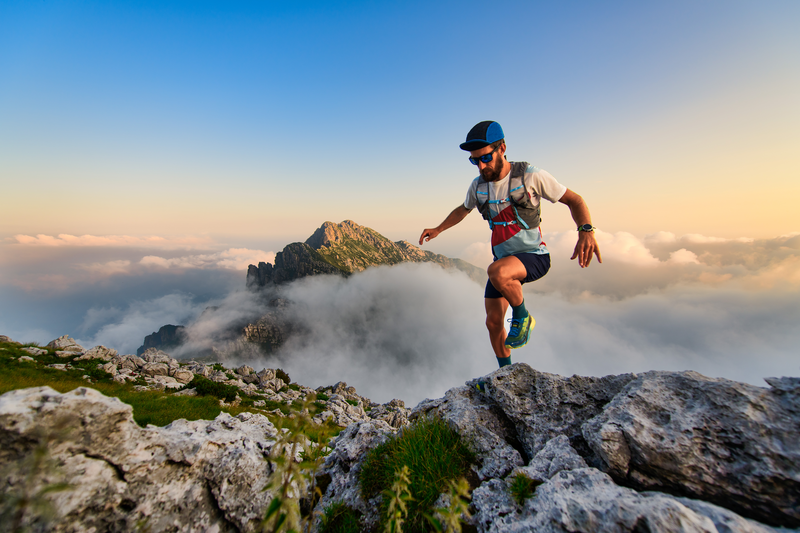
95% of researchers rate our articles as excellent or good
Learn more about the work of our research integrity team to safeguard the quality of each article we publish.
Find out more
ORIGINAL RESEARCH article
Front. Anim. Sci. , 12 July 2021
Sec. Animal Physiology and Management
Volume 2 - 2021 | https://doi.org/10.3389/fanim.2021.706636
This article is part of the Research Topic Animal-Environment Interactions View all 13 articles
The effects of heat stress on dry cows are profound and significantly contribute to lower overall welfare, productivity, and profitability of the dairy sector. Although dry cows are more thermotolerant than lactating cows due to their non-lactating state, similar environmental thresholds are currently used to estimate the degree of heat strain and cooling requirements. Records of dry cow studies conducted over 5 years in Gainesville, Florida, USA were pooled and analyzed to determine environmental thresholds at which dry cows exhibit signs of heat stress in a subtropical climate. Dry-pregnant multiparous dams were actively cooled (CL; shade of a freestall barn, fans and water soakers, n = 107) or not (HT; shade only, n = 111) during the last 7 weeks of gestation, concurrent with the entire dry period. Heat stress environmental indices, including ambient temperature, relative humidity, and temperature-humidity index (THI), and animal-based indices, including respiration rate, rectal temperature and daily dry matter intake were recorded in all studies. Simple correlations were performed between temperature-humidity index and each animal-based indicator. Differences in respiration rate, rectal temperature and dry matter intake between treatments were analyzed by multiple regression. Using segmented regression, temperature-humidity thresholds for significant changes in animal-based indicators of heat stress were estimated. Stronger significant correlations were found between the temperature-humidity index and all animal-based indices measured in HT dry cows (−0.22 ≤ r ≤ 0.35) relative to CL dry cows (−0.13 ≤ r ≤ 0.19). Although exposed to similar temperature-humidity index, rectal temperature (+0.3°C; P < 0.001) and respiration rate (+23 breaths/min; P < 0.001) were elevated in HT dry cows compared with CL cows whereas dry matter intake (−0.4 kg of dry matter/d; P = 0.003) was reduced. Temperature-humidity index thresholds at which respiration rate and rectal temperature began to change were both determined at a THI of 77 in HT dry cows. No significant temperature-humidity threshold was detected for dry matter intake. At a practical level, our results demonstrate that dry cow respiration rate and rectal temperature increased abruptly at a THI of 77 when provided only shade and managed in a subtropical climate. Therefore, in the absence of active cooling, dry cows should be closely monitored when or before THI reaches 77 to avoid further heat-stress related impairments during the dry period and the subsequent lactation and to mitigate potential carry-over effects on the offspring.
Maintaining thermoneutrality is a pre-requisite to reach optimal performance in dairy cattle (West et al., 2003). When environmental pressures such as high ambient temperature coupled with high relative temperature surpass the cow's heat dissipation capacity, core body temperature rises, reflecting heat imbalance. This condition known as heat stress triggers a myriad of physiological and behavioral responses, all aiming to decrease inner heat production and/or increase heat loss to the environment (West et al., 2003). Whether experienced during lactation or the non-lactating dry period, heat stress hinders animal production through decreases in milk yield and quality, fertility, health status, welfare, and longevity, ultimately causing severe economic losses to dairy producers in both harsh and more temperate climates (St-Pierre et al., 2003; Ferreira et al., 2016; Ouellet et al., 2020).
Heat stress in lactating and dry cows can be assessed with animal-based or environmental indicators. Animal-based indicators reflect the state of the animal in a given environment. They refer to any physiological or behavioral changes related to heat stress. On the other hand, environmental indicators refer mainly to the ambient temperature (°C), relative humidity (%), wind speed (m/s), and the calculation of the temperature-humidity index (THI) to which the animal is exposed. Originally developed in humans (Thom, 1959) and extended to dairy cows (Berry et al., 1964), this bioclimatic index combines the effects of ambient temperature and relative humidity on the animal. It is simple to calculate as both components of the equation (i.e., ambient temperature and relative humidity) are easy to measure on-farm or can be retrieved from local weather stations (Ouellet et al., 2019a). In addition, THI can predict the thermal environment of barns accurately since air movement is low and solar radiation is effectively blocked (Li et al., 2009). Animal-based indicators recognize heat stress more accurately than environmental ones, as they account for differences between individuals and differences in management systems (Galán et al., 2018). However, environmental indicators, particularly THI, remains widely used, especially in heat-stress economic studies (St-Pierre et al., 2003; Key and Sneeringer, 2014; Ferreira et al., 2016; Laporta et al., 2020), and for on-farm heat stress abatement strategies (Fournel et al., 2017). In addition, environmental indicators are practical as they allow for assessment of heat stress in retrospective studies including large numbers of animals (Bernabucci et al., 2014; Ouellet et al., 2019a).
A broad spectrum of strategies for improving cow comfort and production during heat stress can be implemented on-farm, but physical modifications of the environment are the most common (Fournel et al., 2017). These physical modifications include heat-abatement technologies that can modify the environment to limit the degree of heat stress such as misters (coupled with fans) and cooling pads or technologies that can enhance heat exchange between the cow and the environment such as soakers (coupled with fans) and fans. Practically, in dairy barns, these technologies are activated when ambient temperature or THI reaches a value at which lactating or dry cows become heat stressed.
Although it was shown recently that considerable variability exists regarding THI thresholds at which cows become heat-stressed (Pinto et al., 2020), a THI of 68 is often used as an indicator of heat stress in both lactating (Zimbelman et al., 2009), and dry cows (Ferreira et al., 2016; Fabris et al., 2019). This threshold was retrieved from a series of 8 studies where milk yield of 100 multiparous high-producing Holstein cows raised in an arid climate decreased by 2.2 kg/day for each 24 h at a daily THI of 68. Hence, the THI threshold of 68 was only determined for a decrease in milk yield in Holstein dairy cows producing more than 35 kg of milk/d raised in an arid climate. Yet, this has not been validated for physiological heat-stress responses of dry cows, which are more thermotolerant relative to lactating cows due to their non-lactating status and lower metabolic heat production (Hahn, 1999).
Heat stress experienced during the dry period affects the dam's subsequent lactation and exerts carry over effects on the progeny during its entire life (Ferreira et al., 2016; Laporta et al., 2020). In order to accurately assess heat stress in dry cows and implement proper cooling regimes on-farm to minimize heat-stress related impairments, there is a need to establish the environmental thresholds at which dry cows start exhibiting signs of heat stress. Therefore, the objectives of this study were to (1) assess the relationships between animal-based indicators and environmental indicators of heat stress in dry cows; and (2) determine environmental thresholds at which dry cows raised in a subtropical climate exhibit signs of heat stress such as increases in rectal temperature and respiration rate and a decrease in dry matter intake. We hypothesize that providing active cooling to dry cows will positively affect the critical THI threshold at which they exhibit physiological signs of heat stress. Moreover, we expect that relative to lactating cows, dry cows will show signs of heat stress at higher THI values since they have a higher upper critical temperature due to their non-lactating state and lower dry matter intake (Hahn, 1999).
Records from dry cows (n = 248) used in this study were obtained from five experiments conducted in 2007, 2009, 2015, 2016, and 2018 at the Dairy Unit of the University of Florida, located in Hague, Florida. Data collected in the experiments were pooled together and analyzed. All treatments and procedures of the experiments were approved by the Institutional Animal Care and Use Committee of the University of Florida.
Pregnant multiparous (parity 2.5 ± 0.4) Holstein cows, blocked by mature-equivalent milk production of the previous lactation and parity, were dried off ~46 d before expected calving date according to standard operating procedures of the University of Florida Dairy Unit. Upon dry-off, pregnant multiparous cows were randomly assigned to one of the two experimental treatments during the entire dry period: actively cooled (CL; n = 120; parity 2.3 ± 0.3) or heat stressed (HT; n = 128; parity 2.5 ± 0.2). The CL cows were housed under the shade of a free-stall barn equipped with fans (J&D Manufacturing, Eau Claire, WI) that ran continuously, and soakers (Rain Bird Manufacturing, Glendale, CA) that turned on for 1.5 min duration at a 5-min interval when ambient temperature exceeded 21°C, while HT cows were only provided with the shade of the free-stall barn. Cows of both treatments were fed the same diet, housed in a sand-bedded free-stall barn and remained in their treatments until calving. All experiments were conducted from May to November at the exception of the 2018 trial, which was conducted from the end of May until October. Studies from do Amaral et al. (2009), Tao et al. (2011), and Fabris et al. (2019) may be referred to for dry cow diet composition, and access to water was ad libitum in all studies.
Experimental pens were equipped with Hobo Pro series Temp probes (Onset Computer Corp., Pocasset, MA), which were set to continuously record air temperature (°C) and relative humidity (%) every 15 min. From these variables, THI was calculated following the equation developed by the National Research Council (1971) and recommended for use in subtropical environments by Dikmen and Hansen (2009):
where T = dry bulb temperature (°C) and RH = relative humidity (%). Temperature-humidity index was then averaged per day, and daily THI was used for further analysis.
In all experiments, rectal temperature (RT, °C) was measured twice daily (~0730 and 1,430 h, to capture the morning and afternoon conditions of the barn) using a GLA M700 digital thermometer (GLA Agricultural Electronics, San Luis Obispo, CA). Rectal temperature was averaged daily. Respiration rate (RR, breaths/min) was measured thrice weekly by counting the flank movements for 1 min for all cows during the duration of the dry period. Vaginal temperatures (VT, °C) were measured every 10 min using i-button (iBUTTONLINK, Whitewater, WI) installed in a modified internal drug-release device without progesterone (CIDR, InterAg, Hamilton, New Zealand) inserted into the vagina. Vaginal temperatures were then averaged hourly. Finally, daily dry matter intake (DMI, kg/d) of individual cows was measured during the entire dry period using a Calan gate system (American Calan Inc., Northwood, NH).
Records of dry cows with missing environmental or physiological data were excluded in the current analysis. Moreover, VT was dropped from the current study as it was only measured in two experiments. After data mining, records from 216 dry cows that were either exposed to active cooling by soakers and fans during the entire dry period (n = 107) or only provided with the shade of the barn (n = 111) were used. Observations collected during the last week before calving was removed from the analysis to avoid bias related to calving as RT and DMI can fluctuate with the approach of parturition (Jensen, 2012; Ouellet et al., 2016).
All statistical analyses were performed in SAS (version 9.4, SAS Institute, Inc.). Data were first tested for covariance (Levene's test) and normality of distribution was tested by evaluating Shapiro-Wilk statistics using the Univariate procedure.
To explore the linear relationship between animal-based and environmental indicators, and between environmental indicators, Pearson correlations were calculated using the CORR procedure. Correlations were carried out by treatment (CL or HT) based on individual observations measured from dry-off until one week pre-calving. Differences among correlations coefficients between treatments were tested with a Fisher-type Z-transformation and significance was set at p ≤ 0.05.
Repeated measures of THI, RR, RT and DMI were analyzed by ANOVA using the MIXED procedure. The model included fixed effects of treatment (TRT) during the dry period (CL or HT), year of the experiment (year), weeks relative to calving (WRC), and animal within TRT as a random effect. Statistical comparisons were made by Tukey-Kramar testing and significance was set at p ≤ 0.05. Finally, two-phase segmented regressions were performed on the least square means retrieved from the mixed models when daily THI was added to the model. Segmented regressions were created using the NLIN procedure to detect THI breakpoints from 68 to 82 at which physiological variables begin to significantly (p < 0.05) rise or decline in CL and HT dry cows.
In CL dry cows, Pearson correlation coefficients (r) observed between THI and animal-based indicators ranged from r = −0.13 to r = 0.19 (Figure 1). Among all correlation coefficients, the strongest relationship was obtained between THI and RT, represented here by the greater correlation coefficients (r = 0.19; P < 0.001), whereas the weakest correlation was obtained between THI and DMI (r = −0.19; P < 0.001). Although all significant (P < 0.001), correlations in CL cows between THI and animal-based indicators were collectively weak.
Figure 1. Relationship between (A) rectal temperature, (B) respiration rate, (C) dry matter intake and temperature-humidity index in dry cows exposed to active continuous cooling (shade, soakers and fans) or heat stress (shade only). Observations were collected from 60 to 7 d before calving. The points • indicate cooling, and the points • indicate heat stress. Lines represent simple linear regression equations, r represents the correlation coefficient, P represents significance of the correlation, and z-score P represents differences between correlations coefficients of both treatments.
All Z-scores were significantly different between CL and HT dry cows (Figure 1; P < 0.001). Relative to CL dry cows, stronger correlations were observed between THI and animal-based indicators in HT dry cows, with Pearson correlations coefficients ranging from r = −0.22 to r = 0. 35 (Figure 1). As observed in CL dry cows, the strongest correlation was obtained between THI and RT (r = 0.35; P < 0.001), followed by the correlation between THI and RR (r = 0.32; P < 0.001). The weakest correlation was measured between THI and DMI (r = −0.22; P < 0.001).
In CL dry cows, Pearson correlation coefficients between the different animal-based indicators ranged from r = −0.14 to r = 0.12. No significant relationship was observed between DMI and RR (r = 0.02; P = 0.46) (Figure 2). A weak, yet significant, positive correlation was observed between RR and RT (r = 0.12; P < 0.001); whereas a weak significant negative correlation was measured between DMI and RT (r = −0.14; P < 0.001).
Figure 2. Relationship between (A) respiration rate and rectal temperature, (B) dry matter intake and rectal temperature, (C) dry matter intake and respiration rate in dry cows exposed to active continuous cooling (shade, soakers and fans) or heat stress (shade only). Observations were collected from 60 to 7 d before calving. The points • indicate cooling, and the points • indicate heat stress. Lines represent simple linear regression equations, r represents the correlation coefficient, P represents significance of the correlation, and z-test P represents differences between correlations coefficients of both treatments.
Relative to CL cows, stronger correlations were measured between the different animal-based indicators in HT cows (Figure 2). Pearson correlation coefficients ranged from r = 0.31 to r = −0.14. The strongest correlation was obtained between RR and RT (r = 0.31; P < 0.001). Negative and weaker correlations were measured between DMI and RT (r = −0.14; P < 0.001), and DMI and RR (r = −0.12; P < 0.001).
Ambient temperature and relative humidity were monitored continuously in all experiments in order to calculate daily THI. Daily THI did not differ between treatments (CLTHI = 76.35 vs. HTTHI = 76.45 ± 0.16; P = 0.16) in all years evaluated (Table 1). Daily THI calculated from May 1 to November 30 in 2007, 2009, 2015, 2016, and 2018, and averaged in both experimental pens (HT and CL) are depicted in Figure 3. The 2007 mean (± SD) daily THI was 74.6 ± 8.2, ranging from 47.1 to 82.5. The 2009 mean (± SD) daily THI was 72.3 ± 6.7, ranging from 48.1 to 82.2. The 2015 mean (± SD) daily THI was 74.0 ± 5.3, ranging from 49.1 to 81.4. The 2016 mean (± SD) daily THI was 73.3 ± 6.9, ranging from 48.1 to 82.5. Finally, the 2018 mean (± SD) daily THI was 77.6 ± 2.4, ranging from 70.4 to 81.5.
Table 1. Temperature-humidity index, respiration rate, rectal temperature and dry matter intake in dry cows exposed to active cooling (CL; shade, soakers and fans, n = 107) or heat stress (HT; shade only, n = 111).
Figure 3. Mean (CL and HT pens) daily temperature-humidity index (THI) calculated according to the equation developed by the National Research Council (1971) with temperature and humidity data collected from May 1 to November 30 (years 2007, 2009, 2015, 2016, and 2018) at the University of Florida Dairy Unit (Hague, FL). Dashed line represents a THI threshold of 68 determined in lactating cows but often used in dry cows to assess heat stress (Zimbelman et al., 2009).
Despite being exposed to similar thermal environments, physiological responses to the environment varied between treatments (Table 1). Notably, HT dry cows had a mean RT that was 0.3°C higher relative to CL dry cows (HT = 39.23 vs. CL = 38.92 ± 0.01°C; P treatment < 0.001). They also had increased RR (HT = 72 vs. CL = 49 ± 0.6 breaths/min: Ptreatment < 0.001) relative to CL dry cows. In addition, HT dry cows had reduced DMI of 0.4 kg/d compared with CL dry cows (HT = 11.01 vs. CL = 11.41 ± 0.06 kg/d; Ptreatment = 0.003).
Temperature-humidity index thresholds were determined when abrupt and significant changes in the animal-based indicators were detected in CL and HT dry cows above a particular THI value (Figure 4). Dry cows exposed to heat-stress had a THI breakpoint of 77 for RT, whereby RT began rising at a rate of 0.12°C for every unit increase in THI above the threshold (Figure 4A). No abrupt and significant change in RT was detected in dry cows provided active cooling (Figure 4A). In addition, HT dry cows had a THI threshold of 77 for RR, whereby RR began rising at a rate of 2.04 breaths/min for every increase of THI (Figure 4B). Relative to HT dry cows, CL dry cows had a lower THI threshold for RR of 75, whereby RR began rising at a rate of 1.98 breaths/min for every increase of THI unit (Figure 4B). No abrupt and significant change in DMI was detected within the THI range of 68 to 82 evaluated herein for CL or HT dry cows.
Figure 4. Segmented regressions of (A) rectal temperature (°C), (B) respiration rate (breaths/min) least square means relative to temperature-humidity index (THI) rate in dry cows exposed to active cooling (shade, soakers and fans, n = 107) or heat stress (shade only, n = 111). Vertical dashed lines indicate breakpoint at which the dependent variables changed significantly in cooled dry cows (—) and in heat-stressed dry cows (—). Δb represents the change in slope between b1 (i.e., slope of data before breakpoint) and b2 (slope of data after breakpoint). ■ and ■ represent 95% confidence interval.
Heat tolerance of dairy cows depends on factors intrinsic to the animal (age, body mass, stage of lactation, physiological status, milk production, genetics), but also to extrinsic factors (the climate in which the animals are managed) (Kadzere et al., 2002). Temperature-humidity index is often used to assess heat tolerance in dairy cows (Galán et al., 2018). It is well-established that dry cows are more thermotolerant compared with lactating cows due to their lower metabolic heat production (Hahn, 1999). However, a THI threshold of 68, which was determined for a decline in milk yield in lactating cows raised in an arid climate, is often used to assess heat stress in dry cows. To the best of our knowledge, no THI threshold at which dry cows start exhibiting signs of heat stress (i.e., increased RR and RT) currently exists. Herein, we establish animal-based and THI associations, differences in physiological responses to heat stress and THI breakpoints for dry cows exposed to chronic heat stress or continuous heat abatement by soakers and fans in a shaded, subtropical environment.
As previously demonstrated in lactating cows (Dikmen and Hansen, 2009) and in dairy calves (Dado-Senn et al., 2020) raised in a subtropical environment, significant correlations between THI and animal-based indicators of heat stress can be assessed in dry cows. Herein, we chose THI over other environmental indicators of heat stress (i.e., ambient temperature, relative humidity) as the cows dissipate heat to the environment via processes of conduction, convection, radiation, and evaporation (West et al., 2003). Whereas, the processes of conduction, convection, and radiation are all dependent on a thermal gradient, evaporation depends on a water gradient. Thus, heat dissipation capacity of the cow is reduced as ambient temperature and relative humidity rise above a critical point. Therefore, heat dissipation capacity of the cow depends on both ambient temperature and relative humidity. These two variables are accounted for in THI calculations. In addition, THI remains the most common method of evaluating the risk of heat stress in cows in research literature (Galán et al., 2018; Hoffmann et al., 2019).
Regardless of the thermal treatment imposed to the dry cows, the strongest correlation between THI and an animal-based indicator of heat stress was obtained between RT and THI. This is not surprising as RT was shown to be an accurate predictor of core body temperature and is the standard for assessing homeothermy and heat stress (Yousef, 1987; Umphrey et al., 2001). Interestingly, correlation coefficients obtained between RT and THI in the current study are lower than what is reported in lactating cows raised in a similar environment, and when the same equation is used in THI calculation (Dikmen and Hansen, 2009). This could potentially indicate that the core body temperature of lactating cows is more responsive to an increase in THI compared with dry cows, and that lactating cows are more vulnerable to harsher environmental conditions relative to dry cows.
In the current study, correlations measured between THI and RT, RR and DMI were stronger in HT dry cows relative to CL dry cows. This was expected as the addition of fans and soakers enhances the heat dissipation of the cows to the environment without impacting the environmental conditions of the barn as demonstrated by similar THI in both treatments. Therefore, the provision of active cooling devices as a heat abatement method can skew the relationship between THI and the animal-based indicators of heat stress. These results are consistent with a previous study conducted in a similar climate by our group where correlations between environmental and animal-based indicators of heat stress were stronger in dairy calves only provided with the shade of the barn compared to dairy calves exposed to continuous cooling by fans (Dado-Senn et al., 2020).
Regardless of the thermal treatment imposed on the dry cows, positive and significant correlations were obtained between RR and RT. This is consistent with previous findings which indicated that an increase in heat load in dry cows can trigger evaporative heat dissipation mechanisms such as an increase in respiration rate (Silanikove, 2000). This was further confirmed in our study, as CL dry cows had a reduced RR compared to the HT dry cows. Negative and significant correlations were observed in both treatments between DMI and RT. Moreover, HT dry cows consumed less feed daily relative to CL dry cows. This decrease in DMI under elevated core body temperature has been reported in both dry cow and lactating cow studies (though to a higher extent in lactating cows), as cattle will consume less feed in order to generate less metabolic heat and to reduce their heat load (Adin et al., 2009; Wheelock et al., 2010; Fabris et al., 2017). Negative correlations were also observed between DMI and RR in both treatments. However, it was only statistically significant for HT dry cows.
In the past twenty years, several THI thresholds at which an abrupt physiological change related to heat stress is triggered were determined in studies conducted in different climates in lactating cows or, more recently, in dairy calves. For instance, the threshold at which a decline in milk yield is observed in an arid climate has been set at THI values ranging from 68 to 72, depending on the production level of the animal with high producing cows being less heat tolerant relative to low producing cows (Ravagnolo et al., 2000; Zimbelman et al., 2009). In contrast, THI values ranging from 60 to 65 were associated with a decline in milk production or milk components production in temperate and continental climates (Brügemann et al., 2011; Hammami et al., 2013; Ouellet et al., 2019b), and a THI value of 52 was recently associated with a reduced rumination time (Müschner-Siemens et al., 2020). In dairy calves exposed to chronic heat stress, THI thresholds of 67, 65, and 82 were respectively determined for an increase in RT and RR and a decrease of milk replacer intake (Dado-Senn et al., 2020). Collectively, THI thresholds at which cattle become heat-stressed varies greatly according to the production level of the animal, the physiological status, the climate where the animal was reared, genetics, and the physiological response measured. These THI thresholds are primarily used in the literature and on-farm to determine whether an animal is susceptible to heat stress (Bernabucci et al., 2014; Ouellet et al., 2019a) or to estimate heat-stress related economic losses (St-Pierre et al., 2003; Key and Sneeringer, 2014; Ferreira et al., 2016; Laporta et al., 2020). In addition, such thresholds can be utilized to determine the proper timing of heat-abatement initiation to prevent production losses (Galán et al., 2018; Pinto et al., 2020).
In order to accurately assess heat stress in dry cows, variation in THI threshold at which the animal begins to experience signs of heat stress must be considered. In the present study, THI thresholds could be detected in RT and RR in HT dry cows, and only for RR in CL dry cows. Regardless of the environment to which the dry cow is exposed, no significant threshold could be detected for DMI. In order to detect a significant THI threshold with the methodology used in the current study (two-phase linear regression), the measured physiological response has to exhibit a plateau under a range of THI values, and then a sudden increase or decrease above a particular THI value. The absence of THI threshold for a decline in DMI could be reflective of the small and steady decrease in DMI as THI increases. However, this suggestion requires further studies with a wider time frame to capture a broader THI range in the analysis.
Interestingly, our results indicated that THI threshold was similar for an increase in RT and RR in HT dry cows. Below a THI of 77, dry cows exposed to heat stress in this study were able to maintain a relatively constant RT and RR. Above this threshold, a significant and abrupt increase in RT and RR was detected. The THI threshold of 77 observed in our data set is considerably higher compared to the threshold of 68 reported for a decrease in milk production in lactating cows, perhaps reflective of the higher thermotolerance of the dry cows. Due to the lack of a dry cow threshold, the THI of 68 has been extensively used in dry cow studies, but our results reinforce the idea that heat threshold determined in lactating cows do not accurately assess heat stress in dry cows. Relative to HT dry cows, a lower THI threshold was identified in CL dry cows for a significant increase in RR, which is counterintuitive considering that they had access to a cooling system. However, throughout the experimental period, RR of CL dry cows remained below a rate of 61 breaths/min, which was recently associated with heat stress in dry cows (Toledo et al., 2020). Thus, although a significant threshold of 75 was identified in CL dry cows, these cows were not suffering from heat stress. At a practical level, our results indicate that dry cows without active cooling in a subtropical climate should be closely monitored for thermal discomfort when THI reaches 77.
Herein, we demonstrate that THI showed moderate correlations with animal-based indicators of heat stress in dry cows when no heat abatement beyond the shade of the barn is provided. Providing heat abatement to dry cows through active cooling provision does not affect the surrounding environment but decreases dry cow rectal temperature and respiration rate and increases dry matter intake relative to dry cows without heat abatement. The THI thresholds at which the animal-based indicators started to change varied depending on the thermal environment to which the cow was exposed but not the physiological response measured. Dry cows not provided with active cooling should be closely monitored when or before THI reaches 77, as the expected rise in RT and RR estimated herein is associated with thermal discomfort and heat stress. It is now clear that the THI threshold established for lactating cows are not suitable to accurately assess heat stress in dry cows. Our results should aid with proper identification and monitoring of heat-stress in dry cows. This is vital to improve dry cow welfare and provide accurate management of dry cows in a subtropical climate.
The original contributions presented in the study are included in the article/supplementary material, further inquiries can be directed to the corresponding author.
The animal study was reviewed and approved by Institutional Animal Care and Use Committee of the University of Florida.
All authors have made a substantial direct and intellectual contribution to the work ranging from inception of the project idea, funding, project implementation and management, data collection and analysis, writing, and editing of the manuscript. All authors have reviewed and approved the final version of this manuscript.
This study was supported by the USDA-Agriculture and Food Research Institute (USDA/NIFA AFRI, Washington, DC) Foundational Program Award 2019-67015-29445 and awarded to JL, and multiple grants that supported the experiments awarded to GED (USDA/NIFA AFRI #2015-67015-23409, #2010-85122-20623, and NSF#1247362).
The authors declare that the research was conducted in the absence of any commercial or financial relationships that could be construed as a potential conflict of interest.
We thank the University of Florida Dairy Unit staff and veterinarians for their assistance in animal care and the graduate students in our programs that collected the animal based data used in this study.
Adin, G., Gelman, A., Solomon, R., Flamenbaum, I., Nikbachat, M., Yosef, E., et al. (2009). Effects of cooling dry cows under heat load conditions on mammary gland enzymatic activity, intake of food water, and performance during the dry period and after parturition. Livest. Sci. 124, 189–195. doi: 10.1016/j.livsci.2009.01.014
Bernabucci, U., Biffani, S., Buggiotti, L., Vitali, A., Lacetera, N., and Nardone, A. (2014). The effects of heat stress in Italian holstein dairy cattle. J. Dairy Sci. 97, 471–486. doi: 10.3168/jds.2013-6611
Berry, I., Shanklin, M., and Johnson, H. (1964). Dairy shelter design based on milk production decline as affected by temperature and humidity. Trans. ASAE 7, 329–331. doi: 10.13031/2013.40772
Brügemann, K., Gernand, E., von Borstel, U. U., and König, S. (2011). Genetic analyses of protein yield in dairy cows applying random regression models with time-dependent and temperature x humidity- dependent covariates. J. Dairy Sci. 94, 4129–4139. doi: 10.3168/jds.2010-4063
Dado-Senn, B., Ouellet, V., Dahl, G. E., and Laporta, J. (2020). Methods for assessing heat stress in preweaned dairy calves exposed to chronic heat stress or continuous cooling. J. Dairy Sci. 103, 8587–8600. doi: 10.3168/jds.2020-18381
Dikmen, S., and Hansen, P. J. (2009). Is the temperature-humidity index the best indicator of heat stress in lactating dairy cows in a subtropical environment? J. Dairy Sci. 92, 109–116. doi: 10.3168/jds.2008-1370
do Amaral, B. C., Connor, E. E., Tao, S., Hayen, M. J., Bubolz, J. W., and Dahl, G. E. (2009). Heat-stress abatement during the dry period: Does cooling improve transition into lactation? J. Dairy Sci. 92, 5988–5999. doi: 10.3168/jds.2009-2343
Fabris, T. F., Laporta, J., Corra, F. N., Torres, Y. M., Kirk, D. J., McLean, D. J., et al. (2017). Effect of nutritional immunomodulation and heat stress during the dry period on subsequent performance of cows. J. Dairy Sci. 8, 6733–6742. doi: 10.3168/jds.2016-12313
Fabris, T. F., Laporta, J., Skibiel, A. L., Corra, F. N., Dado-Senn, B., Wohlgemuth, S. E., et al. (2019). Effect of heat stress during early, late, and entire dry period on dairy cattle. J. Dairy Sci. 102, 5647–5656. doi: 10.3168/jds.2018-15721
Ferreira, F. C., Genari, R. S., Dahl, G. E., and de Vries, A. (2016). Economic feasibility of cooling dry cows across the United States. J. Dairy Sci. 99, 9931–9941. doi: 10.3168/jds.2016-11566
Fournel, S., Ouellet, V., and Charrbonneau, É. (2017). Practices for alleviating heat stress of dairy cows in humid continental climates: a literature review. Animals 7:37. doi: 10.3390/ani7050037
Galán, E., Llonch, P., Villagrá, A., Levit, H., Pinto, S., and del Prado, A. (2018). A systematic review of non-productivity-related animal-based indicators of heat stress resilience in dairy cattle. PLoS ONE 13:e0206520. doi: 10.1371/journal.pone.0206520
Hahn, G. L. (1999). Dynamic responses of cattle to thermal heat loads. J. Anim. Sci. 77, 10–20. doi: 10.2527/1997.77suppl_210x
Hammami, H., Bormann, J., M'hamdi, N., Montaldo, H. H., and Gengler, N. (2013). Evaluation of heat stress effects on production traits and somatic cell score of holsteins in a temperate environment. J. Dairy Sci. 96,1844–1855. doi: 10.3168/jds.2012-5947
Hoffmann, G., Herbut, P., Pinto, S., Heinicke, J., and Kuhla, B. É, Amon, T. (2019). Animal-related, non-invasive indicators for determining heat stress in dairy cows. Biosyst. Eng. 199, 83–96. doi: 10.1016/j.biosystemseng.2019.10.017
Jensen, M. B. (2012). Behaviour around the time of calving in dairy cows. Appl. Anim. Behav. Sci. 139, 195–202. doi: 10.1016/j.applanim.2012.04.002
Kadzere, C. T., Murphy, M. R., Silanikove, N., and Maltz, E. (2002). Heat stress in lactating dairy cows: a review. Livest. Prod. Sci. 77, 59–91. doi: 10.1016/S0301-6226(01)00330-X
Key, N., and Sneeringer, S. (2014). Potential effects of climate change on the productivity of U.S. dairies. Am. J. Agric. Econ. 96,1136–1156. doi: 10.1093/ajae/aau002
Laporta, J., Ferreira, F. C., Ouellet, V., Dado-Senn, B., Almeida, A. K., de Vries, A., et al. (2020). Late-gestation heat stress impairs daughter and granddaughter lifetime performance. J. Dairy Sci. 193, 7555–7568. doi: 10.3168/jds.2020-18154
Li, S., Gebremedhin, K. G., Lee, C. N., and Collier, R. J. (2009). “Evaluation of thermal stress indices for cattle,” in 2009 ASABE Annual Meeting (St. Joseph, MI: ASABE).
Müschner-Siemens, T., Hoffmann, G., Ammon, C., and Amon, T. (2020). Daily rumination time of lactating dairy cows under heat stress conditions. J. Therm. Biol. 88:102484. doi: 10.1016/j.jtherbio.2019.102484
National Research Council (1971). A Guide to Environmental Research on Animals. Washington, DC: National Academy of Science.
Ouellet, V., Bellavance, A. L., Fournel, S., and Charbonneau, É. (2019a). Short communication: summer on-farm environmental condition assessments in Québec tiestall farms and adaptation of temperature humidity index calculated with local meteorological data. J. Dairy Sci. 102, 7503–7508. doi: 10.3168/jds.2018-16159
Ouellet, V., Cabrera, V. E., Fadul-Pacheco, L., and Charbonneau, E. (2019b). The relationship between the number of consecutive days with heat stress and milk production of holstein dairy cows raised in a humid continental climate. J. Dairy Sci. 102,8537–8545. doi: 10.3168/jds.2018-16060
Ouellet, V., Grenier, P., Santschi, D. E., Cabrera, V. E., Fadul-Pacheco, L., and Charbonneau, É. (2020). Projected economic losses from milk performance detriments under heat stress in Quebec dairy herds. Can. J. Anim. 101, 242–56. doi: 10.1139/cjas-2020-0069
Ouellet, V., Vasseur, E., Heuwieser, W., Bufeind, O., Maldagure, X., and Charbonneau, É. (2016). Evaluation of calving indicators measured by automated monitoring devices to predict the onset of calving in Holstein dairy cows. J. Dairy Sci. 99, 1539–1548. doi: 10.3168/jds.2015-10057
Pinto, S., Hoffmann, G., Ammon, C., and Amon, T. (2020). Critical THI thresholds based on the physiological parameters of lactating dairy cows. J. Therm. Biol. 88:102523. doi: 10.1016/j.jtherbio.2020.102523
Ravagnolo, O., Misztal, I., and Hoogenboom, G. (2000). Genetic component of heat stress in dairy cattle, development of heat index function. J. Dairy Sci. 83, 2120–2125. doi: 10.3168/jds.S0022-0302(00)75094-6
Silanikove, N. (2000). Effects of heat stress on the welfare of extensively managed domestic ruminants. Livest. Prod. Sci. 67, 1–18. doi: 10.1016/S0301-6226(00)00162-7
St-Pierre, N. R., Cobanov, B., and Schnitkey, G. (2003). Economic losses from heat stress by US livestock industries. J. Dairy Sci. 86, E52–E77. doi: 10.3168/jds.S0022-0302(03)74040-5
Tao, S., Bubolz, J. W., do Amaral, B. C., Thompson, I. M., Hayen, M. J., Johnson, S. E., et al. (2011). Effect of heat stress during the dry period on mammary gland development. J. Dairy Sci. 94, 5976–5986. doi: 10.3168/jds.2011-4329
Toledo, I. M., Fabris, T. F., Tao, S., and Dahl, G. E. (2020). When do dry cows get heat stressed? Correlations of rectal temperature, respiration rate, and performance. JDS Commun. 1, 21–24. doi: 10.3168/jdsc.2019-18019
Umphrey, J. E., Moss, B. R., Wilcox, C. J., and Van Horn, H. H. (2001). Interrelationships in lactating holsteins of rectal and skin temperatures, milk yield and composition, dry matter intake, body weight, and feed efficiency in summer in Alabama. J. Dairy Sci. 84, 2680–2685. doi: 10.3168/jds.S0022-0302(01)74722-4
West, J. W., Mullinix, B. G., and Bernard, J. K. (2003). Effects of hot, humid weather on milk temperature, dry matter intake, and milk yield of lactating dairy cows. J. Dairy Sci. 8, 232–242. doi: 10.3168/jds.S0022-0302(03)73602-9
Wheelock, J. B., Rhoads, R., VanBaale, M., Sanders, S., and Baumgard, L. H. (2010). Effects of heat stress on energetic metabolism in lactating Holstein cows. J. Dairy Sci. 93,644–655. doi: 10.3168/jds.2009-2295
Yousef, M. K. (1987). Principle of Bioclimatology and Adaptation. Amsterdam: Elsevier Science Publisher.
Zimbelman, R. B., Rhoads, R. P., Rhoads, M. L., Duff, G. C., Baumgard, L. H., and Collier, R. J. (2009). “A re-evaluation of the impact of temperature humidity index (THI) and black globe humidity index (BGHI) on milk production in high producing dairy cows,” in Proceedings of Southwest Nutrition and Management Conference (Tucson, AZ: University of Arizona, Tucson), 158–168.
Keywords: hyperthermia, indicator, dry cow management, environment, heat stress
Citation: Ouellet V, Toledo IM, Dado-Senn B, Dahl GE and Laporta J (2021) Critical Temperature-Humidity Index Thresholds for Dry Cows in a Subtropical Climate. Front. Anim. Sci. 2:706636. doi: 10.3389/fanim.2021.706636
Received: 07 May 2021; Accepted: 18 June 2021;
Published: 12 July 2021.
Edited by:
Pasquale De Palo, University of Bari Aldo Moro, ItalyReviewed by:
Vincenzo Lopreiato, Catholic University of the Sacred Heart, ItalyCopyright © 2021 Ouellet, Toledo, Dado-Senn, Dahl and Laporta. This is an open-access article distributed under the terms of the Creative Commons Attribution License (CC BY). The use, distribution or reproduction in other forums is permitted, provided the original author(s) and the copyright owner(s) are credited and that the original publication in this journal is cited, in accordance with accepted academic practice. No use, distribution or reproduction is permitted which does not comply with these terms.
*Correspondence: Jimena Laporta, amxhcG9ydGFAd2lzYy5lZHU=
Disclaimer: All claims expressed in this article are solely those of the authors and do not necessarily represent those of their affiliated organizations, or those of the publisher, the editors and the reviewers. Any product that may be evaluated in this article or claim that may be made by its manufacturer is not guaranteed or endorsed by the publisher.
Research integrity at Frontiers
Learn more about the work of our research integrity team to safeguard the quality of each article we publish.