- Department of the Sciences of Agriculture, Food, Natural Resources and Engineering, University of Foggia, Foggia, Italy
In the present study individual milk samples were collected from 80 Garganica goats and the population was genotyped for CSN1S1. Seven different alleles were observed with the A allele found as predominant, followed by the F and B allele, and 14 different genotypes were observed. A proteomic approach was used to classify the individual milk on the basis of protein abundance: four levels of αs1-casein (CN) expression were identified as high (HAS-1), intermediate (IAS-1), low (LAS-1), and null (NAS-1). The present paper aimed to investigate the influence of the level of expression of αs1-casein fraction in milk on proteolytic activities, chemical composition, and renneting properties of individual Garganica goat milk. Results from the present study evidenced that the level of expression of αs1-casein fraction in Garganica goat milk affected chemical composition, curd firmness, and indigenous proteolytic enzymes. In particular, fat, protein, and casein content were higher in HAS-1 and IAS-1 compared to LAS-1 and NAS-1. The SCC decreased passing from the HAS-1 to the NAS-1 and the curd firmness displayed the highest value in HAS-1. The activity of plasmin (PL) was higher in HAS-1 and IAS-1 groups compared to the LAS-1 and NAS-1; whereas, PL plasminogen-derived (PG) activity was the highest in NAS-1 group. On the contrary, the activity of elastase was the highest in LAS-1, intermediate in the IAS-1 group, and the lowest in HAS-1 and NAS-1 groups. Present data demonstrated that the level of synthesis of αs1-CN reflects the efficiency of the mammary gland in the activation of the cellular mechanisms and influences the proteolytic activity in milk, especially the PL-PG system.
Introduction
Recent growing attention developed around goat milk, both from multiple scientific implications and market exploitation, has contributed to the enhancement of autochthonous breeds and their productions (Park et al., 2007). Studies on goat milk and meat products are focused on specific compounds able to exert a potentially positive effect on human health beyond basic nutrition (Albenzio et al., 2016). The key aspect of goat milk, in relation not only to its nutritional and health aspects but also to its technological value, seems to lie in the high and complex genetic polymorphism of its proteins, in particular of casein fractions (Selvaggi et al., 2014). Notably, goat milk genetic polymorphisms make such milk a valuable candidate as a substitute for cow milk for people suffering from cow milk allergies (Bevilacqua et al., 2001) or in children suffering from generalized epilepsy (Albenzio et al., 2016). Among the Italian autochthonous breed, the Garganica goat, reared in the Gargano area of the Apulia region (Southern Italy), is characterized by rusticity, longevity, and high adaptability to hard climatic conditions. The principal use of Garganica goat milk is the production of the typical Cacioricotta cheese (Albenzio et al., 2006). The high polymorphism found on CSN1S1 locus in individual Garganica goat milk (Albenzio et al., 2009) has evidenced the possibility to cluster the population into two groups distinguishable on the basis of total casein level. These authors have suggested that the Garganica goat could be selected to produce milk having different uses. For example, milk from animals characterized by the presence strong alleles at CSN1S1 and CSN2 could be used for cheesemaking, while milk from animals showing low or null alleles for CSN1S2 and CSN1S1 could be used for hypoallergenic milk production.
Proteolysis of milk proteins can be attributed to proteases of both indigenous, endogenous, and exogenous origin (Kelly et al., 2006). The plasmin-plasminogen (PL-PG) is the main indigenous proteolytic system composed by PL, its inactive zymogen PG, plasminogen activators (PA), plasminogen activator inhibitors (PAI), and plasmin inhibitors (PI) (Fox and Kelly, 2006). Cathepsin D and elastase are other important milk proteases, associated with somatic cells (Fox and Kelly, 2006; Albenzio and Santillo, 2011). Limited studies are reported on indigenous goat milk protease systems. However, as for bovine milk, plasmin is mainly relevant to the hydrolysis of β-casein and α-casein fractions, whereas elastase and cathepsin D are involved in limited proteolysis of milk caseins (Santillo et al., 2009).
Based on the high polymorphism found in CSN1S1 in the Garganica goat, it is able to influence the total casein level, and it can be hypothesized that the CSN1S1 genotype could also affect the expression and activity of indigenous proteolytic enzymes in goat milk. Therefore, the present paper aimed to investigate i) the CSN1S1 genotype of the Garganica goat population and ii) the influence of the level of expression of αs1-CN fraction on proteolytic activities of individual Garganica goat milk. Furthermore, the chemical composition and renneting properties of goat milk were investigated.
Materials and Methods
Milk Sampling and Analyses
Individual milk samples were collected from 80 Garganica goats reared in the Gargano area, Apulia, Southern Italy. Milk samples were analyzed for total protein, casein, fat, and lactose content using an infrared spectrophotometer (Milko Scan 133B; Foss Electric, Hillerød, Denmark), and for pH value (GLP 21 Crison, Spain). Somatic cell count (SCC) was determined using Fossomatic 90 (Foss Electric) instrument. Milk renneting characteristics as clotting time (r), curd firming time (k20), and curd firmness after 30 min (a30) were measured using a Foss Electric Formagraph (Foss Electric, Hillerød, Denmark). Plasmin (PL) and plasminogen- plasmin derived (PG), cathepsin D, and elastase activities were assayed according to Albenzio et al. (2015).
Milk DNA Analysis
Milk genomic DNA was extracted and analyzed for casein polymorphisms according to the procedure described by Albenzio et al. (2009). Locus CSN1S1 was analyzed by PCR-Restriction Fragment Length Polymorphism (RFLP) to identify alleles F and N* (including the alleles D and N) (Ramunno et al., 2000), and allele C (Cosenza et al., 2008) by Allele-Specific Amplification PCR (ASA-PCR) to identify allele B* (including the alleles B1, B2, B3, B4, L, M, which are not distinguishable from the B* allele) and E (Feligini et al., 2005), and alleles A* and 01 (Cosenza et al., 2003). The applied method does not differentiate among the A, G, H, I, and 02 alleles, therefore the A* allele could include these genetic variants.
2D Electrophoresis on Milk Samples
The first dimensional separation was performed on IPG dry strips (pH 3.9–5.1, 11 cm, Bio-Rad) through an IPG Protean IEF Cell (Bio-Rad, Watford, UK). Goat milk samples were first centrifuged at 4°C for 30 minutes at 2000 x g. Then skimmed milk was suspended in isoelectrofocusing IPG sample buffer (ready-Prep Rehydratation/Sample Buffer, Bio-Rad). The IPG strips were actively rehydrated at 50 V with samples for 2 h, with the following voltage gradient: 250 V for 1.5 h, from 250 to 4000 V for 1 h, and 4000 V for 4 h. Then, the strips were equilibrated for 10 min in a solution containing 0.375 M-Tris–HCl (pH 8.8), 6 M-urea, 200 ml glycerol/l, 20 g SDS/l and 20 g DTT/l, and then with the same solution containing 45 g iodoacetamide/l instead of DTT. The second-dimensional separation was carried out on a Protean II xi vertical slab gel unit (Bio-Rad) using 12% acrylamide separating gels at a voltage of 280 V. A broad range molecular weight electrophoresis calibration kit (Bio-Rad) was used as a standard. Gels were fixed and stained with R250 Coomassie blue (Bio-Rad). The de-stained gels were acquired through Chemi Doc XRS (Bio-Rad) white light conversion screen and quantitative analysis of the spots attributed to αs1-CN was performed by PD Quest 7.4.0 software (Bio-Rad). Landmark spots were used to check spot matching across all gels of the experiment and manual verification was performed to screen out any dust artefacts or incorrectly identified spots. The relative volume of each spot in a gel was normalized as a percentage of the total volume of all spots detected on the gel. Milk samples with strong (BC), intermediate (BE), low (FF) and null (NN) genotypes on αs1-CN were used as reference gels for protein spot detection and quantification; individual milk samples presenting the A* allele were assigned to the four levels on the basis of αs1-CN spots volume. Levels of αs1-CN expression were identified as follows: 41852 ± 870 arbitrary units (A.U.) high (HAS-1), 31248 ± 211 A.U. intermediate (IAS-1), 5457 ± 51 A.U. low (LAS-1), and 1218 ± 43 A.U. null (NAS-1) level of protein expression of the αs1-CN fraction.
Statistical Analysis
The effect of the αs1-CN expression levels was tested on milk chemical composition, renneting characteristics, and enzymatic activities using the GLM procedure of SAS (SAS Institute, 2013). Data were tested for normal distribution before performing statistical analysis. Pearson’s correlation coefficient was used to explore possible associations between the responses. The Cervus software version 3.0.3 (Marshall et al., 1998) was used to estimate allelic frequencies, expected and observed heterozygosity. The chi-square test (χ2) was employed to test the respect of Hardy-Weinberg equilibrium.
Results
Observed heterozygosity at the CSN1S1 locus in the population of the Garganica goat involved in the study was 0.772, whereas expected heterozygosity was 0.723; the locus did not show significant deviations from Hardy-Weinberg proportions (P < 0.01). The allelic frequencies observed at the CSN1S1 locus are reported in Table 1. Seven different alleles were found: the A allele was predominant, followed by the F and B allele which were characterized by halved frequency with respect to the A allele. The null alleles, 0, and N were observed at very low frequencies, although the former presented a frequency 4-times higher than the latter. The distribution of CSN1S1 genotypes is reported in Table 2A. A number of 14 different genotypes were observed. Four heterozygous genotypes presented the low allele F, characterized by different percentage being AF 21.05%, BF 10.53%, CF 8.77%, and F0 1.75%. AF genotype was the most frequent, although it showed frequencies quite similar to the AA and AB genotypes.
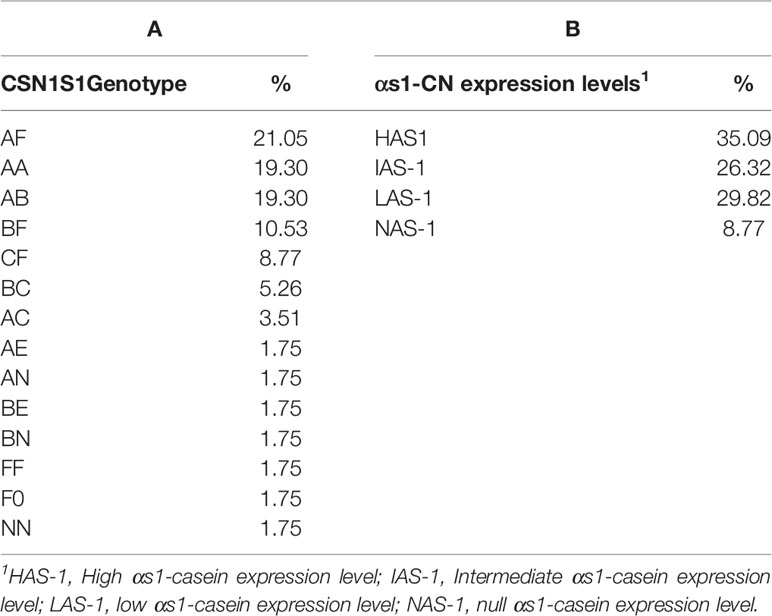
Table 2 CSN1S1 genotype (A) and αs1-casein (CN) expression levels (B) distribution in the Garganica goat population.
The identification of the A allele for the CSN1S1 locus by means of RFLP and ASA-PCR did not permit to distinguish among the strong A, H alleles, the intermediate I allele, the low G allele, and the null 02 allele. Therefore, the proteomic approach was used to discriminate the protein content associated with the individual milk samples. Figure 1 shows reference gels of goat individual milk denoting different level of αs1-CN expression. The milk samples were ascribed to four different groups in relation to protein abundance in high HAS-1, intermediate IAS-1, low LAS-1, and null NAS-1 αs1-CN fraction.
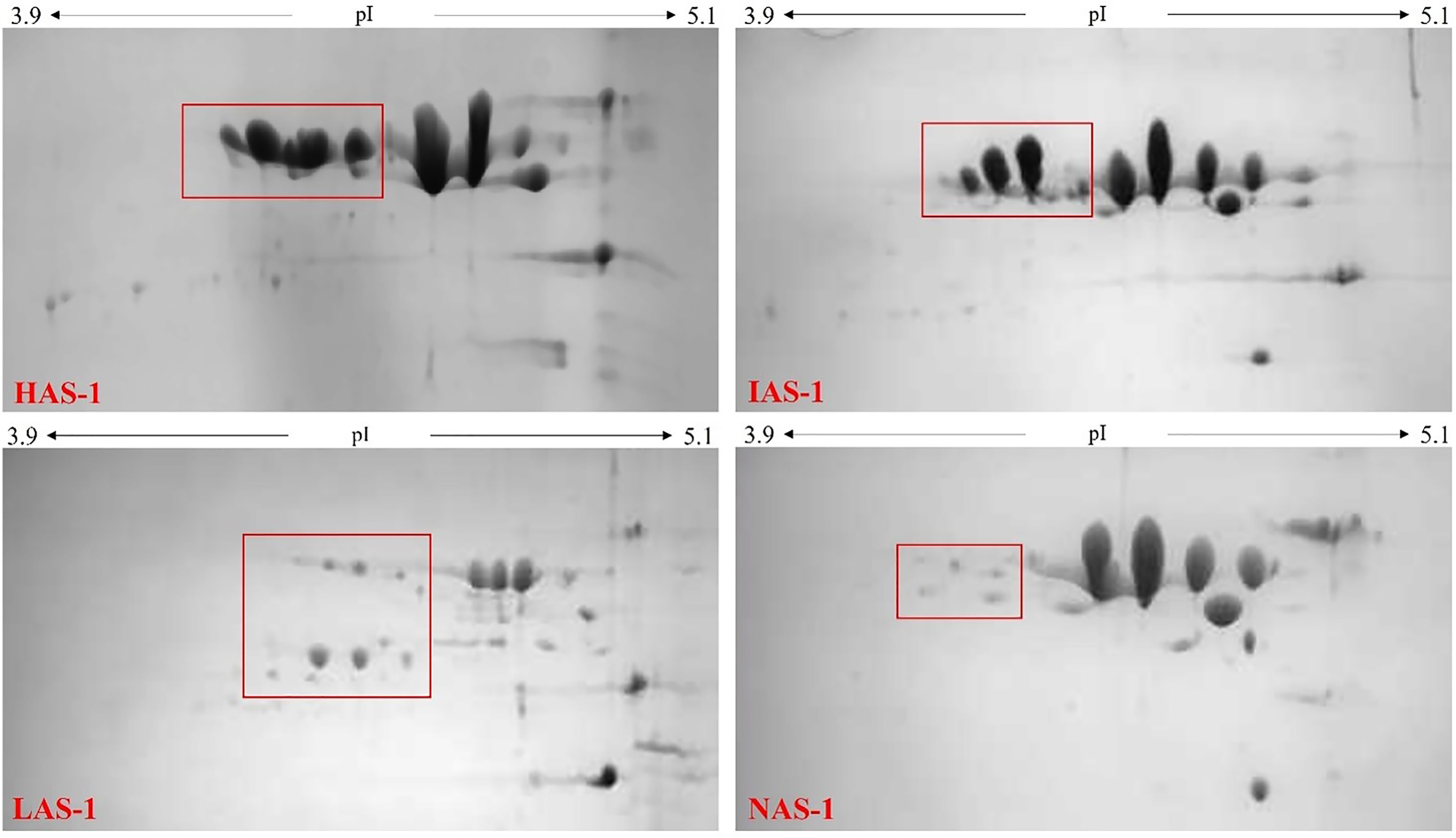
Figure 1 2D-electrophoresis gels of milk with different αs1-casein expression levels: High αs1-casein expression level (HAS-1); Intermediate αs1-casein expression level (IAS-1); Low αs1-casein expression level (LAS-1); Null αs1-casein expression level (NAS-1).
The percentage distribution of milk samples on the basis of αs1-CN abundance is represented in Table 2B, evidencing that high-αs1 (HAS-1) accounted for about 35% followed by low (LAS-1) and intermediate (IAS-1), while the null (NAS-1) was characterized by an incidence of about 9%.
The gross composition of the Garganica goat milk was affected by αs1-CN expression (Table 3). In particular, the SCC showed decreased levels passing from the HAS-1 to the NAS-1 level (P < 0.001). Fat, protein, and casein content had the same behavior with a higher level being found in HAS-1 and IAS-1 groups compared to theI LAS-1 and NAS-1 groups (P < 0.001). On the contrary, an opposite trend was observed for the lactose content (P < 0.001). Among the rheological parameters, the curd firmness (a30) was affected by casein level with the highest value reported for HAS-1 and the lowest for NAS-1 group (P < 0.01).
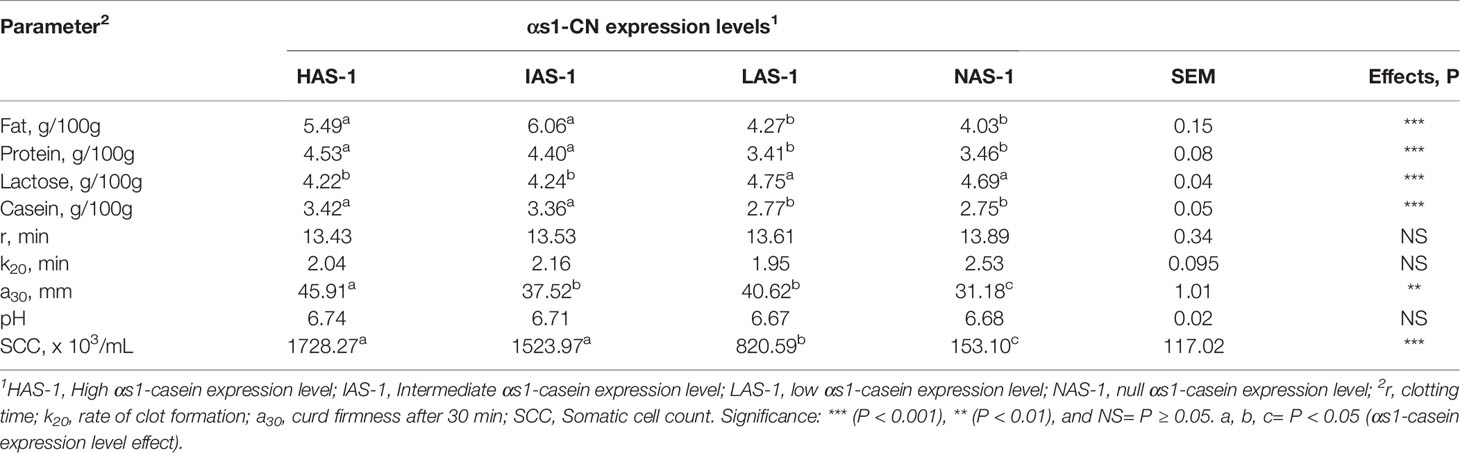
Table 3 Effect of different αs-1 casein expression levels on milk gross composition and clotting parameters.
In regard to the milk indigenous proteolytic enzymes, the activity of plasmin was higher in both HAS-1 and IAS-1 groups compared to the LAS-1 and NAS-1 (P < 0.001, Table 4); whereas, plasmin plasminogen-derived activity exhibited the highest activity in NAS-1 group, being three-fold higher with respect to the other groups (P < 0.001). Consequently, the PG/PL ratio turned out to be the highest in NAS-1; whereas, it was intermediate in LAS-1 and the lowest in HAS-1 and IAS-1 groups (P < 0.01). Finally, the activity of elastase was the highest in LAS-1, intermediate in the IAS-1 group, and the lowest in HAS-1 and NAS-1 groups (P < 0.001).
Discussion
In the Garganica goat, the A, B, C, E, F, N, and 0 alleles were found at the CSN1S1 locus (Albenzio et al., 2009) where the A allele was predominant, followed by the F and B allele. Accordingly, data from the present study confirmed the CSN1S1 alleles and distribution in the goat population was investigated. Moreover, the high frequency of AF genotype evidenced a similarity of the Garganica goat population to the northern European breeds characterized by the high frequency of the low F allele at CSN1S1 locus (Sacchi et al., 2005).
The A* allele could include different alleles associated with high, medium, low, and null levels of as1-CN synthesis, therefore a combined genetic and proteomic approach was useful to obtain information on the effect of αs1-CN fraction on milk characteristics. This study evidenced the effect of αs1-CN expression on gross milk composition parameters. Indeed, the higher levels of fat, protein, and casein content found in HAS-1, IAS-1 groups, with respect to the LAS-1, and NAS-1 groups, were described in previous studies in goat breeds. Some authors (Caravaca et al., 2008) observed a significant effect of the CSN1S1 genotype on the synthesis rate of the corresponding protein. In addition, Mahé et al. (1993) and Manfredi et al. (1995) evidenced the associations between CSN1S1 genotypes and total protein, fat, and casein contents in a French breed. Furthermore, in Saanen goats, AF individuals presented lower milk protein and fat contents with respect to FF individuals, On the contrary, in the Alpine breed, the opposite trend was demonstrated (Manfredi et al., 1995). This contrasting evidence underlines the complex relation between the CSN1S1 genotype and the synthesis level of the main milk components, which can be also influenced by gene-to-gene interaction, environmental, and nutritional factors. In goats, not only the CSN1S1 but also the CSN3 gene was found to be particularly polymorphic, while polymorphisms were described to a lesser extent for CSN1S2 and CSN2 loci (Martin et al., 2002; Moioli et al., 2007). The decreasing levels of SCC passing from the HAS-1 to the NAS-1 may be explained by the major efficiency in milk secretion in goats, characterized by a high level of αS1-CN synthesis that may have led to a major delivery of cellular components in milk through the apocrine secretory mechanism. This phenomenon can be explained by the existence in the goat mammary gland of an apocrine secretory mechanism associated to high cell milk contents, which make difficult the use of SCC as indicators of mammary gland health (Neveu et al., 2002).
Results on milk coagulating characteristics highlighted the complex role of genotype on the mechanism of casein secretion and synthesis, which in turn, rules the ability of caseins to form the protein network in the coagulum. The highest value of the curd firmness found in HAS-1 could be related to the greater milk clotting activity, better inclusion of nutrients in the curd, higher yields of cheese, and more consistent clot reported in goat’s milk from subjects characterized by the presence of strong alleles on αs1-CN, especially AA genotype (Cebo et al., 2012; Bonanno et al., 2013). The authors attributed this result to a better use of food proteins and diets with a high energy content, resulting in a production of milk characterized by larger fat globules and a higher content of fat and proteins. Previous studies (Chanat et al., 1999; Neveu et al., 2002; Akers, 2016) proposed modified transport and secretion processes of milk constituents by mammary epithelial cells, associated with defective as1-CN alleles. Indeed, it was reported that in goats, homozygous for the null allele 0 caseins were more retained in the endoplasmic reticulum of mammary epithelial cells; thus, suggesting that the interaction of CSN1S1 with caseins involved in micelle formation was necessary for efficient transport of these proteins from the endoplasmic reticulum to the Golgi compartment.
The study of indigenous proteolytic systems received minor attention in small ruminants, especially in goat milk. Present data on enzymatic activities detected in Garganica goat milk differed from previous results in which lower levels for the PL-PG system, and higher levels for both elastase and cathepsin D, were found (Santillo et al., 2009). However, the authors referred to bulk milk samples compared to the individual samples involved in the present study. On the contrary, Fantuz et al. (2001) found comparable PL levels (20.04 Units/mL) in individual milk from late-lactating Saanen goat breed. Results on PL activity may be ascribed to the major secretion of αs1-CN in HAS-1 and IAS-1 milk samples leading to a major content of total casein fraction. Plasmin activity is ruled by the presence of casein substrate, which represents the preferred site of protein cleavage (Fox and Kelly, 2006). Indeed, positive correlations were found in the present study between protein and casein content and the corresponding enzyme activity in milk (r = 0.59 P < 0.001 and r = 0.60 P < 0.001 for protein and casein, respectively). On the contrary, the highest level in NAS-1 group of PL and PG-derived activity may be explained by the lower abundance of casein substrate found in milk. A further possible explanation could be the limited conversion of zymogen into its active form. Different mechanisms of conversion of PG to the active enzyme were proposed, among them macrophages can release PAs thus being responsible for the increase of PL activity (Albenzio et al., 2004). It is worth to note that in this study the NAS-1 group presented lower SCC, with respect to the other groups. Previous studies observed a positive correlation between PL and SCC (Albenzio et al., 2015), and negative correlation between PG and SCC (Rebucci et al., 2005). Accordingly, in the present study, positive correlation between PL and SCC (0.27, P < 0.01) and negative correlation between PG and SCC (-0.24 P < 0.05) was found. The higher PG/PL ratio in NAS-1 milk confirmed the lower activation of the principal indigenous proteolytic system. Elastase and cathepsin D activities of the present study confirmed that both enzymes displayed a minor proteolytic activity compared to PL, according to previous reports (Santillo et al., 2009; Moatsou, 2010; Albenzio et al., 2015). Elastase and cathepsin D are mainly present in lysosomes of macrophages and polymorphonuclear leucocytes; therefore, their significance is associated to the mammary gland health status (Albenzio et al., 2009). Notably, IAS-1 and LAS-1 groups displayed higher activity levels of elastase with a concomitant intermediate level of SCC. Accordingly, previous results reported that the highest elastase activity was found in caprine milk with medium SCC level (SCC 701 x 103-1500 x 103 cells mL-1) compared to high and low SCC level (SCC > 1500 x 103 cells mL-1 SCC < 700 x 103 cells mL-1, respectively) (Albenzio et al., 2015).
Conclusion
Garganica goat population involved in the present study showed 7 alleles and 14 genotypes at the CSN1S1 locus; the A allele was the predominant, followed by the F and B allele. The high frequency of AF genotype evidenced a similarity of the Garganica goat population to the northern European breeds. Due to the A* allele including different genetic variants, individual milk samples were grouped according to the level of expression of αs1-CN fraction. Milk chemical composition was affected by the level of expression of αs1-CN fraction, which proved to be linked to the cellular mechanisms, underlying the transport and secretion of milk constituents by the mammary epithelial cells, including fat, protein, and casein content and SCC. Milk composition, in turn, led to ameliorated coagulating properties, in particular, in terms of curd firmness. Plasmin confirmed to be the main contributor to the indigenous proteolytic enzyme pattern in goat milk, with a higher level of activity in correspondence with a higher level of expression of αs1-CN. This last evidence may be significant in the cheese-making characteristic of goat milk, being the plasmin system one of the principal proteolytic agents during cheese ripening.
Data Availability Statement
The raw data supporting the conclusions of this article will be made available by the authors, without undue reservation.
Ethics Statement
Ethical review and approval was not required for the animal study because the study was performed only on milk samples.
Author Contributions
AS and MA conceived the study original design. AS and Fd’A were responsible for sample collection, analytical procedures, data analysis, and wrote the manuscript. MA and MGC revised the manuscript. All authors have read, critically revised, and approved the final version of the manuscript. All authors contributed to the preparation of the manuscript.
Conflict of Interest
The authors declare that the research was conducted in the absence of any commercial or financial relationships that could be construed as a potential conflict of interest.
Publisher’s Note
All claims expressed in this article are solely those of the authors and do not necessarily represent those of their affiliated organizations, or those of the publisher, the editors and the reviewers. Any product that may be evaluated in this article, or claim that may be made by its manufacturer, is not guaranteed or endorsed by the publisher.
References
Akers R. M. (2016). Lactation and the Mammary Gland (Hoboken, New Jersey, U.S.: John Wiley & Sons, Inc.).
Albenzio M., Caroprese M., Marino R., Muscio A., Santillo A., Sevi A. (2006). Characteristics of Garganica Goat Milk and Cacioricotta Cheese. Small Rum. Res. 64, 35–44. doi: 10.1016/j.smallrumres.2005.03.010
Albenzio M., Caroprese M., Santillo A., Marino R., Taibi L., Sevi A. (2004). Effects of Somatic Cell Count and Stage of Lactation on the Plasmin Activity and Cheese-Making Properties of Ewe Milk. J. Dairy Sci. 87, 533–542. doi: 10.3168/jds.S0022-0302(04)73194-X
Albenzio M., Santillo A. (2011). Biochemical Characteristics of Ewe and Goat Milk: Effect on the Quality of Dairy Products. Small Rumin. Res. 101, 33–40. doi: 10.1016/j.smallrumres.2011.09.023
Albenzio M., Santillo A., Avondo M., Nudda A., Chessa S., Pirisi A. (2016). Nutritional Properties of Small Ruminant Food Products and Their Role on Human Health. Small Rumin. Res. 135, 3–12. doi: 10.1016/j.smallrumres.2015.12.016
Albenzio M., Santillo A., d’Angelo F., Sevi A. (2009). Focusing on Casein Gene Cluster and Protein Profile in Garganica Goat Milk. J. Dairy Res. 76, 83–89. doi: 10.1017/S0022029908003853
Albenzio M., Santillo A., Kelly A. L., Caroprese M., Marino R., Sevi A. (2015). Activities of Indigenous Proteolytic Enzymes in Caprine Milk of Different Somatic Cell Counts. J. Dairy Sci. 98 (11), 7587–7594. doi: 10.3168/jds.2015-9762
Bevilacqua C., Martin P., Candalh C., Fauquant J., Piot M., Roucayrol A. M., et al. (2001). Goats’ Milk of Defective αs1-Casein Genotype Decreases Intestinal and Systemic Sensitization to β-Lactoglobulin in Guinea Pigs. J. Dairy Res. 68, 217–227. doi: 10.1017/S0022029901004861
Bonanno A., Di Grigoli A., Montalbano M., Bellina V., Mazza F., Todaro M. (2013). Effects of Diet on Casein and Fatty Acid Profiles of Milk From Goats Differing in Genotype for αs1-Casein Synthesis. Eur. Food Res. Technol. 237, 951–963. doi: 10.1007/s00217-013-2069-8
Caravaca F., Amills M., Jordana J., Angiolillo A., Agüera P., Aranda, et al. (2008). Effect of αs1-Casein (CSN1S1) Genotype on Milk CSN1S1 Content in Malagueña and Murciano-Granadina Goats. J. Dairy Res. 75, 481–484. doi: 10.1017/S0022029908003609
Cebo C., Lopez C., Henry C., Beauvallet C., Ménard O., Bevilacqua C., et al. (2012). Goat αS1-Casein Genotype Affects Milk Fat Globule Physicochemical Properties and the Composition of the Milk Fat Globule Membrane. J. Dairy Sci. 95, 6215–6229. doi: 10.3168/jds.2011-5233
Chanat E., Martin P., Ollivier-Bousquet M. (1999). αs1-Casein is Required for the Efficient Transport of β- and κ-Casein From the Endoplasmic Reticulum to the Golgi Apparatus of Mammary Epithelial Cells. J. Cell Sci. 112, 3399–3412. doi: 10.1242/jcs.112.19.3399
Cosenza G., Illario R., Rando A., Di Gregorio P., Masina P., Ramunno L. (2003). Molecular Characterization of the Goat CSN1S101 Allele. J. Dairy Res. 70 (2), 237–240. doi: 10.1017/S0022029903006101
Cosenza G., Pauciullo A., Gallo D., Colimoro L., D’avino A., Mancusi A., et al. (2008). Genotyping at the CSN1S1 Locus by PCR-RFLP and AS-PCR in a Neapolitan Goat Population. Small Rumin. Res. 74, 84–90. doi: 10.1016/j.smallrumres.2007.03.010
Fantuz F., Polidori F., Cheli F., Baldi A. (2001). Plasminogen Activation System in Goat Milk and its Relation With Composition and Coagulation Properties. J. Dairy Sci. 84, 1786–1790. doi: 10.3168/jds.S0022-0302(01)74616-4
Feligini M., Frati S., Cubric Curik V., Brambilla A., Parma P., Curik I., et al. (2005). Caprine αs1-Casein Polymorphism: Characterization of A, B, E and F Variants by Means of Various Biochemical and Molecular Techniques. Food Technol. Biotechnol. 43 (2), 123–132.
Fox P. F., Kelly A. L. (2006). Indigenous Enzymes in Milk: Overview and Historical Aspects - Part 1. Int. Dairy J. 16, 500–516. doi: 10.1016/j.idairyj.2005.09.013
Kelly A. L., O’Flaherty F., Fox P. F. (2006). Indigenous Proteolytic Enzymes in Milk: A Brief Overview of the Present State of Knowledge. Int. Dairy J. 16, 563–572. doi: 10.1016/j.idairyj.2005.10.019
Mahé M. F., Manfredi E., Ricordeau G., Piacère A., Grosclaude F. (1993). Effects Du Polymorphisme De La Caséine αs1 Caprine Sur Les Performances Laitières: Analyse Intradescendance De Boucs De Race Alpine. Genet. Sel. Evol. 26, 151–157. doi: 10.1186/1297-9686-26-2-151
Manfredi E., Ricordeau G., Barbieri M. E., Amigues Y., Bibé B. (1995). Génotype Caséine αs1 Et Sélection Des Boucs Sur Descendance Dans Les Races Alpine Et Saanen. Genet. Sel. Evol. 27, 451–458. doi: 10.1186/1297-9686-27-5-451
Marshall T. C., Slate J. B. K. E., Kruuk L. E. B., Pemberton J. M. (1998). Statistical Confidence for Likelihood-Based Paternity Inference in Natural Populations. Mol. Ecol. 7 (5), 639–655. doi: 10.1046/j.1365-294x.1998.00374.x
Martin P., Szymanowska M., Zwierzchowski L., Leroux C. (2002). The Impact of Genetic Polymorphisms on the Protein Composition of Ruminant Milks. Reprod. Nutr. Dev. 42, 433–459. doi: 10.1051/rnd:2002036
Moatsou G. (2010). Indigenous Enzymatic Activities in Ovine and Caprine Milks. Intern. J. Dairy Tech. 63 (1), 16–31. doi: 10.1111/j.1471-0307.2009.00552.x
Moioli B., D’Andrea M., Pilla F. (2007). Candidate Genes Affecting Sheepand Goat Milk Quality. Small Rum. Res. 68, 179–192. doi: 10.1016/j.smallrumres.2006.09.008
Neveu C., Riaublanc A., Miranda G., Chich J. F., Martin P. (2002). Is the Apocrine Milk Secretion Process Observed in the Goat Species Rooted in the Perturbation of the Intracellular Transport Mechanism Induced by Defective Alleles at the αS1-Cn Locus? Reprod. Nutr. Dev. 42 (2), 163–172. doi: 10.1051/rnd:2002015
Park Y. W., Juárez M., Ramos M., Haenlein G. F. W. (2007). Physicochemical Characteristics of Goat and Sheep Milk. Small Rumin. Res. 68, 88–113. doi: 10.1016/j.smallrumres.2006.09.013
Ramunno L., Cosenza G., Pappalardo M., Pastore N., Gallo D., Di Gregorio P., et al. (2000). Identification of the Goat CSN1S1F Allele by Means of PCR-RFLP Method. Anim. Genet. 31 (5), 342–342. doi: 10.1046/j.1365-2052.2000.00663.x
Rebucci R., Fusi E., Pecorini C., Pinotti L., Cheli F., Baldi A. (2005). Evaluation of the Biological Activation of Plasmin Plasminogen System in Sheep and Goat Milk. Ital. J. Anim. Sci. 4 (2), 330–332. doi: 10.4081/ijas.2005.2s.330
Sacchi P., Chessa S., Budelli E., Bolla P., Ceriotti G., Soglia D., et al. (2005). Casein Haplotype Structure in Five Italian Goat Breeds. J. Dairy Sci. 88, 1561–1568. doi: 10.3168/jds.S0022-0302(05)72825-3
Santillo A., Kelly A. L., Palermo C., Sevi A., Albenzio M. (2009). Role of Indigenous Enzymes in Proteolysis of Casein in Caprine. Int. Dairy J. 19, 655–660. doi: 10.1016/j.idairyj.2009.06.011
Keywords: goat milk, casein polymorphism, milk chemical composition, clotting properties, proteolytic enzymes
Citation: Santillo A, Ciliberti MG, d’Angelo F and Albenzio M (2022) The Effect of Alpha s1 Genotype on Some Physiological and Chemical Milk Characteristics in Garganica Goat. Front. Anim. Sci. 3:897172. doi: 10.3389/fanim.2022.897172
Received: 15 March 2022; Accepted: 02 May 2022;
Published: 26 May 2022.
Edited by:
Massimo Malacarne, University of Parma, ItalyReviewed by:
Semir Bechir Suheil Gaouar, University of Tlemcen, AlgeriaPaolo Formaggioni, University of Parma, Italy
Copyright © 2022 Santillo, Ciliberti, d’Angelo and Albenzio. This is an open-access article distributed under the terms of the Creative Commons Attribution License (CC BY). The use, distribution or reproduction in other forums is permitted, provided the original author(s) and the copyright owner(s) are credited and that the original publication in this journal is cited, in accordance with accepted academic practice. No use, distribution or reproduction is permitted which does not comply with these terms.
*Correspondence: Antonella Santillo, antonella.santillo@unifg.it