- 1Institute for Chemistry and Biochemistry, Freie Universität Berlin, Berlin, Germany
- 2Department of Molecular Physics, Fritz-Haber-Institut der Max-Planck-Gesellschaft, Berlin, Germany
- 3Biopharmaceuticals, Institute of Pharmacy, Freie Universität Berlin, Berlin, Germany
The increasing antibiotic resistance in bacteria is an alarming phenomenon all around the world. Certain strains have developed resistance against multiple antimicrobial molecules, in which cases, the final option is to use a last-resort drug. These drugs, however, are last-resort for a reason: they can pose serious risk on vital organ functions in the patient. To mitigate the risk of severe side-effects and to reduce the rate of bacterial mutation, co-administration with other molecules that increase their efficacy seems to be the only suitable option. This leads to a reduced dose while maintaining the same level of antibiotic activity within the body. In this study, the effect of heparin derivatives on the antibiotic activity of colistin and their interactions were studied by ion mobility, mass spectrometry, and bacterium growth assays. The results show that during the association of colistin and heparin, they retain their structure while higher-stoichiometry complexes can form. When long-chain heparin is co-administered, multiple colistin molecules can associate with it, which increases the antibiotic activity by ∼40% relative to the sole administration of colistin.
1 Introduction
Antibiotics are in the center of attention in biosciences due to the ever-increasing occurrence of multi-drug resistant bacteria. Based on a recent study, there were nearly five million cases of antimicrobial-resistance-associated deaths in the world within 1 year, where four out of the six deadliest pathogens were Gram-negative bacteria (Murray et al., 2022). In these bacteria, there is a thin peptidoglycan layer between an inner (cytoplasmic) cell membrane and an outer membrane, which makes it more difficult to defeat them with antimicrobial substances.
In the fight against multi-drug resistant bacteria, there are a few so-called “last-resort” molecules (Powers and Trent, 2018; Ahmed, 2021) that are only used in special cases as a result of their strong side effects. However, this allows for one last opportunity to fight off infections from which the patient is otherwise not able to recover on their own. For Gram-negative bacteria, one of these last-resort antibiotics is colistin, or polymyxin E, although certain bacterial strains cultivated from hospitalized patients have already started developing resistance against this molecule as well (Li et al., 2020; Hamel et al., 2021). The uncertainty around the mechanism of colistin’s antibiotic activity has been recently resolved by a study revealing that the low, ∼1% abundance of lipopolysaccharides in the cytoplasmic membrane of Gram-negative bacteria is enough for the interaction with colistin, leading to membrane permeabilization and subsequent lysis (Sabnis et al., 2021). At the same time, its use has been restrained due to its nephrotoxicity (Ordooei Javan et al., 2015). Since the physiological resources of patients needing last-resort antibiotics are often exhausted, it is imperative that drug administration does not overwhelm the organs more than necessary. The most obvious solution is to reduce the dose, but a lower dose leads to lower efficacy and, most likely, to developing resistance against the last-resort drug, too. Therefore, potential solutions include the co-administration of synergistically acting molecules, which in turn also leads to lower doses of the primary antibacterial drug.
In this study, the interaction of colistin with different heparin derivatives, i.e., unfractionated heparin, low-molecular-weight heparin, and a synthetic analogue, fondaparinux was studied. Heparin is an anti-coagulant, and its derivatives are often administered in hospitalized patients (Greinacher et al., 1995; Petitou et al., 2003; Thomas et al., 2015; Karlsson et al., 2021). Furthermore, the role of heparin in the treatment of septic patients is often highlighted, although the understanding of its utility as an antiseptic is still under discussion (Umemura et al., 2016; Li and Ma, 2017; Meziani et al., 2017; Scarlatescu et al., 2017). Heparin is a polyanion, and it is therefore expected to interact with positively charged functional groups, such as the amino group in the diaminobutyric acid residues within colistin. In the studies conducted here, samples of colistin and Kdo2-lipid A, a model lipopolysaccharide with steady concentration were prepared with increasing fondaparinux (i.e., a heparin analogue) concentration. The results indicated that within the clinically administered concentration ranges for each heparin derivative, all of them can increase the efficacy of colistin, with longer chains leading to a 40% higher bacterium growth inhibition than colistin alone in Escherichia coli.
2 Materials and methods
In every experiment, Milli-Q (18.2 MΩ cm) water was used. Colistin sulfate, fondaparinux, Kdo2-lipid A, ammonium acetate (NH4OAc, 7 M), and tetraethylene glycol monooctyl ether (C8E4) were purchased from Sigma. The enoxaparin sample was prepared from a European Pharmacopoeia reference standard. The unfractionated heparin (UFH), lysogeny broth (LB) medium and LB agar plates were obtained from Roth, while glycerol from Thermo Scientific was used. The E. coli K12 AR3110 strain stock was kindly provided by Dr. Chuanxiong Nie (FU Berlin).
2.1 Ion mobility-mass spectrometry studies
2.1.1 Sample preparation
Fondaparinux was used in the mass spectrometry-based studies as a synthetic heparin analog, as enoxaparin and UFH are highly heterodisperse, which prevents their gas-phase study without elaborate purification and separation steps prior to the experiments. The sample was desalted prior to the experiments by using a 5 mL HiTrap desalting column (Cytiva, 1 mL/min flow rate) in a Knauer FPLC. Colistin and fondaparinux aqueous stock solutions of 50 µM were prepared in 250 mM NH4OAc. The 50 µM Kdo2-lipid A stock solution was prepared by dissolving it in 250 mM NH4OAc supplemented with 0.5% C8E4, i.e., at 2x the critical micelle concentration (Landreh et al., 2017).
2.1.2 Ion mobility studies
The stock solutions without further dilution were loaded onto Pd/Pt coated nanoelectrospray capillaries manufactured in-house. A Bruker timsTOF Pro equipped with an electrospray ion source (3500 V source voltage) was used to collect ion mobility data. In this instrument during trapped ion mobility spectroscopy (TIMS), ions are propelled through the mobility cell by a N2 flow. When an electrical field is applied within the cell, ions are separated and stopped at a position (defined by the ions’ respective mobility) where the force of the electrical field counteracts the gas flow’s push on the different ions. When the electrical field is slowly ramped down, ions are sequentially released from the mobility cell based on their inverse reduced ion mobility (1/K0). Each data set was calibrated with the ESI-L Low Concentration Tune Mix (Agilent) to yield collision cross section distributions.
2.1.3 Mass spectrometry-based competition studies
Mass spectrometry was used to gain semi-quantitative information of the competition between Kdo2-lipid A and fondaparinux for colistin. Equal amounts (24 µL) of 50 µM colistin in 250 mM NH4OAc with 0.5% C8E4, 50 µM Kdo2-lipid A stock, and 0–200 µM fondaparinux stocks were mixed together, yielding seven equidistant points on the fondaparinux concentration axis (0, 11.1, 22.2, 33.3, 44.4, 55.6, and 66.7 µM). The samples were introduced into a Bruker timsTOF Pro by a syringe pump connected to an electrospray source (3500 V source voltage). The fixed distance and diameter of the sample capillary allows for the precise observation of tendencies in the ion signal intensities as a function of fondaparinux concentration. Six data sets were recorded at each concentration for reproducibility.
2.2 Bacterium growth studies
Pre-cultures of E. coli K12 AR3110 were made by mixing a bacterial suspension of OD600 = 1.0 with equal volume of 50% sterile aqueous glycerol solution, and 1 mL aliquots were snap-frozen in liquid nitrogen. Before the experiments, a pre-culture aliquot was thawn in a heating block at 30°C over 3.5 min, 200 μL of the pre-culture was added to 9.8 mL of LB medium, and the suspension was thoroughly mixed by inversion and vortexing. 540 μL of this bacterial stock suspension was transferred to prelabelled 1.5 mL reaction tubes. After 12.5 min, 30 µL of the 20x stock solutions of colistin and the respective heparin derivatives (prepared in LB medium) were added to the 540 µL bacterial suspension. After adding all substances, the bacterial suspension was mixed by inverting three times before transferring 4 × 100 µL of the bacterial-substance mixture onto a 96-well plate for technical replicates. 200 μL of water was pipetted in each of the outer wells, which were not used for samples, to maintain the humidity level in the system. The lid was placed on top and the 96-well plate was sealed with Parafilm®. 52 min after thawing the pre-culture, the 96-well plate was placed in a preheated 37°C plate reader (Tecan Infinite). The measurement parameters for the plate reader were: 894 s orbital shaking at 2.5 mm deflection (244.5 rpm), 3 s settle time, five consecutive flashes at 600 nm.
The heparin derivatives (fondaparinux, enoxaparin, and UFH) and colistin were added at physiologically relevant concentrations: the former at 50% and 100% at the maximum clinical blood levels (1.07 μg/mL, 10 μg/mL, and 5.18 μg/mL for fondaparinux, enoxaparin, and unfractionated heparin, respectively, at 100%) (Sule et al., 2009), while the latter at 50% of its minimum inhibitory concentration (0.5 μg/mL) (Morales et al., 2012). The experiments were repeated with a second E. coli culture from the same strain on the next day to account for culture-to-culture variation.
3 Results and discussion
Colistin is an antibiotic that targets lipopolysaccharides in the bacterial cell membranes (Sabnis et al., 2021). However, lipopolysaccharides are much too diverse biomolecules for standard interaction studies; therefore, Kdo2-lipid A, a lipopolysaccharide model was used in the ion mobility-mass spectrometry experiments. To understand the conformational diversity of the in vacuo complexes, the collision cross section distribution curves of the pure substances were recorded first (Figure 1). Colistin (1+) and Kdo2-lipid A (2-) show single peaks in their collision cross section distribution, while fondaparinux (1-), a homogeneous synthetic heparin analogue, appears in two distinct gas-phase conformers with minute collision cross section difference. Therefore, it can be expected that in the colistin-Kdo2-lipid A and the colistin-fondaparinux complexes, distinct populations are separated based on binding sites in the individual molecules and not based on their conformations.
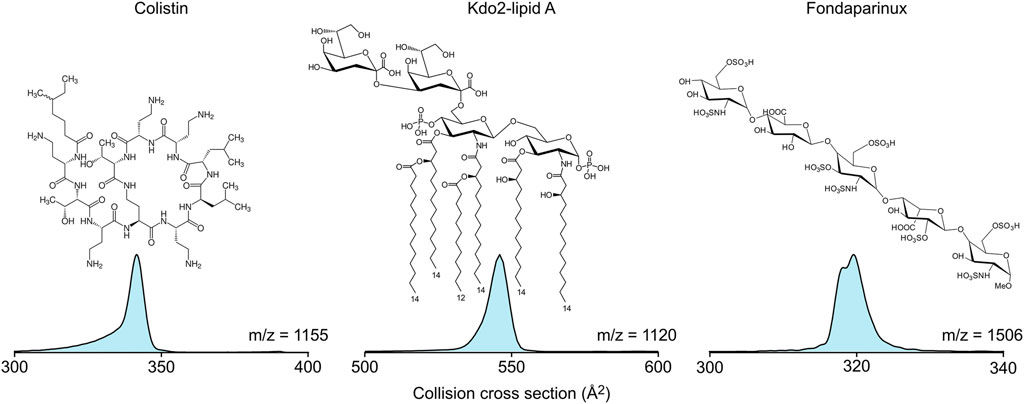
FIGURE 1. The molecular structure and collision cross section distributions of colistin, Kdo2-lipid A, and fondaparinux in their 1+, 2-, and 1- charge states, respectively.
In Figure 2, the collision cross section distribution curves of the colistin-Kdo2-lipid A (m/z = 1,698, 2+) and the colistin-fondaparinux complex (m/z = 1,332, 2+) are shown. Due to the amino groups in colistin, the phosphate groups in Kdo2-lipid A, and the carboxylic- and sulfate groups in fondaparinux, it is expected that the forces stabilizing their complexes rely strongly on electrostatic interactions. The difference between the two complexes is well visible. While there are several distinct populations within the colistin-Kdo2-lipid A complex conformations, the colistin-fondaparinux complex only has a few components. Collision cross sections can yield insights into the nature of interaction specificity as well, as also seen in the isotropic growth of collision cross section in the case of amyloid peptides (Bleiholder et al., 2011). Even though, the association of these molecules is not homodimeric as in the case of amyloid peptides, analogous conclusions can be drawn.
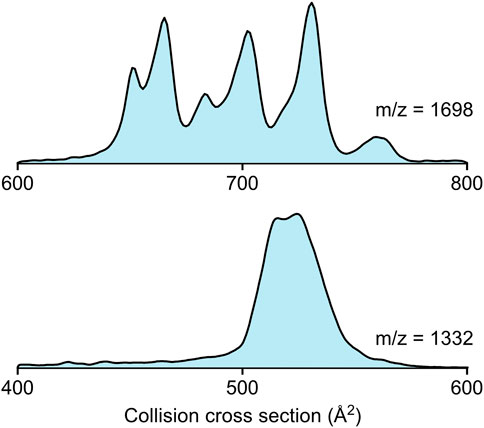
FIGURE 2. The collision cross section distributions of the colistin-Kdo2-lipid A complex (m/z = 1,698, 2+) and the colistin-fondaparinux complex (m/z = 1,332, 2+).
In the case of colistin-Kdo2-lipid A interaction, the collision cross sections extend over a range of ∼100 Å2, which suggests that while certain conformations may not be functional, others are formed based on specific interactions. These specific interactions are crucial for altering the environment in the bacterial cell membrane and eventually for achieving cell lysis. The higher number of complex conformations can be attributed to probable interactions that are different from electrostatic attraction, such as those between the lipid tails and the hydrophobic regions within the colistin molecule. However, it is worth considering that under physiological conditions, colistin interactions with fondaparinux are not hindered sterically as they both flow freely in the blood stream, while Kdo2-lipid A is embedded in the bacterial membrane, which may lead to a significant reduction of complex geometries as opposed to those observed in the gas phase.
In the case of colistin and fondaparinux, the two molecules are very close to each other in collision cross section (342 Å2 and 319 Å2, respectively), and thus the calculation of the isotropic growth (Bleiholder et al., 2011) based on their average collision cross section can give a good estimate on structural alterations. The isotropic growth based on non-specific association would lead to a collision cross section of 525 Å2. The two peaks in the colistin-fondaparinux complex collision cross section distribution (Figure 2 bottom) are at 515 Å2 and 525 Å2, respectively (although, more components are expected to construct the curve), which means that the colistin-fondaparinux interaction leads to complexes that preserve the original structure of the molecules, or only slightly alter them. These findings are promising in the consideration of heparin derivatives as carriers of antibiotic substances, since the molecular structures remain intact.
To understand the competition between Kdo2-lipid A and fondaparinux for colistin, mass spectrometric studies were conducted on solutions containing all three substances with increasing fondaparinux concentration. Figure 3A demonstrates the changes in the colistin-fondaparinux complex signal with respect to fondaparinux concentration. An increasing trend emerges into a plateau as fondaparinux becomes more prominent in the system. It is important to note that these results are semi-quantitative only: the system was only stable in a micelle solution, which can significantly alter the transport efficiency and signal intensity of complex ions. This is due to the harsher conditions (i.e., gas-phase activation) needed to help the complexes escape from the micelles, which can have a deterministic effect on the number of intact complexes.
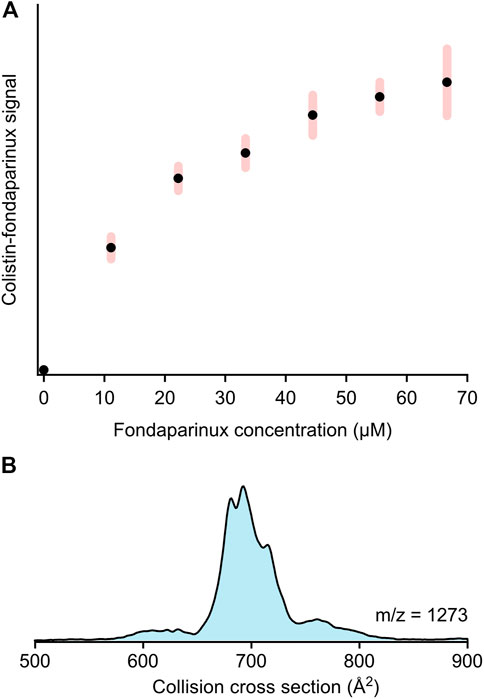
FIGURE 3. (A) The signal intensity trend of the colistin-fondaparinux complex (m/z = 1,332, 2+) with increasing fondaparinux concentration as derived from mass spectrometry-based titration experiments (presented in arbitrary units). The error bars indicate standard deviation (N = 6). (B) The collision cross section distribution of the 2:1 colistin:fondaparinux complex (m/z = 1,273, 3+), indicating the possibility of higher-stoichiometry complexes built up by these molecules.
While the colistin-fondaparinux signal reached a plateau, the signal of free colistin was still significantly higher than that of the complex, and only experienced a slight decrease at high fondaparinux concentrations. The nonlinear concentration dependency of the colistin-fondaparinux signal suggests that higher-stoichiometry complexes are also possible. Complexes containing more than one fondaparinux molecule could not be isolated; although, their existence could still not be ruled out as these higher-stoichiometry complexes could eventually dissociate under the activating conditions. Meanwhile, the 2:1 colistin:fondaparinux complex was detected (Figure 3B), which implies that heparin and its derivatives can potentially concentrate colistin at the bacterial cell membrane if they interact with it.
To understand how heparin affects the antibiotic efficacy of colistin, bacterial growth studies were conducted, and the OD600 values were recorded over several cell cycles. The results of the growth studies in the presence of fondaparinux, enoxaparin, and unfractionated heparin at 50% of the maximum clinically administered blood concentration are demonstrated in Figure 4. The reason behind using clinical blood concentrations instead of matching the mass concentrations is that each of these molecules has an approved concentration range within which they are most effective without any adverse effects, such as heparin-induced thrombocytopenia. The positive control (light gray trace) shows that the culture is sensitive to colistin, and the minimum inhibitory concentration (MIC) results discussed in the literature (1.0 μg/mL) are effective in the case of this strain as well (Morales et al., 2012).
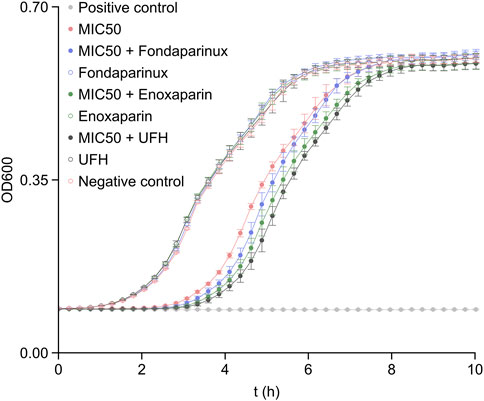
FIGURE 4. The OD600 values recorded in bacterium growth assays with the heparin derivatives being present at 50% of their maximum clinically administered blood concentration (Sule et al., 2009). The error bars indicate standard deviation (N = 8). MIC50 denotes the presence of colistin in 0.5 μg/mL concentration, i.e., at 50% of its MIC (Morales et al., 2012). The positive control was recorded on samples with 1.0 μg/mL colistin concentration (MIC) and the negative control was recorded on samples without colistin and heparin derivatives.
The effect of heparin derivatives on the bacterium cultures was also evaluated as negative control (empty markers). The results indicate that the bacterium strain was not sensitive to heparin derivatives at either of the concentrations (50% or 100% clinically administered levels, the latter shown in Supplementary Figure S1). Therefore, the bacterium strain was suitable for further studies, as the changes in growth rate could not be biased by the heparin derivatives alone.
As visible in Figure 4, each heparin derivative had a positive effect on colistin efficacy against E. coli. Based on the results and the administered mass concentration of the respective heparin derivatives, it is clear that the most deterministic effect on colistin efficacy was caused by the chain length of the heparin derivatives. In fact, increasing their concentration reduced the overall increase in efficacy (Supplementary Figure S1), since the colistin:heparin derivative molecule ratios decreased.
The time-dependent growth-rate inhibition (GRI(t)) was calculated over several cell cycles based on the following equation:
where
The GRI(t) results are shown in Figure 5A. Each heparin derivative at both selected concentrations increases the GRI compared to pure colistin. In the case of fondaparinux (dp5, where dp stands for degree of polymerization, i.e., chain length) and enoxaparin (up to ∼ dp14), the two different concentrations yield very similar growth rate inhibitions (although, the exponential nature of the function must be noted). In comparison, UFH with much longer chains on average than the other two substances shows a significant increase in supporting the antibiotic efficacy of colistin at 50% of its maximum concentration. Therefore, it appears that a higher colistin:heparin chain ratio is more desirable for increasing colistin efficacy. In the mass spectrometry experiments, the 2:1 colistin:fondaparinux complex could be isolated (Figure 3B), leading to the conclusion that higher-stoichiometry complexes exist. With longer heparin chains, multiple colistin molecules are expected to associate with a single chain. Consequently, the colistin:heparin ratio could significantly decrease due to kinetics considerations when twice as many heparin chains are available for interaction. This further solidifies the conclusion that longer heparin chains increase the positive influence on colistin efficacy. If a colistin molecule associated with a heparin chain interacts with the bacterial membrane, there is a higher chance that other colistin molecules complexed with the same chain will also interact with the membrane. As a result, there is an increased probability that at least one colistin molecule reaches a lipopolysaccharide within the cytoplasmic membrane, which leads to the fluidization of the bacterial membrane and subsequent cell lysis (Sabnis et al., 2021). While shorter chains may increase the probability of interaction by a factor of few, long heparin chains can do so by even an order of magnitude.
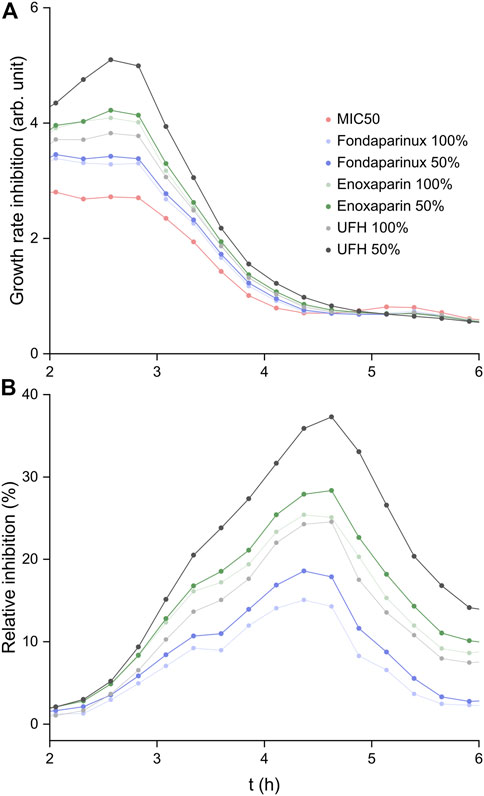
FIGURE 5. (A) The time-dependent growth rate inhibition results calculated based on Hafner et al. (2016). (B) Growth inhibition (%) calculated relative to that of colistin at MIC50.
To understand how heparin derivatives can increase colistin efficacy relative to pure colistin, the relative inhibition percentages (RI) were calculated:
Where
The relative inhibition curves are shown in Figure 5B. In all cases, a relative inhibition of at least 15% was observed at the peak, while administering UFH at 50% of its maximum clinically administered blood concentration led to a ∼40% higher inhibition of E. coli growth compared to colistin alone.
In conclusion, it was demonstrated that heparin derivatives have the potential of increasing the antibiotic efficacy of colistin, a last-resort antibiotic drug. Ion mobility studies showed that the association of colistin and fondaparinux does not lead to significant alterations in the molecule structures, which can be crucial for the antibiotic activity. Mass spectrometric studies showed a plateau in the signal of the colistin-fondaparinux complex, which implies the possibility of complex formation with higher stoichiometries. The 2:1 colistin:fondaparinux complex could be isolated, which confirmed this premise. To understand what effect these interactions have on the antibiotic efficacy of colistin, E. coli growth assays were carried out with three different heparin derivatives: fondaparinux, enoxaparin, and UFH. The bacterial studies showed that longer chains and optimal heparin concentration within the clinically relevant range can largely contribute to the antibiotic activity of colistin. This led to the conclusion that long heparin chains associated with numerous colistin molecules can concentrate the antibiotic substance at the bacterial cell membrane, leading to a much higher probability of cell lysis than colistin alone. This increased the relative inhibition by ∼40% within the cultures when colistin was co-administered with UFH at 50% of its maximum clinically administered blood concentration. As colistin is a last-resort drug that also carries the potential of higher risk of nephrotoxicity, the possibility of reducing the administered amount has serious implications in clinical treatment courses and in the fight against multi-drug-resistant Gram-negative bacteria.
Data availability statement
The raw data supporting the conclusions of this article will be made available by the authors, without undue reservation.
Author contributions
Project plan: GS; experiment plan: GS, DL, and KP, experiment execution: GS, EH, RD, JH, LP, and AZ.; all authors participated in writing the manuscript. All authors contributed to the article and approved the submitted version.
Funding
GS and KP are grateful for the funding by the European Union’s Horizon 2020 Research and Innovation Programme grant number 899687—HS-SEQ. DL acknowledges funding by the Federal Ministry of Education and Science (BMBF; grant number 13XP5111).
Acknowledgments
We thank for the support from the SupraFAB research building realized with funds from the federal government and the city of Berlin.
Conflict of interest
The authors declare that the research was conducted in the absence of any commercial or financial relationships that could be construed as a potential conflict of interest.
Publisher’s note
All claims expressed in this article are solely those of the authors and do not necessarily represent those of their affiliated organizations, or those of the publisher, the editors and the reviewers. Any product that may be evaluated in this article, or claim that may be made by its manufacturer, is not guaranteed or endorsed by the publisher.
Supplementary material
The Supplementary Material for this article can be found online at: https://www.frontiersin.org/articles/10.3389/frans.2023.1154391/full#supplementary-material
References
Ahmed, M. M. E. (2021). Polymyxins: “Last resort” for MDR and/or XDR gram-negative infections. J. Sci. Res. Med. Biol. Sci. 2, 104–122. doi:10.47631/jsrmbs.v2i3.242
Bleiholder, C., Dupuis, N. F., Wyttenbach, T., and Bowers, M. T. (2011). Ion mobility-mass spectrometry reveals a conformational conversion from random assembly to β-sheet in amyloid fibril formation. Nat. Chem. 3, 172–177. doi:10.1038/nchem.945
Greinacher, A., Alban, S., Dummel, V., Franz, G., and Mueller-Eckhardt, C. (1995). Characterization of the structural requirements for a carbohydrate based anticoagulant with a reduced risk of inducing the immunological type of heparin-associated thrombocytopenia. Thromb. Haemost. 74, 886–892. doi:10.1055/s-0038-1649842
Hafner, M., Niepel, M., Chung, M., and Sorger, P. K. (2016). Growth rate inhibition metrics correct for confounders in measuring sensitivity to cancer drugs. Nat. Methods 13, 521–527. doi:10.1038/nmeth.3853
Hamel, M., Rolain, J.-M., and Baron, S. A. (2021). The history of colistin resistance mechanisms in bacteria: Progress and challenges. Microorganisms 9, 442. doi:10.3390/microorganisms9020442
Karlsson, R., Chopra, P., Joshi, A., Yang, Z., Vakhrushev, S. Y., Clausen, T. M., et al. (2021). Dissecting structure-function of 3-O-sulfated heparin and engineered heparan sulfates. Sci. Adv. 7, 6026. doi:10.1126/sciadv.abl6026
Landreh, M., Costeira-Paulo, J., Gault, J., Marklund, E. G., and Robinson, C. v. (2017). Effects of detergent micelles on lipid binding to proteins in electrospray ionization mass spectrometry. Anal. Chem. 89, 7425–7430. doi:10.1021/acs.analchem.7b00922
Li, B., Yin, F., Zhao, X., Guo, Y., Wang, W., Wang, P., et al. (2020). Colistin resistance gene mcr-1 mediates cell permeability and resistance to hydrophobic antibiotics. Front. Microbiol. 10, 3015. doi:10.3389/fmicb.2019.03015
Li, X., and Ma, X. (2017). The role of heparin in sepsis: Much more than just an anticoagulant. Br. J. Haematol. 179, 389–398. doi:10.1111/bjh.14885
Meziani, F., Gando, S., and Vincent, J. L. (2017). Should all patients with sepsis receive anticoagulation? Yes. Intensive Care Med. 43, 452–454. doi:10.1007/s00134-016-4621-z
Morales, A. S., Fragoso De Araújo, J., de Moura Gomes, V. T., Reis Costa, A. T., Prazeres Rodrigues, D., Porfida Ferreira, T. S., et al. (2012). Colistin resistance in escherichia coli and salmonella enterica strains isolated from Swine in Brazil. Sci. World J. 2012, 109795. doi:10.1100/2012/109795
Murray, C. J., Shunji Ikuta, K., Sharara, F., Swetschinski, L., Robles Aguilar, G., Gray, A., et al. (2022). Global burden of bacterial antimicrobial resistance in 2019: A systematic analysis. Lancet 399, 629–655. doi:10.1016/S0140-6736(21)02724-0
Ordooei Javan, A., Shokouhi, S., and Sahraei, Z. (2015). A review on colistin nephrotoxicity. Eur. J. Clin. Pharmacol. 71, 801–810. doi:10.1007/s00228-015-1865-4
Petitou, M., Casu, B., and Lindahl, U. (2003). 1976-1983, a critical period in the history of heparin: The discovery of the antithrombin binding site. Biochimie 85, 83–89. doi:10.1016/S0300-9084(03)00078-6
Powers, M. J., and Trent, M. S. (2018). Phospholipid retention in the absence of asymmetry strengthens the outer membrane permeability barrier to last-resort antibiotics. Proc. Natl. Acad. Sci. U. S. A. 115, E8518–E8527. doi:10.1073/pnas.1806714115
Sabnis, A., Hagart, K. L. H., Klöckner, A., Becce, M., Evans, L. E., Furniss, R. C. D., et al. (2021). Colistin kills bacteria by targeting lipopolysaccharide in the cytoplasmic membrane. Elife 10, e65836. doi:10.7554/ELIFE.65836
Scarlatescu, E., Tomescu, D., and Arama, S. S. (2017). Anticoagulant therapy in sepsis. The importance of timing. J. Crit. Care Med. 3, 63–69. doi:10.1515/jccm-2017-0011
Sule, A. A., Tay, J. C., and Arul, E. (2009). Effect of enoxaparin on peak and trough levels of antifactor Xa in patients with a creatinine clearance of less than 30 mL/min. Int. J. Angiology 18, 184–186. doi:10.1055/s-0031-1278351
Thomas, O., Lybeck, E., Strandberg, K., Tynngåd, N., and Schött, U. (2015). Monitoring low molecular weight heparins at therapeutic levels: Dose-responses of, and correlations and differences between aPTT, anti-factor Xa and thrombin generation assays. PLoS One 10, e0116835. doi:10.1371/JOURNAL.PONE.0116835
Keywords: glycosaminoglycans, mass spectrometry, heparin, colistin, antibiotic activity, ion mobility spectrometry
Citation: Szekeres GP, Hanozin E, Diehn R, Horlebein J, Polewski L, Zappe A, Lauster D and Pagel K (2023) Heparin increases the antibiotic efficacy of colistin. Front. Anal. Sci. 3:1154391. doi: 10.3389/frans.2023.1154391
Received: 30 January 2023; Accepted: 02 May 2023;
Published: 12 May 2023.
Edited by:
Filip Cuyckens, Janssen Research and Development (Belgium), BelgiumReviewed by:
Mariangela Raimondo, National Institute of Health (ISS), ItalyArpad Varga, University of Szeged, Hungary
Copyright © 2023 Szekeres, Hanozin, Diehn, Horlebein, Polewski, Zappe, Lauster and Pagel. This is an open-access article distributed under the terms of the Creative Commons Attribution License (CC BY). The use, distribution or reproduction in other forums is permitted, provided the original author(s) and the copyright owner(s) are credited and that the original publication in this journal is cited, in accordance with accepted academic practice. No use, distribution or reproduction is permitted which does not comply with these terms.
*Correspondence: Gergo Peter Szekeres, Z3BzemVrZXJlc0BmaGkubXBnLmRl