- 1Department of Anatomy and Embryology, Faculty of Veterinary Medicine, Zagazig University, Zagazig, Egypt
- 2Department of Physiology, Faculty of Veterinary Medicine, Zagazig University, Zagazig, Egypt
- 3Department of Histology and Cytology, Faculty of Veterinary Medicine, Zagazig University, Zagazig, Egypt
- 4Department of Surgery, Radiology, and Anesthesiology, Faculty of Veterinary Medicine, Zagazig University, Zagazig, Egypt
- 5Department of Biology, College of Science, King Khalid University, Abha, Saudi Arabia
- 6Department of Theriogenology, Faculty of Veterinary Medicine, South Valley University, Qena, Egypt
- 7Anatomy and Histology Department, Faculty of Medicine, Sana’a University, Sana’a, Yemen
- 8Department of Environmental and Health Research, Umm Al-Qura University, Makkah Al-Mukaramah, Saudi Arabia
Compared to other mammals, Arabian camels are ideal models for exploring the structural adaptations that enable camels to survive in arid environments. Thus, this study aimed to explore how evolutionary adaptation to arid conditions modifies the characteristics of the kidneys in Arabian camels (Camelus dromedarius) compared to oxen. Urine samples were physically and chemically analyzed. Harvested kidneys were subjected to topographical and fast spin echo magnetic resonance (FSE-MR) imaging. Histology, histomorphometry, and Aquaporin-2 (AQP2) expression by immunohistochemistry were also performed. Here, in dromedaries, sodium and potassium values in the urine were much higher (p=0.001, for both), whereas chloride was much lower (p=0.004) than the values of oxen. Compared with oxen, the level of the hormone aldosterone in serum was significantly lower (p=0.002), whereas creatinine and urea were significantly higher (p=0.005 and p=0.001, respectively). Uric acid in dromedaries and oxen did not differ significantly (p=0.349). Like sodium levels (p=0.001) in dromedary serum, chloride was also much higher (p=0.002) than in oxen. The average value of potassium was much lower (p=0.009) than that of oxen. Morphologically, anatomical and FSE MRI studies revealed that minor and major calyces were not found in dromedary kidneys. The renal pelvis was not found in oxen, and the major calyx was directly connected to the ureter. The dromedary kidney contained a wider medullary portion as well as increased diameters for renal corpuscles (RCs), proximal convoluted tubules (PCTs), and collecting tubules (CTs, p<0.05) compared with the oxen. We also noted that AQP2 was significantly expressed in dromedary nephron components, except for RCs, compared with oxen as shown by immunohistochemistry. Overall, these data strongly suggest that the dromedary has a greater ability to adapt to harsh desert conditions in terms of producing highly concentrated urine than oxen.
1 Introduction
Camels (Latin: Camelus) are classified based on the presence of humps into single-humped (Arabian camel; dromedary), two-humped (Bactrian), and non-humped (Lamini) camels (Wu et al., 2014). Arabian dromedaries and Bactrian camels are domesticated species and live in the harsh biome of African and Asian deserts, where they can behaviorally and physiologically adapt to the arid desert conditions by tolerating temperatures over 40° Celsius (104 Fahrenheit) and water loss of more than 25% of total body weight (Hoter et al., 2019). In addition, they can tolerate water deficiency for an extended period (Ouajd and Kamel, 2009). Lamini contain two wild (guanaco and vicuna) and two other domesticated species (llama and alpaca) and live on the high hikes of South America. In contrast to humped species, Lamini camels have not been granted adaptation features to the hot arid ecosystem of the desert (Wu et al., 2014). In addition to being milk, meat, and fiber producers, Arabian camels comparably to other mammals represent ideal models for exploring ecological, physiological, and behavioral adaptations.
It is known that the aptitude to maintain the body’s physiological fluid capacity (fluid conservation) by reducing water loss and metabolizing adipose tissue reserves is the unique feature of the evolutionary adaptation of the Arabian camel (Abdalla, 2020). This feature is achieved through several physiological mechanisms that solely include producing maximally concentrated urine by anatomically distinguished kidneys with certain characteristics for this purpose (Lin et al., 2022). These characteristics include a long loop of Henle and a well-developed medulla with a relative medulla/cortex ratio of 4:1 (Ouajd and Kamel, 2009). Kidneys produce highly concentrated urine by increasing the osmolarity of urine and tubular re-absorption of water, decreasing the glomerular filtration rate, and recycling urea by the specialized epithelial cells lining the renal medulla (Lin et al., 2022). Additionally, the inner medulla contains a highly arranged vasa recta, a thin descending segment of the Henle loop, a thick ascending segment of the Henle loop, and collecting convoluted tubes where the process to generate highly concentrated urine occurs (Abdalla, 2020). Cattle are domesticated herbivorous mammals belonging family Bovidae and have adaptation capability to dry climates and high temperatures (da Costa et al., 2015).
Aquaporins (AQPs) are a critical family of small-sized proteins found on the cell membrane (~30 kDa) and are responsible for facilitating the passive transport of water and certain ions and solutes across transmembrane in a variety of epithelial and nonepithelial cells (Ala et al., 2021). Moreover, accumulated evidence showed that AQPs play a significant role in the control of numerous inflammatory-related disorders as well as lung physiology, angiogenesis, and wound healing. In animals, thirteen variants of AQPs (AQP0 through AQP13) are differentially expressed by body cells. On renal cells, seven AQPs (AQP1, AQP2, AQP3, AQP4, AQP7, AQP8, and AQP11) have been proven to functionally maintain the water balance in the body by facilitating the water reabsorption from the renal tubules, and therefore generating the concentrated urine (JingBao et al., 2014; Kortenoeven and Fenton, 2014; Wang et al., 2018).
It has been shown that cattle excrete about 20 - 40 liters of fluid per day compared with camels which excrete only 1.3 liters on average per day (Breulmann et al., 2007), despite both families being adapted to exist in the harsh conditions of the desert; it is why we were interested in conducting this study to explore the characteristics of their kidneys through anatomical, radiological, histological, and physiological investigations.
2 Materials and methods
2.1 Animals
Twenty healthy adult castrated male dromedaries with mean ± SD age 2.50 ± 0.22 years and weight 444.15 ± 26.08 kg, and 20 healthy adult oxen (1.90 ± 0.19 years, weight 480.27 ± 12.25 kg) were involved in this study. These animals were slaughtered in public governmental slaughterhouses (El-Sharkia, Egypt). Before slaughter, the dromedaries and oxen were routinely and thoroughly examined by registered veterinarians for any abnormalities. In this study, only apparently healthy animals were included. This study was carried out under the guidance of rules of the Ethical Committee and Animal Welfare of the Faculty of Veterinary Medicine, Zagazig University, Egypt, and with the approval of the Institutional Animal Care and Use Committee (Approval # ZU-IACUC/2/F/164/2022) of Zagazig University.
2.2 Sampling and preparation of sera and urine
Before slaughtering, blood samples (10 ml each) were aseptically harvested via jugular venipuncture in sterile silicone-coated vacutainers and transported in a sterile polyethylene cool box. For all animals, the sera samples were separated from harvested blood samples by centrifugation at 3000 rpm for 10 minutes and were kept at -20°C unless otherwise freshly used. Immediately after the slaughtering, the urinary bladders were carefully harvested, and the containing urine was collected in sterile 50-mL tubes.
2.3 Urine analysis
Urine samples were transferred in sterile containers and directly subjected to physical, microscopical, and chemical using Medi-Test (Combi-10® SGL, Macherey-Nagel, Germany) examination as well as examined for electrolyte values as described previously (Coles, 1986).
2.4 Serum biochemistry analysis
Serum aldosterone was measured using an enzyme-linked immunosorbent assay (ELISA) kit and a microplate reader (LDN kit, Nordhorn, Germany). Calorimetric analysis of serum urea, uric acid, and creatinine was conducted using commercial kits following the manufacturer’s instructions and as described before (Larsen, 1972; Hamada et al., 2008; Dart et al., 2020). All these kits were purchased from BioMed Diagnostic Co., Cairo, Egypt. Easylyte Plus Ions Analyzer (Medica Corporation, The Netherlands) was used to measure the serum electrolytes (sodium, potassium, and chloride).
2.5 Anatomical and morphometric analysis of kidneys
Biometric estimation of the left and right kidneys in both species was performed. The kidneys were carefully dissected and then immersed in a 10% formaldehyde solution for external and internal anatomical description. The relative thickness measurement of the cortex and medulla was made by sliding caliper.
2.6 Fast spin echo magnetic resonance imaging
In all dromedaries and oxen, kidneys were removed immediately after slaughter and were subjected to MR scanning within 4-hours post-harvesting. The MR imaging was made using an internal magnetom of 1.5 - tesla field strength (Philips, Intra, USA) with fast spin echo (FSE) T1 (relaxation time) – weighted sequences, 350 ms repetition time (TR), 0.8 s echo time (ET), and one excitation (E). On MR images, normal renal biometrics were assessed, and the renal structures visualized were identified and labeled.
2.7 Histological examination
Renal specimens were fixed in 10% buffered neutral formalin and then dehydrated and cleared in xylene. All specimens were infiltrated with soft melted paraffin and embedded in hard paraffin. Paraffin sections were exposed to Harris’s Hematoxylin and Eosin staining for the general histological renal structures (Suvarna et al., 2019).
2.8 Histomorphometry
Six dromedaries and oxen were involved in the morphometrical analysis. Per each animal, six representative fields were used and quantitatively analyzed using ImageJ software (Fiji ImageJ; 1.51 n, NIH). Diameters of the RCs, PCTs, DCTs, the thick segment of the loop of Henle, the thin segment of the loop of Henle, and collecting tubules were estimated on hematoxylin and eosin-stained photomicrographs at 400x magnification of kidney of oxen and dromedaries.
2.9 Immunohistochemistry
Renal sections were mounted, deparaffinized by xylene, rehydrated, and then washed in phosphate buffer saline (PBS). The sections were immersed in 0.3% hydrogen peroxide in water. The sections were then blocked by incubation in 10% normal rabbit serum for 1 hour. The sections were then incubated with anti-rabbit aquaporin-2 polyclonal antibody (NB110-74682; dilution 1:200, Novus Biologicals, CO, USA) overnight at 4°C. The next day, the labeled sections were then incubated with horseradish peroxidase (HRP)-conjugated anti-rabbit secondary antibody for 2 hours at room temperature. After washing, Diaminobenzidine (DAB) was used as chromogen in which labeled sections were incubated for 2-4 minutes at room temperature. Afterward, sections were washed, then counterstained with Mayer’s hematoxylin, then mounted with Canada Balsam (Suvarna et al., 2019). All the stained sections were then examined with a standard light microscope (Olympus BX 21) and imaged by a high-resolution camera (canon®) at the Department of Histology and Cytology, Zagazig University.
2.10 Statistical analysis
Independent student’s t-test was used for statistical analysis and to compare between kidneys of oxen and dromedaries for the measured histological parameters (diameter of RCs, diameter of PCT, diameter of DCT, diameter of thick segment of loop of Henle, diameter of thin segment of loop of Henle and diameter of collecting tubules), anatomical and MRI biometrics. Data were expressed as mean ± SE. Statistical analysis was performed by GraphPad prism 8.0.2 (GraphPad Software, Inc, USA). A value of p<0.05 was considered statistically significant.
3 Results
3.1 Urine findings
Data retrieved from urine analysis in both dromedaries and oxen are listed in Table 1. As observed, dromedary urine was pale yellow, whereas oxen urine was light yellow. In both species, urine appeared clear with a uriniferous odor and had an alkaline pH. There were no proteins, sugars, ascorbic acid, bile salts, bile pigments, crystals, and epithelial cells detected in urine samples of dromedaries and oxen. There were only traces of urobilinogen detected in both species. There was no significant variation in the number of pus cells and red blood cells (RBCs) (p=0.29 and p=0.42, respectively) in the urine of dromedaries compared with those in the oxen. In dromedaries, the mean values of sodium and potassium were significantly higher (p<0.01, for both), whereas urine chloride was significantly lower (p<0.01) than those of oxen (Figure 1).
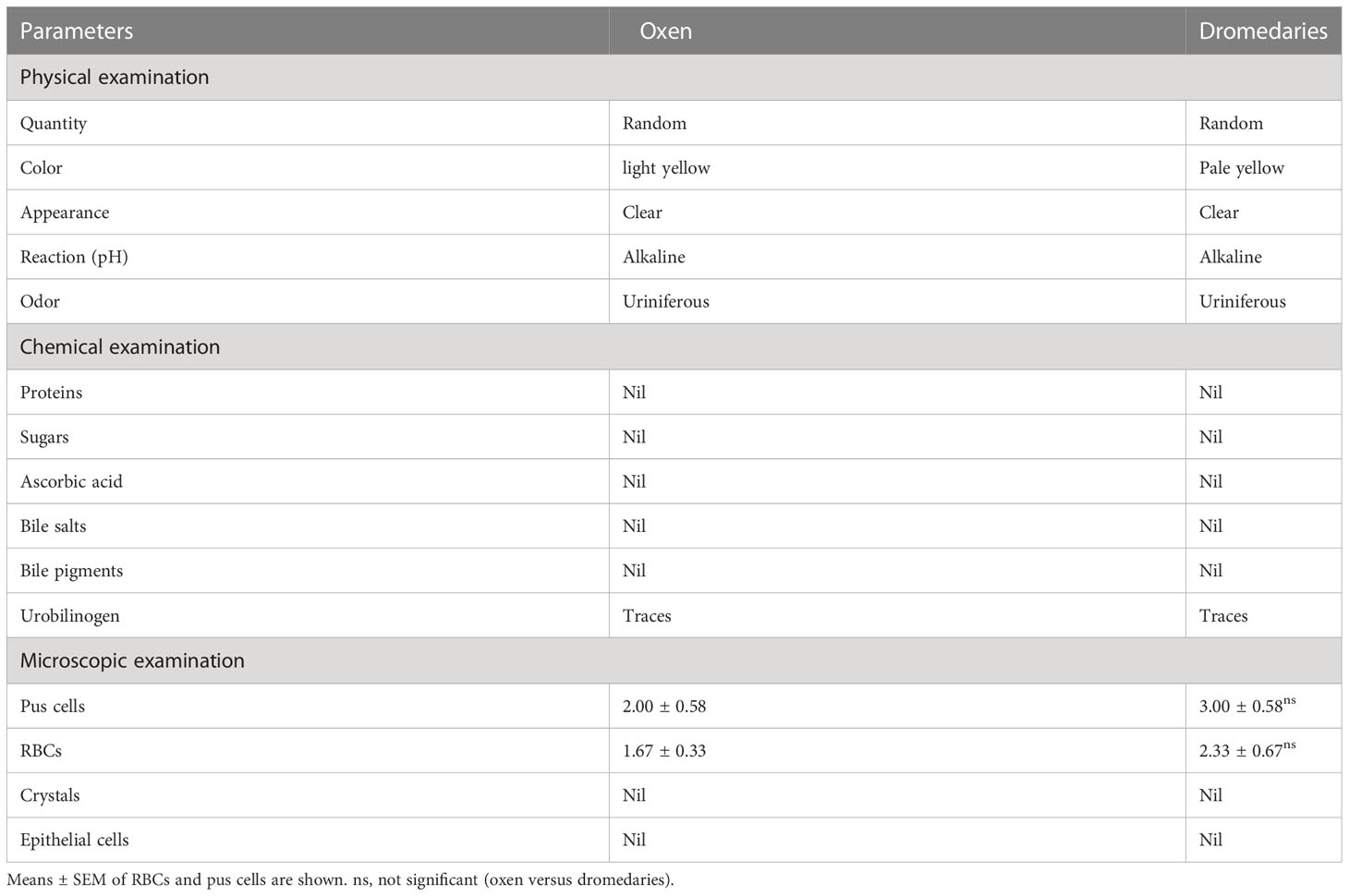
Table 1 Physical, chemical, and microscopical characteristics of the urine in oxen and dromedaries (n=20 each).
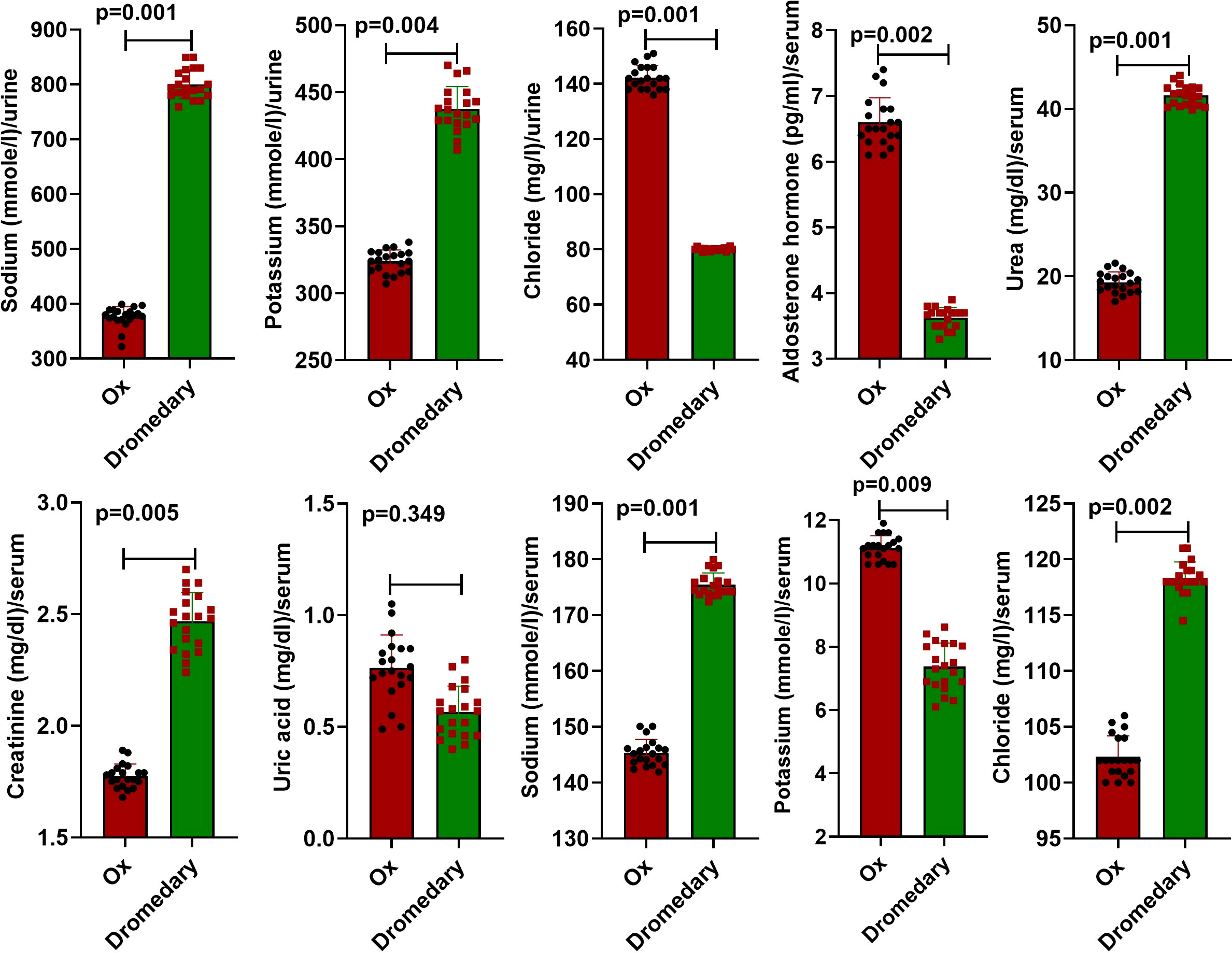
Figure 1 Biochemical characteristics of the urine and serum in oxen and dromedaries (n = 20 each). Data are shown as Means ± SD.
3.2 Serum findings
Compared to oxen, the serum level of aldosterone hormone was significantly lower (p<0.01), while serum levels of creatinine and urea were significantly higher (p<0.01, for both). Uric acid values in both dromedaries and oxen were not differed significantly (p>0.05). In addition to serum sodium level, the mean value of serum chloride in dromedaries was greatly higher (p<0.01) compared to oxen. The mean value of serum potassium was significantly lower (p<0.01) than that in oxen (Figure 1).
3.3 Anatomical and morphometric findings
In dromedaries and oxen, kidneys were found dorsally in the sub-lumbar region and surrounded by adipose tissue (perirenal fat). Biometrical characteristics of the left and right kidneys in oxen and camels are listed in Table 2.
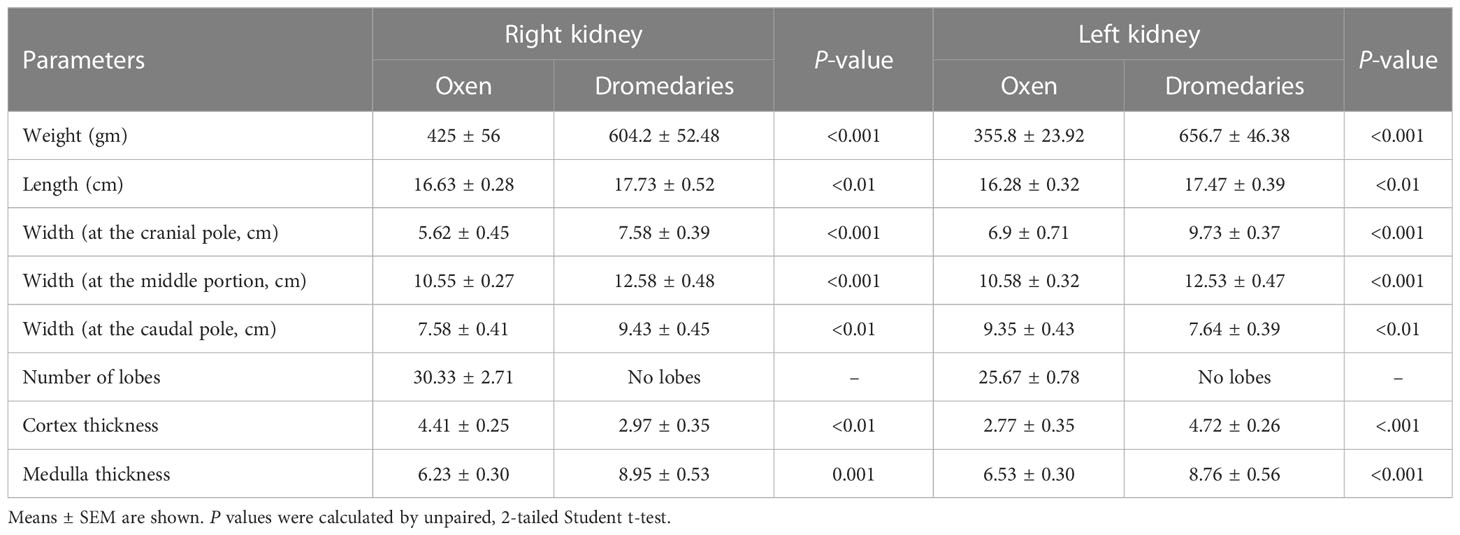
Table 2 Biometrical characteristics of the left and right kidneys in the oxen (n=20) and dromedaries (n=20).
3.3.1 Kidneys of the oxen
Kidneys appeared light brown and were divided into lobes by interlobar fissures. These fissures were filled with adipose tissue and only separated the renal cortex as deep as the renal pyramids (Figure 2). The right and left kidneys appeared differently in shape, weight, and dimensions. The right kidney shape was oval to bean and contained 30.33 ± 2.71 lobes. It had two (dorsal and ventral) surfaces, two (lateral and medial) borders, and two (cranial and caudal) poles. The left kidney was pyramidal in shape and had 25.67 ± 0.78 lobes. It had three (dorsal, right, and left ventral) surfaces, two (lateral and medial) boundaries, and two (cranial and caudal) poles. The renal hilus appeared as a concave depression at the mid-region of the ventral surface and extended to the proximal third of the medial border (Figures 2, 3). In a longitudinal section, three zones, the capsule, cortex, and medulla, appeared differently in color and texture. There were two major calyces and a considerable number of minor calyces. There was no renal pelvis, and the major calyces were connected directly to the ureter (Figure 4A). The fibrous capsule enveloping the kidney was a transparent, strong layer, and easily peeled. The cortex appeared as a brownish layer with a coarse texture and radiant appearance. The cortex was extended between renal pyramids to form the renal columns. The medulla consisted of renal pyramids with prominent renal papillae endings. The base of the renal pyramids was found close to the cortical layer and the apices were toward the hilus (Figure 4A). It was noted that the renal papilla opened in approximately 18 - 22 funnel-shaped channels (minor calyces). The minor calyces were surrounded by adipose tissue in the renal sinus and opened into the major calyces that opened directly into the ureter at the hilus (Figure 4A).
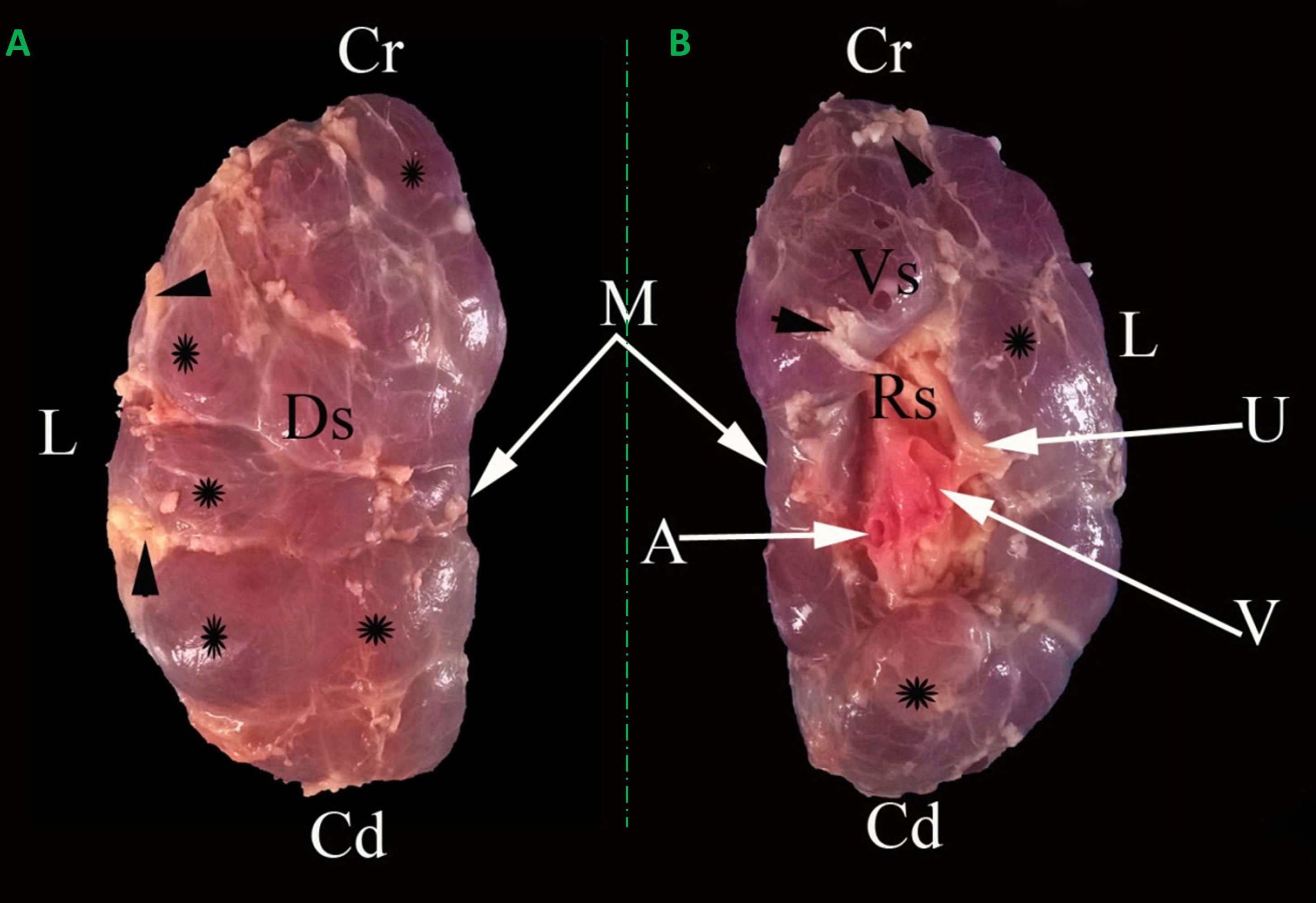
Figure 2 Representative dorsal (A) and ventral (B) macroscopical views of the encapsulated right kidney of oxen. Cr: Cranial pole; Cd: caudal pole; Ds: dorsal surface; Vs: ventral surface; M: medial border; L: lateral border; A: renal artery; V: renal vein; U: ureter; Rs: renal sinus; renal lobules (*); interlobar fissures filled with adipose tissue (arrowheads).
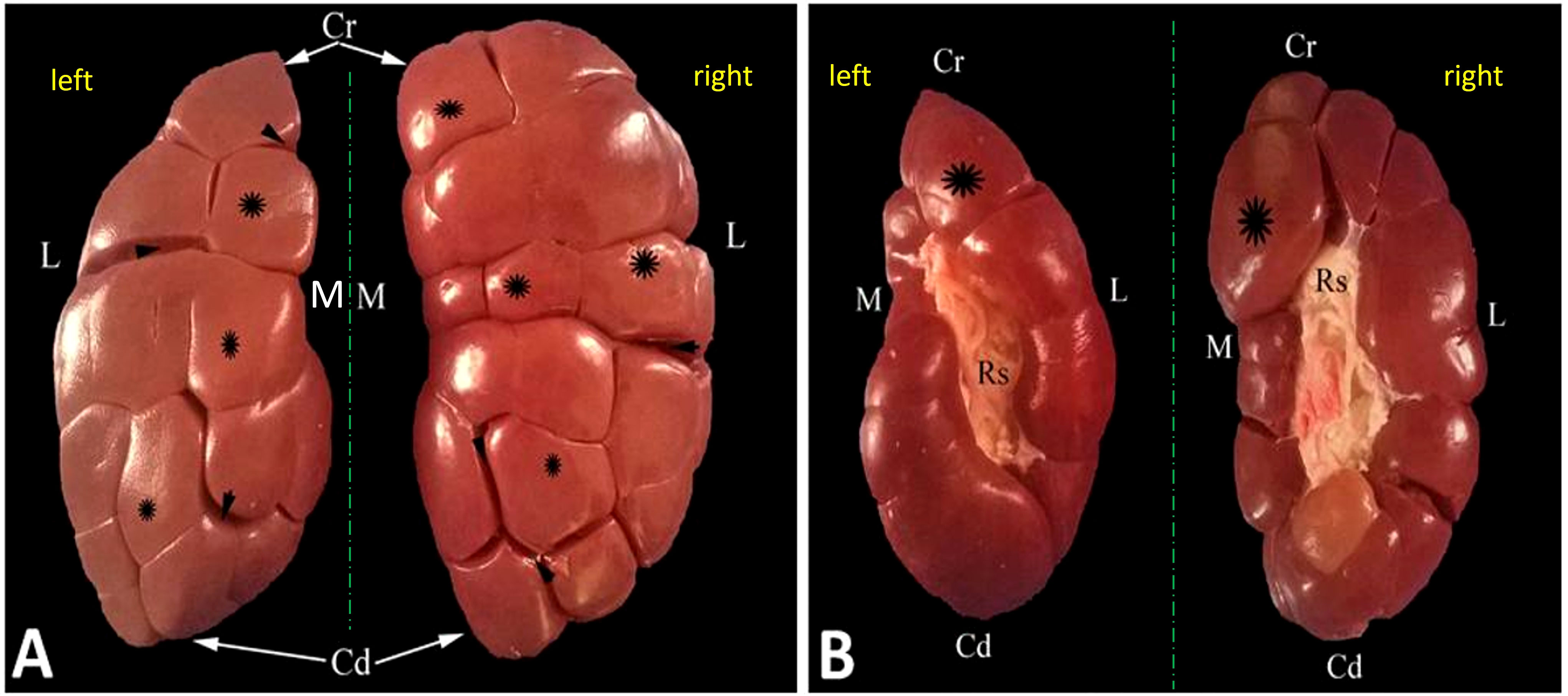
Figure 3 Representative dorsal (A) and ventral (B) macroscopical views of the decapsulated right and left kidneys of oxen. Cr, cranial pole; Cd, caudal pole; M, medial border; L, lateral border; Rs, renal sinus filled with adipose tissue; renal lobules (asterisk); interlobar fissures (arrowheads).
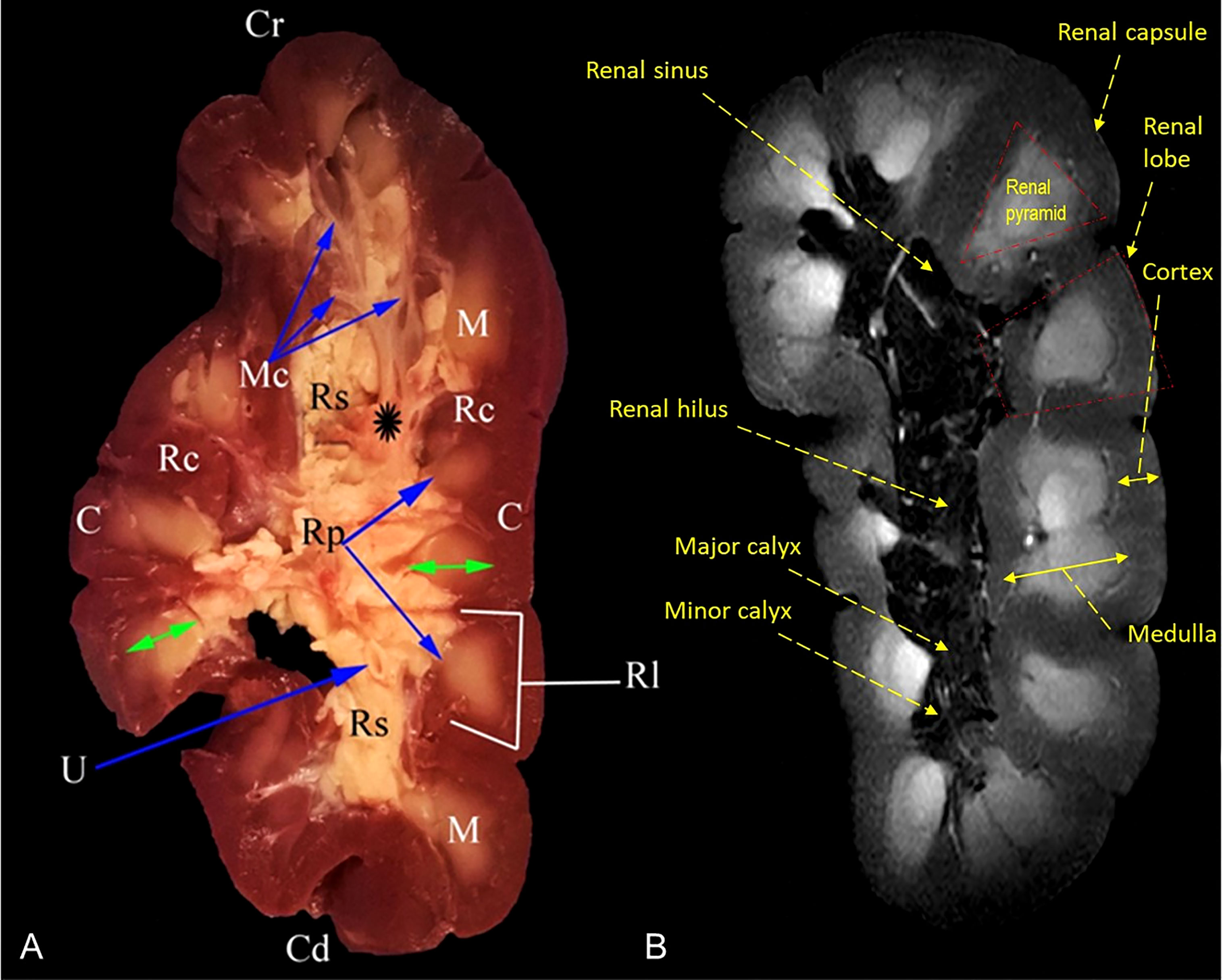
Figure 4 Representative mid-longitudinally sectioned gross image (A) and corresponding MR image (B) of the left kidney of oxen. Cr, cranial pole; Cd, caudal pole; RI, renal lobule; C, cortex; Rc, renal column; M, medulla; renal pyramid (double arrowhead); Rp, renal papillae; Rs, renal sinus; Mc: minor calyx; major calyx (asterisk symbol); U: ureter within fat tissue into the renal sinus.
3.3.2 Kidneys of the dromedary
Kidneys appeared firm, reddish brown, with a smooth surface, and differed in shape, weight, and dimensions. The right kidney appeared bean-shaped, whereas the left one was human ear-shaped. Both kidneys had dorsal and ventral surfaces, lateral and medial borders, and cranial and caudal ends. The hilus appeared as a concave depression at the middle third of the medial boundary (Figure 5). In a longitudinal section, three zones (capsule, cortex, and medulla) appeared unevenly in color and texture. The cortex-medulla borderline was clear and serrated. The average medullary thickness was 4 times more than the cortex thickness. The medulla consisted of renal pyramids from which a renal crest and the renal pelvis were connected. A pronounced secondary renal pyramid was formed on the side-bottom of the adjacent renal pyramid. The pyramids’ bases were towards the cortex while the apices were towards the medulla interna. The renal pelvis was crescentic-shaped, and its long axis appeared to follow the renal long axis. The smooth medial wall of the main renal cavity was adjacent to the adipose connective tissue of the renal sinus where the ureter emerged from the center of the medial wall of the main cavity of the renal pelvis (Figure 6A). About 12 prominent mucous folds were found dorsoventrally to the renal pelvis and the medulla externa between the renal pyramids. In the renal pelvis, the correspondent dorsoventrum protruded into the medullary boundaries (M. interna and M. externa) where the medulla intra and externa connected to form the fornix (Figure 6A).
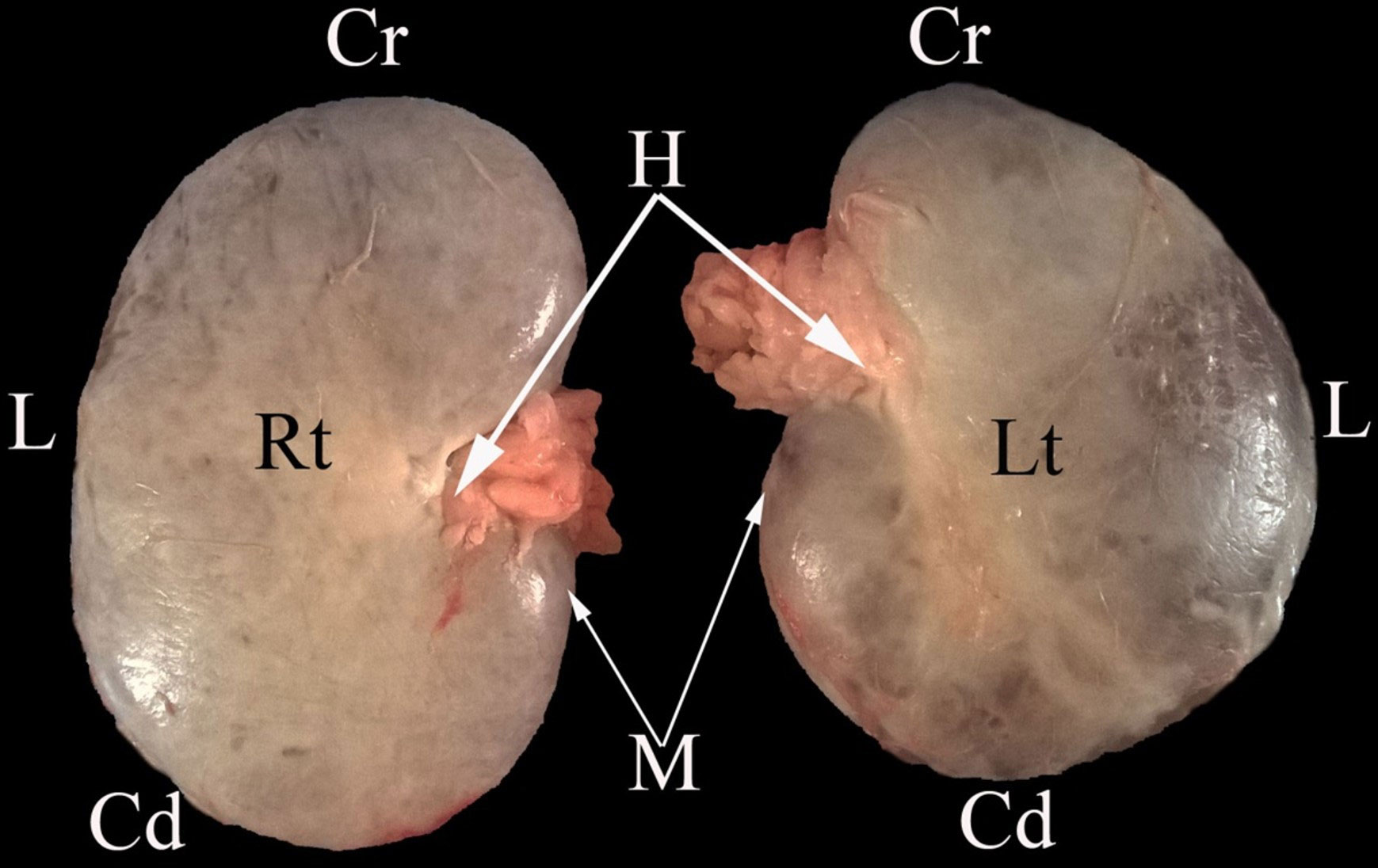
Figure 5 A representative ventral macroscopical view of the encapsulated kidney of dromedaries. Rt, right kidney; Lt, left kidney; Cr, cranial pole; Cd, caudal pole; M, medial border; L, lateral border; H, renal hilus.
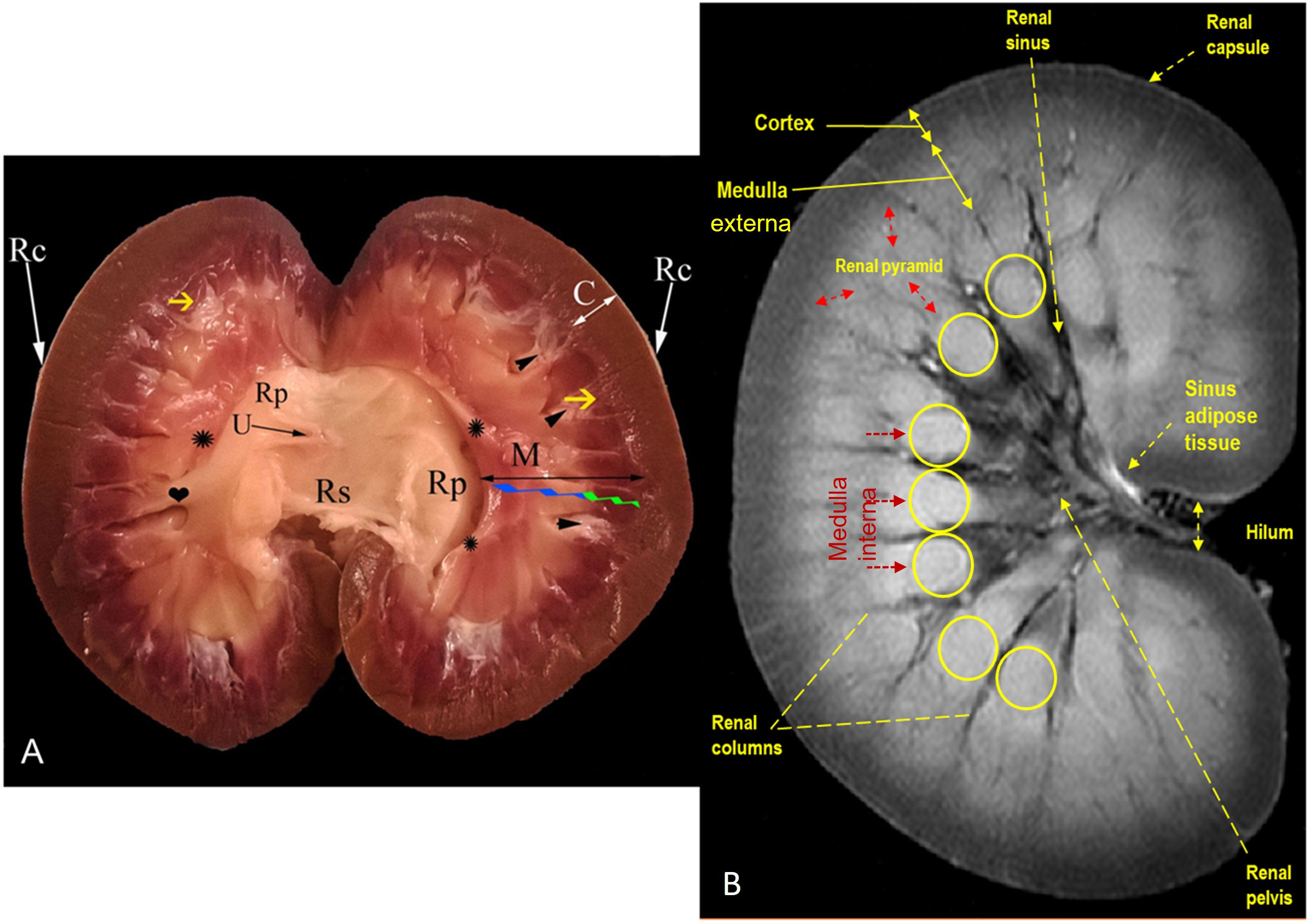
Figure 6 Representative mid-longitudinally sectioned gross image (A) and corresponding MR image (B) of the left kidney of dromedaries. Rc, renal capsule; C: cortex; M: medulla; medulla externa (green zigzag line); medulla interna (blue zigzag line); renal pyramid (black double arrowhead); renal crest formed by converging of renal pyramids (asterisk symbols); Rp: renal pelvis; collateral recesses (heart symbol); secondary pyramid (yellow arrow); fornix (arrowhead); U: ureter within fat tissue into the renal sinus; Rs: renal sinus containing fat and ureter
3.4 Fast spin echo MRI findings
MRI provided good discrimination between the renal adjacent soft tissues according to their physical density difference. Herein, we found that on MRI, the renal cortex appeared gray (low signal intensity, hypointense), while fat and renal pyramids appeared bright (high signal, hyperintense). The description of the renal structures viewed on MR images and their corresponding mid-longitudinally sectioned gross images of the left kidney in dromedaries and oxen are presented in Figures 4B, 6B, respectively.
3.5 Histology and histomorphometry
3.5.1 Renal cortex
In oxen, the kidney was enveloped by a thin collagenous capsule. The cortical labyrinth showed different portions of nephrons (RCs, PCTs, and DCTs). The RCs were mainly distributed in the mid-cortex and cortico-medullary junction, but not in the subcapsular region. The RCs appeared round-shaped and consisted of a double-layer bowman’s capsule: parietal layer (lined by a simple squamous epithelium) and visceral layer (lined with podocytes and a cluster of glomerular capillaries). PCTs were lined by cuboidal epithelial cells with rounded nuclei, acidophilic cytoplasm, and well-developed brush borders. While DCTs were shorter in length, infrequently seen, and lined with simple cuboidal epithelium characterized by paler cytoplasm and lower height with no brush borders (Figures 7A, C). While in dromedaries, the renal capsule was thicker than that of the oxen. The RCs, relative to that in oxen, were larger (p<0.05) (Figure 7E), and RCs appeared ovoid-shaped and were found mainly in sub-capsular and mid-cortical zones (Figures 7B, D). PCTs were considerably wider (p<0.01) compared with that in oxen (Figure 7E).
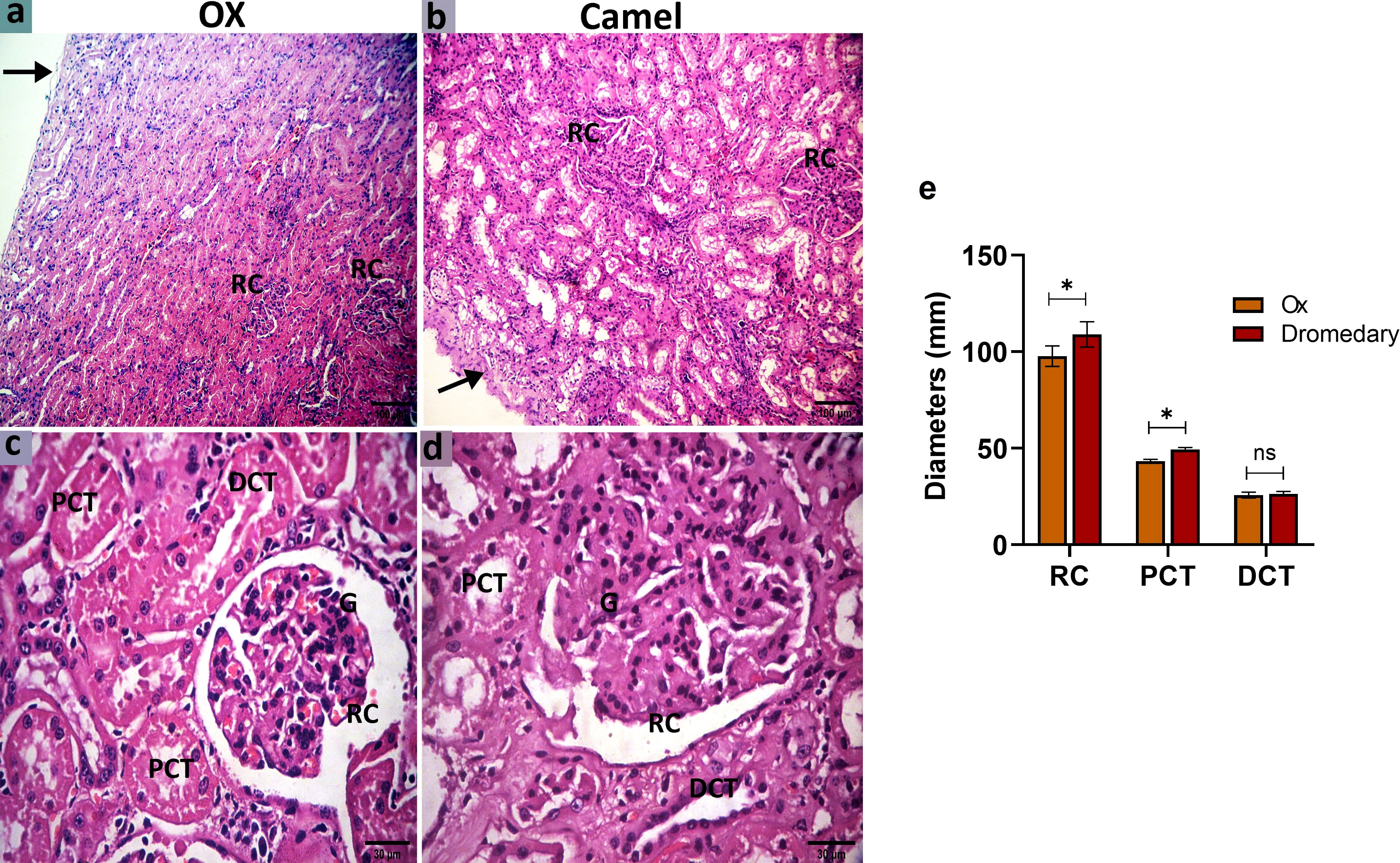
Figure 7 Histologic sections of the oxen and dromedary kidney show that the dromedary kidney contains larger and wider RCs and PCTs. The renal cortex of the oxen and dromedary (a and b, respectively). (A) a thinner capsule (arrow) and smaller renal corpuscles (RCs) in the ox. (B) a thicker capsule (arrow), and larger RCs in the dromedary. scale bar, 100 μm; hematoxylin and eosin stain. The renal cortex of the oxen and dromedary (c and d, respectively). (C, D) RC, glomerulus-G, proximal convoluted tubules-PCT, and distal convoluted tubules-DCT. Scale bar, 30 μm; hematoxylin and eosin stain. (E) Morphometry analysis for diameters of RC, PCT, and DCT. Data shown are represented as mean ± SD.*p<0.05.
3.5.2 Renal medulla
Oxen exhibited numerous collecting tubules and different parts of the loop of Henle. In the loop of Henle, the thin segment was lined by simple squamous epithelial cells with thin cytoplasm, while the thick segment was lined by simple cuboidal epithelial cells with acidophilic thick cytoplasm and rounded nuclei. The collecting tubules were found wider and lined by simple cuboidal epithelial cells with clear cellular boundaries and spherical nuclei (Figures 8A, C). In dromedaries, the collecting tubules were separated by abundant vasa recta (Figures 8B, D). Thin and thick segments of the loop of Henle were to some extent wider, but the collecting tubules were significantly larger in diameter (p<0.05) compared to those in oxen (Figure 8E).
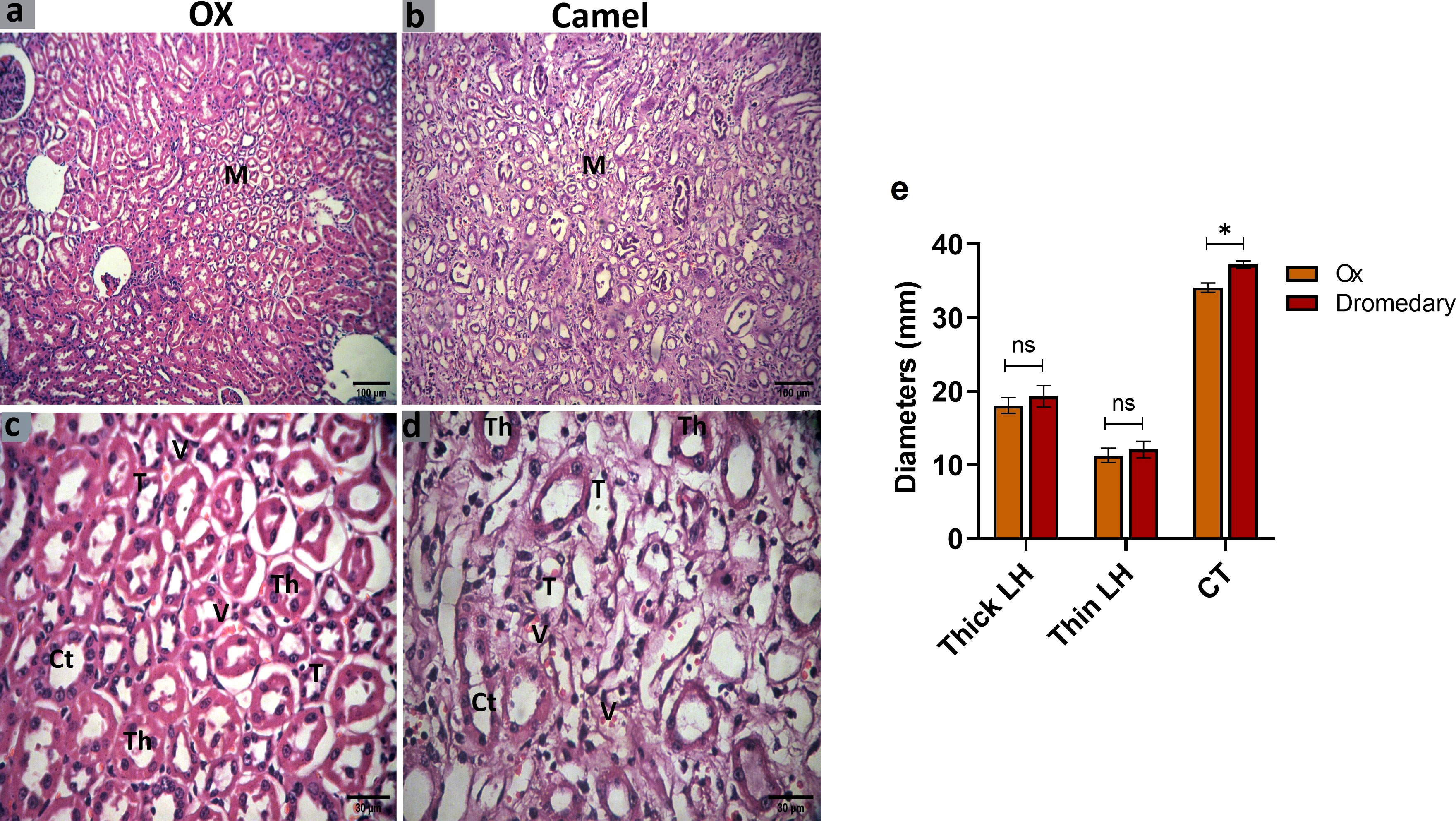
Figure 8 Histologic sections of the oxen and dromedary kidney show that the dromedary kidney contains wider CTs. The renal medulla (M) of the oxen and dromedary (A, B, respectively). Scale bar, 100 μm; hematoxylin and eosin stain. collecting tubules-CT, the thin segment of the loop of Henle-T, the thick segment of the loop of Henle-Th, and vasa recta-V. The renal medulla of the oxen and dromedary (C, D, respectively). Scale bar, 30 μm; hematoxylin and eosin stain. (E) Morphometry analysis for diameters of thin and thick segments of the loop of Henle, and CT. Data shown are represented as mean ± SD.*p<0.05.
3.6 Immunohistochemical findings
We further evaluated the renal AQP2 expression which potentially mediates the water reabsorption from the renal tubules and consequently leads to concentrated urine. Immunohistochemical analysis for AQP2 protein expression in the renal tissues of the ox and dromedary is shown in Figures 9, 10. As we noted, the nephron of the oxen kidney showed a negative expression for AQP2 in glomeruli, mild expression in the basal borders of the DCTs lining epithelium, and strong expression in PCTs. In addition to the negative AQP2 expression in glomeruli of dromedary’s nephrons, a strong expression in the cytoplasm of cells lining DCTs and in membranous-cytoplasmic expression in epithelium of PCTs were observed. Moreover, in the ox’s kidney, moderate cytoplasmic and membranous AQP2 expressions in the epithelium layer lining the collecting tubules were observed and were mainly distributed over the basolateral borders. In the dromedary, the lining epithelium of collecting tubules showed strong expression, and moderate expression was noted in the loop of Henle.
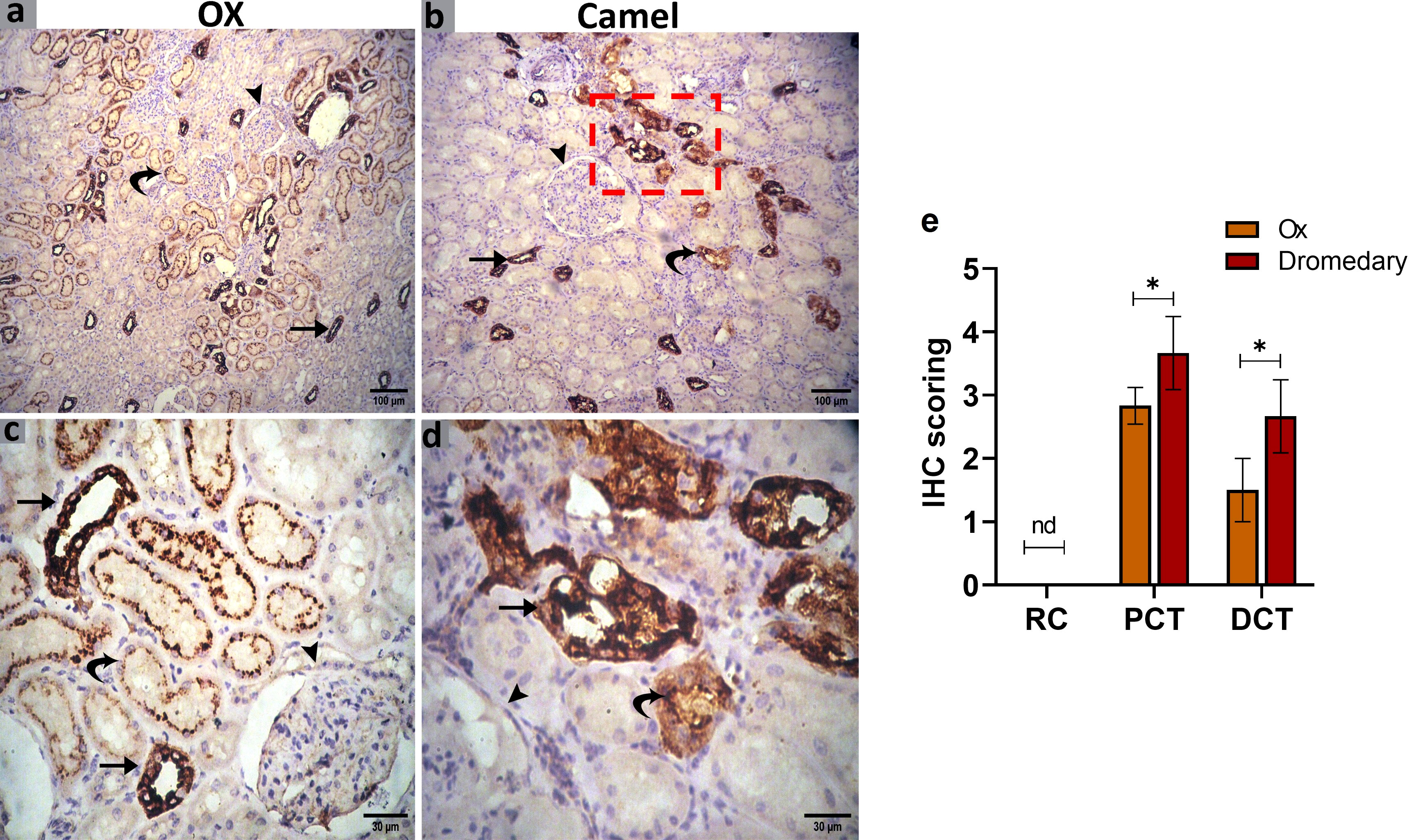
Figure 9 (A, C) Immunohistochemical analysis for AQP2 protein expression in the renal tissues of the oxen. A negative expression in the glomerulus (arrowhead), a mild expression in the distal convoluted tubules (DCT, curved arrow), and moderate expression in the proximal convoluted tubules (PCT, arrows) were noted. Scale bar, 100 μm and 30 μm. (B, D) Immunohistochemical analysis for AQP2 protein expression in the renal tissues of the dromedary kidney. A negative expression in glomeruli (arrowhead), moderate expression in the DCT (curved arrow), and a strong expression in the PCT (arrows) were detected. Scale bar, 100 μm and 30 μm. (E) Expression scoring for the expression of AQP2 along the nephron components in kidneys of oxen and dromedaries. A minimum of 3 slides were assessed for each species. Data shown are represented as mean ± SD.*p<0.05.
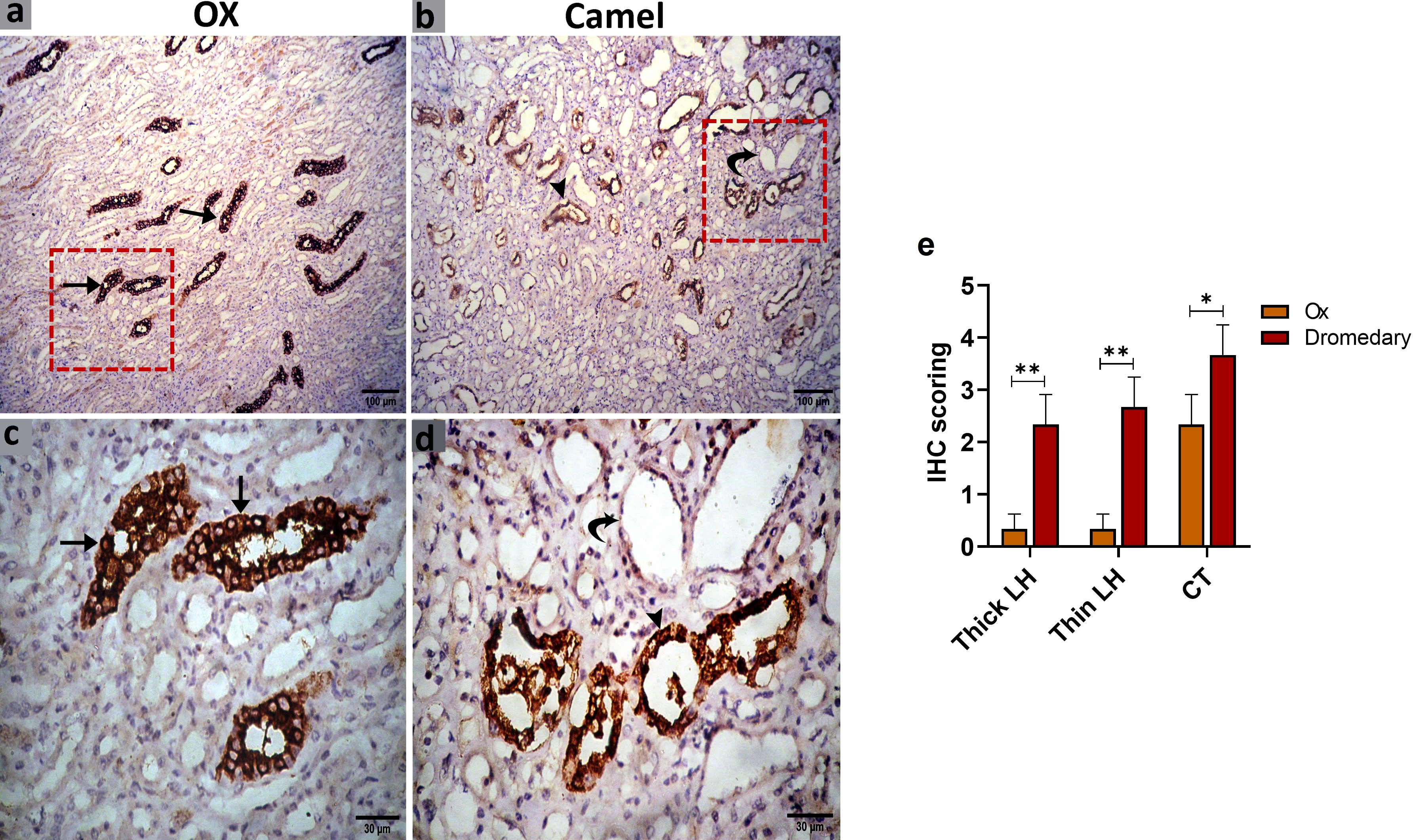
Figure 10 (A, C) Immunohistochemical analysis for AQP2 protein expression in the renal tissues of the oxen. a moderate expression in the epithelium lining the collecting tubules (CT, arrows) was noted. (B, D) Immunohistochemical analysis for AQP2 protein expression in the renal tissues of the dromedary kidney. A strong expression in the lining epithelium of CT (arrowheads) and moderate expression in the loop of Henle (curved arrows) was observed. (E) Expression scoring for the expression of AQP2 in kidneys of oxen and dromedaries. A minimum of 3 slides were assessed for each species. Data shown are represented as mean ± SD.*p<0.05, **p<0.01.
4 Discussion
Urinalysis is used to reflect the health status of the urinary system and to investigate various types of renal disorders (El-Deeb and Buczinski, 2015). In the current study, urinalysis by test strip revealed that normal urine of both dromedaries and oxen was yellow in color, and the pH was alkaline. Similarly, a previous study (Gole, 2020), has shown that normal camel urine is yellow to amber and has a pH range of 7-8.5. Moreover, it has been demonstrated in cattle that the normal color of urine is yellow to light amber and attributed this to the presence of constantly released urochromes (Zanetti et al., 2008). Normal cattle have an alkaline urinary pH ranging from 7.4 to 8.4 pH (Mavangira et al., 2010). In both species, herein, there were no protein, glucose, bile salts, pigments, and urobilinogen detected in their normal urine samples. According to a few references about urine in cattle, protein is not found in the urine or normally contains only traces of proteins (Trang et al., 2014; Herman et al., 2019). Microscopically, samples of dromedaries and oxen urine revealed the absence of crystals and epithelial cells, in addition to traces of RBCs and pus cells, with no statistically significant differences between the urine of both species. It has been proposed that the camel can sustain surviving in harsh dry conditions without dehydration by conserving water and electrolyte balance inside its body (Abdalla, 2020). Pursuantly to this data, we found that the dromedaries contained higher levels of sodium and potassium ions in urine, and sodium and chloride ions in serum compared to those in urine and serum samples of oxen. The reduction in the volume of plasma in response to water restriction leads to an increase in the osmolarity of the blood plasma, which leads to a raising concentration of electrolytes in plasma (Kaliber et al., 2016), especially sodium and chloride ions. Moreover, after water intake following hypovolemia, the sudden flow of water fills the space outside and inside the cells, thus it is crucial to retain as much sodium as possible to prevent diluting body fluids (Abdoun et al., 2010). It is well known that the camel is the best-adapted animal to harsh desert conditions. About 235000 heads of camels (Camelus dromedarius) are present in Egypt (Saoud, 1999) and found mostly in the arid and semi-arid parts. Such regions are characterized by harsh environmental conditions including water scarcity. It is common for grazing animals to go for several days with little or no drinking water (Abdel Rahman et al., 2002). Many studies dealt with the physiological responses of camels to water deprivation (Ben-Goumi et al., 1993; Assad et al., 1997).
Renal function was also affected by dehydration. Serum sodium levels were significantly increased (p<0.001) across dehydration in the dehydrated group compared to the control and the values were nearly similar to that studied in the current work (175.3 ± 2.2 mmole/l) (Al Haj, 2013).
The lower concentration of potassium in the serum of dromedaries compared to oxen may attribute to a decrease in the concentration of the hormone aldosterone in dromedaries. In mammals, the hormone aldosterone promotes sodium absorption and potassium secretion by means of a renal distant tube collection channel system. Potassium increases the secretion of aldosterone by the adrenal cortex, and aldosterone lowers serum potassium by stimulating its kidney excretion (Verma et al., 2022).
In contrast to oxen, the significantly lower serum concentration of serum aldosterone hormone in dromedaries could be attributed to a decrease in serum potassium and an increase in serum sodium levels. Bekele et al. (2013) proposed that an increase in the concentration of sodium in plasma inhibits the secretion of aldosterone during dehydration, thereby avoiding a hyperosmotic load (Bekele et al., 2013).
Blood creatinine is directly proportional to the individual’s muscle mass. A stable amount of creatinine is presented to the kidneys daily for excretion. Creatinine concentration can vary based on several factors including the animal’s diet, muscle mass, and gender (Deen, 2013). Eyob et al. (2018) reported maximum values for creatinine that reach 2.97 mg/dl in Somali camels which are attributed to high muscle mass. In addition, this increase may be attributed to the dry season on which samples were collected as previously reported by Worku et al. (2021) who attributed this increase to camel recycling of a substantial quantity of protein for body use during the dry season, which may boost total protein levels in the body blood. The current study found that dromedaries had higher concentrations of serum urea and creatinine than oxen, but there was no difference in serum uric acid values between the two species. Urea concentrations in the dromedary’s blood may be high due to strong kidney filtration. Consistently, the results of previous studies have revealed that camels have higher urea concentrations than sheep (Badakhshan and Mirmahmoudi, 2016). In the latter study, it was explained that increased water re-absorption in nephron collecting tubules, and since urea is a highly permeable molecule, it is expected to increase. Moreover, hypovolemia due to lack of water leads to a decrease in renal blood flow, which leads to a decrease in the rate of renal filtration and accordingly a high concentration of urea in the blood. Creatine in the blood is produced from the phosphocreatine in muscle tissues to produce spontaneous, irreversible, and non-enzymatic energy during tissue metabolism.
In oxen, the kidney appeared light brownish and lobulated, while the kidney of the dromedary was reddish-brown and without external lobulation similar to small ruminants, horses, pigs, dogs & cats (Frandson et al., 2009; Dyce et al., 2010). Statistically, here, all the biometric parameters of the left kidney were greater than the right kidney. In this study, the dromedary kidney did not have minor and major calyces, and the medullary pyramids converge forming a thick renal crest projected into the renal pelvis (multilobed, mono-papillary). Similar findings were observed in equine, caprine, ovine, and canine kidneys and were different from the kidneys of bovines and pigs (multilobed, multi-papillary) (Rowen and Wilke, 2009). In dromedaries, the structure of the renal pelvis is somewhat complicated due to the formation of three-dimensional extensions emanating from the main pelvis cavity (body) (Abdalla, 2020). Conversely, the ox had no renal pelvis, and the major calyx was directly connected to the ureter (Sawad, 2006). Previous studies have shown that the formation of concentrated urine and hence water conservation relies on three main features. These features include the relative thickness of the renal medulla (Vimtrup and Schmidt-Nielsen, 1952), the architecture of the renal pelvis (Pfeiffer, 1968), and the cortical tubules (Tharwat, 2020). The camel which inhabits arid environments with water shortage for a prolonged time or were accustomed to drinking salty water or eating highly salty food can excrete highly concentrated urine. However, ox which inhabits moist climates or regions with adequate water supply, generally, excrete more diluted urine. These properties must be relevant to the thickness of the renal medulla. Consistently, as we observed, the medullary portion was broader in the dromedary than in the ox. The renal medulla of the dromedary had the anatomical basis to create a gradient of high osmotic pressure that was necessary to produce hypertonic urine (Xu et al., 2009; Bello et al., 2013; Tharwat, 2020). Because of the large renal pelvic-medullary interface in the camel; urea could be recycled leading to the build of medullary osmotic concentration (Zguigal and Ouhsine, 2004), this was contrary to the bovine kidney where there was no renal pelvis.
Our study showed that the kidney of the ox was enveloped by a thin capsule of collagenous tissue, in contrast to the dromedaries. Similar findings were documented by Eissa et al. (2018).The RCs were distributed in the middle of the cortex section and cortico-medullary junction. However, in dromedaries, RCs were found mainly in sub-capsular and mid-cortical zones. Similar observations were stated by Bargooth et al. (2020) who added that the cortical layer can reach up to half of the kidney size in camels. RCs are composed of a double layer of the Bowman’s capsule enclosing a narrow urinary space with a glomerulus as mentioned in rats (Al-Samawy, 2012). Eissa et al. (2019) reported that simple squamous, as well as simple cuboidal epithelia formed the parietal layer of Bowman’s capsule which varied from our current work. The RCs and glomeruli in dromedary kidneys are larger than those recognized in the kidneys of the ox. These findings were supported by the approved idea that dromedaries suffering dehydration have a 73% reduction of the reuptake of sodium from tubular lumens resulting in concentrating the urine and better preserving water in contrast to the bovine kidney which is unable to form concentrated urine (Rowen and Wilke, 2009; Adem et al., 2013). In this study, PCTs were lined with simple cuboidal epithelium and found larger in the case of dromedaries than oxen. Similarly, in a previous study, the PCTs of dromedaries showed wide lumens with spherical nuclei in their lining cells (Bargooth et al., 2020; Ishaya et al., 2021). The DCTs were dissimilar to the PCTs in the epithelium lining which had large nuclei and possesses no brush border. Also, the DCTs were shorter than the PCTs, and fewer in number in the regional cortex (Al-Samawy, 2012). Additionally, in the current study, the collecting tubules were lined by cuboidal epithelium which was characterized by clear cellular boundaries and spherical nuclei when compared with the cells of the PCTs and DCTs. We found that the dromedary had a longer loop of Henle, wider collecting tubules, and numerous vasa recta than the ox. It is suggested that dromedaries can concentrate their urine and preserve water via a long and well-specialized loop of Henle-collecting tubule distance that promotes maximum water reabsorption from the urine (Bargooth et al., 2020). The thickness ratio of the renal medulla/cortex was 4:1 which indicated that Henle’s loops in the dromedary’s kidneys were very long (Al-Samawy, 2012). Aquaporin 2 (AQP2) is a small, integral tetrameric plasma membrane protein that is expressed in mammalian kidneys and is vital for hypertonic urine production (Kortenoeven and Fenton, 2014). In our study, the protein expression of AQP2 in the ox kidneys showed a strong expression of PCTs while in dromedaries it showed moderate expression of DCTs and a strong expression in the PCTs. Wang et al. (2018) documented that that epithelial cells of the DCT were strongly reacted to AQP2. However, Brandt et al. (2012) and Wang et al. (2018) claimed that AQP2 was expressed only in the lining epithelium of collecting tubules. The strong expression of AQP2 in PCT lining cells may indicate their potential contribution to the hemostasis of fluid, electrolytes, and nutrients. This homeostasis is carried out by reabsorbing around 70% of water and NaCl, a greater proportion of the Sodium bicarbonate (NaHCO3), and nearly all the nutrients in the ultrafiltrate (Curthoys and Moe, 2014). Also, as we noted, the expression of AQP2 was localized in the epithelium lining the collecting tubules, especially in the plasma membrane (Michalek et al., 2014). The immune expression in the case of dromedaries showed strong expression of the lining epithelium of collecting tubules and the moderate reaction of the loop of Henle (Khafaga et al., 2021). Altogether, these data strongly suggest that dromedaries can produce highly concentrated urine, which was ensured by Alvira-Iraizoz et al. (2021) who revealed that water retention in the kidney indirectly facilitated by the AQP2-mediated water reabsorption. However, the specific function and underlying mechanisms of this observation are not clear and should further be investigated.
5 Conclusion
There were obvious differences between dromedaries and oxen for concentrations of inorganic ions in both serum and urine. dromedaries had higher concentrations of sodium and potassium in the urine as well as urea, creatinine, sodium, and chloride in the blood compared to oxen. Moreover, the thicker renal medulla and more prevalence of AQP2 expression in the dromedary’s kidney than in oxen were also evident. However, aldosterone levels were lower in the serum of dromedaries than in oxen. To this end, the kidneys of the dromedary are more compatible with the production of concentrated urine than the ox. Therefore, the dromedary can be usefully employed as a target animal model that helps to understand the process of water conservation in mammals.
Data availability statement
The original contributions presented in the study are included in the article. Further inquiries can be directed to the corresponding author.
Ethics statement
This study was carried out under the guidance of rules of the Ethical Committee and Animal Welfare of the Faculty of Veterinary Medicine, Zagazig University, Egypt, and with the approval of the Institutional Animal Care and Use Committee (Approval # ZU-IACUC/2/F/164/2022) of Zagazig University. Written informed consent was obtained from the owners for the participation of their animals in this study.
Author contributions
Conceptualization, EE-H, AB, NG, AA-I and MA. methodology, EE-H, AB, NG, AA-I and MA. software, EE-H, AB, NG, AA-I, AA and MA. validation, EE-H, AB, NG, AA-I, AA, AA-D and MA. formal analysis, EE-H, AB, NG, AA-I and MA. investigation, EE-H, AB, NG, AA-I, AA-D., MAA and MA. data curation, EE-H, AB, NG, AA-I AA, AA-D and MA. writing—original draft preparation, EE-H, AB, NG, AA-I and MA. writing—review and editing, EE-H, AB, NG, AA-I and MA. visualization, EE-H, AB, NG, AA-I, IE-R., MAA and MA. supervision, EE-H, AB, NG, AA-I and MA. project administration, EE-H, AB, NG, AA-I and MA. funding acquisition, AA, AA-D., IE-R, and MAA. All authors contributed to the article and approved the submitted version.
Funding
This research was supported by the Small Groups Project under grant number (RGP.1/84/43) funded by the Deanship of Scientific Research at King Khalid University. The authors would like to introduce great thanks to the Deanship of Scientific Research at Umm Al-Qura university for supporting this work with grant number (23UQU4320609DSR04).
Conflict of interest
The authors declare that the research was conducted in the absence of any commercial or financial relationships that could be construed as a potential conflict of interest.
Publisher’s note
All claims expressed in this article are solely those of the authors and do not necessarily represent those of their affiliated organizations, or those of the publisher, the editors and the reviewers. Any product that may be evaluated in this article, or claim that may be made by its manufacturer, is not guaranteed or endorsed by the publisher.
References
Abdalla M. A. (2020). Anatomical features in the kidney involved in water conservation through urine concentration in dromedaries (Camelus dromedarius). Heliyon. 6, e03139. doi: 10.1016/j.heliyon.2019.e03139
Abdel Rahman H., El-Sherif M. M. A., Omar S. S., El Sayed M. A., Ibrahim N. H. (2002). Effect of drinking diluted seawater on some physiological aspects of camels. J. Anim. Sci. 80 (Supp. 1). 85, Supp 1.
Abdoun K., Alameen A., Elmagbol W., Makkawi T., Al-Haidary A. (2010). Effect of dehydration and rehydration on serum and forestomach osmolality, Na and K concentrations in camelus dromedaries. Online J. Vet. Res. 14, 143–152.
Adem A., Al Haj M., Benedict S., Yasin J., Nagelkerke N., Nyberg F., et al. (2013). ANP and BNP responses to dehydration in the one-humped camel and effects of blocking the renin-angiotensin system. PloS One 8, e57806. doi: 10.1371/journal.pone.0057806
Ala M., Mohammad Jafari R., Hajiabbasi A., Dehpour A. R. (2021). Aquaporins and diseases pathogenesis: From trivial to undeniable involvements, a disease-based point of view. J. Cell Physiol. 236 (9), 6115–6135. doi: 10.1002/jcp.30318
Al Haj M. (2013). Effects of dehydration and blockade of the renin-angiotensin system in the one-humped camel (Camelus dromedarius). Acta Universitatis Upsaliensis Digital Compr. Summaries Uppsala Dissertations Faculty Pharm. 178, 52.
Al-Samawy E. R. M. (2012). Morphological and histological study of the kidneys on the albino rats. Al-Anbar J. Vet. Sci. 5.
Alvira-Iraizoz F., Gillard B. T., Lin P., Paterson A., Pauža A. G., Ali M. A., et al. (2021). Multiomic analysis of the Arabian camel (Camelus dromedarius) kidney reveals a role for cholesterol in water conservation. Commun. Biol. 4 (1), 779. doi: 10.1038/s42003-021-02327-3
Assad F. S., Bayoumi M. T., El-Sherif M. A. (1997). The role of some ecosystem stress on some blood characteristics of camels raised under semi-arid conditions. Egyp Appl. Sci. 12 (4), 1–10.
Badakhshan Y., Mirmahmoudi R. (2016). Blood metabolites of one-humped camel (Camelus dromedarius) versus sheep during summer heat stress. I J. V M 10, 65–71. doi: 10.22059/IJVM.2016.57052
Bargooth A. F., Al-A´araji A. S., Medakel M. S. (2020). A histological study of the renal corpuscle nephron distribution in Iraqi camels (Camelus dromedaries). Indian J. Foren Med. Toxicol. 14, 2481–2484.
Bekele T., Olsson K., Olsson U., Dahlborn K. (2013). Physiological and behavioral responses to different watering intervals in lactating camels (Camelus dromedarius). Am. J. Physiol. Regul. Integr. Comp. Physiol. 305, R639–R646. doi: 10.1152/ajpregu.00015.2013
Bello A., Lawal U. F., Onyeanusi B. I., Sonfada M. L., Umaru M. A., Onu J. E., et al. (2013). Prenatal development of the kidney of one-humped camel (Camelus dromedarius) – a histomorphometric study. Afr J. BioMed. Res. 16, 31–37. doi: 10.4314/AJBR.V16I1
Ben-Goumi M., Riad F., Giry J., Farge F., Safwate A., Davicco M. J., et al. (1993). Hormonal control of water and sodium in plasma and urine of camels during dehydration and rehydration. Gen. Comp. Endocrinolo 89 (3), 378–386. doi: 10.1006/gcen.1993.1045
Brandt L. E., Bohn A. A., Charles J. B., Ehrhart E. J. (2012). Localization of canine, feline, and mouse renal membrane proteins. Vet. Pathol. 49, 693–703. doi: 10.1177/0300985811410720
Breulmann M., Boer B., Wernery U., Wernery R., El-Shaer H., Alhadrami G., et al. (2007). A proposal towards combating desertifcation via establishment of camel farms based on fodder production from indigenous plants and halophytes Vol. 14 (UAE: UNESCO-Doha).
Curthoys N. P., Moe O. W. (2014). Proximal tubule function and response to acidosis. Clin. J. Am. Soc. Nephrol 9, 1627–1638. doi: 10.2215/CJN.10391012
da Costa A. N., Feitosa J. V., Montezuma P. A. Jr, de Souza P. T., de Araújo A. A. (2015). Rectal temperatures, respiratory rates, production, and reproduction performances of crossbred girolando cows under heat stress in northeastern Brazil. Int. J. Biometeorol 59 (11), 1647–1653. doi: 10.1007/s00484-015-0971-4
Dart A. B., Wicklow B., Scholey J., Sellers E. A., Dyck J., Mahmud F., et al. (2020). An evaluation of renin-angiotensin system markers in youth with type 2 diabetes and associations with renal outcomes. Pediatr. Diabetes 21, 1102–1109. doi: 10.1111/pedi.13081
Deen A. (2013). Serum creatinine, urea nitrogen and endogenous creatinine clearance based glomerular filtration rate in camels to evaluate renal functions. Camel: Inter J. Vet. Sci. 1 (1), 1–12.
Dyce K. M., WOa S., Wensing C. J. (2010). Textbook of veterinary anatomy (Philadelphia. London. New York: W.B. Saunders Company).
Eissa L., Ismail H. I., Elhassan M. M. O., Ali H. A. (2019). Basement membranes in the kidney of the dromedary camel (Camelus dromedarius): An immunohistochemical and ultrastructural study. Acta Histochem. 121 (4), 419–429. doi: 10.1016/j.acthis.2019.03.005
Eissa L., Ismail I., Elhassan M., Yaseen R., Ali H. (2018). Comparative histology and histometry of the renal capsule in dromedary she camel (Camelus dromedarius), cow (Bos indicus) and ewe (Ovis aires). J. Camel Res. Prod. 2 (1), 2018.
El-Deeb W. M., Buczinski S. (2015). The diagnostic and prognostic importance of oxidative stress biomarkers and acute phase proteins in urinary tract infection (UTI) in camels. PeerJ. 3, e1363. doi: 10.7717/peerj.1363
Eyob I., Gezahegne M., Alemayew L., Simenew K. (2018). Serum biochemical parameters of apparently healthy camels (Camelus dromedarius) of Ethiopia. Euro J. Biolo Sci. 10 (3), 80–85.
Frandson R. D., Wilke W. L., Fails A. D. (2009). Anatomy and physiology of farm animals. In: Wiley Blackwell.
Gole F. (2020). Review on health benefits of camel urine: Therapeutics effects and potential impact on public health around East hararghe district. Am. J. Pure Appl. Biosci. 2, 183–191. doi: 10.34104/ajpab.020.01830191
Hamada T., Igawa O., Shigemasa C., Hisatome I. (2008). [How do we set the standard value of serum uric acid levels?]. Nihon Rinsho 66 (4), 723–727.
Herman N., Bourges-Abella N., Braun J. P., Ancel C., Schelcher F., Trumel C. (2019). Urinalysis and determination of the urine protein-to-creatinine ratio reference interval in healthy cows. J. Vet. Intern. Med. 33, 999–1008. doi: 10.1111/jvim.15452
Hoter A., Rizk S., Naim H. Y. (2019). Cellular and molecular adaptation of Arabian camel to heat stress. Front. Genet. 19 (10), 588. doi: 10.3389/fgene.2019.00588
Ishaya L., DibaL N., Chiroma S., Attah M. (2021). Comparative anatomical study of the kidneys in cattle (Bos taurus) and camel (Camelus dromederus). J. Morphol Sci. 38, 386–391. doi: 10.51929/jms.38.67.2021
JingBao W., HaiYan Li, ZuXian H., BaoPing S., JianLin W. (2014). Renal expression and functions of AQP1 and AQP2 in bactrian camel (Camelus bactrianus). J. Camel Pract. Res. 21 (2), 153. doi: 10.5958/2277-8934.2014.00027.7
Kaliber M., Koluman N., Silanikove N. (2016). Physiological and behavioral basis for the successful adaptation of goats to severe water restriction under hot environmental conditions. Animal. 10, 82–88. doi: 10.1017/S1751731115001652
Khafaga A. F., Elewa Y. H. A., Atta M. S., Noreldin A. E. (2021). Aging-related functional and structural changes in renal tissues: Lesson from a camel model. Microsc Microanal 10, 1–13. doi: 10.1017/S1431927621000210
Kortenoeven M. L., Fenton R. A. (2014). Renal aquaporins and water balance disorders. Biochim. Biophys. Acta 1840, 1533–1549. doi: 10.1016/j.bbagen.2013.12.002
Larsen K. (1972). Creatinine assay in the presence of protein with LKB 8600 reaction rate analyser. Clin. Chim. Acta 38, 475–476. doi: 10.1016/0009-8981(72)90146-5
Lin P., Gillard B. T., Pauža A. G., Iraizoz F. A., Ali M. A., Mecawi A. S., et al. (2022). Transcriptomic plasticity of the hypothalamic osmoregulatory control centre of the Arabian dromedary camel. Commun. Biol. 5 (1), 1008. doi: 10.1038/s42003-022-03857-0
Mavangira V., Cornish J. M., Angelos J. A. (2010). Effect of ammonium chloride supplementation on urine pH and urinary fractional excretion of electrolytes in goats. J. Am. Vet. Med. Assoc. 237, 1299–1304. doi: 10.2460/javma.237.11.1299
Michalek K., Laszczynska M., Ciechanowicz A. K., Herosimczyk A., Rotter I., Oganowska M., et al. (2014). Immunohistochemical identification of aquaporin 2 in the kidneys of young beef cattle. Biotech. Histochem. 89, 342–347. doi: 10.3109/10520295.2013.858828
Ouajd S., Kamel B. (2009). Physiological particularities of dromedary (Camelus dromedarius) and experimental implications. Scand. J. Lab. Anim. Sci. 36, 19–29.
Pfeiffer E. W. (1968). Comparative anatomical observations of the mammalian renal pelvis and medulla. J. Anat. 102, 321–331.
Rowen D. F., Wilke W. L. (2009). Anatomy and physiology of farm animals. Ed. Blackwell W.-. (Iowa, USA: A John Wiley & Sons, Inc). F. AD.
Saoud A. E. (1999). The camel in the desert (Egypt: Desert Research Center, Ministry of Agriculture).
Sawad A. A. (2006). Functional anatomy of the kidney of the buffaloes. Iraqi J. Vet. Med. 42 (1), 92–98. doi: 10.33762/bvetr.2006.58814
Suvarna K. S., Layton C., John D. (2019). Bancroft’s theory and practice of histological techniques (New York, London: Elsevier Ltd).
Tharwat M. (2020). Ultrasonography of the kidneys in healthy and diseased camels (Camelus dromedarius). Vet. Med. Int. 2020, 7814927. doi: 10.1155/2020/7814927
Trang N. T., Hirai T., Nabeta R., Fuke N., Yamaguchi R. (2014). Membranoproliferative glomerulonephritis in a calf with nephrotic syndrome. J. Comp. Pathol. 151, 162–165. doi: 10.1016/j.jcpa.2014.05.001
Verma A., Vaidya A., Subudhi S., Waikar S. S. (2022). Aldosterone in chronic kidney disease and renal outcomes. Eur. Heart J. 43 (38), 3781–3791. doi: 10.1093/eurheartj/ehac352
Vimtrup B., Schmidt-Nielsen B. (1952). The histology of the kidney of kangaroo rats. Anat Rec. 114, 515–528. doi: 10.1002/ar.1091140402
Wang J., Yang M., Wang Z., j Wu, Chen J., Yan W., et al. (2018). Expression of aquaporin 2, aquaporin 3 and aquaporin 4 in renal medulla of bactrian camel (Camelus bactrianus). Int. J. Morphol 36 (1), 303–309. doi: 10.4067/S0717-95022018000100303
Worku K., Kechero Y., Janssens G. P. (2021). Measuring seasonal and agro-ecological effects on nutritional status in tropical ranging dairy cows. J. Dairy Sci. 104 (4), 4341–4349. doi: 10.3168/jds.2020-18995
Wu H., Guang X., Al-Fageeh M. B., Cao J., Pan S., Zhou H., et al. (2014). Camelid genomes reveal evolution and adaptation to desert environments. Nat. Commun. 5, 5188. doi: 10.1038/ncomms6188
Xu C., Bao H., Qi F., Liu Y., Qin J., Gandahi J., et al. (2009). Morpho-histological investigation of kidney of bactrian camel (camelus bactrianus). J. Camel Pract. Res. 16, 1–6.
Zanetti G., Paparella S., Trinchieri A., Prezioso D., Rocco F., Naber K. G. (2008). Infections and urolithiasis: Current clinical evidence in prophylaxis and antibiotic therapy. Arch. Ital Urol Androl 80, 5–12.
Keywords: dromedary, kidney, oxen, concentrated urine, AQP2, evolutionary adaptation
Citation: El-Hady E, Behairy A, Goda NA, Abdelbaset-Ismail A, Ahmed AE, Al-Doaiss AA, Abd El-Rahim I, Alshehri MA and Aref M (2023) Comparative physiological, morphological, histological, and AQP2 immunohistochemical analysis of the Arabian camels (Camelus dromedarius) and oxen kidney: Effects of adaptation to arid environments. Front. Anim. Sci. 4:1078159. doi: 10.3389/fanim.2023.1078159
Received: 24 October 2022; Accepted: 27 February 2023;
Published: 27 March 2023.
Edited by:
Adem Kara, Erzurum Technical University, TürkiyeReviewed by:
Abdelkareem Ahmed, Botswana University of Agriculture and Natural Resources, BotswanaAvinash Premraj, Management of Scientific Centers & Presidential Camels, United Arab Emirates
Copyright © 2023 El-Hady, Behairy, Goda, Abdelbaset-Ismail, Ahmed, Al-Doaiss, Abd El-Rahim, Alshehri and Aref. This is an open-access article distributed under the terms of the Creative Commons Attribution License (CC BY). The use, distribution or reproduction in other forums is permitted, provided the original author(s) and the copyright owner(s) are credited and that the original publication in this journal is cited, in accordance with accepted academic practice. No use, distribution or reproduction is permitted which does not comply with these terms.
*Correspondence: Ahmed Abdelbaset-Ismail, YWFpc21hZWlsQHZldC56dS5lZHUuZWc=