- Laboratory for the Analysis of Medicines, Center for Interdisciplinary Research on Medicines (CIRM), University of Liege, Liege, Belgium
Phosphorothioate (PS) oligonucleotides have drawn more and more attention lately due to their great therapeutic potential. The presence of one (or several) phosphorothioate moiety (ies) improves pharmacokinetic properties but at the same time creates an additional chiral center for each phosphorothioate linkage, and thus diastereomers. It is therefore important to develop analytical strategies to monitor individual species to enable more in-depth investigations. In this study, a PVA coated capillary with a background electrolyte made of 100 mM phosphoric acid adjusted to pH 3.0 with triethanolamine was used. A design of experimental approach provides the optimal conditions for the separation of the eight isobaric diastereomers bearing one phosphorothioate linkage (Mix 1), and the separation of the 12 isobaric diastereomers of a mixture made of oligonucleotides with two phosphorothioate linkages (Mix 2). Remarkably, full separation in Mix 1 could be achieved using a combination of a cationic cyclodextrin (2-hydroxy-3-N,N,N-trimethylamino) propyl-γ-CD chloride, and an anionic cyclodextrin (carboxymethyl-
1 Introduction
Capillary electrophoresis (CE) is a powerful analytical technique widely used in various fields of research and industry for the separation of charged molecules based on their electrophoretic mobility in a capillary filled with an electrolyte solution (Schmitt-Kopplin, 2016).
The separation of enantiomers and diastereomers, compounds with identical molecular formulas and structural similarities but distinct three-dimensional spatial arrangements, has been a longstanding challenge in analytical chemistry and biochemistry. It is essential, especially in pharmacy and molecular biology, where the biological activity of a molecule often depends on its chirality. One breakthrough approach in achieving this separation is the use of CE and cyclodextrins (CDs) in the background electrolyte. CDs, cyclic oligosaccharides composed of glucose units, possess a cavity that can encapsulate molecules, forming inclusion complexes. Over the past few decades, the application of cyclodextrins in CE has revolutionized chiral separation, providing an efficient and high-resolution technique for resolving complex mixtures of chiral compounds (Chankvetadze et al., 1994; Fillet et al., 2000; Rudaz et al., 2000; Fradi et al., 2006; 2012; Marini et al., 2007; Lomsadze et al., 2009).
Phosphorothioate oligonucleotides are structural variants of nucleic acids, in which at least one of the oxygen atoms in the phosphate backbone is replaced with a sulfur atom, creating an additional chiral center for each phosphorothioate (PS) linkage and thus diastereomers (Figure 1). These diastereomers play a crucial role in biotechnology as they can be incorporated into synthetic DNA and RNA sequences, offering enhanced stability and resistance to enzymatic degradation (Iwamoto et al., 2017). Their unique properties have made them valuable tools for various applications, such as antisense oligonucleotide therapeutics and PCR amplification. Understanding the distinct characteristics of phosphorothioate oligonucleotide diastereomers is essential for exploiting their potential in the design of nucleic acid-based technologies and therapies (Genna et al., 2023).
In a recent study, preliminary investigations for the separation of short phosphorothioate oligonucleotide diastereomers with closely related structures were conducted (Demelenne et al., 2020; Ghassemi et al., 2022). We observed that to have a successful separation applying one type of CD is not efficient. An acidic BGE containing a dual CD system containing 25 mM (2-hydroxy-3-N,N,N-trimethylamino)propyl-γ-CD and 10 mM carboxymethyl-β-cyclodextrin was determined as the most appropriate system for the qualitative evaluation of the short oligonucleotides investigated. In the present study, we focused our attention on chemo- and stereoselectivity in two very challenging mixtures containing isomeric phosphorothioate oligonucleotides of identical molecular mass. A Design of Experiment (DoE) approach was used to model the effects of two types of positively charged CD derivatives on the separation.
Full Factorial Design (FFD) is a powerful approach that explores all possible combinations of factors and their levels in a minimum number of experiments. It was applied to investigate the effect of positively charged cyclodextrins, namely, (2-hydroxy-3-N, N, N-trimethylamino) propyl-γ-CD (QA-γ-CD) and (2-hydroxy-3-N, N, N-trimethylamino) propyl-
2 Material and methods
2.1 Chemicals
All lyophilized oligonucleotide samples were supplied by Integrated DNA Technologies (Coralville, IA, United States of America). Carboxymethyl-
Triethanolamine (TEA) and methanol were supplied by Merck (Darmstadt, Germany). Phosphoric acid 85% was purchased from VWR Chemicals (Leuven, Belgium). Ultrapure water was provided by Milli-Q equipment (Millipore, Bedford, MA, United States) and Chromafil syringe filters (0.20 µm) were from Macherey-Nagel (Düren, Germany). All chemicals were of analytical grade and used without further purification.
2.2 Oligonucleotides
The oligonucleotides investigated had the following sequence: 5′-TCGTG-3’. Variations were related to the amount and position of PS linkages. The individual lyophilized oligonucleotides were reconstituted separately in ultrapure water at 1 mM concentration. Subsequently, all samples were diluted to a final concentration of 50 µM in water. To prepare Mix 1, comprising PS 1_1, PS 1_2, PS 1_3, and PS 1_4 (as detailed in Table 1), these compounds were diluted in water to obtain a concentration of 50 µM for each component. Mix 2, comprising PS 2_1,3, PS 2_2,3 and PS 2_3,4 was prepared using the same approach.
2.3 Equipment
Separations were carried out on a G7100 CE system (Agilent Technologies, Waldbronn, Germany) coupled to a Diode-Array Detector (DAD). A PVA-coated fused-silica capillary of 64.5 cm long (50 µm ID, 56 cm effective length) was used to perform the experiments (Agilent Technologies, Germany). Hydrodynamic injection was used for all samples by applying a pressure of 50 mbar for 6 s, following by an injection of BGE at 50 mbar for 4 s. The separation voltage was set at −30 kV during the experiments. The UV-detection wavelength was set at 254 ± 4 nm. Instrument control and data acquisition were achieved using the Agilent Openlab version 2.7 software.
2.4 CE operating conditions
A new capillary was conditioned first by flushing water, followed by BGE, for 15 min each. At the beginning of each day, the capillary was rinsed with water and then by BGE, both for 10 min. In addition, before each run, water and BGE were successively flushed both for 5 min. The capillary was washed at the end of each sequence with water for 30 min and then dried with air for 5 min. Rinses were performed at a pressure of approximately 1 bar. The BGE consisted of 100 mM phosphoric acid adjusted to pH 3.0
2.5 Computation
Experimental design (Supplementary Table S1) and data analysis were performed using JMP software version 17.2.0 (SAS Institute, Inc., Cary, NC, United States).
3 Results and discussion
Several critical parameters play a pivotal role in the CE separation of phosphorothioate oligonucleotide diastereomers using CDs as chiral selectors, such as the choice of BGE, the BGE pH, the capillary length and voltage, and of course, the type and concentration of the CD, possibly used in combination with another one. In our previous article (Ghassemi et al., 2022), various BGEs as well as charged, negatively charged and neutral CDs were investigated for the separation of 5-mer oligonucleotides containing 0, 1, 2, or 3 phosphorothioate linkages. Combining a positively charged cyclodextrin (QA-b-CD or QA-g-CD) with a weakly negatively charged (CM-b-CD) cyclodextrin improved chemo- and enantio-selectivity in a BGE made of 100 mM phosphoric acid adjusted to pH 3.
3.1 Application of previously selected conditions for mix 1 and mix 2
First, we applied the dual CD system conditions selected in a previous study dealing with another mixture of short oligonucleotides (Ghassemi et al., 2022). The BGE was made of a mixture of QA-γ-CD and CM-β-CD or QA-β-CD and CM-β-CD, in 100 mM phosphoric acid-TEA pH 3.0 buffer.
By employing charged CD derivatives, the influence of electrostatic interactions can alter the separation mechanism and profile. Consequently, utilizing chiral selectors possessing an opposite charge to that of the analytes can be highly advantageous for enhancing resolution. Indeed, a wider separation window provides greater flexibility for effectively separating compounds with limited resolution (Blanco et al., 2003).
The combination of 25 mM QA-β-CD or 25 mM QA-γ-CD as a positively charged CD and 10 mM CM-β-CD as a weakly negatively charged CD was first tested (data not shown). By using 25 mM QA-β-CD and 10 mM CM-β-CD, half of the peaks in Mix 1 (4 out of 8) and 9 out of 12 peaks for Mix 2 were observed. A combination of 25 mM of QA-γ-CD and 10 mM CM-β-CD provided better separation of the compounds of Mix 1 (7 out of eight peaks were detected).
To obtain a lower current, shorter migration times and a more stable baseline, a five times lower concentration of the positively charged CDs was tested. The results obtained for Mix 1 and Mix 2 are shown in Figures 2A, B. Each sample was also injected independently in order to assign the peaks.
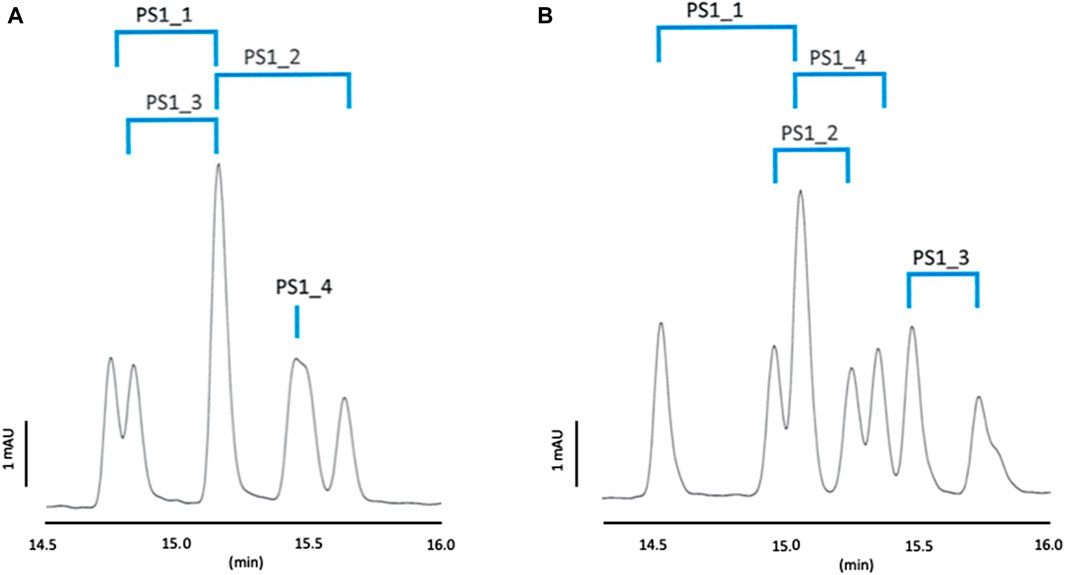
Figure 2. Separation profiles of Mix 1. BGE: 100 mM phosphoric acid adjusted with TEA to pH 3.0 containing 10 mM CM-β-CD and 5 mM QA-β-CD (A) or 5 mM QA-γ-CD (B), capillary temperature: 25°C, current
As can be seen in Figure 2A with QA-β-CD derivative, the PS1_4 diastereomeric pair was not separated and 5 peaks out of eight could be observed, which confirms the poor chemoselectivity provided by this cyclodextrin. On the contrary, all diastereomeric pairs were separated with QA-γ-CD (see Figure 2B), and 7 out of the eight peaks were visible. QA-γ−CD showed thus better chemo- and stereoselectivity, and its concentration should be further optimized for Mix 1. It is also worth mentioning to note that the two studied cationic cyclodextrins do not show the same PS selectivity and profile.
For Mix 2, QA-β-CD could separate the 4 diastereomers of PS2_1,3 and PS2_2,3 but not those of PS2_3,4 (Figure 3A). QA-γ-CD could separate the 4 diastereomers of PS2_1,3 but not those of PS2_2,3 and PS2_3,4 (Figure 3B). In both cases, 9 out of the 12 peaks were observed. As for Mix 1, QA-β-CD and QA-γ-CD do not show the same chemo- and stereoselectivity. QA-β-CD shows the strongest affinity for PS2_2,3, while QA-γ-CD shows the strongest affinity for PS2_3,4. So it could be interesting to test their combination to optimize Mix 2 separation.
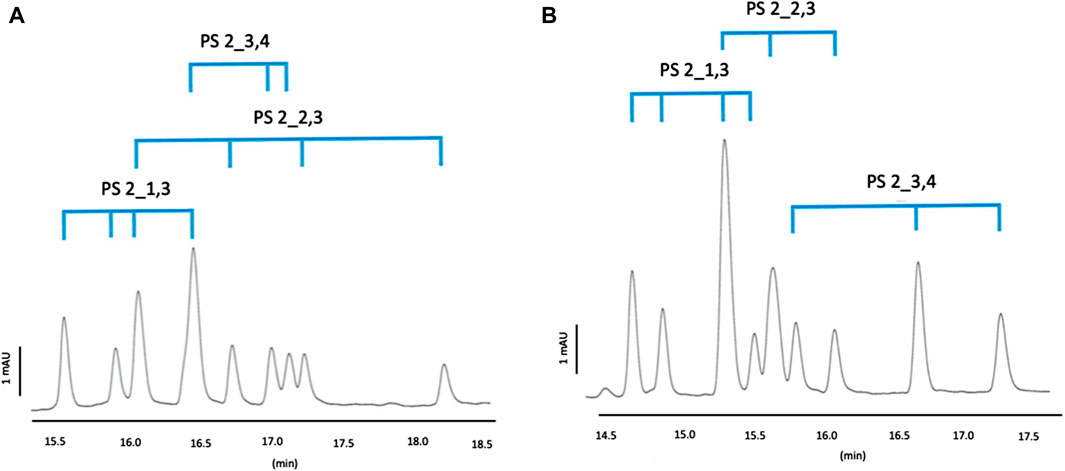
Figure 3. Separation profiles of Mix 2, BGE: 100 mM phosphoric acid adjusted by TEA pH 3.0 containing 10 mM CM-β-CD and 5 mM QA-β-CD (A) or 5 mM QA-γ-CD (B), capillary temperature: 25°C, current
Overall, QA-γ-CD showed more promising separation for Mix 1 and inversely, QA-β-CD for Mix 2. Since at lower CD concentrations, an improvement in the resolution of critical pairs and much lower current were observed, it was decided to select the 5 mM concentration for both cationic CDs in further experiments.
3.2 Impact of capillary temperature and methanol on separation
To further investigate the critical experimental parameters, we considered three critical pairs in Mix 1 and four critical pairs in Mix 2 (the critical pair selection was based on full separation profiles obtained in DoE runs, see Section 3.3). Both positional isomers and diastereomers were involved in critical pairs for both mixtures considering that the separation of diastereomers identified as critical pairs was the most complicated.
Temperature can significantly influence the complexation between the chiral selector (CD) and the analytes, impacting both the efficiency and selectivity of the separation. Higher capillary temperatures can enhance analyte mobility, potentially reducing analysis times, but too high temperatures may compromise cyclodextrin complex stability, leading to lower peak resolution. Conversely, lower temperatures can strengthen interactions and improve selectivity at the expense of longer analysis times. Lowering the temperature to 15°C led to a slight improvement in selectivity. Therefore, 15°C was selected for further experiments.
Methanol is often employed to adjust the electrophoretic mobility of analytes, and its concentration in the electrolyte can influence the interactions between the chiral selector and the analytes. An increase in methanol concentration typically leads to reduced viscosity and decreases the electroosmotic flow, which can result in longer analysis times and potentially improved separation efficiency. Methanol contents of 5%–10% were investigated since too high amounts can disrupt the formation of stable complexes between the cyclodextrin and the diastereomers, leading to peak distortion or even loss of resolution. In this study, the addition of methanol did not lead to any increase in resolution but improved the stability of the method, and the methanol content was kept at 7.5%.
3.3 Full factorial design of experiment
In the context of optimizing the CE separation of diastereomers using a combination of cationic and slightly anionic CDs, DoE plays a major role in systematically exploring the intricate interplay of factors that can influence the separation. A full factorial design allows researchers to investigate several factors simultaneously at various levels (Jiju, 2023). These levels refer to specific values or settings at which each factor is tested. According to the abovementioned results, the concentrations of the positively charged CDs were considered the main factors in the elaboration of the FFD at five levels (0, 2.5, 5.0, 7.5, and 10 mM) (Supplementary Table S1). This design comprised 28 runs with three replicates at the central point to evaluate repeatability. All analytes were analysed separately as well as in mixture. Other parameters considered as constant factors were the voltage (−30 kV), the percentage of organic modifier (methanol at 7.5%), the temperature (15°C) and CM-β-CD concentration (10 mM). The concentration of the anionic cyclodextrin was not investigated further, as we had already shown in a previous study (16), and with additional tests (up to 45 mM), concentrations higher than 10 mM only increased viscosity and migration time and had no significant effect on the separation profile.
By choosing the number of peaks and resolution of critical pairs in each mixture as responses (namely, resolution between peaks 3–4, 4-5, and 6-7 in Mix 1 and peaks 2–3, 3–4, 5-6 and 6-7 in Mix 2), we evaluated the data obtained in the 28 runs. The desirability value is a crucial concept to assess the overall quality of a set of experimental conditions with different units or scales, which are combined into a single value. By assigning weights to each response variable to express their relative importance, we used desirability functions to optimize the separation, seeking conditions that maximize the overall desirability value.
Each response was modelled according to the following equation:
where β0 is the intercept, β1 and β2 the main effect terms, β12 the interaction term, X1 the QA-β-CD concentration, X2 the QA-γ-CD concentration and ε the error term.
The coefficients of the model with their statistical significance are presented in Supplementary Table S2. The p-value represents the probability of obtaining a result as extreme or more extreme than the observed one, assuming the proposed null hypothesis is true. A factor is considered as significant if its p-value is lower than 0.05.
As can be seen in Supplementary Table S2, for Mix 1, QA-γ-CD concentration has a positive impact on the resolution of critical pair 3-4 through its main term, while the interaction between CD concentrations has a significant impact on critical pair 4-5 and the peak number. For Mix 2, significant impacts of QA-β-CD concentration on the separation of critical pairs 2-3 and 3-4 and of QA-γ-CD concentration on critical pairs 2-3 and 6-7 can be observed. The interaction between the two cationic CD concentrations shows a significant effect on all responses, except on critical pairs 6–7. Overall, it can be concluded that the interaction between these two CD concentrations has an impact on the separation of compounds in Mix 2, particularly.
Table 2 presents the predicted Rs values with the confidence intervals for the critical pairs under the optimum concentrations of each cationic CD for the chemo- and stereo-separation with a desirability of 0.9 in Mix 1 (0.35 mM QA-β-CD and 9.98 mM QA-γ-CD) and a desirability value of 0.75 in Mix 2 (10 mM QA-β-CD and 2.91 mM QA-γ-CD). For practical reasons, we used a BGE made of 10 mM QA-γ-CD for Mix 1 and of 10 mM QA-β-CD and 3 mM QA-γ-CD for Mix 2.
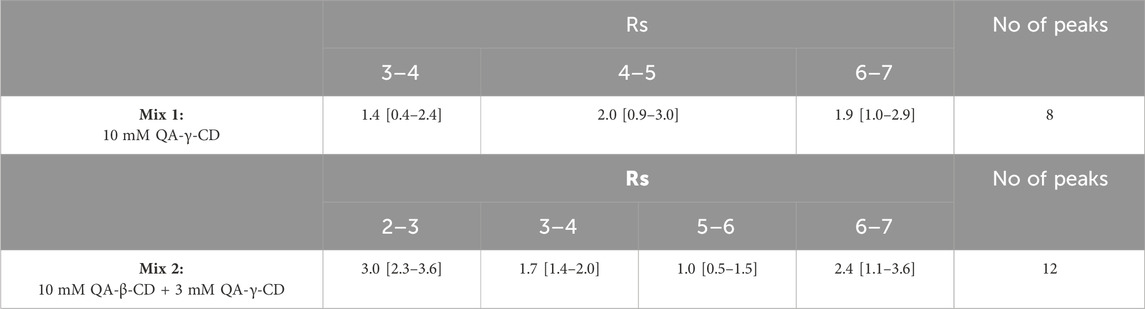
Table 2. Predicted resolution values with the confidence intervals for the critical pairs under the optimum concentrations of each cationic CD for the two oligonucleotide mixtures.
Those conditions were tested experimentally. Figures 4, 5 show the electropherograms under the optimal CD concentrations for Mix 1 and Mix 2.
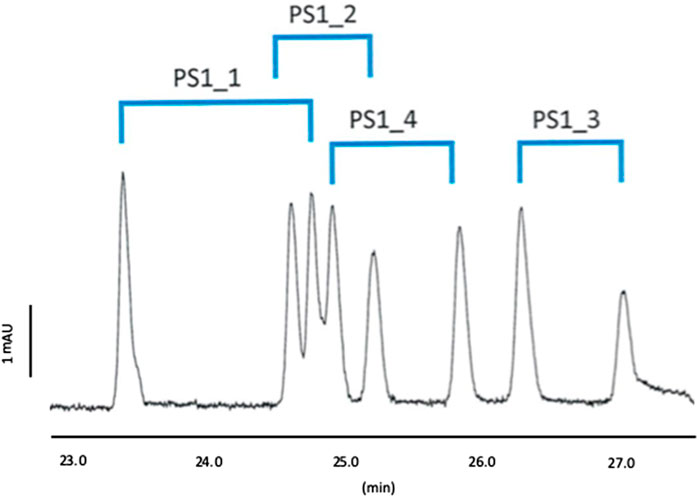
Figure 4. Separation profile of Mix 1; BGE: 100 mM phosphoric acid adjusted by TEA pH 3.0 with 7.5% MeOH containing 10 mM CM-β-CD and 10 mM QA-γ-CD; Capillary temperature: 15°C.
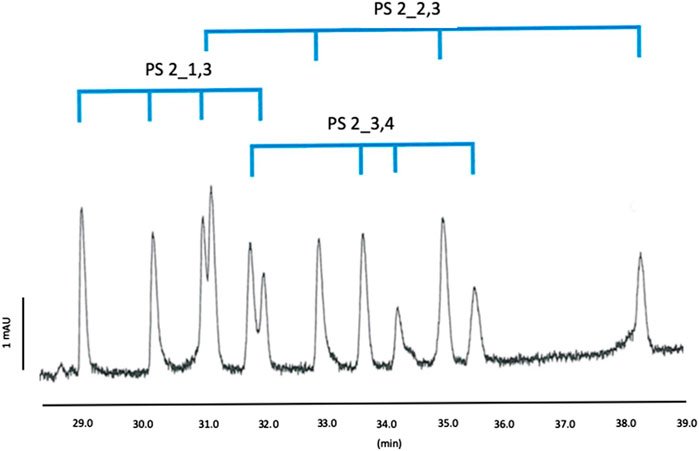
Figure 5. Separation profile of Mix 2, BGE: 100 mM phosphoric acid adjusted by TEA pH 3.0 with 7.5% MeOH containing 10 mM CM-β-CD, 10 mM QA-β-CD and 3 mM QA-γ-CD; Capillary temperature: 15°C.
As the optimal conditions are located at the limits of the experimental domain, CD concentrations higher than 10 mM were tested in order to further increase the resolution values. Nevertheless, no improvement was observed, confirming that the DoE fulfilled its objectives.
Accordingly, we could confirm the prediction of the DoE, allowing the detection of all the expected peaks, i.e. 8 peaks for Mix 1 and 12 peaks for Mix 2, as shown in Figures 4, 5.
4 Conclusion
By combining cyclodextrins with different charge states and testing two different cavity sizes (beta and gamma), it has become possible to exploit a wider spectrum of interactions, in order to achieve better separation selectivity, particularly for mixture 2; bearing in mind that larger cyclodextrin cavities (gammaCD) generally result in longer migration times due to the stronger formation of inclusion complexes. Overall, the interaction between the nature and concentration of cyclodextrins, cavity size and their use in combination is complex and requires careful optimization. By systematically applying the principles of DoE, we have identified the optimum conditions for obtaining better separation of isobaric phosphorothioate oligonucleotides as a function of their chiral centers and overall molecular architectures.
Data availability statement
The raw data supporting the conclusion of this article will be made available by the authors, without undue reservation.
Author contributions
MG: Investigation, Methodology, Writing–original draft. VH: Investigation, Methodology, Writing–review and editing. JC: Writing–review and editing. A-CS: Methodology, Writing–review and editing, Supervision. MF: Conceptualization, Funding acquisition, Investigation, Project administration, Resources, Writing–original draft, Writing–review and editing, Supervision.
Funding
The author(s) declare financial support was received for the research, authorship, and/or publication of this article. The authors thank the University of Liege and the Foundation Léon Frédéricq for the financial support.
Conflict of interest
The authors declare that the research was conducted in the absence of any commercial or financial relationships that could be construed as a potential conflict of interest.
The author(s) declared that they were an editorial board member of Frontiers, at the time of submission. This had no impact on the peer review process and the final decision.
Publisher’s note
All claims expressed in this article are solely those of the authors and do not necessarily represent those of their affiliated organizations, or those of the publisher, the editors and the reviewers. Any product that may be evaluated in this article, or claim that may be made by its manufacturer, is not guaranteed or endorsed by the publisher.
Supplementary material
The Supplementary Material for this article can be found online at: https://www.frontiersin.org/articles/10.3389/frans.2024.1421463/full#supplementary-material
References
Blanco, M., and Valverde, I. (2003). Choice of chiral selector for enantioseparation by capillary electrophoresis. TrAC 22 (7), 428–439. doi:10.1016/S0165-9936(03)00705-2
Chankvetadze, B., Endresz, G., and Blaschke, G. (1994). About some aspects of the use of charged cyclodextrins for capillary electrophoresis enantioseparation. Electrophoresis 15 (1), 804–807. doi:10.1002/elps.11501501113
Demelenne, A., Gou, M. J., Nys, G., Parulski, C., Crommen, J., Servais, A. C., et al. (2020). Evaluation of hydrophilic interaction liquid chromatography, capillary zone electrophoresis and drift tube ion-mobility quadrupole time of flight mass spectrometry for the characterization of phosphodiester and phosphorothioate oligonucleotides. J. Chromatogr. A 1614, 460716. doi:10.1016/j.chroma.2019.460716
Fillet, M., Hubert, P., and Crommen, J. (2000). Enantiomeric separations of drugs using mixtures of charged and neutral cyclodextrins. J. Chromatogr. A 875 (1-2), 123–134. doi:10.1016/s0021-9673(00)00084-4
Fradi, I., Servais, A. C., Lamalle, C., Kallel, M., Abidi, M., Crommen, J., et al. (2012). Chemo-and enantio-selective method for the analysis of amino acids by capillary electrophoresis with in-capillary derivatization. J. Chromatogr. A 1267, 121–126. doi:10.1016/j.chroma.2012.05.098
Fradi, I., Servais, A. C., Pedrini, M., Chiap, P., Iványi, R., Crommen, J., et al. (2006). Enantiomeric separation of acidic compounds using single-isomer amino cyclodextrin derivatives in nonaqueous capillary electrophoresis. Electrophoresis 27 (17), 3434–3442. doi:10.1002/elps.200500643
Genna, V., Iglesias-Fernández, J., Reyes-Fraile, L., Villegas, N., Guckian, K., Seth, P., et al. (2023). Controlled sulfur-based engineering confers mouldability to phosphorothioate antisense oligonucleotides. Nucleic Acids Res. 51 (10), 4713–4725. doi:10.1093/nar/gkad309
Ghassemi, K. M., Demelenne, A., Crommen, J., Servais, A. C., and Fillet, M. (2022). Improvement of chemo- and stereoselectivity for phosphorothioate oligonucleotides in capillary electrophoresis by addition of cyclodextrins. J. Chromatogr. A 1676, 463270. doi:10.1016/j.chroma.2022.463270
Iwamoto, N., Butler, D. C. D., Svrzikapa, N., Mohapatra, S., Zlatev, I., Sah, D. W. Y., et al. (2017). Control of phosphorothioate stereochemistry substantially increases the efficacy of antisense oligonucleotides. Nat. Biotechnol. 35 (9), 845–851. doi:10.1038/nbt.3948
Jiju, A. (2023) Design of experiments for engineers and scientists. 3. Elsevier, 65–87. ISBN 9780443151736. doi:10.1016/B978-0-443-15173-6.00009-3
Lomsadze, K., Martínez-Girón, A. B., Castro-Puyana, M., Chankvetadze, L., Crego, A. L., Salgado, A., et al. (2009). About the role of enantioselective selector-selectand interactions and the mobilities of diastereomeric associates in enantiomer separations using CE. Electrophoresis 30 (16), 2803–2811. doi:10.1002/elps.200900076
Marini, R. D., Rozet, E., Vander, H. Y., Ziemons, E., Boulanger, B., Bouklouze, A., et al. (2007). Robustness testing of a chiral NACE method for R-timolol determination in S-timolol maleate and uncertainty assessment from quantitative data. JPBA 44 (3), 640–651. doi:10.1016/j.jpba.2006.08.018
Rudaz, S., Cherkaoui, S., Dayer, P., Fanali, S., and Veuthey, J. L. (2000). Simultaneous stereoselective analysis of tramadol and its main phase I metabolites by on-line capillary zone electrophoresis–electrospray ionization mass spectrometry. J. Chromatogr. A 868 (2), 295–303. doi:10.1016/S0021-9673(99)01257-1
Keywords: phosphorothioate oligonucleotide, diastereomeric separation, capillary electrophoresis, cyclodextrin, design of experiment
Citation: Ghassemi MK, Hurlet V, Crommen J, Servais A-C and Fillet M (2024) Separation of isobaric phosphorothioate oligonucleotides in capillary electrophoresis: study of the influence of cationic cyclodextrins on chemo and stereoselectivity. Front. Anal. Sci. 4:1421463. doi: 10.3389/frans.2024.1421463
Received: 22 April 2024; Accepted: 24 May 2024;
Published: 13 June 2024.
Edited by:
Ann Van Eeckhaut, Vrije Universiteit Brussel, BelgiumReviewed by:
Emmanuelle Lipka, Lille University, FranceGerhard Scriba, Friedrich Schiller University Jena, Germany
Copyright © 2024 Ghassemi, Hurlet, Crommen, Servais and Fillet. This is an open-access article distributed under the terms of the Creative Commons Attribution License (CC BY). The use, distribution or reproduction in other forums is permitted, provided the original author(s) and the copyright owner(s) are credited and that the original publication in this journal is cited, in accordance with accepted academic practice. No use, distribution or reproduction is permitted which does not comply with these terms.
*Correspondence: Marianne Fillet, Marianne.fillet@uliege.be