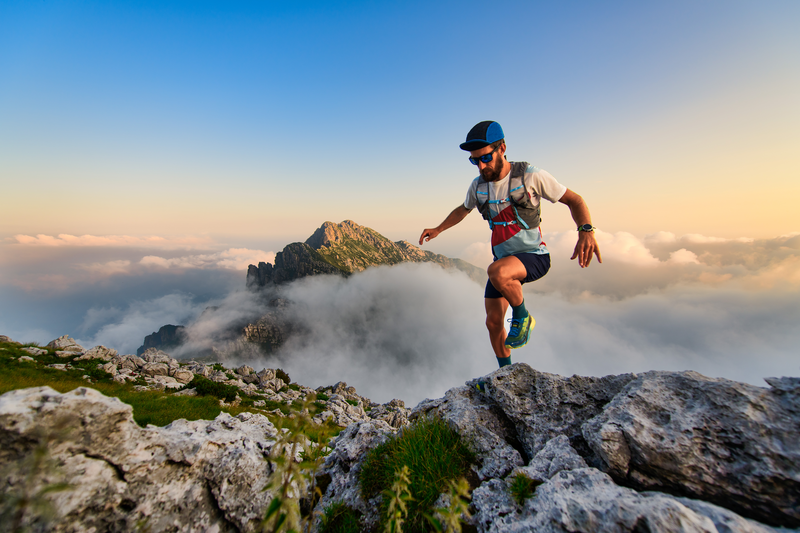
94% of researchers rate our articles as excellent or good
Learn more about the work of our research integrity team to safeguard the quality of each article we publish.
Find out more
ORIGINAL RESEARCH article
Front. Anim. Sci. , 16 January 2023
Sec. Animal Nutrition
Volume 4 - 2023 | https://doi.org/10.3389/fanim.2023.1125061
Oral supplementation of β-glucans may be able to improve the health of companion animals. However, little is understood regarding the effects of yeast β-glucan on diet processing and intestinal function. Therefore, the objectives of this research were to determine the carry through of yeast β-glucan during extruded diet production and its impact on diet utilization by dogs. Three diets were formulated to contain increasing levels of a yeast β-glucan blend at 0, 0.012 and 0.023% inclusion. Processing inputs were held constant during extrusion to allow for evaluation of output parameters and physical characteristics of kibble. Yeast β-glucan concentration was analyzed in extruded diets using the glucan enzymatic method, resulting in >100% recovery. Twenty-four Labrador Retrievers were assigned to one of three dietary groups of 8 dogs each with an equal distribution of sex and age. Dogs were fed dietary treatments for 24-d adaption followed by 4-d total fecal collection. Feces were scored on a 1-5 scale, with 1 representing liquid diarrhea and 5 hard pellet-like with a fecal score of 3.5-4 considered ideal. Fresh fecal samples were collected for analysis of short chain fatty acid concentrations. Apparent total tract digestibility was calculated by total fecal collection (TFC) and titanium (TI) marker methods. Data were analyzed using a mixed model procedure in software (version 9.4, SAS Institute, Inc., Cary, NC). Dry bulk density, kibble diameter, and kibble length did not differ among dietary treatments. Intake was similar among dietary treatments (P > 0.05). Dogs required about 26% more food than estimated [130*BWkg0.75] to maintain body weight among all treatments. Fecal score was not different (P > 0.05) among dietary treatments but was lower than ideal at an average of 2.6. Nutrient digestibility was not affected (P > 0.05) by inclusion of the yeast β-glucan. By method, the TFC procedure resulted in higher (P< 0.05) digestibility values when compared to the TI procedure. In addition, yeast β-glucan did not alter short or branched chain fatty acid proportions. Overall, processing parameters, physical characteristics of kibble, stool quality, nutrient digestibility, and intestinal health in dogs were not affected by the yeast β-glucan blend.
Due the humanization of pets, owners are looking for additional ways to improve the health and lifespan of their companions. Oral supplementation of β-glucans may be able to support this desire. β-glucans are major structural components of the cell walls of yeast, fungi, and some bacteria. Cereals, such as barley and oat, also contain β-glucans as part of their endosperm cell wall (Volman et al., 2008). Various functional properties have been reported for β-glucans depending on their source and structure (Brown and Gordon, 2005; El Khoury et al., 2012). Cereal β-glucans are linear chains of glucose molecules with β-1,3 and β-1,4 linkages. These β-glucans have been reported to impact intestinal function by acting as a dietary fiber, altering cholesterol metabolism (El Khoury et al., 2012). In contrast, yeast β-glucans are composed of β-1,3 linkages and β-1,6 linked branches which are known for their ability to modulate the immune system (Novak and Vetvicka, 2008). Therefore, numerous studies have investigated the effects of yeast β-glucans on immune response (Stuyven et al., 2010; Haladova et al., 2011; Vetvicka and Oliveira, 2014; Vojtek et al., 2017) and cereal β-glucans on intestinal function and diet digestibility in dogs (Ferreira et al., 2018). However, there is limited information regarding the impact yeast β-glucans may have on stool quality, diet digestibility, and intestinal health when fed to dogs.
In addition, most of the studies which have evaluated yeast β-glucan in dogs have delivered them via oral supplements (Stuyven et al., 2010; Haladova et al., 2011; Vojtek et al., 2017; Lin et al., 2020). There are a few studies which mention the incorporation of β-glucan within the diet (Ferreira et al., 2018; Vetvicka and Oliveira, 2014), but it is unclear if the β-glucan was processed as part of the diet matrix or added topically during feeding. Previous studies have reported a decrease in β-glucan content following extrusion processing (Huth et al., 2000). Therefore, the first objective of this study was to determine the retention of yeast β-glucan following extrusion processing as well as effects of yeast β-glucan on processing parameters and kibble characteristics. The second objective was to evaluate potential effects of yeast β-glucan consumption within the animal such as stool quality, diet digestibility, and intestinal health markers.
The experimental protocol was reviewed and approved by the Institutional Animal Care and Use Committee (IACUC) at Four Rivers Kennel (Walker, MO) under protocol #FRK-34.
Three different diets with increasing levels of a yeast β-glucan blend (Wellmune for Pet™, Kerry Incorporated, Beloit, WI) were formulated to be iso-nutritional and meet the AAFCO nutritional requirements for adult dogs. The β-glucan evaluated in this study was derived from a strain of baker’s yeast, Saccharomyces cerevisiae. The control diet contained 0% yeast β-glucan blend (0X) while the 0.5X and 1X treatments contained either 0.012% or 0.023% yeast β-glucan blend, respectively. The target dose (1X) was intended to evaluate about one-third of the predetermined dosage for adult humans (250 mg/day) based on previous studies (Feldman et al., 2009; Talbott and Talbott, 2009; Talbott and Talbott, 2010; Fuller et al., 2012). This inclusion level was then decreased (0.5X) in order to evaluate if half of the target dose would elicit a response in dogs. The doses for this study were determined based on Meng (2016) who evaluated similar dosages in children, which resulted in reduced illness. Therefore, formulated inclusion levels were intended to result in a daily dosage of 1.4 mg/BWkg and 2.8 mg/BWkg yeast β-glucan blend for the 0.5X and 1X treatment, respectively.
Titanium dioxide (0.40%) was added to serve as an indigestible marker to estimate apparent total tract nutrient digestibility. The dry raw materials, except for the yeast β-glucan blend, comprised the base ration which was supplied by a commercial mill (Fairview Mills, Seneca, KS; Table 1). For the 0.5X and 1X treatments, yeast β-glucan was mixed into the base ration in a series of dilutions before incorporation into a 123 kg batch in order to achieve a uniform distribution.
Each diet was produced using a single screw extruder (model E525, ExtruTech, Inc., Sabetha, KS, USA). The pre-conditioner (model ADP 145, ExtruTech, Inc., Sabetha, KS, USA) was configured with 12 45° back and 57 neutral beaters on each of the two shafts. The extruder profile and barrel temperatures were based on a typical commercial pet food configuration. At the end of the extruder barrel there were two round die inserts with an interior diameter of 9.5 mm. The target in-barrel moisture was approximately 25%. Fixed input parameters were kept constant during the processing of all treatments, including pre-conditioner (PC) cylinder speed (185 rpm), PC water (66.5 kg/hr), PC steam (56 kg/hr), extruder (EX) water (0 kg/hr), EX steam (0 kg/hr), EX screw speed (300 rpm), and EX knife speed (850 rpm; Table 2).
Table 2 Processing inputs for the pre-conditioner and extruder used to produce canine diets with increasing levels of yeast β-glucan blend are reported as average ± standard deviation.
During processing, PC and EX parameters were collected from sensor readouts every 10 minutes to evaluate potential effects of β-glucan inclusion on the process. Output variables included PC discharge temperature, EX motor load, and EX die temperature and pressure. In addition, a digital caliper was used to measure kibble diameter and length from five randomly selected pieces every 15 min for each diet production off the extruder and off the dryer. Wet and dry bulk density was also measured off the extruder and off the dryer every 15 mins during the processing of each treatment. Bulk density was measured using a 1 L cup in which kibble was leveled and weighed on a digital scale with 0.1 g sensitivity.
After extrusion, kibble was pneumatically conveyed through a 20 cm clean air hood system and deposited onto an oscillating belt spreader. The kibble was dried on a 1.5 m wide single pass two zone dryer (model AFI, ExtruTech, Sabetha, KS, USA) to achieve a less than 10% moisture content. Kibble was dried at approximately 110°C for 24 minutes. Dried kibble was coated with chicken fat protected with natural antioxidants and a dry powdered flavor designed for dogs using a ribbon mixer.
β-glucan concentration in the 0.5X and 1X treatments was analyzed to determine percent recovery after extrusion processing using the Glucan Enzymatic Method (GEM™). Briefly, the diet samples were first gelatinized in aqueous potassium hydroxide (KOH.) The gelatinized samples were then diluted with buffer and treated with lyticase and pustulanase endoglucanases that partially break down and solubilize β-glucan. Exo-1,3-β-glucanase and β-glucosidase (Megazyme International, Wicklow, Ireland) were then added to completely digest the solubilized β-glucan fragments to glucose. The glucose was then quantified using the glucose oxidase/peroxidase (GOPOD) system.
For this study, 24 healthy adult (5.1 ± 2.9 years) Labrador Retrievers (12 intact males, 12 intact females) were enrolled. The dogs had an average body weight of 29.1 ± 3.6 kg. Daily food amounts to maintain body weight were determined by kennel staff (Four Rivers, Nevada, MO). Body weight of each dog was measured prior to start of the study and weekly throughout the trial. The digestibility trial included a 24-day diet adaption phase followed by a 4-day total fecal collection. Dogs were blocked by sex and age prior to random allocation to diets.
The dogs were individually housed overnight and during feeding in temperature-controlled kennels. Depending on weather, dogs were housed in outside yards for 6-8 hours per day during the adaptation period. All dogs were fed their assigned diet once daily in the morning and had free access to automated waterers both inside and outside. Any feed refusals were weighed and recorded daily for accurate calculation of feed intake. During the 4-day collection period, dogs remained kenneled to allow for total fecal collection. The fecal samples were weighed and scored on a 1-5 scale with 0.5 increments [1-liquid diarrhea to 5-dry hard pellets (Carciofi et al., 2008)]. A score of 3.5-4.0 was considered ideal. Feces were stored in a labeled whirl-pak bag in a freezer until further processed. In addition, the pH of a fresh sample (within 15 minutes of defecation) was recorded in triplicate with a calibrated glass-electrode pH probe (FC240B, Hanna Instruments, Smithfield, RI), and 2-g aliquots were transferred into three plastic microcentrifuge tubes using a spatula and stored at -80°C for short chain fatty acid (SCFA) and branched chain fatty acid (BCFA) analysis.
After the collection period, feces from each dog were composited and dried at 55°C in a forced air oven until constant weight was achieved (24-48 h). Dried samples were ground to pass through a 1 mm screen in a laboratory fixed blade impact mill (ZM 200, Retsch, Verder Scientific, Haan, Germany). Titanium dioxide (TiO2) concentration was measured in food and feces using a spectrophotometer plate reader (Gen5TM, Biotek® Instruments, Inc.Winooski, VT) at 410 nm (Myers et al., 2004). Apparent total tract digestibility (ATTD) was estimated by total fecal collection (TFC) and titanium (TI) using the following equations:
Food and partially-dried fecal samples were analyzed in duplicate for moisture (AOAC 930.15), ash (AOAC 942.05), fat by acid hydrolysis (AH) and hexane extraction (AOAC 960.39), gross energy (Parr 6200 Calorimeter, Parr Instrument Company, Moline, IL), and total dietary fiber (AOAC 991.43). Crude protein was determined by Dumas combustion (AOAC 990.03) using a nitrogen analyzer (FP928, LECO Corporation, Saint Joseph, MI).
Fecal SCFA and BCFA concentrations were determined by gas-liquid chromatography (Erwin et al., 1961) using a capillary column (30 m x 0.25 mm internal diameter; 0.25 µm film thickness; Aligent Technologies, Santa Clara, CA). The system was equipped using helium as a carrier gas with a constant flow rate of 40 cm/s and utilizing a 25:1 split ratio injector with injection size of 0.5 µL. A flame ionization detector was configured with hydrogen as the makeup gas with a flow rate of 40 mL/min to clarify peak resolution. The detector and injector temperatures were set at 250°C, and the initial oven temperature was set to 80°C with a ramp rate of 10°C/min to 200°C. The peak area of chromatograms was analyzed using integrative software (GC solution version 2.42.00, Shimadzu, Kyoto, Japan). Concentrations of SCFA (acetate, propionate, and butyrate) and BCFA (isobutyrate, valerate, and isovalerate) were quantified by comparing the sample peak area to a known standard of 10 mM concentration (Volatile Free Acid Mix, Sigma-Aldrich, St. Louis, MO) and correcting for fecal dry matter content.
Data was analyzed using a mixed procedure in SAS (version 9.4, SAS Institute, Inc., Cary, NC). Tukey’s post hoc test was applied for the least-squares means separation, with significance considered at P< 0.05. The feeding trial was conducted as a randomized complete block design in which dogs were blocked by sex and age. Sex and age were considered random effects in the model for analysis of data from the feeding trial. Comparison of TFC and TI methods to calculate ATTD was analyzed using a t-test.
The PC discharge temperature was greatest for the 0.5X treatment at 96.9°C and lowest for the 0X treatment at 92.9°C, with 1X intermediate at 95°C (P < 0.0001; Table 3). The percent motor load remained constant for all dietary treatments at 25%. The 0X treatment had a higher die temperature at 117.4°C compared to the 0.5X and 1X treatments at 112.1°C and 110.9°C, respectively (P = 0.0002). However, the die pressure (300 psi) did not fluctuate during processing of dietary treatments. The wet and dry bulk density was similar among treatments with averages of 369.9 and 340 g/L, respectively (Table 3). Kibble diameter off the extruder was consistent among dietary treatments at an average of 16.8 mm. However, kibble length off the extruder was longest for the 0X treatment at 8.5 mm and shortest for the 0.5X treatment at 7.9 mm, with 1X intermediate at 8.1 mm (P = 0.0164). Kibble diameter and length off the dryer was similar among dietary treatments with averages of 16.5 and 7.2 mm, respectively.
Table 3 Processing outputs and physical characteristics of canine diets with increasing levels of yeast β-glucan blend.
The calculated yeast β-glucan inclusion prior to extrusion was 14.8 g/136 kg for the 0.5X treatment and 28.4 g/136 kg for the 1X treatment (Table 4). The yeast β-glucan concentration analyzed after extrusion using the GEM™ method for the 0.5X and 1X treatments was 16.2 and 35.0 g/136 kg, respectively. This resulted in a 109.5 and 123.2% recovery of the yeast β-glucan in the 0.5X and 1X treatments, respectively.
Table 4 Estimated inclusion of yeast β-glucan from blend in diets before extrusion and analyzed inclusion of β-glucan after extrusion processing.
Moisture content of dietary treatments was <10%. On a dry matter (DM) basis, the dietary treatments were approximately 91% organic matter (OM), 31% crude protein (CP), 12% fat, 9% total dietary fiber (TDF), and 4430 kcal/kg gross energy (GE) (Table 5).
Table 5 Analyzed chemical composition of canine diets with increasing levels of yeast β-glucan blend.
Food intake was provided in amount to maintain body weight and did not differ (P > 0.05) among dietary treatments for an average of 553 g/d (Table 6). Wet fecal output by dogs was similar among dietary treatments (P > 0.05) with an average of 295 g/d (Table 6). Fecal dry matter percent and dry fecal output of dogs were also not different among dietary treatments (P > 0.05) with averages of 30.5% and 89 g/d, respectively. Defecation by dogs fed dietary treatments was similar (P > 0.05) among treatments at an average of 1.8 times per day. Dogs had an average fecal score of 2.6 among dietary treatments. In addition, fecal pH of dogs fed dietary treatments was similar (P > 0.05) at an average of 6.14 (Table 6).
Table 6 Food intake and stool quality parameters of dogs fed diets with increasing levels of yeast β-glucan blend.
Overall, the TFC method resulted in significantly higher digestibility values when compared to the TI method (Table 7). There were no differences (P > 0.05) in nutrient digestibility among dietary treatments with either calculation method (Table 8). For the TI method, dietary treatments resulted in an average of 80.1%, 87.0%, 79.5%, 95.2%, 40.3%, and 86.5% for DM, OM, crude protein, AH fat, TDF, and GE digestibility, respectively (Table 8).
Table 7 Comparison of total fecal collection (TFC) and titanium (TI) methods to calculate apparent total tract digestibility.
Table 8 Apparent total tract digestibility of dogs fed diets with increasing levels of yeast β-glucan blend estimated by total fecal collection and titanium as a dietary marker.
Total SCFA (range 619 – 669 μmol/g DM) and total BCFA (range 35 – 52 μmol/g DM) concentrations in fresh fecal samples were not different (P > 0.05) among dietary treatments (Table 9). In addition, the inclusion of the yeast β-glucan blend did not impact (P > 0.05) the relative proportions of acetate (average; 62.7%), propionate (average; 28.0%), or butyrate (average; 9.3%). The relative proportions of isovalerate, isobutyrate, and valerate (average; 46.6%, 29.8%, and 23.5%, respectively) were also not different (P > 0.05) among treatments (Table 9).
The primary purpose of this study was to evaluate the impact of this specific yeast β-glucan on the immune response in dogs challenged with vaccine (Fries-Craft et al., 2023). The current work was intended to explore the potential side effects from yeast β-glucan on extrusion processing, stool quality, diet digestibility, or intestinal health in dogs. This information should provide valuable insight to complement previous studies evaluating yeast β-glucan that primarily focused on immune outcomes.
In addition, previous studies which have evaluated yeast β-glucan in dogs have done so with greater doses, ranging from 4 mg/BWkg to 25 mg/BWkg, than those used in the current study. This could be seen as a limitation in the current study resulting in the non-significant results. However, this study could help to determine the level at which yeast β-glucan begins to elicit a response in the dog.
The intent of this study was to hold input processing parameters constant in order to determine if the yeast β-glucan blend would affect output processing parameters or physical characteristics of the kibble. Since the PC cylinder speed, PC steam, PC water, and EX screw speed were maintained constant during production of all dietary treatments, the only input difference was the β-glucan addition into the diet matrix. This permits conclusions regarding any differences in output variables among treatments with the β-glucan inclusion and not the adjustment of processing inputs.
As a fiber source, yeast β-glucan may have significant impact on extrusion parameters and final product quality. Fibers are dispersed phase fillers and are known to have poor functionality in extrusion, meaning they result in less expansion of the final product (Guy, 2001). In addition, fibrous ingredients have hydration properties and tend to increase the bulk density of the products. Therefore, the addition of fiber often requires different extruder configurations and processing conditions to achieve the target product (Rokey, 2006). However, in this study, the only difference in processing parameters was the PC discharge temperature and EX die temperature. The increased PC discharge temperature with the inclusion of yeast β-glucan was not considered to be of practical importance due to the maintenance of PC conditions. In addition, the decrease in EX die temperature does not seem to be of practical importance as a decrease in die temperature would correlate to less kibble expansion, which was not observed in the current study. The physiological properties of β-glucan are attributed to its effect on increasing viscosity (Mejia et al., 2020), which may impact processing. However, the maintenance of the EX motor load among dietary treatments indicated that the yeast β-glucan did not affect the viscosity of the diet matrix during processing (De Pilli et al., 2011).
Bulk density is a common and reliable quality control measurement used during the production of dry expanded pet food, which provides a quick and tangible measure of how well the product is cooked or expanded (Rokey, 2006). The dry bulk density of dietary treatments (average; 340 g/L) resembled that of typical commercial kibble which have densities between 280 and 400 g/L (Rokey, 2006). Therefore, with similar bulk density among dietary treatments the yeast β-glucan blend did not affect kibble cook or expansion. In addition, the lack of size change for diameter and length of dry kibble among dietary treatments also indicates that the yeast β-glucan did not affect kibble expansion. The significant decrease in kibble length off the extruder in the 0.5X treatment compared to the 0X treatment was resolved following kibble drying, indicating no practical difference. The physical characteristic consistency among dietary treatments may be due to the low inclusion level of the yeast β-glucan. Future studies should evaluate higher inclusion levels as they may impact results.
Some previous studies have reported a decrease in β-glucan content following extrusion processing. Huth et al. (2000) reported that increasing the feed moisture from 15% to 22.5% at a constant extrusion temperature of 150°C resulted in an 8% reduction of β-glucan. In addition, the increase in extrusion temperature to 170°C with 22.5% feed moisture reduced the β-glucan content by 10% (Huth et al., 2000). These results indicate that multiple processing conditions during extrusion may affect β-glucan retention. However, Fadel et al. (1988) reported a numerical increase in β-glucan following extrusion.
The β-glucan concentration of the diets in the current study seemed to increase after extrusion. However, this may be due to the method used to determine β-glucan concentration in the diet matrix before extrusion. The estimation of β-glucan in the diets before processing was calculated solely on the β-glucan concentration supplied from the experimental product. Therefore, this may have been an underestimation as other ingredients could have contributed to the β-glucan concentration in the diets. For example, rice and wheat are reported to contain low levels of β-glucan (Demirbas, 2005), which were included in dietary treatments. Of note, the previous studies evaluated β-glucan derived from different sources and in different diet matrices than the current study, which may lead to varying results. Nevertheless, these results indicated that this β-glucan can successfully be incorporated into extruded pet food.
The <10% moisture content in dietary treatments was by design as the drying process was controlled during production. The similar nutrient composition among dietary treatments was also expected since the only difference in ingredient composition between diets was the yeast β-glucan blend, which was included at very low levels. If the yeast β-glucan blend was included at higher levels, it may be predicted to increase the dietary fiber content as β-glucans are a known source of soluble fiber (El Khoury et al., 2012). However, in this study there was only a slight increase (< 0.6%) in total dietary fiber with the inclusion of the yeast β-glucan blend.
Similar intake among dietary treatments was expected as food intake was controlled to maintain body weight. In addition, there were no feed refusals among dietary treatments and dogs consumed the diets readily. Rummell et al. (2022) also reported no difference in feed intake for dogs fed a diet supplemented with a concentrated yeast β-glucan compared to a non-supplemented diet. However, the average amount of food required to maintain body weight was about 26% more than anticipated among all treatments based on the equation for normal active dogs [130*BWkg0.75; (NRC, 2006)]. This was likely due to the high activity and stress level of the dogs. Because of this, dogs consumed 1.9 mg/BWkg and 3.8 mg/BWkg per day of the yeast β-glucan blend for the 0.5X and 1X treatments, respectively. This was greater than the targeted doses of 1.4 mg/BWkg and 2.8 mg/BWkg.
It may be expected that the yeast β-glucan would increase moisture content of stool as soluble fiber has increased water holding capacity (McRorie and McKeown, 2017). However, total fecal output, fecal dry matter percent, and fecal score were consistent among dietary treatments. The maintenance of stool quality between the control and yeast β-glucan treatments is consistent with the work of Lin et al. (2020) who reported no difference in fecal DM, fecal score, or fecal pH of dogs orally supplemented with yeast β-glucan compared to non-supplemented dogs. Rummell et al. (2022) also reported consistent fecal scores in dogs fed yeast β-glucan compared to a control. The average fecal score among dogs was lower than ideal at 2.6, indicating loose stools. The loose stools were likely due to the increased stress resulting from confinement during the fecal collection period (Sokolow et al., 2005).
The variation in digestibility among the TFC and TI methods is likely because the TFC method requires collection of all fecal excrement for accurate calculation. However, dogs may step in feces or perform coprophagy making the collection of all fecal material difficult (Alvarenga et al., 2019). In the case of the current study, the loose fecal samples were often stepped in by the dogs resulting in the inability to collect the entire sample. Therefore, the TFC method likely overestimated digestibility due to an underrepresentation of total fecal output. Acuff and Aldrich (2021) also reported numerically higher digestibility values when comparing the TFC to the TI method in dogs. Due to these concerns, the TI method likely resulted in more accurate digestibility estimates in the current study.
The similar digestibility values among treatments indicates that the yeast β-glucan evaluated in this study did not affect nutrient digestibility. This is an important finding since most studies in dogs have only evaluated the effect of yeast β-glucan on the immune system and not potential effects on diet digestibility (Stuyven et al., 2010; Haladova et al., 2011; Vetvicka and Oliveira, 2014; Vojtek et al., 2017). The effect of cereal β-glucans on diet digestibility in dogs has been previously studied (Ferreira et al., 2018); however, the results do not provide accurate comparisons for the current study. That is because the structure of β-glucans derived from cereals differ from those of yeast, resulting in unique functional properties (Brown and Gordon, 2005; El Khoury et al., 2012).
β-glucans are recognized as prebiotics, which are known to promote the growth of beneficial microbiota in the gastrointestinal tract. When consumed, β-glucans escape digestion in the stomach resulting in fermentation by colon microbiota into butyrate, acetate, and propionate (Bobade et al., 2022). Therefore, it was anticipated that the inclusion of β-glucan in the diets would increase production of SCFA. However, the maintenance of SCFA and BCFA concentrations among dietary treatments indicates that the yeast β-glucan did not affect intestinal fermentation. Rummell at al. (2022) also reported no difference in SCFA or BCFA in fecal samples from dogs fed a diet supplemented with 7 mg/BWkg yeast β-glucan compared to a control diet.
A canine in vitro study evaluated the effect of a Saccharomyces cerevisiae-based product containing β-glucan and mannan-oligosaccharides (MOS) on SCFA and BCFA production at increasing inclusion levels (Van den Abbeele et al., 2020). The product contained 27.5% β-glucan resulting in β-glucan inclusion levels of 0.014, 0.028, and 0.055%. These authors reported an increase in acetate and butyrate production with the 0.028 and 0.055% β-glucan supplementation compared to a control. However, there was no difference in acetate or butyrate production among the control and the 0.014% supplementation. Propionate production increased with all levels of supplementation in samples representing the proximal colon while the only significant difference in samples from the distal colon were found with the 0.055% supplementation. In addition, a significant increase in BCFA levels were observed in the distal colon with the 0.028 and 0.055% supplementation. Their results suggest that the inclusion level of β-glucan required to elicit differences in fermentation patterns may be higher than what was included in the current study. In addition, the changes in fermentation patterns from the previous study may be due to the MOS and not the β-glucan. The diet matrix of the current study could also have led to the varying results. Of note, SCFA and BCFA concentrations in the current study were analyzed in fecal samples which may not provide a direct comparison to the previous study. It appears from Van den Abbeele et al. (2020), that increased yeast β-glucan supplementation may be required to see a significant effect further along the digestive tract.
From this study, it can be concluded that this specific yeast β-glucan blend can be successfully incorporated into an extruded pet food without effect on process or on carry through of the active compound. In addition, this study demonstrated that the inclusion of yeast β-glucan at low dosages does not impact stool quality or negatively affect diet digestibility when fed to dogs. However, if the goal of inclusion is to improve intestinal health, greater inclusion levels may be required when fed to dogs. Of note, the mean body weight of children evaluated in the Meng (2016) study was about 15 kg less than the mean body weight of dogs evaluated in the current study, which may have impacted the effectiveness of the dose. For the next study in dogs, it would be recommended to evaluate the predetermined dose for adult humans at 250 mg/day, which would be about 3x greater than the target dose evaluated in this study.
The original contributions presented in the study are included in the article/Supplementary Material. Further inquiries can be directed to the corresponding author.
The animal study was reviewed and approved by the Institutional Animal Care and Use Committee (IACUC) at Four Rivers Kennel (Walker, MO) under protocol #FRK-34.
CA and LK-K formulated the diets and designed the study. LK-K collected samples and performed sample analysis. LK-K performed statistical analysis and primary writing. CA provided editing and interpretation. All authors contributed to the article and approved the submitted version.
We gratefully acknowledge the financial support provided by Kerry Incorporated, Beloit, WI.
The authors declare that the research was conducted in the absence of any commercial or financial relationships that could be construed as a potential conflict of interest.
All claims expressed in this article are solely those of the authors and do not necessarily represent those of their affiliated organizations, or those of the publisher, the editors and the reviewers. Any product that may be evaluated in this article, or claim that may be made by its manufacturer, is not guaranteed or endorsed by the publisher.
Acuff H. L., Aldrich C. G. (2021). Evaluation of graded levels of bacillus coagulans GBI-30, 6086 on apparent nutrient digestibility, stool quality, and intestinal health indicators in healthy adult dogs. J. Anim. Sci. 99, 1–11. doi: 10.1093/jas/skab137
Alvarenga I. C., Aldrich C. G., Ou Z. (2019). Comparison of four digestibility markers to estimate fecal output of dogs. J. Anim. Sci. 97, 1036–1041. doi: 10.1093/jas/skz020
Bobade H., Gupta A., Sharma S. (2022). “Chapter 20 - beta-glucan,” in Nutraceuticals and health care (Cambridge, MA: Academic Press), 343–358. Available at: doi: 10.1016/B978-0-323-89779-2.00013-2.
Brown G. D., Gordon S. (2005). Immune recognition of fungal beta-glucans. Cell Microbiol. 7, 471–479. doi: 10.1111/j.1462-5822.2005.00505.x
Carciofi A. C., Takakura F. S., de-Oliveira L. D., Teshima E., Jeremias J. T., Brunetto M. A., et al. (2008). Effects of six carbohydrate sources on cat diet digestibility and post-prandial glucose and insulin response. J. Ani. Phys. Ani. Nutr. 92, 326–336. doi: 10.1111/j.1439-0396.2007.00794.x
Demirbas A. (2005). β-glucan and mineral nutrient contents of cereals grown in Turkey. Food Chem. 90, 773–777. doi: 10.1016/j.foodchem.2004.06.003
De Pilli T., Derossi A., Talja R. A., Jouppila K., Severini C. (2011). Study of starch-lipid complexes in model system and real food produced using extrusion-cooking technology. Innov. Food. Sci. Emerg. Technol. 12, 610–616. doi: 10.1016/j.ifset.2011.07.011
El Khoury D., Cuda C., Luhovyy B. L., Anderson G. H. (2012). Beta glucan: health benefits in obesity and metabolic syndrome. J. Nutr. Metab. 2012, 851362. doi: 10.1155/2012/851362
Erwin E. S., Marco G. J., Emery E. M. (1961). Volatile fatty acid analyses of blood and rumen fluid by gas chromatography. J. Dairy Sci. 44, 1768–1771. doi: 10.3168/jds.S0022-0302(61)89956-6
Fadel J. G., Newman C. W., Newman R. K., Graham H. (1988). Effects of extrusion cooking of barley on ileal and fecal digestibilities of dietary components in pigs. Can. J. Ani. Sci. 69, 891–897. doi: 10.4141/cjas88-098
Feldman S., Schwartz H., Kalman D. (2009). Randomized phase II clinical trials of wellmune WGP® for immune support during cold and flu season. J. Appl. Res. Clin. Expt. Ther. 9, 30–42.
Ferreira L. G., Endrighi M., Lisenko K. G., Oliveira M.R.D.de, Damasceno M. R., Claudino J. A., et al. (2018). Oat beta-glucan as a dietary supplement for dogs. PLoS One 13, e0201133. doi: 10.1371/journal.pone.0201133
Fries-Craft K., Kilburn-Kappeler L. R., Aldrich G., Bobeck E. A. (2023). Dietary yeast beta 1,3/1,6 glucan supplemented to adult Labrador retrievers alters peripheral blood immune cell responses to vaccination challenge without affecting protective immunity. J. Ani. Sci. Submitted.
Fuller R., Butt H., Noakes P. S. (2012). Influence of yeast-derived 1,3/1,6 glucopolysaccharide on circulating cytokines and chemokines with respect to upper respiratory tract infections. Nutr. 28, 665–669. doi: 10.1016/j.nut.2011.11.012
Haladová E., Mojžišová J., Smrčo P., Ondrejková A., Vojtek B., Prokeš M., et al. (2011). Immunomodulatory effect of glucan on specific and nonspecific immunity after vaccination in puppies. Acta Vet. Hung. 59, 77–86. doi: 10.1556/AVet.59.2011.1.7
Huth M., Dongowski G., Gebhardt E., Flamme W. (2000). Functional properties of dietary fibre enriched extrudates from barley. J. Cereal Sci. 32, 115–128. doi: 10.1006/jcrs.2000.0330
Lin C. Y., Carroll M. Q., Miller M. J., Rabot R., Swanson K. S. (2020). Supplementation of yeast cell wall fraction tends to improve intestinal health in adult dogs undergoing an abrupt diet transition. Front. Vet. Sci. 7. doi: 10.3389/fvets.2020.597939
McRorie J. W., McKeown N. M. (2017). Understanding the physics of functional fibers in the gastrointestinal tract: an evidence-based approach to resolving enduring misconceptions about insoluble and soluble fiber. J. Acad. Nutr. Dietetics. 117, 251–264. doi: 10.1016/j.jand.2016.09.021
Mejía S. M. V., Francisco A., Bohrer B. M. (2020). A comprehensive review on cereal b-glucan: extraction, characterization, causes of degradation, and food application. Crit. Rev. Food Sci. Nutr. 60, 3693–3704. doi: 10.1080/10408398.2019.1706444
Meng F. (2016). Baker’s yeast beta-glucan decreases episodes of common childhood illness in 1 to 4 year old children during cold season in China. J. Nutr. Food. Sci. 6, 518. doi: 10.4172/2155-9600.1000519
Myers W. D., Ludden P. A., Nayigihugu V., Hess B. W. (2004). Technical note: A procedure for the preparation and quantitative analysis of samples for titanium dioxide. J. Anim. Sci. 82, 179–183. doi: 10.2527/2004.821179x
Novak M., Vetvicka V. (2008). Beta-glucans, history, and the present: immunomodulatory aspects and mechanisms of action. J. Immunotoxicol. 5, 47–57. doi: 10.1080/15476910802019045
NRC (2006). Nutrient requirements of dogs and cats (Washington, D.C.: The National Academies Press). doi: 10.17226/10668
Rokey G. J. (2006). Pet food production: process description (Pet Food Production). Online article in Animal Feed of Engormix
Rummell R. M., Steele M. A., Templeman J. R., Yohe T. T., Akhtar N., Lambie J. G., et al. (2022). A proof of principle study investigating the effects of supplemental concentrated brewer’s yeast on markers of gut permeability, inflammation, and fecal metabolites in healthy non-challenged adult sled dogs. J. Ani. Sci. 100, 1–11. doi: 10.1093/jas/skac281
Sokolow S. H., Rand C., Marks S., Drazenovich N. L., Kather E. J., Foley J. (2005). Epidemiologic evaluation of diarrhea in dogs in an animal shelter. Amer. J. Vet. Res. 66, 1018–1024. doi: 10.2460/ajvr.2005.66.1018
Stuyven E., Verdonck F., Hoek I.V., Daminet S., Duchateau L., Remon J. P., et al. (2010). Oral administration of beta-1,3/1,6-glucan to dogs temporally changes total and antigen-specific IgA and IgM. Clin. Vaccine Immunol. 17, 281–285. doi: 10.1128/CVI.00344-09
Talbott S., Talbott J. (2009). Effect of beta 1, 3/1, 6 glucan on upper respiratory tract infection symptoms and mood state in marathon athletes. J. Sports Sci. Med. 8, 509–515.
Talbott S., Talbott J. (2010). Beta 1,3/1,6 glucan decreases upper respiratory tract infection symptoms and improves psychological well-being in moderate to highly-stressed subjects. Agro. Food Industry Hi-Tech. 21, 21–24.
Van den Abbeele P., Duysburgh C., Rakebrandt M., Marzorati M. (2020). Dried yeast cell walls high in beta-glucan and mannan-oligosaccharides positively affect microbial composition and activity in the canine gastrointestinal tract in vitro. J. Anim. Sci. 98, 1–10. doi: 10.1093/JAS/SKAA173
Vetvicka V., Oliveira C. (2014). β(1-3)(1-6)-D-glucans modulate immune status and blood glucose levels in dogs. Br. J. Pharm. Res. 4, 981–991. doi: 10.9734/BJPR/2014/7862.
Vojtek B., Mojžišová J., Smrčo P., Drážovská M. (2017). Effects of orally administered β-1,3/1,6-glucan on vaccination responses and immunological parameters in dogs. Food Ag. Immun. 28, 993–1002. doi: 10.1080/09540105.2017.1324407
Keywords: canine, digestibility, extrusion, stool quality, yeast β-glucan
Citation: Kilburn-Kappeler LR and Aldrich CG (2023) Evaluation of a yeast β-glucan blend in a pet food application to determine its impact on stool quality, apparent nutrient digestibility, and intestinal health when fed to dogs. Front. Anim. Sci. 4:1125061. doi: 10.3389/fanim.2023.1125061
Received: 15 December 2022; Accepted: 03 January 2023;
Published: 16 January 2023.
Edited by:
Luciano Pinotti, University of Milan, ItalyReviewed by:
Monica Isabella Cutrignelli, University of Naples Federico, ItalyCopyright © 2023 Kilburn-Kappeler and Aldrich. This is an open-access article distributed under the terms of the Creative Commons Attribution License (CC BY). The use, distribution or reproduction in other forums is permitted, provided the original author(s) and the copyright owner(s) are credited and that the original publication in this journal is cited, in accordance with accepted academic practice. No use, distribution or reproduction is permitted which does not comply with these terms.
*Correspondence: Charles G. Aldrich, YWxkcmljaDRAa3N1LmVkdQ==
Disclaimer: All claims expressed in this article are solely those of the authors and do not necessarily represent those of their affiliated organizations, or those of the publisher, the editors and the reviewers. Any product that may be evaluated in this article or claim that may be made by its manufacturer is not guaranteed or endorsed by the publisher.
Research integrity at Frontiers
Learn more about the work of our research integrity team to safeguard the quality of each article we publish.