- 1Animal Nutrition Group, Department of Animal Sciences, Wageningen University & Research, Wageningen, Netherlands
- 2Behaviour Ecology Group, Department of Animal Sciences, Wageningen University & Research, Wageningen, Netherlands
Overweight and obesity are common in global pet cat populations which makes it important to understand how properties of food affect appetite (food motivation). In four experiments, we studied this by using a model of operant conditioning for assessing appetite in which cats could press a lever for food rewards. There was no effect of protein status on motivation for protein, when evaluated in a cross-over design with cats receiving low protein (LP) or high protein (HP) foods for 14 days. Cats obtained similar numbers of HP and LP rewards, irrespective of whether their daily food was HP or LP (mixed-effects model, P = 0.550 for food × reward, P = 0.151 for reward). High dietary protein reduced food motivation when we regressed protein levels in 12 commercial foods (12.0 to 27.4 g crude protein/MJ metabolizable energy; P = 0.022) fed for 2 days and tested at 5 h postprandially on the third day whereas fiber levels were without effect (3.8 to 17.8 g non-starch polysaccharides/MJ; P = 0.992). Dietary fiber may reduce appetite depending on its physicochemical properties and we tested the effect of a gelling fiber (alginate), viscous fiber (psyllium) and a fermentable fiber (inulin). Cats received test foods as well as control foods for 3 days and were tested on the third day at 3 h (alginate), 5 h (psyllium) or 8 h (inulin) postprandially. Enriching the food with alginate (P = 0.379) or psyllium (P = 0.153) did not affect the number of rewards obtained, but the feeding of the inulin-enriched food did make the cats obtain fewer rewards than when they received the control food (P = 0.001). Finally, cooking or grinding of dietary meat increased the number of rewards obtained by cats, on day 3 at 3 h postprandial, without evidence for additive effects of these treatments (P = 0.014 for grinding × cooking). This study shows that dietary content of protein or fiber, and the grinding or cooking of meat, all affect appetite in cats as expected, though some predicted effects remained undetected and clearly details regarding food properties matter. These and future findings can guide the designing of foods that promote satiety and prevent over-eating in meal-fed cats.
Introduction
The domestic cat (Felis silvestris catus) has adopted a sedentary lifestyle that is common to pets, with foods readily and superfluous available, and an energy consumption that often exceeds expenditure. Overconsumption, relative to energy expenditure, underlies the development of overweight and obesity, which is unfortunately common in pet cat populations around the world with estimates ranging from 19 to 48% to 4 to 13%, respectively (reviewed in Hoelmkjaer and Bjornvad, 2015). Excess weight is detrimental to the cat's health and quality of life (German, 2006; Hoelmkjaer and Bjornvad, 2015), which makes it important to understand the regulation of food intake in cats and the properties of foods affecting it, as to prevent and remedy overweight in pet cats.
Food intake is regulated by intertwined brain systems that control homeostasis and reward or hedonism (Saper et al., 2002). In this framework, the homeostatic system controls the levels of energy and nutrients within the body, for example by increasing the motivation to eat, and the hedonic system underlies the pleasure of food consumption. The systems together ensure intake of adequate nutrition, i.e. affective states express the rewarding effects of foods (liking) and, consequently, modulate the motivation (wanting) to obtain and select between foods. Protein intake is regulated as such in various animal species (Simpson and Raubenheimer, 1997), and cats target to eat around 50% of the total energy in the form of protein (Hewson-Hughes et al., 2011; Salaun et al., 2017). This is irrespective of moisture content, texture (Hewson-Hughes et al., 2013), and the organoleptic properties (Hewson-Hughes et al., 2016) of foods. Though one study reported a lower protein target of 30% of total energy when foods were standardized for palatability (Hall et al., 2018), cats clearly crave protein and likely have the central control systems for protein liking and wanting as observed in rats (Chaumontet et al., 2018). This predicts strong motivation for high-protein foods when cats have a low protein status, which has not been tested experimentally so far.
Energy and nutrient homeostasis, including food intake, is regulated on the long-term and short-term, like on a meal-to-meal basis. Food consumption changes the motivational state of animals, suppressing appetite and experiencing satiety. The extent and duration of appetite suppression depend on the amount and properties of the food (e.g. see Berthoud, 2008). Per unit of energy, proteins appear more satiating than digestible carbohydrates and fats (Morrison et al., 2012). Fibers (non-digestible carbohydrates) potently promote satiety too, at least in humans (Wanders et al., 2011) and pigs (De Leeuw et al., 2008). This involves various underlying mechanisms (for a review see Bosch et al., 2007) that relate to the formation of gels in the stomach (e.g. alginate; Paxman et al., 2008) and small intestine (e.g. psyllium; Brum et al., 2016) or via the promotion of large intestinal fermentation (e.g. inulin; Korczak and Slavin, 2018). The satiating properties of foods can be increased also by its physical structure. In humans, for example, a meal with ground steamed chicken suppressed hunger less than a meal with the solid equivalent (Martens et al., 2011). Though some studies in cats suggest that fibers increase satiety (Hours et al., 2016), and that protein and fiber levels affect voluntary food intake (Servet et al., 2008), more needs to be known about the impact of food properties on appetite in cats.
We hypothesized that the effects of food properties on appetite described above would also apply to cats and we therefore quantified these effects on appetite (food motivation) in cats, by using a model of operant conditioning in which cats could press a lever for food rewards. In four experiments, we assessed if dietary protein level influences motivation for specifically protein, if dietary protein and fiber levels of commercial foods relate to appetite, if the fibers alginate, psyllium or inulin suppress appetite, and if manipulating the physical structure of food by grinding and/or cooking of meat alters its potential to suppress appetite.
Materials and Methods
The present experimental procedures did not involve animal suffering and were therefore not considered as animal experiments as defined in the Dutch Experiments on Animals Act (2014), as assessed by the Animal Welfare Body of Wageningen University (Wageningen, The Netherlands).
Cats, Housing and Care
The study cats belonged to a colony of 32 adult European short-hair cats at “Carus” research facility (Wageningen University & Research, the Netherlands). Cats were housed in groups of the same sex of up to eight individuals in rooms of 10.4 m2 with access to a fenced outdoor area of 5.4 m2. Usable space was increased with multiple shelves varying in height, and with hammocks and multiple litter boxes and water bowls. Scratching posts, rush matting, pieces of carpet and wood were provided for the deposition of olfactory and visual signals and for claw abrasion. Toys were provided and exchanged on a regular basis. Rooms had daylight and electric lighting was on from 7:00 to 19:00. Cats were fed individually in metabolic cages in the morning and afternoon with a dry extruded food (Perfect Fit™ In-Home, Mars Petcare, Verden, Germany) and at a level that met metabolizable energy (ME) requirements for maintaining weight. Food leftovers were recorded after each meal to calculate the individual food intakes. The cats' health was checked daily by biotechnicians. Body weight and condition were determined before and after the experiments and, for the first experiment, also between the two feeding periods. In the first two experiments, the body condition was scored using the Waltham S.H.A.P.E. system (Alexander et al., 2010), after which the procedures at the facilities changed to the Nestlé PURINA Body Condition System (Laflamme, 1997). Fecal consistency was scored throughout the study, using the Waltham system (Moxham, 2001), and the abdomen of the cats were checked for intestinal upset if caretakes found feces with scores of 4 or higher. Cats were removed from the study to ensure animal welfare when feces consistency remained poor for 2 consecutive days or in the case of bloating. When cats participated in studies, foods and feeding routines were modified according to the study requirements as explained below.
Operant Test Apparatus
The four experiments involved that cats were tested for food motivation, and this was assessed by the number of lever presses cats were willing to make to get a food reward. For this, we used a self-build operant conditioning apparatus (Figure 1; W × D × H: 70 × 81 × 54 cm) that was furnished with a speaker and a red button covered by Perspex lids to create a lever. A hardboard divider (D × H: 30 × 39 cm) separated a second lever, which was not used for the presented work. An Arduino open-source electronics platform enabled sound cues and the scheduling of food rewards in relation to lever pressing, and it recorded the number of presses made by the cats. A stepper motor operated a wheel by which food rewards (kibbles) were dispensed on a small tray located above the lever. Successful presses and food reward dispensing were signaled by sound cue (1,000 Hz for 0.1 s and 2,000 Hz for 0.2 s, respectively). The apparatus was placed in an experimental room that was familiar to the cats and in the same corridor as their living room.
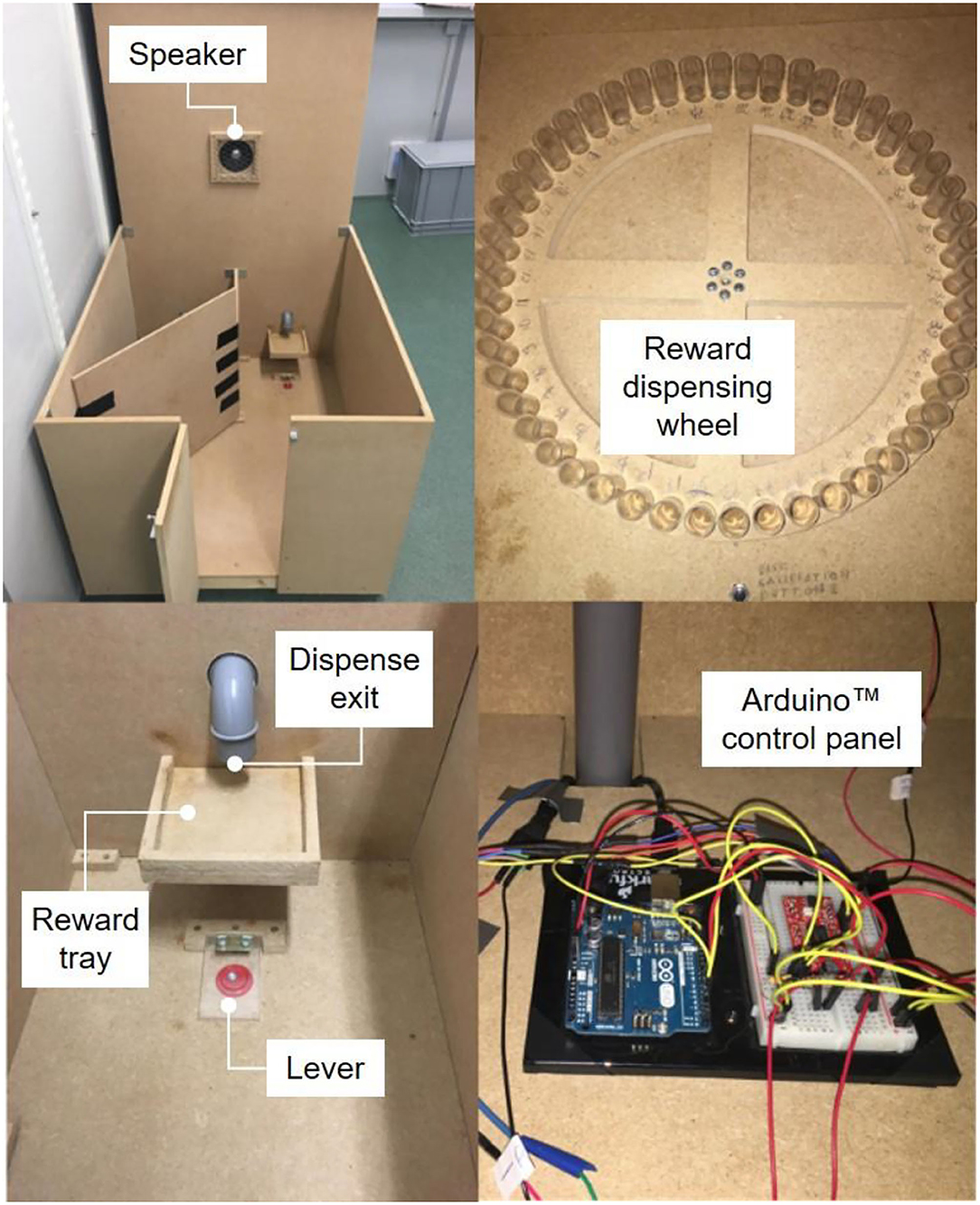
Figure 1. Operant conditioning apparatus for testing food motivation in cats. The speaker emits a sound of 1,000 Hz for 0.1 s when the lever is pressed but no reward is dispensed and a sound of 2,000 Hz for 0.2 s when a food reward (one kibble) is dispensed. The reward is dispensed from the wheel at the top of the apparatus to the reward tray. The Arduino open-source electronics platform enabled the sound cues and scheduling of food rewards (Photo credit: Anqi Li).
Training and Testing
All cats were trained to press the lever for food rewards by means of shaping their behavior via clicker training. Training was tailored to individual performances, meaning that some cats received more training time than others. The test protocols were occasionally tweaked between experiments, but protocols were standardized within experiments. Cats were typically eager to press the lever and were not forced to do so. The cats were tested with a single commodity reinforcement schedule, with workload increasing after each reinforcement (variable ratio). Starting with 1 lever press reinforcement, the number of presses required to obtain successive rewards was increased by 1 or 2, depending on the variability in eagerness to press among cats and on the experiment. The test ended when the maximum time had passed (15 min) or the cats were not motivated anymore to operate the lever and walked away or jumped out from the apparatus for a third time, or showed no intention to press the lever for more than 2 min. We recorded the number of presses, rewards and time intervals.
Experiment 1: Protein Status
Daily foods of low protein (LP) or high protein (HP) were fed to cats that were tested for food motivation by lever pressing for food rewards of HP or LP. The experiment tested if cats with low protein status were more food motivated, especially for high protein rewards. Eight cats (3♂, 5♀; 3.2 to 5.8 years old, 3.0 to 4.1 kg BW, body condition score B to E) were randomly assigned to one of two groups. In a cross-over design, groups were fed either the LP or the HP food for 14 days in period 1 and then switched to the other food for 14 days in period 2. The first 3 days of each period were used to adapt cats to their test food. Cats were fed at maintenance level in two equal meals between 7:30 and 8:30, and between 15:00 and 16:00. The last 6 days of each period were used to measure food motivation once per day at 5 h after their morning meal. The first 3 out of 6 food motivation tests were done with LP rewards and the last 3 with HP rewards, with this order being reversed in period 2. Records for cats consuming <90% of their morning meal on the day of testing were excluded from further analyses, as to minimize the effect of accidental low food intake on measured food motivation.
The daily food was prepared by mixing a commercial wet food that contained 50% ME in protein with pregelatinized corn starch or with acid casein, a liquid palatability enhancer and water (the latter for LP food only). Food portions were weighed, tagged and stored at −18°C until the start of the study. Foods were thawed at room temperature overnight before feeding. Food leftovers were weighed to determine food intake. The rewards that cats could earn by lever pressing were commercial dry extruded kibbles standardized to a weight of 0.15 g/kibble with either a HP or a LP content. Foods and rewards were chemically analyzed for proximate composition and from this the ME contents and protein energy were calculated. Protein energy was 34.7% for the LP food and 71.0% for the HP food and 27.7% for the LP rewards and 40.5% for the HP rewards (Table 1). Statistical analyses were carried out using a mixed-effects model in the PROC MIXED procedure in SAS (version 9.4; SAS Inst. Inc., Cary, NC). The model included the fixed effects of food (LP, HP), reward (LP, HP), and food × reward interaction and period (1, 2) as a random effect. Day of testing was included in the repeated statement and cat within group as subject. Based on the lowest Akaike's information criterion, a first-order autoregressive covariance structure [AR(1)] was selected to account for within-cat variation. Assumed normality of the error term (residuals) was examined using the Shapiro-Wilks test in PROC UNIVARIATE and confirmed.
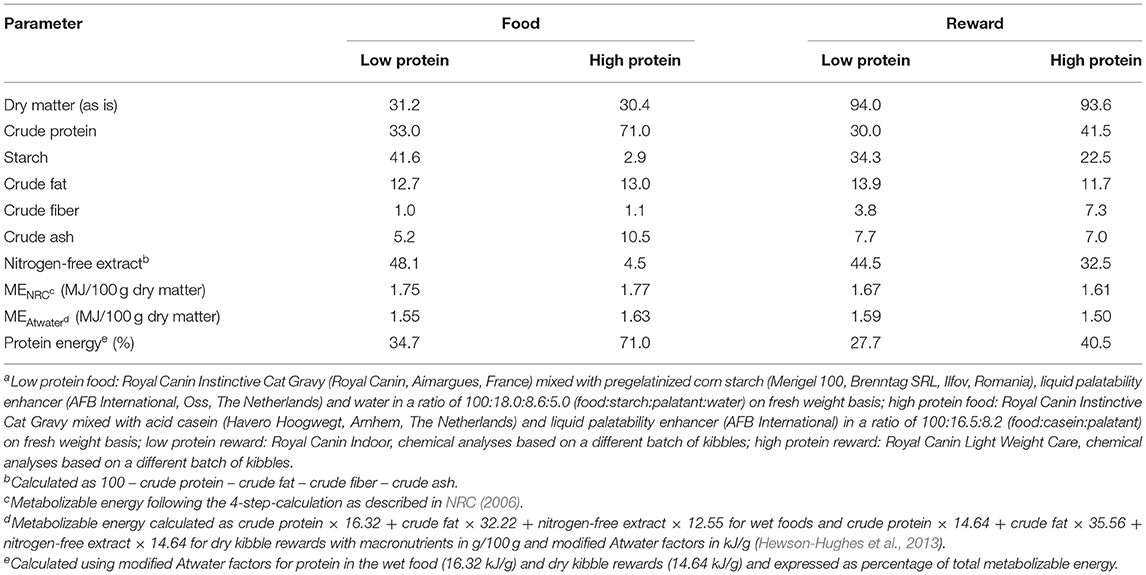
Table 1. Analyzed chemical composition of low and high protein foods and low and high protein kibble rewardsa (g/100 g dry matter unless defined differently).
Experiment 2: Protein and Fiber Levels
Commercial foods were fed to the cats for 3 days and food motivation was assessed on the third day, again using the model of lever pressing for food rewards. Twelve cats (6♂, 6♀; 3.5 to 6.3 years old, 3.5 to 6.2 kg BW, body condition score D to E) were fed each of the 12 foods in a Latin-square design with 12 periods of 3 days. A maximum of four cats could be tested within 1 h. Cats were therefore divided in three groups of four cats, of which the second and third groups started respectively one and two days later than the first group. Cats were fed at maintenance level in two equal meals between 8:00 and 9:00, and between 15:00 and 16:00. Food leftovers in the morning were weighed and added to the afternoon meal. The first 2 days of each period were used to adapt cats to their test food. Food motivation was measured on the last day of each period at 5 h after their morning meal. Rewards were commercial dry extruded kibbles (Perfect Fit™ In-Home). Records for cats consuming <90% of their morning meal on the day of testing were excluded from further analyses.
A dataset was created containing dry cat foods available on the Dutch market. Labeled crude protein and crude fiber contents were expressed per unit of ME as described in NRC (2006). From the 408 foods in the dataset, 12 foods were selected to obtain dietary treatments with graded levels of protein and fiber contents. Foods were chemically analyzed for compositions, and from this the non-starch polysaccharide (NSP) and metabolizable energy (ME) contents were calculated. Foods varied in CP from 12.0 to 26.5 g/MJ ME and in NSP from 3.8 to 17.8 g/MJ ME (Table 2). Three statistical models were implemented, using the PROC MIXED procedure, which included both the protein and NSP contents (in g/MJ ME) as explanatory co-variates (i.e. multiple regression), protein content only and NSP content only. Cat within group and period (1 to 12) were included as random effects in each model. Assumed normality of the error term (residuals) was tested using PROC UNIVARIATE and confirmed.
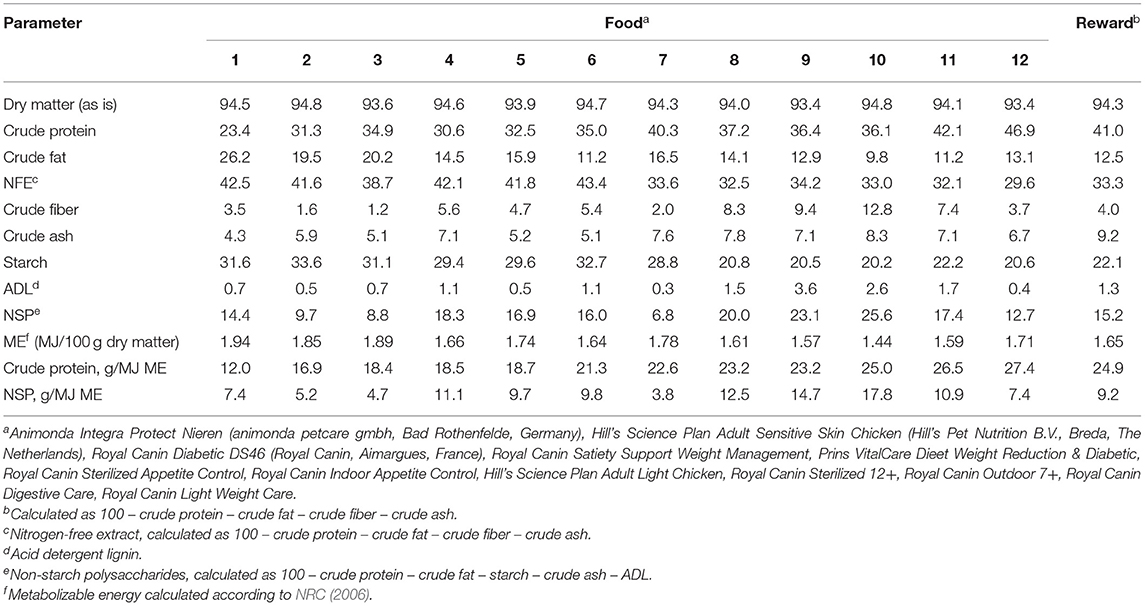
Table 2. Analyzed chemical composition of commercial dry extruded foods (g/100 g dry matter unless defined differently).
Experiment 3: Fiber Types
Dietary fiber content was tested for effects on food motivation following the 3-day procedures as described for the commercial foods. Within an experimental group, each of the 3 fiber treatments were tested against control treatments in a cross-over design with some cats starting with the control treatment and others with the fiber treatment. Each of the two periods included 2 d of adaptation, and the testing for food motivation after the morning meal on day 3. Eight cats (4♂, 4♀; 5.3 to 5.4 years old, 3.6 to 5.7 kg BW, body condition score 5 to 5.5) were assigned to four groups blocked by sex. All groups tested all 3 fiber sources, but in a different order and the fourth group started one day later than the other three groups. Three fiber sources were selected that differed in physicochemical properties and in the ways to suppress appetite, being alginate, psyllium and inulin. Fibers potentially suppress appetite at different moments after the meal, which were assumed to be around 3 h for alginate, 5 h for psyllium and 8 h for inulin based on gastrointestinal transit times in cats. With a gastric half-emptying time of 2.5 to 3.9 h (Wyse et al., 2003; Husnik et al., 2017) and a small intestinal transit time of about 2.5 h (Chandler et al., 1997, 1999), the amount of fiber that would have entered the colon and been fermented might have been insufficient at 5 h after the meal. Food motivation was measured at these time points after the morning meal. Rewards were commercial dry extruded kibbles (Perfect Fit In-Home, Masterfoods, Verden, Germany).
Fiber sources were mixed each morning with a low-fiber wet food (0.02 g crude fiber/100 g dry matter; Table 3) to reach effective dosages for appetite suppression reported in humans [i.e. 0.06 g/kg BW0.75 for alginate, Paxman et al. (2008); 0.36 g/kg BW0.75 for psyllium, Brum et al. (2016); 0.22 g/kg BW0.75 for inulin, Korczak and Slavin (2018)]. Effective dosages in humans were extrapolated to cats on a metabolic BW basis (75 to 4 kg). The mixtures were fed together with a commercial low-fiber content dry food (1.1 g crude fiber/100 g dry matter) in the morning between 7:45 and 8:45 at 40% of the estimated ME requirements. For each fiber-enriched food, a control food was made consisting of the wet food and dry food (Table 3). In the afternoon between 16:45 and 17:45, only the dry food was fed to reach the remaining 60% of the ME requirements. ME contents were calculated based on compositional data on the labels. Food leftover for each cat was recorded to calculate the morning and total intake. Records for cats consuming <90% of their morning meal on the day of testing were excluded from further analyses. Data analyses for each fiber type were carried out using the PROC MIXED procedure with models including the food (control, fiber) as fixed effect and cat within group and period (1, 2) as random effects. Assumed normality of the error term (residuals) was tested using PROC UNIVARIATE and confirmed.
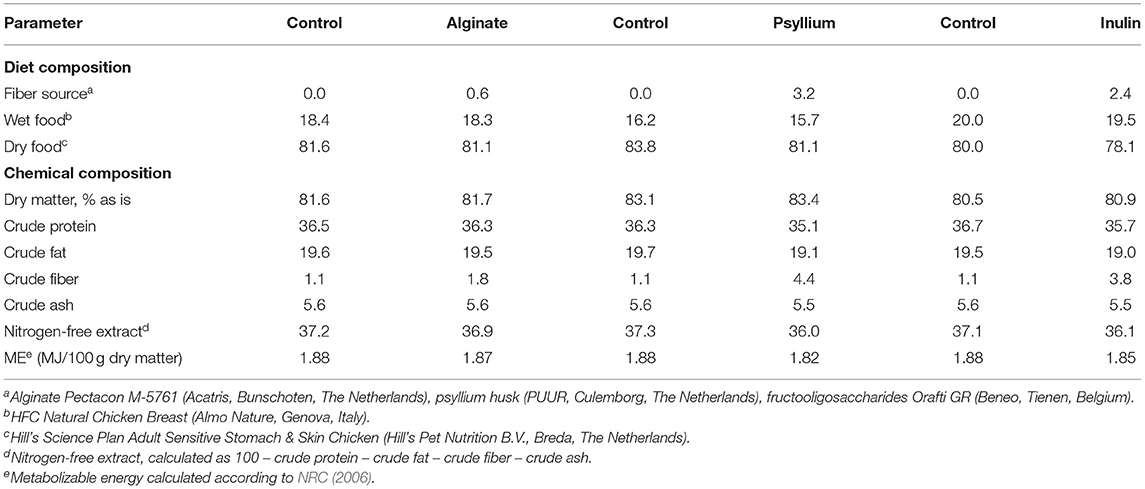
Table 3. Composition (% as is) and calculated chemical composition (g/100 g dry matter unless defined differently) of fiber-enriched foods and their respective control foods fed as morning meal.
Experiment 4: Food Physical Structure
The physical structure of food was altered by grinding and/or cooking of the meat and tested for effects on food motivation following the described 3-day procedures. Eight cats (4♂, 4♀; 5.2 to 5.2 years old, 3.1 to 5.6 kg BW, body condition score 5 to 6) were assigned to four groups of two cats. Groups were fed each of four meats in a Latin-square design with four periods of 3 days. The second, third and fourth groups started respectively one, two and three days later than the first group. Cats were fed at maintenance level in three meals with meats being fed in the morning at 30% of the estimated required energy and the commercial wet food was fed in the early and late afternoon at respectively 20 and 50% of the requirement. To allow food motivation measurements at exactly 3 h after the morning meal, cats within a group were successively fed with the first cat being fed at 8:00, the second at 8:30, etc. In the afternoon the feeding times were 13:00, 13:30, etc. and 16:00, 16:30, etc. Rewards were commercial dry extruded kibbles (Perfect Fit In-Home).
Chicken breast (Pectoralis major) was trimmed from all visible fat and connective tissue. Half of the meat was cut into strips of 1 × 1 × 2 cm (S) and the other half was ground to a particle size ≤ 2 mm (G) using a manual meat grinder with 3 mm die (AMG600 Electric Meat Grinder, Bestron, ‘s-Hertogenbosch, The Netherlands). Half of the meat in strips and half of the ground meat were cooked (C) in zip-lock bags in a water bath at ~75°C for 90 min whereas the other parts remained raw (R). Cooked meats were cooled down to room temperature (~20°C). All water released from the meat after cooking remained in the respective portion. Finally, all the individual food portions were weighed, tagged and stored at −18°C until the start of the study. Metabolizable energy (ME) content of the meat (1.76 MJ/100 g dry matter) was calculated using modified Atwater factors for highly digestible foods (NRC, 2006) and compositional data according to the Dutch food database LEDA (Netherlands Nutrition Centre., 2019). As chicken breast does not provide all nutrients that cats require, a wet canned food (1.87 MJ/100 g dry matter; Whiskas tuna flavor, Masterfoods, Verden, Germany) was also fed to the cats. Meats were thawed at room temperature overnight before feeding. Food leftovers were weighed to determine food intake. The statistical model in PROC MIXED included the fixed effects of grinding (strips, ground), cooking (raw, cooked), and grinding × cooking interaction and cat within group and period (1 to 4) as random effects. Assumed normality of the error term (residuals) was tested using PROC UNIVARIATE and confirmed.
Results and Discussion
Food motivation plays a role in cats becoming overweight and may require a certain amount of control by dietary strategies. To develop such strategies, we need to understand the regulation of food intake by dietary factors and here we studied the effects of dietary content of protein and/or fiber, and the physical properties of dietary meat on food motivation.
Experiment 1: Protein Status
The reward value of protein depends on an individual's internal protein status, at least in rats (Chaumontet et al., 2018), and here we tested this with cats. In a cross-over design, eight cats received a low protein (LP) or a high protein (HP) food for 14 days and, from day 8, were assessed daily for food motivation by lever pressing for HP or LP kibbles. Two female cats were taken out from the study during their second period because of loose feces. One cat had low food consumptions of both foods (99 to 60% of the daily amount, 22% on average for 12 morning meals) and two cats had occasional low consumptions (87 to 84% for three meals; 33% for one meal), which resulted in exclusion of four out of 60 records from the dataset. In the first experimental period cats gained on average (±stdev) 1.3% (±1.9%) in BW (ranging from −0.9 to 4.6%) and in the second period this was 0.9% (±2.5%; −1.8 to 3.4%). In 56 15-min trials, the five cats pressed on average 178 (±84) times per trial to obtain in total 13 (±3) food rewards. Cats obtained similar numbers of HP and LP rewards, irrespective of whether their daily food was HP or LP (mixed-effects model, P = 0.550 for food × reward, P = 0.151 for reward) (Figure 2), which suggests that protein content did not affect satiety after the meal. Proteins are more satiating than digestible carbohydrates, in humans and animal models (Morrison et al., 2012), and the satiating potential of protein was detected in our second experiment (see below). The reward value of protein was unaffected when cats were in a low-protein status, and this did not impact on the general motivation for rewards (food P = 0.324). Our findings are not in line with those in rats where protein-restriction was suggested to induce a reward system–driven appetite for protein (Chaumontet et al., 2018). Relative to feeding rats a normal-protein (NP) diet (14% of energy from protein), feeding a LP diet (6%) for 2 weeks altered gene expressions in the nucleus accumbens that are involved in reward pathways, contribute to food intake behavior and preference for protein when having a choice between HP (55%), NP and LP feeds for 24 h (Chaumontet et al., 2018). We may have missed such effects due to the reduced statistical power as five instead of eight cats finished the study. Future studies can consider lowering the protein content of the LP food to increase the contrasts between treatments and potentially elicit a stronger response as observed in our study.
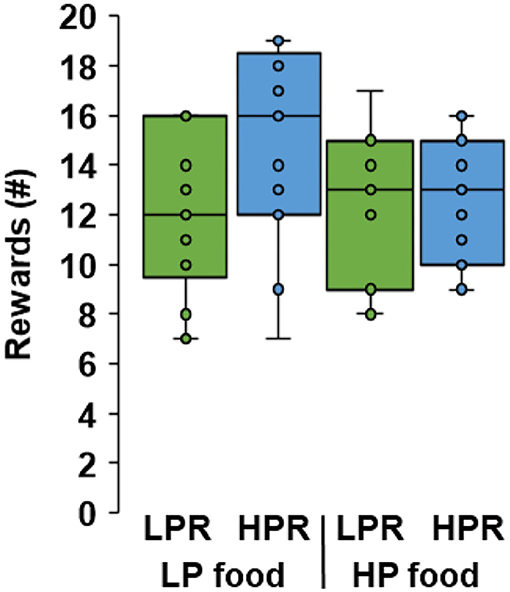
Figure 2. Boxplots for number of rewards obtained by lever pressing for kibbles with low (LPR) or high (HPR) protein content. Cats (n = 5) were adapted to wet foods with a low protein (LP) or a high protein (HP) content and tested for motivation around 5 h after their morning meal. Similar numbers of HP and LP rewards were obtained irrespective of food provided (P = 0.550 for food × reward). Boxes show the interquartile ranges and whiskers show the minimum and maximum values.
Experiment 2: Protein and Fiber Levels
Dietary proteins and fibers promote satiety (Bosch et al., 2007; Morrison et al., 2012) and are thus expected to reduce food motivation. We tested whether protein and fiber levels in 12 commercial foods explained variation in motivation for food. The cats' motivation to lever press for food rewards was assessed 5 h after the morning meals of the third day of being fed a commercial food. Ten out of 12 cats remained healthy throughout the study. One cat was taken out of the study as a precautionary measure in period 10 due to minor signs of bloating and low food intake, and one cat was excluded from further analyses due to substantial leftovers throughout the study (32 out of 36 days). Food intake was 98% of the provided meals or higher for 10 cats and 96% for one cat. The morning meals before the motivation tests were completely consumed for all foods except for foods 9 and 10. For food 10, two cats consumed 94 or 97% of their morning meal whereas for food 9 six cats consumed 98% to 85% of their morning meal with three cats having consumed <90% of the meal provided. These two foods 9 and 10 had highest fiber contents (14.7 and 17.8 g NSP/MJ ME), which may have been the least palatable, although other studies do not report low palatability of commercial high-fiber foods (Servet et al., 2008; Hours et al., 2016). As foods 9 and 10 also had low energy densities (1.57 and 1.44 MJ/100 g DM), meal sizes were on average 8 and 18% larger than for the other foods, which may have promoted stronger feelings of within-meal satiety, limiting meal intake. Over the experimental period during which the cats received the commercial foods they gained on average 1.1% (±1.3%) in BW (ranging from −0.6 to 3.2%) with cats having a BW of 3.2 to 6.1 kg and a body condition score of C to E at the end of the experiment. The 10 cats pressed on average 244 (±239) times in 124 15-min trials to obtain in total 18 (±8) food rewards. The multiple regression analysis of dietary protein (crude protein, CP) and fiber (non-starch polysaccharide, NSP) on the number of food rewards showed that these decreased with increasing protein level at an estimated rate of −0.25 reward per g CP/MJ ME (95%-CI −0.47 to −0.04; P = 0.022) whereas fiber was without effect (0.00 reward per g NSP/MJ ME, −0.27 to 0.27; P = 0.992). Single regression analyses (Figure 3) confirmed this, with slopes being different from 0 for CP level (−0.25, 95%-CI −0.46 to −0.05; P = 0.014) but not for NSP (−0.12, 95%-CI −0.37 to 0.14; P = 0.360). Dietary protein content, in the range of 12.0 to 27.4 g CP/MJ ME, reduced food motivation 5 h postprandial and this satiating potential is in line with findings in humans (Veldhorst et al., 2008; Leidy et al., 2015). The effect was detectable irrespective of the considerable variation among the 12 commercial foods in ingredients and nutrients other than protein and fiber. Increasing protein content promotes satiety, but other aspects of foods high protein (e.g., impacting health) should be considered when developing cat foods. Dietary fiber content, in the range of 3.8 to 17.8 g NSP/MJ ME, did not relate to the cats' food motivation. Fibers promote satiety depending on their physicochemical properties like gelling, viscosity and fermentability (De Leeuw et al., 2008; Wanders et al., 2011), but these properties were not measured in this experiment. It can be speculated that fibers present in the selected commercial foods were merely bulky in nature with little gelling action and impact on digesta viscosity nor being highly fermentable. Moreover, the moment of testing at 5 h after the morning meal may not have been optimal for finding an effect of fermentation. With a gastric half-emptying time of 2.5 to 3.9 h (Wyse et al., 2003; Husnik et al., 2017) and a small intestinal transit time of about 2.5 h (Chandler et al., 1997, 1999), the amount of fiber that would have entered the colon and been fermented might have been insufficient at 5 h after the meal. Cat foods designed to aid in weight management generally have high protein and fiber levels (German, 2016). In dogs, this combination was more effective in stimulating satiety than high-protein only (Weber et al., 2007). It cannot be excluded that this is different for cats and that protein levels are in particular important to promote satiety, at least when cats are fed restrictively. The application of these findings to cats fed unrestricted amounts of food, however, is limited as voluntary intake can actually be increased when protein levels are high (Wei et al., 2011). This may be controlled by decreasing protein levels and increasing fiber levels (Servet et al., 2008). This experiment did not demonstrate an effect of fiber level on satiety, which may in part have been due to the specific fiber types as evident from the next experiment.
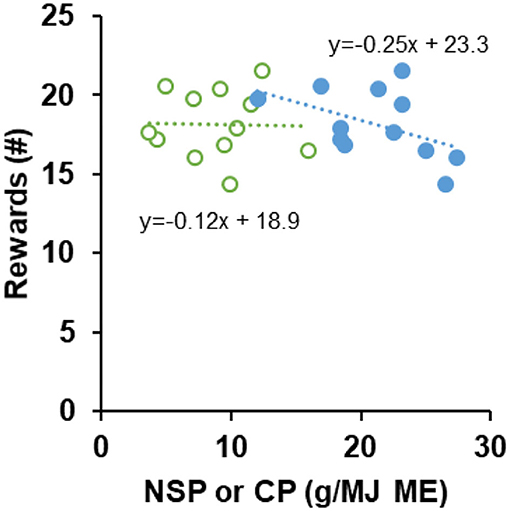
Figure 3. Number of rewards obtained by lever pressing for kibbles. Cats (n = 10 to 11) were adapted to dry extruded foods with differing levels of non-starch polysaccharides (NSP, green open markers) and crude protein (CP, blue filled markers) and tested for motivation around 5 h after their morning meal. Slopes of regression lines were different from 0 for protein level (P = 0.014) but not for NSP level (P = 0.360).
Experiment 3: Fiber Types
Fibers vary in their potential to prolong satiety depending on their physicochemical properties (De Leeuw et al., 2008; Wanders et al., 2011). We tested the effect of a gelling fiber (alginate), viscous fiber (psyllium) and a fermentable fiber (inulin) on food motivation in cats. The cats received test foods as well as control foods for 3 days and pressed lever for food rewards at 3 h (alginate), 5 h (psyllium) or 8 h (inulin) after the morning meal on the third day. All cats remained healthy throughout the study. Food intake was 99% or higher, which also included the morning meals before the food motivation tests. On average, cats lost 1.5% (±2.1%) of their BW (−5.1 to 1.8%) and had a BW between 3.4 and 5.8 kg and body condition score between 4.5 and 5 at the end of the experiment. The eight cats pressed on average 188 (±132) times in 48 15-min trials to obtain in total 13 (±5) food rewards. Enriching the food with alginate or psyllium did not affect the number of rewards that cats obtained (P = 0.379 and P = 0.153, respectively). The feeding of the inulin-enriched food did result in fewer rewards obtained than when cats received the control food (P = 0.001; Figure 4). In humans, similar levels of dietary alginate and psyllium suppressed, respectively, energy intake (Paxman et al., 2008) and hunger (Brum et al., 2016). The present lack of effect of alginate and psyllium on food motivation may be explained by species differences in digestive physiology and appetite regulation, levels of effective dosages and timing of testing for appetite. For alginate, the effectiveness to gel in the stomach of the cats should be considered. We mixed the alginate with only a small amount of wet food and fed it together with a dry food. Liquids are required to elicit gelling of alginate, but the liquid does not necessarily need to come from the food. A dry pelleted feed with 5% alginate was effective in gelling stomach digesta in growing pigs (Souza da Silva, 2013), which was mixed with gastric juice and/or drinking water. It remains speculative to what degree alginate was successful in creating a strong gel matrix in our cats, and in slowing stomach emptying of the complete food mixture. The alginate was mixed with the wet food and then fed together with the dry food. If alginate only gelled the wet food without impacting the dry food in the stomach, the resulting contrasts in gastric distention and emptying would have been smaller than intended. Furthermore, at the moment of testing at 3 h after the morning meal, the difference between treatments in stomach distention and delayed nutrient absorption due to entrapment in the gel matrix might have been too small to create a contrast in feelings of satiety. An increase in digesta viscosity by adding psyllium did also not affect the cats' food motivation at 5 h postprandially. The timing of measuring food motivation is not expected to play an overly strong role in this. Psyllium reduced appetite in human, relative to the placebo, and this was retained from shortly after the meal until the next meal 4.5 h later (Brum et al., 2016). The effects of dietary fibers on satiety in cats seem relatively subtle and subject to a range of modulating factors that are in part well-established, like physicochemical properties, whereas other factors are less clear. Having multiple measurements for a dietary treatment for each cat could reduce the variability and increase the resolution to reveal potentially more subtle satiating effects that alginate or psyllium could promote. Dietary fermentable fibers reduced appetite in various species including humans (Korczak and Slavin, 2018), dogs (Bosch et al., 2009a,b) and pigs (Souza da Silva et al., 2012) and various underlying appetite-regulating mechanisms have been described (for a review see e.g. Bosch et al., 2007). The large intestine of cats is relatively short and non-sacculated, but it hosts an active microbial community that is capable of fermenting fibers, including inulin (Sunvold et al., 1995; Bosch et al., 2017), and potentially stimulating mechanisms that promote satiety. The supplemented inulin was indeed effective in reducing motivation for food in cats measured 8 h after the morning meal. Fermentable fibers differ in their rate of fermentation as well as in the fermentation products formed (Bosch et al., 2017), and findings like ours do not necessarily apply across different fermentable fibers. This is open to further research and it would be interesting to study the effectivity of different fermentable fibers in reducing voluntary food intake in cats that are fed unrestricted amounts of food.
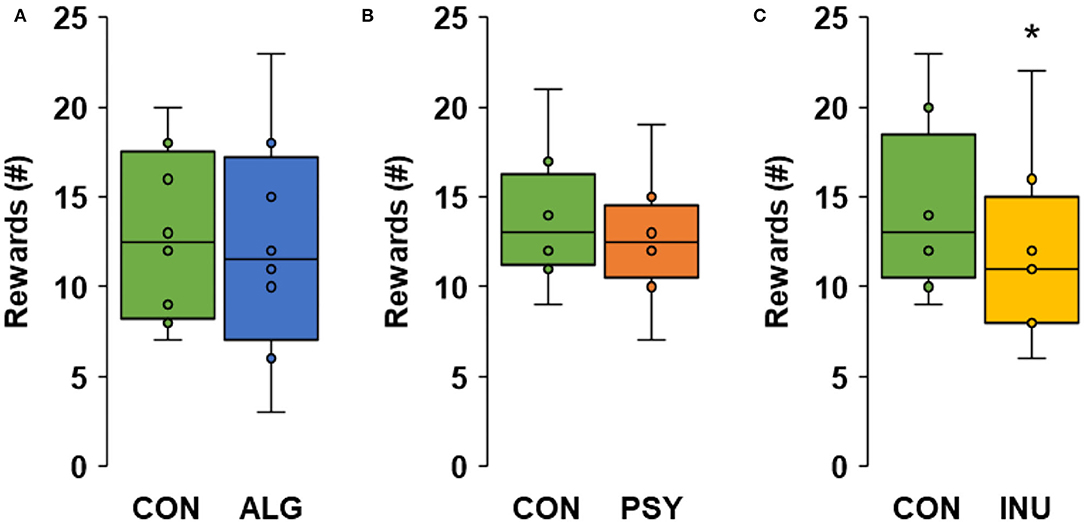
Figure 4. Boxplots for the number of rewards obtained by lever pressing of cats (n = 8) after a morning meal without (CON) or with alginate [ALG; test at 3 h post meal; (A)], psyllium [PSY; 5 h; (B)] or inulin [INU; 8 h; (C)]. Boxes show the interquartile ranges, whiskers show the minimum and maximum values, and the asterisk indicates difference from control (P = 0.001).
Experiment 4: Food Physical Structure
The physical structure of food regulates appetite in humans (Martens et al., 2011) and we tested whether in cats the cooking and grinding of dietary meat changes its satiating properties. All eight cats remained healthy throughout the experiment, they received the test foods for 3 days and were assessed for food motivation at 3 h after the morning meal on the third day. Food intake was complete, including the morning meals before the motivation tests. On average, cats gained 4.9% (±4.4%) of their BW (-0.4 to 10.6%; 3.1 to 6.0 kg BW, body condition score 5 to 6). Cats obtained only few rewards by lever pressing when the meat in their daily diet was raw and in strips (P = 0.014 for grinding × cooking; Figure 5). All other foods, with the meat cooked and/or ground, resulted in similarly stronger food motivation, without evidence for additive effects. The digestive properties of the meat changes with cooking and grinding in a way that it facilitates digestion of nutrients through chemical changes like denaturation and physical changes like increased surface area. The accelerated digestion of processed meat showed in a decreased diet-induced thermogenesis (i.e. energetic costs for meal digestion and assimilation; Secor, 2009) when it was cooked (12.4%), ground (12.7%) or cooked and ground (23.4%), and fed to Burmese pythons (Python molurus) (Boback et al., 2007). The additive effect of the combination of cooking and grinding on the speed of digestion in pythons did not show in the food motivation of our cats, which might relate to species differences in digestive physiology, type of meats used, or degree of grinding. We did not observe an effect of cooked meat provided as strips or ground, as it was observed in human subjects who consumed a piece of cooked meat with a glass of water or had the meat ground and liquified in water (Martens et al., 2011). Such liquified meat will have a more rapid passage through the stomach than our cooked meat that was ground to a particle size ≤ 2 mm and fed as a solid product. Our finding that cooking as well as grinding reduced the satiating potential of meat for cats is in particular relevant for the processing of ingredients and complete foods. Commercial cat foods typically contain ingredients that are finely ground and heated to improve the properties of the final product, including safety and shelf-life. Such processing treatments would make nutrients easier digestible, but potentially with unfavorable effects on the satiating properties of the food. Such a relationship has not been studied in cats to date and research is warranted in which the digestion kinetics of nutrients in ingredients and foods are manipulated and tested for their impact on satiety and energy intake in cats.
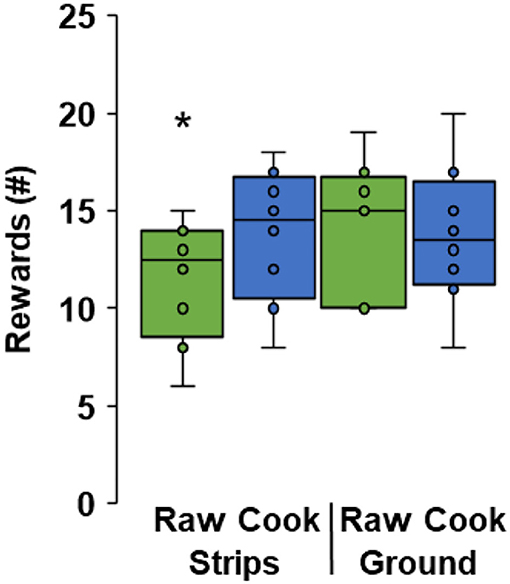
Figure 5. Boxplots for the number of rewards obtained by lever pressing for kibbles. Cats (n = 8) were adapted to raw or cooked meat fed in strips (1 × 1 × 2 cm) or ground (particle size ≤ 2 mm) and tested for food motivation 3 h after their morning meal. Boxes show the interquartile ranges, whiskers show the minimum and maximum values, and the asterisk indicates difference between raw stripped meat and other treatments (P = 0.014 for grinding × cooking).
Conclusions
Dietary protein content, supplementation of inulin and the grinding or cooking of meat, all affected food motivation in cats as expected, though some other expected effects of fiber and protein remained undetected. We showed that different food properties impact on motivation for food, as quantified with a model of operant conditioning, and such findings can guide the designing of cat foods that aim to promote satiety in meal-fed cats. High dietary protein levels in commercial foods were associated with reduced motivation for food and supplementing food with inulin reduced food motivation 8 h after the meal. The physical structure of food is also relevant in appetite regulation with either cooking or grinding already reducing the satiating property of meat. Unexpectedly, motivation for protein-rich rewards was not changed by dietary protein level and food motivation was not affected by the fiber content of commercial foods nor by the dietary fibers alginate and psyllium. Limitations in the resolution of our measuring methods could play a role, and the unexpected outcomes warrant further study to elucidate how food properties affect voluntary food intake and energy balance in cats.
Data Availability Statement
The raw data supporting the conclusions of this article will be made available by the authors, without undue reservation.
Ethics Statement
The animal study was reviewed and approved by Animal Welfare Body of Wageningen University.
Author Contributions
GB and BB contributed to conception of the studies. GB, BB, and MG contributed to the design of the experiments. GB performed the statistical analyses and wrote the initial manuscript draft. BB and MG reviewed and edited the manuscript. All authors contributed to manuscript revision, read, and approved the submitted version.
Funding
This research was funded by Wageningen University & Research.
Conflict of Interest
The authors declare that the research was conducted in the absence of any commercial or financial relationships that could be construed as a potential conflict of interest.
Publisher's Note
All claims expressed in this article are solely those of the authors and do not necessarily represent those of their affiliated organizations, or those of the publisher, the editors and the reviewers. Any product that may be evaluated in this article, or claim that may be made by its manufacturer, is not guaranteed or endorsed by the publisher.
Acknowledgments
We greatly acknowledge the efforts of Joyce Wuister, Mathias Cox, Anika Evertse, Carlijn de Groot, Anqi Li, Hu Zhenpeng, Diana Jaramillo Cherres, and Tianrui Xia in co-developing the used materials and methods as well as performing the studies and collecting data.
References
Alexander, L., Buckley, C., Merrill, R., Morris, P. J., and Stevenson, A. (2010). WALTHAM Pocket Book of Healthy Weight Maintenance for Cats and Dogs. London: Beyond Design Solutions Ltd.
Berthoud, H. R. (2008). Vagal and hormonal gut-brain communication: from satiation to satisfaction. Neurogastroent. Motil. 20, S64–S72. doi: 10.1111/j.1365-2982.2008.01104.x
Boback, S. M., Cox, C. L., Ott, B. D., Carmody, R., Wrangham, R. W., and Secor, S. M. (2007). Cooking and grinding reduces the cost of meat digestion. Comp. Biochem. Physiol. A. 148, 651–656. doi: 10.1016/j.cbpa.2007.08.014
Bosch, G., Beerda, B., Hendriks, W. H., van der Poel, A. F. B., and Verstegen, M. W. A. (2007). Impact of nutrition on canine behaviour: current status and possible mechanisms. Nutr. Res. Rev. 20, 180–194. doi: 10.1017/S095442240781331X
Bosch, G., Beerda, B., van de Hoek, E., Hesta, M., van der Poel, A. F. B., Janssens, G. P. J., et al. (2009a). Effect of dietary fibre type on physical activity and behaviour in kennelled dogs. Appl. Anim. Behav. Sci. 121, 32–41. doi: 10.1016/j.applanim.2009.08.001
Bosch, G., Heesen, L., de Melo Santos, K., Cone, J. W., Pellikaan, W. F., and Hendriks, W. H. (2017). Evaluation of an in vitro fibre fermentation method using feline faecal inocula: inter-individual variation. J. Nutr. Sci. 6, e24. doi: 10.1017/jns.2017.21
Bosch, G., Verbrugghe, A., Hesta, M., Holst, J. J., van der Poel, A. F. B., Janssens, G. P. J., et al. (2009b). The effects of dietary fibre type on satiety-related hormones and voluntary food intake in dogs. Br. J. Nutr. 102, 318–325. doi: 10.1017/S0007114508149194
Brum, J. M., Gibb, R. D., Peters, J. C., and Mattes, R. D. (2016). Satiety effects of psyllium in healthy volunteers. Appetite. 105, 27–36. doi: 10.1016/j.appet.2016.04.041
Chandler, M. L., Guilford, W. G., and Lawoko, C. R. O. (1997). Radiopaque markers to evaluate gastric emptying and small intestinal transit time in healthy cats. J. Vet. Intern. Med. 11, 361–364. doi: 10.1111/j.1939-1676.1997.tb00481.x
Chandler, M. L., Guilford, W. G., Lawoko, C. R. O., and Whittem, T. (1999). Gastric emptying and intestinal transit times of radiopaque markers in cats fed a high-fiber diet with and without low-dose intravenous diazepam. Vet. Radiol. Ultrasoun. 40, 3–8. doi: 10.1111/j.1740-8261.1999.tb01831.x
Chaumontet, C., Recio, I., Fromentin, G., Benoit, S., Piedcoq, J., Darcel, N., et al. (2018). The protein status of rats affects the rewarding value of meals due to their protein content. J. Nutr. 148, 989–998. doi: 10.1093/jn/nxy060
De Leeuw, J. A., Bolhuis, E. J., Bosch, G., and Gerrits, W. J. J. (2008). Effects of dietary fibre on behaviour and satiety in pigs. Proc. Nutr. Soc. 67:334–342. doi: 10.1017/S002966510800863X
German, A. J. (2006). The growing problem of obesity in dogs and cats. J. Nutr. 136, S1940–S1946. doi: 10.1093/jn/136.7.1940S
German, A. J. (2016). Weight management in obese pets: the tailoring concept and how it can improve results. Acta Vet. Scand. 58, 57. doi: 10.1186/s13028-016-0238-z
Hall, J. A., Vondran, J. C., Vanchina, M. A., and Jewell, D. E. (2018). When fed foods with similar palatability, healthy adult dogs and cats choose different macronutrient compositions. J. Exp. Biol. 221, jeb173450. doi: 10.1242/jeb.173450
Hewson-Hughes, A. K., Colyer, A., Simpson, S. J., and Raubenheimer, D. (2016). Balancing macronutrient intake in a mammalian carnivore: disentangling the influences of flavour and nutrition. R. Soc. Open Sci. 3, 160081. doi: 10.1098/rsos.160081
Hewson-Hughes, A. K., Hewson-Hughes, V. L., Colyer, A., Miller, A. T., Hall, S. R., Raubenheimer, D., et al. (2013). Consistent proportional macronutrient intake selected by adult domestic cats (Felis catus) despite variations in macronutrient and moisture content of foods offered. J. Comp. Physiol. B. 183, 525–536. doi: 10.1007/s00360-012-0727-y
Hewson-Hughes, A. K., Hewson-Hughes, V. L., Miller, A. T., Hall, S. R., Simpson, S. J., and Raubenheimer, D. (2011). Geometric analysis of macronutrient selection in the adult domestic cat, felis catus. J. Exp. Biol. 214, 1039–1041. doi: 10.1242/jeb.049429
Hoelmkjaer, K. M., and Bjornvad, C. R. (2015). Management of obesity in cats. Vet. Med. 5, 97–107. doi: 10.2147/VMRR.S40869
Hours, M. A., Sagols, E., Junien-Castagna, A., Feugier, A., Moniot, D., Daniel, I., et al. (2016). Comparison of voluntary food intake and palatability of commercial weight loss diets in healthy dogs and cats. BMC Vet. Res. 12, 274. doi: 10.1186/s12917-016-0899-x
Husnik, R., Fletcher, J. M., Gaschen, L., and Gaschen, F. P. (2017). Validation of ultrasonography for assessment of gastric emptying time in healthy cats by radionuclide scintigraphy. J. Vet. Intern. Med. 31, 394–401. doi: 10.1111/jvim.14674
Korczak, R., and Slavin, J. L. (2018). Fructooligosaccharides and appetite. Curr. Opin. Clin. Nutr. Metab. Care 21, 377–380. doi: 10.1097/MCO.0000000000000502
Laflamme, D. P. (1997). Development and validation of a body condition system for cats: a clinical tool. Feline Pract. 25, 13–17.
Leidy, H. J., Clifton, P. M., Astrup, A., Wycherley, T. P., Westerterp-Plantenga, M. S., Luscombe-Marsh, N. D., et al. (2015). The role of protein in weight loss and maintenance. Am. J. Clin. Nutr. 101, S1320–1329. doi: 10.3945/ajcn.114.084038
Martens, M. J. I., Lemmens, S. G. T., Born, J. M., and Westerterp-Plantenga, M. S. (2011). A solid high-protein meal evokes stronger hunger suppression than a liquefied high-protein meal. Obesity 19, 522–527. doi: 10.1038/oby.2010.258
Morrison, C. D., Reed, S. D., and Henagan, T. M. (2012). Homeostatic regulation of protein intake: in search of a mechanism. Am. J. Physiol. Regul. Integr. Comp. Physiol. 302, R917–928. doi: 10.1152/ajpregu.00609.2011
Moxham, G. (2001). WALTHAM feces scoring system - A tool for veterinarians and pet owners: how does your pet rate? WALTHAM Focus 11, 24–25. doi: 10.1111/j.1939-1676.2008.0204.x
Netherlands Nutrition Centre. (2019). Dutch Food Database LEDA (Levensmiddelendatabank). The Hague: Netherlands Nutrition Centre.
Paxman, J. R., Richardson, J. C., Dettmar, P. W., and Corfe, B. M. (2008). Daily ingestion of alginate reduces energy intake in free-living subjects. Appetite 51, 713–719. doi: 10.1016/j.appet.2008.06.013
Salaun, F., Blanchard, G., Le Paih, L., and Niceron, C. (2017). Impact of macronutrient composition and palatability in wet diets on food selection in cats. J. Anim. Physiol. Anim. Nutr. 101, 320–328. doi: 10.1111/jpn.12542
Saper, C. B., Chou, T. C., and Elmquist, J. K. (2002). The need to feed: homeostatic and hedonic control of eating. Neuron 36, 199–211. doi: 10.1016/S0896-6273(02)00969-8
Secor, S. M. (2009). Specific dynamic action: a review of the postprandial metabolic response. J. Comp. Physiol. B. 179, 1–56. doi: 10.1007/s00360-008-0283-7
Servet, E., Soulard, Y., Venet, C., Biourge, V., and German, A. J. (2008). Ability of diets to generate ‘satiety' in cats. J. Vet. Intern. Med. 22, 1482.
Simpson, S. J., and Raubenheimer, D. (1997). Integrative models of nutrient balancing: application to insects and vertebrates. Nutr. Res. Rev. 10, 151–179. doi: 10.1079/NRR19970009
Souza da Silva, C. (2013). Fermentation in the Gut to Prolong Satiety. Wageningen: Wageningen University.
Souza da Silva, C., Van den Borne, J. J. G. C., Gerrits, W. J. J., Kemp, B., and Bolhuis, E. J. (2012). Effects of dietary fibers with different physicochemical properties on feeding motivation in adult female pigs. Physiol. Behav. 107, 218–230. doi: 10.1016/j.physbeh.2012.07.001
Sunvold, G. D., Hussein, H. S., Fahey Jr, G. C., Merchen, N. R., and Reinhart, G. A. (1995). In vitro fermentation of cellulose, beet pulp, citrus pulp, and citrus pectin using fecal inoculum from cats, dogs, horses, humans, and pigs and ruminal fluid from cattle. J. Anim. Sci. 73, 3639–3648. doi: 10.2527/1995.73123639x
Veldhorst, M., Smeets, A., Soenen, S., Hochstenbach-Waelen, A., Hursel, R., Diepvens, K., et al. (2008). Protein-induced satiety: effects and mechanisms of different proteins. Physiol. Behav. 94, 300–307. doi: 10.1016/j.physbeh.2008.01.003
Wanders, A. J., van den Borne, J. J. G. C., de Graaf, C., Hulshof, T., Jonathan, M. C., Kristensen, M., et al. (2011). Effects of dietary fibre on subjective appetite, energy intake and body weight: a systematic review of randomized controlled trials. Obes Rev. 12, 724–739. doi: 10.1111/j.1467-789X.2011.00895.x
Weber, M., Bissot, T., Servet, E., Sergheraert, R., Biourge, V., and German, A. J. (2007). A high-protein, high-fiber diet designed for weight loss improves satiety in dogs. J. Vet. Intern. Med. 21, 1203–1208. doi: 10.1111/j.1939-1676.2007.tb01939.x
Wei, A., Fascetti, A. J., Liu, K. J., Villaverde, C., Green, A. S., Manzanilla, E. G., et al. (2011). Influence of a high-protein diet on energy balance in obese cats allowed ad libitum access to food. J. Anim. Physiol. Anim. Nutr. 95, 359–367. doi: 10.1111/j.1439-0396.2010.01062.x
Keywords: fiber, food motivation, food structure, operant conditioning, protein, satiety
Citation: Bosch G, Gilbert M and Beerda B (2022) Properties of Foods That Impact Appetite Regulation in Cats. Front. Anim. Sci. 3:873924. doi: 10.3389/fanim.2022.873924
Received: 11 February 2022; Accepted: 23 March 2022;
Published: 14 April 2022.
Edited by:
Anna Katharine Shoveller, University of Guelph, CanadaReviewed by:
Maria Cattai De Godoy, University of Illinois at Urbana-Champaign, United StatesEmma Natasha Bermingham, AgResearch Ltd, New Zealand
Copyright © 2022 Bosch, Gilbert and Beerda. This is an open-access article distributed under the terms of the Creative Commons Attribution License (CC BY). The use, distribution or reproduction in other forums is permitted, provided the original author(s) and the copyright owner(s) are credited and that the original publication in this journal is cited, in accordance with accepted academic practice. No use, distribution or reproduction is permitted which does not comply with these terms.
*Correspondence: Guido Bosch, guido.bosch@wur.nl