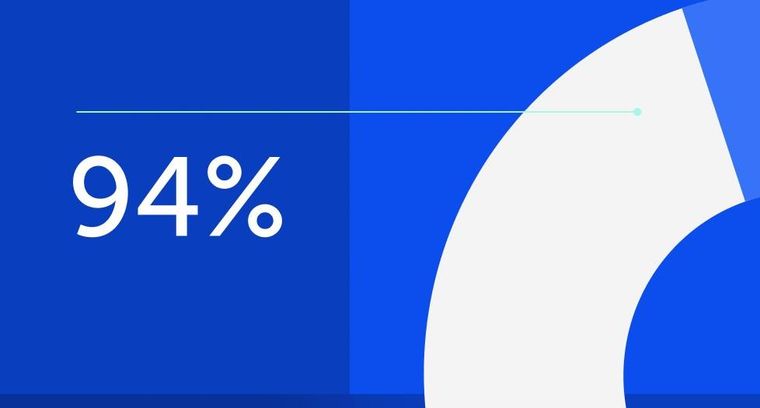
94% of researchers rate our articles as excellent or good
Learn more about the work of our research integrity team to safeguard the quality of each article we publish.
Find out more
REVIEW article
Front. Anim. Sci., 18 November 2021
Sec. Animal Physiology and Management
Volume 2 - 2021 | https://doi.org/10.3389/fanim.2021.775311
This article is part of the Research TopicEnvironmental Management of Poultry – Advances and Perspectives on Improving Animal Well-beingView all 6 articles
Environmental stressors can promote the vulnerability of animals to infections; it is therefore, essential to understand how stressors affect the immune system, the adaptive capacity of animals to respond, and effective techniques in managing stress. This review highlights scientific evidence regarding environmental stress challenge models and the potential effectiveness of vitamin supplementation. The major environmental stressors discussed are heat and cold stress, feed restriction, stocking density, and pollutants. Much work has been done to identify the effects of environmental stress in broilers and layers, while few involved other types of poultry. Studies indicated that chickens' performance, health, and welfare are compromised when challenged with environmental stress. These stressors result in physiological alterations, behavioral changes, decreased egg and meat quality, tissue and intestinal damage, and high mortalities. The application of vitamins with other nutritional approaches can help in combating these environmental stressors in chickens. Poultry birds do not synthesize sufficient vitamins during stressful periods. It is therefore suggested that chicken diets are supplemented with vitamins when subjected to environmental stress. Combination of vitamins are considered more efficient than the use of individual vitamins in alleviating environmental stress in chickens.
It is essential to maximize poultry production to meet the increasing demand for animal protein due to the increase in global human population (Tona, 2018). One of the major determinants of successful poultry production is the ability to combat environmental stress challenges in broiler chickens. Stress occurs as a result of a biological response to an internal or external stimulus that poses threats to the normal physiological equilibrium of an organism (Elitok, 2018). Commercial poultry production is faced with a variety of stresses, including environmental, nutritional, and internal stress which decrease production and reproductive performance and affect the health status of poultry birds (Surai and Fisinin, 2016). Poultry suffer from various environmental stressors such as heat stress (Tsiouris et al., 2018), cold stress (Zhang et al., 2011), feed restriction (Janczak et al., 2007), stocking density, pollutants, and many more.
Despite the advances in housing and management technologies to combat the effects of environmental stress, poultry production is still gravely impacted by stressors (Abo Ghanima et al., 2020). Consequently, the use of nutritional manipulation during periods of prolonged heat stress helps strengthen the application of modern technology to alleviate stressors in poultry farms, thereby maintaining the health and production performance of flocks (Abdel-Moneim et al., 2021). Dietary manipulations such as dietary supplementation with amino acids, vitamins, minerals, polyphenols, and phytogenics are widely applied to ameliorate the detrimental impacts of environmental stress in poultry (Abd El-Hack et al., 2020). These additives have proven efficacious following their pharmacological and nutritional attributes, minimum or no side effects, anti-oxidative and immune promoting functions, acid-base homeostasis, and improved production performance in poultry (Olgun et al., 2021).
Vitamins are required for essential cell activities such as metabolism, growth, and development. In addition to these conventional functions, vitamins A, D, E, and C have vital roles in normal functioning of the immune system (Mishra and Jha, 2019). Vitamins has been one of the least-studied group of nutrients, but recent evidence has demonstrated the role of vitamins in improving the performance and survivability of poultry during stress conditions (Balnave, 2004). For instance, the vitamin requirement of poultry is increased during heat stress conditions, and poultry birds do not synthesize sufficient vitamins during stressful periods (Abidin and Khatoon, 2013). Thus, it is recommended that broilers' diet should be complemented with vitamins via exogenous supply to maintain normal body functions. Most of the vitamin requirement values that are currently used in feed formulation have been determined between 1980's and 1990's (NRC, 1994). Hence, current vitamin requirement values may no longer be able to achieve desired production efficiency without interfering with the health of the birds. In aged laying hens, the existing dietary nutrient criteria adopted by farmers may not be enough for the birds exposed to a variety of stress. Lots of efforts such as dietary supplementation of vitamin D and biotin have been engaged to improve the production performance of aged laying hens when exposed to stress (Burton et al., 1983). Exogenous vitamins serve as antioxidants by hunting reactive oxygen species (ROS), providing antioxidative functions that are beneficial in fighting stress (Min et al., 2018). The aim of this review is to highlight scientific evidence regarding environmental stress challenge such as heat and cold stress, feed restriction, stocking density, and pollutants in chickens, and the potential effectiveness of vitamin supplementation in combating the mentioned stress challenges.
The thermal environment in chicken housing is a primary determinant of production performance and efficiency (Purswell et al., 2012). Exposure of chickens to chronic environmental temperatures (high or low) during the period of production may have an adverse effect on growth performance, meat yield, immune response, and mortality (Washburn, 1985; Howlider and Rose, 1989). A high ambient temperature increases chickens' energy requirement and results in significant losses from a less efficient conversion of feed to meat or egg, which detrimentally impacts the health and productivity of chickens. Thermal stress incites a classic family of molecular chaperones known as heat shock proteins (HSPs) which play protective roles against tissue damage (Calderwood, 2010).
Heat stress (HS) is a commonly known physical environmental stressor that can affect the performance, health, and welfare of poultry (Tsiouris et al., 2018). HS occurs when heat produced in the body surpasses its dissipation capacity, and the body becomes unable to get rid of excess heat (Lara and Rostagno, 2013). HS has been reported as a key environmental factor that decreases the growth performance of chickens (Goo et al., 2019). Various physiological interruptions, such as systemic immune dysregulation, endocrine disorders, respiratory alkalosis, and electrolyte imbalance are experienced by poultry exposed to HS (Teeter et al., 1985; Sohail et al., 2010; Lara and Rostagno, 2013) and these ultimately decrease their growth performance and intestinal barrier function (Goo et al., 2019). By impairing thermoregulation, HS can increase mortality rates (Saiz del Barrio et al., 2020) and change the physiological and behavioral responses of birds. For example, birds spend less time on eating and walking, while more time is spent on drinking, resting, and panting under HS challenge conditions (Mack et al., 2013). In a study conducted by Branco et al. (2021), birds subjected to HS were prostrate, experienced shorter behavioral responses such as laying down and eating. Birds strove for adaptation under HS circumstances by increasing water intake (that helps promote heat loss), decreasing feed consumption and increasing laying down to reduce heat production through movement and increase panting to enhance evaporative cooling (Daghir, 2009; Mahmoud et al., 2015).
HS condition has been confirmed to increase HSP expression (Vinoth et al., 2015), rectal temperature, heterophil:lymphocyte ratios (H/L ratio), and plasma corticosterone (Soleimani et al., 2011). An upsurge in corticosterone as a response to HS causes muscle protein breakdown and provides amino acid substrates to liver gluconeogenesis that is responsible for energy supply (Ma et al., 2021). The rise in corticosterone levels might increase oxidative stress, induce cell death in follicular cells, leading to a decrease in follicle numbers and subsequently reducing egg production (Li G. M. et al., 2020). In layers, Allahverdi et al. (2013) observed that high temperatures significantly decreased egg quality, egg weight, shell weight, egg specific gravity, and shell thickness. Aside from the reduction in egg quality, HS has been found to delay sexual maturity in layers (Sharma et al., 2020). Zhao et al. (2021) found that high-temperature levels damage the viability of granulosa cells in laying hens. Broilers are more sensitive to HS than layers (Zahoor et al., 2017). Previous evidence revealed that the negative effect of HS on body temperature, plasma creatine, and skeletal muscles are higher in broilers than layers (Sandercock et al., 2006). In broilers, HS has been reported to cause oxidative stress and weaken antioxidant status resulting from increased lipid peroxidation and decreased superoxide dismutase (SOD) activity (Vinoth et al., 2015). Researchers have studied the effects of HS on blood biochemistry parameters in chickens. Gharib et al. (2005) observed that plasma albumin, serum calcium (Ca) levels, red blood cell count decreased significantly in pullets challenged with HS. Bueno et al. (2017) showed that cyclic HS does not alter uric acid, total protein, albumin, globulin but increased aspartate aminotransferase (AST) activity.
HS can negatively affect poultry gut health (Tsiouris et al., 2018), as it has been associated with various changes in the intestinal morphology, gut microbiota, and intestinal integrity (Song et al., 2014). During HS, chickens attempt to dissipate the accumulated body temperature, resulting in a reduction in blood flow to the internal organs, in which the gastrointestinal tracts (GIT) get affected, then results in slackening of the tight junctions, leading to higher intestinal permeability (Gupta et al., 2017) which affects the intestinal barrier function (Goo et al., 2019). HS impaired the small intestinal mucosa as indicated by reduced villous height and crypt depth (Shakeri et al., 2019). Similarly, Santos et al. (2015) and Song et al. (2013) reported that destructions of crypt depth, stripping and reduction of villus height, and reduction in epithelial cell area ratio were observed among heat-stressed birds. HS promotes the proliferation of pathogenic bacteria such as Escherichia coli, Salmonella spp. in chickens (Kammon et al., 2019). Tsiouris et al. (2018) reported that outbreak of necrotic enteritis was prompted in chickens in response to HS. Broilers subjected to HS had reduced pH values of intestinal digesta, related to the alteration of protective microbiota (Tsiouris et al., 2018). It is suggested that high temperature could change the cecum microbiota diversity and disrupt the homeostasis of gut bacteria in chicken by enhancing the growth of Bacteroidetes and decreasing Euryarchaeota (Wang et al., 2020). The GIT of chickens comprises the most receptive organs to HS (Akinyemi et al., 2020); however, the reaction differs within different intestinal segments. In the chicken jejunum, heat-stressed broilers had a significant mRNA up-regulation of HSF3 (heat shock factor), while in ileum both HSF1 and HSF3 mRNA levels were increased after HS exposure. Expression of HSFs and HSPs, due to high heat exposure were more pronounced in the chicken ileum compared to the jejunum (Varasteh et al., 2015). HSF1 and HSF3 are the major members of the HSF family engaged in regulating HSPs in chicken under heat-stress challenge. HSPs are grouped based on their molecular weights. Among HSP classes, HSP60, HSP70, and HSP90 are mostly studied (Shehata et al., 2020).
Furthermore, HS has been reported to reduce immune function and intestinal development, which can degrade birds' innate protective mechanisms making them vulnerable to pathogens. It has been indicated that HS can increase the effect of E. coli on intestinal inflammatory injury of chickens by increasing the TLR4-NF-κB signaling pathway (Tang et al., 2021). The results of Quinteiro-Filho et al. (2017) affirmed that HS activated hypothalamus–pituitary–adrenal (HPA) axis, increased Salmonella enteritidis infection, and impaired the chicken immune system. The HPA axis is a major part of the neuroendocrine system that helps in regulating body processes and controlling reactions to stress (Kaiser et al., 2009). The activation of the HPA axis quickens the release of a key hormone (corticosterone) during stress which stimulates a reduction in IgA levels and toll-like receptors (Quinteiro-Filho et al., 2017). An additional study by Honda et al. (2015) identified that HS altered the immune cell profile of broiler chickens by reducing the B-lymphocyte and increasing the T-cytotoxic suppressor and T-helper lymphocytes in the blood. HS can also affect the weight of immune organs of chickens. Quinteiro-Filho et al. (2010) reported that HS decreased the relative weight of some immune organs (bursa, spleen, and thymus). In general, HS in chickens is linked with reduced feed intake, declined growth performance, feed efficiency, egg production, and quality, and increased oxidative stress in birds (Khan et al., 2012). Oxidative stress in poultry could result from the accumulation of stress, such as nutritional or HS, which alters birds' overall performance (Shojadoost et al., 2021). The detailed characteristics of some of the included studies on HS are summarized in Table 1.
Another important environmental stressor is cold stress (CS). CS occurs when the surrounding temperature drop below 18°C (Dhanalakshmi et al., 2007). In such case, the animal's body might find it difficult to warm itself, resulting in serious cold-associated disease, tissue damage, and eventual death (Dhanalakshmi et al., 2007). Adult birds can withstand CS because they can control their body temperature and generate heat by metabolism (Mozo et al., 2005). However, neonatal chicks are susceptible to cold temperature and cannot survive the harsh condition until they develop mature organs for thermogenesis (Mujahid, 2010). Mujahid and Furuse, 2009a observed that the plasma corticosterone of neonatal chicks was not affected when exposed to CS, which may imply that the HPA-axis of such chicks was immature. The inactiveness of HPA-axis might be associated with hypothermia and lipid peroxidation in chicks. Mujahid and Furuse (2009b) reported that neonatal chicks exposed to cold temperature (20°C for 3 h) were unable to maintain thermostability despite the availability of feed. This resultantly affected the behavior and decreased distress vocalization in those chicks. In addition, low temperature (20°C for 12 h) increased lipid peroxidation in the brain and hearts of neonatal chicks (Mujahid and Furuse, 2009a).
Exposure of growing chickens (21 to 41 d old) to CS condition at 15.5°C significantly increased feed intake, feed conversion ratio, and mortality while the body weight and overall productivity were generally reduced in response to CS (Mendes et al., 1997). The two major causes of death in a cold environment are ascites and cardiomyopathy (Mendes et al., 1997). Additional results revealed that both acute (12 ± 1°C kept for 1, 3, 6, 12, 24 h) and chronic CS (12 ± 1°C kept for 5, 10, 20 d) could trigger oxidative stress in the duodenum and change nitric oxide synthase (iNOS), linking with the intestinal damage process in 15 d old broilers (Zhang et al., 2011). Chen et al. (2015) concluded that CS prompted oxidative stress and produced free radicals which initiated the up-generation of liver fatty acid-binding protein (L-FABP). The L-FABP acts to inactivate free radicals and promote fatty acid intake to manage low temperature through lipid synthesis (Chen et al., 2015). Moreover, the H/L ratio was significantly higher in birds challenged with CS, negatively affecting their welfare (Campo et al., 2008). Zhao et al. (2013) observed a significant decrease in the height of jejunum villus in CS challenged birds and concluded that CS could cause a change in immune function and intestinal damage in chickens. The effect of CS on the messenger RNA (mRNA) levels of corticotrophin-releasing hormone (CRH) and thyrotropin-releasing hormone (TRH) in the hypothalami of broilers showed that birds subjected to acute CS had significantly increased mRNA levels of TRH in the hypothalamus (Wang and Xu, 2008). CS has also been reported to negatively affect meat quality (Dadgar et al., 2012). Dadgar et al. (2012) observed a depletion of glycogen reserve in broilers thigh muscle, revealing a dark, firm, dry quality defect when exposed to low temperature of −8°C. A cold environmental temperature that is below 16°C has adverse effects on animal's productive performance. Specifically, in birds, cold temperature can lead to a variety of negative effects such as increased feed intake, decreased egg production and nutrient digestibility, and reduced individual body weight and feed efficiency (Xie et al., 2017; Olfati et al., 2018; Li D. et al., 2020). Blahová et al. (2007) recorded that cold temperature influenced the level of triiodothyronine, hemoglobin, haematocrit, abdominal fat content, and heart weight in male broiler chickens significantly. The results clearly confirmed that during growth, low temperature adversely affected some performance indices and blood systems in broiler chickens (Blahová et al., 2007). A recent study detected that under CS, the intestinal barrier function might be damaged and bacterial translocation might take place (Zhou H. J. et al., 2021). Chickens subjected to CS for 72 h had increased blood endotoxin, aspartate aminotransferase, glucose, and low-density lipoprotein cholesterol levels significantly (Zhou H. J. et al., 2021). High ascites-related-mortality and intestinal bacterial infection is rampant under CS challenge condition (Qureshi et al., 2018). In summary, CS enhances susceptibility to infections and accrues huge economic loss in broiler production. The detailed characteristics of the included studies on CS are summarized in Table 2.
Feed restriction has been identified as one of the causes of physiological stress in chickens (Najafi et al., 2015), especially in young birds because they are in rapid growth stages and have high metabolic requirements (Mench, 2002). Most times, feed restriction is initiated during the first 3 weeks of age (Soleimani et al., 2012; Trocino et al., 2015) and can induce stress, as indicated by elevated corticosterone concentrations, enhanced abnormal behaviors, and impaired welfare and health of chickens (Yan et al., 2021). Feed deprivation can be applied in different ways either quantitative or qualitative. The reduction in the amount of the feeds is known as quantitative feed restriction while decreasing the energy content in a given feed is known as qualitative feed restriction (Urdaneta-Rincon and Leeson, 2002). Feed restriction has been previously reported to reduce body weight gain, body weight, feed consumption, and meat quality (Urdaneta-Rincon and Leeson, 2002; Abu-Dieyeh, 2006; Cornejo et al., 2007; Hassanabadi, 2008; Boostani et al., 2010; Azis and Afriani, 2017). The negative effect of feed restriction in chickens might depend on the extent, timing, and intensity (Trocino et al., 2020). Chicks subjected to 95, 90, or 85% feed restriction from 5 to 42 d of age had lowered body weights compared to the ad libitum fed group. Broilers challenged with qualitative feed restriction (fed mash only) from 1 to 7 wk had reduced breast meat yield at 42 and 49 d (Urdaneta-Rincon and Leeson, 2002). Gratta et al. (2019) observed that early and late feed restriction (80%) from 13 to 22 d of age and 23 to 37 d of age impaired the growth performance of broiler chickens. An experiment conducted by Hassanabi and Moghaddam (2006), showed that restricted group of birds fed a mixture of 50:50 rice hulls, with the supplementation of trace minerals and vitamins from 4 to 11 d of age had reduced serum thyroxin and average final body weight on d 11 compared with ad libitum fed group, but was compensated on d 42 of age. Similary, growth performance parameters such as feed intake and body weight were significantly reduced in 3 to 5 d old birds when fed 8 hours per day from 7 to 21, 14 to 28, 21 to 35 d of age than those in ad libitum group (Boostani et al., 2010). Carcass weight, breast weight and yield of broilers were significantly decreased in restricted birds from 7 to 21 d of age, which confirm the adverse effects of eary restriction on meat quality (Boostani et al., 2010). Englmaierová et al. (2021) showed that the reduction in diet level (20% and 30%) from 29 to 57 d of age negatively affected the dressing percentage and breast yield while the leg yield and muscle fibers increased with increasing restriction levels in cockrels.
Feed-restricted broilers may result in high levels of plasma corticosterone as a stress indicator (Soleimani et al., 2012; Najafi et al., 2018). Janczak et al. (2007) reported that feed restriction increased the plasma and fecal corticosterone in birds. There is also a possibility of corticosterone transfer from stressed layer hens into their eggs, thus altering the growth and welfare of chicks (Janczak et al., 2007). Najafi et al. (2015) noted a significant linear relationship between plasma circulating corticosterone, H/L ratio, dopamine concentrations, and feed restriction. Aside establishing feed restriction as a chronic stress inducer, Yan et al. (2021) observed alteration in the gut microbiota composition in response to severe feed restriction. Hangalapura et al. (2005) revealed that severe feed restriction promoted reactive oxygen intermediates and decreased the relative weight of immune organs (spleen and bursa). Other stress behaviors associated with feed restrictions include object pecking, increased sitting, lying, decreased standing and feeding, and increased H/L ratio, which impeded growth rate and immune functions (Sandilands et al., 2005; Bowling et al., 2018; Trocino et al., 2020).
There is extensive evidence that feed restriction can result in physiological stress responses (De Jong et al., 2002; Janczak et al., 2007). De Jong et al. (2002) observed an increased in body temperature and a clear repeated sound in the heart rate of feed-restricted birds, whereas such a rhythm was blunted in ad libitum-fed birds. Feed-restricted broilers beyond 4 days had lowered counts of red blood cells, hemoglobin, and mean cell heamoglobin concentration compared to birds fed ad libitum (Bratte, 2011). Feed restriction decreased thyroid hormone triiodothyronine, thyroxine, and albumin in broiler chickens (Azis et al., 2012). Yang et al. (2010) reported a decrease in SOD activity, swollen mitochondria, and reduced cristae liver in feed-restricted groups, which may affect lipid metabolism in chickens. In addition, feed restriction significantly altered glucose, albumin, chlorine, ALT, and cholesterol in birds (Demir et al., 2004). Activities of jejunal alkaline phosphatase and pancreatic trypsin, amylase, and lipase were reduced significantly in response to feed restriction (Palo et al., 1995). Significant changes of blood biochemical indices caused by feed restriction implies that feed restriction poses a serious stress on chickens during the growth period (Rajman et al., 2006).
Stocking density (SD) is a critical environmental factor, identified as one of the causes of physiological stress among poultry species which has become a widespread concern (Li X. M. et al., 2019). SD has serious implications for the broiler industry because profits can be maximized as the number of birds per unit area increases. It is possible that assigned SD are focused on profit maximization at the expense of chickens' health (Estevez, 2007). SD directly influences the health, welfare, and performance of birds. With the rapid growth rate of present-day broilers, high SD is highly associated with leg deformities such as footpad dermatitis, lameness, hock burns, and abnormal gaits (Bilal et al., 2021). In layers, high SD has been confirmed to increase eggshell lightness, broken eggs, reduced laying rates, shell color, strength, thickness, and relative weight (Wang et al., 2020). Previous studies have reported that SD stress can affect the performance and behavior of chickens (Eugen et al., 2019; Li W. et al., 2019). Behaviors such as increased anxiety (Li W. et al., 2019), fearfulness (Eugen et al., 2019), cannibalism (Turkyilmaz, 2006), decreased locomotion, ground pecking and preening patterns (Hall, 2001), decreased feed intake, walking and drinking (Ibrahim et al., 2018), increased resting (Son, 2013) are associated with high SD. On growth performance, increasing SD above 30 kg of BW/m2 negatively affected live performance (Dozier et al., 2006) growth rate, feed consumption, and feed conversion of chickens at 28 d of age (Dozier et al., 2006). Another study concluded that increasing the SD rate from 28 to 40 kg of BW/m2 had negative effects on the performance and welfare of broiler chickens (Abudabos et al., 2013). High density stocked birds had lower body weight and high levels of plasma corticosterone which indicated stress and impaired welfare in birds (Eugen et al., 2019). Tong et al. (2012) observed a linear decrease in individual body weight and feed intake with increasing SD from 1to 28 d of age in a local chicken breed. On the contrary, body weight increased with increasing SD in chickens raised from d 1 to 10 as reported by Guardia et al. (2011). The findings of Guardia et al. (2011) showed that broilers' body weight was not affected by SD from d 10 to 24 and d 24 to 32; however, from d 32 to 39, high SD adversely affected broilers' body weight and feed conversion ratio. More findings on broilers affirmed that high SD decreased body weight and overall performance during the grower and finisher phases (Magnuson et al., 2020).
Moreover, SD significantly affects physiological stress indicators of broiler chicks. As SD increased, stronger stress responses were seen, including poor performance (Qaid et al., 2016). High SD was associated with decreased locomotor activity and increased H/L ratio, bursa weight, and oxidative stress (Simitzis et al., 2012). Another evidence showed that heterophils, H/L ratio, and serum corticosterone were greater in birds stocked at 10 birds/m2 than in those stocked at 6–7 birds/m2 (Kang et al., 2016). Elevation of hemoglobin was clearly seen in the high-density-stocked group due to overcrowding stress. Less oxygen is supplied when birds are overcrowded, leading to hypoxia, a stimulus for erythropoietin production, resulting in erythropoiesis in stressed chickens (Lokhande et al., 2009). Previous literature showed a positive correlation between SD and physiological stress response (i.e., stress indicators) (Bozkurt et al., 2008). High SD challenged hens experienced a significant increase in plasma insulin and glucose levels (Mirfendereski and Jahanian, 2015). Lallo et al. (2012) observed that plasma protein and rectal temperature increased with increasing SD. Blood biochemical parameters such as Ca, phosphorus (P), potassium (K), blood glucose and AST were found to decrease with increasing SD (Pandurang et al., 2011; Lallo et al., 2012; Mirfendereski and Jahanian, 2015). Özbey (2007) also established that SD can affect blood biochemical indices and antibody titers. Chegini et al. (2018) specified that plasma immunoglobulins, corticosterone, and nitric oxide concentrations were higher in chickens stocked at a high density compared to those at normal density. It has been previously studied by Cengiz et al. (2015) that SD stress can activate the HPA axis in the affected birds and thereafter increase corticosterone, prompting the release of pro-inflammatory cytokines. Elevated SD from 28 to 40 kg BW/m2 stimulated a state of hemodilution and a decrease in packed cell volume (PCV) in broiler chickens (Abudabos et al., 2013).
High SD has also been reported to affect carcass traits and meat quality (Li W. et al., 2019). Broiler chickens stocked at a high density had significantly reduced breast muscle yield, tibial length, tibial width, and tibial weight. Elevated SD decreased breast filet weight and its relative yield and breast tender weight (Dozier et al., 2006). High density stocked birds had reduced tibia quality compared to low-density stocked ones (Wang et al., 2020). In addition, Wang et al. (2014) observed a reduction in drip loss of broilers breast muscle, triggered by high SD, while Pekel et al. (2020) reported that the length of breast filet was significantly increased among stressed broilers. Drip loss can show quality deviations as the volume of drip loss is an indicator of oxidative stress in muscles (Wang et al., 2014). Other recent findings showed high drip loss and breast muscle redness shortly after slaughter in the high SD birds compared to other treatments groups (Wu et al., 2020). Bilgili and Hess (1995) observed that carcass quality was reduced in female chickens subjected to SD stress while breast filet yield was reduced in male chickens. The mRNA expression level of myostatin, a protein involved in the regulation of fat mass hens was increased with increasing SD in broiler breeder hens (Li X. M. et al., 2019). Conversely, some previous studies have revealed that SD stress did not affect meat quality or carcass traits in chickens (Simitzis et al., 2012; Tong et al., 2012; Goo et al., 2019). Variation in results might be due to differences in production systems.
Chickens stocked at high densities may be susceptible to severe oxidative stress (Simitzis et al., 2012; Wu et al., 2018). Metabolic alterations resulting from SD stress can cause excessive production of ROS that leads to oxidative damage of biomolecules (Fang et al., 2002). Antioxidant enzymes such as SOD, catalase (CAT), glutathione reductase and glutathione peroxidases (Gpx) utilize synergistic actions in scavenging free radicals and ROS (Fang et al., 2002). Elevated SD increased malondialdehyde (MDA) significantly while the SOD and CAT activity remained unaffected (Jobe et al., 2019). As reported by Simsek et al. (2009), high SD increased serum MDA level and reduced GSH-Px but did not change serum CAT and GSH levels. A current study found that high SD significantly decreased total antioxidant capacity, GPx activity and increased plasma MDA (Miao et al., 2021). High SD elevated glutathione concentration in the plasma, breast, and thigh of growers but decreased it in the liver and thigh of the finishers (Magnuson et al., 2020). The detailed characteristics of the included studies on SD are summarized in Table 3.
Environmental pollutants such as Arsenic (As), copper (Cu), ammonia (NH3), hydrogen sulfide (H2S) can induce oxidative stress when inhaled by chickens (Hu et al., 2018; Wang et al., 2018; Xing et al., 2019). These pollutants can inhibit antioxidant enzymes like CAT and GPx (Sawyer et al., 2002). Wang et al. (2018) reported that exposure to As and Cu provoked an imbalanced oxidant status in the intestines of chickens which may impair the functioning of the GIT (Wang et al., 2018). Higher MDA and hydroxyl radical content and reduced CAT and GPx were seen in chickens exposed to Cu and As, which affirmed the occurrence of lipid peroxidation of the heavy metals and a close connection with oxidative stress (Zhao et al., 2018). Similarly, Nie et al. (2020) observed that As and Cu could prompt redox imbalance in the chicken brain, accompanied by apoptosis in brain cells and neurological damage. Other negative effects of As and Cu were alteration of the inflammatory and immune-regulated cytokines, causing toxicity in the chicken thymus (Liu et al., 2018). While Cu is needed for several cellular processes and enzymes in the organisms, an excess amount produces cellular damage leading to several adverse effects and diseases (Öhrvik et al., 2017). Experimental studies suggested that exposure of chickens to excessive Cu triggered oxidative stress.
Ammonia (NH3) is one of the environment pollutants of greatest concern and its emissions play a vital role in the formation of secondary inorganic aerosols (Artíñano et al., 2018). The rise in NH3 concentrations depends on the housing systems. Concentrations of NH3 are usually higher in aviaries and floor systems because manure is not regularly removed compared to furnished cages systems (David et al., 2015). Evidence showed that an upsurge of NH3 could adversely affect the health, behavior, and performance of chickens, and can predispose them to respiratory infections and pathogens (Yahav, 2004; David et al., 2015). An increase in NH3 levels triggered inflammation in broiler chickens' respiratory tracts. 15 ppm NH3 altered tracheal microbiota composition that led to the tracheal injury, enhancing their susceptibility to pathogens (Zhou Y. et al., 2021). Wang et al. (2019a) found that NH3 exposure could cause oxidative stress, intestinal inflammation and disrupt the chicken intestine integrity. Previous findings have shown that a high concentration of NH3 increased serum biochemical indices such as AST, ALT, AKP, GGT, CK and ALB in chickens, which signified a negative effect on the immune organs (Lu et al., 2017; Shah et al., 2020). Liu et al. (2020) indicated that exposure of chickens to a high concentration of NH3 disrupted the ecological balance of the lung tissue flora, increased some pathogenic bacteria such as Escherichia/Shigella and damaged their lung tissue.
Hydrogen sulfide has been widely researched as an environmental stressor that causes respiratory and immune damage in chickens (Li X. et al., 2020; Yin et al., 2020; Song et al., 2021). Results showed that increasing H2S concentrations decreased cell viability, and differentially inhibited neutrophil viability and neutrophil extracellular trap (NET) (Yin et al., 2020). Neutrophils perform immunity roles through the formation of NET (Selders et al., 2017). H2S damaged broiler chicken's trachea by dysregulating necroptosis and apoptosis (Li X. et al., 2020). A previous study revealed that H2S induced oxidative stress in the chicken trachea, indicated by increased levels of hydrogen peroxide (H2O2) and MDA, and decreased SOD, GSH, and CAT activity, which resulted in inflammatory injury mediated by FOS/IL8 signaling (Chen et al., 2019). Likewise, H2S decreased CAT and total antioxidant capacity (T-AOC) activities and increased H2O2 and nitric oxide content in broilers' myocardial region and in the bursa (Hu et al., 2018; Wang et al., 2019b). Chi et al. (2018) suggested that H2S triggers inflammatory responses and oxidative stress by enhancing ROS production, then impairing energy metabolism and immune system in the chickens. Aside from cellular and oxidative damage, concentrated H2S negatively affects growth performance parameters such as average daily gain and body weight, the carcass yield, and increased water loss rate of breast and leg (Wang et al., 2011).
The vitamin requirement of poultry is increased during stress conditions, and poultry birds do not synthesize sufficient vitamins at this period. Dietary supplementation of vitamins has been widely accepted for growth performance and maintenance in chickens. We further discuss the roles of different vitamins during environmental stress in chickens.
Vitamin A is a fat- for bone health and development soluble vitamin, which when added to the diet, is converted to retinoid within the intestine, liver, and other tissues (Huang et al., 2018). Vitamin A is obtained from plant diets as provitamin A carotenoids while from animal sources as retinoids. Forms of provitamin A carotenoids includes α-carotene, β-carotene and βcryptoxanthin (Maqbool et al., 2018). Emerging literature reported that vitamin A is involved in the development of the immune system and plays an essential role in cellular and humoral immune response (Aydelotte, 1963). Vitamin A is involved in the proliferation and differentiation of epithelial cells (Thomas et al., 2005). Vitamin A has been established to enhance normal intestinal barrier function, regulates the composition of the intestinal bacteria and subsequently helps in maintaining innate immunity and gut integrity in humans (Quadro et al., 2000; Thurnham et al., 2000). Pang et al. (2021) showed that the dietary supplementation of Vitamin A significantly changed the gut microbiota and ameliorated a chronic gastrointestinal tract inflammation know as Ulcerative colitis in mice. Supplementation of Vitamin A promoted the restoration of the damaged small intestine in rats (Xia et al., 2019) while Vitamin A deficiency altered Bacteriodes/Firmicutes ratio, and decreased rates of short chain fatty acid (Tian et al., 2018).
In poultry diets, the importance of vitamin A has been given wide attention because of production losses associated with vitamin A deficiencies, including growth dysfunction, weakness, ruffled feathers, decreased egg production, and immune depression (Sklan et al., 1994; Shojadoost et al., 2021). Vitamin A insufficiency often leads to serious infection, through substitution of many secretory epithelia cells with non-secretory ones (Aydelotte, 1963). Depression of the chick antibody production and proliferative responses of T lymphocytes have been associated with lack of Vitamin A in the chicks diet (Sklan et al., 1994). Vitamin A deficiency was also associated with the significant reduction in ovarian stroma and follicles weights (Chen et al., 2016). On the other hand, previous works have established that excessive Vitamin A has adverse effects on chicken health (March et al., 1972; Lessard et al., 1997). Excessive or insufficient vitamin A increased vulnerability of the chicks to Eschericia coli infection, followed by depressed immune responses (Friedman et al., 1991). Yuan et al. (2014) observed that the addition of vitamin A at 135,000 IU/kg differentially decreased peripheral blood T-cell production at week 24, adversely affected the liver function and reproductive performance in broilers breeders. Similarly, Sklan et al. (1994) revealed that the supplementation of Vitamin A above 6,000 IU/kg negavely affected the immune system of broilers.
The roles of vitamin A have been studied in heat-stressed chickens (Lin et al., 2002; Kucuk et al., 2003) but sparsely studied in other types of environmental stress. Vitamin A has been supplemented in HS challenged birds and found to be beneficial in terms of laying performance and immune function in layer chickens (Lin et al., 2002). Lin et al. (2002) found that high level of vitamin A supplementation (9,000 IU/kg) improved the feed intake and laying rate of heat-stressed hens compared to the control group (3,000 IU/kg). Likewise, Abd El-Hack et al. (2019) observed that Vitamin A addition at 8,000 IU/kg significantly increased the feed intake and feed conversion ratio of layers raised under high temperature. Dietary supplementation of vitamin A in combination with zinc has been suggested as an effective way of managing heat-stress-related production depression in broilers (Kucuk et al., 2003).
It is required to supplement B vitamins in chickens' meal to ensure maximum production potential. Different forms of vitamin B such as riboflavin, pantothenic acid, folate, B12 and niacin improved production performance of chickens fed with low density diet (Suckeveris et al., 2020). Vitamin B1 (thiamine) can be classified as part of the anti-stress vitamins due to its strong antioxidant ability (Lukienko et al., 2000). Miladinović et al. (2021) affirmed the antioxidant and anti-inflammatory properties of vitamin B1 in quail brains treated with organophosphate pesticides. Vitamin B1 deficiency caused neurological damage and severe cardiovascular infection because of excessive production of ROS in the brain (Miladinović et al., 2021). Thiamine plays a key role in glucose metabolism and functioning of the tissue, organ, and body (Kerns et al., 2015). Owing to its multidimensional roles in biochemical reactions, thiamine is essential for body growth and development (Lonsdale, 2019). Vitamin B3 (niacin) helps in the prevention of metabolic diseases in animals. Supplementation of 6–12 g niacin has been established to defend dairy cattle from chronic HS (Panda et al., 2017). Niacin is an essential nutrient needed by the body for proper metabolism and digestion, and also support blood circulation and the brain (Moneva et al., 2008). Riboflavin is involved in the reduction of oxidized glutathione and its deficiency led to an increase in lipid peroxidation and a decrease in antioxidant ability (Saedisomeolia and Ashoori, 2018). Previous studies have confirmed riboflavin as a strong antioxidant that protect the body against oxidative stress (Wang et al., 2014). Kumar et al. (2020) showed that a combination of riboflavin and selenium nanoparticles can alleviate high temperature effects and arsenic pollution in fish. An additional study revealed that riboflavin lessens oxidative stress and tissue damage in diabetic mice (Alam et al., 2015). Many studies involved the in ovo feeding of vitamin B in chickens (Momeneh and Torki, 2018; Teymouri et al., 2019). Teymouri et al. (2019) suggested that in ovo feeding of the vitamin B12 positively influenced the performance of broiler chickens. Momeneh and Torki (2018) recorded that in ovo injection of vitamin B6 and B12 ameliorated the ethanol induced oxidative stress in chicken embryos.
Another type of vitamin B known as folate (vitamin B9), is an essential component of an healthy diet, known to improve cardiovascular diseases through its homocysteine lowering potential and necessary for normal body functioning (Nakano et al., 2001; Patel and Sobczyńska-Malefora, 2016). Folic acid is the synthetic form of folate and is supplemented in diets to increase folate levels (Powers, 2007). It exhibits similar antioxidant effect as vitamin C and E (Gursu et al., 2004; Sinbad et al., 2019). Gouda et al. (2020) stated that the dietary supplementation of the combination of folic acid and vitamin C (1.5 mg/kg) significantly improved the growth performance, immune status, blood biochemistry parameters, and antioxidant capacity of broilers raised under HS conditions. In quail, dietary supplementation of folic acid (2 mg/kg) alleviated the negative effects of CS on growth performance, lipid peroxidation, and serum concentrations of homocysteine (Sahin et al., 2003a). In mouse, administration of folic acid ameliorated the inhibitory effects of HS on the pre-implantation embryos development (Koyama et al., 2012), served a protective role by reducing the CAT, SOD, and glucose level (Padmanabhan et al., 2018; Mutavdzin et al., 2019). The ameliorative effects of vitamin B in chickens challenged with environmental stress are rarely studied. Given the importance of vitamin B, it may be worthwhile to supplement chickens' diet with vitamin B especially during stress conditions.
Vitamin C is an alternative functional nutrient adopted for stress amelioration in farm animals because of its antioxidant ability. Vitamin C is water-soluble in form. It prevents cells from oxidative damage and improves cell immune function (Buettner, 1993; Chambial et al., 2013). Under normal conditions, poultry synthesizes sufficient vitamin C needed to meet physiological needs; however with HS exposure, vitamin C requirement increases and the body's capacity becomes inadequate (Attia et al., 2016). Thus, it is recommended that under stressful conditions, poultry diets should be supplemented with vitamin C to augment the body's synthesis. Several studies have investigated the effect of vitamin C supplementation in chickens challenged with HS. Attia et al. (2009) showed that vitamin C supplementation improves the humoral immune response and eradicates the negative effects of HS on broiler chickens. Supplementation of vitamin C also improved growth performance in heat-stressed broiler chickens under standard animal density conditions (Saiz del Barrio et al., 2020). In a study conducted by Ferket and Qureshi (1992) on the efficacy of different vitamins and electrolyte treatments in drinking water for heat-stressed broilers, the authors observed that vitamin C treatment increased body weight gain and feed conversion by 3 and 5%, respectively and reduced the rate of HS induced death by 63%. In addition, dietary vitamin C supplementation for HS-challenged broiler chicks positively affected the relative weights and immune status of bursa, thymus, and spleen (Naseem et al., 2005). Also, supplementation of vitamin C at 200 ppm was shown to be beneficial for improving weight gains, nutrient digestibility, carcass traits, and bone resistance and helped to reach the full genetic potential of commercial broilers (Lohakare et al., 2005). Similarly, Abidin and Khatoon (2013) found that vitamin C ameliorated heat stress-induced complications such as infertility, poor semen quality, poor immunity, and mortality in birds. Related functions have been observed in vitamins C and E; both positively affected the immune system by improving the production of antibody, macrophage activity, and humoral immunity in chickens. It is proposed that the combination of vitamins C and E in broiler chicken diets might improve their immune system functions and performance (Shakeri et al., 2020).
Furthermore, results revealed that vitamin C alleviated the adverse effects of CS and HS in poultry performance by decreasing synthesis and secretion of corticosteroids (McDowell, 1989; Kutlu and Forbes, 1993). A previous study observed that the combination of 250 mg vitamin C and 400 μg chromium per kg of diet prevented rise in blood glucose, total cholesterol induced by CS (Khukhodziinai et al., 2021). Similar diet administered by Khukhodziinai et al. (2021) resulted in optimum performance in layers challenged with CS (Sahin and Sahin, 2001). Rajabi and Torki (2021) found that the dietary supplementation of 240 mg/kg vitamin C and zinc improved the egg quality of layers challenged with cold temperature between 13 and 15°C. Sahin and Sahin (2001) concluded that the depressive effect of CS in chickens can be alleviated by vitamin C and chromium. Inconsistent findings have also been reported on the modulatory roles of vitamin C in chickens subjected to high SD (Jahanian and Mirfendereski, 2015; Shewita et al., 2019). Jahanian and Mirfendereski (2015) found that 500 mg/kg vitamin C supplementation had no significant effect on the growth performance of broiler chickens subjected to high SD but decreased plasma and yolk MDA. A recent study by Yu et al. (2021) observed that 200 mg/kg had no marked effects on the growth performance, meat quality, and intestinal permeability of broiler chickens stocked at high density (12 birds/m2). Shewita et al. (2019) reported that 200 mg/kg vitamin C improved final body weight, feed intake and decreased mortality in broilers reared in high SD (15 birds/m2). The inconsistency in the findings might be attributed to the strain of the animal or different SD used (Yu et al., 2021). Vitamin C was also reported to decrease plasma corticosterone caused by high SD in broilers (Mirfendereski and Jahanian, 2015). Vitamin C could ameliorate the negative effects of environmental stress by increasing the antioxidant ability of the birds (Jahanian and Mirfendereski, 2015; Zangeneh et al., 2020).
Vitamin D is a fat-soluble vitamin that is gotten by either exposing the skin to sunlight or by dietary supplementation (Lamberg-Allardt, 2006). Vitamin D is available in animal-derived (vitamin D3, cholecalciferol) and plant-derived (vitamin D2, ergocalciferol) forms (Jones, 2018). It is transported in the blood by the vitamin D binding protein into the liver for hydroxylation to 25-hydroxyvitamin D3 (25(OH)D3), which can then be converted to its active metabolite 1,25(OH)2D in numerous cell types (Christakos et al., 2010). Vitamin D plays a role in the maintenance of Ca and P for bone health and development (Lamberg-Allardt, 2006). Also, it is involved in sustaining normal immune function and mediates between innate and adaptive immunity by influencing vitamin D receptors (VDR) (Li et al., 2014). Vitamin D can impact the gut function by binding to its VDR and maintain the epithelia integrity of the organism (Fakhoury et al., 2020). Vitamin D has been confirmed as a prohormone due to its involvement in the physiology of stressed birds (Huff et al., 2000). The addition of vitamin D in turkey diets improved their body weight, disease resistance, and white blood cell counts despite being challenged with dexamethasone (DEX) and Escherichia coli repeatedly (Huff et al., 2000). In mice, vitamin D3 alleviated oxidative stress induced by lipopolysaccharide challenge through regulation of antioxidant and oxidant exymes gene (Xu et al., 2015). Geng et al. (2018) also affirmed that vitamin D3 supplementation protected laying hens against immunological stress caused by Escherichia coli lipopolysaccharide challenge. A recent study revealed that dietary supplementation of an active metabolite of Vitamin D3 for layer chickens reversed the effect of high SD on T-AOC, MDA content, and antioxidative catalase activity (Wang et al., 2021). Additional evidence suggests that dietary vitamin D is effective in reducing the severity of lameness caused by environmental stress through tibial dyschondroplasia reduction (Nääs et al., 2012). Vitamin D supplementation promotes gene expression related to antioxidation and serves as therapy in cold-related cases (Lei et al., 2017). Other studies have established the antioxidant and anti-inflammatory roles of vitamin D in rat (Manna et al., 2017; El-Boshy et al., 2019). No study has recorded the protective roles of vitamin D in chickens challenged with different forms of environmental stress.
Vitamin E is a chain-breaking antioxidant that protects the membranes of cells from being scavenged by lipid peroxyl radicals (Gao et al., 2010). Supplementation of vitamin E in chicken diets is necessary because they cannot synthesize vitamin E on their own under normal and stress conditions (Shakeri et al., 2020). Vitamin E supplementation can enhance immune system and decrease influence of corticosterone induced by stress, aside protecting cells against oxidative damage (Attia et al., 2016). Min et al. (2018) found that dietary supplementation with vitamin E increased body weight and GSH-Px mRNA expression of breeder roosters challenged by oxidative stress when compared to the control treatment, suggesting that vitamin E could improve antioxidant ability and immune performance in oxidative-stressed chickens (Min et al., 2018). An additional study indicated that vitamin E supplementation improved the performance of broiler chickens by alleviating the oxidative stress induced by DEX treatment (Singh et al., 2006). Aside from growth performance, the effectiveness of vitamin E as an anti-stress substance has also been proven by Niu et al. (2009) who reported that heat-stressed broiler chickens had improved immune response when fed with vitamin E supplemented diets (Niu et al., 2009). A different study affirmed that vitamin E helps in promoting humoral immune response which may influence the growth of lymphoid cells and immunocompetence of chicks (Surai et al., 2019). In layers, Puthpongsiriporn et al. (2001) reported that dietary supplementation of vitamin E at 65 IU/kg diet enhanced higher egg mass, egg quality, and egg yolk formation during HS. Further results revealed that lipid peroxidation in yolk and plasma resulting from HS can be ameliorated with 65 IU/kg of vitamin E supplementation (Puthpongsiriporn et al., 2001). Research on breeders and cockrels indicated that an increase in vitamin E supplementation in animal diet increased resistance to various forms of stress (Qureshi et al., 2018). A previous report concluded that dietary supplementation of 250 mg vitamin E/kg of diet provided during and after HS is effective for alleviating, the symptoms and adverse effects of chronic HS in chickens (Bollengier-Lee et al., 1999).
In addition, vitamin E supplementation in the diet of broilers exposed to CS caused a significant reduction in ascites-related mortality (Akşit et al., 2008; Qureshi et al., 2020). Increase in antibody production was observed in broiler chickens challenged with extreme cold stress (5 ± 2°C) after vitamins fortification (Sandhu et al., 2013). Qureshi et al. (2020) found that the intestinal health of broiler chickens subjected to CS was improved with the addition of 250 mg/kg vitamin E in their diet. Correspondingly, pathological changes on the chicken intestine (duodenum, jejunum and ileum) induced by oxidative stress in response to virus infection were fully alleviated by addition of vitamin E (Rehman et al., 2018). Birds fed vitamin E had a significantly lower LPS-induced inflammatory response, as indicated by lower IL6 RNA expression levels, suggesting a protective effect from natural-type vitamin E when a chicken encounters a bacterial component (Kaiser et al., 2012). The roles of dietary supplementation of vitamin E in ameliorating the negative effects of SD in chickens have been studied. 200 mg/kg vitamin E diet improved the growth performance, liver functions, and decrease the pathogen counts in broilers stocked at high density (Desoky, 2018). Selvam et al. (2017) reported that vitamin E positively influenced the body weight, feed conversion ratio, liver GSH, and MDA levels of broiler chickens reared under high SD. In a study conducted by El-Gogary et al. (2015), vitamin E protected the lymphoid organs such as spleen, bursa, thymus from the negative effects of high SD by enhancing the organs to produce more lymphocytes that aided in improving the birds immunity. The positive effects of dietary vitamin supplementation in chickens challenged with different forms of environmental stress are summarized in Table 4.
Table 4. Summary of the positive effects of dietary vitamin supplementation in chickens challenged with different environmental stress.
In poultry nutrition, the application of vitamins in combination with micro minerals is considered more effective in combating environmental stress (Asli et al., 2007; Horváth and Babinszky, 2018). It had been reviewed that vitamins combated ROS, promoted antioxidant enzymes, and attenuated lipid peroxidation when supplied to diets in quantities as; vitamin A (9000–15,000 IU/kg diet), vitamin E (150–500 mg/ kg diet), and vitamin C (150–500 mg/kg diet) (Horváth and Babinszky, 2018).
During HS conditions, the combination of Vitamin E along with Zinc (30–60 mg/kg) demonstrated synergistic effects on the growth and production performance of both broilers and laying hens (Shakeri et al., 2020). This was attributed to the enhanced anti-oxidative properties derived from such combinations against HS effects. In a study performed to investigate the possible interactions between zinc sources and vitamin E levels, it was revealed that supplying a mixture of 60 mg/kg of zinc-amino acid complex, and 50 IU/kg of vitamin E significantly improved the body weight gain, feed conversion ratio, villus length, and villus to crypt depth ratio in heat-stressed broilers, providing an overall enhancing effect on the growth performance and intestinal health of broiler chickens (De Grande et al., 2021). Also, dietary supplementation of zinc-L-selenomethione with vitamin E (120 mg/kg feed) was reported to improve egg characteristics in old breeders, and the hatchability traits of young breeders (Urso et al., 2015). Combination of zinc (30 mg/kg diet) and Vitamin B6 (8 mg pyridoxine/kg diet) to 28 weeks old Hy-Line laying hens synergistically improved the feed conversion ratio, egg production, eggshell weights, haugh unit, and plasma contents of Ca and phosphorous concentrations (Kucuk et al., 2008). Supplementing 1,000 mg/kg betaine, 200 mg/kg ascorbic acid, and 150 mg/kg α-Tocopherol acetate, along with their possible combinations to dual-purpose hens under HS improved the production parameters (body weight, body weight gain, laying rate, survivability, egg mass, and feed intake) of hens (Attia et al., 2016). The co-administration of 1,200 IU/kg retinol, 30 mg/kg ascorbic acid and 50 mg/kg α-Tocopherol ameliorated HS and enhanced pullets productivity under thermal stress conditions (Sinkalu and Ayo, 2016). Studies have also shown that different vitamins and minerals can have beneficial synergistic effects on chickens exposed to environmental pollutants (Kalavathi et al., 2011; Hashem et al., 2019). Combination of 200 mg/kg vitamins E and 120 mg/kg zinc exerted a synergistic effect by protecting the jejuna mucosa of broiler chickens polluted with silver particles against oxidative stress (Song et al., 2017). Hashem et al. (2019) showed that the mixing of 0.5 mg/kg selenium and 100 mg/kg vitamin E together alleviated the oxidative stress caused by an environmental pollutant (100 mg/kg cadmium) in broilers.Vitamins C and E can also function synergistically to alleviate the negative effects of copper toxicity. The combination of 250 mg/kg vitamin C and 250 mg/kg vitamin E mitigated oxidative stress and reduced renal toxicity in the chickens challenged with copper toxicity (300 mg/kg) (Hashem et al., 2021). 200 mg/kg vitamin C and 300 mg/kg vitamin E also mitigated the toxic effect of arsenic in broiler chickens (Kalavathi et al., 2011). Vitamin C, E, Zn, and Cu have been recognized as interconnected antioxidant defense systems that protect the cells from oxidative damage (Evans and Halliwell, 2001). Altogether, synergistic effects have been reported on the growth performance, production indices, immunity, antioxidant status, and intestinal health of chickens supplied with vitamins under stressful conditions (Attia et al., 2016).
Additionally, there have been instances of antagonism where the combination of ascorbate and α-tocopherol delayed oxymyoglobin and lipid oxidation (Yin et al., 1993). An earlier report had shown that different levels of vitamins A and D interacted to impair skeletal development in poultry (Rohde et al., 1999). At high concentrations, it is explained that excess vitamin A may impede Vitamin D absorption, transport, actions, conversion to its active form and could further stimulate its metabolic degradation (Rohde et al., 1999). Poults fed normal vitamin D levels (900 ICU/kg, NRC estimated requirement) with high vitamin A (400,000 IU/kg) suffered from severe lameness, growth depression, lowered bone mineral content, and rachitic like condition; but feeding normal vitamin A levels (4,000 IU/kg, NRC estimated requirement) with high vitamin D (900,000 ICU/kg), caused hypervitaminosis D, with symptoms of renal tubular mineralization, and moderate growth depression (Metz et al., 1985). In another study, feeding Vitamin D2 with increasing amounts of vitamin A (retinyl acetate) caused a progressive but significant decline in total bone ash, and impairments on intestinal and bone functions (Rohde et al., 1999). Also, broilers fed 1-α-HydroxSycholecalciferol [a synthetic form of cholecalciferol (vitamin D3)] showed a linear decline in plasma cholecalciferol levels with increasing Ca inclusion levels, suggesting the potential of 1-α-Hydroxycholecalciferol supplementation to cause Ca toxicity or antagonism during the growing phase (Warren et al., 2020). In 87-wk-old laying hens, supplementing basal diets with varying levels of vitamins A (0, 7,000, and 14,000 IU/kg) and Vitamin K3 (0, 2.0, and 4.0 mg/kg) improved the eggshell quality, yolk color and antioxidant enzymes status in the eggshell gland of aged laying hens (Guo et al., 2021).
Moreso, supplying vitamins at supraphysiological doses may also incur detrimental effects. Vitamin D3 toxicity is associated with an unregulated increase in plasma Ca and P levels, mineralization of tissues and organs, decrease in bone calcification, structural damage, and organs dysfunction including cardiac and renal failure (Morrow, 2001; Pande et al., 2015; Kumar et al., 2017). Similarly, an in vitro experiment using chicken bone marrow-derived mesenchymal stem cells also showed that these stem cells were highly sensitive to exogenous calcitriol and excess doses inhibited mineralization and caused loss of cell proliferation (Pande et al., 2015). Altogether, the mode of action of vitamins in potentiating these effects is still an avenue to be explored, to gain a better understanding of the application and utilization of vitamins in environmental stress conditions.
Despite the demonstrated negative effects of environmental stress in chickens, environmental stress at the early stage of growth might be beneficial to chickens. We discussed the positive effects and cross adaptation in chickens. A previous study revealed that subjecting female broilers to feed restriction (60%) at 4, 5 and 6 d of age improved heat tolerance in subsequent life (Zulkifli et al., 2000). Short-term feed restriction before HS challenge can mitigate HS negative effects on broilers. Feed restriction of broiler chickens (75 and 50%) from 4 to 8 weeks of age, improved heat resistance of broiler chickens when exposed to 40°C at 8 weeks old (Abudabos et al., 2013). Feed restriction has been one of the effective ways to promote heat resistance in broilers because the feed deprivation decreased heat production (Lin et al., 2006). A previous study also affirmed that exposure of broilers and layers to feed restriction improved humoral immune response and their physiological ability to withstand subsequent acute HS (Mahmoud and Yaseen, 2005). Early feed restriction (80%) from 13 to 21d of age reduced and controlled muscle fiber degeneration compared to ad libitum fed chickens (Radaelli et al., 2017). Tsiouris et al. (2014) observed that feed restricted birds had protective effects against necrotic enteritis associated with the alteration of the intestinal ecosystem. 85% quantitative and qualitative feed restriction exerted beneficial effects on some cytokines in broiler chickens (Jang et al., 2009). Feed restriction has also been used to improve feed efficiency, manipulate growth performance in chickens and reduced metabolic diseases such as ascites (Özkan et al., 2006; Trocino et al., 2020).
Emerging studies have shown the possibility of enhancing thermotolerance in chickens exposed to high temperature during pre and post hatching (Yahav and McMurtry, 2001; Al-Zhgoul et al., 2013). Yahav and McMurtry (2001) observed that exposure of 3d old chicks to high temperature (36 and 37°C) reduced triiodothyronine (T3) concentration and enabled chicks to withstand heat stress later. Similarly, thermal manipulation of chicken eggs at 38.8°C for 18 h/day during embryonic development (10–18 d), followed by high temperature challenge (41°C) for 6 h daily at the early and late growing stage significantly decreased the concentrations of T3 and improved the growth performance of the treated chicks (Al-Zhgoul et al., 2013). The reduction in T3 observed in the above studies implies lowered metabolic rate and improved thermotolerance acquisition (Yahav, 2000). Yahav et al. (2004) also revealed that thermal manipulation at 39°C for 3 h during the late embryogenesis stage (16–18) improved thermotolerance acquisition and reduced the level of corticosterone of 3 d old chicks. The improved thermotolerance was characterized by increased expression of signaling protein (Saleh and Al-Zghoul, 2019), enhanced breast muscle yield, lowered stress status as indicated by reduced H/L ratio compared to the control group (Loyau et al., 2013) coupled with reduced levels of GPx, SOD, and catalase (Al-Zgoul et al., 2019). Application of pre or post hatch thermal manipulation during the development of thermal regulation system of broilers ameliorated the adverse effects of chronic HS on broilers in the early stage of life (Vinoth et al., 2015; Zaboli et al., 2017) through stimulation of physiological memory due to epigenic temperature adaptation approach (Yahav, 2009).
In addition, appropriate cold simulation has been shown to improve the survival rate of broilers (Shinder et al., 2002). Acute cold temperature exposure during the last stage of broilers embryogenesis improved their ability to adapt to CS in subsequent life (Shinder et al., 2009). Cold temperature stimulation from 8 to 42d result to cold acclimation, characterized by reduction of pro inflammatory cytokines caused by consequent CS (Su et al., 2018). Cold acclimation enhanced the immune system of birds in alleviating cold injury induced by CS (Su et al., 2020).
The adverse effect of environmental stress on chicken's health, welfare, and performance cannot be overemphasized. Environmental stressors can cause an upsurge in the bodily secretion of stress hormone which negatively affects growth and leads to mortality in severe cases. However, effective management techniques are key to raising healthy chickens and profit maximization in the poultry industry. To enhance chickens' adaptability under stress conditions, it is essential to understand the functions of different vitamins and appropriate dosage in chicken diets to alleviate stress. The synergistic effects of different vitamin and minerals could promote growth performance and reduce the effect of environmental stress in chicken.
FA: writing—original draft preparation and revision and editing of the manuscript. DA: conceptualization, review and editing, and funding acquisition. All authors have read and agreed to the published version of the manuscript.
This work was supported by Dalhousie University start-up grant and Natural Sciences and Engineering Council of Canada (NSERC).
The authors declare that the research was conducted in the absence of any commercial or financial relationships that could be construed as a potential conflict of interest.
All claims expressed in this article are solely those of the authors and do not necessarily represent those of their affiliated organizations, or those of the publisher, the editors and the reviewers. Any product that may be evaluated in this article, or claim that may be made by its manufacturer, is not guaranteed or endorsed by the publisher.
The authors would like to appreciate Victoria A. Uyanga for her contributions to the work.
Abd El-Hack, M. E., Abdelnour, S. A., Taha, A. E., Khafaga, A. F., Arif, M., Ayasan, T., et al. (2020). Herbs as thermoregulatory agents in poultry: An overview. Sci. Total Environ. 703:134399. doi: 10.1016/j.scitotenv.2019.134399
Abd El-Hack, M. E. A., Alagawany, M., Mahrose, K. M., Arif, M., Saeed, M., Arain, M. A., et al. (2019). Productive performance, egg quality, hematological parameters and serum chemistry of laying hens fed diets supplemented with certain fat-soluble vitamins, individually or combined, during summer season. Anim. Nutri. 5:49. doi: 10.1016/j.aninu.2018.04.008
Abdel-Moneim, A. E., Shehata, A. M., Khidr, R. E., Paswan, V. K., Ibrahim, N. S., El-Ghoul, A. A., et al. (2021). Nutritional manipulation to combat heat stress in poultry—a comprehensive review. J. Therm. Biol. 98:102915. doi: 10.1016/j.jtherbio.2021.102915
Abidin, Z., and Khatoon, A. (2013). Heat stress in poultry and the beneficial effects of ascorbic acid (vitamin C) supplementation during periods of heat stress. World's Poultr. Sci. J. 69, 135–152. doi: 10.1017/S0043933913000123
Abo Ghanima, M. M., Abd El-Hack, M. E., Othman, S. I., Taha, A. E., Allam, A. A., and Eid Abdel-Moneim, A. M. (2020). Impact of different rearing systems on growth, carcass traits, oxidative stress biomarkers, and humoral immunity of broilers exposed to heat stress. Poult. Sci., 99, 3070–3078. doi: 10.1016/j.psj.2020.03.011
Abudabos, A. M., Samara, E. M., Hussein, E. O. S., Al-Ghadi, M. Q., and Al-Atiyat, R. M. (2013). Impacts of stocking density on the performance and welfare of broiler chickens. Ital. J. Anim. Sci. 12:11. doi: 10.4081/ijas.2013.e11
Abu-Dieyeh, Z. H. M. (2006). Effect of chronic heat stress and long-term feed restriction on broiler performance. Int. J. Poultry Sci. 5, 1682–8356 doi: 10.3923/ijps.2006.185.190
Akinyemi, F. T., Bello, S.F., Uyanga, V.A, Oretomiloye, C., and Meng, H. (2020). Heat Stress and gut microbiota: effects on poultry productivity. Int. J. Poult. Sci. 19, 294–302 doi: 10.3923/ijps.2020.294.302
Akşit, M., Altan, Ö., Karul, A. B., Balkaya, M., and Özdemir, D. (2008). Effects of cold temperature and vitamin E supplementation on oxidative stress, Troponin-T level, and other ascites-related traits in broilers. Archiv Fur Geflugelkunde. 72, 221–230.
Alam, M. M., Iqbal, S., and Naseem, I. (2015). Ameliorative effect of riboflavin on hyperglycemia, oxidative stress and DNA damage in type-2 diabetic mice: Mechanistic and therapeutic strategies. Arch. Biochem. Biophys. 584, 10–19. doi: 10.1016/j.abb.2015.08.013
Allahverdi, A., Feizi, A., Takhtfooladi, H.A., and Nikpiran, A. (2013). Effects of heat stress on acid-base imbalance, plasma calcium concentration, egg production and egg quality in commercial layers. Glob. Veterinaria. 10, 203–207. doi: 10.5829/idosi.gv.2013.10.2.7286
Al-Zgoul, M. B., Sukker, H., and Ababneh, M. M. (2019). Effect of thermal manipulation of broilers embryos on the response to heat-induced oxidative stress. Poult. Sci. 98, 991–1001. doi: 10.3382/ps/pey379
Al-Zhgoul, M. B., Dalab, A. E. S., Ababneh, M. M., Jawasreh, K. I., Busadah, K. A., and Ismail, Z. B. (2013). Thermal manipulation during chicken embryogenesis results in enhanced Hsp70 gene expression and the acquisition of thermotolerance. Res. Vet. Sci. 95, 502–507. doi: 10.1016/j.rvsc.2013.05.012
Artíñano, B., Pujadas, M., Alonso-Blanco, E., Becerril-Valle, M., Coz, E., Gómez-Moreno, F. J., et al. (2018). Real-time monitoring of atmospheric ammonia during a pollution episode in Madrid (Spain). Atmos. Environ., 189, 80–88. doi: 10.1016/j.atmosenv.2018.06.037
Asli, M.M., Shariatmadari, F., Hosseini, S., and Lotfollahian, H. (2007). Effect of probiotics, yeast, vitamin e and vitamin c supplements on performance and immune response of laying hen during high environmental temperature. Int. J. Poult. Sci. 6, 895–900. doi: 10.3923/ijps.2007.895.900
Attia, Y.A., Abd El-Hamid Ael, H., Abedalla, A.A., Berika, M.A., Al-Harthi, M.A., Kucuk, O., et al. (2016). Laying performance, digestibility and plasma hormones in laying hens exposed to chronic heat stress as affected by betaine, vitamin c, and/or vitamin e supplementation. Springerplus 5:1619. doi: 10.1186/s40064-016-3304-0
Attia, Y. A., Hassan, R. A., and Qota, E. M. A. (2009). Recovery from adverse effects of heat stress on slow-growing chicks in the tropics 1: effect of ascorbic acid and different levels of betaine. Trop. Anim. Health Prod. 41:9. doi: 10.1007/s11250-008-9256-9
Aydelotte, M. B. (1963). Vitamin A deficiency in chickens. Br. J. Nutri. 17:21. doi: 10.1079/BJN19630021
Azis, A., Abbas, H., Heryandi, Y., and Kusnadib, E. (2012). Thyroid hormone and blood metabolites concentrations of broiler chickens subjected to feeding time restriction. Media Peternakan. 35, 32–372012. doi: 10.5398/medpet.2012.35.1.32
Azis, A., and Afriani, A. (2017). Effect of feeding time restriction during the growing period on growth performance of broiler chickens. Asian J. Poultr. Sci. 11:74. doi: 10.3923/ajpsaj.2017.70.74
Balnave, D. (2004). Challenges of accurately defining the nutrient requirements of heat-stressed poultry. Poult. Sci. 83, 5–14. doi: 10.1093/ps/83.1.5
Bilal, R.M., Hassan, F.-,u., Farag, M.R., Nasir, T.A., Ragni, M., Mahgoub, H.A.M., et al. (2021). Thermal stress and high stocking densities in poultry farms: potential effects and mitigation strategies. J. Therm. Biol. 99:102944. doi: 10.1016/j.jtherbio.2021.102944
Bilgili, S. F., and Hess, J. B. (1995). Placement density influences broiler carcass grade and meat yields1. J. Appl. Poult. Res. 4, 384–389. doi: 10.1093/japr/4.4.384
Blahová, J., Dobšíkov,á, R., Strakov,á, E., and Such,ý, P. (2007). Effect of low environmental temperature on performance and blood system in broiler chickens (Gallus domesticus). Acta Veterinaria Brno. 76:17. doi: 10.2754/avb200776S8S017
Bollengier-Lee, S., Williams, P. E. V., and Whitehead, C. C. (1999). Optimal dietary concentration of vitamin E for alleviating the effect of heat stress on egg production in laying hens. Br. Poult. Sci. 40:7917. doi: 10.1080/00071669987917
Boostani, A., Ashayerizadeh, A., Mahmoodian Fard, H.R., and Kamalzadeh, A. (2010). Comparison of the effects of several feed restriction periods to control ascites on performance, carcass characteristics and hematological indices of broiler chickens. Brazil. J. Poultry Sci. 12, 170–177. doi: 10.1590/S1516-635X2010000300006
Bowling, M., Forder, R., Hughes, R. J., Weaver, S., and Hynd, P. I. (2018). Effect of restricted feed intake in broiler breeder hens on their stress levels and the growth and immunology of their offspring. Transl. Anim. Sci. 2:64. doi: 10.1093/tas/txy064
Bozkurt, Z., Bayram, I., Bülbül, A., and Aktepe, O. C. (2008). Effects of strain, cage density and position on immune response to vaccines and blood parameters in layer pullets. Kafkas Univ. Vet. Fak. Derg. 14:2.
Branco, T., Moura, D. J., de de Alencar Nääs, I., da Silva Lima, N. D., Klein, D. R., Oliveira, S. R., et al. (2021). The sequential behavior pattern analysis of broiler chickens exposed to heat stress. AgriEng. 3, 447–457. doi: 10.3390/agriengineering3030030
Bratte, L. (2011). Influence of early skip-a-day feed withdrawal on the haematological indices, serum protein and nutrient digestibility of broilers. Pakistan J. Nutri. 10, 831–835. doi: 10.3923/pjn.2011.831.835
Bueno, J. P. R., Nascimento, M. R. B., de, M., Martins, J. M. da, S., Marchini, C. F. P., Gotardo, L. R. M., et al. (2017). Effect of age and cyclical heat stress on the serum biochemical profile of broiler chickens, Semina: Ciências Agrárias, Londrina. 38, 1383–1392. doi: 10.5433/1679-0359.2017v38n3p1383
Buettner, G. R. (1993). The pecking order of free radicals and antioxidants: lipid peroxidation, α-tocopherol, and ascorbate. Arch. Biochem. Biophys. 300:1074. doi: 10.1006/abbi.1993.1074
Burton, G. W., Joyce, A., and Ingold, K. U. (1983). Is vitamin E the only lipid-soluble, chain-breaking antioxidant in human blood plasma and erythrocyte membranes? Arch. Biochem. Biophys. 221, 281–290. doi: 10.1016/0003-9861(83)90145-5
Calderwood, S.K. (2010). Heat shock proteins in breast cancer progression–a suitable case for treatment? Int. J. Hypertherm. 26, 681–685. doi: 10.3109/02656736.2010.490254
Campo, J. L., Prieto, M. T., and Dávila, S. G. (2008). Effects of housing system and cold stress on heterophil-to-lymphocyte ratio, fluctuating asymmetry, and tonic immobility duration of chickens. Poult. Sci. 87, 621–626. doi: 10.3382/ps.2007-00466
Cengiz, Ö., Köksal, B. H., Tatl,i, O., Sevim, Ö., Ahsan, U., Üner, A. G., et al. (2015). Effect of dietary probiotic and high stocking density on the performance, carcass yield, gut microflora, and stress indicators of broilers. Poult. Sci. 94, 2395–2403. doi: 10.3382/ps/pev194
Chambial, S., Dwivedi, S., Shukla, K. K., John, P. J., and Sharma, P. (2013). Vitamin C in disease prevention and cure: an overview. In Indian J. Clinic. Biochemistr. 28:3. doi: 10.1007/s12291-013-0375-3
Chegini, S., Kiani, A., and Rokni, H. (2018). Alleviation of thermal and overcrowding stress in finishing broilers by dietary propolis supplementation, Italian J. Anim. Sci. 17, 377–385, doi: 10.1080/1828051X.2017.1360753
Chen, F., Jiang, Z., Jiang, S., Li, L., Lin, X., Gou, Z., et al. (2016). Dietary vitamin A supplementation improved reproductive performance by regulating ovarian expression of hormone receptors, caspase-3 and Fas in broiler breeders. Poultry Sci. 95, 30–40. doi: 10.3382/ps/pev305
Chen, M., Li, X., Shi, Q., Zhang, Z., and Xu, S. (2019). Hydrogen sulfide exposure triggers chicken trachea inflammatory injury through oxidative stress-mediated FOS/IL8 signaling. J. Hazard. Mater. 368, 243–254. doi: 10.1016/j.jhazmat.2019.01.054
Chen, X. Y., Li, R., and Geng, Z. Y. (2015). Cold stress initiates the Nrf2/UGT1A1/L-FABP signaling pathway in chickens. Poult. Sci. 94, 2597–2603. doi: 10.3382/ps/pev253
Chi, Q., Chi, X., Hu, X., Wang, S., Zhang, H., and Li, S. (2018). The effects of atmospheric hydrogen sulfide on peripheral blood lymphocytes of chickens: Perspectives on inflammation, oxidative stress and energy metabolism. Environ. Res. 167, 1–6. doi: 10.1016/j.envres.2018.06.051
Christakos, S., Ajibade, D.V., Dhawan, P., Fechner, A.J., and Mady, L.J. (2010). Vitamin d: metabolism. Endocrinol. Metabol. Clinic. NA 39, 243–253. doi: 10.1016/j.ecl.2010.02.002
Cornejo, S., Gadelha, A. C., Pokniak, J., and Villouta, G. (2007). Qualitative feed restriction on productive performance and lipid metabolism in broiler chickens. Arq. Bras. Med. Vet. Zootec. 59, 1554–1562. doi: 10.1590/S0102-09352007000600031
Dadgar, S., Crowe, T. G., Classen, H. L., Watts, J. M., and Shand, P. J. (2012). Broiler chicken thigh and breast muscle responses to cold stress during simulated transport before slaughter. Poult. Sci. 91, 1454–1464. doi: 10.3382/ps.2011-01520
Daghir, N. J. (2009). Nutritional strategies to reduce heat stress in broilers and broiler breeders. Lohmann Inform. 44, 6–15.
David, B., Mejdell, C., Michel, V., Lund, V., and Moe, R. O. (2015). Air quality in alternative housing systems may have an impact on laying hen welfare. part ii-ammonia. Anim. Open Access J. MDPI. 5, 886–896. doi: 10.3390/ani5030368
De Grande, A., Ducatelle, R., Delezie, E., Rapp, C., De Smet, S., Michiels, J., et al. (2021). Effect of vitamin E level and dietary zinc source on performance and intestinal health parameters in male broilers exposed to a temperature challenge in the finisher period. J. Anim. Physiol. Anim. Nutr. 105, 777–786, doi: 10.1111/jpn.13492
De Jong, I. C., van Voorst, S., Ehlhardt, D. A., and Blokhuis, H. J. (2002). Effects of restricted feeding on physiological stress parameters in growing broiler breeders. Br. Poult. Sci., 43, 157–168. doi: 10.1080/00071660120121355
Demir, E., Sarica, S., Sekeroglu, A., Ozcan, M. A., and Seker, Y. (2004). Effects of early and late feed restriction or feed withdrawal on growth performance, ascites and blood constituents of broiler chickens. Acta Agricult. Scand. Section A—Anim. Sci. 54, 152–158. doi: 10.1080/09064700410004852
Desoky, A. (2018). Growth performance and immune response of broiler chickens reared under high stocking density and vitamin e supplementation. Egypt. Poultr. Sci. J. 38, 607–620.
Dhanalakshmi, S., Devi, R. S., Srikumar, R., Manikandan, S., and Thangaraj, R. (2007). Protective effect of triphala on cold stress-induced behavioral and biochemical abnormalities in rats. Yakugaku Zasshi. 127:11. doi: 10.1248/yakushi.127.1863
Dozier, W. A., Thaxton, J. P., Purswell, J. L., Olanrewaju, H. A., Branton, S. L., and Roush, W. B. (2006). Stocking density effects on male broilers grown to 1.8 kilograms of body weight. Poult. Sci. 85, 344–351. doi: 10.1093/ps/85.2.344
El-Boshy, M., BaSalamah, M. A., Ahmad, J., Idris, S., Mahbub, A., Abdelghany, A. H., et al. (2019). Vitamin D protects against oxidative stress, inflammation and hepatorenal damage induced by acute paracetamol toxicity in rat. Free Radic. Biol. Med. 141, 310–321. doi: 10.1016/j.freeradbiomed.2019.06.030
El-Garhy, O. (2021). Effect of stocking density, dietary vitamin d3 and probiotic supplementation on carcass traits and blood parameters of broiler chickens. Annal. Agric. Sci. 59, 51–60. doi: 10.21608/assjm.2021.183645
El-Gogary, M. R., Ismail, F. S. A., and El-Nadi, M. I. (2015). Effect of Vitamin E supplementation and stocking density on broiler performance, carcass traits and histological responses of lymphoid organs. Asian J. Poult. Sci. 9, 70–84. doi: 10.3923/ajpsaj.2015.70.84
Elitok, B. (2018). Importance of stress factors in poultry. Juniper Online J. Case Stud. 7, 20–22. doi: 10.19080/JOJCS.2018.07.555723
Englmaierová, M., Skrivan, M., Taubner, T., Skrivanová, V., and Cermák, L. (2021). Effect of housing system and feed restriction on meat quality of medium-growing chickens. Poult. Sci. 100:101223. doi: 10.1016/j.psj.2021.101223
Estevez, I. (2007). Density allowances for broilers: where to set the limits? Poult. Sci. 86, 1265–1272. doi: 10.1093/ps/86.6.1265
Eugen, K. V., Nordquist, R. E., Zeinstra, E., and Staay, F. J. V. (2019). Stocking density affects stress and anxious behavior in the laying hen chick during rearing. Animals 9:53. doi: 10.3390/ani9020053
Evans, P., and Halliwell, B. (2001). Micronutrients: oxidant/antioxidant status. Br. J. Nutr. 85, S67–S74. doi: 10.1049/BJN2000296
Fakhoury, H. M. A., Kvietys, P. R., AlKattan, W., Anouti, F., al Elahi, M. A., Karras, S. N., et al. (2020). Vitamin D and intestinal homeostasis: barrier, microbiota, and immune modulation. J. Steroid Biochem. Mol. Biol. 200:105663. doi: 10.1016/j.jsbmb.2020.105663
Fang, Y. Z., Yang, S., and Wu, G. (2002). Free radicals, antioxidants, and nutrition. Nutrition. 18, 872–879. doi: 10.1016/S0899-9007(02)00916-4
Ferket, P. R., and Qureshi, M. A. (1992). Performance and immunity of heat-stressed broilers fed vitamin- and electrolyte-supplemented drinking water. Poult. Sci. 71, 88–97. doi: 10.3382/ps.0710088
Friedman, A., Meidovsky, A., Leitner, G., and Sklan, D. (1991). Decreased resistance and immune response to escherichia coli infection in chicks with low or high intakes of vitamin A. J. Nutr. 121, 395–400. doi: 10.1093/jn/121.3.395
Gao, J., Lin, H., Wang, X. J., Song, Z. G., and Jiao, H. C. (2010). Vitamin E supplementation alleviates the oxidative stress induced by dexamethasone treatment and improves meat quality in broiler chickens. Poult. Sci. 89, 318–327. doi: 10.3382/ps.2009-00216
Geng, A. L., Liu, H. G., Zhang, Y., Zhang, J., Wang, H. H., Chu, Q., and Yan, Z. X. (2020). Effects of indoor stocking density on performance, egg quality, and welfare status of a native chicken during 22 to 38 weeks. Poult. Sci. 99, 163–171. doi: 10.3382/ps/pez543
Geng, Y., Ma, Q., Wang, Z., and Guo, Y. (2018). Dietary vitamin D3 supplementation protects laying hens against lipopolysaccharide-induced immunological stress. Nutri. Metabol. 15, 1–14. doi: 10.1186/s12986-018-0293-8
Gharib, H. B. A., El- Menawey, M. A., Attalla, A. A., and Stino, F. K. R. (2005). Response of commercial layers to housing at different cage densities and heat stress conditions. 1- Physiological indicators and immune response. Egypt. J. Anim. Product. 42, 47–70. doi: 10.21608/ejap.2005.93011
Goo, D., Kim, J. H., Park, G. H., Delos Reyes, J. B., and Kil, D. Y. (2019). Effect of heat stress and stocking density ongrowth performance, breast meat quality,and intestinal barrier function in broiler chickens. Animals. 9:107. doi: 10.3390/ani9030107
Gouda, A., Amer, S. A., Gabr, S., and Tolba, S. A. (2020). Effect of dietary supplemental ascorbic acid and folic acid on the growth performance, redox status, and immune status of broiler chickens under heat stress. Trop. Anim. Health Prod. 52, 2987–2996. doi: 10.1007/s11250-020-02316-4
Gratta, F., Birolo, M., Sacchetto, R., Radaelli, G., Xiccato, G., Ballarin, C., et al. (2019). Effect of feed restriction timing on live performance, breast myopathy occurrence, and muscle fiber degeneration in 2 broiler chicken genetic lines. Poult. Sci. 98, 5465–5476. doi: 10.3382/ps/pez352
Guardia, S., Konsak, B., Combes, S., Levenez, F., Cauquil, L., Guillot, J. F., et al. (2011). Effects of stocking density on the growth performance and digestive microbiota of broiler chickens. Poult. Sci. 90, 1878–1889. doi: 10.3382/ps.2010-01311
Guo, S., Niu, J., Xv, J., Fang, B., Zhang, Z., Zhao, D., et al. (2021). Interactive effects of vitamins a and k3 on laying performance, egg quality, tibia attributes and antioxidative status of aged roman pink laying hens. Animal.15:100242. doi: 10.1016/j.animal.2021.100242
Gupta, A., Chauhan, N.R., Chowdhury, D., Singh, A., Meena, R. C., Chakrabarti, A., and Singh, S. B. (2017). Heat stress modulated gastrointestinal barrier dysfunction: Role of tight junctions and heat shock proteins. Scand. J. Gastroentero. 52, 1315–1319 doi: 10.1080/00365521.2017.1377285
Gursu, M. F., Onderci, M., Gulcu, F., and Sahin, K. (2004). Effects of vitamin C and folic acid supplementation on serum paraoxonase activity and metabolites induced by heat stress in vivo. Nutri. Res. 24, 157–164. doi: 10.1016/j.nutres.2003.11.008
Hajati, H., Hassanabadi, A., Golian, A., Moghaddam, H. N., and Nassiri, M.R. (2015). The effect of grape seed extract and vitamin c feed supplementation on some blood parameters and HSP70 gene expression of broiler chickens suffering from chronic heat stress, Italian. J. Anim. Sci. 14:3273. doi: 10.4081/ijas.2015.3273
Hall, A.L. (2001). The effect of stocking density on the welfare and behaviour of broiler chickens reared commercially. Anim. Welfare. 10, 23–40.
Hangalapura, B. N., Nieuwland, M. G., De Vries Reilingh, G., Buyse, J., Van Den Brand, H., Kemp, B., et al. (2005). Severe feed restriction enhances innate immunity but suppresses cellular immunity in chicken lines divergently selected for antibody responses. Poult. Sci. 84, 1520–1529. doi: 10.1093/ps/84.10.1520
Hashem, M., Gamal El-Dein, I., and Eltahawy, S. (2019). Clinicopathological studies on the ameliorative effects of selenium and vitamin e against cadmium toxicity in chickens. Zagazig Vet. J. 47, 277–287. doi: 10.21608/zvjz.2019.13192.1045
Hashem, M. A., Hamied, S. S. A., el Ahmed,, E. M. A., Amer, S. A., and Hassan, A. M. (2021). Alleviating effects of vitamins c and e supplementation on oxidative stress, hematobiochemical, and histopathological alterations caused by copper toxicity in broiler chickens. Animals. 11:1739. doi: 10.3390/ani11061739
Hassanabadi, A. (2008). The effects of early age feed restriction on performance and carcass characteristics of male broiler chickens. J. Anim. Veterinary Adv. 7, 372–3762008
Hassanabi, A., and Moghaddam, H. N. (2006). Effect of early feed restriction on performance characteristics and serum thyroxin of broiler chickens. Int. J. Poult. Sci. 5, 1156–1159. doi: 10.3923/ijps.2006.1156.1159
Holick, M. F., and Clark, M. B. (1978). The photobiogenesis and metabolism of vitamin D. Fed. Proc. 37:12.
Honda, B. T., Calefi, A. S., Costola-de-Souza, C., Quinteiro-Filho, W. M., da Silva Fonseca, J. G., de Paula, V. F., et al. (2015). Effects of heat stress on peripheral T and B lymphocyte profiles and IgG and IgM serum levels in broiler chickens vaccinated for Newcastle disease virus. Poult. Sci. 94, 2375–2381. doi: 10.3382/ps/pev192
Horváth, M., and Babinszky, L. (2018). Impact of selected antioxidant vitamins (vitamin a, e and c) and micro minerals (zn, se) on the antioxidant status and performance under high environmental temperature in poultry. A review. Anim. Sci. 68, 152–160. doi: 10.1080/09064702.2019.1611913
Houshmand, M., Azhar, K., Zulkifli, I., Bejo, M. H., and Kamyab, A. (2012). Effects of prebiotic, protein level, and stocking density on performance, immunity, and stress indicators of broilers. Poult. Sci. 91, 393–401. doi: 10.3382/ps.2010-01050
Howlider, M.A.R., and Rose, S.P. (1989). Rearing temperature and the meat yield of broilers. Br. Poult. Sci. 30, 61–67. doi: 10.1080/00071668908417125
Hu, X., Chi, Q., Wang, D., Chi, X., Teng, X., and Li, S. (2018). Hydrogen sulfide inhalation-induced immune damage is involved in oxidative stress, inflammation, apoptosis and the Th1/Th2 imbalance in broiler bursa of Fabricius. Ecotoxicol. Environ. Saf. 164, 201–209. doi: 10.1016/j.ecoenv.2018.08.029
Huang, Z., Liu, Y., Qi, G., Brand, D., and Zheng, S. (2018). Role of Vitamin A in the immune system. J. Clinic. Med. 7:258. doi: 10.3390/jcm7090258
Huff, G. R., Huff, W. E., Balog, J. M., and Rath, N. C. (2000). The effect of vitamin D3 on resistance to stress-related infection in an experimental model of turkey osteomyelitis complex. Poult. Sci. 79, 672–679. doi: 10.1093/ps/79.5.672
Ibrahim, R. R., Khalil, F., Mostafa, A. S., and Emeash, H. H. (2018). Efficacy of probiotic in improving welfare and mitigating overcrowding stress in broilers. J. Adv. Veterinary Res. 8, 73–78.
Jahanian, R., and Mirfendereski, E. (2015). Effect of high stocking density on performance, egg quality, and plasma and yolk antioxidant capacity in laying hens supplemented with organic chromium and vitamin C. Livest. Sci., 177, 117–124. doi: 10.1016/j.livsci.2015.04.022
Janczak, A. M., Torjesen, P., Palme, R., and Bakken, M. (2007). Effects of stress in hens on the behaviour of their offspring. Appl. Anim. Behav. Sci. 107:16. doi: 10.1016/j.applanim.2006.09.016
Jang, I., Kang, S., Ko, Y., Moon, Y., and Sohn, S. (2009). Effect of qualitative and quantitative feed restriction on growth performance and immune function in broiler chickens. Anim. Biosci. 22, 388–395. doi: 10.5713/ajas.2009.80513
Jobe, M. C., Ncobela, C. N., Kunene, N. W., and Opoku, A. R. (2019). Effects of Cassia abbreviata extract and stocking density on growth performance, oxidative stress and liver function of indigenous chickens. Trop. Anim. Health Prod. 51, 2567–2574. doi: 10.1007/s11250-019-01979-y
Jones, G. (2018). The discovery and synthesis of the nutritional factor vitamin d. Int. J. Paleopathol. 23, 96–99. doi: 10.1016/j.ijpp.2018.01.002
Kaiser, M. G., Block, S. S., Ciraci, C., Fang, W., Sifri, M., and Lamont, S. J. (2012). Effects of dietary vitamin E type and level on lipopolysaccharide-induced cytokine mRNA expression in broiler chicks. Poult. Sci. 91, 1893–1898. doi: 10.3382/ps.2011-02116
Kaiser, P., Wu, Z., Rothwell, L., Fife, M., Gibson, M., Poh, T.Y., et al. (2009). Prospects for understanding immune-endocrine interactions in the chicken. Gen. Comp. Endocrinol. 163, 83–9 doi: 10.1016/j.ygcen.2008.09.013
Kalavathi, S., Kumar, A. A., Reddy, A. G., Srilatha, C., and Reddy, A. R. (2011). Sodium Arsenite toxicity in broiler chicks and its amelioration: haemato-biochemical and pathological studies. Indian J. Vet. Pathol. 35, 171–176.
Kammon, A., Alzentani, S., Tarhuni, O., and Asheg, A. (2019). Effect of some organic acids on body weight, immunity and cecal bacterial count of chicken during heat stress. Int. J. Poult. Sci. 18, 293–300. doi: 10.3923/ijps.2019.293.300
Kang, H. K., Park, S. B., Kim, S. H., and Kim, C. H. (2016). Effects of stock density on the laying performance, blood parameter, corticosterone, litter quality, gas emission and bone mineral density of laying hens in floor pens. Poult. Sci. 95, 2764–2770. doi: 10.3382/ps/pew264
Kerns, J. C., Arundel, C., and Chawla, L. S. (2015). Thiamin deficiency in people with obesity. Adv. Nutri. 6, 147–153. doi: 10.3945/an.114.007526
Khan, R. U., Naz, S., Nikousefat, Z., Selvaggi, M., Laudadio, V., and Tufarelli, V. (2012). Effect of ascorbic acid in heat-stressed poultry. World's Poultry Sci. J. 68:3. doi: 10.1017/S004393391200058X
Khukhodziinai, M., Ayub Ali, M. C., Lallianchhunga, R., Goswami, T. C, Tolenkhomba, and Mayengbam, P. (2021). Supplementation of chromium and vitamin C to alleviate cold stress in white leghorn chicken. J. Entomol. Zool. Stud. 9, 1295–1298.
Koyama, H., Ikeda, S., Sugimoto, M., and Kume, S. (2012). Effects of folic acid on the development and oxidative stress of mouse embryos exposed to heat stress. Reprod. Domest. Anim. 47, 921–927. doi: 10.1111/j.1439-0531.2012.01992.x
Kucuk, O., Kahraman, A., Kurt, I., Yildiz, N., and Onmaz, A. C. (2008). A combination of zinc and pyridoxine supplementation to the diet of laying hens improves performance and egg quality. Biol. Trace Elem. Res. 126:165. doi: 10.1007/s12011-008-8190-z
Kucuk, O., Sahin, N., and Sahin, K. (2003). Supplemental zinc and vitamin A can alleviate negative effects of heat stress in broiler chickens. Biol. Trace Elem. Res. 94:225. doi: 10.1385/BTER:94:3:225
Kumar, N., Gupta, S. K., Chandan, N. K., Bhushan, S., Singh, D. K., Kumar, P., et al. (2020). Mitigation potential of selenium nanoparticles and riboflavin against arsenic and elevated temperature stress in Pangasianodon hypophthalmus. Scientific Rep. 10, 1–17. doi: 10.1038/s41598-020-74911-2
Kumar, R., Brar, R. S., and Banga, H. S. (2017). Hypervitaminosis d(3) in broiler chicks: Histopathological, immunomodulatory and immunohistochemical approach. Iran J.Vet. Res. 18, 170–176.
Kutlu, H. R., and Forbes, J. M. (1993). Changes in growth and blood parameters in heat-stressed broiler chicks in response to dietary ascorbic acid. Livestock Product. Sci. 36:50. doi: 10.1016/0301-6226(93)90050-R
Lallo, C.H.O., Williams, M., Campbell, M., and Palmer, D.W. (2012). Effects of stocking density on performance and economic implications for broilers grown to 42 days in open sided house in Trinidad. Trop. Agric. 89, 170–180.
Lamberg-Allardt, C. (2006). Vitamin D in foods and as supplements. Progr. Biophysic. Molecul. Biol. 92:17. doi: 10.1016/j.pbiomolbio.2006.02.017
Lara, L.J., and Rostagno, M.H. (2013). Impact of heat stress on poultry production. Animals. 3, 356–369. doi: 10.3390/ani3020356
Lei, G. S., Zhang, C., Cheng, B. H., and Lee, C. H. (2017). Mechanisms of action of vitamin D as supplemental therapy for Pneumocystis pneumonia. Antimicrob. Agents Chemother. 61:10. doi: 10.1128/AAC.01226-17
Lessard, M., Hutchings, D., and Cave, N. A. (1997). Cell-mediated and humoral immune responses in broiler chickens maintained on diets containing different levels of vitamin A. Poult. Sci. 76, 1368–1378. doi: 10.1093/ps/76.10.1368
Li, D., Tong, Q., Shi, Z., Zheng, W., Wang, Y., Li, B., et al. (2020). Effects of cold stress and ammonia concentration on productive performance and egg quality traits of laying hens. Anim. Open Access J. 10:2252. doi: 10.3390/ani10122252
Li, G. M., Liu, L. P., Yin, B., Liu, Y. Y., Dong, W. W., Gong, S., et al. (2020). Heat stress decreases egg production of laying hens by inducing apoptosis of follicular cells via activating the FasL/Fas and TNF-α systems. Poult. Sci. 99, 6084–6093. doi: 10.1016/j.psj.2020.07.024
Li, W., Wei, F., Xu, B., Sun, Q., Deng, W., Ma, H., et al. (2019). Effect of stocking density and alpha-lipoic acid on the growth performance, physiological and oxidative stress and immune response of broilers. Asian-Austr. J. Ani. Sci. 32, 1914–1922. doi: 10.5713/ajas.18.0939
Li, X., Chen, M., Shi, Q., Zhang, H., and Xu, S. (2020). Hydrogen sulfide exposure induces apoptosis and necroptosis through lncRNA3037/miR-15a/BCL2-A20 signaling in broiler trachea. Sci. Total Environ. 699:134296. doi: 10.1016/j.scitotenv.2019.134296
Li, X. M., Zhang, M. H., Liu, S. M., Feng, J. H., Ma, D. D., Liu, Q. X., et al. (2019). Effects of stocking density on growth performance, growth regulatory factors, and endocrine hormones in broilers under appropriate environments. Poult. Sci. 98, 6611–6617. doi: 10.3382/ps/pez505
Li, Y. C., Chen, Y., Liu, W., and Thadhani, R. (2014). MicroRNA-mediated mechanism of vitamin D regulation of innate immune response. J. Steroid Biochemistr. Mol. Biol. 144:14. doi: 10.1016/j.jsbmb.2013.09.014
Lin, H., Jiao, H., Buyse, J., and Decuypere, E. (2006). Strategies for preventing heat stress in poultry. World's Poultry Sci. J. 62, 71–86. doi: 10.1079/WPS200585
Lin, H., Wang, L. F., Song, J. L., Xie, Y. M., and Yang, Q. M. (2002). Effect of dietary supplemental levels of vitamin A on the egg production and immune responses of heat-stressed laying hens. Poult. Sci., 81, 458–465. doi: 10.1093/ps/81.4.458
Liu, J., Zhao, H., Wang, Y., Shao, Y., Zhang, L., and Xing, M. (2018). Impacts of simultaneous exposure to arsenic (III) and copper (II) on inflammatory response, immune homeostasis, and heat shock response in chicken thymus. Int. Immunopharmacol., 64, 60–68. doi: 10.1016/j.intimp.2018.08.021
Liu, Q. X., Zhou, Y., Li, X. M., Ma, D. D., Xing, S., Feng, J. H., et al. (2020). Ammonia induce lung tissue injury in broilers by activating NLRP3 inflammasome via Escherichia/Shigella. Poult. Sci. 99, 3402–3410. doi: 10.1016/j.psj.2020.03.019
Lohakare, J. D., Ryu, M. H., Hahn, T. W., Lee, J. K., and Chae, B. J. (2005). Effects of supplemental ascorbic acid on the performance and immunity of commercial broilers. J. Appl. Poultr. Res. 14:10. doi: 10.1093/japr/14.1.10
Lokhande, P. T., Kulkarni, G. B., Ravikanth, K., Maini, S., and Rekhe, D. S. (2009). Growth and haematological alterations in broiler chicken during overcrowding stress. Vet. World. 2, 432–434.
Lonsdale, D. (2019). Thiamin and protein folding. Med. Hypotheses, 129:109252. doi: 10.1016/j.mehy.2019.109252
Loyau, T., Berri, C., Bedrani, L., Métayer-Coustard, S., Praud, C., Duclos, M. J., et al. (2013). Thermal manipulation of the embryo modifies the physiology and body composition of broiler chickens reared in floor pens without affecting breast meat processing quality. J. Anim. Sci. 91, 3674–3685. doi: 10.2527/jas.2013-6445
Lu, M., Bai, J., Wei, F., Xu, B., Sun, Q., Li, J., et al. (2017). Effects of alpha-lipoic acid supplementation on growth performance, antioxidant capacity and biochemical parameters for ammonia-exposed broilers. Anim. Sci. J. 88, 1220–1225. doi: 10.1111/asj.12759
Lukienko, P. I., Mernichenko, N. G., Zverinskii, I., and v Zabrodskaya, S. (2000). Antioxidant properties of thiamine. Pharmacol. Toxicol. 130, 303–305. doi: 10.1007/BF02682257
Ma, B., Zhang, L., Li, J., Xing, T., Jiang, Y., and Gao, F. (2021). Heat stress alters muscle protein and amino acid metabolism and accelerates liver gluconeogenesis for energy supply in broilers. Poult. Sci. 100:90. doi: 10.1016/j.psj.2020.09.090
Mack, L. A., Felver-Gant, J. N., Dennis, R. L., and Cheng, H. W. (2013). Genetic variations alter production and behavioral responses following heat stress in 2 strains of laying hens. Poult. Sci. 92:2589. doi: 10.3382/ps.2012-02589
Magnuson, A. D., Liu, G., Sun, T., Tolba, S. A., Xi, L., Whelan, R., et al. (2020). Supplemental methionine and stocking density affect antioxidant status, fatty acid profiles, and growth performance of broiler chickens. J. Anim. Sci. 98:skaa092. doi: 10.1093/jas/skaa092
Mahmoud, KZ, and Yaseen, A.M. (2005). Effect of feed withdrawal and heat acclimatization on stress responses of male broiler and layer-type chickens (Gallus gallus domesticus). Asian-australas. J. Anim. Sci., 18, 1445–1450. doi: 10.5713/ajas.2005.1445
Mahmoud, U. T., Abdel-Rahman, M. A. M., Darwish, M. H. A., Applegate, T. J., and Cheng, H. (2015). Behavioral changes and feathering score in heat stressed broiler chickens fed diets containing different levels of propolis. Appl. Anim. Behav. Sci., 166, 98–105. doi: 10.1016/j.applanim.2015.03.003
Manna, P., Achari, A. E., and Jain, S. K. (2017). Vitamin D supplementation inhibits oxidative stress and upregulate SIRT1/AMPK/GLUT4 cascade in high glucose-treated 3T3L1 adipocytes and in adipose tissue of high fat diet-fed diabetic mice. Arch. Biochem. Biophys. 615, 22–34. doi: 10.1016/j.abb.2017.01.002
Maqbool, M. A., Aslam, M., Akbar, W., and Iqbal, Z. (2018). Biological importance of vitamins for human health: a review. JABS 2, 2518–4210.
March, B. E., Coates, V., and Goudie, C. (1972). Delayed hatching time of chicks from dams fed excess vitamin A and from eggs injected with vitamin A. Poult. Sci. 51, 891–896. doi: 10.3382/ps.0510891
McDowell, L. (1989). Analítico: Vitamins in Animal Nutrition; Comparative Aspects to Human Nutrition. London: Academic Press.
Mench, J. (2002). Broiler breeders: feed restriction and welfare. World's Poultr. Sci. J. 58, 23–29. doi: 10.1079/WPS20020004
Mendes, A. A., Watkins, S. E., England, J. A., Saleh, E. A., Waldroup, A. L., and Waldroup, P. W. (1997). Influence of dietary lysine levels and arginine:lysine ratios on performance of broilers exposed to heat or cold stress during the period of three to six weeks of age. Poult. Sci. 76:472. doi: 10.1093/ps/76.3.472
Metz, A. L., Walser, M. M., and Olson, W. G. (1985). The interaction of dietary vitamin a and vitamin d related to skeletal development in the turkey poult. J. Nutr. 115, 929–935, doi: 10.1093/jn/115.7.929
Miao, Z. Q., Dong, Y. Y., Qin, X., Yuan, J. M., Han, M. M., Zhang, K. K., et al. (2021). Dietary supplementation of methionine mitigates oxidative stress in broilers under high stocking density. Poult. Sci. 100:101231. doi: 10.1016/j.psj.2021.101231
Miladinović, D., Prevendar, C, A., Pekovi,ć, S., Daci,ć, S., Ivanovi,ć, S., Santibanez, J. F., et al. (2021). Recovery of brain cholinesterases and effect on parameters of oxidative stres and apoptosis in quails (Coturnix japonica) after chlorpyrifos and vitamin B1 administration. Chem. Biol. Interact. 333:109312. doi: 10.1016/j.cbi.2020.109312
Min, Y. N., Niu, Z. Y., Sun, T. T., Wang, Z. P., Jiao, P. X., Zi, B. B., et al. (2018). Vitamin E and Vitamin C supplementation improves antioxidant status and immune function in oxidative-stressed breeder roosters by up-regulating expression of GSH-Px gene. Poult. Sci. 97, 1238–1244. doi: 10.3382/ps/pex417
Mirfendereski, E., and Jahanian, R. (2015). Effects of dietary organic chromium and vitamin C supplementation on performance, immune responses, blood metabolites, and stress status of laying hens subjected to high stocking density. Poult. Sci. 94, 281–288. doi: 10.3382/ps/peu074
Mishra, B., and Jha, R. (2019). Oxidative stress in the poultry gut: potential challenges and interventions. Front. Veterinary Sci. 6:60. doi: 10.3389/fvets.2019.00060
Momeneh, T., and Torki, M. (2018). Effects of in ovo injection of vitamins B6 and B12 in fertile eggs subjected to ethanol stress on hatching traits, performance and visceral organs of broiler chicks reared under cold stress condition. Iran J. Appl. Anim. Sci. 8, 491–498.
Moneva, P., Popova-Ralcheva, S., Gudev, D., Sredkova, V., and Yanchev, I. (2008). Agricultural academy. Bulgarian J. Agricult. Sci. 14, 480–490.
Mozo, J., Emre, Y., Bouillaud, F., Ricquier, D., and Criscuolo, F. (2005). Thermoregulation: what role for UCPs in mammals and birds? Biosci. Rep. 25, 227–249. doi: 10.1007/s10540-005-2887-4
Mujahid, A. (2010). Acute cold-induced thermogenesis in neonatal chicks (Gallus gallus). Comparative Biochemistry and Physiology. Mol. Integrat. Physiol. 156, 34–41. doi: 10.1016/j.cbpa.2009.12.004
Mujahid, A., and Furuse, M. (2009a). Oxidative damage in different tissues of neonatal chicks exposed to low environmental temperature. comparative biochemistry and physiology. Mol. Integrat. Physiol. 152, 604–608. doi: 10.1016/j.cbpa.2009.01.011
Mujahid, A., and Furuse, M. (2009b). Behavioral responses of neonatal chicks exposed to low environmental temperature. Poult. Sci. 88, 917–922. doi: 10.3382/ps.2008-00472
Mutavdzin, S., Gopcevic, K., Stankovic, S., Uzelac, J. J., Borovic, M. L., and Djuric, D. (2019). The effects of folic acid administration on cardiac oxidative stress and cardiovascular biomarkers in diabetic rats. Oxid. Med. Cell. Longev. 19:549. doi: 10.1155/2019/1342549
Nääs, I., de, A., Baracho, M. dos, S., Bueno, L. G. F., de Moura, D. J., Vercelino, R. do, A., et al. (2012). Use of vitamin D to reduce lameness in broilers reared in harsh environments. Revista Brasileira de Ciencia Avicola, 14:2. doi: 10.1590/S1516-635X2012000300002
Najafi, P., Zulkifli, I., and Soleimani, A. F. (2018). Inhibition of corticosterone synthesis and its effect on acute phase proteins, heat shock protein 70, and interleukin-6 in broiler chickens subjected to feed restriction. Poult. Sci. 97, 1441–1447. doi: 10.3382/ps/pex364
Najafi, P., Zulkifli, I., Soleimani, A. F., and Kashiani, P. (2015). The effect of different degrees of feed restriction on heat shock protein 70, acute phase proteins, and other blood parameters in female broiler breeders. Poult. Sci. 94, 2322–2329. doi: 10.3382/ps/pev246
Nakano, E., Higgins, J. A., and Powers, H. J. (2001). Folate protects against oxidative modification of human LDL. Br. J. Nutr. 86, 637–639. doi: 10.1079/BJN2001478
Naseem, S., Younus, M., Anwar, B., Ghafoor, A., Aslam, A., and Akhter, S. (2005). Effect of ascorbic acid and acetylsalicylic acid supplementation on performance of broiler chicks exposed to heat stress. Int. J. Poult. Sci. 4:904. doi: 10.3923/ijps.2005.900.904
Nie, X., Wang, Y., Zhao, H., Guo, M., Liu, Y., and Xing, M. (2020). As3+ or/and Cu2+ exposure triggers oxidative stress imbalance, induces inflammatory response and apoptosis in chicken brain. Ecotoxicol. Environ. Saf. 203, 110993. doi: 10.1016/j.ecoenv.2020.110993
Niu, Z. Y., Liu, F. Z., Yan, Q. L., and Li, W. C. (2009). Effects of different levels of vitamin E on growth performance and immune responses of broilers under heat stress. Poult. Sci. 88, 2101–2107. doi: 10.3382/ps.2009-00220
NRC (1994). National Research Council. Washington, DC: Nutrient Requirements of Poultry, eighth ed. National Academic Press.
Öhrvik, H., Aaseth, J., and Horn, N. (2017). Orchestration of dynamic copper navigation—new and missing pieces. Metallomics: Integr. Biomet. Sci. 9, 1204–1229. doi: 10.1039/C7MT00010C
Olfati, A., Mojtahedin, A., Sadeghi, T., Akbari, M., and Martínez-Pastor, F. (2018). Comparison of growth performance and immune responses of broiler chicks reared under heat stress, cold stress and thermoneutral conditions. Span. J. Agricult. Res. 16:e0505. doi: 10.5424/sjar/2018162-12753
Olgun, O., Abdulqader, A.F., and Karabacak, A. (2021). The importance of nutrition in preventing heat stress at poultry. World's Poultry Sci. J. 19, 1–18. doi: 10.1080/00439339.2021.1938340
Özbey, O, and Esen, F. (2007). The effects of breeding systems and stocking density on some blood parameters of rock partridges (Alectoris graeca). Poult. Sci. 86, 420–422. doi: 10.1093/ps/86.2.420
Özkan, S., Plavnik, I., and Yahav, S. (2006). Effects of early feed restriction on performance and ascites development in broiler chickens subsequently raised at low ambient temperature. J. Appl. Poultry Res. 15, 9–19. doi: 10.1093/japr/15.1.9
Padmanabhan, S., Waly, M. I., Taranikanti, V., Guizani, N., Ali, A., Rahman, M. S., et al. (2018). Folate/Vitamin b12 supplementation combats oxidative stress-associated carcinogenesis in a rat model of colon cancer. Nutri. Cancer. 71, 100–110. doi: 10.1080/01635581.2018.1513047
Palo, P. E., Sell, J. L., Piquer, F. J., Vilaseca, L., and Soto-Salanova, M. F. (1995). Effect of early nutrient restriction on broiler chickens. 2. Performance and digestive enzyme activities. Poultry Sci. 74, 1470–1483. doi: 10.3382/ps.0741470
Panda, S., Panda, N., Panigrahy, K. K., Gupta, S. K., Mishra, S. P., and Laishram, M. (2017). Role of niacin supplementation in dairy cattle: a review. Asian J. Dairy Food Res. 36:7949. doi: 10.18805/ajdfr.v36i02.7949
Pande, V. V., Chousalkar, K. C., Bhanugopan, M. S., and Quinn, J. C. (2015). Super pharmacological levels of calcitriol (1,25-(oh)2 d3) inhibits mineral deposition and decreases cell proliferation in a strain dependent manner in chicken mesenchymal stem cells undergoing osteogenic differentiation in vitro. Poultry Sci. 94, 2784–2796. doi: 10.3382/ps/pev284
Pandurang, L., Kulkarni, G., Gangane, G., More, P., Ravikanth, K., Maini, S., et al. (2011). Overcrowding stress management in broiler chicken with herbal antistressor. Iranian J. Appl. Anim. Sci. 1, 49–55.
Pang, B., Jin, H., Liao, N., Li, J., Jiang, C., and Shi, J. (2021). Vitamin A supplementation ameliorates ulcerative colitis in gut microbiota–dependent manner. Food Res. Int. 148:110568. doi: 10.1016/j.foodres.2021.110568
Patel, K. R., and Sobczyńska-Malefora, A. (2016). The adverse effects of an excessive folic acid intake. Euro. J. Clinic. Nutri. 71, 159–163. doi: 10.1038/ejcn.2016.194
Pekel, A. Y., Tatli, O., Sevim, Ö., Kuter, E., Ahsan, U., Khamseh, E. K., et al. (2020). Effects of reducing dietary amino acid density and stocking density on growth performance, carcass characteristics, meat quality, and occurrence of white striping in broiler chickens. Poult. Sci., 99, 7178–7191. doi: 10.1016/j.psj.2020.08.077
Powers, H. J. (2007). Folic acid under scrutiny. Br. J. Nutr. 98, 665–666. doi: 10.1017/S0007114507795326
Purswell, J.L., Dozier III, W.A., Olanrewaju, H.A., Davis, J.D., Xin, H., and Gates, R.S. (2012). “Effect of temperature-humidity index on live performance in broiler chickens grown from 49 to 63 days of age,” in 2012 IX International Livestock Environment Symposium (ILES IX) (p. 3). American Society of Agricultural and Biological Engineers.
Puthpongsiriporn, U., Scheideler, S. E., Sell, J. L., and Beck, M. M. (2001). Effects of vitamin E and C supplementation on performance, in vitro lymphocyte proliferation, and antioxidant status of laying hens during heat stress. Poult. Sci. 80, 1190–1200. doi: 10.1093/ps/80.8.1190
Qaid, M., Albatshan, H., Shafey, T., Hussein, E., and Abudabos, A. M. (2016). Effect of stocking density on the performance and immunity of 1-to 14-d- old broiler chicks. Revista Brasileira de Ciencia Avicola 18:289. doi: 10.1590/1806-9061-2016-0289
Quadro, L., Gamble, M. V., Vogel, S., Lima, A. A. M., Piantedosi, R., Moore, S. R., et al. (2000). Retinol and retinol-binding protein: gut integrity and circulating immunoglobulins. J. Infect. Dis. 182, S97–S102. doi: 10.1086/315920
Quinteiro-Filho, W. M, Ribeiro, A., Ferraz-de-Paula, V., Pinheiro, M. L., and Sakai, M. (2010). Heat stress impairs performance parameters, induces intestinal injury, and decreases macrophage activity in broiler chickens. Poult Sci. 89, 1905–1914 doi: 10.3382/ps.2010-00812
Quinteiro-Filho, W. M., Calefi, A. S., Cruz, D., Aloia, T., Zager, A., Astolfi-Ferreira, C. S., et al. (2017). Heat stress decreases expression of the cytokines, avian β-defensins 4 and 6 and Toll-like receptor 2 in broiler chickens infected with Salmonella Enteritidis. Vet. Immunol. Immunopathol. 186, 19–28. doi: 10.1016/j.vetimm.2017.02.006
Qureshi, S., Khan, H., Amin, U., Mir, M., Adil, S., and Sofi, A. (2020). Influence of cold stress and its remedial measures on jejunal Histomorhology of broiler chicken. J. Pharmarceutic. Innov.9, 225–229. doi: 10.20546/ijcmas.2020.904.137
Qureshi, S., Khan, H. M., Mir, M. S., Raja, T. A., Khan, A. A., Ali, H., et al. (2018). Effect of cold stress and various suitable remedies on performance of broiler chicken. J. World's Poultr. Res. 8:3.
Radaelli, G., Piccirillo, A., Birolo, M., Bertotto, D., Gratta, F., Ballarin, C., et al. (2017). Effect of age on the occurrence of muscle fiber degeneration associated with myopathies in broiler chickens submitted to feed restriction. Poultr. Sci. 96, 309–319. doi: 10.3382/ps/pew270
Rahmani, M., Golian, A., Kermanshahi, H., and Reza Bassami, M. (2017). Effects of curcumin or nanocurcumin on blood biochemical parameters, intestinal morphology and microbial population of broiler chickens reared under normal and cold stress conditions. J. Appl. Anim. Res. 46, 200–209. doi: 10.1080/09712119.2017.1284077
Rajabi, M., and Torki, M. (2021). Effect of dietary supplemental vitamin C and zinc sulfate on productive performance, egg quality traits and blood parameters of laying hens reared under cold stress condition. J. Appl. Anim. Res. 49, 309–317, doi: 10.1080/09712119.2021.1949999
Rajman, M., Juráni, M., Lamosová, D., Mácajová, M., Sedlacková, M., Kost'ál, L., et al. (2006). The effects of feed restriction on plasma biochemistry in growing meat type chickens (Gallus gallus). Comp. Biochem. Physiol. Part A Mol. Integr. 145, 363–371. doi: 10.1016/j.cbpa.2006.07.004
Rehman, Z. U., Che, L., Ren, S., Liao, Y., Qiu, X., Yu, S., et al. (2018). Supplementation of Vitamin E protects chickens from newcastle disease virus-mediated exacerbation of intestinal oxidative stress and tissue damage. Cell. Physiol. Biochem. 47, 1655–1666. doi: 10.1159/000490984
Rohde, C. M., Manatt, M., Clagett-Dame, M., and DeLuca, H. F. (1999). Vitamin a antagonizes the action of vitamin d in rats. J. Nutri. 129, 2246–2250. doi: 10.1093/jn/129.12.2246
Saedisomeolia, A., and Ashoori, M. (2018). riboflavin in human health: a review of current evidences. Adv. Food Nutr. Res. 83, 57–81. doi: 10.1016/bs.afnr.2017.11.002
Sahin, K., Onderci, M., Sahin, N., Ferit Gursu, M., and Aydin, S. (2003a). Cold-induced elevation of homocysteine and lipid peroxidation can be alleviated by dietary folic acid supplementation. Nutri. Res. 23, 357–365. doi: 10.1016/S0271-5317(02)00539-0
Sahin, K., Sahin, N., and Kucuk, O. (2003b). Effects of chromium, and ascorbic acid supplementation on growth, carcass traits, serum metabolites, and antioxidant status of broiler chickens reared at a high ambient temperature (32°C). Nutri. Res. 23, 225–238. doi: 10.1016/S0271-5317(02)00513-4
Sahin, N., and Sahin, K. (2001). Optimal dietary concentrations of vitamin C and chromium picolinate for alleviating the effect of low ambient temperature (6.2°C) on egg production, some egg characteristics, and nutrient digestibility in laying hens. Vet. Med. 46, 9–10. doi: 10.17221/7887-VETMED
Saiz del Barrio, A., Mansilla, W. D., Navarro-Villa, A., Mica, J. H., Smeets, J. H., den Hartog, L. A., et al. (2020). Effect of mineral and vitamin C mix on growth performance and blood corticosterone concentrations in heat-stressed broilers. J. Appl.Poultr. Res. 29, 23–33. doi: 10.1016/j.japr.2019.11.001
Saleh, K. M., and Al-Zghoul, M. B. (2019). Thermal manipulation during broiler chicken embryogenesis modulates the splenic cytokines' mRNA expression. Jordan J. Biol. Sci. 12, 595–601.
Sandercock, D. A., Hunter, R. R., Mitchell, M. A., and Hocking, P. M. (2006). Thermoregulatory capacity and muscle membrane integrity are compromised in broilers compared with layers at the same age or body weight. Br. Poultry Sci. 47, 322–329. doi: 10.1080/00071660600732346
Sandhu, M.A., Raza, F., Afzal, F., and Ahmad Anjum, M.S. (2013). Managing immunocompetence of broiler chicken through vitamin E supplementation at low ambient temperature. Int. J. Agric. Biol. 15, 1051–1058
Sandilands, V., Tolkamp, B. J., and Kyriazakis, I. (2005). Behaviour of food restricted broilers during rearing and lay - effects of an alternative feeding method. Physiol. Behav. 85:1. doi: 10.1016/j.physbeh.2005.03.001
Santos, R. R., Awati, A., Roubos-van den Hil, P. J., Tersteeg-Zijderveld, M. H., Koolmees, P. A., and Fink-Gremmels, J. (2015). Quantitative histo-morphometric analysis of heat-stress-related damage in the small intestines of broiler chickens. Avian Pathol. J. W.V.P.A, 44, 19–22. doi: 10.1080/03079457.2014.988122
Sawyer, D.B., Siwik, D.A., Xiao, L., Pimentel, D.R., Singh, K., and Colucci, W.S. (2002). Role of oxidative stress in myocardial hypertrophy and failure. J. Mol. Cell. Cardiol. 34. 379–388 doi: 10.1006/jmcc.2002.1526
Selders, G. S., Fetz, A. E., Radic, M. Z., and Bowlin, G. L. (2017). An overview of the role of neutrophils in innate immunity, inflammation and host-biomaterial integration. Regenerat. Biomater. 4, 55–68. doi: 10.1093/rb/rbw041
Selvam, R., Saravanakumar, M., Suresh, S., Sureshbabu, G., Sasikumar, M., and Prashanth, D. (2017). Effect of vitamin e supplementation and high stocking density on the performance and stress parameters. Broilers. 19, 587–594. doi: 10.1590/1806-9061-2016-0417
Shah, S., Chen, J., Han, Q., Xu, Y., Ishfaq, M., and Teng, X. (2020). Ammonia inhalation impaired immune function and mitochondrial integrity in the broilers bursa of fabricius: Implication of oxidative stress and apoptosis. Ecotoxicol. Environ. Safety 190:110078. doi: 10.1016/j.ecoenv.2019.110078
Shakeri, M., Cottrell, J. J., Wilkinson, S., Zhao, W., Le, H. H., McQuade, R., et al. (2019). Dietary betaine improves intestinal barrier function and ameliorates the impact of heat stress in multiple vital organs as measured by evans blue dye in broiler chickens. Anim. Open Access J. MDPI 10:38. doi: 10.3390/ani10010038
Shakeri, M., Oskoueian, E., Le, H. H., and Shakeri, M. (2020). Strategies to combat heat stress in broiler chickens: unveiling the roles of selenium, vitamin E and vitamin C. Veterinary Sci. 7:71. doi: 10.3390/vetsci7020071
Sharma, A., Kumar, B., Dash, S., Singh, S., and Verma, R. (2020). Heat shock protein B1 expression is associated with age at sexual maturity in Rhode Island Red and Punjab Red layers under heat stress. Int. J. Biometeorol. 64, 1133–1143. doi: 10.1007/s00484-020-01887-z
Shehata, A. M., Saadeldin, I. M., Tukur, H. A., and Habashy, W. S. (2020). Modulation of heat-shock proteins mediates chicken cell survival against thermal stress. Anim. Open Access J. MDPI. 10:2407. doi: 10.3390/ani10122407
Shewita, R. S., El-Naggar, K., and El Naby, W, Abd, S. H. (2019). Influence of dietary vitamin c on growth performance, blood biochemical parameters and transcript levels of heat shock proteins in high stocking density reared broiler chickens. Slovenian Veterinar. Res. Suppl. 56, 129–138. doi: 10.26873/SVR-750-2019
Shinder, D., Luger, D., Rusal, M., Rzepakovsky, V., Bresler, V, and Yahav, S. (2002). Early cold conditioning in broiler chickens (Gallus domesticus): thermotolerance and growth responses. J. Therm. Biol. 27, 517–523. doi: 10.1016/S0306-4565(02)00025-6
Shinder, D., Rusal, M., Giloh, M., and Yahav, S. (2009). Effect of repetitive acute cold exposures during the last phase of broiler embryogenesis on cold resistance through the life span. Poult. Sci. 88, 636–646. doi: 10.3382/ps.2008-00213
Shojadoost, B., Yitbarek, A., Alizadeh, M., Kulkarni, R. R., Astill, J., Boodhoo, N., et al. (2021). Centennial review: effects of vitamins A, D, E, and C on the chicken immune system. Poultry Sci. 100:27. doi: 10.1016/j.psj.2020.12.027
Simitzis, P. E., Kalogeraki, E., Goliomytis, M., Charismiadou, M. A., Triantaphyllopoulos, K., Ayoutanti, A., et al. (2012). Impact of stocking density on broiler growth performance, meat characteristics, behavioural components and indicators of physiological and oxidative stress. Br. Poult. Sci. 53:5930. doi: 10.1080/00071668.2012.745930
Simsek, U. G., DalkIlIc, B., CIftc,I, M., and Yuce, A. (2009). The influences of different stocking densities on some welfare indicators, lipid peroxidation (MDA) and antioxidant enzyme activities (GSH, GSH-Px, CAT) in broiler chickens. J. Anim. Veterinary Adv. 8, 1568–1572.
Sinbad, O. O., Folorunsho, A. A., Olabisi, O. L., Abimbola, A. O, and Temitope, J. E. (2019). Vitamins as Antioxidants. J. Food Sci. Nutri. Res. 02:03. doi: 10.26502/jfsnr.2642-11000021
Singh, H., Sodhi, S., and Kaur, R. (2006). Effects of dietary supplements of selenium, vitamin E or combinations of the two on antibody responses of broilers. Br. Poult. Sci. 47:79. doi: 10.1080/00071660601040079
Sinkalu, V. O., and Ayo, J. O. (2016). Combined effects of retinol, ascorbic acid and α-tocopherol on diurnal variations in rectal temperature of Black Harco pullets subjected to heat stress. Int. J. Biometeorol. 62, 9–15. doi: 10.1007/s00484-016-1157-4
Sklan, D., Melamed, D., and Friedman, A. (1994). The effect of varying levels of dietary vitamin a on immune response in the chick. Poult. Sci. 73, 843–847. doi: 10.3382/ps.0730843
Sohail, M.U., Ijaz, A., Yousaf, M.S., and Ashraf, K.;, Zaneb, H.;, Aleem, M.;, Rehman, H. (2010). Alleviation of cyclic heat stress in broilers by dietary supplementation of mannan-oligosaccharide and Lactobacillus-based probiotic: dynamics of cortisol, thyroid hormones, cholesterol, C-reactive protein, and humoral immunity. Poult. Sci. 89, 1934–1938. doi: 10.3382/ps.2010-00751
Soleimani, A. F., Zulkifli, I., Hair-Bejo, M., Omar, A. R., and Raha, A. R. (2012). The role of heat shock protein 70 in resistance to Salmonella enteritidis in broiler chickens subjected to neonatal feed restriction and thermal stress. Poult. Sci. 91, 340–345. doi: 10.3382/ps.2011-01703
Soleimani, A. F., Zulkifli, I., Omar, A. R., and Raha, A. R. (2011). Physiological responses of 3 chicken breeds to acute heat stress. Poult. Sci. 90, 1435–1440. doi: 10.3382/ps.2011-01381
Son, J. H. (2013). The effect of stocking density on the behaviour and welfare indexes of broiler chickens. J. Agric. Sci. Technol. 3, 307–311
Song, J., Jiao, L. F., Xiao, K., Luan, Z. S., Hu, C. H., Shi, B., et al. (2013). Cello-oligosaccharide ameliorates heat stress-induced impairment of intestinal microflora, morphology and barrier integrity in broilers. Anim. Feed Sci. Technol. 185, 175–181. doi: 10.1016/j.anifeedsci.2013.08.001
Song, J., Xiao, K., Ke, Y. L., Jiao, L. F., Hu, C. H., Diao, Q. Y., et al. (2014). Effect of a probiotic mixture on intestinal microflora, morphology, and barrier integrity of broilers subjected to heat stress. Poult. Sci. 93, 581–588. doi: 10.3382/ps.2013-03455
Song, Z., Lv, J., Sheikhahmadi, A., Uerlings, J., and Everaert, N. (2017). Attenuating Effect of zinc and vitamin e on the intestinal oxidative stress induced by silver nanoparticles in broiler chickens. Biologic. Trace Elem. Res. 180, 306–313. doi: 10.1007/s12011-017-1016-0
Song, N., Wang, W., Wang, Y., Guan, Y., Xu, S., and Guo, M. Y. (2021). Hydrogen sulfide of air induces macrophage extracellular traps to aggravate inflammatory injury via the regulation of miR-15b-5p on MAPK and insulin signals in trachea of chickens. Sci. Total Environ., 771:145407. doi: 10.1016/j.scitotenv.2021.145407
Su, Y., Li, S., Xin, H., Li, J., Li, X., Zhang, R., et al. (2020). Proper cold stimulation starting at an earlier age can enhance immunity and improve adaptability to cold stress in broilers. Poult. Sci. 99, 129–141. doi: 10.3382/ps/pez570
Su, Y., Zhang, X., Xin, H., Li, S., Li, J., Zhang, R., et al. (2018). Effects of prior cold stimulation on inflammatory and immune regulation in ileum of cold-stressed broilers. Poult. Sci. 97, 4228–4237. doi: 10.3382/ps/pey308
Suckeveris, D., Burin Jr, A., Oliveira, A., Nascimento, F°, M., Pereira, R., Luvizotto Jr, J., et al. (2020). Supra-nutritional levels of selected b vitamins in animal or vegetable diets for broiler chicken. Brazilian Journal of Poultry Science, 22, 1–10. doi: 10.1590/1806-9061-2019-1024
Surai Peter, F., Kochish, I. I., Romanov, M. N., and Griffin, D. K. (2019). Nutritional modulation of the antioxidant capacities in poultry: the case of vitamin E. Poult. Sci. 98, 4030–4041. doi: 10.3382/ps/pez072
Surai, P. F., and Fisinin, V. I. (2016). Vitagenes in poultry production: Part 3. Vitagene concept development. World's Poultry Sci. J. 72:751. doi: 10.1017/S0043933916000751
Tang, L.-P., Li, W.-H., Liu, Y.-L., Lun, J.-C., and He, Y.-M. (2021). Heat stress aggravates intestinal inflammation through TLR4-NF-κB signaling pathway in Ma chickens infected with Escherichia coli O157:H7. Poult. Sci. 100, 101030. doi: 10.1016/j.psj.2021.101030
Teeter, R. G., Smith, M. O., Owens, F. N., Arp, S. C., Sangiah, S., and Breazile, J. E. (1985). Chronic heat stress and respiratory alkalosis: Occurrence and treatment in broiler chicks. Poult. Sci. 64, 1060–1064. doi: 10.3382/ps.0641060
Teymouri, B., Ghiasi Ghalehkandi, J., Hassanpour, S., and Aghdam-Shahryar, H. (2019). Effect of In Ovo Feeding of the Vitamin B12 on Hatchability, Performance and Blood Constitutes in Broiler Chicken. Int. J. Peptide Res0 Therapeutics 26, 381–387. doi: 10.1007/s10989-019-09844-0
Thomas, S., Prabhu, R., and Balasubramanian, K. (2005). Retinoid metabolism in the rat small intestine. Br. J. Nutri. 93, 59–63. doi: 10.1079/BJN20041306
Thurnham, D. I., Northrop-Clewes, C. A., McCullough, F. S. W., Das, B. S., and Lunn, P. G. (2000). Innate immunity, gut integrity, and vitamin a in gambian and indian infants. J. Infect. Dis. 182, S23–S28. doi: 10.1086/315912
Tian, Y., Nichols, R. G., Cai, J., Patterson, A. D., and Cantorna, M. T. (2018). Vitamin A deficiency in mice alters host and gut microbial metabolism leading to altered energy homeostasis. J. Nutr. Biochem. 54, 28–34. doi: 10.1016/j.jnutbio.2017.10.011
Tona, G. O. (2018). Current and Future Improvements in Livestock Nutrition and Feed Resources. Anim. Husbandry Nutri. 18:73088. doi: 10.5772/intechopen.73088
Tong, H. B., Lu, J., Zou, J. M., Wang, Q., and Shi, S. R. (2012). Effects of stocking density on growth performance, carcass yield, and immune status of a local chicken breed. Poult. Sci. 91, 667–673. doi: 10.3382/ps.2011-01597
Trocino, A., Piccirillo, A., Birolo, M., Radaelli, G., Bertotto, D., Filiou, E., et al. (2015). Effect of genotype, gender and feed restriction on growth, meat quality and the occurrence of white striping and wooden breast in broiler chickens. Poult. Sci., 94, 2996–3004. doi: 10.3382/ps/pev296
Trocino, A., White, P., Bordignon, F., Ferrante, V., Bertotto, D., Birolo, M., et al. (2020). Effect of feed restriction on the behaviour and welfare of broiler chickens. Animals 10:50830. doi: 10.3390/ani10050830
Tsiouris, V., Georgopoulou, I., Batzios, C., Pappaioannou, N., Ducatelle, R., and Fortomaris, P. (2014). Temporary feed restriction partially protects broilers from necrotic enteritis. Avian Pathol. 43, 139–145. doi: 10.1080/03079457.2014.889278
Tsiouris, V., Georgopoulou, I., Batzios, C., Pappaioannou, N., Ducatelle, R., and Fortomaris, P. (2015). The effect of cold stress on the pathogenesis of necrotic enteritis in broiler chicks. Avian Pathol. 44, 430–435. doi: 10.1080/03079457.2015.1083094
Tsiouris, V., Georgopoulou, I., Batzios, C., Pappaioannou, N., Ducatelle, R., and Fortomaris, P. (2018). Heat stress as a predisposing factor for necrotic enteritis in broiler chicks. Avian Pathol. 47:24574. doi: 10.1080/03079457.2018.1524574
Turkyilmaz, M. K. (2006). The effect of stocking density on stress reaction in broiler chickens during summer Turk. J. Vet. Anim. Sci. 1, 31–36.
Urdaneta-Rincon, M., and Leeson, S. (2002). Quantitative and qualitative feed restriction on growth characteristics of male broiler chickens. Poult. Sci. 81, 679–688. doi: 10.1093/ps/81.5.679
Urso, U.R.A., Dahlke, F., Maiorka, A., Bueno, I.J.M., Schneider, A.F., Surek, D., et al. (2015). Vitamin e and selenium in broiler breeder diets: Effect on live performance, hatching process, and chick quality. Poultry Sci. 94, 976–983. doi: 10.3382/ps/pev042
Varasteh, S., Braber, S., Akbari, P., Garssen, J., and Fink-Gremmels, J. (2015). Differences in Susceptibility to Heat Stress along the Chicken Intestine and the Protective Effects of Galacto-Oligosaccharides. PLoS ONE 10:e0138975. doi: 10.1371/journal.pone.0138975
Vinoth, A., Thirunalasundari, T., Tharian, J. A., Shanmugam, M., and Rajkumar, U. (2015). Effect of thermal manipulation during embryogenesis on liver heat shock protein expression in chronic heat stressed colored broiler chickens. J. Therm. Biol. 53, 162–171. doi: 10.1016/j.jtherbio.2015.10.010
Wang, B., Min, Z., Yuan, J., Zhang, B., and Guo, Y. (2014). Effects of dietary tryptophan and stocking density on the performance, meat quality, and metabolic status of broilers. J. Anim. Sci. Biotechnol. 5:44. doi: 10.1186/2049-1891-5-44
Wang, J., Qiu, L., Gong, H., Celi, P., Yan, L., Ding, X., et al. (2020). Effect of dietary 25-hydroxycholecalciferol supplementation and high stocking density on performance, egg quality, and tibia quality in laying hens. Poult. Sci. 99, 2608–2615. doi: 10.1016/j.psj.2019.12.054
Wang, J., Zhang, C., Zhang, T., Yan, L., Qiu, L., Yin, H., et al. (2021). Dietary 25-hydroxyvitamin D improves intestinal health and microbiota of laying hens under high stocking density. Poultry Sci. 10:32. doi: 10.1016/j.psj.2021.101132
Wang, J. W., and Xu, S. W. (2008). Effects of cold stress on the messenger ribonucleic acid levels of corticotrophin-releasing hormone and thyrotropin-releasing hormone in hypothalami of broilers. Poult. Sci., 87, 973–978. doi: 10.3382/ps.2007-00281
Wang, S., Chi, Q., Hu, X., Cong, Y., and Li, S. (2019b). Hydrogen sulfide-induced oxidative stress leads to excessive mitochondrial fission to activate apoptosis in broiler myocardia. Ecotoxicol. Environ. Saf. 183:109578. doi: 10.1016/j.ecoenv.2019.109578
Wang, Y., Huang, M., Meng, Q., and Wang, Y. (2011). Effects of atmospheric hydrogen sulfide concentration on growth and meat quality in broiler chickens. Poult. Sci. 90, 2409–2414. doi: 10.3382/ps.2011-01387
Wang, Y., Zhao, H., Liu, J., Shao, Y., Li, J., Luo, L., et al. (2018). Copper and arsenic-induced oxidative stress and immune imbalance are associated with activation of heat shock proteins in chicken intestines. Int. Immunopharmacol. 60, 64–75. doi: 10.1016/j.intimp.2018.04.038
Wang, S., Li, X., Wang, W., Zhang, H., and Xu, S. (2019a). Application of transcriptome analysis: Oxidative stress, inflammation and microtubule activity disorder caused by ammonia exposure may be the primary factors of intestinal microvilli deficiency in chicken. Sci. Total Environ. 696:134035. doi: 10.1016/j.scitotenv.2019.134035
Warren, M.F., Vu, T.C., Toomer, O.T., Fernandez, J.D., and Livingston, K.A. (2020). Efficacy of 1-α-hydroxycholecalciferol supplementation in young broiler feed suggests reducing calcium levels at grower phase. Front. Veterinary Sci. 7:245. doi: 10.3389/fvets.2020.00245
Washburn, K.W. (1985). “Breeding of poultry in hot and cold environments,” in Stress physiology in livestock. Volume III. Poultry. pp.111-122 ref.45 Boca Raton, Fla. (USA): CRC Press.
Wu, Y., Li, J., Qin, X., Sun, S., Xiao, Z., Dong, X., et al. (2018). Proteome and microbiota analysis reveals alterations of liver-gut axis under different stocking density of Peking ducks. PLoS ONE 13:1989985. doi: 10.1371/journal.pone.0198985
Wu, Y., Wang, Y., Wu, W., Yin, D., Sun, X., Guo, X., et al. (2020). Effects of nicotinamide and sodium butyrate on meat quality and muscle ubiquitination degradation genes in broilers reared at a high stocking density. Poult. Sci. 99, 1462–1470. doi: 10.1016/j.psj.2019.10.070
Xia, S.-L., Ying, S.-J., Lin, Q.-R., Wang, X.-Q., Hong, W.-J., Lin, Z.-J., et al. (2019). Association of ulcerative colitis with foxp3 gene polymorphisms and its colonic expression in Chinese patients. Gastroenterol. Res. Pract. 2019, 1–10. doi: 10.1155/2019/4052168
Xie, S., Yang, X., Gao, Y., Jiao, W., Li, X., Li, Y., et al. (2017). Performance differences of Rhode Island Red, Bashang Long-tail Chicken, and their reciprocal crossbreds under natural cold stress. Asian-australas. J. Anim. Sci., 30, 1507–1514. doi: 10.5713/ajas.16.0957
Xing, H., Peng, M., Li, Z., Chen, J., Zhang, H., and Teng, X. (2019). Ammonia inhalation-mediated mir-202-5p leads to cardiac autophagy through PTEN/AKT/mTOR pathway. Chemosphere235, 858–866. doi: 10.1016/j.chemosphere.2019.06.235
Xu, S., Chen, Y. H., Tan, Z. X., Xie, D. D., Zhang, C., Xia, M. Z., et al. (2015). Vitamin D3 pretreatment alleviates renal oxidative stress in lipopolysaccharide-induced acute kidney injury. J. Steroid Biochem. Mol. Biol. 152, 133–141. doi: 10.1016/j.jsbmb.2015.05.009
Yahav, S. (2000). Domestic fowl-strategies to confront environmental conditions. Avian Poult. Biol. Rev. 11, 81–95.
Yahav, S. (2004). Ammonia affects performance and thermoregulation of male broiler chickens. Anim. Res. 53, 289–293. doi: 10.1051/animres:2004015
Yahav, S. (2009). Alleviating heat stress in domestic fowl: different strategies. Worlds Poult. Sci. J. 65, 719–732. doi: 10.1017/S004393390900049X
Yahav, S., Collin, A., Shinder, D., and Picard, M. (2004). Thermal manipulations during broiler chick embryogenesis: effects of timing and temperature. Poult. Sci. 83, 1959–1963. doi: 10.1093/ps/83.12.1959
Yahav, S., and McMurtry, J. P. (2001). Thermotolerance acquisition in broiler chickens by temperature conditioning early in life–the effect of timing and ambient temperature. Poult. Sci. 80, 1662–1666. doi: 10.1093/ps/80.12.1662
Yan, C., Xiao, J., Chen, D., Turner, S. P., Li, Z., Liu, H., et al. (2021). Feed restriction induced changes in behavior, corticosterone, and microbial programming in slow- and fast-growing chicken breeds. Animals: an open access journal from MDPI 11:141. doi: 10.3390/ani11010141
Yang, X., Zhuang, J., Rao, K., Li, X., and Zhao, R. (2010). Effect of early feed restriction on hepatic lipid metabolism and expression of lipogenic genes in broiler chickens. Res. Vet. Sci. 89, 438–444. doi: 10.1016/j.rvsc.2010.04.003
Yin, K., Cui, Y., Qu, Y., Zhang, J., Zhang, H., and Lin, H. (2020). Hydrogen sulfide upregulates miR-16-5p targeting PiK3R1 and RAF1 to inhibit neutrophil extracellular trap formation in chickens. Ecotoxicol. Environ. Saf. 194:110412. doi: 10.1016/j.ecoenv.2020.110412
Yin, M. C., Faustman, C., Riesen, J. W., and Williams, S. N. (1993). α-tocopherol and ascorbate delay oxymyoglobin and phospholipid oxidation in vitro. J. Food Sci. 58, 1273–1276. doi: 10.1111/j.1365-2621.1993.tb06164.x
Yu, D. G., Namgung, N., Kim, J. H., Won, S. Y., Choi, W. J., and Kil, D. Y. (2021). Effects of stocking density and dietary vitamin C on performance, meat quality, intestinal permeability, and stress indicators in broiler chickens. J. Anim. Sci. Technol. 63:815. doi: 10.5187/jast.2021.e77
Yuan, J., Roshdy, A. R., Guo, Y., Wang, Y., and Guo, S. (2014). Effect of Dietary Vitamin A on Reproductive Performance and Immune Response of Broiler Breeders. PLoS ONE, 9:105677. doi: 10.1371/journal.pone.0105677
Zaboli, G. R., Rahimi, S., Shariatmadari, F., Torshizi, M. A. K., Baghbanzadeh, A., and Mehri, M. (2017). Thermal manipulation during Pre and Post-Hatch on thermotolerance of male broiler chickens exposed to chronic heat stress. Poult. Sci. 96, 478–485. doi: 10.3382/ps/pew344
Zahoor, I., de Koning, D. J., and Hocking, P. M. (2017). Transcriptional profile of breast muscle in heat stressed layers is similar to that of broiler chickens at control temperature. Genetics Select. Evol. 49:69. doi: 10.1186/s12711-017-0346-x
Zangeneh, S., Torki, M., Abdolmohammadi, A., and Saki, A. (2020). Dietary addition of lysophospholipids and vitamin c affects growth performance, serum metabolites, antioxidant capacity and carcass characteristics of broiler chickens reared under low ambient temperature. Anim. Product. Sci. 60, 1557–1566. doi: 10.1071/AN18495
Zhang, H. Y., Piao, X. S., Zhang, Q., Li, P., Yi, J. Q., Liu, J. D., et al. (2013). The effects of Forsythia suspensa extract and berberine on growth performance, immunity, antioxidant activities, and intestinal microbiota in broilers under high stocking density. Poult. Sci. 92, 1981–1988. doi: 10.3382/ps.2013-03081
Zhang, Z. W., Lv, Z. H., Li, J. L., Li, S., Xu, S. W., and Wang, X. L. (2011). Effects of cold stress on nitric oxide in duodenum of chicks. Poult. Sci. 90, 1555–1561. doi: 10.3382/ps.2010-01333
Zhao, F. Q., Zhang, Z. W., Yao, H. D., Wang, L. L., Liu, T., Yu, X. Y., et al. (2013). Effects of cold stress on mRNA expression of immunoglobulin and cytokine in the small intestine of broilers. Res. Vet. Sci. 95:21. doi: 10.1016/j.rvsc.2013.01.021
Zhao, H., Wang, Y., Shao, Y., Liu, J., Wang, S., and Xing, M. (2018). Oxidative stress-induced skeletal muscle injury involves in NF-κB/p53-activated immunosuppression and apoptosis response in copper (II) or/and arsenite-exposed chicken. Chemosphere 210, 76–84. doi: 10.1016/j.chemosphere.2018.06.165
Zhao, Q., Xue, W., Zhang, S., Guo, Y., Li, Y., Wu, X., et al. (2021). The functions of Patchouli and Elsholtzia in the repair of hen follicular granular cells after heat stress, Poultry Science 21:101306, doi: 10.1016/j.psj.2021.101306
Zhou, H. J., Kong, L. L., Zhu, L. X., Hu, X. Y., Busye, J., and Song, Z. G. (2021). Effects of cold stress on growth performance, serum biochemistry, intestinal barrier molecules, and adenosine monophosphate-activated protein kinase in broilers. Animal 15:100138. doi: 10.1016/j.animal.2020.100138
Zhou, Y., Zhang, M., Liu, Q., and Feng, J. (2021). The alterations of tracheal microbiota and inflammation caused by different levels of ammonia exposure in broiler chickens. Poult. Sci. 100, 685–696. doi: 10.1016/j.psj.2020.11.026
Keywords: chickens, temperature stress, stocking density, feed restriction, environmental pollutants, vitamins
Citation: Akinyemi F and Adewole D (2021) Environmental Stress in Chickens and the Potential Effectiveness of Dietary Vitamin Supplementation. Front. Anim. Sci. 2:775311. doi: 10.3389/fanim.2021.775311
Received: 13 September 2021; Accepted: 26 October 2021;
Published: 18 November 2021.
Edited by:
Elizabeth Bobeck, Iowa State University, United StatesReviewed by:
Kyung-Woo Lee, Konkuk University, South KoreaCopyright © 2021 Akinyemi and Adewole. This is an open-access article distributed under the terms of the Creative Commons Attribution License (CC BY). The use, distribution or reproduction in other forums is permitted, provided the original author(s) and the copyright owner(s) are credited and that the original publication in this journal is cited, in accordance with accepted academic practice. No use, distribution or reproduction is permitted which does not comply with these terms.
*Correspondence: Deborah Adewole, ZGVib3JhaC5hZGV3b2xlQGRhbC5jYQ==
Disclaimer: All claims expressed in this article are solely those of the authors and do not necessarily represent those of their affiliated organizations, or those of the publisher, the editors and the reviewers. Any product that may be evaluated in this article or claim that may be made by its manufacturer is not guaranteed or endorsed by the publisher.
Research integrity at Frontiers
Learn more about the work of our research integrity team to safeguard the quality of each article we publish.