- 1Department of Anesthesia and Intensive Care, Akershus University Hospital, Lørenskog, Norway
- 2Institute of Clinical Medicine, Faculty of Medicine, University of Oslo, Oslo, Norway
- 3Health Services Research Unit, Akershus University Hospital, Lørenskog, Norway
- 4Department of Anesthesiology, Division of Emergencies and Critical Care, Oslo University Hospital, Oslo, Norway
Background: In robot-assisted laparoscopic prostatectomy surgery patients are tilted 30°–40° head-down. Knowledge of cerebral autoregulation and determinants of cerebral blood flow in this setting is limited, though postoperative cognitive impairment has been reported. This observational study describes the hemodynamic determinants of cerebral blood flow and dynamics of cerebral perfusion pressure during surgery in the Trendelenburg position and the correlations with postoperative cognition measures.
Materials and methods: We included patients scheduled for robot-assisted laparoscopic prostatectomy without known cerebrovascular disease. Cardiac index, mean arterial pressure, central venous pressure, optic nerve sheath diameter as a surrogate for intracranial pressure, ultrasound-measured internal carotid artery (ICA) blood flow and PaCO2 were recorded at six time points (awake, anesthetized, immediately after tilt, 1 h of tilt, just before end of tilt, and before emerging from anaesthesia). Comprehensive cognitive tests were performed before surgery and 10 and 180 days post-surgery. Data was evaluated using linear regression models.
Results: Forty-four males with a mean age of 67 years were included. Duration of anesthesia was 226 min [IQR 201,266] with 165 min [134,199] in head-down tilt. ICA flow decreased after induction of anesthesia (483 vs. 344 ml/min) and remained lowered before increasing at return to horizontal position (331 vs. 407 ml/min). Cerebral perfusion pressure decreased after 1 h tilt (from 73 to 62 mmHg) and remained lowered (66 mmHg) also after return to horizontal position. Optic nerve sheath diameter increased from mean 5.8 mm to 6.4 mm during the course of surgery. ICA flow correlated positively with cardiac index (β 0.367. 1 L/min/m2 increase corresponding to 92 ml/min increased ICA flow). PaCO2 had a positive effect on ICA flow (β 0.145. 1 kPa increase corresponding to 49 ml/min increased ICA flow), while mean arterial pressure had a negative effect (β −0.203. 10 mmHg increase corresponding to a 29 ml/min decline in ICA flow). We found no evidence of postoperative cognitive dysfunction.
Conclusion: ICA flow and cerebral perfusion pressure were significantly reduced during robot-assisted laparoscopic prostatectomy surgery. ICA flow positively correlated with cardiac index and PaCO2, but negatively with mean arterial pressure. Postoperative cognitive function was not impaired.
1 Introduction
Over the past decade, robot-assisted surgery has increasingly become common practice for numerous surgical procedures (1, 2). Robot-assisted laparoscopic prostatectomy offers lower risk of postoperative erectile dysfunction, incontinence and urethral stricture, and it allows for more rapid postoperative recovery (3). The procedure utilizes insufflation of the abdominal cavity with CO2 (pneumoperitoneum) and steep Trendelenburg position to improve surgical access to the prostate. This entails significant changes in systemic and cerebral hemodynamics as well as intrathoracic pressures and constitutes a challenge for anesthesia providers. Knowledge of the determinants of cerebral blood flow during this procedure is currently limited, and several studies have reported substantial postoperative cognitive deficits and visual field defects in the days immediately following surgery (4–6). However, any long-term effects of prolonged Trendelenburg positioning on cognitive function have yet to be determined.
While the Trendelenburg position provides surgical advantages, studies suggest that it induces increased intracranial pressure (6, 7) and concomitant reduced cerebral perfusion pressure (7–9) and possibly reduced cerebral blood flow. Deterioration of cerebral autoregulation intraoperatively may result in increased rates of postoperative cognitive dysfunction (10). In a recent study by Yu et al. cerebral blood flow was found to be reduced in the tilted phase during robot-assisted laparoscopic prostatectomy (11). However, monitoring cerebral blood flow changes peroperatively may be not clinically relevant if not evaluated together with patient-centered outcomes like long-term cognitive function. To optimize perioperative care of an increasingly elderly surgical population it is important to assess postoperative cognitive dysfunction and its possible connections to changes in intracranial pressure and cerebral blood flow during surgery.
Cerebral blood flow is regulated by a complex combination of physiological responses and feedback mechanisms (12). A prevalent belief is that these autoregulatory mechanisms will ensure constant cerebral blood flow if mean arterial pressure is between 60 and 150 mmHg with stable PaCO2 levels (13, 14). However, studies have shown that changes in cardiac output may elicit significant changes in cerebral blood flow despite a mean arterial pressure within the believed “autoregulatory range” (15–17). Previous studies have been contradictory on whether cerebral autoregulation is affected during surgery in the Trendelenburg position, with one study reporting progressive attenuation of cerebral autoregulation during prolonged steep Trendelenburg position (18), and another reporting no impairment (19).
This observational study aimed to investigate the changes in cerebral circulatory physiology during robot-assisted laparoscopic prostatectomy and describe the determinants of cerebral blood flow. Specifically, we wanted to compare the effects of cardiac output and mean arterial pressure on cerebral blood flow. Further, we employed a comprehensive state-of-the-art cognitive testing with a substantial follow-up time, to detect whether cognition was impaired following surgery.
2 Methods and materials
2.1 Study design and eligibility criteria
The study was designed as a prospective, non-randomized observational study. We invited all patients who were scheduled for robot-assisted laparoscopic prostatectomy surgery at Akershus University Hospital from June 10th to December 23rd, 2022. Patients who met the exclusion criteria listed in Supplementary Table S1 were not included.
After oral and written information, written consent was obtained before the first presurgical cognitive test. Our recruitment process and protocol were in accordance with the Declaration of Helsinki and approved by the Regional Ethics Committee (Oslo, Norway; ref. No.: 230764) and the Institutional Data Protection Officer (ref. No.: 2021_14).
2.2 Anesthesia procedure
The study was purely observational. Premedication, anesthesia, mechanical ventilation, and postoperative analgesia were given according to our department's standard regime for robot-assisted laparoscopic prostatectomy. Oral premedication was given approximately 60 min before surgery, consisting of paracetamol (2 g, 1.5 g if age ≥75 years or weight ≤60 kg), dexamethasone (16 mg, 12 mg if age ≥75 years or weight ≤60 kg), oxycodone, prolonged release formulation (10 mg, 5 mg if age ≥75 years or weight ≤60 kg). Dexamethasone was omitted in patients with diabetes mellitus unless they were deemed well-regulated with HbA1C ≤ 48 mmol/mol or had type II diabetes regulated by low-dose metformin (500 mg × 2–3/day) or managed by dietary restrictions alone.
Anesthesia was induced with fentanyl, 100–150 µg, remifentanil and propofol using target control anesthesia. Muscle relaxation was provided using rocuronium (0.6 mg/kg). Anesthesia was maintained with an infusion of propofol and remifentanil, targeting a Bispectral IndexTM (BISTM, Medtronic Inc, Dublin, Ireland) of 40–60. Bolus doses of rocuronium were used to maintain deep neuromuscular blockade throughout the procedure (TOF = 0 and PTC ≤4). Sugammadex was used as reversal agent if TOF < 90% at the end of surgery. Bolus doses of ephedrine 5–10 mg i.v. and phenylephrine 0.05–0.1 mg i.v. were given in the event of hypotension. In select cases, an infusion of norepinephrine was started. Parecoxib 40 mg i.v. was administered approximately 30 min before extubation unless contraindicated. Ondansetron 4 mg i.v. was administered to patients with a high risk of developing postoperative nausea and vomiting. Normovolemia was maintained with an infusion of isotonic Ringer's Acetate with a rate of 3 ml/kg.
2.3 Recordings
Baseline measurements and recordings were obtained before induction of anesthesia (T1). Further measurements and recordings were taken immediately after induction of anesthesia and mechanical ventilation (T2), after the introduction of pneumoperitoneum and tilting the patient in the Trendelenburg position (T3), after 60 min in Trendelenburg position (T4), just before the end of surgery (T5), and after the patient was tilted back to horizontal position, but still under general anesthesia and mechanical ventilation (T6) (Table 1).
Standard anesthesia monitoring for the procedure included an arterial catheter for continuous blood pressure measurements, continuous electrocardiogram, finger pulse oximetry and measurements of end-tidal CO2, oxygen concentrations, and mean airway pressure from the ventilator circuit (Zeus, Drägerwerk AG & Co. KGaA, Lübeck, Germany).
Results from arterial blood gas analyses collected for study purposes were concealed from the treating anesthesia team if values did not exceed predetermined safety thresholds (Supplementary Table S2).
Central venous pressure was measured through a short 1.1 mm diameter cannula placed in the left external jugular vein (20). Standard breath-to-breath spirometry from the ventilator circuit provided tidal volumes, ventilatory minute volumes (L/min), peak airway pressures, and mean airway pressures. The laparoscope (AirSeal® iFS, CONMED Corporation, Largo. USA) continuously measured and displayed intraabdominal pressure. The degree of tilt of the operating table was electronically controlled and displayed on the table panel.
Doppler ultrasound measurements were obtained using a high-frequency linear probe over the right internal carotid artery (ICA) (VenueTM, General Electric Company, Boston, USA). We obtained time-averaged mean frequency (TAMEAN) and luminal cross-sectional area in both systole and diastole. All ultrasound measurements were repeated 3 times at each measurement time point (T1-T6). As recordings were of maximum 4 s, the ventilator frequency was set to a minimum of 15 breaths per minute during the recording to ensure that the ultrasound measurements included a full ventilatory cycle. The ventilation frequency was returned to their prior setting as soon as ultrasound measurements were completed. Arterial line signals were analyzed using LiDCO RapidTM (Masimo Corporation), which provided a nominal cardiac output, pulse pressure variation, nominal stroke volume, and stroke volume variation.
Optic nerve sheath diameter (ONSD) was measured using ultrasound after induction of anesthesia (T2) and near the end of surgery (T5). We measured ONSD in the transverse and sagittal plane in both eyes, the median value was reported.
Data was collected and stored using an electronic database (REDCap®, Vanderbilt University, Nashville, USA).
2.4 Cognitive testing
Cognitive testing was performed using the Cambridge Neuropsychological Test Automated Battery (CANTAB®) (Cambridge Cognition Ltd) software, which tests different domains of cognitive function (attention, memory, visuo-spatial processing, language and executive functions) (21). Testing was performed at least one day before surgery and repeated approximately 10 and 180 days after surgery. Cognitive testing was performed in a controlled and quiet environment at our hospital or at a healthcare facility closer to the patient's residence. The principal investigator or dedicated research staff had oversight over the entire test routine to ensure proper execution and standardization of testing conditions. Both the principal investigator and the research staff had received training from a neuropsychiatrist.
A total of 11 cognitive variables from the testing were analyzed. We analyzed Motor Screening Task Mean Latency, Paired Associates Learning First Attempt Memory Score, Paired Associates Learning Total Errors Adjusted, Reaction Time Task Median Five-Choice Reaction Time, Rapid Visual Information Processing, Rapid Visual Information Processing Median Response Latency, Rapid Visual Information Processing Probability of False Alarm, Spatial Working Memory Between Errors and Spatial Working Memory Strategy.
Variables were chosen based on recommendations from the CANTAB Connect Research: Test Administration User Guide (22), recommendations from the CANTAB® team and review of previous studies using CANTAB® to test for postoperative cognitive dysfunction (21, 23).
2.5 Endpoints
Our primary endpoints were changes in ICA flow, cerebral perfusion pressure, ONSD and postoperative changes in the cognitive testing variables. Secondary endpoints were correlations between ICA flow and other hemodynamic variables.
2.6 Calculations
For time-averaged mean frequency and ICA cross-sectional area in systole and diastole, the median value of all three measurements at each time point was used. For ONSD, the median value of the ONSD for all four measurements was used.
ICA flow (ml/min) was calculated by multiplying the median time-averaged mean frequency (cm/sec) by the average cross-sectional area (cm2) of the internal carotid artery (median diastolic area · 0.67 + median systolic area · 0.33), multiplied by 60. Cerebral perfusion pressure was calculated by subtracting the central venous pressure from mean arterial pressure. Cardiac index was calculated as cardiac output/body surface area. Ideal body weight (kg) was calculated as patient height in cm – 100.
2.7 Statistical analysis
The study was by design a non-randomized prospective observational study. No formal sample size calculation was performed. Temporal changes in ICA flow, ONSD, cardiorespiratory variables and outcomes from cognitive testing were assessed using a linear mixed model analysis fit by REML with t-tests using Satterthwaite's method. Participant ID was set as the random effect to account for inter-subject correlation and measurement time was set as the fixed effect.
Independent variables potentially associated with changes in ICA flow in the timeframe T2–T6 were analyzed in a linear mixed model. They were chosen a priori and included age, body mass index, cardiac index, heart rate, stroke volume index, mean arterial pressure, central venous pressure, mean airway pressure, PaCO2, and tidal volume divided by ideal body weight. Partial pressure of arterial oxygen (PaO2) was not used in this analysis, as we deemed it heavily influenced by pre-oxygenation before induction and extubation. A forward stepwise regression procedure set to minimize BIC was run to select important predictors. These were subsequently included in a linear mixed model with participant ID as a random effect. The underlying relationships among the contributing variables were also integrated in a Structural Equation Model (SEM) and presented as a path diagram.
We performed a linear mixed model analysis for variables potentially associated with changes in cognitive outcomes, modeling the variation in each endpoint with time as a fixed effect, the first cognitive test as a reference point, and study ID as a random effect. Secondly, we performed a mixed model regression including the following fixed effects: Total tilt time, the integral of cerebral perfusion pressure between T3 and T5, the integral of ICA flow between T3 and T5, the time between T2 and T6 (corresponding to the duration of anesthesia), the difference in ONSD between T2 and T5, and the CANTAB testing time point.
The assumptions of normal distribution and equal variance were assessed using quantile-quantile plots and a frequency distributions test. Normally distributed data are reported as means with 95% confidence interval shown in round brackets (x-y), and otherwise as medians and quartiles shown in square brackets [x,y]. The analyses were considered exploratory in nature, and therefore claims of significance are made cautiously.
Statistical analyses were performed using dedicated statistical software (R Foundation for statistical Computing, Vienna, Austria), using the “lmer” package for linear mixed models and “lavaan” for Structural Equation Modeling. Preparation of the data, stepwise regression procedure, analysis on means, medians, distributions and preparation of figures were done using JMP (JMP Pro17 ®, JMP Statistical Discovery, Buckinghamshire, UK).
3 Results
3.1 Participants
Eighty-four males were screened for eligibility, of which 44 were enrolled in the study. Of the 40 patients who were screened but not enrolled, 17 were excluded due to logistical reasons, and nine were excluded as they had a non-Scandinavian language as their native language. Nine did not consent to participate or failed to respond to contact from the principal investigator. Five were excluded due to known cerebrovascular or carotid disease. No patient was excluded because of findings of carotid stenosis after enrollment.
Of the 44 included patients, we performed intraoperative recordings on 37, the remaining seven undergoing cognitive testing only. Of the patients undergoing cognitive testing only, five were excluded from intraoperative measuring due to logistical reasons. The remaining two patients were excluded due to intraoperative complications. One due to hypotension and bradycardia, the other due to subcutaneous emphysema affecting the neck. Of the 37 patients who had intraoperative measurements, 32 also completed cognitive tests 1 and 2, and 25 patients completed all three tests. Reasons for incomplete follow-up were logistical reasons (n = 4), failure to respond to contact (n = 3), or illness/surgical complications hindering cognitive testing (n = 5).
3.2 Patient characteristics
The mean age of the included patients was 67 years (65–69), mean BMI was 27 kg/m2 (26–28) and median ASA physical status was 2 [2,2]. The most prevalent co-morbidities were hypertension (43%), coronary artery disease (16%), and diabetes mellitus (7%). Sixty-one percent had other co-morbidities. Supplementary Table S3 provides additional patient characteristics.
3.3 Outcome data
The total time with head-down tilt during surgery was 165 min [134,199], the duration of operation was 182 min [161,225], while general anesthesia lasted 226 min [201,266]. The total propofol and remifentanil doses were 4.9 mg/kg/h [4.5, 5.3] and 0.16 µg/kg/min [0.14, 0.18] respectively. Vasopressors were used in 78% of patients at T3, 16% at T5, and 73% at T6. Body temperatures were 36.2 C° [35.7, 36.6] and 36.5 C° [36.1, 36.9] at the start and end of the surgery respectively.
Descriptive statistics and results of a linear mixed model of variation of observed peroperative variables with time are presented in Tables 2, 3. There was a significant approximately 30% decline in ICA flow after induction of anesthesia and onset of mechanical ventilation. In the period from established head down tilt to end of surgery we observed no further changes in ICA flow, but there was a moderate increase in ICA flow when the patients were tilted back to horizontal position.
Cerebral perfusion pressure declined significantly following induction of anesthesia and did not change further immediately upon tilting. However, at all following timepoints cerebral perfusion pressure was lowered compared to immediately after tilting (Tables 2, 3). ONSD increased significantly over the course of the surgery.
We observed a significant decrease in ICA cross-sectional area from the awake state to after induction of anesthesia, with no significant changes in any of the subsequent time points. Similarly, blood velocity (time-averaged mean frequency) decreased 23% following induction of anesthesia, with no further changes apart from a trend towards recovery. Figure 1 provides an overview of changes in hemodynamic variables during surgery.
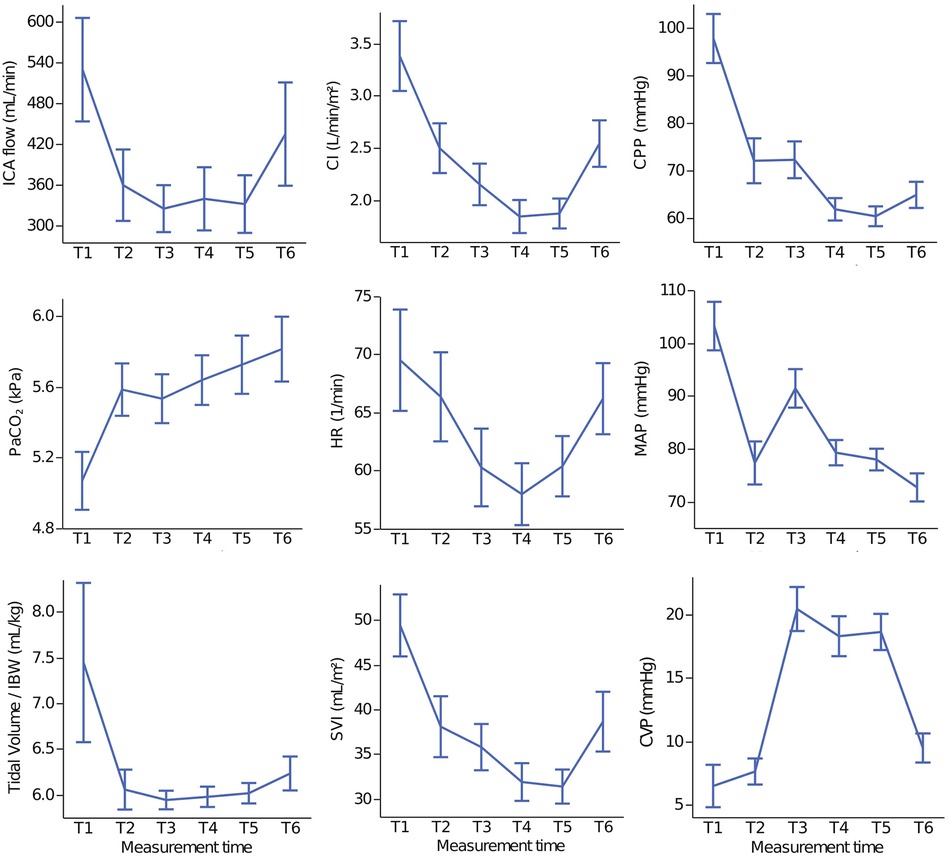
Figure 1. Overview of peroperative physiological changes. Peroperative variables at different stages of robot-assisted laparoscopic prostatectomy in 37 males. Values are means and 95% confidence intervals. T1: awake, T2: anesthetized horizontal position, T3: immediately after head-down tilt, T4: one hour of head-down tilt, T5: immediately before end of head-down tilt, T6: before emerging from anaesthesia, horizontal position. CI, cardiac index; CPP, cerebral perfusion pressure; CVP, central venous pressure; HR, heart rate; IBW, ideal body weight; ICA, internal carotid artery; MAP, mean arterial pressure PaCO2, partial pressure of arterial carbon dioxide; SVI, stroke volume index.
3.4 Correlations and associations
Cardiac index and mean arterial pressure were the predictors of ICA flow selected in the forward stepwise regression procedure set to minimize BIC. In a final multivariate linear mixed model, cardiac index was the strongest predictor of ICA flow. Its positive association had a log worth of 4.82 (p < 0.0001), more than twice the 2.30 log worth (p < 0.0005) of the negative association between mean arterial pressure and ICA flow.
We found that cardiac index had a moderate positive effect on ICA flow (β 0.367, p < 0.0001) with 1 L/min/m2 increased cardiac index corresponding to 92 ml/min increased ICA flow. PaCO2 had a small positive effect (β 0.145, p = 0.045), a 1 kPa increase in PaCO2 corresponding to 49 ml/min increased ICA flow, while mean arterial pressure had a small negative effect (β −0.203, p = 0.007), a 10 mmHg increase corresponding to a decline in ICA flow of 29 ml/min. In Figure 2, the predicted ICA flow response to changes in cardiac index and mean arterial pressure is illustrated for each individual and on a group level based on mixed-model multiple regression.
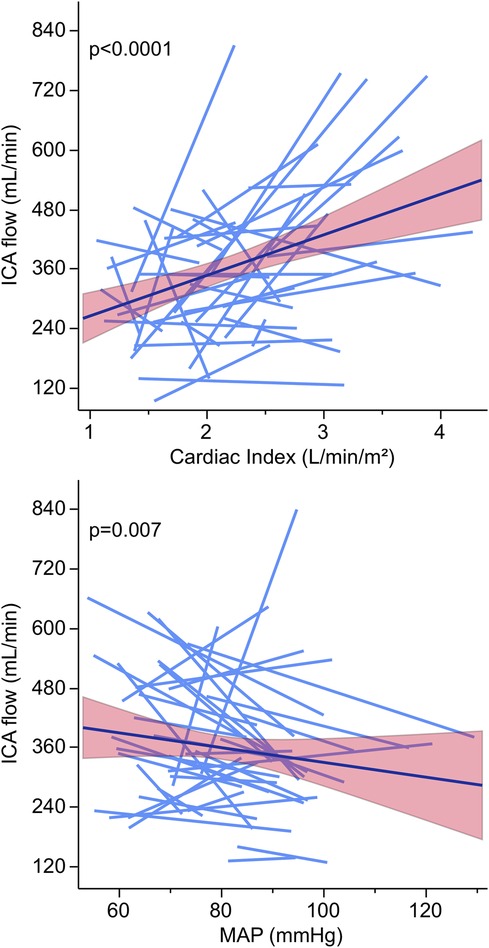
Figure 2. Associations between internal carotid artery flow blood flow, cardiac index and mean arterial pressure. Data from 37 males undergoing robot-assisted laparoscopic prostatectomy. Blue regression lines show individual patients’ change in ICA flow; black regression lines represents the overall group response with a 95% confidence fit for the slope. Upper panel: Relationship between ICA flow and cardiac index, 1 L/min/m2 increased cardiac index corresponding to 92 ml/min increased ICA flow. Lower panel: Relationship between ICA flow and mean arterial pressure, 10 mmHg increased mean arterial pressure corresponding to a decline in ICA flow of 29 ml/min. ICA, internal carotid artery; MAP, mean arterial pressure.
Structural Equation Modeling also found ICA flow to have a significant positive relationship with cardiac index and PaCO2, while having a significant negative relationship with mean arterial pressure (Figure 3). The general structural equation model had a moderate fit (Chi-Squared/df = 8.995, CFI = 0.882, RMSEA = 0.213), the analysis potentially being limited by heterogeneity due to unaccounted-for variation in time present in the data. The proportion of variance of ICA flow not explained by the effect of other variables was 0.83.
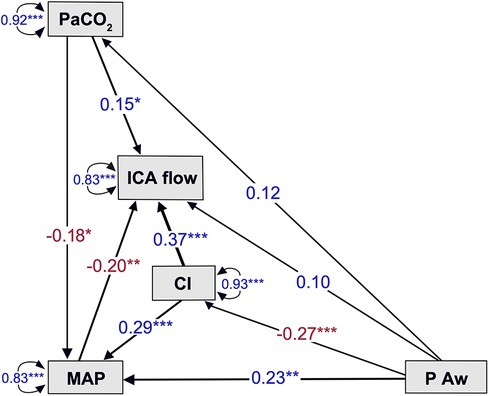
Figure 3. Determining factors of blood flow and their underlying relationships. Structural equation model of relationships between internal carotid artery flow, mean arterial pressure, cardiac index, airway pressure and partial pressure of CO2 in the arterial blood. Arrows indicate regression effects and directions of how one variable affect another. Red numbers indicate a negative relationship and blue numbers indicate a positive relationship between variables. Double-headed arrows represent variances in the unit of the variable not explained by relations to other variables. ***: p < 0.0001, **: p < 0.01, *: p < 0.05.
3.5 Cognition
Focus and outcomes of the employed cognitive tests are shown in Table 4. We observed improvements 10 days after surgery in Rapid Visual Information Processing (1.1%, p = 0.015) and Motor Screening Task Mean Latency 10.7%, p < 0.001), and at 180-days for Rapid Visual Information Processing (2.2%, p < 0.001), Motor Screening Task Mean Latency (10.8%, p = 0.001), Reaction Time Task Median Five-Choice Reaction Time (4.3%, p = 0.003) and Spatial Working Memory Between Errors (28%, p = 0.043).
Total time spent under anesthesia and total Trendelenburg time were respectively negative and positive predictors of Spatial Working Memory Strategy, but these results should be interpreted with a high degree of caution considering the large number of tests and exploratory nature of the analysis. Data are presented in Supplementary Table S4.
4 Discussion
The main findings in this observational study of patients undergoing robot-assisted laparoscopic prostatectomy surgery were a significant decline in ICA flow and cerebral perfusion pressure after induction of anesthesia. While ICA flow thereafter remained stable, a further reduction in cerebral perfusion pressure was found after one hour in Trendelenburg position, remaining so throughout the procedure. There was a significant increase in optic nerve sheath diameter (ONSD) in the head-down tilted position, indicating an increase in intracranial pressure. ICA flow showed a moderate positive association to cardiac index and to a smaller degree with PaCO2, while interestingly, a negative association was found between ICA flow and mean arterial pressure. There were slight improvements in some cognitive testing outcomes after 10 and 180 days, however, none of the variables observed during surgery predicted cognitive outcomes with certainty.
The observation of reduced cerebral flow after induction of anesthesia is well-established and can be explained by the reduced cerebral oxygen demand induced by propofol (24). Our finding of preserved ICA blood flow after tilting supports previous work on patients in Trendelenburg position, blood velocity in the median cerebral artery being preserved or increased after tilting (18). At first look our findings contradict the observation of reduced ICA flow after tilting reported by Yu et al. (11). However, in their study PaCO2 was actively held between 30 and 35 cmH2O, and a decrease in cardiac index was observed over time. In the present study ICA flow was positively correlated to cardiac index but also to PaCO2, which increased during surgery from 5.6 kPa (42 mmHg). Therefore the results by Yu et al. concur with the findings in the present study.
The increase in ONSD points toward a significant increase in intracranial pressure during the procedure (25). Our finding is in line with most previous robot-assisted laparoscopic prostatectomy studies (26–29). One study has reported unchanged ONSD after robot-assisted laparoscopic prostatectomy (30), however, they did not apply positive end-expiratory pressure during anesthesia. Positive end-expiratory pressure has been shown to increase intracranial pressure in animals during head-down tilting (7). Also, intrathoracic pressure will increase because of the abdominal content shift during head-down tilt, increasing central venous pressure with a concomitant increase in hydrostatic venous pressure on the brain, reducing venous return. This changes the relationship between hydrostatic and osmotic pressures in the brain. An increase in extracellular fluid will occur and over time institute cerebral edema.
In patients undergoing robot-assisted laparoscopic prostatectomy, intracranial pressure estimated by the zero flow pressure method, calculated from transcranial doppler velocity measurements, showed a close relationship between cerebral perfusion pressure calculated as mean arterial pressure minus central venous pressure and mean arterial pressure-estimated intracranial pressure by the Belfort formula (8), even after extended time spent in the Trendelenburg position. Reducing mean airway pressures either by reducing positive end-expiratory pressure or tidal volumes may reduce intracranial pressure in the head-down tilted patient. In our observational study, positive end-expiratory pressure during tilt was moderate (8 mBar) and tidal volumes were low (6 ml/kg Ideal body weight).
Baseline recordings of ONSD in the present study were higher than previously reported from normal populations (31). This is likely explained by the fact that male sex, advanced age and positive end-expiratory pressure, factors universal in our study population, all significantly increase ONSD (32). We observed that cerebral perfusion pressure after 1 h in Trendelenburg position was reduced, and remained lowered, from a median of 73 mmHg after induction to 61 mmHg right before the patient was tilted back to horizontal position. The decline in cerebral perfusion pressure was due to a decreasing mean arterial pressure, as central venous pressure was unchanged during tilt (Table 2). However, values varied markedly between individuals during tilting, central venous pressure ranging from 10 to 28 mmHg and cerebral perfusion pressure from 49 to 99 mmHg.
With the patient under general anesthesia, subjected to pneumoperitoneum and in the Trendelenburg position we found a significant positive association between cardiac index and cerebral blood flow, with a moderate effect size. Although this association has previously been described in both anesthetized and non-anesthetized individuals in the supine or anti-Trendelenburg position (16, 17) it is a novel finding for patients in the Trendelenburg position. This underscores the importance of optimizing patients’ cardiac output during surgery.
With a stable mean arterial pressure within the classically perceived “autoregulatory range” (13) in all patients, we found a negative association between mean arterial pressure and cerebral blood flow during general anesthesia, although with a small effect size. Skytioti et al. and Olesen et al. reported the opposite during minor and major gastrointestinal surgery, in reverse Trendelenburg and horizontal position, respectively (17, 33, 34). The major difference between those and the present study is the utilization of the extreme Trendelenburg position.
Previous studies have provided contradictory conclusions on cerebral autoregulation during robot-assisted laparoscopic prostatectomy, depending on methodology. Schram et al. observed disturbed cerebral autoregulation during robot-assisted laparoscopic prostatectomy when estimated by an autoregulation index using median cerebral artery blood velocity, i.e., not cerebral blood flow (18). In contrast, Beck et al. found no significant reduction in cerebral autoregulation during robot-assisted laparoscopic prostatectomy when estimated by a cerebral oxygenation index, calculated from a moving correlation coefficient between near-infrared spectroscopy measurements and mean arterial pressure (19). Results from the present study, using ICA flow to estimate cerebral blood flow and external jugular venous pressure to estimate central venous pressure, suggest an at least in part functioning cerebral autoregulation (preserved cerebral blood flow despite declining cerebral perfusion pressure, Figure 1), limiting the effects of hydrostatic pressure in the brain.
While a large variation in older adults’ ability to autoregulate cerebral blood flow during changes in blood pressure has been demonstrated (35), cerebral autoregulation has been found to be mostly intact during propofol and remifentanil anesthesia when maintaining normal PaCO2 (36). However, hypercapnia during anesthesia increases cerebral CO2 reactivity (33). We found ICA flow to be positively associated with PaCO2, an increase of 1 kPa CO2 corresponding to an increase in ICA flow of 49 ml/min. A decline in PaCO2 would however also reduce the hydrostatic pressure of the cerebral capillary system, potentially reducing the risk of cerebral oedema.
We correlated intraoperative cardiorespiratory measurements with data on long-term cognitive outcomes after robot-assisted laparoscopic prostatectomy, using a formalized test battery including tests of reaction time, visual memory, spatial working memory and ability of sustained attention - all necessary abilities in daily life. Mainly small changes in cognitive outcomes from preoperative baseline values were detected at postoperative day 10 and 180, and only the test for spatial working memory changed to a degree of clinical relevance. Evidence of a learning effect has been reported for this specific test (37, 38).
Previous studies showing postoperative cognitive dysfunction following robot-assisted laparoscopic prostatectomy performed their testing one to seven days post-surgery (4, 5, 39). Two of the studies reported a correlation between intraoperative pulsatility index and ONSD, surrogate markers for intracranial pressure, and incidence of postoperative cognitive dysfunction (4, 5). Our data suggests a complete return to baseline cognition before the 10-day mark and sustained cognitive abilities in patients tested at the 180-day mark, despite major changes in intracranial pressure, cerebral blood flow and cerebral perfusion pressure during robot-assisted laparoscopic prostatectomy surgery. We further found no consistent correlation between any of the cognitive outcomes and any data recorded during surgery.
4.1 Limitations
Although ICA has no extracranial branches, they only supply 80% of the cerebral blood flow, the remainder coming from the vertebral arteries. Our study utilized unilateral measurements of the ICA since the contralateral side was used for central venous pressure measurements. All analyses of ultrasound images, which require some manual evaluation, were done in a non-blinded fashion. Vasopressors were not administered by protocol but at the discretion of the attending doctor or nurse anesthetist.
The choice to exclude non-Scandinavian native language speakers due to the cognitive testing may limit external validity. Further, the first cognitive test was performed only days before surgery, when the cancer diagnosis and anticipation of surgery may have contributed negatively to the results. Selection bias may have played a role at the 180-day testing as we had a substantial loss to follow up. This observational study may have been underpowered for statistical analyses of cognitive test results.
5 Conclusion
This study demonstrated that ICA flow and cerebral perfusion pressure in patients undergoing robot-assisted radical prostatectomy decrease significantly after induction of anesthesia. No further decline in ICA flow was observed after application of pneumoperitoneum or head down tilt to 30 degrees, despite cerebral perfusion pressure continuing to decline. ICA flow was strongly positively predicted by changes in cardiac index and PaCO2, while the correlation between ICA flow and mean arterial pressure was slightly negative. To prevent reduced cerebral blood flow during surgery in the Trendelenburg position, monitoring of patients’ cardiac output seems more important than the traditional sole focus on mean arterial pressure. No apparent decline in cognitive test results were observed 10 and 180 days after surgery.
Data availability statement
Data were used under license for the current study and so are not publicly available, further inquiries can be directed to the corresponding author.
Ethics statement
The studies involving humans were approved by Regional Ethics Committee (Oslo, Norway; ref. No.: 230764) and the Institutional Data Protection Officer (ref. No.: 2021_14). The studies were conducted in accordance with the local legislation and institutional requirements. The participants provided their written informed consent to participate in this study.
Author contributions
FN: Writing – original draft, Writing – review & editing, Conceptualization, Data curation, Formal Analysis, Funding acquisition, Investigation, Methodology, Project administration, Validation. ST: Data curation, Investigation, Validation, Writing – review & editing. A-VI: Investigation, Methodology, Writing – review & editing. LØ: Investigation, Methodology, Validation, Writing – review & editing. OT: Data curation, Formal Analysis, Validation, Writing – review & editing. SS: Conceptualization, Formal Analysis, Funding acquisition, Methodology, Project administration, Software, Supervision, Visualization, Writing – review & editing. JH: Conceptualization, Funding acquisition, Methodology, Project administration, Resources, Supervision, Writing – original draft, Writing – review & editing.
Funding
The author(s) declare financial support was received for the research, authorship, and/or publication of this article. The project was funded by the South-Eastern Norway Regional Health Authority (Grant no. 2022088) and Akershus University Hospital, both public entities.
Acknowledgments
We thank senior consultant of urological surgery Gunder Magne Lilleaasen and nurse anesthetist Gitte Lise Olsen for assistance in planning the logistics of the study, and specialist nurse Mette Bach for her assistance in data collection and patient logistics. We greatly appreciate the included patients’ willingness to contribute to the study.
Conflict of interest
The authors declare that the research was conducted in the absence of any commercial or financial relationships that could be construed as a potential conflict of interest.
Publisher's note
All claims expressed in this article are solely those of the authors and do not necessarily represent those of their affiliated organizations, or those of the publisher, the editors and the reviewers. Any product that may be evaluated in this article, or claim that may be made by its manufacturer, is not guaranteed or endorsed by the publisher.
Supplementary material
The Supplementary Material for this article can be found online at: https://www.frontiersin.org/articles/10.3389/fanes.2024.1395973/full#supplementary-material
Abbreviations
CO2, Carbon dioxide; ICA, internal carotid artery; ONSD, optic nerve sheath diameter; PaCO2, partial pressure of arterial carbon dioxide; TAMEAN, time-averaged mean velocity inside; MOTML, Motor Screening Task Mean Latency; PALFAMS, Paired Associates Learning First Attempt Memory Score; PALTEA, Paired Associates Learning Total Errors Adjusted; RTIFMDRT, Reaction Time Task Median Five-Choice Reaction Time; RVPA, Rapid Visual Information Processing A; RVPMDL, Rapid Visual Information Processing Median Response Latency; RVPPFA, Rapid Visual Information Processing Probability of False Alarm; SWMBE, Spatial Working Memory Between Errors; SWMS, Spatial Working Memory Strategy.
References
1. Matanes E, Boulus S, Lowenstein L. The implementation of robotic surgery in Israel. Isr Med Assoc J. (2015) 17(9):563–6. PMID: 26625547.26625547
2. Sheetz KH, Claflin J, Dimick JB. Trends in the adoption of robotic surgery for common surgical procedures. JAMA Netw Open. (2020) 3(1):e1918911. doi: 10.1001/jamanetworkopen.2019.18911
3. Seo HJ, Lee NR, Son SK, Kim DK, Rha KH, Lee SH. Comparison of robot-assisted radical prostatectomy and open radical prostatectomy outcomes: a systematic review and meta-analysis. Yonsei Med J. (2016) 57(5):1165–77. doi: 10.3349/ymj.2016.57.5.1165
4. Aceto P, Russo A, Galletta C, Schipa C, Romanò B, Luca E, et al. Relationship between middle cerebral artery pulsatility index and delayed neurocognitive recovery in patients undergoing robot-assisted laparoscopic prostatectomy. J Clin Med. (2023) 12(3):1–10. doi: 10.3390/jcm12031070
5. Chen K, Wang L, Wang Q, Liu X, Lu Y, Li Y, et al. Effects of pneumoperitoneum and steep trendelenburg position on cerebral hemodynamics during robotic-assisted laparoscopic radical prostatectomy: a randomized controlled study. Medicine (Baltimore). (2019) 98(21):e15794. doi: 10.1097/MD.0000000000015794
6. Taketani Y, Mayama C, Suzuki N, Wada A, Oka T, Inamochi K, et al. Transient but significant visual field defects after robot-assisted laparoscopic radical prostatectomy in deep Trendelenburg position. PLoS One. (2015) 10(4):e0123361. doi: 10.1371/journal.pone.0123361
7. Bedirli N, Emmez G, Ünal Y, Tönge M, Emmez H. Effects of positive end-expiratory pressure on intracranial pressure during pneumoperitoneum and trendelenburg position in a porcine model. Turk J Med Sci. (2017) 47(5):1610–5. doi: 10.3906/sag-1609-17
8. Kalmar AF, Dewaele F, Foubert L, Hendrickx JF, Heeremans EH, Struys MM, et al. Cerebral haemodynamic physiology during steep trendelenburg position and CO(2) pneumoperitoneum. Br J Anaesth. (2012) 108(3):478–84. doi: 10.1093/bja/aer448
9. Kalmar AF, Foubert L, Hendrickx JF, Mottrie A, Absalom A, Mortier EP, et al. Influence of steep trendelenburg position and CO(2) pneumoperitoneum on cardiovascular, cerebrovascular, and respiratory homeostasis during robotic prostatectomy. Br J Anaesth. (2010) 104(4):433–9. doi: 10.1093/bja/aeq018
10. Fu H, Fan L, Wang T. Perioperative neurocognition in elderly patients. Curr Opin Anaesthesiol. (2018) 31(1):24–9. doi: 10.1097/ACO.0000000000000536
11. Yu J, Park JY, Hong JH, Hwang JH, Kim YK. Effect of pneumoperitoneum and trendelenburg position on internal carotid artery blood flow measured by ultrasound during robotic prostatectomy. Clin Physiol Funct Imaging. (2022) 42(2):139–45. doi: 10.1111/cpf.12742
12. Claassen J, Thijssen DHJ, Panerai RB, Faraci FM. Regulation of cerebral blood flow in humans: physiology and clinical implications of autoregulation. Physiol Rev. (2021) 101(4):1487–559. doi: 10.1152/physrev.00022.2020
13. Lassen NA. Cerebral blood flow and oxygen consumption in man. Physiol Rev. (1959) 39(2):183–238. doi: 10.1152/physrev.1959.39.2.183
14. Meng L, Gelb AW. Regulation of cerebral autoregulation by carbon dioxide. Anesthesiology. (2015) 122(1):196–205. doi: 10.1097/ALN.0000000000000506
15. Levine BD, Giller CA, Lane LD, Buckey JC, Blomqvist CG. Cerebral versus systemic hemodynamics during graded orthostatic stress in humans. Circulation. (1994) 90(1):298–306. doi: 10.1161/01.CIR.90.1.298
16. Skytioti M, Søvik S, Elstad M. Internal carotid artery blood flow in healthy awake subjects is reduced by simulated hypovolemia and noninvasive mechanical ventilation. Physiol Rep. (2016) 4(19):1–10. doi: 10.14814/phy2.12969
17. Skytioti M, Elstad M, Søvik S. Internal carotid artery blood flow response to anesthesia, pneumoperitoneum, and head-up tilt during laparoscopic cholecystectomy. Anesthesiology. (2019) 131(3):512–20. doi: 10.1097/ALN.0000000000002838
18. Schramm P, Treiber AH, Berres M, Pestel G, Engelhard K, Werner C, et al. Time course of cerebrovascular autoregulation during extreme trendelenburg position for robotic-assisted prostatic surgery. Anaesthesia. (2014) 69(1):58–63. doi: 10.1111/anae.12477
19. Beck S, Ragab H, Hoop D, Meßner-Schmitt A, Rademacher C, Kahl U, et al. Comparing the effect of positioning on cerebral autoregulation during radical prostatectomy: a prospective observational study. J Clin Monit Comput. (2021) 35(4):891–901. doi: 10.1007/s10877-020-00549-0
20. Parker JL, Flucker CJR, Harvey N, Maguire AM, Russell WC, Thompson JP. Comparison of external jugular and central venous pressures in mechanically ventilated patients. Anaesthesia. (2002) 57(6):584–605. doi: 10.1046/j.1365-2044.2002.02509_4.x
21. Haller G, Chan MTV, Combescure C, Lopez U, Pichon I, Licker M, et al. The international ENIGMA-II substudy on postoperative cognitive disorders (ISEP). Sci Rep. (2021) 11(1):1–12. doi: 10.1038/s41598-021-91014-8
23. Turowicz A, Czapiga A, Malinowski M, Dorobisz T, Czapla B, Janczak D. Lower extremity artery disease and cognitive impairment. Artery Res. (2022) 28(1):9–14. doi: 10.1007/s44200-021-00010-w
24. Schlünzen L, Juul N, Hansen KV, Cold GE. Regional cerebral blood flow and glucose metabolism during propofol anaesthesia in healthy subjects studied with positron emission tomography. Acta Anaesthesiol Scand. (2012) 56(2):248–55. doi: 10.1111/j.1399-6576.2011.02561.x
25. Chen LM, Wang LJ, Hu Y, Jiang XH, Wang YZ, Xing YQ. Ultrasonic measurement of optic nerve sheath diameter: a non-invasive surrogate approach for dynamic, real-time evaluation of intracranial pressure. Br J Ophthalmol. (2019) 103(4):437–41. doi: 10.1136/bjophthalmol-2018-312934
26. Kim MS, Bai SJ, Lee JR, Choi YD, Kim YJ, Choi SH. Increase in intracranial pressure during carbon dioxide pneumoperitoneum with steep trendelenburg positioning proven by ultrasonographic measurement of optic nerve sheath diameter. J Endourol. (2014) 28(7):801–6. doi: 10.1089/end.2014.0019
27. Balkan B, Emir NS, Demirayak B, Çetingök H, Bayrak B. The effect of robotic surgery on intraocular pressure and optic nerve sheath diameter: a prospective study. Braz J Anesthesiol. (2021) 71(6):607–11. doi: 10.1016/j.bjane.2021.02.035
28. Choi ES, Jeon YT, Sohn HM, Kim DW, Choi SJ, In CB. Comparison of the effects of desflurane and total intravenous anesthesia on the optic nerve sheath diameter in robot assisted laparoscopic radical prostatectomy: a randomized controlled trial. Medicine (Baltimore). (2018) 97(41):e12772. doi: 10.1097/MD.0000000000012772
29. Bang YJ, Jeong H, Heo BY, Shin BS, Sim WS, Kim DK, et al. Effects of increased optic nerve sheath diameter on inadequate emergence from anesthesia in patients undergoing robot-assisted laparoscopic prostatectomy: a prospective observational study. Diagnostics (Basel). (2021) 11(12):1–11. doi: 10.3390/diagnostics11122260
30. Verdonck P, Kalmar AF, Suy K, Geeraerts T, Vercauteren M, Mottrie A, et al. Optic nerve sheath diameter remains constant during robot assisted laparoscopic radical prostatectomy. PLoS One. (2014) 9(11):e111916. doi: 10.1371/journal.pone.0111916
31. Schroeder C, Katsanos AH, Richter D, Tsivgoulis G, Gold R, Krogias C. Quantification of optic nerve and sheath diameter by transorbital sonography: a systematic review and metanalysis. J Neuroimaging. (2020) 30(2):165–74. doi: 10.1111/jon.12691
32. Ertl M, Knüppel C, Veitweber M, Wagner A, Pfister K, Wendl C, et al. Normal age- and sex-related values of the optic nerve sheath diameter and its dependency on position and positive End-expiratory pressure. Ultrasound Med Biol. (2020) 46(12):3279–85. doi: 10.1016/j.ultrasmedbio.2020.08.025
33. Olesen ND, Egesborg AH, Frederiksen HJ, Kitchen CC, Svendsen LB, Olsen NV, et al. Influence of blood pressure on internal carotid artery blood flow during combined propofol-remifentanil and thoracic epidural anesthesia. J Anaesthesiol Clin Pharmacol. (2022) 38(4):580–7. doi: 10.4103/joacp.JOACP_575_20
34. Olesen ND, Frederiksen HJ, Storkholm JH, Hansen CP, Svendsen LB, Olsen NV, et al. Internal carotid artery blood flow is enhanced by elevating blood pressure during combined propofol-remifentanil and thoracic epidural anaesthesia: a randomised cross-over trial. Eur J Anaesthesiol. (2020) 37(6):482–90. doi: 10.1097/EJA.0000000000001189
35. Liu J, Tseng BY, Khan MA, Tarumi T, Hill C, Mirshams N, et al. Individual variability of cerebral autoregulation, posterior cerebral circulation and white matter hyperintensity. J Physiol. (2016) 594(11):3141–55. doi: 10.1113/JP271068
36. Dagal A, Lam AM. Cerebral autoregulation and anesthesia. Curr Opin Anaesthesiol. (2009) 22(5):547–52. doi: 10.1097/ACO.0b013e32833020be
37. Cacciamani F, Salvadori N, Eusebi P, Lisetti V, Luchetti E, Calabresi P, et al. Evidence of practice effect in CANTAB spatial working memory test in a cohort of patients with mild cognitive impairment. Appl Neuropsychol Adult. (2018) 25(3):237–48. doi: 10.1080/23279095.2017.1286346
38. Gonçalves MM, Pinho MS, Simões MR. Test-retest reliability analysis of the Cambridge neuropsychological automated tests for the assessment of dementia in older people living in retirement homes. Appl Neuropsychol Adult. (2016) 23(4):251–63. doi: 10.1080/23279095.2015.1053889
39. Beck S, Zins L, Holthusen C, Rademacher C, von Breunig F, Tennstedt P, et al. Comparison of cognitive function after robot-assisted prostatectomy and open retropubic radical prostatectomy: a prospective observational single-center study. Urology. (2020) 139:110–7. doi: 10.1016/j.urology.2019.12.045
Keywords: cerebrovascular circulation, cerebral autoregulation, robot-assisted laparoscopic surgery, Trendelenburg position, postoperative cognitive dysfunction
Citation: Nordum FH, Tveit SH, Idland A-V, Øyen LA, Thomas OMT, Søvik S and Hyldebrandt JA (2024) Predictors of cerebral blood flow during surgery in the Trendelenburg position, and their correlations to postoperative cognitive function. Front. Anesthesiol. 3:1395973. doi: 10.3389/fanes.2024.1395973
Received: 4 March 2024; Accepted: 14 August 2024;
Published: 29 August 2024.
Edited by:
Niels Damkjær Olesen, University of Copenhagen, DenmarkReviewed by:
Jing Ye, Guangzhou Medical University, ChinaAlbert Gjedde, University of Copenhagen, Denmark
Copyright: © 2024 Nordum, Tveit, Idland, Øyen, Thomas, Søvik and Hyldebrandt. This is an open-access article distributed under the terms of the Creative Commons Attribution License (CC BY). The use, distribution or reproduction in other forums is permitted, provided the original author(s) and the copyright owner(s) are credited and that the original publication in this journal is cited, in accordance with accepted academic practice. No use, distribution or reproduction is permitted which does not comply with these terms.
*Correspondence: Signe Søvik, signe.sovik@medisin.uio.no