was brought to the collective consciousness in the late 1980s with the Bruntland report of the United Nations. The report titled “Our Common Future” referred to sustainable development as development that meets the needs of the present without compromising the ability of future generations to meet their own needs (UN-WCED, 1987). At the time, the economic growth and development that occurred post World War II had proceeded with little regard to the serious environmental degradation it was causing (i.e., industrial pollution). Thus, the emphasis was to link economic development to the protection of environmental resources (air and water quality primarily). Over time, social sustainability was added as a third pillar or dimension to address concerns associated with the fulfillment of human capacity in a more just and equitable society (Kuhlman and Farrington, 2010). In an attempt to clarify terminology, Glavič and Lukman (2007) referred to sustainable development as a process or evolution of human society, which (a) should align with environmental and natural processes (i.e., the law of nature and biology), (b) recognize the limitation of resources (economic, societal, and environmental) and (c) can be applied on local, regional, national and international levels based on political will. However, how to precisely define, how to measure, and how to operationalize sustainable development in various societal domains remain a work in progress within the scientific community (Gibbes et al., 2020; Ruggerio, 2021). In the supra-national policy realms, however, world leaders gathered under the auspices of the United Nations in 2015 have adopted a 15-year agenda of 17 SDGs (UN-DESA, 2022) supported by 169 targets and 232 indicators (UN-SD, 2022). These goals are not one-size-fits-all approaches to sustainable development. On the contrary, governments of the 178 signatory nations are expected to take ownership and establish frameworks that are relevant in their national contexts and priorities. The SDGs center on partnerships to address poverty, malnutrition, economic growth, social issues of justice and equity, and fulfillment of human capacity while addressing climate change and other environmental concerns. They offer a framework for a holistic exploration of benefits, synergies, tradeoffs, and adverse side-effects of technological innovation in agricultural and food systems (Herrero et al., 2020; Herrero et al., 2021) and as a guide to their transformation (FAO, 2018b). As illustrated in Figure 1, few SDGs are left untouched by the livestock sector at the global scale (FAO, 2018c).
Figure 1 The 17 Sustainable Development Goals and the contribution of livestock categorized as opportunity (), challenge (
) or neutral (
) as per FAO (2018a).
Dairy farming is site specific and enabled by economic, environmental, and social contexts. Thus, one way to define its sustainability is by deduction from the broader (legal) definition of sustainable agriculture of the United States (USDA-NAL, 1999), which is “an integrated system of plants and animals that have site-specific applications that will over the long term (a) satisfy human food and fiber needs, (b) enhance environmental quality and the natural resource base upon which the agricultural economy depends, (c) make most efficient use of non-renewable resources and on-farm resources, and integrate when appropriate natural biological cycles and controls, (d) sustain the economic viability of farm operation and (e) enhance the quality of life of the farmer and the society at large.” Thus, in addition to its contribution to food security, dairy farming is sustainable to the extent that it (a) yields profitable farm income, (b) promotes environmental stewardship, and (c) enhances quality of human life. Some authors have suggested that the contributions of dairy farming (and dairy products) to human health and nutrition be included as a relevant indicator of the sector’s sustainable development (Clay et al., 2020) and its contribution to the SDGs (FAO, 2018c; Mottet et al., 2020).
Interestingly, the roles and contributions of dairy farming to sustainable food systems are the subject of debates that are unfolding in distinct ways in high-income countries compared to low-and-middle-income countries (UN, 2021). In high-income countries, milk is produced for the most part as a commodity in specialized operations. The increased awareness of the high environmental impact of intensive animal agriculture relative to food crops (Eshel et al., 2014), the rise of health concerns associated with excess consumption of animal-based products (Tilman and Clark, 2014; Willett et al., 2019), and animal welfare (Weary and von Keyserlingk, 2017) are examples of concerns that are leading consumers to prefer food deemed better for the environment, considered healthier, causing no harm to animals, not too expensive, and supportive of local economies (Schiano and Drake, 2021). The latter authors noted that the public perception has been driven mainly by marketing messages focused on idealistic narratives. By contrast in low-and-middle-income countries, milk and meat are produced for the most part in less-specialized operations where the multi-functional dimensions of livestock systems are still in full display (Herrero et al., 2013). For example, they remain essential to food security, diet diversification, and the livelihoods of smallholders (Fraval et al., 2019); they may contribute to sustainable intensification and risk management (Thornton and Herrero, 2015), and may contribute to women’s empowerment and gender equity (Doss et al., 2017). Recent FAO publications have illustrated how livestock agriculture practiced in the context of agroecological principles has contributed to the sustainability of food and agriculture (FAO, 2018a) and provided case studies of its contributions to the 17 SDGs (FAO, 2018c). Notably, certain traits that characterize the mixed crop-livestock systems of low-and-middle-income countries — such as greater diversification, greater reliance on family labor, deeper roots in local economies, and lower purchased inputs compared to specialized systems — are the foundation of their sustainability and resilience.
Dairy systems are extremely diverse not only across regions of the world but even within narrowly-defined geographical areas. There are many ways to define and characterize them. Descriptors are often used to provide a snapshot or a trait that encapsulates a dominant feature; the emphasis may be on the mode of production (e.g., pasture-based, organic, or conventional systems) or the size of the operation (e.g., family, small-scale or industrial systems). Similarly in low-and-middle-income countries, terms such as subsistence, market-oriented, pastoral, or peri-urban are frequently used to characterize dairy systems. Although these descriptors are useful, it is upon the analyst to define the system in unequivocal terms as a prerequisite to address their sustainability. Thus, for our purpose, a dairy system refers (implicitly or explicitly) to an entity with bio-physical or conceptual boundaries made of internal interacting components (sub-systems), organized to complete one or more functions (goals), and characterized by a set of behavioral relations (interactions) with its surroundings (“outside” entities). Purpose, elements, and interconnections have been identified as the hallmarks of system thinking, the requisite to systems research (Arnold and Wade, 2015). Intended to tackle complexities, system analysis remains limited however by the model it relies upon. As noted by Jones et al. (2017) systems are an abstraction (simplification) of the real world, made by “specialists” for specific purposes.
According to Drinkwater et al. (2016) sustainability emerges from the complex relationships among the economic, environmental, and social components of a system and therefore cannot be reduced to performance on a single component. Beede (2013) identified three “schools of thought” related to the sustainability of animal agriculture. The first school is centered on the historical and fundamental function of agriculture, as an economic activity to supply food and fiber to a growing population. This approach emphasizes market drivers of supply and demand. To sustain the economic viability of the farm, farmers are expected to manage resources efficiently. Greater efficiency (defined as greater output per unit of input) is more desirable because it is viewed as the source of greater profitability for farmers and greater affordability for consumers. In addition, greater efficiency is viewed as the most relevant way to address environmental concerns. In the second school, there is a recognition that farming practices have an environmental cost that cannot be ignored. In this case, the stewardship of natural resources over time is at the core of sustainability. For self-interest and the “common good” in the long term, farmers should maintain the natural resource base upon which the agricultural economy depends. The third school places agriculture in a societal and institutional context. Agriculture is viewed as a component of food systems, which is only one of the sectors of a national economy. In this context, it is sustainable insofar as it “enhances … the society at large.” In this most integrated school of thought, agriculture is shaped in part by societal concerns. The livelihood that farmers derive from their activities and practices is predicated on doing so in a manner consistent with societal priorities established either through local market forces (e.g., consumers’ preferences and concerns), national policies (e.g., agricultural subsidies, food safety regulations, investment in R&D), and the global context (e.g., international trade agreements). Beede (2013) presents these schools as a five-decade-long evolution of ways of conceptualizing sustainability, privileging first economic, then environmental, and more recently the social concerns. To clarify, we believe that economic, environmental, and social concerns always exist at farm, food system, and societal levels and they are in constant interplay within and across levels (see Figure 2). Finally, it should be noted that insofar as these schools of thought emphasize food security, environmental protection, and social concerns, they agree with the definition of sustainable agriculture (as noted by italicized text above), and they align directly with multiple SDGs.
Figure 2 Dairy farm sustainability viewed as nested within agricultural sustainability, food systems sustainability, and the national commitment of a country to achieve the Sustainable Development Goals. The dotted arrows are meant to illustrate that basic life sciences (e.g., biochemistry and genetics) serve as the foundation for the research of biological farm components (e.g., cow and crop) whereas the basic social sciences (e.g., economic and psychology) serve as the foundation of the research in the social farm components (e.g., economic viability and a farmer’s decision-making process); The colored circles are meant to illustrate the three pillars of sustainability (economic, environmental and social) with varying degrees of shade to illustrate that relevant indicators within each pillar may differ across systems; The solid arrows are meant to illustrate that sustainability is not influenced only by the interactions of the pillars within a system but also by the drivers of the systems within which it is nested; The five roman numerals borrowed from Mottet et al. (2020) are meant to illustrate the need for added dimensions of assessment as a result of broadening the scope (boundaries) of the system; and the gray scale boxes are meant to illustrate the scientific paradigms as a gradual sliding tendency.
The reductionist research paradigm focuses mainly on system components whereas holism emphasizes interactions (Gershenson, 2013). The most appropriate approach to address a researchable question depends on how the problem is defined and how the “real world” is modeled. Here, we use the leakage of nitrogen from dairy systems to suggest that reductionist approaches are necessary to address dairy sustainability when defined as an issue of managing economic resources (i.e., the first school of thought) or minimizing environmental impact (i.e., the second school of thought) but insufficient to address the same issue in its most integrated and contemporary conceptualization (i.e., the third school of thought). Arguably disciplinary research can be used to address the efficiency of nitrogen utilization of dairy system components. For example, nutritionists may research ration formulations to avoid unnecessary purchases of expensive protein supplements while maximizing productivity and minimizing nitrogen losses in cow manure (Arndt et al., 2015). Similarly, agronomists may research ways to optimize nitrogen fertilizer purchases and cropping systems while maximizing productivity, minimizing the cost of production, and the risk of leaching or runoff (Martin et al., 2017). However, focusing independently on the cows and the crops is insufficient to address the issue at the level of the farm viewed as an integrated system. The nitrogen loss associated with a urination event of a cow is drastically different if the cow is standing on a pasture or the concrete floor of a barn. This example is meant to illustrate that when multiple disciplines come together and thus system boundaries are expanded, a new model must be drawn to align elements, purpose, and interconnections with the real-world system to simulate. Both empirical studies (Powell et al., 2017) and computer simulation (Pellerin et al., 2017) have been used to address nitrogen loss at a farm level. Thus, interdisciplinary research allows for addressing problems at a greater level of integration than is possible within a discipline. In turn, if a dairy farm is viewed not as a system but a sub-system of a broader social organization (e.g., the third school of thought), addressing the leakage of nitrogen from the farm is no longer a uniquely natural sciences problem because various stakeholders with various (synergetic or antagonistic) interests bring their own perspective on what the problem is, how it affects them, and how it might be solved. For example, local residents may become incommoded by the ammonia emitted from manure, businesses relying on recreational activities on local lakes and rivers may become concerned by the degradation of water quality associated with nitrogen runoffs, or public health authorities may get concerned about nitrate contamination of water from wells. Bringing the viewpoint of distinct stakeholders to bear turns the nitrogen losses from a farm into a “wicked” problem. As outlined by Peterson (2013), wicked problems have emerged from the social sciences as a category of problems with the following characteristics: (a) No definitive formulation of the problem exists (i.e., various stakeholders have “equally valid” definitions of the problem), (b) stakeholders have radically different frames of reference concerning the problem (i.e., people’s values clashes with one another), (c) the “solution” is not true or false, but rather better or worse (i.e., there is not a single analytical solution), (d) the underlying cause-and-effect relationships are complex, systemic, and either unknown or highly uncertain (i.e., complex interdependencies make it difficult to predict behavior change). As such, a wicked problem is essentially not “solvable”, but can be managed over time. The controversy about the use of recombinant bovine growth hormone (Thompson, 2020), which can be used to enhance milk production and feed conversion efficiency of dairy cows provides an example of a technology with contradictory outcomes depending on the perspective (economic, environmental, or social) used to define its impact on sustainability.
Gershenson (2013) posited that holism rather than reductionism is better suited to address philosophical and social problems. Thus, achieving the multi-dimensionality necessary for a systemic assessment of sustainability inclusive of contrasting views and the ethical concerns of multiple stakeholders requires long-term team efforts that include natural scientists, social scientists, and non-academic partners (Wilmer et al., 2018). This type of research has been referred to as transdisciplinary (Pohl, 2011). Transdisciplinary research does not rely on scientific discoveries alone to address issues, but it is inclusive and respectful of other forms of knowledge, emphasizing the co-creation of knowledge and shared governance. Although transdisciplinary research is still in an early stage of development and there remain considerable barriers to its implementation (Brandt et al., 2013; Kurian, 2017), some have advocated the need for such an approach to guide the research focused on sustainable development at the global scale (Shrivastava et al., 2020), the transition toward greater sustainability of agriculture (Reganold et al., 2011), the dairy sector in the U.S. (von Keyserlingk et al., 2013), and smallholder farming systems in low-and-middle-income countries (Dubé et al., 2012).
Thus, the scientific approaches related to dairy systems sustainability lie on a spectrum (Figure 2). At one end sustainability research is made of a series of discrete issues to be addressed by disciplinary research (inward looking) and at the other end lies the transdisciplinary efforts to address the concerns of multiple stakeholders (outward looking). Hence, Figure 2 shows the sustainability of dairy farming as nested within the sustainability of agriculture, which itself is a subset of the sustainability of the food systems, which in turn could be construed as a subset of the national commitments of a country to achieve the SDGs. Indicators of sustainability must differ at each level. Two examples will be used here to illustrate that indicators that are used in disciplinary research to improve the efficiency of the existing system are inadequate in the context of transdisciplinary research aimed at transitioning (transforming) the system.
SDG 13 urges national governments to integrate climate change measures into national policies, strategies, and planning to combat its detrimental impacts. As one of the eight indicators of this goal, indicator 13.2.2. refers to [reduction of] total greenhouse gas emissions per year (UN-SD, 2022). However, FAO-GDP (2018) reported that the combination of increased cow population (+11%) and productivity (+15%) resulted in a 30% increase in global milk supply between 2005 and 2015. Although the global milk carbon footprint was reduced by 11% (from 2.8 to 2.5 kg CO2-eq/kg fat-protein-corrected milk), the improvement fell short of compensating for the increase in the size of the industry resulting in an 18% increase in total emission during that 10-year period. The report however suggested that without improvement in efficiency the total greenhouse gas emission from the sector would have increased by 38%. A similar approach was used to study the California dairy systems by Naranjo et al. (2020) who reported a 46% reduction in cradle-to-farmgate milk carbon footprint from 1964 to 2014 (2.11 vs. 1.14 kg CO2-eq per kg of energy-corrected milk) but a 166% increase in total emission (8.2 vs. 21.8 Mt of CO2-eq per year). The authors also noted that without improvement in efficiency, the total emission in 2014 would have been 39.7 Mt of CO2-eq. Focusing on milk carbon footprint may be useful in many respects, but alone it is insufficient to address climate change. Despite the current emphasis on reducing milk carbon footprint (Hagemann et al., 2011; Grossi et al., 2019) there are concerns about the limitations and biases of the current life cycle assessment (LCA) methodology upon which these findings are derived. First, quantifying emissions remains a serious limitation, especially in developing countries (Munidasa et al., 2021). Second, the LCA methodology is still at an early stage to account for potential carbon sinks such as carbon sequestration in soil organic matter (IDF, 2022) or opportunities for carbon offset through manure bio-digestion (Aguirre-Villegas and Larson, 2017). Third, concerns have been raised also about the functional unit (Salou et al., 2017; Letelier et al., 2022a), the emission allocation methods (Mazzetto et al., 2022; Letelier et al., 2022a), as well as biases that appear when standard milk LCA procedures are applied to dual-purpose breed (Zehetmeier et al., 2012) or applied to low-income countries systems where livestock have multiple functionalities (Weiler et al., 2014). The strong emphasis on milk carbon footprint in the research realms may have had implications for policy-making. In a review of the national-level dairy policy of 23 countries York et al. (2018) indicated that policies aimed at reducing emission intensity (i.e., milk carbon footprint) were most preferred (n = 58 of 62) but those aimed at reducing sector size were largely ignored (n = 4 of 62). As suggested above, the national priorities and the multiple contributions of the dairy sector to the SDGs might provide a contextualized framework to address questions related to the desired size of the sector. To achieve a net zero emission by 2050 (as agreed upon by the Paris Accords) the sector will have to decrease its annual emissions and find ways to compensate for inevitable emissions (FAO-GDP, 2018).
“End hunger, achieve food security and improved nutrition, and promote sustainable agriculture” is the official wording of SDG 2. This goal includes 14 indicators focused on 4 main areas, two of which are of direct interest here to discuss the contribution of milk protein production to ending all forms of malnutrition and ensuring sustainable food production systems. Using FAO data, we found that across 163 countries national supply expressed as g per capita per day of plant-source protein was (means ± standard deviation) 44.3 ± 9.0 and 35 ± 19.8 for animal-source protein (Wattiaux, 2017). Notwithstanding considerable losses and wastes (FAO, 2019), these levels of supply are likely sufficient to meet human protein requirement- which has been set at 0.99 g per kg per day (Leser, 2013) or approximately 64 g for an individual with a body weight of 65 kg- in most parts of the world except for some sub-Saharan countries. The production of milk and thus milk protein contributes to SDG 2, but especially in low-and-middle income countries where supply is still limited (FAO, 2013). Given that nitrogen is a major driver of agricultural production (Erisman et al., 2008) and its leakage into the environment has exceeded the safe planetary boundaries to avoid major disruption to earth systems (Steffen et al., 2015), the efficiency with which various forms of nitrogen inputs are converted to human-edible protein (HEP) should be considered carefully. However, the adoption of a disciplinary lens versus a transdisciplinary lens may lead to divergent if not contradictory pathways going forward. In the case of the dairy sector, protein nutrition of dairy cattle has been a subject of study for many decades (Schwab and Broderick, 2017) and one of the main paradigms of the dairy nutrition discipline has been to improve nitrogen use efficiency (NUE) defined as the nitrogen secreted in the milk (essentially as high-quality HEP) divided by nitrogen intake of the cow on a daily basis (Calsamiglia et al., 2010; Broderick, 2018). The rationale to continue this line of research is based on the findings that a third or less of the nitrogen consumed by a cow is converted into milk protein and the remaining two-thirds or more are excreted in the manure either as fecal or urinary N (Spanghero and Kowalski, 2021; Bougouin et al., 2022). The sub-optimal conversion efficiency was also highlighted in the study of Spek et al. (2013) in which cows in North America and Northern Europe were found to produce more urinary nitrogen (essentially in the form of urea) than milk nitrogen in the form of HEP on a daily basis. Dietary supplementation of amino acids (Lobos et al., 2021) or avoiding excess of dietary crude protein (Letelier et al., 2022b) are two examples of disciplinary research efforts intended to increase NUE of dairy cows. In contrast, other researchers have approached the issue of NUE as a part of the broader debate of using resources to produce feed (for animals) vs. food (for humans) (Gill, 2013; Sijpestijn et al., 2022). Some researchers have proposed to measure the efficiency of animal protein production as HEP in the product divided by HEP consumed by the animal (CAST, 1999; Wilkinson and Lee, 2018) or a similar output/input ratio based on human-digestible essential amino acids (Patel et al., 2016). In both cases, a ratio greater than 1.0 indicates that the animal system is a net contributor to human supply and thus is not competing against humans. In contrast, values less than 1.0 indicate that the animal system is taking valuable forms of nitrogen away from the human supply chain. Wilkinson and Lee (2018) demonstrated that with increasing human-inedible feed resources in the diet of dairy cows, the net contribution of the dairy systems to HEP supply increases, but both animal productivity (milk protein production per cow per day) and the NUE decrease. Similarly, research by van Hal et al. (2019) suggested that relying on human-inedible food sources only (i.e., grass, food waste, and by-product feed) as feed sources for livestock could yield as much as 31 g of HEP per European per day, with dairy being consistently selected as the most desirable form of production compared to pig meat, poultry meat plus eggs, poultry meat, or beef meat. Furthermore, given the low quality of the feed resources, medium-to-low-producing animals were found to be best suited to convert these feed resources into animal products rather than high-productivity animals. Thus, relying on HEP to assess what may be deemed as desirable for society at large may come in conflict with the disciplinary research paradigm suggesting that a decline in animal productivity may have negative profitability implications for the farmer and a lower NUE may have negative environmental implications. As noted by Gill (2013), there is no one ideal solution to these dilemmas (i.e., wicked problems). We posit, however, that the national priorities and the multiple contributions of the dairy sector to the SDGs might provide a contextualized framework to guide related research and policy-making.
The concerns of the dairy research community for sustainability and the SDGs are still at an early stage and more needs to be done. In a search of the Agricola, Web of Science, CABI abstracts, and Scopus databases we found only 11 non-duplicate hits from a search of peer-reviewed studies published since 2015 including “milk or dairy” in the title “sustainability” in the abstract, and “sustainable development goals” in any field. Notwithstanding substantial differences in the drivers of the sustainability of the dairy sector across nations of the world, there are issues of universal concerns that merit attention such as the reduction of milk lost or wasted (FAO, 2011) and the contribution of milk and dairy products to sustainable diets (Miller et al., 2020), child growth and development (Balehegn et al., 2019; Miller et al., 2021) and human health across stages of life (FAO, 2013; Givens, 2020). At the farm level, however, science-based knowledge is needed for sound decision-making by dairy farmers and other stakeholders operating in a variety of contexts. Work is needed to make current systems more efficient while exploring transition pathways that are more systemic and transformative. A diversity of research approaches should be employed at various scales to foster the co-existence of diverse dairy systems that contribute to the sustainability of agriculture and food systems within national priorities to achieve the SDGs. Adaptation of current tools such as the one proposed by Mottet et al. (2020) and creation of new indicators will be needed to capture the holistic contributions of dairy production to the SDGs across the globe.
The original contributions presented in the study are included in the article. Further inquiries can be directed to the corresponding author.
The author declares his full and complete contributions to all the phases of development of this manuscript.
The author is grateful to MaryGrace Erickson and Dante Pizzaro for their editorial suggestions.
The author declares that the research was conducted in the absence of any commercial or financial relationships that could be construed as a potential conflict of interest.
All claims expressed in this article are solely those of the authors and do not necessarily represent those of their affiliated organizations, or those of the publisher, the editors and the reviewers. Any product that may be evaluated in this article, or claim that may be made by its manufacturer, is not guaranteed or endorsed by the publisher.
Aguirre-Villegas H. A., Larson R. A. (2017). Evaluating greenhouse gas emissions from dairy manure management practices using survey data and lifecycle tools. J. Cleaner Prod. 143, 169–179. doi: 10.1016/j.jclepro.2016.12.133
Arndt C., Powell J. M., Aguerre M. J., Wattiaux M. A. (2015). Performance, digestion, nitrogen balance, and emission of manure ammonia, enteric methane, and carbon dioxide in lactating cows fed diets with varying alfalfa silage-to-corn silage ratios. J. Dairy Sci. 98 (1), 418–430. doi: 10.3168/jds.2014-8298
Arnold R. D., Wade J. P. (2015). A definition of systems thinking: A systems approach. Proc. Comput. Sci. 44, 669–678. doi: 10.1016/j.procs.2015.03.050
Balehegn M., Mekuriaw Z., Miller L., Mckune S., Adesogan A. T. (2019). Animal-sourced foods for improved cognitive development. Anim. Front. 9 (4), 50–57. doi: 10.1093/af/vfz039
Beede D. K. (2013). “Chapter 18. animal agriculture: How can it be sustainable in the future?,” in Sustainable animal agriculture. Ed. Kebreab E. (Boston, MA; USA: CABI), 284–311.
Bougouin A., Hristov A., Dijkstra J., Aguerre M. J., Ahvenjärvi S., Arndt C., et al. (2022). Prediction of nitrogen excretion from data on dairy cows fed a wide range of diets compiled in an intercontinental database: A meta-analysis. J. Dairy Sci. 105 (9), 7462–7481. doi: 10.3168/jds.2021-20885
Brandt P., Ernst A., Gralla F., Luederitz C., Lang D. J., Newig J., et al. (2013). A review of transdisciplinary research in sustainability science. Ecol. Econ. 92, 1–15. doi: 10.1016/j.ecolecon.2013.04.008
Broderick G. A. (2018). Review: Optimizing ruminant conversion of feed protein to human food protein. Animal 12 (8), 1722–1734. doi: 10.1017/S1751731117002592
Calsamiglia S., Ferret A., Reynolds C. K., Kristensen N. B., van Vuuren A. M. (2010). Strategies for optimizing nitrogen use by ruminants. Animal 4 (Special Issue 07), 1184–1196. doi: 10.1017/S1751731110000911
CAST (1999) Animal agriculture and global food supply. task force report no 135 (Council for Agricural science and Technology). Available at: https://www.cast-science.org/publication/animal-agriculture-and-global-food-supply/ (Accessed December 26 2022).
Clay N., Garnett T., Lorimer J. (2020). Dairy intensification: Drivers, impacts and alternatives. Ambio 49 (1), 35–48. doi: 10.1007/s13280-019-01177-y
Doss C., Meinzen-Dick R., Quisumbing A., Theis S. (2018). Women in agriculture: Four myths. Global Food Secur 16, 69–74. doi: 10.1016/j.gfs.2017.10.001
Drinkwater L. E., Friedman D., Buck L. (2016). Systems research for agriculture: Innovative solutions to complex challenges. SARE Handbook Series 13. (College Park, MD, USA: Sustainable Agriculture Research and Education (SARE)).
Dubé L., Pingali P., Webb P. (2012). Paths of convergence for agriculture, health, and wealth. Proc. Natl. Acad. Sci. 109 (31), 12294–12301. doi: 10.1073/pnas.0912951109
Erisman J. W., Sutton M. A., Galloway J., Klimont Z., Winiwarter W. (2008). How a century of ammonia synthesis changed the world. Nat. Geosci 1 (10), 636–639. doi: 10.1038/ngeo325
Eshel G., Shepon A., Makov T., Milo R. (2014). Land, irrigation water, greenhouse gas, and reactive nitrogen burdens of meat, eggs, and dairy production in the united states. Proc. Natl. Acad. Sci 111 (33), 11996–12001. doi: 10.1073/pnas.1402183111
FAO (2013). Milk and dairy products in human nutrition (Rome, Italy: FAO). Available at: https://www.fao.org/3/i3396e/i3396e.pdf.
FAO (2018a) Livestock and agroecology: How they can support the transition towards sustainable food and agriculture (Rome, Italy). Available at: https://www.fao.org/3/i8926en/I8926EN.pdf (Accessed December 26 2022).
FAO (2018b). Transforming food and agriculture to achieve the SDGs: 20 interconnected actions to guide decision-makers. 2nd ed. (Rome, Italy: Food and Agriculture Organization (FAO) of the United Nations).
FAO (2018c). World livestock: Tranforming the livestock sector through the sustainabale development goals (Rome: Licence CC BY-NC-SA 3.0 IGO).
FAO (2019) The state of food and agriculture. moving forward on food loss and waste reduction (FAO Rome). Available at: https://www.fao.org/3/ca6030en/ca6030en.pdf (Accessed December 26 2022).
FAO-GDP (2018) Climate change and the global dairy cattle sector - the role of the dairy sector in a low-carbon future. Available at: https://www.fao.org/3/CA2929EN/ca2929en.pdf.
Fraval S., Hammond J., Bogard J. R., Ng'endo M., van Etten J., Herrero M., et al. (2019). Food access deficiencies in Sub-saharan Africa: Prevalence and implications for agricultural interventions. Front. Sustain. Food Syst. 3, 104. doi: 10.3389/fsufs.2019.00104
Gershenson C. (2013). The implications of interactions for science and philosophy. Found. Sci. 18 (4), 781–790. doi: 10.1007/s10699-012-9305-8
Gibbes C., Hopkins A. L., Díaz A. I., Jimenez-Osornio J. (2020). Defining and measuring sustainability: A systematic review of studies in rural Latin America and the Caribbean. Environ. Dev. Sustain. 22 (1), 447–468. doi: 10.1007/s10668-018-0209-9
Gill M. (2013). “Converting feed into human food: the multiple dimensions of efficiency,” in Optimization of feed use efficiency in ruminant production systems. Eds. Makkar H. P. S., Beever D. E. (Bangkok, Thailand: FAO Animal Production and Health Proceedings, No. 16. Rome, FAO and Asian-Australasian Association of Animal Production Societies).
Givens D. I. (2020). MILK symposium review: The importance of milk and dairy foods in the diets of infants, adolescents, pregnant women, adults, and the elderly. J. Dairy Sci. 103 (11), 9681–9699. doi: 10.3168/jds.2020-18296
Glavič P., Lukman R. (2007). Review of sustainability terms and their definitions. J. Cleaner Prod. 15 (18), 1875–1885. doi: 10.1016/j.jclepro.2006.12.006
Grossi G., Goglio P., Vitali A., Williams A. G. (2019). Livestock and climate change: impact of livestock on climate and mitigation strategies. Anim. Front. 9 (1), 69–76. doi: 10.1093/af/vfy034
Hagemann M., Hemme T., Ndambi A., Alqaisi O., Sultana M. N. (2011). Benchmarking of greenhouse gas emissions of bovine milk production systems for 38 countries. Anim. Feed Sci. Technol. 166-167, 46–58. doi: 10.1016/j.anifeedsci.2011.04.002
Herrero M., Grace D., Njuki J., Johnson N., Enahoro D., Silvestri S., et al. (2013). The roles of livestock in developing countries. Animal 7 Supplement S1 (March), 3–18. doi: 10.1017/S1751731112001954
Herrero M., Thornton P. K., Mason-D'Croz D., Palmer J., Bodirsky B. L., Pradhan P., et al. (2021). Articulating the effect of food systems innovation on the sustainable development goals. Lancet Planetary Health 5 (1), e50–e62. doi: 10.1016/S2542-5196(20)30277-1
Herrero M., Thornton P. K., Mason-D’Croz D., Palmer J., Benton T. G., Bodirsky B. L., et al. (2020). Innovation can accelerate the transition towards a sustainable food system. Nat. Food 1 (5), 266–272. doi: 10.1038/s43016-020-0074-1
IDF (2022). “Life cycle assessment guidelines for calcuating carbon sequestration in cattle production systems,” in Bulletin of the IDF no 519/2022 (Brussels, Belgium: International Dairy Federation).
Jones J. W., Antle J. M., Basso B., Boote K. J., Conant R. T., Foster I., et al. (2017). Brief history of agricultural systems modeling. Agric. Syst. 155, 240–254. doi: 10.1016/j.agsy.2016.05.014
Kuhlman T., Farrington J. (2010). What is sustainability? Sustainability 2 (11), 3436–3448. doi: 10.3390/su2113436
Kurian M. (2017). The water-energy-food nexus: Trade-offs, thresholds and transdisciplinary approaches to sustainable development. Environ. Sci. Policy 68, 97–106. doi: 10.1016/j.envsci.2016.11.006
Leser S. (2013). The 2013 FAO report on dietary protein quality evaluation in human nutrition: Recommendations and implications. Nutr. Bull. 38 (4), 421–428. doi: 10.1111/nbu.12063
Letelier P., Aguirre-Villegas H. A., Chacón Navarro M., Wattiaux M. A. (2022a). Milk, meat, and human edible protein from dual-purpose cattle in Costa Rica: Impact of functional unit and co-product handling methods on predicted enteric methane allocation. Livestock Sci. 263, 105013. doi: 10.1016/j.livsci.2022.105013
Letelier P., Zanton G. I., Wattiaux M. A. (2022b). Production performance of Holstein cows at 4 stages of lactation fed 4 dietary crude protein concentrations. J. Dairy Sci. 105, 9581–9596. doi: 10.3168/jds.2022-22146
Lobos N. E., Wattiaux M. A., Broderick G. A. (2021). Effect of rumen-protected lysine supplementation of diets based on corn protein fed to lactating dairy cows. J. Dairy Sci. 104 (6), 6620–6632. doi: 10.3168/jds.2020-19835
Martin N. P., Russelle M. P., Powell J. M., Sniffen C. J., Smith S. I., Tricarico J. M., et al. (2017). Invited review: Sustainable forage and grain crop production for the US dairy industry. J. Dairy Sci. 100 (12), 9479–9494. doi: 10.3168/jds.2017-13080
Mazzetto A. M., Falconer S., Ledgard S. (2022). Mapping the carbon footprint of milk production from cattle: A systematic review. J. Dairy Sci. 105 (12), 9713–9725. doi: 10.3168/jds.2022-22117
Miller G. D., Kanter M., Rycken L., Comerford K. B., Gardner N. M., Brown K. A. (2021). Food systems transformation for child health and well-being: the essential role of dairy. Int. J. Environ. Res. Public Health 18 (19), 14. doi: 10.3390/ijerph181910535
Miller G. D., Slimko M., Tricarico J., Peerless D. (2020). Food system sustainability: A dairy perspective. Nutr. Today 55 (2), 82–85. doi: 10.1097/NT.0000000000000401
Mottet A., Bicksler A., Lucantoni D., De Rosa F., Scherf B., Scopel E., et al. (2020). Assessing transitions to sustainable agricultural and food systems: A tool for agroecology performance evaluation (TAPE). Front. Sustain. Food Syst. 4, 252. doi: 10.3389/fsufs.2020.579154
Munidasa S., Eckard R., Sun X., Cullen B., McGill D., Chen D., et al. (2021). Challenges and opportunities for quantifying greenhouse gas emissions through dairy cattle research in developing countries. J. Dairy Res. 88 (1), 3–7. doi: 10.1017/S0022029921000182
Naranjo A., Johnson A., Rossow H., Kebreab E.. (2020). Greenhouse gas, water, and land footprint per unit of production of the California dairy industry over 50 years. J. Dairy Sci. 103 (4), 3760–3773. doi: 10.3168/jds.2019-16576
Patel M., Sonesson U., Hessle A. (2016). Upgrading plant amino acids through cattle to improve the nutritional value for humans: effects of different production systems. animal 11 (3), 519–528. doi: 10.1017/S1751731116001610
Pellerin D., Charbonneau E., Fadul-Pacheco L., Soucy O., Wattiaux M. A. (2017). Economic effect of reducing nitrogen and phosphorus mass balance on Wisconsin and quèbec dairy farms. J. Dairy Sci. 100 (10), 8614–8629. doi: 10.3168/jds.2016-11984
Peterson H. C. (2013). “Chapter 1 sustainability: A wicked problem,” in Sustainability of animal agriculture. Ed. Kebreab E. (Boston, MA: CABI International), 1–9.
Pohl C. (2011). What is progress in transdisciplinary research? Futures 43 (6), 618–626. doi: 10.1016/j.futures.2011.03.001
Powell J. M., Barros T., Danes M., Aguerre M., Wattiaux M., Reed K. (2017). Nitrogen use efficiencies to grow, feed, and recycle manure from the major diet components fed to dairy cows in the USA. Agriculture Ecosyst. Environ. 239, 274–282. doi: 10.1016/j.agee.2017.01.023
Reganold J. P., Jackson-Smith D., Batie S. S., Harwood R. R., Kornegay J. L., Bucks D., et al. (2011). Transforming U.S. agriculture. Science 332 (6030), 670–671. doi: 10.1126/science.1202462
Ruggerio C. A. (2021). Sustainability and sustainable development: A review of principles and definitions. Sci. Total Environ. 786, 147481. doi: 10.1016/j.scitotenv.2021.147481
Salou T., Le Mouël C., van der Werf H. M. G. (2017). Environmental impacts of dairy system intensification: the functional unit matters! J. Cleaner Prod. 140, 445–454. doi: 10.1016/j.jclepro.2016.05.019
Schiano A. N., Drake M. A. (2021). Invited review: Sustainability: Different perspectives, inherent conflict. J. Dairy Sci. 104 (11), 11386–11400. doi: 10.3168/jds.2021-20360
Schwab C. G., Broderick G. A. (2017). A 100-year review: Protein and amino acid nutrition in dairy cows. J. Dairy Sci. 100 (12), 10094–10112. doi: 10.3168/jds.2017-13320
Segerkvist K. A., Hansson H., Sonesson U., Gunnarsson S. (2020). Research on environmental, economic, and social sustainability in dairy farming: A systematic mapping of current literature. Sustainability (Basel, Switzerland) 12 (14), 5502. doi: 10.3390/su12145502
Shrivastava P., Stafford Smith M., O’Brien K., Zsolnai L. (2020). Transforming sustainability science to generate positive social and environmental change globally. One Earth 2 (4), 329–340. doi: 10.1016/j.oneear.2020.04.010
Sijpestijn G. F., Wezel A., Chriki S. (2022). Can agroecology help in meeting our 2050 protein requirements? Livestock Sci. 256, 104822. doi: 10.1016/j.livsci.2022.104822
Spanghero M., Kowalski Z. M. (2021). Updating analysis of nitrogen balance experiments in dairy cows. J. Dairy Sci. 104 (7), 7725–7737. doi: 10.3168/jds.2020-19656
Spek J. W., Dijkstra J., Duinkerken G.v., Hendriks W. H., Bannink A. (2013). Prediction of urinary nitrogen and urinary urea nitrogen excretion by lactating dairy cattle in northwestern Europe and north America: a meta-analysis. J. Dairy Sci. 96 (7), 4310–4322. doi: 10.3168/jds.2012-6265
Steffen W., Richardson K., Rockström J., Cornell S.E., Fetzer I., Bennett E. M., et al. (2015). Planetary boundaries: Guiding human development on a changing planet. Science 347 (6223), 736–736. doi: 10.1126/science.aaa9629
Thompson P. B. (2020). “Biotechnology, policy and the problem of unintended consequences: The case of rBST,” in Food and agrcultural biotechnology in ehtical perspective, 3rd ed (Cham, Switzerland: Springer Nature), 53–77.
Thornton P. K., Herrero M. (2015). Adapting to climate change in the mixed crop and livestock farming systems in sub-Saharan Africa. Nat. Climate Change 5 (9), 830–836. doi: 10.1038/nclimate2754
Tilman D., Clark M. (2014). Global diets link environmental sustainability and human health. Nature 515 (7528), 518–522. doi: 10.1038/nature13959
UN (2021) United nations food summit 2021 scientific group reports. Available at: https://www.un.org/en/food-systems-summit/documentation (Accessed December 28 2022).
UN-DESA (2022) The 17 goals (New York: UNDESA (United Nations Department of Economic and Social Affairs). Available at: https://sdgs.un.org/goals (Accessed December 19, 2022).
UN-SD (2022) SDG indicators: Global indicator framework for the sustainable development goals and targets of the 2030 agenda for sustainable development (New York: UNSD (United Nations Statisitcs Division). Available at: https://unstats.un.org/sdgs/indicators/indicators-list/ (Accessed December 13, 2022).
UN-WCED (1987) Report of the world commission on environment and development: Our common future. Available at: https://sustainabledevelopment.un.org/content/documents/5987our-common-future.pdf (Accessed December 27 2022).
USDA-NAL (1999) Sustainable agriculture: Definitions of terms. special reference briefs series no. SRB 99-02 (USDA-NAL (United States Department of Agriculture - National Agricultural Library). Available at: https://www.nal.usda.gov/afsic/sustainable-agriculture-definitions-and-terms (Accessed December 19, 2022).
van Hal O., de Boer I. J. M., Muller A., de Vries S., Erb K. H., Schader C., et al. (2019). Upcycling food leftovers and grass resources through livestock: Impact of livestock system and productivity. J. Cleaner Prod. 219, 485–496. doi: 10.1016/j.jclepro.2019.01.329
von Keyserlingk M. A. G., Martin N. P., Kebreab E., Knowlton K. F., Grant R. J., Stephenson M., et al. (2013). Invited review: Sustainability of the US dairy industry. J. Dairy Sci. 96 (9), 5405–5425. doi: 10.3168/jds.2012-6354
Wattiaux M. A. (2017). “Dairy sector across the world: National trends and opportunity for sustainable growth,” in Large Dairy herd management. Ed. Beede D. K. (Champaign, IL: American Dairy Science Association), 1–18.
Weary D. M., von Keyserlingk M. A. G. (2017). Public concerns about dairy-cow welfare: how should the industry respond? Anim. Prod. Sci. 57 (7), 1201–1209. doi: 10.1071/AN16680
Weiler V., Udo H. M. J., Viets T., Crane T. A., De Boer I. J. M. (2014). Handling multi-functionality of livestock in a life cycle assessment: the case of smallholder dairying in Kenya. Curr. Opin. Environ. Sustain. 8, 29–38. doi: 10.1016/j.cosust.2014.07.009
Wilkinson J. M., Lee M. R. F. (2018). Review: Use of human-edible animal feeds by ruminant livestock. Animal 12 (8), 1735–1743. doi: 10.1017/S175173111700218X
Willett W., Rockström J., Loken B., Springmann M., Lang T., Vermeulen S., et al. (2019). Food in the anthropocene: the EAT–lancet commission on healthy diets from sustainable food systems. Lancet 393 (10170), 447–492. doi: 10.1016/S0140-6736(18)31788-4
Wilmer H., Derner J. D., Fernández-Giménez M. E., Briske D. D., Augustine D. J., Porensky L. M. (2018). Collaborative adaptive rangeland management fosters management-science partnerships. Rangeland Ecol. Manage. 71 (5), 646–657. doi: 10.1016/j.rama.2017.07.008
York L., Heffernan C., Rymer C. (2018). A systematic review of policy approaches to dairy sector greenhouse gas (GHG) emission reduction. J. Cleaner Prod. 172, 2216–2224. doi: 10.1016/j.jclepro.2017.11.190
Keywords: research methods, milk carbon footprint, climate change, nitrogen, human edible protein, developing countries, low-income countries, high-income countries
Citation: Wattiaux MA (2023) Sustainability of dairy systems through the lenses of the sustainable development goals. Front. Anim. Sci. 4:1135381. doi: 10.3389/fanim.2023.1135381
Received: 31 December 2022; Accepted: 14 February 2023;
Published: 07 March 2023.
Edited by:
Melissa Duplessis, Agriculture and Agri-Food Canada (AAFC), CanadaReviewed by:
John Moreki, Botswana University of Agriculture and Natural Resources, BotswanaCopyright © 2023 Wattiaux. This is an open-access article distributed under the terms of the Creative Commons Attribution License (CC BY). The use, distribution or reproduction in other forums is permitted, provided the original author(s) and the copyright owner(s) are credited and that the original publication in this journal is cited, in accordance with accepted academic practice. No use, distribution or reproduction is permitted which does not comply with these terms.
*Correspondence: Michel A. Wattiaux, d2F0dGlhdXhAd2lzYy5lZHU=
Disclaimer: All claims expressed in this article are solely those of the authors and do not necessarily represent those of their affiliated organizations, or those of the publisher, the editors and the reviewers. Any product that may be evaluated in this article or claim that may be made by its manufacturer is not guaranteed or endorsed by the publisher.
Research integrity at Frontiers
Learn more about the work of our research integrity team to safeguard the quality of each article we publish.