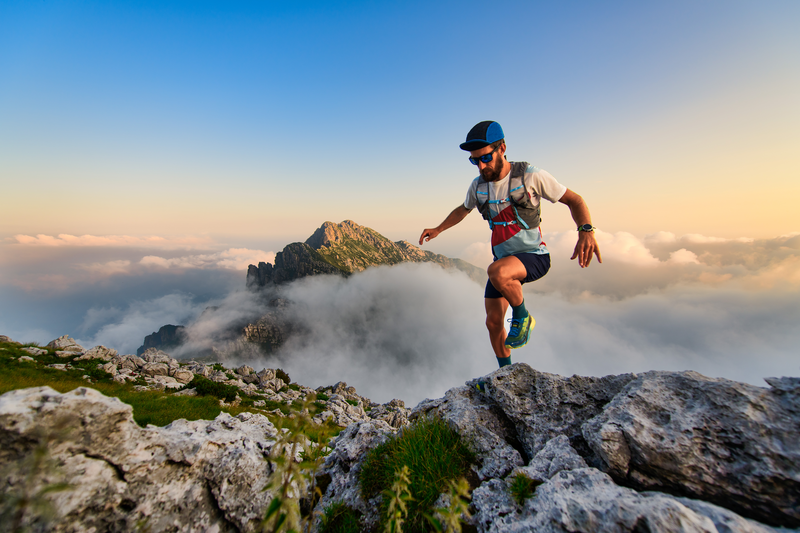
94% of researchers rate our articles as excellent or good
Learn more about the work of our research integrity team to safeguard the quality of each article we publish.
Find out more
REVIEW article
Front. Anesthesiol. , 20 February 2025
Sec. Perioperative Medicine
Volume 4 - 2025 | https://doi.org/10.3389/fanes.2025.1454904
This article is part of the Research Topic Patient Blood Management and Transfusion Strategies in Perioperative Settings View all articles
Newborns and infants undergoing complex heart surgery often encounter post-operative bleeding episodes and coagulopathy following cardiopulmonary bypass (CPB). Addressing these challenges necessitates a comprehensive understanding of the intricate mechanisms involved, including thrombin generation, platelet activation, fibrinolysis, and the pivotal role of fibrinogen. This article explores these critical components within the context of pediatric patients undergoing cardiac surgery with CPB, drawing from Hoffman and Monroe's cell-based coagulation approach. By shedding light on these key aspects, we aim to equip clinicians with essential insights for designing a well-guided patient blood management strategy in this type of surgery.
Bleeding and coagulation disturbances are common following surgery for congenital heart disease and the use of the cardiopulmonary bypass (CPB) in neonates and infants (1). The magnitude of these disturbances may directly relate to poor postoperative outcomes including the need for extracorporeal membrane oxygenation (ECMO) and renal replacement therapy. Numerous predictors for excessive bleeding loss and coagulopathy during Pediatric cardiac surgeries are described in published literature. Excessive bleeding loss, reducing coagulation factors will lead to coagulopathy which is influenced by conditions such as a low body temperature, re-sternotomy, prolonged cardiopulmonary by-pass duration, and patient body weight <8 Kg (2, 3).
The hemostasis and coagulation in pediatric cardiac surgery patients present particularities. They tend to have more coagulation problems due to their small blood volume, and the use of CPB machine, inducing an inflammatory reaction. The other challenges are related to the surgical procedure, and the particularities of their coagulation system compared to the adult population. In addition, compared to adult patients, the circuit size is larger in the pediatric population considering their body weight and volume, therefore increasing the effect of hemodilution in the patient. Furthermore, in pediatric patients, the bypass time is often longer and increases the patient's risk of coagulation disorders. Therefore, coagulopathy in this population is more challenging.
Previous publications found that the perioperative management of blood transfusions in pediatric patients undergoing complex cardiac surgeries varies between teams and institutions (4). Also, blood derivative products, adjunct drugs for perioperative coagulation therapy in patient management protocols, and algorithms used by pediatric cardiac anesthesiologist teams might be different. These differences include the specific surgical team techniques, transfusion strategies, blood derivative products availability, threshold for transfusion of blood products, and population variability. This might be an important point to consider when attempting to harmonize practice within institutions.
As the troubles of hemostasis and coagulation in pediatric patients may cause serious derangements in the perioperative course, a thorough understanding of the normal mechanisms of the coagulation as well as the possible specific coagulation disorders encountered in these procedures warrants a better-designed management strategy for bleeding and coagulopathy, and guide the specific interventions needed for replacement therapies.
Two approaches have been described to explain the mechanism of coagulation. The traditional approach describing the intrinsic and extrinsic phases of coagulation presented some limits because, in vivo, these phases could not act independently as they did in vitro (5). A more recent and realistic approach described by Hoffman and Monroe gives a more accurate explanation of the events happening simultaneously during bleeding episodes and leading to clot formation (6). Following blood loss during the perioperative period for complex pediatric cardiac surgery, platelet activation will help with the initiation and propagation of the thrombus formed. While fibrinogen will be needed early, a constant equilibrium should be maintained between thrombin generation, the level of Antithrombin, and later the activation of the fibrinolytic system. These findings applied to the coagulation derangement occurring during pediatric cardiac surgery could help also in the management strategies to use for these patients. In this review, we present the general mechanism of the coagulation based on the cellular approach of coagulation and we discuss the main phases of this process impacting bleeding and coagulopathy during pediatric cardiac surgery.
The basic coagulation model with separation into the major components, the intrinsic and extrinsic cascades, has been used for decades to explain the process from the initiation of coagulation to the formation and stabilization of the fibrin clot (5). After progress in the understanding of biochemistry and coagulation factors, a more accurate model was developed, describing three phases of coagulation occurring on different cell surfaces including the initiation phase, occurring on the tissue-factor bearing fibroblasts followed by amplification and propagation phases, occurring on the activated platelet surface (6).
In the intact and uninjured tissue, fibroblasts and cells containing tissue factor (TF), otherwise known as tissue platelet factor, reside in the subendothelial space, separated from the vessel lumen. Following vascular injury, these subendothelial TF-bearing cells express TF (Tissue factor) which binds to and activates FVII. Activated factor VII activates factors IX and X on the surface of activated platelets (7). The location of factor VIIa on the platelet surface could along with factors IXa and Xa augment the pro-coagulant effect of this pathway as these factors are protected from inhibition by plasma protease inhibitors (7). The complex formed by factor III, factor VIIa, factor Xa, and calcium will play a significant role in this process. Activated factor X binds to factor Va to form the prothrombinase complex. Activated factors X and V can also directly activate factor II (prothrombin), leading to the formation of thrombin. The amount of thrombin formed during this phase is small and will initiate a feedback loop leading to the amplification and the propagation phase with the generation of significant thrombin (6).
During the amplification phase, two important events occur: the small amount of thrombin generated during the initiation phase amplifies coagulation by enhancing platelet adhesion and activation with the activation of additional amounts of factors V, VIII, and XI. The factor VIIa/III complex and factor XIa activate factor IX. Activated factor IX binds to factor VIIIa to form the “tenase complex”. This tenase complex will then activate more factor X than had previously been activated by the TF, factor VIIa, factor Xa, calcium complex, and later create a wide cascade of reactions accelerating thrombin generation. This thrombin burst is well known as the propagation phase and takes place at the platelet surface after the tenase and the prothrombinase complexes bind. Thrombin formed will then convert fibrinogen to fibrin and in addition, will activate FXIII to form FXIIIa which cross-links the fibrin stands, stabilizing the clot. The result of these concurrent steps is the formation of a mural platelet thrombus that forms a scaffold to initiate coagulation, hemostasis, and healing of the injured vessel (Figure 1). To prevent intravascular coagulation and control the thrombotic aspects of the coagulation cascade, there are 3 main anti-thrombotic processes including anti-thrombin III, tissue factor pathway inhibitor (TFPI), which inhibits the TF, factor VIIa, factor Xa, calcium complex, and the protein C pathway with its cofactor protein S.
Figure 1. The cell-based model of hemostasis and modulating factors during pediatric complex cardiac surgery with cardiopulmonary bypass. CPB, cardiopulmonary bypass; GA, general anesthesia; MUF, modified ultrafiltration; TG, thrombin generation; TAFI, thrombin activatable fibrinolysis inhibitor; TXA, tranexamic acid; EACA, epsilon amino caproic acid; 3F-PCC, three factors prothrombin complex concentrate; 4F-PCC, four factors prothrombin complex concentrate; tenase complex, factor IXa and factor VIIIa; prothrombinase complex, FVa and FXa.
There are significant age-specific variations in both the thrombotic (procoagulant) and anticoagulant aspects of the pathways (Table 1). From birth to 6 months, contact activators [factors XII, XI, high molecular weight kininogen (HMWK), prekallikrein (PK)], and the vitamin K-dependent factors are low. Factors V and XIII are also low at birth but increase significantly by day 5 (8, 9). Other procoagulant protein factors including factor VIII and VWF are elevated at birth compared to the adult population. Anticoagulant factors including TFPI, Anti-thrombin III, and protein C are low at birth and in preterm neonates. While TFPI remains low during most of childhood, Anti-thrombin III concentrations increase progressively, reaching adult concentration at 3 months of age. Protein C levels attain adult values at 6 months of age (8–10). The other inhibitors of coagulation are alpha-2-macroglobulin (Alpha 2 M) and C1 esterase inhibitor (C1-Inh), with a markedly inhibitory effect in the pediatric population (10, 11). The author suggested that they may play a major role in the maintenance of the balance between pro and anti-coagulant factors since they are elevated in the fetus and the newborn until 16 years of age and decrease later.
Classically, three main events in the coagulation process might influence outcomes in pediatric patients undergoing open heart with CPB: Thrombin generation, platelet activation, and fibrinolysis.
In a study by Chandlers (12) et al. in adults, using enzyme immunoassay, thrombin generation increased 20 folds 5 min after the start of CPB with additional rise after taking down the aortic cross-clamp and reperfusion of the heart and lung. There was an additional rise in thrombin and total fibrin generation after heparin reversal using protamine, while there was no change in thrombin generation after sternotomy or heparin administration. Thrombin and fibrin generation returned to baseline 2 h after surgery. Another adult study by Brister et al., demonstrated that the use of heparin did not prevent the rise of prothrombin fragments 1 and 2 and TAT despite having an activated clotting time >400 s (13). In that study, thrombin generation increased after neutralization of heparin using protamine sulfate and continued to be elevated for 24 h following the procedure. The authors concluded that high-dose heparin did not prevent thrombin generation during CPB and that thrombin bound to fibrin or CPB tubing is resistant to antithrombin III/heparin inhibition and thus causes thrombin generation. Therefore, these patients are at risk, not only of bleeding but also of thrombotic events (14).
During cardiac surgery, Thrombin is continuously generated as shown in different series leading to the thrombin burst (12–15). At the same time, there is a decrease of the Antithrombin level, particularly with the effect of hemodilution from the cardiopulmonary bypass circuit priming fluid (12). This fact is important to mention because the pediatric population has naturally a reduction in anticoagulant factors as mentioned previously. In addition, this could also be one of the reasons for carefuly increasing the procoagulant balance. When doing so, it is highly suggested to also consider AT repletion. To increase efficiently Antithrombin level during CPB, a substitution by either Antithrombin or FFP is needed. Additionally, CPB induces activation of the hemostatic system comprising the coagulation system, endothelium, regulatory protein, platelet, and fibrinolysis (16). Before initiating CPB, heparin is given to prevent blood clotting from the effect of CPB. Achievement of an adequate heparin level is assessed using Activating Clotting Time monitoring (ACT). There is an increased need for perioperative hemostasis monitoring in periods of increasing burst of thrombin generation such as early after starting CPB, after reperfusion of the heart and lungs, and after Protamine reversal (17).
Andreasen et al. assessed changes in thrombin generation in 29 children from 0.6 to 135 months after cardiac surgery and the ex-vivo response to blood products and hemostatic agents. The author found impaired hemostasis in patients after cardiac surgery (15). After analyzing thrombin generation in the patient's platelet-poor and platelet-rich plasma, they found a significantly prolonged lag time, a decreased peak thrombin generation, and decreased endogenous thrombin generation potential after CPB compared to baseline. The ex-vivo addition of platelet fibrinogen concentrate and rFVIIa restored the impaired hemostasis (15). In another study, 25 infants under 10 Kg undergoing cardiac surgery using CPB, Ashikhmina et al. demonstrated a decline in thrombin generation and antithrombin III (AT III) activity that did not recover completely after platelets and cryoprecipitate transfusion (18). In an ex-vivo study, Guzzetta et al. investigated the effect of rFVIIa and 3-factor PCC on thrombin generation in eleven neonates during cardiac surgery with CPB using a computer simulation model. 3-factor PCC successfully corrected the lag time, thrombin generation rate, and peak thrombin generation. 3F-PCC showed a potent procoagulant activity over rFVIIa (14). Despite the ex-vivo design of this study, the take-home point was that thrombin generation impairment was common during CPB, starting from CPB and continuing through the postoperative period. We discussed the use of Prothrombin Concentrate for management of coagulopathy in pediatric patients undergoing cardiac surgery with CPB in a previous publication (19).
Thrombin generation essays can demonstrate the balance existing between pro and anticoagulant factors. It gives an idea of the whole coagulation process. This can present advantages that plead for the usefulness of Thrombin essays for the assessment and correction of coagulation defects during CPB. They are effective for assessment and targeted intervention to correct coagulation disturbances. However, the feasibility and usefulness of these tools in the clinical setting present many challenges including accessibility, cost, and turnaround time. Future prospective and in vivo studies are needed to address specifically these questions. Thromboelastography (TEG) and Rotational thromboelastography (ROTEM) at this time present valuable alternatives since they can provide information on thrombin generation during cardiac surgery. The value of the ROTEM parameters; alpha angle and the maximum clot firmness correlated significantly with thrombin peak and endogenous thrombin potential (ETP) in a study assessing correlations of thrombin generation essays and ROTEM in adult patients (20). Furthermore, a significant correlation was found between CFT, alpha angle, and MCF in EXTEM, INTEM, and APTEM with platelets, INR, and fibrinogen and also between INTEM-CT and aPTT (21). In clinical, these ROTEM parameters could serve as surrogates to give an overview of thrombin generation for pediatric patients undergoing complex cardiac surgery.
Cyanotic children with congenital cardiac disease present more coagulation abnormalities compared to non-cyanotic ones. These coagulation disturbances might lead to thromboembolic and bleeding complications. A study aimed to evaluate Rotational thromboelastometry and whole impedance aggregometry in cyanotic congenital heart disease patients (CCHD) compared to a group without chronic cyanotic disease found that the first group presented relevant hypocoagulable disorders related to cyanosis duration. Blood analysis was done before heparin administration. While hemoglobin and hematocrit were higher in patients with CCHD, CT (Clotting time) and CFT (Clotting firmness time) in INTEM and EXTEM tests were reduced (22).
Patients with cyanotic congenital heart disease commonly present with changes in the coagulation profile including secondary erythroses, reduction in plasma volume, coagulation factors, platelet and fibrinogen deficiency, blood hyperviscosity, therefore increasing the risk of thrombosis and hemorrhagic events (23–25). Expert recommendations for perioperative PBM for these patients include RBC transfusion and coagulopathy management and, have been described previously (26, 27).
Blood hyperviscosity increases the risk of cardiovascular events, thrombosis, and stroke in patients with CCHD. Also, atrial fibrillation, arterial hypertension, and microcytosis are independent factors associated with stroke. During the perioperative period, special considerations are needed for pediatric patients with CCHD that include a thorough understanding of patient's medical history, physical examination, and relevant lab work. The identification of symptoms of hyperviscosity (dizziness, chest pain, abdominal pain, headache, etc.), coagulation profile, liver function with specific attention to blood collection are required to avoid false results (23). Phlebotomy should be considered only when significant hyperviscosity symptoms are present because of its potential to precipitate seizures, stroke, and other cardiovascular events (23). In the intraoperative period, phlebotomy is done either to relieve symptoms or for autologous blood donation with adequate replacement by plasma or albumin.
During massive hemorrhage, fibrinogen reaches critically low plasma levels sooner than other coagulation factors (28). In adults, a fibrinogen concentration of less than 220 mg/dl is associated with a significant risk of bleeding after cardiac surgery (29). Infants and neonates have low fibrinogen concentrations that reach adult values by one year of age. In addition, the fibrinogen network and clot formation that occurs with fetal fibrinogen in neonates and young infants is not the same as the one seen in adults. The clot formed in these younger pediatric patients is composed of highly aligned fibers instead of the highly branched network formed in adults, leading to a more porous and less effective clot (30). Furthermore, the interaction between adult and fetal forms of fibrinogen as well as neonatal clotting factors is not completely understood, which may explain why neonatal clot formation is not fully restored by fibrinogen transfusion. During Pediatric cardiac surgery, a good correlation was found between fibrinogen levels and the Fibtem essay using Rotational thromboelastometry (31, 32). Cryoprecipitate is a fractionated plasma product containing Fibrinogen (Factor I), Von Willebrand Factor, Factor VIII, and small amount of Factor XIII and Fibronectine.
Fibrinogen concentrate and Cryoprecipitate are used in pediatric patients undergoing cardiac surgery for fibrinogen compensation.
Early in hemostasis, platelets bind to the site of the damaged blood vessels and actively participate in the initiation and propagation of thrombus formation (33).
Platelets derive from megakaryocytes and circulate freely in the circulation in the normal vessel wall. After vascular injury, vasoconstriction, and liberation of inflammatory mediators, VWF is exposed and the platelet will quickly respond, undergoing activation and aggregation that will further lead to clot formation. Arriving at the injury site, the platelet will adhere to the endothelial lesion with the Glycoprotein Ib-IX-V-receptor, using Von Willebrand factor as a mediator. Activated platelet will change their shape into a pseudopod form upon the adhesion to the injured site and their surface will serve for carrying activated factors (6). As mentioned, this may explain the efficacy and safety of factor VIIa.
During CPB, qualitative and quantitative platelet disorders are linked to two main actions, preoperative platelet dysfunction and intraoperative platelet activation and destruction.
A prospective observational study of fifty-seven children undergoing cardiac surgery assessed platelet count and function using point-of-care testing and demonstrated a marked drop in platelet count postoperatively (34). Preoperative platelet dysfunction may predict blood product transfusion in children undergoing cardiac surgery and influence the expected coagulation disturbance found after CPB. Preoperative thrombocytopenia predicted longer closure time and the increased need for transfusion of PRBCs and FFP (35). Unfortunately, the cohort of patients included in that study had a limited number of patients with congenital heart disease (35). Cyanotic congenital heart disease alters the coagulation profile of patients, with platelet dysfunction being particularly prevalent in this subset of patients (23, 36, 37). Hypothermia and blood contact with the CPB circuit also target this activation (17). This may lead to the consumption of coagulation factors that is exaggerated in patients who undergo reoperations (38). Using ROTEM testing, parameters like HEPTEM maximum clot firmness (MCF) and Clot formation time (CFT) were highly predictive of intraoperative platelet dysfunction during CPB (39). Another study found an association between CPB time and postoperative bleeding, platelet dysfunction, and fibrinolysis. The authors suggested that these results could be since longer CPB time initiates plasmin generation through heparin, raises the Plasminogen activator inhibitor-tissue type plasminogen complex (PAI-1-tPA complex), and down-regulates platelet function (40).
In an in vitro study to measure the impact of hypothermia on platelet activation, analyses were performed on heparinized whole blood incubated at temperatures ranging from 17 to 37 degrees Celsius (41). The authors studied the effect of these temperatures on aggregation, P-Selectin expression, GP IIb/IIa activation, and PMP formation of unstimulated and ADP-stimulated platelets of 36 subjects using flow cytometry. In both unstimulated and ADP-stimulated samples, platelet aggregation started during moderate to deep hypothermia. The threshold was 25.2°C–25.8°C for unstimulated and ADP-stimulated samples, respectively. Hypothermia-induced P-selectin expression and GPII/IIIa activation were marked in deep hypothermia for both unstimulated and ADP-stimulated samples with different responses in mild hypothermia. The authors suggested that hypothermia-induced platelet activation may develop in most patients undergoing cardiac surgery (42). The consequence of this leads to excessive platelet activation, clot formation, and increasing thromboembolic complications.
Platelet dysfunction after CPB in the pediatric population is responsible for bleeding and the need for transfusion. The risk factors have been described in previous publications including hypothermia, hemodilution, the use of CPB, and perioperative use of antiplatelet agents. Classically, the diagnosis of platelet dysfunction is made with light aggregometry in the laboratory. Unfortunately, the use of this device in the operating setting is limited because of the longer turnaround time and the high amount of blood needed to perform the test. During surgery, Point-of-care devices such as multiple-electrode aggregometry (MEA) are more suitable. The latest generation of the ROTEM enables to combine analysis of ROTEM® with impedance aggregometry (ROTEM platelet®) (22) Classically, cyanotic patients will present with high platelet preoperatively but will suffer from high bleeding postoperatively due to the multifactorial origin of bleeding and hemostatic dysfunction in these patients as previously mentioned.
Hypothermia during pediatric cardiac surgery can occur for many reasons including vasodilatation of general anesthesia agents (Fentanyl, Propofol, opioids, etc.), and impairment of hypothalamic thermoregulation. While hypothermia in these patients is needed for brain ischemia protection and myocardial protection, it's also contribute to platelet dysfunction. Mild to modest hypothermia has been used in Pediatric cardiac surgery by different teams (32–34°C) (43); (24–25°C) (44). In recent studies, authors suggested benefits from normothermia in pediatric patients undergoing congenital cardiac surgery. This strategy will need to be well documented in large-scale studies (45).
The NATA guidelines for the management of platelet dysfunction in pediatric patient undergoing complex cardiac surgery with CPB at this time is based on consensus due to the few evidence available. They recommend platelet transfusion in the presence of excessive bleeding post-CPB despite adequate heparin reversal (26).
Cardiopulmonary bypass activates the fibrinolytic system (46–48). This is mediated by thrombin generation and continues in the postoperative period, decreasing progressively. Modified ultrafiltration (MUF) after separation from CPB may reduce fibrinolysis and significantly reduce blood loss and blood transfusion in adults after CPB (47). In a study to prospectively evaluate fibrinolysis in children during cardiac surgery using thromboelastography, 278 consecutive children undergoing cardiac surgery were evaluated (49). Evidence of fibrinolysis using TEG was found in 3% of patients pre-CPB, 16% during CPB, and 3% post-CPB. In another study, Ignjatovic et al. investigated fibrinolytic markers for plasmin generation and fibrinolysis in pediatric patients undergoing surgery with CPB (43). Fibrinogen level fluctuated throughout the perioperative period, with the greatest decreases found after initiation of CPB, post-protamine administration, and 6 h post-surgery. The Thrombin activatable fibrinolysis inhibitor (TAFI) level decreased by 40% after heparin administration, remained low throughout the surgery, and increased after protamine administration, but not more than the baseline level, up to six hours after the procedure.
In the same study, using fibrin monomer (FM), a more sensitive marker of fibrinolysis than D-dimer, they found an increased fibrin monomer (FM) level at the end of CPB, indicating increased fibrinolytic activity despite the action of TAFI on clot stabilization. The authors suggest that these mechanisms could explain the significant bleeding event observed in this population after CPB. This increased fibrinolysis increases blood product requirements during the postoperative period.
Thrombin can mediate increased fibrinolysis by activating the TAFI (50). The combined action of reduced thrombin generation and increased fibrinolysis in pediatric patients after CPB contributes to increased coagulopathy (51).
In an attempt to reduce fibrinolysis during CPB, antifibrinolytic agents were introduced. After the withdrawal of aprotinin from the market following the BART study in 2008 (Blood conservation using Antifibrinolytics in a Randomized trial) (52) clinicians tried other antifibrinolytic agents for pediatric cardiac surgery patients. Tranexamic acid (TXA) is an analog of the amino acid lysine competing with plasminogen for binding sites on fibrin and preventing plasmin-induced platelet activation. Lysine analogs like TXA and Epsilon aminocaproic acid (EACA) are the agents most used despite the lack of evidence regarding the optimal dosing and benefit in the pediatric population (53). TXA is by far more potent than EACA (54).
In adult cardiac surgery, TXA reduces the risk of bleeding without a higher risk of death or thrombotic complications within 30 days after surgery. In pediatric patients, Yu Zhang et al., in a retrospective cohort of 2026 consecutive patients who underwent surgical repair of the atrial septal defect, ventricular septal defect, or complete repair of tetralogy of Fallot, showed that TXA significantly reduced the mean difference of the total postoperative blood loss but not the allogenic transfusion requirement, although the correct dosage was not well defined (55). In a study comparing TXA and EACA, both drugs were similar in their effects on perioperative blood loss as well as in major clinical outcome criteria (56). In another study, TXA was found to reduce postoperative blood loss but not the allogeneic transfusion requirements in pediatric patients undergoing cardiac surgery, particularly in infants weighing less than 10 Kg and cyanotic lesions. In a retrospective review, Giordano et al. found a significant reduction of intraoperative blood requirement in the TXA group with a greater impact in the congenital cardiac heart surgery population compared with a previous series who did not receive TXA (57).
Seizure or “seizure-like” activity is a possible complication following the use of antifibrinolytic agents. In systematic reviews and meta-analyses, there were wide differences in dosage and incidence of seizures associated with the use of these agents, suggesting weak evidence to support the routine use of TXA and EACA in pediatric cardiac surgery patients (53, 58, 59).
Higher doses of TXA may be associated with seizures. Chauhan et al., compared different doses of TXA in 150 consecutive children with congenital heart disease (60). The author compared 5 dosing regimens, randomly assigning patients to each group. The dose of 10 mg/Kg at induction, 10 mg/Kg on CPB, and 10 mg/Kg after protamine administration yielded the best results. In another study including 231 patients, TXA was given as a bolus of 20 mg/Kg after anesthesia induction and 20 mg/Kg after protamine administration (57). In this study, TXA significantly reduced blood loss, the intra- and postoperative amount, and the number of RBC transfusions, and no concerns about safety were raised. In a large nationwide cohort, authors found an association with seizures but suggested that this could be avoided by prolonging the delivery of anesthetic during the early postop period (58). The study by Sharma suggested that seizures occurred in patients receiving a cumulative dose of TXA exceeding 80 mg/Kg (61). Other potential complications of TXA are thromboembolism and renal dysfunction.
A study on the pharmacokinetics of TXA in neonates, infants, and children undergoing cardiac surgery with CPB did not propose an effective dosage and protocol (62). Institutional protocols and experiences may provide a foundation for finding optimal dosage and eventually reducing side effects and maximizing the benefits of this therapy. NATA recommendations suggest for children less than 1 year old, a loading dose of 30 mg/Kg and a continuous infusion of 10 mg/Kg/h until the end of the surgery. For children of 1 y.o and more, 10 mg/Kg of loading dose and 10 mg/Kg/h until the end of the surgery (26).
The mechanisms of hemostasis and coagulation during pediatric cardiac surgery are complex. Their intricacies and the particularities related to the pediatric population must guide the clinical reasoning following CPB. While taking care of pediatric cardiac surgery patients these three phases (Thrombin generation, Platelet activation, Fibrinolysis) should be closely monitored to anticipate and manage a possible coagulation defect. This review presented the cell-based model of coagulation in bleeding-related conditions during pediatric cardiac surgery under CPB. Platelet activation taking place early in this process will allow the other factors to go through all the phases of the coagulation process, platelet aggregation, amplification, the formation of the thrombin spark, and later formation of the cascade of reactions which will lead to the clot formation. We emphasized also the importance of well-managing fibrinolysis taking place after CPB and continuing throughout the postoperative course. We also provide an overview of strategies and recommendations to manage these disorders. This information will help guide perioperative coagulation management strategies and reasoning in pediatric patients undergoing cardiac surgery under CPB as well as study interventions to implement for improving outcomes for this patient group.
DM: Conceptualization, Data curation, Formal Analysis, Funding acquisition, Investigation, Methodology, Project administration, Resources, Software, Supervision, Validation, Visualization, Writing – original draft, Writing – review & editing.
The author(s) declare that no financial support was received for the research, authorship, and/or publication of this article.
The authors declare that the research was conducted in the absence of any commercial or financial relationships that could be construed as a potential conflict of interest.
All claims expressed in this article are solely those of the authors and do not necessarily represent those of their affiliated organizations, or those of the publisher, the editors and the reviewers. Any product that may be evaluated in this article, or claim that may be made by its manufacturer, is not guaranteed or endorsed by the publisher.
1. Guzzetta NA, Allen NN, Wilson EC, Foster GS, Ehrlich AC, Miller BE. Excessive postoperative bleeding and outcomes in neonates undergoing cardiopulmonary bypass. Anesth Analg. (2015) 120(2):405–10. doi: 10.1213/ANE.0000000000000531
2. Williams GD, Bratton SL, Ramamoorthy C. Factors associated with blood loss and blood product transfusions: a multivariate analysis in children after open-heart surgery. Anesth Analg. (1999) 89(1):57–64. doi: 10.1097/00000539-199907000-00011
3. Miller BE, Mochizuki T, Levy JH, Bailey JM, Tosone SR, Tam VK, et al. Predicting and treating coagulopathies after cardiopulmonary bypass in children. Anesth Analg. (1997) 85(6):1196–202. doi: 10.1213/00000539-199712000-00003
4. Mazine A, Rached-D’Astous S, Ducruet T, Lacroix J, Poirier N, Injury PAL, et al. Blood transfusions after pediatric cardiac operations: a north American multicenter prospective study. Ann Thorac Surg. (2015) 100(2):671–7. doi: 10.1016/j.athoracsur.2015.04.033
5. Macfarlane RG. An enzyme cascade in the blood clotting mechanism, and its function as a biochemical amplifier. Nature. (1964) 202:498–9. doi: 10.1038/202498a0
6. Hoffman M, Monroe DM 3rd. A cell-based model of hemostasis. Thromb Haemost. (2001) 85(6):958–65. doi: 10.1055/s-0037-1615947
7. Hoffman M, Monroe DM 3rd, Roberts HR. Activated factor VII activates factors IX and X on the surface of activated platelets: thoughts on the mechanism of action of high-dose activated factor VII. Blood Coagul Fibrinolysis. (1998) 9(Suppl 1):S61–5.9819030
8. Andrew M, Vegh P, Johnston M, Bowker J, Ofosu F, Mitchell L. Maturation of the hemostatic system during childhood. Blood. (1992) 80(8):1998–2005. doi: 10.1182/blood.V80.8.1998.1998
9. Guzzetta NA, Miller BE. Principles of hemostasis in children: models and maturation. Paediatr Anaesth. (2011) 21(1):3–9. doi: 10.1111/j.1460-9592.2010.03410.x
10. Andrew M, Paes B, Milner R, Johnston M, Mitchell L, Tollefsen DM, et al. Development of the human coagulation system in the full-term infant. Blood. (1987) 70(1):165–72. doi: 10.1182/blood.V70.1.165.165
11. Ling X, Delorme M, Berry L, Ofosu F, Mitchell L, Paes B, et al. alpha 2-macroglobulin remains as important as antithrombin III for thrombin regulation in cord plasma in the presence of endothelial cell surfaces. Pediatr Res. (1995) 37(3):373–8. doi: 10.1203/00006450-199503000-00020
12. Chandler WL, Velan T. Estimating the rate of thrombin and fibrin generation in vivo during cardiopulmonary bypass. Blood. (2003) 101(11):4355–62. doi: 10.1182/blood-2002-08-2400
13. Brister SJ, Ofosu FA, Buchanan MR. Thrombin generation during cardiac surgery: is heparin the ideal anticoagulant? Thromb Haemost. (1993) 70(2):259–62. doi: 10.1055/s-0038-1649561
14. Guzzetta NA, Szlam F, Kiser AS, Fernandez JD, Szlam AD, Leong T, et al. Augmentation of thrombin generation in neonates undergoing cardiopulmonary bypass. Br J Anaesth. (2014) 112(2):319–27. doi: 10.1093/bja/aet355
15. Andreasen JB, Ravn HB, Hvas AM. Changes in thrombin generation in children after cardiac surgery and ex-vivo response to blood products and haemostatic agents. Blood Coagul Fibrinolysis. (2016) 27(1):24–30. doi: 10.1097/MBC.0000000000000379
16. Koster A, Fischer T, Praus M, Haberzettl H, Kuebler WM, Hetzer R, et al. Hemostatic activation and inflammatory response during cardiopulmonary bypass: impact of heparin management. Anesthesiology. (2002) 97(4):837–41. doi: 10.1097/00000542-200210000-00014
17. Sniecinski RM, Chandler WL. Activation of the hemostatic system during cardiopulmonary bypass. Anesth Analg. (2011) 113(6):1319–33. doi: 10.1213/ANE.0b013e3182354b7e
18. Ashikhmina E, Johnson PM, Aganga DO, Nuttall GA, Lahr BD, Schaff HV, et al. Thrombin generation and antithrombin activity in infants undergoing cardiopulmonary bypass-an exploratory study. J Cardiothorac Vasc Anesth. (2020) 34(8):2083–90. doi: 10.1053/j.jvca.2019.12.008
19. Munlemvo DM, Tobias JD, Chenault KM, Naguib A. Prothrombin complex concentrates to treat coagulation disturbances: an overview with a focus on use in infants and children. Cardiol Res. (2022) 13(1):18. doi: 10.14740/cr1342
20. Schneider T, Siegemund T, Siegemund R, Petros S. Thrombin generation and rotational thromboelastometry in the healthy adult population. Hämostaseologie. (2015) 35(02):181–6. doi: 10.5482/HAMO-14-08-0033
21. Theusinger OM, Schröder CM, Eismon J, Emmert MY, Seifert B, Spahn DR, et al. The influence of laboratory coagulation tests and clotting factor levels on rotation thromboelastometry (ROTEM®) during major surgery with hemorrhage. Anesth Analg. (2013) 117(2):314–21. doi: 10.1213/ANE.0b013e31829569ac
22. Perez M-H, Longchamp D, Amiet V, Natterer J, Ferry T, Boegli Y, et al. Point-of-care HEMOstasis in children with congenital heart disease, the POCHEMO study: rotational thromboelastometry and impedance aggregometry in children with cyanotic and non-cyanotic congenital heart disease. Int J Cardiol Congenit Heart Dis. (2022) 8:100383. doi: 10.1016/j.ijcchd.2022.100383
23. Zabala LM, Guzzetta NA. Cyanotic congenital heart disease (CCHD): focus on hypoxemia, secondary erythrocytosis, and coagulation alterations. Pediatric Anesthesia. (2015) 25(10):981–9. doi: 10.1111/pan.12705
24. Cordina RL, Celermajer DS. Chronic cyanosis and vascular function: implications for patients with cyanotic congenital heart disease. Cardiol Young. (2010) 20(3):242–53. doi: 10.1017/S1047951110000466
25. Jensen A, Johansson P, Idorn L, Sørensen K, Thilén U, Nagy E, et al. The haematocrit–an important factor causing impaired haemostasis in patients with cyanotic congenital heart disease. Int J Cardiol. (2013) 167(4):1317–21. doi: 10.1016/j.ijcard.2012.03.181
26. Faraoni D, Meier J, New HV, Van der Linden PJ, Hunt BJ. Patient blood management for neonates and children undergoing cardiac surgery: 2019 NATA guidelines. J Cardiothorac Vasc Anesth. (2019 Dec 1) 33(12):3249–63. doi: 10.1053/j.jvca.2019.03.036
27. Cholette JM, Willems A, Valentine SL, Bateman ST, Schwartz SM. Recommendations on RBC transfusion in infants and children with acquired and congenital heart disease from the pediatric critical care transfusion and anemia expertise initiative. Pediatr Crit Care Med. (2018) 19(9S):S137–48. doi: 10.1097/PCC.0000000000001603
28. Grottke O, Mallaiah S, Karkouti K, Saner F, Haas T. Fibrinogen supplementation and its indications. Semin Thromb Hemost. (2020) 46(01):038–49. doi: 10.1055/s-0039-1696946
29. Ranucci M, Pistuddi V, Baryshnikova E, Colella D, Bianchi P. Fibrinogen levels after cardiac surgical procedures: association with postoperative bleeding, trigger values, and target values. Ann Thorac Surg. (2016) 102(1):78–85. doi: 10.1016/j.athoracsur.2016.01.005
30. Guzzetta NA, Williams GD. Current use of factor concentrates in pediatric cardiac anesthesia. Paediatr Anaesth. (2017) 27(7):678–87. doi: 10.1111/pan.13158
31. Haas T, Spielmann N, Mauch J, Madjdpour C, Speer O, Schmugge M, et al. Comparison of thromboelastometry (ROTEM®) with standard plasmatic coagulation testing in paediatric surgery. Br J Anaesth. (2012) 108(1):36–41. doi: 10.1093/bja/aer342
32. Oswald E, Stalzer B, Heitz E, Weiss M, Schmugge M, Strasak A, et al. Thromboelastometry (ROTEM®) in children: age-related reference ranges and correlations with standard coagulation tests. Br J Anaesth. (2010) 105(6):827–35. doi: 10.1093/bja/aeq258
33. Monroe DM, Hoffman M, Roberts HR. Platelets and thrombin generation. Arterioscler Thromb Vasc Biol. (2002) 22(9):1381–9. doi: 10.1161/01.ATV.0000031340.68494.34
34. Jakob SM, Ruokonen E, Grounds RM, Sarapohja T, Garratt C, Pocock SJ, et al. Dexmedetomidine vs midazolam or propofol for sedation during prolonged mechanical ventilation: two randomized controlled trials. JAMA. (2012) 307(11):1151–60. doi: 10.1001/jama.2012.304
35. Zubair MM, Bailly DK, Lantz G, Sunstrom RE, Saharan S, Boshkov LK, et al. Preoperative platelet dysfunction predicts blood product transfusion in children undergoing cardiac surgery. Interact Cardiovasc Thorac Surg. (2015) 20(1):24–30. doi: 10.1093/icvts/ivu315
36. Maurer HM, McCue CM, Caul J, Still WJS. Impairment in platelet aggregation in congenital heart disease. Blood. (1972) 40(2):207–16. doi: 10.1182/blood.V40.2.207.207
37. Tempe DK, Virmani S. Coagulation abnormalities in patients with cyanotic congenital heart disease. J Cardiothorac Vasc Anesth. (2002) 16(6):752–65. doi: 10.1053/jcan.2002.128436
38. Hall TS, Sines JC, Spotnitz AJ. Hemorrhage related reexploration following open heart surgery: the impact of pre-operative and post-operative coagulation testing. Cardiovasc Surg. (2002) 10(2):146–53. doi: 10.1177/096721090201000210
39. Romlin BS, Söderlund F, Wåhlander H, Hallhagen S, Wessman C, Baghaei F, et al. Perioperative monitoring of platelet function in paediatric cardiac surgery by thromboelastometry, or platelet aggregometry? Br J Anaesth. (2016) 116(6):822–8. doi: 10.1093/bja/aew053
40. Varghese SJ, Unni MK, Mukundan N, Rai R. Platelet functions in cardiopulmonary bypass surgery. Med J Armed Forces India. (2005) 61(4):316–21. doi: 10.1016/S0377-1237(05)80053-X
41. Nieuwland R, Berckmans RJ, Rotteveel-Eijkman RC, Maquelin KN, Roozendaal KJ, Jansen PG, et al. Cell-derived microparticles generated in patients during cardiopulmonary bypass are highly procoagulant. Circulation. (1997) 96(10):3534–41. doi: 10.1161/01.CIR.96.10.3534
42. Straub A, Breuer M, Wendel HP, Peter K, Dietz K, Ziemer G. Critical temperature ranges of hypothermia-induced platelet activation: possible implications for cooling patients in cardiac surgery. Thromb Haemost. (2007) 97(4):608–16. doi: 10.1160/TH06-10-0563
43. Otto KA. Therapeutic hypothermia applicable to cardiac surgery. Vet Anaesth Analg. (2015) 42(6):559–69. doi: 10.1111/vaa.12299
44. Naguib AN, Carrillo SA, Corridore M, Bigelow AM, Walczak A, Tram NK, et al. A ROTEM-guided algorithm aimed to reduce blood product utilization during neonatal and infant cardiac surgery. The Journal of ExtraCorporeal Technology. (2023) 55(2):60–9. doi: 10.1051/ject/2023017
45. Shamsuddin AM, Nikman AM, Ali S, Zain MR, Wong AR, Corno AF. Normothermia for pediatric and congenital heart surgery: an expanded horizon. Front Pediatr. (2015) 3:23. doi: 10.3389/fped.2015.00023
46. Hunt BJ, Parratt RN, Segal HC, Sheikh S, Kallis P, Yacoub M. Activation of coagulation and fibrinolysis during cardiothoracic operations. Ann Thorac Surg. (1998) 65(3):712–8. doi: 10.1016/S0003-4975(97)01345-3
47. Leyh RG, Bartels C, Joubert-Hübner E, Bechtel JFM, Sievers HH. Influence of modified ultrafiltration on coagulation, fibrinolysis and blood loss in adult cardiac surgery. Eur J Cardiothorac Surg. (2001) 19(2):145–51. doi: 10.1016/S1010-7940(00)00633-3
48. Ignjatovic V, Chandramouli A, Than J, Summerhayes R, Newall F, Horton S, et al. Plasmin generation and fibrinolysis in pediatric patients undergoing cardiopulmonary bypass surgery. Pediatr Cardiol. (2012) 33(2):280–5. doi: 10.1007/s00246-011-0122-6
49. Williams GD, Bratton SL, Nielsen NJ, Ramamoorthy C. Fibrinolysis in pediatric patients undergoing cardiopulmonary bypass. J Cardiothorac Vasc Anesth. (1998) 12(6):633–8. doi: 10.1016/S1053-0770(98)90233-6
50. Bouma BN, Mosnier LO. Thrombin activatable fibrinolysis inhibitor (TAFI)–how does thrombin regulate fibrinolysis? Ann Med. (2006) 38(6):378–88. doi: 10.1080/07853890600852898
51. Ignjatovic V, Than J, Summerhayes R, Newall F, Horton S, Cochrane A, et al. Hemostatic response in paediatric patients undergoing cardiopulmonary bypass surgery. Pediatr Cardiol. (2011) 32(5):621–7. doi: 10.1007/s00246-011-9929-4
52. Fergusson DA, Hébert PC, Mazer CD, Fremes S, MacAdams C, Murkin JM, et al. A comparison of aprotinin and lysine analogues in high-risk cardiac surgery. N Engl J Med. (2008) 358(22):2319–31. doi: 10.1056/NEJMoa0802395
53. Faraoni D, Willems A, Melot C, De Hert S, Van der Linden P. Efficacy of tranexamic acid in paediatric cardiac surgery: a systematic review and meta-analysis. Eur J Cardiothorac Surg. (2012) 42(5):781–6. doi: 10.1093/ejcts/ezs127
54. Makhija N, Sarupria A, Kumar Choudhary S, Das S, Lakshmy R, Kiran U. Comparison of epsilon aminocaproic acid and tranexamic acid in thoracic aortic surgery: clinical efficacy and safety. J Cardiothorac Vasc Anesth. (2013) 27(6):1201–7. doi: 10.1053/j.jvca.2013.04.003
55. Zhang Y, Zhang X, Wang Y, Shi J, Yuan S, Duan F, et al. Efficacy and safety of tranexamic acid in pediatric patients undergoing cardiac surgery: a single-center experience. Front Pediatr. (2019) 7:181. doi: 10.3389/fped.2019.00181
56. Martin K, Breuer T, Gertler R, Hapfelmeier A, Schreiber C, Lange R, et al. Tranexamic acid versus ɛ-aminocaproic acid: efficacy and safety in paediatric cardiac surgery. Eur J Cardiothorac Surg. (2011) 39(6):892–7. doi: 10.1016/j.ejcts.2010.09.041
57. Giordano R, Palma G, Poli V, Palumbo S, Russolillo V, Cioffi S, et al. Tranexamic acid therapy in pediatric cardiac surgery: a single-center study. Ann Thorac Surg. (2012) 94(4):1302–6. doi: 10.1016/j.athoracsur.2012.04.078
58. Maeda T, Sasabuchi Y, Matsui H, Ohnishi Y, Miyata S, Yasunaga H. Safety of tranexamic acid in pediatric cardiac surgery: a nationwide database study. J Cardiothorac Vasc Anesth. (2017) 31(2):549–53. doi: 10.1053/j.jvca.2016.10.001
59. Nishijima DK, Monuteaux MC, Faraoni D, Goobie SM, Lee L, Galante J, et al. Tranexamic acid use in United States children’s hospitals. J Emerg Med. (2016) 50(6):868–74.e1. doi: 10.1016/j.jemermed.2016.02.004
60. Chauhan S, Bisoi A, Kumar N, Mittal D, Kale S, Kiran U, et al. Dose comparison of tranexamic acid in pediatric cardiac surgery. Asian Cardiovasc Thorac Ann. (2004) 12(2):121–4. doi: 10.1177/021849230401200208
61. Sharma V, Katznelson R, Jerath A, Garrido-Olivares L, Carroll J, Rao V, et al. The association between tranexamic acid and convulsive seizures after cardiac surgery: a multivariate analysis in 11 529 patients. Anaesthesia. (2014) 69(2):124–30. doi: 10.1111/anae.12516
Keywords: hemostasis, coagulation cascade, coagulopathy, pediatric cardiac anesthesiology, patient blood management
Citation: Munlemvo DM (2025) The cell-based approach of hemostasis, coagulopathy, and the implications for the pediatric cardiac anesthesiologist. Front. Anesthesiol. 4:1454904. doi: 10.3389/fanes.2025.1454904
Received: 25 June 2024; Accepted: 10 January 2025;
Published: 20 February 2025.
Edited by:
Dominik P. Guensch, Insel Gruppe AG, SwitzerlandReviewed by:
Gabor Erdoes, University Hospital of Bern, SwitzerlandCopyright: © 2025 Munlemvo. This is an open-access article distributed under the terms of the Creative Commons Attribution License (CC BY). The use, distribution or reproduction in other forums is permitted, provided the original author(s) and the copyright owner(s) are credited and that the original publication in this journal is cited, in accordance with accepted academic practice. No use, distribution or reproduction is permitted which does not comply with these terms.
*Correspondence: Dolly M. Munlemvo, ZG9sbHkubXVubGVtdm9AZ21haWwuY29t; bXVubGVtdm8uMUBvc3UuZWR1
Disclaimer: All claims expressed in this article are solely those of the authors and do not necessarily represent those of their affiliated organizations, or those of the publisher, the editors and the reviewers. Any product that may be evaluated in this article or claim that may be made by its manufacturer is not guaranteed or endorsed by the publisher.
Research integrity at Frontiers
Learn more about the work of our research integrity team to safeguard the quality of each article we publish.