- 1Department of Veterinary Medicine, University of Bari Aldo Moro, Valenzano, Italy
- 2Medical Statistics, Department of Biomedical Science and Human Oncology, University of Bari, Bari, Italy
- 3Department of Agriculture and Forest Sciences (DAFNE), University of Tuscia, Viterbo, Italy
- 4Italian Brown Breeders Association, Bussolengo, Italy
Climate change is impacting worldwide efficiency and welfare standards in livestock production systems. Considering the sensibility to heat stress reported for different milk production patterns in Italian Brown Swiss, this study aims to evaluate the effect of heat waves (HWs)of different lengths on some milk production traits (fat-corrected milk, energy-corrected milk, protein and fat yield, protein percentage, cheese production at 24 h, and cheese yield). A 10-year dataset (2009–2018), containing 202,776 test-day records from 23,296 Brown Swiss cows, was used. The dataset was merged both with the daily maximum temperature–humidity index (THI) recorded by weather stations and with the daily maximum THI threshold for each trait in Italian Brown Swiss cows. The study considered 4 different HWs according to their length: 2, 3, 4, and 5 consecutive days before the test-day over the weighted THI threshold. Milk production traits were determined as the difference in losses compared to those after only 1 day before the test-day over the weighted maximum THI. All traits showed to be affected by HWs. Particularly, protein percentage losses increased from −0.047% to −0.070% after 2 consecutive days over the daily THI threshold, reaching −0.10% to −0.14% after 5 days (p < 0.01), showing a worsening trend with the increasing length of HWs. First parity cows showed to be more sensitive to HWs than other parity classes, recording greater losses after shorter HWs, compared to multiparous cows, for protein yield and, consequently, for cheese production at 24 h. This suggests a less efficient metabolic response to heat stress and exposure time in primiparous, compared to multiparous cows, probably due to their incomplete growth process that overlaps milk production, making it more difficult for them to dissipate heat. Although actions to mitigate heat stress are always needed in livestock, this study points out that often time exposure to warm periods worsens milk production traits in Brown Swiss cows.
Introduction
The current and forecasted future scenario on global warming leads animal scientists to focus on several challenges on livestock efficiency, animal welfare, and economic perspective. Several studies stated that prolonged periods of excessively hot weather, such as heat waves (HWs), are expected to increase in frequency, intensity, and duration (Beniston et al., 2007; Vitali et al., 2020). Comprehensive knowledge and analysis of the effects of this phenomenon on animal efficiency is the basis for improving future livestock systems' efficiency. Dairy cows have been considered heat stressed when their capacity for heat dissipation exceeds the range specified for normal activity (Pinto et al., 2020). High-yielding cows are the most discharged due to the production of a large amount of metabolic heat (Godyń et al., 2019). The activation of adaptive systems (Kadzere et al., 2002), in these animals, are not able to cope with the high temperature and high relative humidity, resulting in cow's body temperature increase (Allen et al., 2015), impaired thermoregulation, and excessive heat load (Rhoads et al., 2009). Consequently, the supposed climate scenario may have some negative consequences from a different point of view: health, welfare, and overall efficiency. Heat stress increases the risk of production losses (Bernabucci et al., 2014; Dahl et al., 2019; Maggiolino et al., 2020), infections (Olde Riekerink et al., 2007), and mortality (Vitali et al., 2015).
For the estimation of heat stress effects on dairy cows, many indices have been proposed in the literature (Herbut, 2018). The most suitable in intensive systems is the temperature–humidity index (THI) (Hammami et al., 2013; Heinicke et al., 2018), a combination of dry bulb temperature and relative humidity (Maggiolino et al., 2020). The THI thresholds are suitable for estimating the intensity of thermal discomfort, and different thresholds in different breeds by different research were proposed. Commonly, THI of 72 is considered the upper limit threshold in Holstein cows, after which the milk production starts to decrease (Heinicke et al., 2018). Mader et al. (2006) defined a range of THI between 70 and 74 to be a potential heat stress condition for cattle. Many studies showed considerable variability in THI thresholds regarding production losses such as THI 72 (Ravagnolo et al., 2000; Bohmanova et al., 2007), THI 68 (Herbut et al., 2015), THI 65 (Zimbelman and Collier, 2011), and THI 60 (Brügemann et al., 2012). Moreover, Maggiolino et al. (2020) reported higher THI thresholds for Brown Swiss cows, showing higher heat tolerance of this breed. Although several studies have been carried out on different breeds and in a different geographical area, aiming to estimate the THI thresholds over which cows are heat stressed, few studies considered how HWs can affect dairy cows' production, and often they investigated the association of HWs with mortality (Vitali et al., 2009, 2015). The HW is generally defined as a prolonged period of excessively hot weather. The lack of an official definition of HW is based on average weather conditions in the area and on normal seasonal temperatures (Robinson, 2001; Beniston et al., 2007; Russo et al., 2014). The International Panel on Climate Change (IPCC) defined it as the longest period in the year of at least 5 consecutive days with a maximum temperature at least 5°C higher than the climatology of the same calendar day. The Environment Canada (2013) defined it as 3 consecutive days of temperatures of at least 32°C daily maximum temperature, but a variety of other definitions exist for an HW. Hahn (1999) defined an HW as a period of at least 3 days characterized by THI greater or equal to 70 for all hours.
In our study, we considered “heat wave” a period of at least 2 consecutive days when the daily maximum THI has exceeded the average maximum THI threshold over which the production worsened for each parameter considered for Brown Swiss cows as defined by Maggiolino et al. (2020).
The authors' hypothesis is that the number of consecutive days with maximum THI higher than the maximum THI threshold can negatively affect production traits, particularly compared to a single day over the maximum THI threshold before the test day. So, the aim of this study is to highlight the potential effect on production losses of HWs lasting 2, 3, 4, and 5 consecutive days over the maximum THI threshold, compared to the losses of only 1 day over the maximum THI threshold.
Materials and Methods
Data were provided by the Italian Brown Swiss Breeders Association (Verona, Italy) and consisted of 1,048,570 test-day records from 85,379 Brown Swiss cows. Records were collected from 749 dairy farms from 2009 through 2018. The first editing of the raw dataset followed the same exclusion criteria as already reported in our previous study (Maggiolino et al., 2020). Briefly, data from all farms located over 700 m above sea level, all farms with less than 10 lactating cows, all cows that did not have at least 5 test-day records per lactation, all multiparous cows that did not have first lactation data available, all cows that did not have at least 15 test-day records per farm among all lactations, all test-day records over 365 days in milk (DIM) were excluded. After applying the inclusion and exclusion criteria, we had 202,776 records for the analysis on the maximum THI.
The 4% fat-corrected milk (FCM) yield was calculated for each test-day record according to the formula reported by Gaines and Davidson (1923):
The energy-corrected milk (ECM) yield was calculated for each test-day record according to the formula reported by Yan et al. (2011):
Cheese yield was calculated according to the formula reported by Van Slyke and Publow (1910) modified for Parmigiano Reggiano cheese:
where fat recovery factor is 0.93; factor accounting for casein loss is 0.1; 1.09 represents the constituents of cheese solids non-casein and non-fat, corresponding to 9% of the sum; M is the moisture of fresh cheese, assumed to be 39.5% as for 24-h Parmigiano Reggiano cheese (Formaggioni et al., 2015).
Cheese production at 24 h (4) was calculated following the formula reported by Formaggioni et al. (2015) obtained by adapting the Van Slyke and Publow (1910) formula to 24-h Parmigiano Reggiano cheese characteristics, both for each test-day record as follow:
where MY24h is daily milk yield (24 h) and is calculated as follow:
where 0.84 is the fat recovery factor, 0.944 is the theoretical case in factor recovery,
and SY is the standard yield and is calculated as follow:
Statistical Analysis
Two sets of analyses were carried out as reported in our previous study (Maggiolino et al., 2020) and also briefly reported here. First, a mixed linear model with repeated measures was fitted to obtain least squares estimates of THI effect on production traits:
where Y is the outcome (MY, FCM, kg/day), fat (%), fat yield (kg/day), protein (%), protein yield (kg/day), cheese yield, equivalent cheese milk, and cheese milk production in 24 h; farm (yc) is the fixed effect of the herd nested within the year of calving (yc); mc (yc) is the fixed effect of the month of calving (mc) nested within the year of calving; cdim is the fixed effect of DIM class; This is the fixed effect of THI maximum class; ord is the fixed effect of parity class; thir × ord is the fixed effect of the THI class by parity class; cdim × ord is the fixed effect of the DIM class by parity class; μ is the random factor that corrects for repeated measures of cows; and e is the random residual effect. Five DIM classes were defined as follows: from 0 to 30, from 31 to 90, from 91 to 180, from 181 to 270, and from 271 to 365. The weather dataset was divided into 41 THI maximum classes, with the first class beginning at THI = 50, and the subsequent classes were set at each 1-point THI thereafter until the last class, which had a THI = 90. Parity was grouped into 4 classes: first lactation, second lactation, third lactation, and fourth lactation or more.
In the second set of analyses, the least squares mean estimated for the levels of the interaction effect between the THI class and the parity class in the model (7) were used as the dependent variable to estimate changepoints in the relationship between products. These change points would then determine variables and heat load. The comfort threshold(s) in these populations. The following model was used:
where at time i, yi is an observation of the dependent variable (e.g., least squared means of production), xi T is a k × 1 vector of regressors, with the first component usually equal to unity; β is the k × 1 vector of regression coefficients, which may vary over time (THI); and ui are iid (0, σ2) (i.e., ui is a k × 1 vector of residuals, which are independent and identically distributed with mean 0 and SD σ2). In this second phase, we tested the null hypothesis that the regression coefficients remain constant (reduced model) compared with the alternative hypothesis that at least 1 coefficient varies over time (complete model). If the increase in the sum of square errors from the complete to the reduced model is significantly large, then the null hypothesis can be rejected, concluding that the complete model works well and a significant breakpoint does exist (i.e., a significant change in milk outcome parameter due to THI has been detected). The analyses were repeated by regressing the least squares means on the THI for each of the 15 days before the test-day record. The weighted THI was calculated according to the R2 weight of each THI observed.
After all analyses, we decided to consider and report the THImax effect on all patterns investigated, as this index gave the most consistent results compared with the other ones calculated and considered.
After this, a mixed linear model with repeated measures was fitted to obtain least squares estimates of outcome production in the presence or absence of HWs. HWs are defined as the number of consecutive days exceeding the THI threshold compared to a single day over the threshold. Therefore, test-days with THI below the threshold have been deleted. The weighted threshold values found in our previous study (Maggiolino et al., 2020) were used (Table 1). The effect of four different possible HWs was evaluated: 2 days (2 consecutive days before test-day); 3 days (3 consecutive days before test-day); 4 days (4 consecutive days before test-day); and 5 days (5 consecutive days before test-day). All losses were calculated and compared to losses after only 1 day over the weighted THImax. The farm and the year of calving were included among the random effects because they are considered hierarchically as a second level unit with respect to the cow. HW, the month of calving, DIM class, parity class, and HW by parity class were the fixed effects in the model. The least square means of outcome production by HWs and class parity were compared, and paired multiple comparison was adjusted by Tukey.
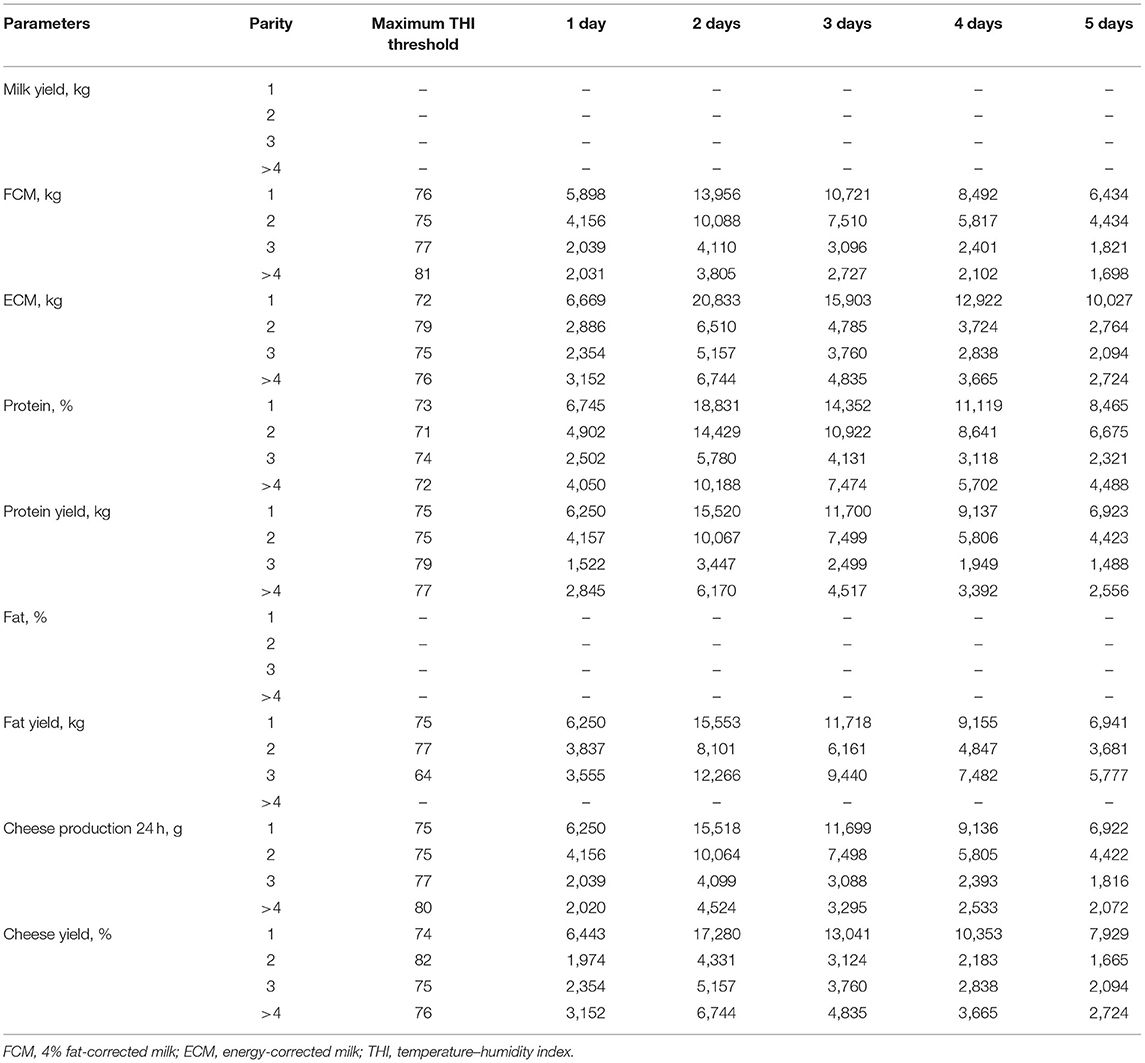
Table 1. Maximum daily THI threshold values and number of records for each production trait and according to parity class.
All tests of statistical significance were two-tailed, and p < 0.05 were considered significant. Statistical analyses were performed using the SAS/STAT® Statistics version 9.4 (SAS, 2011; SAS Institute Inc., Cary, NC, USA). The R software (http://cran.r-project.org) and the “strucchange” package (Zeileis et al., 2002) were used in the breakpoint analyses.
Results
Estimated variations of milk production traits after HWs of 2, 3, 4, and 5 days over the maximum THI threshold, compared to a single day exceeding the maximum THI threshold, are reported in Table 2. The FCM yield showed a progressive reduction (p < 0.05), according to the HW duration. Estimated losses ranged from −319 g for HW lasting 2 days to −532 g after HW of 5 days over the maximum daily THI thresholds. Considering ECM, the yield depression was significant for all the duration of HWs (p < 0.001), ranging from −1.86 to −3.05 kg after 2 and 5 days lasting HW, respectively. In addition, protein percentage, compared to the decreased production at only 1 day over the maximum daily THI threshold, showed a worsening trend as longer was the HW duration (p < 0.01), with recorded values of −0.05% after 2 days HW, until more than doubling these losses after HW lasting 5 days (−0.11%). The protein yield (g/day) did not show differences, in comparison with the depression after 1 day over the daily maximum THI, at 2 days of HW (p > 0.05) but showed a significant and progressive worsening of this pattern after 3 days lasting HW (−11.25 g; p < 0.05), 4 days lasting HW (−23.54 g; p < 0.001), and 5 days lasting ones (−34.35 g; p < 0.001). Fat yield decreased according to the HW duration, compared to a single day over the maximum daily THI threshold (p < 0.01). Brown Swiss cows lose 19.32 g/day after 3 days lasting HW, until 48.92 g/day after HW of 4 days. Cheese production at 24 h did not show differences for HW durations ranging from 2 to 4 days (p > 0.05), but after a 5-day HW a reduction of 42.42 g was recorded (p < 0.05). On the other hand, cheese yield was affected by the HW duration, in comparison with a single day over the daily maximum THI threshold (p < 0.001). Cheese yield losses ranged from −2.59 g after a 2-day HW to −3.42 g for a 5-day HW.
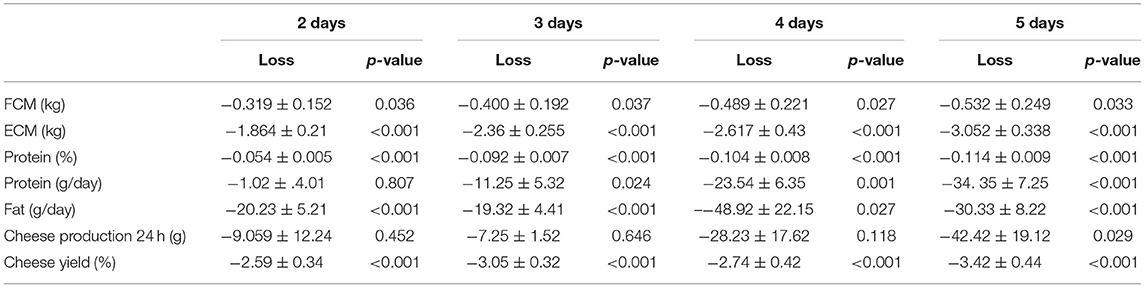
Table 2. Relative variation of fat-corrected milk (FCM; kg), energy-corrected milk (ECM; kg), protein content (%), protein yield (g/day), fat yield (g/day), cheese production at 24 h (g) and cheese yield (%) after heat waves lasting 2, 3, 4, and 5 consecutive days, in comparison with the losses estimated after a single day of daily maximum temperature–humidity index (THI) over the threshold.
Table 3 shows results describing the effect of HW duration (from 2 to 5 consecutive days), compared to the impaired normalized milk yield production (FCM and ECM) due to a single day of daily maximum THI over the threshold, in different classes of parity. Multiparous cows did not show a significant FCM yield reduction in the case of HW compared to a single day over the THI threshold (p > 0.05). Differently, primiparous cows had a lower FCM yield for HW lasting from 3 (−0.359 g; p < 0.05) to 5 days (−0.617 g; p < 0.01). The ECM yield was affected by HWs, regardless of the length of the HW, compared to 1 day over the daily maximum THI threshold (p < 0.001), ranging from losses of −1.232 to losses of −3.097 kg/day. The only exception was in the second-parity cows that had a similar ECM yield for a 2-day HW, though this parity category also impaired productive efficiency for HW longer than 2 days (p < 0.001).
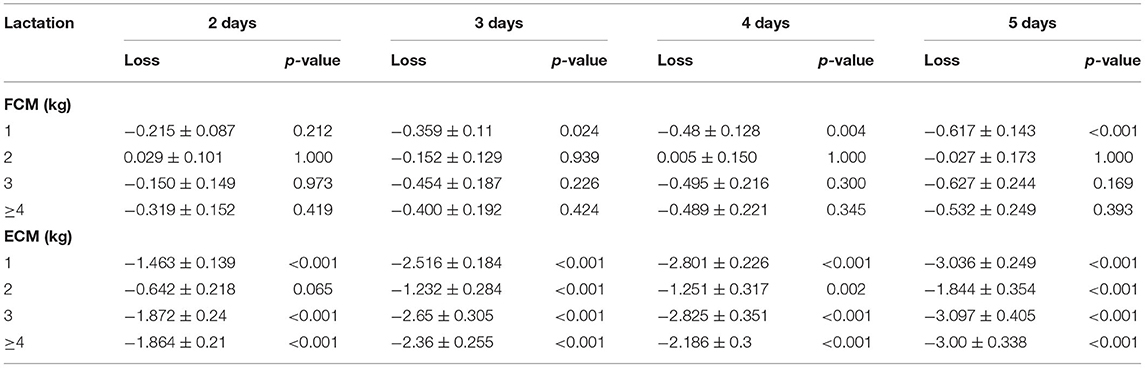
Table 3. Relative variation of fat-corrected milk (FCM) and energy-corrected milk (ECM), after heat waves lasting 2, 3, 4, and 5 consecutive days, compared with the losses estimated after a single day of daily maximum temperature–humidity index (THI) over the threshold, for the different parity classes.
The effect of HW duration in comparison to a single day over the THI threshold on protein percentage, protein, and fat yield traits is reported in Table 4. The protein percentage was negatively affected by HWs, independent from their duration and in all the parity classes considered (p < 0.01), ranging from −0.047% to −0.141% in comparison to a single day over the THI threshold. Protein yield was significantly affected by a 3-day HW in primiparous (p < 0.01) and third-parity cows (p < 0.05), and by 4-day HW in primiparous (p < 0.001), third- (p < 0.01) and fourth- or greater parity cows (p < 0.05), and by a 5-day HW in primiparous (p < 0.01) and fourth-parity cows (p < 0.01). The fat yield in second-parity cows was not affected by HWs. Differently, primiparous and third-parity cows showed losses due to HW lasting from 2 to 5 days (p < 0.01), with depression in fat yield ranging from −15.35 to −37.89 g/day.
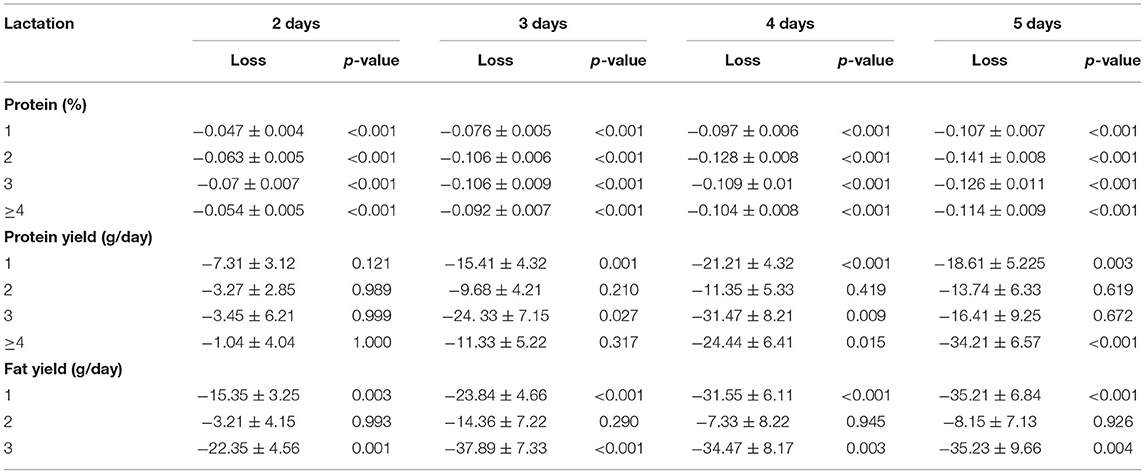
Table 4. Relative variation of protein concentration (%), protein yield (g/day), and fat yield (g/day), after heat waves lasting 2, 3, 4, and 5 consecutive days, in comparison with the losses estimated after a single day of daily maximum THI over the threshold, for the different parity classes.
Table 5 shows cheese production at 24 h and cheese yield estimated patterns as affected by the HW duration (from 2 to 5 consecutive days), compared to the impaired values due to a single day of daily maximum THI over the threshold, in different classes of parity secondiparous cows showed to be not affected by HW in both the considered traits (p > 0.05).
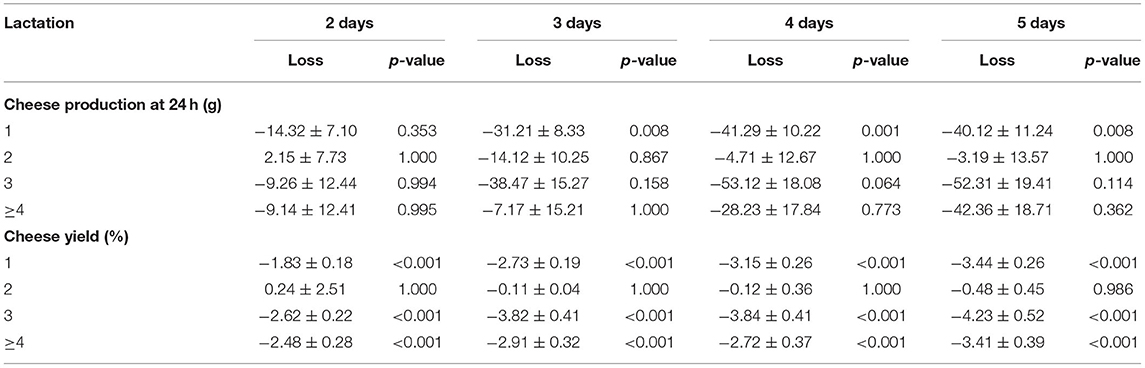
Table 5. Relative variation of cheese production at 24 h (g), cheese yield (%) after heat waves lasting 2, 3, 4, and 5 consecutive days, in comparison with the losses estimated after a single day of daily maximum THI over the threshold, for the different parity classes.
Cheese production at 24 h showed differences in losses only in primiparous cows after 3- to 5-day HW (p < 0.01), with values ranging from −31.21 to −41.29 g. Differently, cheese yield showed to be affected by HWs lasting from 2 to 5 days (p < 0.001) with losses higher than those due to only 1 day over the daily maximum THI threshold, in all the considered parity classes, with the exception of the second- and third-parity decreasing rate of cheese yield according to the parity classes and HW duration, ranged from −1.83% to −4.23%.
Discussion
The temperature-humidity index is a commonly used index for the heat stress evaluation in animals, and particularly in dairy cows, due to its ease of calculation (Ouellet et al., 2019) and to its good accurate prediction suitability in the assessment of animal exposure to heat stress when they are in barns and not exposed to high air movement (Li et al., 2009). Although several studies have been conducted on heat stress and its effect on milk traits in different dairy cow breeds as Holstein (Bohmanova et al., 2007; Sánchez et al., 2009; Aguilar et al., 2010; Bernabucci et al., 2014) and Brown Swiss (Maggiolino et al., 2020), often they tried to show the THI thresholds over which there was a real heat stress effect on physiology, behavior, and/or productive patterns.
Previous research (Maggiolino et al., 2020) reported no maximum THI threshold for milk yield in Brown Swiss, and for this reason, milk yield was not included in this study. In fact, the 2-phase regression approach applied to estimate the THI thresholds did not fit for milk yield; however, the milk yield normalization (FCM and ECM) showed to best fit with it. However, only primiparous cows showed FCM losses after HWs of 4 and 5 days of duration, whereas multiparous cows did not show any FCM losses when the HW duration increased. This result confirms a greater sensibility of cows at first lactation to a hot environment (Maggiolino et al., 2020). In contrast, when ECM was considered, they showed to be highly sensitive to HW across all parities, with losses that increased even more than 3 kg/day after a 5-day HW. The ECM showed more sensitivity, and so greater losses than FCM because of its inclusion in the formula of both fat and protein. Results obtained on ECM are consistent with those recorded on protein and fat in milk because these traits were significantly affected by the HW duration. Probably, the combination of poor heat tolerance for protein and fat milk constituents resulted in a great effect of HWs on ECM. Although protein percentage proved to be very sensitive to HWs, protein yield was affected by HWs only in the first- and third-parity cows by HWs over 3 days. The decrease in protein yield due to heat stress could be related to the drop in caseins synthesis (Bernabucci et al., 2002, 2015). Cheese yield estimates the weight of cheese obtained from 100 kg of milk, and the total cheese production in 24 h, used for Parmigiano Reggiano cheese production, represents the weight of cheese produced from 100 kg of milk after 24 h. Both these parameters were involved in our study because they represent useful tools to monitor the Brown Swiss efficiency in the cheese-making process and in dairy performance (Formaggioni et al., 2015), considering that this breed is mainly intended for cheese production (Maggiolino et al., 2020). Moreover, these parameters are strictly linked to protein yield. Cheese production worsens with increasing HW duration. A 5-day HW can induce higher losses in animals, and particularly primiparous showed higher sensitivity to HW lasting 3 or more days. Primiparous cows appear to be more sensitive to heat stress for protein yield and, consequently, cheese yield, confirming what was reported by Maggiolino et al. (2020). They showed that primiparous cows were characterized by a lower maximum THI threshold compared to multiparous, showing greater sensitivity to heat stress. When the THI threshold is exceeded for 3 days or more, cheese yield losses are higher than only for 1 day. Cows can usually efficiently dissipate heat if they are under a THI threshold. This condition typically occurs during nighttime (Vitali et al., 2020), but if hours over the THI threshold are exceeded during a day, they can make heat dissipation impossible. During a day (in 24 h), usually cows can have part of the day over and part under the THI threshold. This second condition usually happens during night hours that are characterized by lower temperature and so lower THI. When this happens, cows can dissipate heat held during day hours over the THI threshold. There might be a difference among breeds, parities, and individuals in the efficiency in dissipating loaded heat during the day period under the THI threshold. However, cattle initially respond to mild heat stress by sweating, panting, drinking more, and seeking shade when possible. When THI increases and reaches the upper critical value and imbalance between heat production and dissipation capacity is induced, the risk of milk production losses increases (Bernabucci et al., 2014), dry matter intake decreases (Fodor et al., 2018), and infectious and metabolic diseases and mortality risks increase (Olde Riekerink et al., 2007; Vitali et al., 2015).
Gaughan et al. (2008) reported that there are upper and lower thresholds, at which cattle accumulate or dissipate heat, respectively. These thresholds change due to the metabolic differences between growth and lactation, with greater heat accumulation in animals that are involved in both metabolic needs. Considering this, when heat stress lasts only 1 or 2 days and THI decreases below the lower threshold, usually occurring during the nighttime, cows can dissipate the accumulated heat, with no negative effects on productive performance and/or feeding intake (Fox and Tylutki, 1998).
There is an increased occurrence of HWs worldwide (Meehl and Tebaldi, 2004), and also in the Mediterranean Basin regions. This is expected to cause additional heat stress in dairy cows, as reflected by our results. As reported by Vitali et al. (2015), HWs may also reach a length of 8–10 days, with production losses that must be calculated and prevented. Obviously, under such conditions, heat stress is correlated not only with impaired milk traits production but also with an increased cattle mortality (Bishop-Williams et al., 2015; Vitali et al., 2015) and culling rate due to reduced fertility (Pinedo et al., 2010).
During the last quarter century, livestock efforts focused on improving production efficiency and nutritional requirements in animals exposed to adverse environmental conditions, applying selection pressure on milk and milk constituents' yield rather than improving stress resistance. Although this approach increased dairy cows' productivity, it also increased their sensitivity (reduced their thermal plasticity) in warm microenvironmental conditions (Collier et al., 2018).
In conclusion, the present study contributes to deepening knowledge on how THI persistence over the THI threshold can affect productive traits and how the HW duration is able to get worse in Brown Swiss dairy cows' production. This is even more true considering that there were more 2, 3, 4, and 5-day HW lengths than only 1 day over the THI threshold (as reported in Table 1). Although current cooling system technologies should be applied to cope with dairy cow's heat stress (Collier et al., 2018), genetic improvement, also based on genomic approach, for increasing heat tolerance should be considered as a potential strategy to help for mitigating the negative effects of increased frequency of HWs (Garner et al., 2016; Nguyen et al., 2016).
Moreover, further studies are needed to investigate sensitivity to heat load. It is necessary to pay more attention and better investigate how to improve animal welfare and efficiency and breeding selection.
Data Availability Statement
The data analyzed in this study is subject to the following licenses/restrictions: the database if part of the official test-day records database and is intellectual propriety of breeder association that allow use for research purpose upon official request. Requests to access these datasets should be directed to dmluY2Vuem8ubGFuZGlAdW5pYmEuaXQ=.
Ethics Statement
Ethical review and approval was not required for the animal study because this study have been carried out on official fenotypic data collected by the official national animal recording system in its routine activity for breeder associations. All data are available in the national central database upon official request.
Author Contributions
AM, PD, and VL designed the study. AM, VL, ES, AB, and NB performed the dataset collection and analysis preparation. NB conducted the statistical analysis. AM and NB drafted the manuscript. PD and UB supervised and reviewed the manuscript. All authors contributed to the article and approved the submitted version.
Conflict of Interest
Author ES was employed by Italian Breeder Association.
The remaining authors declare that the research was conducted in the absence of any commercial or financial relationships that could be construed as a potential conflict of interest.
Publisher's Note
All claims expressed in this article are solely those of the authors and do not necessarily represent those of their affiliated organizations, or those of the publisher, the editors and the reviewers. Any product that may be evaluated in this article, or claim that may be made by its manufacturer, is not guaranteed or endorsed by the publisher.
Acknowledgments
The authors are grateful to Giovanna Calzaretti and Francecsco Giannico (Department of Veterinary Medicine, University of Bari Aldo Moro) for their technical support. Moreover, the authors are grateful to Marco Giazzi and Marco Tadini, the president and vice president, respectively, of the Meteonetwork Association (https://www.meteonetwork.it) and to the Italian Air Force weather service (http://www.aeronautica.difesa.it) for giving us access to all weather station data.
References
Aguilar, I., Misztal, I., and Tsuruta, S. (2010). Short communication: genetic trends of milk yield under heat stress for US Holsteins. J. Dairy Sci. 93, 1754–1758. doi: 10.3168/jds.2009-2756
Allen, J. D., Hall, L. W., Collier, R. J., and Smith, J. F. (2015). Effect of core body temperature, time of day, and climate conditions on behavioral patterns of lactating dairy cows experiencing mild to moderate heat stress. J. Dairy Sci. 98, 118–127. doi: 10.3168/jds.2013-7704
Beniston, M., Stephenson, D. B., Christensen, O. B., Ferro, C. T., Frei, C., et al. (2007). Future extreme events in European climate: an exploration of regional climate model projections. Clim. Change 81, 71–95. doi: 10.1007/s10584-006-9226-z
Bernabucci, U., Basiricò, L., Morera, P., Dipasquale, D., Vitali, A., Piccioli Cappelli, F., et al. (2015). Effect of summer season on milk protein fractions in Holstein cows. J. Dairy Sci. 98, 1815–1827. doi: 10.3168/jds.2014-8788
Bernabucci, U., Biffani, S., Buggiotti, L., Vitali, A., Lacetera, N., and Nardone, A. (2014). The effects of heat stress in Italian Holstein dairy cattle. J. Dairy Sci. 97, 471–486. doi: 10.3168/jds.2013-6611
Bernabucci, U., Lacetera, N., Ronchi, B., and Nardone A. (2002). Effects of the hot season on milk protein fractions in Holstein cows. Anim. Res. 51, 25–33. doi: 10.1051/animres:2002006
Bishop-Williams, K. E., Berke, O., Pearl, D. L., Hand, K., and Kelton, D. F. (2015). Heat stress related dairy cow mortality during heat waves and control periods in rural Southern Ontario from 2010–2012. BMC Vet. Res. 11, 291. doi: 10.1186/s12917-015-0607-2
Bohmanova, J., Misztal, I., and Cole, J. B. (2007). Temperature-humidity indices as indicators of milk production losses due to heat stress. J. Dairy Sci. 90, 1947–1956. doi: 10.3168/jds.2006-513
Brügemann, K., Gernand, E., König Von Borstel, U., and König, S. (2012). Defining and evaluating heat stress thresholds in different dairy cow production systems. Anim. Breed. 55, 13–24. doi: 10.5194/aab-55-13-2012
Collier, R. J., Baumgard, L. H., Zimbelman, R. B., and Xiao, Y. (2018). Heat stress: physiology of acclimation and adaptation. Anim. Front. 9, 12–19. doi: 10.1093/af/vfy031
Dahl, G. E., Skibiel, A. L., and Laporta, J. (2019). In utero heat stress programs reduced performance and health in calves. Vet. Clin. North Am. Food Anim. Pract. 35, 343–353. doi: 10.1016/j.cvfa.2019.02.005
Environment Canada (2013). Historical Climate Data. Retreived from: http://climate.weather.gc.ca/ (accessed May 21, 2013)
Fodor, N., Foskolos, A., Topp, C. F. E., Moorby, J. M., Pásztor, L., and Foyer, C. H. (2018). Spatially explicit estimation of heat stress-related impacts of climate change on the milk production of dairy cows in the United Kingdom. PLoS ONE 13:e0197076. doi: 10.1371/journal.pone.0197076
Formaggioni, P., Summer, A., Malacarne, M., Franceschi, P., and Mucchetti, G. (2015). Italian and Italian-style hard cooked cheeses: Predictive formulas for Parmigiano-Reggiano 24-h cheese yield. Int. Dairy J. 51, 52–58. doi: 10.1016/j.idairyj.2015.07.008
Fox, D. G., and Tylutki, T. P. (1998). Accounting for the effects of environment on the nutrient requirements of dairy cattle. J. Dairy Sci. 81, 3085–3095. doi: 10.3168/jds.S0022-0302(98)75873-4
Gaines, W. L., and Davidson, F. A. (1923). Relationship Between Percentage Fat Content and Yield of Milk. Illinois Agricultural Experimentation Station Bulletin. Urbana-Champaign: University of Illinois.
Garner, J. B., Douglas, M. L., Williams, S. R. O., Wales, W. J., Marett, L. C., Nguyen, T. T. T., et al. (2016). Genomic selection improves heat tolerance in dairy cattle. Sci. Rep. 6:34114. doi: 10.1038/srep34114
Gaughan, J. B., Mader, T. L., Holt, S. M., and Lisle, A. (2008). A new heat load index for feedlot cattle. J. Anim. Sci. 86, 226–234. doi: 10.2527/jas.2007-0305
Godyń, D., Herbut, P., and Angrecka, S. (2019). Measurements of peripheral and deep body temperature in cattle—a review. J. Therm. Biol. 79, 42–49. doi: 10.1016/j.jtherbio.2018.11.011
Hahn, G. L. (1999). Dynamic responses of cattle to thermal heat loads. J. Anim. Sci. 77, 10–20. doi: 10.2527/1997.77suppl_210x
Hammami, H., Bormann, J., M'hamdi, N., Montaldo, H. H., and Gengler, N. (2013). Evaluation of heat stress effects on production traits and somatic cell score of Holsteins in a temperate environment. J. Dairy Sci. 96, 1844–1855. doi: 10.3168/jds.2012-5947
Heinicke, J., Hoffmann, G., Ammon, C., Amon, B., and Amon, T. (2018). Effects of the daily heat load duration exceeding determined heat load thresholds on activity traits of lactating dairy cows. J. Therm. Biol. 77, 67–74. doi: 10.1016/j.jtherbio.2018.08.012
Herbut, P. (2018). Environmental parameters to assessing of heat stress in dairy cattle—a review. Int. J. Biometeorol. 62, 2089–2097. doi: 10.1007/s00484-018-1629-9
Herbut, P., Bieda, W., and Angrecka, S. (2015). Influence of hygrothermal conditions on milk production in a free stall barn during hot weather. Anim. Sci. Pap. Rep. 33, 49–58.
Kadzere, C. T., Murphy, M. R., Silanikove, N., and Maltz, E. (2002). Heat stress in lactating dairy cows: a review. Livestock Prod. Sci. 77, 59–91. doi: 10.1016/S0301-6226(01)00330-X
Li, S., Gebremedhin, K. G., Lee, C. N., and Collier, R. J. (2009). Evaluation of Thermal Stress Indices for Cattle. ASABE 2009, Vol. 4, (Reno, NV: American Society of Agricultural and Biological Engineers Annual International Meeting), 2283–2302.
Mader, T. L., Davis, M. S., and Brown-Brandl, T. (2006). Environmental factors influencing heat stress in feedlot cattle1,2. J. Anim. Sci. 84, 712–719. doi: 10.2527/2006.843712x
Maggiolino, A., Dahl, G. E., Bartolomeo, N., Bernabucci, U., Vitali, A., Serio, G., et al. (2020). Estimation of maximum thermo-hygrometric index thresholds affecting milk production in Italian Brown Swiss cattle. J. Dairy Sci. 103, 8541–8553. doi: 10.3168/jds.2020-18622
Meehl, G. A., and Tebaldi, C. (2004). More intense, more frequent, and longer lasting heat waves in the 21st century. Science 305, 994–997. doi: 10.1126/science.1098704
Nguyen, T. P. L., Seddaiu, G., Virdis, S. G. P., Tidore, C., Pasqui, M., and Roggero, P. P. (2016). Perceiving to learn or learning to perceive? Understanding farmers' perceptions and adaptation to climate uncertainties. Agric. Syst. 143, 205–216. doi: 10.1016/j.agsy.2016.01.001
Olde Riekerink, R. G. M., Barkema, H. W., and Stryhn, H. (2007). The effect of season on somatic cell count and the incidence of clinical mastitis. J. Dairy Sci. 90, 1704–1715. doi: 10.3168/jds.2006-567
Ouellet, V., Cabrera, V. E., Fadul-Pacheco, L., and Charbonneau, É. (2019). The relationship between the number of consecutive days with heat stress and milk production of Holstein dairy cows raised in a humid continental climate. J. Dairy Sci. 102, 8537–8545. doi: 10.3168/jds.2018-16060
Pinedo, P. J., De Vries, A., and Webb, D. W. (2010). Dynamics of culling risk with disposal codes reported by Dairy Herd Improvement dairy herds. J. Dairy Sci. 93, 2250–2261. doi: 10.3168/jds.2009-2572
Pinto, S., Hoffmann, G., Ammon, C., and Amon, T. (2020). Critical THI thresholds based on the physiological parameters of lactating dairy cows. J. Therm. Biol. 88:102523. doi: 10.1016/j.jtherbio.2020.102523
Ravagnolo, O., Misztal, I., and Hoogenboom, G. (2000). Genetic component of heat stress in dairy cattle, development of heat index function. J. Dairy Sci. 83, 2120–2125. doi: 10.3168/jds.S0022-0302(00)75094-6
Rhoads, M. L., Rhoads, R. P., Vanbaale, M. J., Collier, R. J., Sanders, S. R., Weber, W. J., et al. (2009). Effects of heat stress and plane of nutrition on lactating Holstein cows: I. Production, metabolism, and aspects of circulating somatotropin1. J. Dairy Sci. 92, 1986–1997. doi: 10.3168/jds.2008-1641
Robinson, P. J. (2001). On the definition of a heat wave. J. Appl. Meteorol. 40, 762–775. doi: 10.1175/1520-0450(2001)040<0762:OTDOAH>2.0.CO;2
Russo, S., Dosio, A., Graversen, R. G., Sillmann, J., Carrao, H., Dunbar, M. B., et al. (2014). Magnitude of extreme heat waves in present climate and their projection in a warming world. J. Geophys. Res. Atmos. 119, 500–512. doi: 10.1002/2014JD022098
Sánchez, J. P., Misztal, I., Aguilar, I., Zumbach, B., and Rekaya, R. (2009). Genetic determination of the onset of heat stress on daily milk production in the US Holstein cattle. J. Dairy Sci. 92, 4035–4045. doi: 10.3168/jds.2008-1626
Van Slyke, L. L., and Publow, C. A. (1910). The Science andd Practice of Cheese-Making. New York, N.Y.: Orange Judd Co.
Vitali, A., Felici, A., Esposito, S., Bernabucci, U., Bertocchi, L., Maresca, C., et al. (2015). The effect of heat waves on dairy cow mortality. J. Dairy Sci. 98, 4572–4579. doi: 10.3168/jds.2015-9331
Vitali, A., Felici, A., Lees, A. M., Giacinti, G., Maresca, C., Bernabucci, U., et al. (2020). Heat load increases the risk of clinical mastitis in dairy cattle. J. Dairy Sci. 103, 8378–8387. doi: 10.3168/jds.2019-17748
Vitali, A., Segnalini, M., Bertocchi, L., Bernabucci, U., Nardone, A., and Lacetera, N. (2009). Seasonal pattern of mortality and relationships between mortality and temperature-humidity index in dairy cows. J. Dairy Sci. 92, 3781–3790. doi: 10.3168/jds.2009-2127
Yan, M.-J., Humphreys, J., and Holden, N. M. (2011). An evaluation of life cycle assessment of European milk production. J. Environ. Manage. 92, 372–379. doi: 10.1016/j.jenvman.2010.10.025
Zeileis, A., Leisch, F., Hornik, K., and Kleiber, C. (2002). strucchange: an R package for testing for structural change in linear regression models. J. Stat. Softw. 7, 1–38. doi: 10.18637/jss.v007.i02
Keywords: heat waves, heat stress, THI, Brown Swiss, dairy cow
Citation: Maggiolino A, Landi V, Bartolomeo N, Bernabucci U, Santus E, Bragaglio A and De Palo P (2022) Effect of Heat Waves on Some Italian Brown Swiss Dairy Cows' Production Patterns. Front. Anim. Sci. 2:800680. doi: 10.3389/fanim.2021.800680
Received: 23 October 2021; Accepted: 06 December 2021;
Published: 17 January 2022.
Edited by:
Edward Narayan, The University of Queensland, AustraliaReviewed by:
John Gaughan, The University of Queensland, AustraliaMassimo Malacarne, University of Parma, Italy
Copyright © 2022 Maggiolino, Landi, Bartolomeo, Bernabucci, Santus, Bragaglio and De Palo. This is an open-access article distributed under the terms of the Creative Commons Attribution License (CC BY). The use, distribution or reproduction in other forums is permitted, provided the original author(s) and the copyright owner(s) are credited and that the original publication in this journal is cited, in accordance with accepted academic practice. No use, distribution or reproduction is permitted which does not comply with these terms.
*Correspondence: Vincenzo Landi, dmluY2Vuem8ubGFuZGlAdW5pYmEuaXQ=