- 1Department of Anesthesia, Rigshospitalet, University of Copenhagen, Copenhagen, Denmark
- 2Department of Surgical Gastroenterology, Rigshospitalet, University of Copenhagen, Copenhagen, Denmark
Purpose: During abdominal surgery, manipulation of internal organs may induce a “mesenteric traction syndrome” (MTS) including a triad of flushing, hypotension, and tachycardia that lasts for approximately 30 min. We evaluated whether MTS affects internal carotid artery blood flow.
Methods: This prospective cohort study included 27 patients aged 65 ± 11 years (mean ± SD) undergoing stomach resection (n = 12), esophageal resection (n = 14), or gastro-entero anastomosis (n = 1) during propofol-remifentanil and thoracic epidural anesthesia. Duplex ultrasound determined internal carotid artery blood flow, laser Doppler flowmetry assessed forehead skin blood flow, and near-infrared spectroscopy determined cerebral oxygenation. Development of MTS was defined by flushing within 60 min after incision.
Results: Seven patients developed MTS 22 [20–26; median (IQR)] min after incision and at that time the change in heart rate (to 87 ± 13 vs. 70 ± 11 bpm; P = 0.0007) and skin blood flow (to 214 (134–269) vs. 84 (59-112 PU; P = 0.0044) was higher in the patients who developed MTS as compared to those who did not, while mean arterial pressure (63 ± 13 vs. 64 ± 14 mmHg; P = 0.1433), cerebral oxygenation (69 ± 9% vs. 63 ± 10%; P = 0.2485), and internal carotid artery flow (225 ± 53 vs. 203 ± 69 ml/min; P = 0.9529) were similar.
Conclusion: Hemodynamic perturbations are observed in some patients in response to manipulation of the viscera, but the development of MTS appears not to influence internal carotid artery flow.
Clinical trial registration: https://clinicaltrials.gov/ct2/show/NCT02951273?term=NCT02951273&rank=1, identifier: NCT02951273.
1. Introduction
Traction of the viscera during abdominal surgery may provoke a so-called mesenteric traction syndrome (MTS) described as a triad of flushing, tachycardia, and hypotension [1–4]. MTS is part of the inflammatory response to surgery [5] and with marked flushing, MTS associates with postoperative complications [1]. The incidence of MTS is reported in approximately 50% of patients undergoing open upper gastrointestinal, pancreatic, and abdominal aortic surgery [1–4, 6] and develops about 15–20 min after incision and lasts for some 30 min [4, 6]. The syndrome is characterized by vasodilation with an increase in cardiac output [3, 4] but the effect of MTS on cerebral blood flow (CBF) is unclear.
Development of MTS is reported to elevate near-infrared-spectroscopy determined cerebral oxygenation ScO2 [4], which may relate to an increase in CBF or be due to an increase in forehead skin blood flow (SkBF) as changes in the extracranial circulation influence ScO2 [7]. Furthermore, MTS appears not to affect transcranial Doppler-determined middle cerebral artery blood velocity [4], but the evaluation is limited in response to vasodilation that may affect the diameter of the middle cerebral artery whereby any increase in blood flow is underestimated [8]. MTS likely relates to the release of prostaglandin I2 (PGI2) as MTS is absent following cyclooxygenase inhibition [2, 3, 9]. Infusion of PGI2 to healthy subjects provokes similar hemodynamic changes as MTS with no effect on CBF [10], although PGI2 dilates cerebral vessels in vitro [11, 12]. Furthermore, the effect of PGI2 on CBF could be influenced by anesthesia and other vasodilators potentially released during MTS. Thus, we designed the study to evaluate whether CBF is elevated in response to MTS or whether the increase in ScO2 is due to the increase in SkBF. An increase in CBF in response to MTS could be clinically important as it may elevate intracranial pressure and provoke cerebral edema [13–15].
This study evaluated whether the development of MTS in patients undergoing stomach or esophageal resection affects internal carotid artery (ICA) blood flow and we hypothesized that ICA flow, SkBF, and ScO2 would increase in response to MTS. Duplex ultrasound was used to evaluate ICA flow which correlates to an evaluation by phase contrast magnetic resonance imaging [16].
2. Materials and methods
2.1. Participants
A total of 30 consecutive patients planned for open stomach- or esophageal resection aged 18 years or older were included in this prospective cohort study (from December 2016 to July 2017) and provided written informed consent as approved by the ethical committee of Copenhagen (H-16036250, 18 October 2016) in accordance with the Declaration of Helsinki. The study was preregistered on ClinicalTrials.gov (NCT02951273; principal investigator: N.D.O.; 1 November 2016) and the manuscript adheres to the STROBE guideline. Results from this study concerning the effect of induction of anesthesia, phenylephrine treatment of anesthesia-induced hypotension, and cerebral CO2 reactivity is published elsewhere [17]. Exclusion criteria were intake of anti-inflammatory medication including non-steroidal anti-inflammatory drugs and corticosteroids, robot-assisted procedure, >15% ICA obstruction as evaluated by duplex ultrasound 1 to 3 days prior to surgery, and neurologic disease that may affect CBF including stroke, dementia, and epilepsy. Any use of acetylsalicylic acid was discontinued for 3 days prior to surgery and no anti-inflammatory medication was used during anesthesia.
2.2. Surgical setting
Surgery was after an overnight fast with patients allowed to drink “clear” fluids until 2 h before the scheduled time for the operation and no premedication was administered. Under the cover of local anesthesia, a radial artery and epidural catheter (T8–T10) was placed. Epidural anesthesia was by 15–30 mg bupivacaine, followed by infusion of bupivacaine with morphine (2.5 and 0.05 mg/ml; 5 ml/h) and an hourly bolus dose administration of 15 mg bupivacaine. Anesthesia was induced by propofol and maintained by propofol and remifentanil. Muscle relaxation was by cisatracurium or suxamethonium allowing for tracheal intubation and ventilation aimed at an end-tidal CO2 tension of 4.0–5.0 kPa (Dräger Primus, Drägerwerk, Lübeck, Germany). A central venous catheter was inserted in the internal jugular vein contralateral to the ICA insonation site. Blood volume was maintained by human albumin 5% and lactated Ringer's solution. A reduction in mean arterial pressure (MAP) to below 60 mmHg was treated by administration of a bolus dose of ephedrine or phenylephrine or infusion of noradrenaline on the choice of the anesthesiologist.
2.3. Definition of a mesenteric traction syndrome
Development of MTS was defined as flushing within 60 min after incision in patients who underwent manipulation of the abdominal viscera. Flushing was described as level “I” if around the eyes and on the cheeks and level “II” if it covered the entire face [1, 2, 4, 6].
2.4. Measurements
Radial artery catheterization allowed for the evaluation of blood gas variables (ABL 725, Radiometer, Copenhagen, Denmark) as well as MAP, heart rate (HR), stroke volume (SV), cardiac output (CO), and total peripheral resistance (TPR; Finometer, Finapres Medical Systems, Amsterdam, The Netherlands). Duplex ultrasound determined ICA flow using a linear transducer at 8–12 MHz (Logiq E, transducer 12L, GE Medical System, Jiangsu, China). The evaluation was ≥1.5 cm distal to the bifurcation with the head rotated to the contralateral side. At an insonation angle ≤ 60° pulsed-wave Doppler determined the angle-corrected time-averaged maximum blood velocity that corresponds to two times the mean velocity [18]. Software determined diameter (Brachial Analyzer for Research v. 6, Medical Imaging Applications LLC, Coralville, IA, USA) and two to three recordings over approximately 20 s were conducted, and the mean reported with flow calculated as follows [19]:
Conductance was ICA flow divided by MAP.
The ScO2 was determined on the forehead ipsilateral to the evaluation of ICA flow and right deltoid muscle oxygenation (INVOS 5100C, Somanetics, Troy, MI, USA) using an optode with a 3 and 4 cm emitter-receiver separation and wavelengths of 730 and 808 nm. The arterial CO2 tension (PaCO2) influences CBF [20], and thus, ICA flow and ScO2 are reported after correction for any changes in PaCO2 from before induction of anesthesia using the individual CO2 reactivity as determined in the present study (CO2 reactivity for the ICA: 18 [14–24]%/kPa and ScO2: 3.6 (3.1–4.5)%/kPa, respectively, as published elsewhere [17]) and the corrected flow was calculated as follows:
The same formula was used for ScO2.
Laser Doppler flowmetry with an integrated oxygenation sensor evaluated forehead SkBF, oxy- and deoxyhemoglobin concentration (O2HBskin and HBskin), and thus skin oxygenation [O2HBskin/(O2HBskin + HBskin)] close to the hairline at a depth of 1–2 mm (VMS-LDF and VMS-OXY, Moor Instruments, Axminster, UK). The depth of anesthesia was assessed by the bispectral index (BIS Complete Monitoring Systems, Covidien, USA). Arterial gas variables and ICA flow were evaluated 5 min before and after incision and 0, 20, 40, and 70 min after flushing and correspondingly after 20, 40, 60, and 90 min after incision in patients who did not develop MTS.
Central hemodynamic and skin variables were sampled at 100 Hz (Powerlab, ADInstruments, Bella Vista, Australia) and ScO2, muscle oxygenation, and bispectral index every 5 s on a pc, and values were averaged over 2 min. Volume balance and vasopressor administration from 0 to 90 min after the incision was recorded from the anesthesia chart. All measurements were conducted prior to one-lung ventilation for patients undergoing esophageal resection.
2.5. Statistical analysis
2.5.1. Sample size calculation
Reperfusion of a grafted liver during orthotopic liver transplantation is followed by a hemodynamic response similar to that manifesting with the development of MTS and is accompanied by an approximately a 23% increase in CBF when taking into account the concomitant increase in PaCO2 [21] and a sample size calculation indicated that at least six patients who developed MTS were required to detect a similar increase in ICA flow with an SD for the change of 15% [22] at a 5% significance level and a power of 80%.
2.5.2. Outcome
The primary outcome was a change in ICA flow while secondary outcomes were change in ScO2, SkBF, and skin oxygenation from before incision to the start of flushing in patients who developed MTS as compared to those who did not (i.e., the interaction of time point and group according to development of MTS, see below).
2.5.3. Statistics
An analysis of variables was performed by a repeated measures mixed model, fit by restricted maximum likelihood in a structured covariance model with fixed effects, (1) time point (Time; 5 min before and after incision and 0, 20, 40, and 70 min after flushing and correspondingly 20, 40, 60, and 90 min after incision in patients without MTS), (2) group according to development of MTS, and (3) the interaction of time point and group, and the subject variable as a random effect (Proc mixed; SAS 9.4, SAS Institute, Cary, NC, USA). The interaction factor was evaluated at the five time points following baseline in order to evaluate whether changes from baseline were different in the patients who developed MTS as compared to those who did not and the Bonferroni method was used to correct for multiple testing (P < 0.01 for the interaction factor). If the factor “time point” was significant, we evaluated changes from baseline for both groups combined using the mixed model with correction for multiple comparisons by the Tukey-Kramer method. In case the interaction factor was significant, changes over time were evaluated for patients with and without MTS separately using a repeated measures mixed model with the fixed effect time point and the subject variable as a random effect in order to evaluate changes from baseline with correction for multiple comparisons by the Tukey-Kramer method. Analysis of SkBF, O2HBskin, HBskin, plasma lactate, the fraction of inspired O2, and infusion of propofol and remifentanil were conducted after logarithmic transformation. Differences in age, height, weight, and body mass index among patients with and without MTS were evaluated by unpaired t-test. Differences between the groups regarding volume balance and vasopressor administration from 0 to 90 min after the incision was evaluated by Wilcoxon-Mann-Whitney's test. Furthermore, differences in preoperative risk factors, gender, and length of stay were evaluated by Fisher's exact test. The figure was drawn in SigmaPlot 14.0 (Systat Software Inc., CA, USA). Values are presented as mean ± SD or median (IQR) for not normally distributed data, estimates by mixed models for the interaction factor upon flushing are mean (95% CI), and changes are reported as absolute values. Statistical significance was set at P < 0.05.
3. Results
Three patients were excluded because (1) the central venous catheter was placed at the chosen ICA insonation side while contralateral visualization was poor due to vessel tortuosity, (2) cerebral CO2 reactivity was not evaluated due to early one-lung ventilation, or (3) use of sevoflurane. Seven patients developed MTS 22 [20–26] min after the start of surgery and flushing was level I in all cases. Patient characteristics are reported in Table 1 while the surgical procedure, volume balance, and vasopressor administration during the first 90 min of surgery are reported in Table 2. The patients who developed MTS had a higher weight, height, and body mass index than those who did not develop MTS, while age, gender, and preoperative risk factors were similar. For one patient, surgery was converted from stomach resection to gastro-entero anastomosis due to carcinosis. There was a trend toward a higher incidence of MTS during esophageal- as compared to stomach resection.
At baseline, HR was slightly higher in the patients who later developed MTS (Table 3). HR increased upon flushing and remained elevated for 40 min in patients with MTS, whereas HR increased from 20 to 40 min after incision in the patients without MTS. The increase in HR was greater in the patients with MTS as compared to those without from 0 to 40 min after flushing. After incision and until the end of the study, TPR was reduced in the patients who developed MTS while TPR was reduced only at 20 min after incision in the patients without MTS, and the reduction in TPR was greater in the patients who developed MTS (from flushing until the end of the study). The MAP and SV were stable and similar in the two groups. In contrast, CO increased from 0 to 20 min after flushing while it was maintained in the patients without MTS, but the change in CO was not significantly different in the two groups. Development of MTS led to an increase in SkBF (by 122 PU; 95% CI: 53–191; P = 0.0007) upon flushing with an elevation in O2HBskin from 0 to 20 min after flushing whereas, in patients without MTS, SkBF was maintained while O2HBskin was stable until it decreased at 60 and 90 min after incision (Figure 1). Skin and muscle oxygenation were unaffected and similar in the two groups whereas HBskin was stable in patients with MTS while HBskin decreased at the end of the study in the patients without MTS.
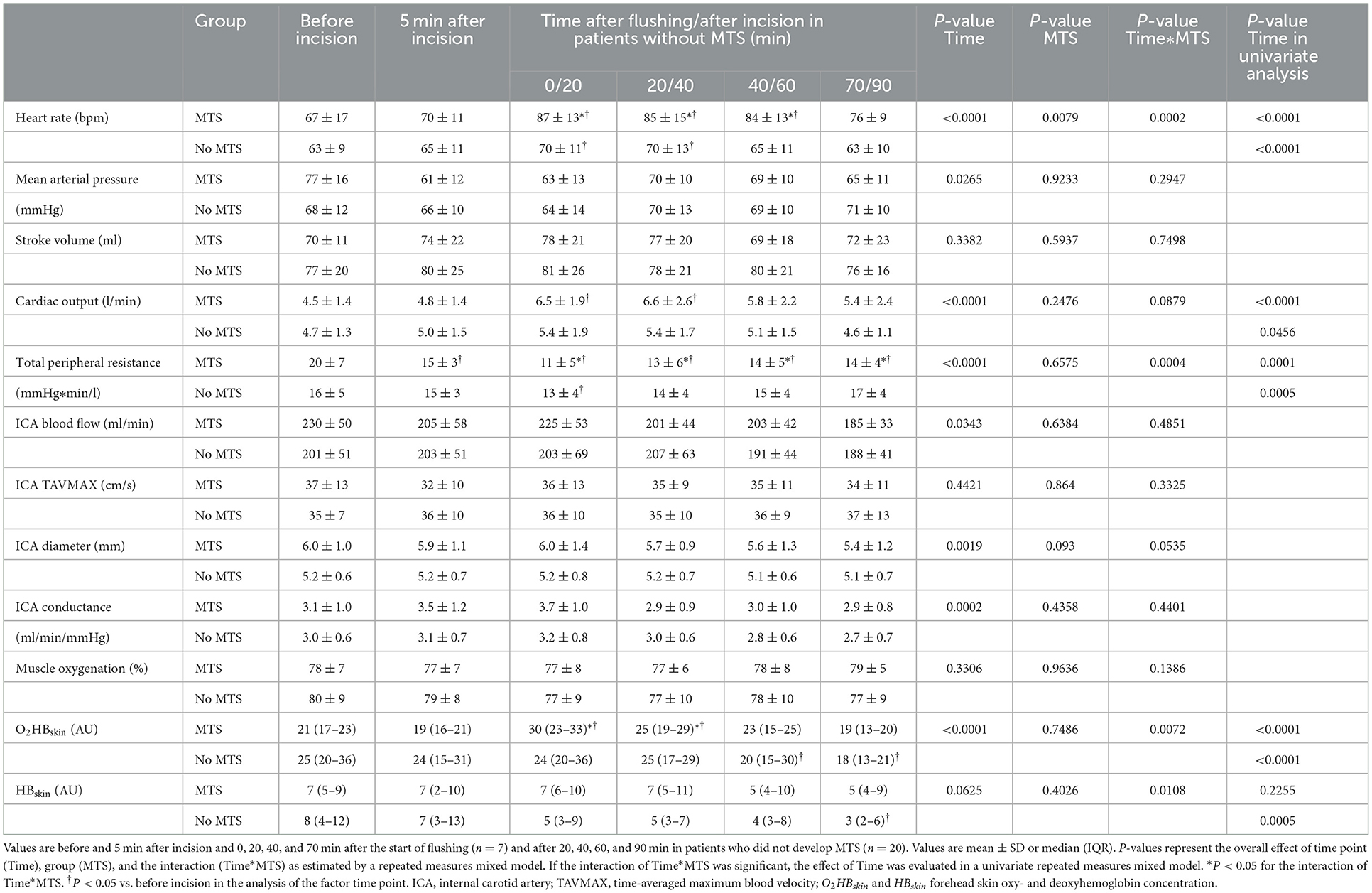
Table 3. Hemodynamic variables in patients who developed mesenteric traction syndrome (MTS) and those who did not.
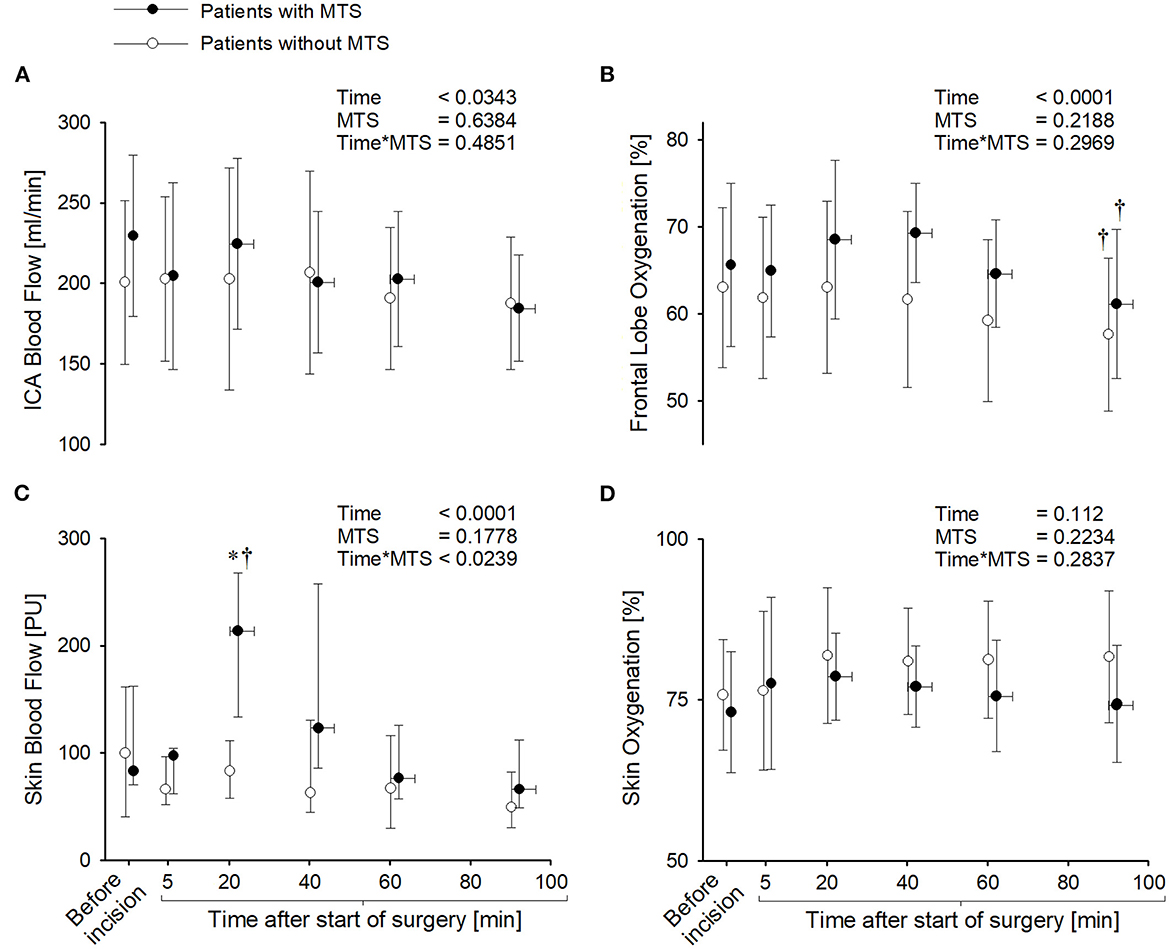
Figure 1. Internal carotid artery (ICA) blood flow (A), near-infrared spectroscopy determined frontal lobe oxygenation (B), and forehead skin blood flow and oxygenation (C, D) before and 5 min after incision and 0, 20, 40, and 70 min after the start of flushing (n = 7) and after 20, 40, 60, and 90 min in patients who did not develop mesenteric traction syndrome (MTS) (n = 20). Values are mean ± SD except for skin blood flow which is reported as median (IQR). P-values represent the overall effect of time point (Time), group (MTS), and the interaction (Time*MTS) as estimated by a repeated measures mixed model. If the interaction of Time*MTS was significant, the effect of Time was evaluated in a univariate repeated measures mixed model. *P < 0.05 for the interaction of Time*MTS. †P < 0.05 vs. before incision in the analysis of the factor time point.
The ScO2 was similar in the two groups and ScO2 was stable during surgery until it decreased at the end of the study. There was no significant change in ICA blood flow in response to MTS (by −1 ml/min; 95% CI: −37–35; P = 0.9529) as ICA blood velocity, diameter, blood flow, and conductance were similar in the two groups, and were unaffected during surgery. The PaO2 was lower at baseline in the patients who developed MTS and PaO2 decreased similarly in both groups from 0 to 20 min after flushing and correspondingly, 20 to 40 min after incision in the patients without MTS (Table 4). The fraction of inspired O2 was reduced from 40 to 60 min after incision in the patients who did not develop MTS but there was no significant difference in the fraction of inspired O2 between the two groups, and the arterial saturation was above 92% in all patients throughout the study. There was no difference between the two groups in PaCO2, hemoglobin concentration, and O2 content, and values were not significantly affected during surgery. Arterial lactate concentration was similar in the two groups, and it increased from 20 to 40 min after flushing and correspondingly from 40 to 60 min after incision in patients without MTS. Infusion of propofol and remifentanil was similar in patients with and without MTS, and the bispectral index was stable. Fluid balance and vasopressor administration were similar in patients with and without MTS, and no patient received red blood cells for the duration of the study. Length of stay was 9 (8–9) days in patients with MTS and 8 (7–10; P = 0.7986) days in those without and there was no mortality within 1 month.
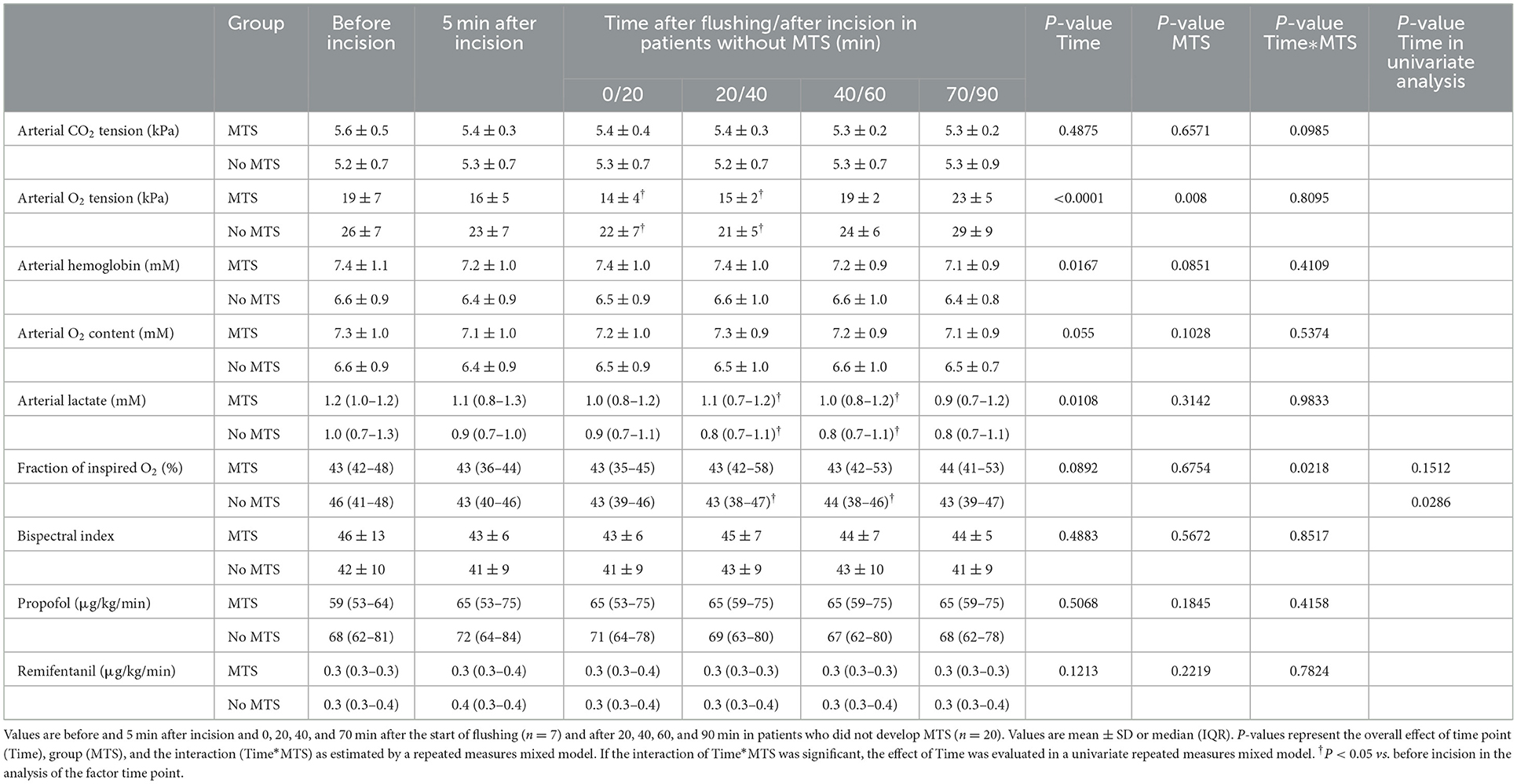
Table 4. Blood and anesthetic variables in patients who developed a mesenteric traction syndrome (MTS) and those who did not.
4. Discussion
This study in patients planned for stomach- or esophageal- resection evaluated whether MTS affects ICA blood flow. The middle cerebral artery blood velocity is reported to be unaffected by MTS [4], but that observation could be influenced by vasodilation and thus duplex ultrasound was used to evaluate blood flow in the ICA which supplies most of CBF. We found that ICA flow was unaffected by MTS with level I flushing while SkBF and CO became elevated in reflection of a reduction in total peripheral resistance. Furthermore, HR increased while MAP was stable when flushing manifested, as MAP was maintained above 60 mmHg by administration of vasopressors, and there was no significant change in ScO2 or skin oxygenation.
MTS likely relates to the release of PGI2 [2, 3, 9] and in support of maintained ICA flow in response to MTS, administration of PGI2 to healthy subjects does not affect CBF [10]. The syndrome is generally accompanied by hypotension whereby the effect of MTS on CBF and central hemodynamics may have been attenuated by the administration of vasopressors in the present study. Furthermore, the patients who developed MTS only presented mild flushing which results in smaller hemodynamic changes than MTS with marked flushing [1, 3, 6]. Development of MTS is reported to elevate ScO2 likely due to increased SkBF [4]. Here, ScO2 was not significantly affected in response to MTS which may reflect the mild degree of flushing and administration of vasopressors [7]. Development of MTS with marked flushing is associated with postoperative complications [1, 23] but MTS appears not to provoke cerebral hyperperfusion.
The degree of traction on the viscera seems important for the development of MTS as the incidence is reported low in laparoscopic surgery [1] whereas MTS is observed in about 90% of patients when the small intestine is exposed [9]. In our study, the incidence of MTS tended to be lower during stomach, as compared to esophageal resection which could relate to less manipulation of the abdominal viscera during stomach resection. However, similar incidences of MTS of about 50–75% in the two procedures are reported which are higher than in the present study [24]. Patients who developed MTS were taller and with larger body mass index which could relate to more forcible manipulation of the viscera in overweight patients, but the difference may be a chance finding as such differences are not reported [4, 25]. At baseline, the patients who later developed MTS had slightly higher HR and lower arterial O2 tension despite a similar fraction of inspired O2 which may be chance findings or could relate to the higher weight of the patients who developed MTS. As none of the patients became hypoxic during the study, we take the difference in arterial O2 tension to have little influence on the hemodynamic changes. No studies have evaluated the influence of preoperative comorbidities on the incidence of MTS [24] and here we found no differences in age or preoperative comorbidities in the two groups. Propofol anesthesia approximately halves CBF by a reduction in neuronal activity [26, 27] but the infusion of propofol and remifentanil was similar in the two groups of patients, and the bispectral index was stable.
Reperfusion of a grafted liver during orthotopic liver transplantation causes central hemodynamic changes similar to MTS with marked vasodilation, but reperfusion of the transplanted liver also elevates CBF even when the increase in PaCO2 is taken into account [21, 28, 29]. The different cerebrovascular responses may relate to the release of different vasoactive metabolites as reperfusion of a transplanted liver elevates prostaglandin I2 as observed in MTS but also increases nitrous oxide, endothelin, and thromboxane, in addition to unknown metabolites [30].
4.1. Limitations
The effect of MTS on skin variables and ScO2 may have been confounded by vasopressor administration [7] but the administered doses were similar in the two groups. Laser Doppler flowmetry does not provide an absolute value of SkBF and the study could have benefitted by evaluation using laser speckle contrast imaging [31]. The evaluation of MTS would be improved by the determination of plasma PGI2, but no cut-off level for the development of MTS is established. The evaluation of ICA flow was unilateral but the contralateral regulation of CBF is similar in response to both propofol anesthesia [26] and administration of, for example, nitroglycerin [32] and L-arginine [33] and we take any difference to be small. We did not evaluate vertebral artery flow which supplies about 20–25% of CBF and may present different regulations from the ICA [34]. The fraction of inspired O2 was not protocolled but was maintained during the study. We did not evaluate the force of traction or the degree to which the abdominal organs were exposed. The study may have been too small to detect an effect of MTS on cardiac output, ICA diameter, or administration of vasopressors.
4.2. Conclusion
Development of MTS with flushing level I causes vasodilation as observed by a reduction in TPR and increased SkBF but appears not to affect ICA flow.
Data availability statement
The raw data supporting the conclusions of this article will be made available by the authors, without undue reservation.
Ethics statement
The studies involving human participants were reviewed and approved by the Ethical Committee of Copenhagen, Denmark. The patients/participants provided their written informed consent to participate in this study.
Author contributions
NO: conception and design of the study, acquisition, analysis and interpretation of data, and writing of the first draft of the manuscript. AE: acquisition of data and revision of the manuscript. H-JF and LS: design of the study and revision of the manuscript. NS: conception and design of the study, interpretation of data, and revision of the manuscript. All authors have provided final approval of the version to be published and are accountable for all aspects of the manuscript, and including the accuracy and validity of the contents.
Funding
The study was funded by the University of Copenhagen.
Acknowledgments
We thank the surgical and anesthetic personnel at Rigshospitalet, Copenhagen, Denmark for their invaluable help.
Conflict of interest
The authors declare that the research was conducted in the absence of any commercial or financial relationships that could be construed as a potential conflict of interest.
Publisher's note
All claims expressed in this article are solely those of the authors and do not necessarily represent those of their affiliated organizations, or those of the publisher, the editors and the reviewers. Any product that may be evaluated in this article, or claim that may be made by its manufacturer, is not guaranteed or endorsed by the publisher.
References
1. Ambrus R, Svendsen LB, Secher NH, Goetze JP, Runitz K, Achiam MP. Severe postoperative complications may be related to mesenteric traction syndrome during open esophagectomy. Scand J Surg. (2017) 106:241–8. doi: 10.1177/1457496916683098
2. Fujimoto Y, Nomura Y, Hirakawa K, Hotta A, Nakamoto A, Yoshikawa N, et al. Flurbiprofen axetil provides a prophylactic benefit against mesenteric traction syndrome associated with remifentanil infusion during laparotomy. J Anesth. (2012) 26:490–5. doi: 10.1007/s00540-012-1368-8
3. Gottlieb A, Skrinska VA, O'Hara P, Boutros AR, Melia M, Beck GJ. The role of prostacyclin in the mesenteric traction syndrome during anesthesia for abdominal aortic reconstructive surgery. Ann Surg. (1989) 209:363–7. doi: 10.1097/00000658-198903000-00018
4. Olesen ND, Sorensen H, Ambrus R, Svendsen LB, Lund A, Secher NH, et al. mesenteric traction syndrome affects near-infrared spectroscopy evaluated cerebral oxygenation because skin blood flow increases. J Clin Monit Comput. (2018) 32:261–8. doi: 10.1007/s10877-017-0014-2
5. Desborough JP. The stress response to trauma and surgery. Br J Anaesth. (2000) 85:109–17. doi: 10.1093/bja/85.1.109
6. Nomura Y, Funai Y, Fujimoto Y, Hori N, Hirakawa K, Hotta A, et al. Remifentanil increases the incidence of mesenteric traction syndrome: preliminary randomized controlled trial. J Anesth. (2010) 24:669–74. doi: 10.1007/s00540-010-0998-y
7. Sørensen H, Rasmussen P, Siebenmann C, Zaar M, Hvidtfeldt M, Ogoh S, et al. Extra-cerebral oxygenation influence on near-infrared-spectroscopy-determined frontal lobe oxygenation in healthy volunteers: a comparison between INVOS-4100 and NIRO-200NX. Clin Physiol Funct Imaging. (2015) 35:177–84. doi: 10.1111/cpf.12142
8. Olesen ND, Fischer M, Secher NH. Sodium nitroprusside dilates cerebral vessels and enhances internal carotid artery flow in young men. J Physiol. (2018) 596:3967–76. doi: 10.1113/JP275887
9. Takada M, Taruishi C, Sudani T, Suzuki A, Iida H. Intravenous flurbiprofen axetil can stabilize the hemodynamic instability due to mesenteric traction syndrome–evaluation with continuous measurement of the systemic vascular resistance index using a FloTrac(R) sensor. J Cardiothorac Vasc Anesth. (2013) 27:696–702. doi: 10.1053/j.jvca.2012.11.019
10. Wienecke T, Olesen J, Oturai PS, Ashina M. Prostacyclin (epoprostenol) induces headache in healthy subjects. Pain. (2008) 139:106–16. doi: 10.1016/j.pain.2008.03.018
11. Boullin DJ, Bunting S, Blaso WP, Hunt TM, Moncada S. Responses of human and baboon arteries to prostaglandin endoperoxides and biologically generated and synthetic prostacyclin: their relevance to cerebral arterial spasm in man. Br J Clin Pharmacol. (1979) 7:139–47. doi: 10.1111/j.1365-2125.1979.tb00914.x
12. Uski T, Andersson KE, Brandt L, Edvinsson L, Ljunggren B. Responses of isolated feline and human cerebral arteries to prostacyclin and some of its metabolites. J Cereb Blood Flow Metab. (1983) 3:238–45. doi: 10.1038/jcbfm.1983.32
13. Larsen FS, Ejlersen E, Hansen BA, Knudsen GM, Tygstrup N, Secher NH. Functional loss of cerebral blood flow autoregulation in patients with fulminant hepatic failure. J Hepatol. (1995) 23:212–7. doi: 10.1016/0168-8278(95)80338-6
14. Simard JM, Bellefleur M. Systemic arterial hypertension in head trauma. Am J Cardiol. (1989) 63:32c−5c. doi: 10.1016/0002-9149(89)90403-7
15. Sundt TM, Sharbrough FW, Piepgras DG, Kearns TP, Messick JM, O'Fallon WM. Correlation of cerebral blood flow and electroencephalographic changes during carotid endarterectomy: with results of surgery and hemodynamics of cerebral ischemia. Mayo Clin Proc. (1981) 56:533–43.
16. Khan MA, Liu J, Tarumi T, Lawley JS, Liu P, Zhu DC, et al. Measurement of cerebral blood flow using phase contrast magnetic resonance imaging and duplex ultrasonography. J Cereb Blood Flow Metab. (2017) 37:541–9. doi: 10.1177/0271678X16631149
17. Olesen ND, Frederiksen HJ, Storkholm JH, Hansen CP, Svendsen LB, Olsen NV, et al. Internal carotid artery blood flow is enhanced by elevating blood pressure during propofol-remifentanil anesthesia. J Anaesthesiol Clin Pharmacol. (2022) 38:580–7. doi: 10.4103/joacp.JOACP_575_20
18. Evans DH. On the measurement of the mean velocity of blood flow over the cardiac cycle using Doppler ultrasound. Ultrasound Med Biol. (1985) 11:735–41. doi: 10.1016/0301-5629(85)90107-3
19. Thomas KN, Lewis NC, Hill BG, Ainslie PN. Technical recommendations for the use of carotid duplex ultrasound for the assessment of extracranial blood flow. Am J Physiol Regul Integr Comp Physiol. (2015) 309:R707–20. doi: 10.1152/ajpregu.00211.2015
20. Mariappan R, Mehta J, Chui J, Manninen P, Venkatraghavan L. Cerebrovascular reactivity to carbon dioxide under anesthesia: a qualitative systematic review. J Neurosurg Anesthesiol. (2015) 27:123–35. doi: 10.1097/ANA.0000000000000092
21. Skak C, Rasmussen A, Kirkegaard P, Secher NH. Cerebral oxygen saturation and blood flow during liver transplantation. Anesth Analg. (1997) 84:730–3. doi: 10.1097/00000539-199704000-00005
22. Sato K, Ogoh S, Hirasawa A, Oue A, Sadamoto T. The distribution of blood flow in the carotid and vertebral arteries during dynamic exercise in humans. J Physiol. (2011) 589:2847–56. doi: 10.1113/jphysiol.2010.204461
23. Olsen AA, Strandby RB, Nerup N, Johansson PI, Svendsen LB, Achiam MP. Severe mesenteric traction syndrome is associated with increased systemic inflammatory response, endothelial dysfunction, and major postoperative morbidity. Langenbeck's Arch Surg. (2021) 406:2457–67. doi: 10.1007/s00423-021-02111-1
24. Olsen AA, Bazancir LA, Dahl S, Fukumori D, Shiwaku H, Svendsen LB, et al. Mesenteric traction syndrome - Incidence, impact, and treatment: A systematic scoping review of the literature. Anaesth Crit Care Pain Med. (2022) 42:101162. doi: 10.1016/j.accpm.2022.101162
25. Brinkmann A, Seeling W, Rockemann M, Junge JH, Radermacher P, Wiedeck H, et al. Changes in gastric intramucosal pH following mesenteric traction in patients undergoing pancreas surgery. Dig Surg. (1999) 16:117–24. doi: 10.1159/000018703
26. Kaisti KK, Langsjo JW, Aalto S, Oikonen V, Sipila H, Teras M, et al. Effects of sevoflurane, propofol, and adjunct nitrous oxide on regional cerebral blood flow, oxygen consumption, and blood volume in humans. Anesthesiology. (2003) 99:603–13. doi: 10.1097/00000542-200309000-00015
27. Song XX Yu BW. Anesthetic effects of propofol in the healthy human brain: functional imaging evidence. J Anesth. (2015) 29:279–88. doi: 10.1007/s00540-014-1889-4
28. Larsen FS, Ejlersen E, Strauss G, Rasmussen A, Kirkegaard P, Hansen BA, et al. Cerebrovascular metabolic autoregulation is impaired during liver transplantation. Transplantation. (1999) 68:1472–6. doi: 10.1097/00007890-199911270-00007
29. Aggarwal S, Kang Y, DeWolf A, Scott V, Martin M, Policare R. Transcranial Doppler: monitoring of cerebral blood flow velocity during liver transplantation. Transplant Proc. (1993) 25:1799–800.
30. Hei ZQ, Huang HQ, Luo CF Li SR, Luo GJ. Changes of nitric oxide and endothelin, thromboxane A2 and prostaglandin in cirrhotic patients undergoing liver transplantation. World J Gastroenterol. (2006) 12:4049–51. doi: 10.3748/wjg.v12.i25.4049
31. Ambrus R, Strandby RB, Svendsen LB, Achiam MP, Steffensen JF, Sondergaard Svendsen MB. Laser speckle contrast imaging for monitoring changes in microvascular blood flow. Eur Surg Res. (2016) 56:87–96. doi: 10.1159/000442790
32. Bednarczyk EM, Wack DS, Kassab MY, Burch K, Trinidad K, Haka M, et al. Brain blood flow in the nitroglycerin (GTN) model of migraine: measurement using positron emission tomography and transcranial Doppler. Cephalalgia. (2002) 22:749–57. doi: 10.1046/j.1468-2982.2002.00440.x
33. Pretnar-Oblak J, Zaletel M, Zvan B, Sabovic M, Pogacnik T. Cerebrovascular reactivity to L-arginine in patients with lacunar infarctions. Cerebrovasc dis. (2006) 21:180–6. doi: 10.1159/000090530
Keywords: anesthesia, internal carotid artery, cerebrovascular circulation, near-infrared spectroscopy, skin blood flow, surgery, mesenteric traction syndrome, cerebral blood flow
Citation: Olesen ND, Egesborg AH, Frederiksen HJ, Svendsen LB and Secher NH (2023) The effect of a mesenteric traction syndrome on internal carotid artery blood flow. Front. Anesthesiol. 2:1101013. doi: 10.3389/fanes.2023.1101013
Received: 17 November 2022; Accepted: 16 January 2023;
Published: 15 February 2023.
Edited by:
Hossam El Beheiry, University of Toronto, CanadaReviewed by:
Silvestro Roatta, University of Turin, ItalyDominik P. Guensch, Insel Gruppe AG, Switzerland
Copyright © 2023 Olesen, Egesborg, Frederiksen, Svendsen and Secher. This is an open-access article distributed under the terms of the Creative Commons Attribution License (CC BY). The use, distribution or reproduction in other forums is permitted, provided the original author(s) and the copyright owner(s) are credited and that the original publication in this journal is cited, in accordance with accepted academic practice. No use, distribution or reproduction is permitted which does not comply with these terms.
*Correspondence: Niels D. Olesen, bmllbHMuZGFta2phZXIub2xlc2VuLjAxJiN4MDAwNDA7cmVnaW9uaC5kaw==