- Department of Chemistry, Georgia State University, Atlanta, GA, United States
While traditional Chinese medicine (TCM) is considered a valuable resource for drug discovery and form a potential basis for drug development, they also carry substantial safety risks due to adverse drug reactions and a lack of understanding of their mechanisms of action. However, due to their highly complex composition, valid analytical methodologies for analyzing TCMs must be developed and promoted. An extensive search of published research and review of scientific papers implies that the increased efficiency and sensitivity of capillary electrophoresis (CE) has attracted much research attention. This review provides an in-depth assessment of CE applications for TCM analysis published in the open literature in the last decade (2011–2021). Our survey findings showed that capillary zone electrophoresis (CZE) with ultraviolet (UV) detection is a capillary electromigration technique frequently utilized for the efficient separation, identification, and quantitation of various active components in highly complex matrices. Different extraction methods, modifiers to the background electrolyte, preconcentration techniques, and mass spectrometry (MS) detectors are used to enhance CE separation selectivity and TCM sensitivity.
1 Introduction
Traditional Chinese medicine (TCM) is a health and wellness approach that the Chinese have practiced for thousands of years. Since ancient times, TCM has been recognized and is currently used in many countries worldwide for many reasons. The benefits of TCM suggest its potential as a therapeutic option for patients with various diseases. Recently, TCM has attracted increased attention and acceptance due to its better therapeutic effects, lower toxicity, and affordability. Hence, analyzing bioactive components in TCMs must be reviewed and enhanced to promote the development and use of TCMs worldwide (Xu et al., 2021; Leong et al., 2020). As reported by DrugBank, 97 FDA-approved drugs are derived from TCMs (Fang et al., 2020). One example is ephedrine, which is obtained from the TCM herb Ma Huang (Herba Ephedrae) and is now used as an anti-asthmatic drug (Chen et al., 2012). Another example is artemisinin (qinghaosu), derived from the Chinese herb qinghao (Herba Artemisiae annuae), which is now considered the first-line drug for the treatment of malaria (Tu, 2011). Nevertheless, the knowledge of Chinese herbs and their combinations as prescriptions is built upon many years of testing and identifying their clinical efficacy for disease treatment. Therefore, isolating the active ingredients in TCM will allow the dissection of their mechanisms of action, opening new therapeutic possibilities (Harvey et al., 2015; Xu et al., 2019). However, due to their propensity to provide immediate therapeutic outcomes while also carrying considerable safety risks, TCMs have recently been termed “two sides of the coin” (Tu et al., 2021). Also, due to their extensive worldwide, TCM quality issues might lead to medical mishaps in practice. Hence, quality control is critical in TCM.
1.1 Occurrence and structural diversity
TCM contains various bioactive ingredients that may vary among plant species based on their natural sources. Figure 1 lists the chemical structures of compounds that the authors of this review considered to be the most representative of TCM herbs. This list is by no means comprehensive and only shows structurally diverse active components (flavonoids, phenolic acids, saponins, coumarins, anthraquinones, carbohydrates, alkaloids, glycosides, terpenoids, lignans, organic acids, and amino acids) found in TCMs that have been claimed to be helpful in various disorders. For example, the perennial herb, phillygenin, contains lignan and glucoside (e.g., epipinoresinol-4-O-β-glucoside, pinoresinol-4-O-β-glucoside, lariciresinol, pinoresinol, isolariciresinol, and valadinol D), which are often used to alleviate pyrexia, gonorrhea, inflammation, pharyngitis, tonsillitis, ulcers, and other ailments (Liang et al., 2018). More than 30 phenolic acids have been isolated from Danshen and are well known for their antioxidant, antiosteoporosis, anti-atherosclerosis, antidiabetic, antihypertension, antiwrinkle, and neuroprotective effects, as well as potential therapeutic effects against human immunodeficiency virus (Pang et al., 2016). The Magnolia officinalis stem bark used in TCM contains geometric isomers of lignan magnoliol and honokiol, which have long been used to treat thrombotic strokes, typhoid fever, and headaches (Han et al., 2012). Paclitaxel is a diterpenoid pseudoalkaloid isolated from Taxus brevifolia and primarily used to treat tumors (Alves et al., 2018). The roots of Scutellaria baicalensis in TCM have a wide range of pharmacological effects on the neurological system and immunological system, providing liver protection and antitumor, antibacterial, antiviral, and antioxidant effects due to the flavonoid, terpenoid, volatile oil, and polysaccharide active components (Zhao et al., 2019). Recent studies on Cynomorii Herba, which includes flavonoids, organic acids, polysaccharides, triterpenes, steroidal compounds, volatile oil, phenolic acids, steroids, alkaloids, and condensed tannins, have reported a variety of bioactivities, including anticancer, antioxidant, antiaging, antibacterial, and HCV protease and glucosidase inhibitory actions (Jin et al., 2020).
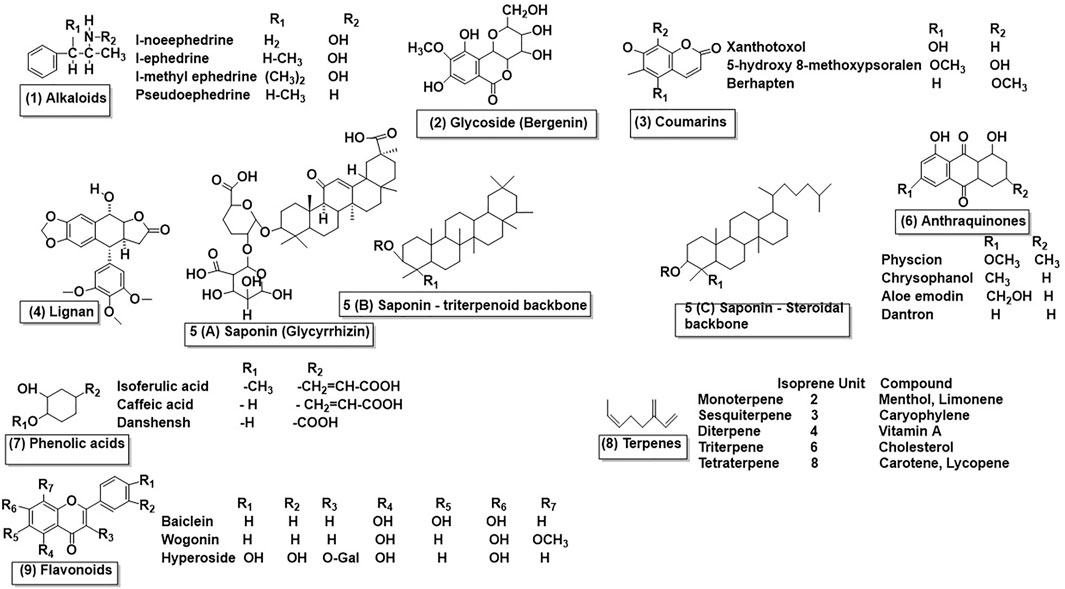
FIGURE 1. Structural classification of the active components of traditional Chinese medicine (TCMs). (1) Alkaloids. (2) Glycoside (bergenin). (3) Coumarins. (4) Lignan. (5) A-Saponin (glycyrrhizin). (5) B-Saponin triterpenoid backbone. (5) C-Saponin steroidal backbone. (6) Anthraquinones. (7) Phenolic acids. (8) Terpenes. (9) Flavonoids.
1.2 Identification of studies on TCM analysis by capillary electrophoresis
Studies in the last decade have examined and reviewed the status of capillary electrophoresis (CE) for the separation, subsequent determination, quantitation, and quality control of TCMs (Table 1). CE and CE-mass spectrometry (MS) have attracted research attention (Ma et al., 2018; Gackowski et al., 2021; Przybylska et al., 2021), as detailed in our search discussed in the following sentences. In particular, CE has played a vital role in TCM analysis as a reliable and sensitive analytical tool; thus, CE is a suitable platform for TCM identification, separation, and quality control. Additionally, CE has gained prominence in recent years due to its high separation efficiency, minimal solvent consumption, small sample volume, low running cost, repeatability, and adaptability of various separation modes. Moreover, CE has recently been classified as an eco-friendly, powerful tool for analyzing different types of active constituents present in TCM, medicinal plants, and natural products used in TCM (Gackowski et al., 2021).
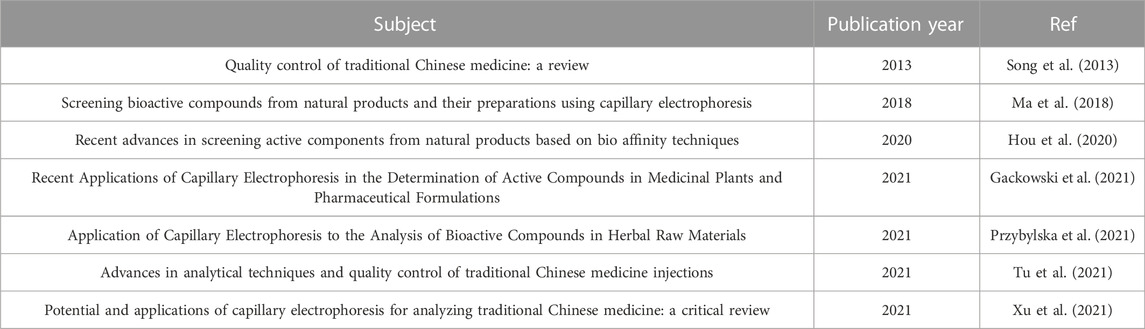
TABLE 1. Reviews published in the last 10 years (2011–2021) on capillary electrophoresis methods for the analysis of TCM.
Supplementary Figure S1 depicts the flowchart for the literature search. Four databases (PubMed, ScienceDirect, SciFinder, and Web of Science) were searched using five search terms (“CE analysis of TCM,” “CE-MS for TCM,” “quality control of TCM,” “hyphenated CE techniques,” and “characterization of TCM by CE”) from 2011 to 2021. The data search identified 1,749 articles from ScienceDirect, 230 articles from PubMed, 250 articles from SciFinder, and 308 articles from Web of Science. The abstracts and the citations of all 2,537 articles were retrieved through EndNote.
The exclusion criteria of duplicate findings; non-English language; reports, abstracts, papers including enzyme inhibition, and papers not meeting the requirements of TCM and CE analysis published in 2011–2021 were removed to finalize the articles of interest. We only included studies that used electromigration techniques like capillary zone electrophoresis (CZE), micellar electrokinetic chromatography (MEKC), and capillary electrochromatography (CEC) coupled with UV-Vis, MS, and other detectors for the analysis of various active constituents of TCM. A total of 20% (n = 518) of articles published before 2011 and the duplicates of publications that represent 8% (n = 197) of articles were removed using EndNote. Additionally, 3% (n = 75) of publications in the Chinese language, abstracts, and reports were not included in this review. Papers describing only TCM without mentioning CE as the analytical technique (25.3%, n = 641) and the application of CE for the analysis of various components other than TCM such as in soil, water, environment, food, plasma, proteins, enzymes, blood serum, urine, and drugs (37%, n = 934) were also eliminated. Finally, 2.6% (n = 67) of articles describing the application of CE for enzyme inhibition were also disqualified from the final selection. After removing all the articles, 98 research articles and seven review papers (a total of 105 articles) published in the last decade based on TCM analysis by CE were selected for inclusion in the review of various active components in TCM using CE. These numbers indicated the considerable research activity in TCM analysis by CE published in 33 journals in the past decade. As illustrated in Figure 2, among 98 research publications on the CE technique for TCM, 21 were published in the Journal of Separation Science, 15 in Electrophoresis, eight in the Journal of Chromatography A, seven in the Journal of Pharmaceutical and Biomedical Analysis, and fewer than five in various journals.
The pie chart shown in Figure 3A shows the percentage of papers published for various types of active TCM ingredients, while the bar plots (Figure 3B) show the trends in the numbers of publications vs the years of publication for the final 98 research articles. The pie chart indicated that alkaloids are the main types of TCM constituents (34%), followed by flavonoids (22%) and polyphenols (16%) as the second most widely analyzed TCM constituents assessed by CE during the past 10 years. Less than 10% of papers were published for all other classes of TCM ingredients, including saponins, coumarins, anthraquinones, glycosides, organic acids, amino acids, and terpenoids. In addition, the bar plots show several noteworthy trends in papers on CZE, MEKC, and CEC techniques for the analysis of TCM published in the last 10 years. First, significant CZE articles were published in all years except in the past 2 years, where a publication drop was noted, possibly due to the coronavirus disease 2019 (COVID-19) pandemic. Second, the trend indicated that CZE and MEKC research articles were published almost every year, while for CEC, only a few articles were published in 2011, 2012, 2016, 2019, and 2020. Third, the number of publications (68) on CZE was significantly higher compared to those for MEKC (25) and CEC (6). This higher rate of CZE publications is due to high separation efficiency, low detection limit, short analysis times, lower injection system demand, and lower complexity of CZE compared to MEKC or CEC. Nevertheless, the latter two modes of CE are still in the developmental stage, which warrants research in developing new and selective pseudostationary phases and columns.
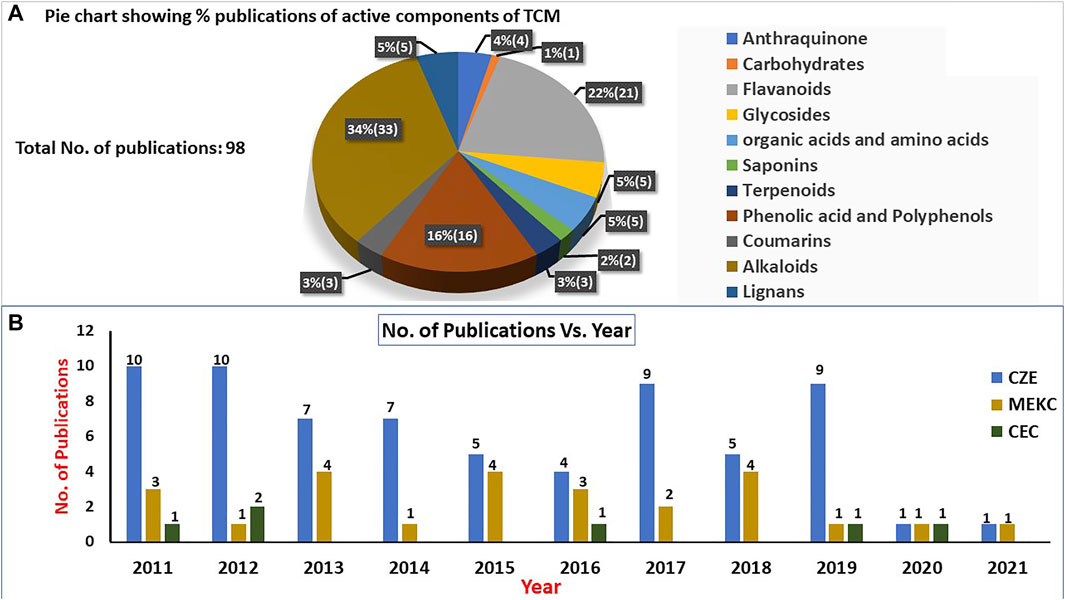
FIGURE 3. Pie chart showing (A) the relative distributions of traditional Chinese medicine (TCM) compounds analyzed by capillary electrophoresis (CE) and bar plot (B) showing the numbers of publications on various modes of capillary electrophoresis (CE) [capillary zone electrophoresis (CZE), micellar electrokinetic chromatography (MEKC), and capillary electrochromatography (CEC)] from 2011 to 2021 for TCM analysis.
1.3 Scope of review
This review aimed to highlight the developments of CE in TCM from 2011 to 2021. The other review articles mentioned in Table 1 do not address the same topic. A recently published review by Xu et al. (2021) in another journal covered the application of CE for TCM analysis but with a limited number of papers analyzing TCM. However, the present review covers CE analysis for all types of active constituents present in TCM, medicinal plants, and current natural products used as TCM. Furthermore, this review offers updates on the CE analysis of TCM over the last 10 years, emphasizing key milestones. This review also compares the applicability of various CE modes with MS and other detection methods. To our knowledge, this is the first comprehensive study to integrate the papers published in the last 10 years on CE for TCM analysis in this journal that also covers the applications of CE.
The flowchart in Supplementary Figure S2 summarizes the recent advances highlighted in this review of the application of CE for analyzing TCMs. Section 2 focuses on the principles of three commonly used modes of CE (CZE, MEKC, and CEC) used for TCM. Section 3 discusses the methods for sample preparation and preconcentration techniques used in the past decade for TCM. Section 4 has several subsections discussing the types of buffer chemistry, micelles, and stationary phases used for CZE, MEKC, and CEC, respectively, to analyze TCM. Section 5 reports the combination of CE with MS and other detection methods. Valuable data are compiled on the type of TCMs separated, sample matrices, BGEs, micelles, and stationary phases used in CZE, MEKC, and CEC in separate tables to make it easier for the reader to search for a specific application. The review ends with a commentary on the overall conclusions and future perspectives on the expected developments in CE and CE-MS for TCM applications in Section 6.
2 Overview of capillary electromigration techniques for TCM
The microscale CE technique primarily identifies, separates, and quantifies various chemical constituents in complex TCM mixtures. Consequently, CE with UV detection has been considered the most advanced technology for the analysis of herbal raw materials in the past 20 years (Przybylska et al., 2021). The typical separation modes of CE utilized for TCM are CZE, MEKC, and CEC, whereas capillary isotachophoresis (CITP) is used as a preconcentration technique.
The CZE mode is the simplest of the CE techniques. In this mode, the analytes are separated inside an open tubular capillary filled with a buffer or electrolyte solution. Therefore, CZE is also called free solution CE. The separation in CZE is based on the differences in migration velocities due to the analyte’s charge, mass, and size, in response to an applied voltage-generating electric field across the capillary. The movement of analyte ions in an electric field is called electrophoretic flow. The electroosmotic flow (EOF) represents a bulk flow of all ions and neutrals in the liquids inside the capillary, even though the migration of buffer cations is the primary driving force for the EOF. The buffer pH is crucial for the charged states of the analytes. Several classes of non-dissociated (uncharged or neutral TCM molecules) are not separated from each other but can migrate through the bulk solution’s movement by EOF and elute at the dead time. Because of its simplicity, CZE-UV is the most extensively utilized mode in TCM. CZE has been primarily used to quantify secondary metabolites in plant extracts and active components in herbal medicines, mainly with UV detection. Although the molecular absorption method of detection has been used to determine most TCM analytes, alternative detection methods such as fluorometric, electrochemical methods (conductometry, amperometry, and potentiometry), chemiluminescence, as well MS methods have also been reported. In CE-MS, an ionization source is present at the cathodic end of the capillary to generate gas-phase ions analyzed by a mass analyzer to generate a mass spectrum, providing information about the mass-to-charge ratio of the ionic species.
Tables 2–Tables 3 list types of TCM tested by CZE. To achieve a good separation of TCM by CZE, multiple variables must be optimized, including capillary type, pH, voltage, injection mode, buffer composition, and concentration, as well as the types and concentrations of buffer additives. The MEKC mode of CE allows for the resolution of neutral and charged molecules and can be used to analyze a wide range of active TCM ingredients, such as flavonoids, in herbal raw materials (Table 4). The separation in MEKC mode is more complex than that in CZE mode because surfactant and/or cyclodextrin are often added to the running buffer in the former mode of CE. Therefore, the MEKC running buffer is reinforced with charged or uncharged surfactants present at higher concentrations than their critical micelle concentration (CMC), resulting in micelle formation. Because of the influence of dispersed surfactants, the micelles form a pseudostationary phase (PSP) that allows the differential separation of analyzed compounds based on the charge-to-mass ratio and hydrophobicity.
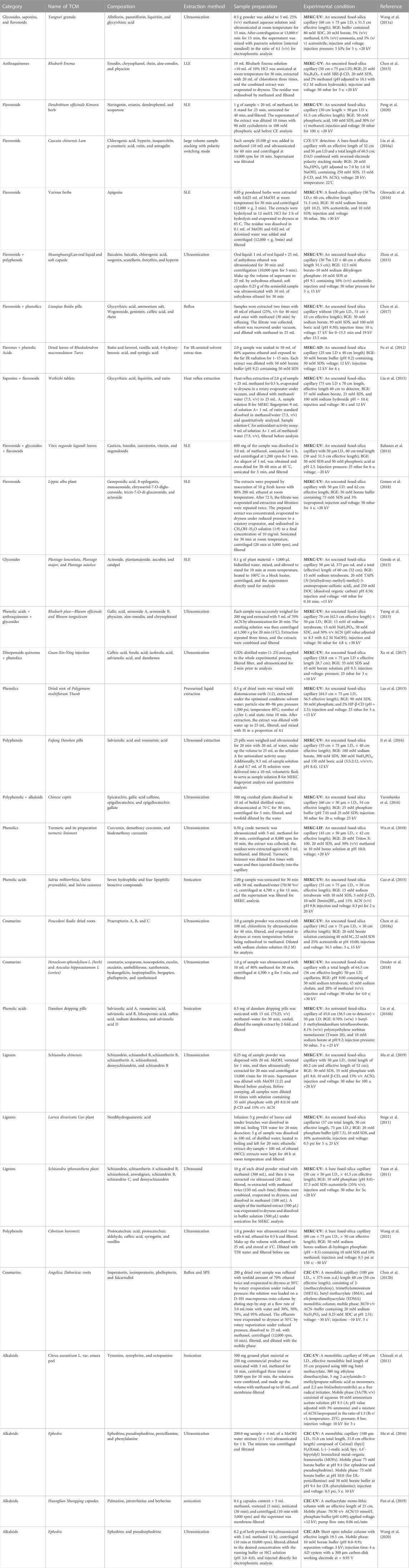
TABLE 2. Application of capillary zone electrophoresis (CZE) for the analysis of various classes of alkaloids, flavonoids, anthraquinones and terpenoids.
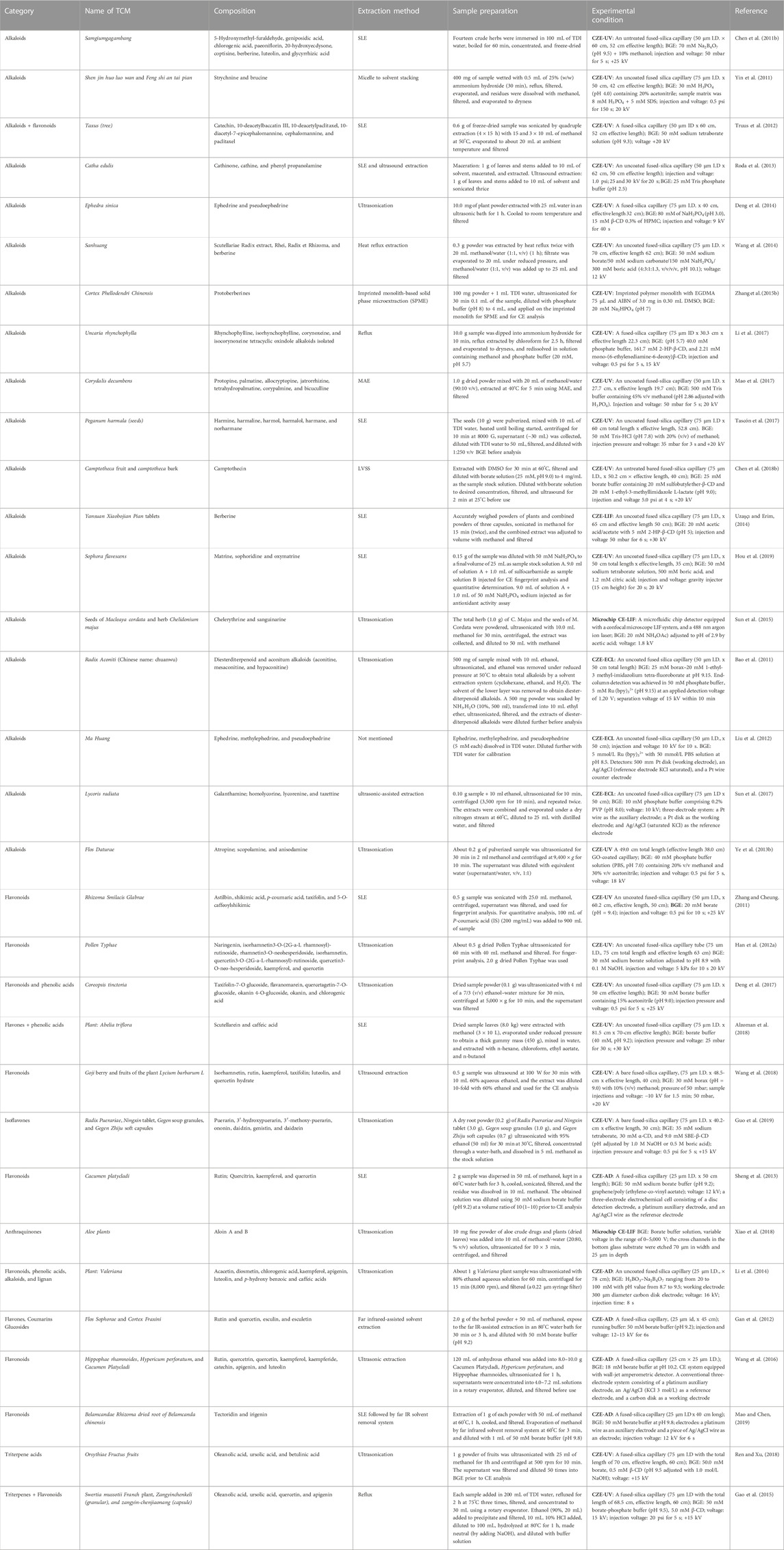
TABLE 3. Application of capillary zone electrophoresis (CZE) for the analysis of various classes of alkaloids, flavonoids, anthraquinones, and terpenoids.
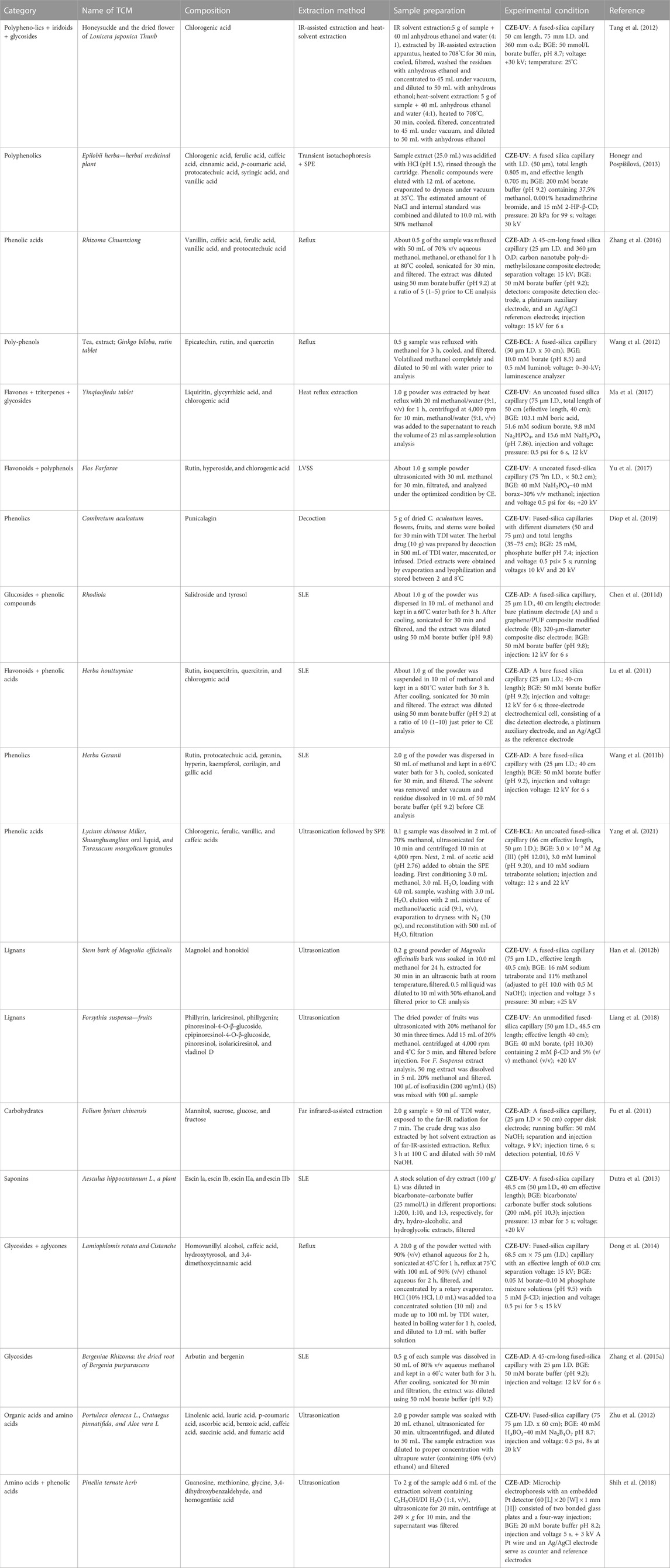
TABLE 4. Application of capillary zone electrophoresis (CZE) for the analysis of polyphenols/phenolics, lignans, glycoside, saponins, organic acids, amino acids, and carbohydrates.
The CEC technique is the third CE mode, which unites aspects of HPLC and CZE to separate charged and neutral analytes. The EOF is used to drive bulk fluid movement in CE. Because CEC incorporates a true stationary phase like HPLC, various methodologies can provide different selectivity beyond what can be achieved with HPLC or CE in a capillary column (coated or packed with particles or a monolith) to generate EOF. Due to its high efficiency, high selectivity, and low sample consumption, the use of CEC has been reported for complex TCM samples (Table 4, rows 27–31). Additionally, the reverse-phase mechanism of HPLC makes this method reliable and adaptable for variable structural classes of TCM compounds, as shown in Figure 1. Moreover, CEC is entirely suitable for pharmaceutical analysis and can replace other less selective techniques for complex mixtures of compounds in TCM (Gackowski et al., 2021).
3 Sample preparation and preconcentration techniques used for TCM
TCM is a complicated sample matrix containing a wide range of active ingredients; thus, sample preparation is critical. Furthermore, TCMs from different sources (plant materials, shrubs, herbs, etc.) contain complex compositions that can interfere with and compromise sensitivity. CE is a specialized technique in separation sciences for the analysis of highly complex TCMs. Its application may be limited due to its poor sensitivity, short optical path, small capillary diameters, and limited sample volume. Preconcentration techniques have been added to CE systems to resolve these concerns. For many TCMs, sample pretreatment is crucial to allow a short analysis time, minimize sample consumption, reduce analysis costs, and improve the limit of detection (LOD). The analysis of active components in the TCM requires drying, making powder, homogenization, mixing with compatible solvents, and centrifugation before extraction and preconcentration. These steps should be ecologically friendly, non-toxic, rapid, and autonomous; however, cost-effectiveness, high sensitivity, and reliability are highly desirable in many situations. Meeting all the aforementioned desired characteristics requires time and effort. Herein, we discuss several essential extraction and preconcentration techniques for various active components in the CE analysis of TCMs. The extraction techniques include solid–liquid extraction (SLE) (Hou et al., 2019), ultrasonication (Guo et al., 2019); solid phase extraction (SPE) (Hou et al., 2019), solid phase microextraction (SPME) (Zhang et al., 2015), liquid–liquid extraction (LLE) (Chen et al., 2015), ultrasound-assisted extraction (UAE) (Wang et al., 2018), far infrared-assisted extraction (FIAE) (Mao and Chen, 2019), and other extraction methods such as matrix-assisted solid-phase dispersion microextraction and pressurized liquid extraction (PLE) (Lao et al., 2013), used for extracting active ingredients from natural origin, herbs, and TCM formulations (Honegr and Pospíšilová, 2013). Furthermore, microwave-assisted extraction (MAE) (Li et al., 2017) and reflux (Gao et al., 2015) have been reported. The preconcentration methods include ITP (Chen et al., 2012), field-amplified sample stacking (FASS) (Wang et al., 2018), field-amplified sample injection (FASI) (Duan et al., 2012), large volume sample stacking (LVSS) (Wang et al., 2021), and micelle to solvent stacking (MSS) (Yin et al., 2011). Some review papers for online sample preconcentration provide more extensive information on the theory and applications, such as FASS and t-ITP (Kitagawa and Otsuka, 2014; Kawai, 2017), sample stacking (Šlampová et al., 2018), and SPE (Dugheri et al., 2021) is recommended. Recently, an outstanding study was published that offered a comprehensive summary of all the major separation approaches investigated for TCM (Jin et al., 2019).
3.1 Solid–liquid extraction (SLE)
A schematic model of SLE shows a simple extraction process in which the solute (i.e., a component of interest) is extracted from a mixture of solids by dissolving it in an appropriate solvent (Supplementary Figure S3). In the first phase, the sample is dried and ground to increase its surface area and, consequently, its extraction rate. Following extraction, filtering separates the soluble chemicals from the solids. The extraction efficiency can be influenced by various factors such as particle size, solid-to-liquid ratio, solvent polarity, time, temperature, and mechanical agitation.
The SLE is the most extensively utilized approach for extracting polar TCM (polyphenols, flavonoids, glycosides, amino acids, and alkaloids) in medicinal plant materials, shrubs, herbs, and TCM formulations employing different polar solvents in the CE analysis of various TCM samples. The common SLE solvents used for TCM sample matrices include water, methanol, sodium dihydrogen phosphate solutions, aqueous and anhydrous ethanol, acetonitrile as pure solvents, and the combination of two or more solvents. Methanol is the most widely used solvent in many publications. Before conducting SLE, TCMs from various complex sources must be processed and ground into fine powders. This simple technique has been utilized to extract alkaloids in Samgiumgagambang (Chen et al., 2011), Taxus (Truus et al., 2012), Catha edulis (Roda et al., 2013), Peganum harmala (Tascón et al., 2017), berberine (Uzaşçı and Erim, 2014), and Sophora flavescens (Hou et al., 2019). Flavonoids and phenolic compounds were also extracted from Abelia triflora (Alzoman et al., 2018), Rhizoma Smilacis Glabrae (Zhang and Cheung, 2011), Rhodiola (Chen et al., 2011), Herba Houttuyniae (Lu et al., 2011), and Herba Geranii (Wang et al., 2011) by simple SLE. Dutra et al. extracted saponins from Aesculus hippocastanum L (Dutra et al., 2013), while Zhang et al. extracted glycosides from the dried root of Bergenia purpurascens (Zhang et al., 2015). SPE was also significant in extracting essential alkaloids and amino acids from Toki, Bukuryo, Sojutsu, Takusha, Shakuyaku, and Senkyu by CE-MS (Iino et al., 2011) and saponins from licorice (Li et al., 2015). Non-aqueous (NA)-CE-MS with SPE preconcentration technique has also been reported for the extraction of alkaloids from radix Stephaniae tetrandrae and rhizomes of Menispermum dauricum (Chen et al., 2013).
3.2 Solid phase extraction (SPE) and solid phase microextraction (SPME)
The fundamental premise of SPE is the transport of targets from the aqueous phase to the active sites of the surrounding solid phase (Zhang et al., 2022). SPE can be used in conjunction with CE in one of four ways: offline (manual SPE), at-line (automatic sample transfer), in-line (SPE integrated into CE), or online (SPE attached to CE via an interface) (Ramautar et al., 2016). Most of the articles in this review reported offline approaches. Offline SPE is executed in cartridges/columns on a bed of stationary phase (sorbents or cartridge) elements on a vacuum manifold. A schematic diagram of SPE is shown in Supplementary Figure S4.
Yang et al. used an ultrasonicated and centrifuged methanolic extract of Taraxacum mongolicum granules as an SPE loading sample eluted with a 2 mL mixture of methanol/acetic acid (9: 1, v/v) for measurement of CE chemiluminescence (CL) (Yang et al., 2021). Zhang and others proposed a unique imprinted SPME technique with a lower limit of detection (LLOD) compared to the conventional SPE technique. The LLOD of 0.1 μg/mL of three common protoberberines was obtained using a molecularly imprinted monolith as the specific sorbent (Zhang et al., 2015; Zhang et al., 2015). The active ingredients (glycyrrhizin and licorice saponin) from the water extract of licorice were isolated and purified by SPE cartridge, while online CE-ESI-MS was used for structure determination (Li et al., 2015).
As a microextraction method, SPME can extract and preconcentrate the analytes of interest from TCMs in a single step, making sampling easier. In addition to C18, numerous commercial SPME coatings are accessible, including polydimethylsiloxane, polyacrylate, and divinylbenzene. However, the minimal extraction capability of these coatings hampers the use of SPME in TCM extraction (Reyes et al., 2018). Previous rebinding studies used ground particles to synthesize two types of berberine-imprinted polymers using a typical polymerization process (Chen et al., 2011). The extraction selectivity was only attained in chloroform and acetonitrile, limiting the use of MIPs. Zhang et al. devised a novel molecularly imprinted SPME with CE to extract and identify protoberberines in complicated herb samples. To overcome this issue, the authors used berberine as a template to create a unique molecularly imprinted monolith in an enhanced pipette tip. In aqueous solutions, this monolith showed excellent selectivity for berberine, with a LOD as low as 0.1 μg/mL, demonstrating the outstanding application of SPME for berberine (Zhang et al., 2015).
3.3 Ultrasonication and ultrasonic assisted extraction (UAE)
In ultrasound and ultrasonic assisted extraction (UAE), the sample is placed in a vessel, the solvent is added to the vessel and ultrasound waves produce mechanical energy to the sample. The tube is then placed in an ultrasonication instrument. The ultrasonic mechanical action and cavitation improve the rate, frequency, and solvent penetration. Figure 4 illustrates a proposed mechanism (Zhari et al., 2020) during herb extraction by UAE. The sample should finally be cleaned up by filtration. The commonly used solvents in ultrasonication include water, methanol, ethanol, and ethyl ether. Ultrasonication and UAE are the most widely used extraction techniques in most publications to extract active components from various TCMs. For example, TCMs such as Chinese coptis (Yaroshenko et al., 2016), Macleaya cordata seeds, the herb Chelidonium majus (Sun et al., 2015), Flos Daturae (Ye et al., 2013a), Pollen Typhae (Han et al., 2012), Cuscuta chinensis Lam (Liu et al., 2016), Coreopsis tinctoria (Deng et al., 2017), Yufeng Ningxin-Radix Puerariae, the dry root of Pueraria lobata, Yufeng Ningxin tablet, Gegen soup granules, Gegen Zhiju soft capsules (Guo et al., 2019), aloe plants and aloe-containing beverages (Xiao et al., 2018), Valeriana (Li et al., 2014), and Forsythiae Fructus fruits (Gan et al., 2012) have all reported ultrasound methods for extraction. Bao et al. compared two extraction methods with ultrasonication following basification to extract aconitum alkaloids from the dried root of Radix Aconiti (Bao et al., 2011). UAE was applied to extract Lycoris radiata alkaloids using the CE-CL technique (Sun et al., 2017). An effective UAE was also quickly adopted in the sample preparation of TCMs with various physicochemical effects, including ultrasonic cavitation, fragmentation, erosion, and shear forces (Vinatoru et al., 2017). This technique was efficiently utilized to extract active components such as Fufang Danshen (Ji et al., 2016), Schisandra sphenanthera (Yuan et al., 2011), Evodiae Fructus (Liu et al., 2019), Fritillariae Thunbergii Bulbus (Wang et al., 2019), Fructus Psoraleae (Zhang and Chen, 2012), Catha edulis (Roda et al., 2013), and Goji berry (Wang et al., 2018).
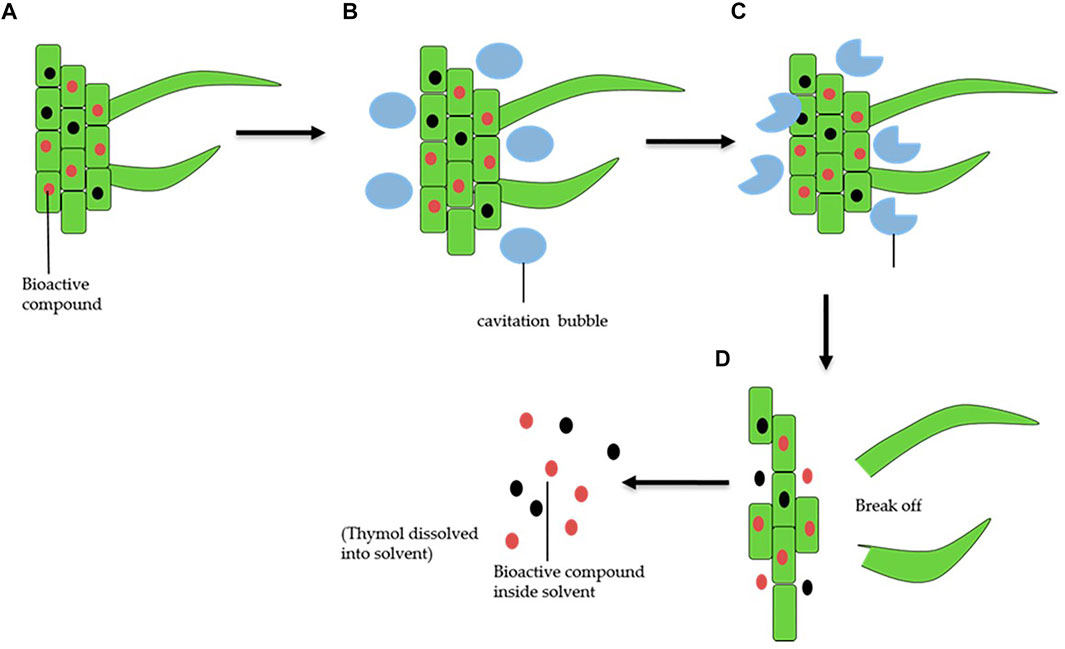
FIGURE 4. Possible mechanism of TCM extraction using ultrasonic assisted extraction (UAE). (A) Image of solid herb particles inside a solvent. (B) Agitation bubble formation. (C) Cavitation bubble breakdown. (D) Release of chemical components into the solvent. Reproduced under CC-BY-4.0 from Zahari et al. (2020).
3.4 Far infrared-assisted extraction (FIAE)
Infrared (IR) radiation is a type of electromagnetic radiation that exists in the electromagnetic spectrum between visible light and microwaves. Fu et al. applied FIAE to isolate flavones and phenolic acids in the dried leaves of Rhododendron mucronulatum Turcz by MEKC with amperometric detection (AD). The extraction duration was significantly decreased to 6 min with FIAE compared to hot solvent extraction (HSE) time of 3 h (Fu et al., 2012). Carbohydrates are abundant in herbal medicines as major water-soluble components. The extraction time was reduced to 7 min with the help of far-IR radiation, compared to 3 h for HSE (Fu et al., 2011). Furthermore, the extraction efficacy of the FIAE method was higher than that of the heat-reflux extraction method (Tang et al., 2012). Mao et al. developed a far IR solvent removal system for the methanolic extract of the dried root of Belamcanda chinensis (Mao and Chen, 2019).
3.5 Isotachophoresis (ITP) and transient ITP (tITP)
The online combination of ITP and CZE offers a high preconcentration capability and improved detection sensitivity. Traditional ITP has poor separation ability due to analyte zone overlap. tITP alleviates this limitation and has been used for sample preconcentration in the CE analysis of TCM (Honegr and Pospíšilová, 2013). According to the Kohlrausch regulatory function, in ITP, the concentrations of the various sample components are regulated by the concentration of the leading electrolyte (LE). All sample components begin to move in isotachophoresis zones. The transient leading ion is propelled at the interface between the LE and the BGE due to the higher electric field strength of BGEs as the electrolyte acts as a terminating electrolyte. A combination of online tITP for sample preconcentration and SPE for pre-cleaning was developed to achieve the desired sensitivity of organic acids in Epilobii herba, an herbal medicinal plant (Honegr and Pospíšilová, 2013). This method allows the BGE co-ion to serve as a terminating ion for induced tITP and also a co-ion for electrophoretic separation. Preconcentration procedures allowed huge plugs of low-concentration samples to be injected without column overloading, resulting in low detection limits and no loss of separation efficiency.
3.6 Large volume sample stacking (LVSS)
To surmount the challenge of low concentration sensitivity in CZE, sample stacking is often used as a practical online preconcentration approach (Yin et al., 2011; Chen et al., 2018a). Several sample stacking approaches have been extensively utilized, including the normal stacking mode (NSM) (Acosta et al., 2014) used in FASS (Kitagawa et al., 2013), pH-mediated stacking (Kong et al., 2017), and LVSS (Kitagawa et al., 2017). Yu et al. noted that LVSS is ideal for detecting trace amounts of materials in complex sample matrices of TCM, with ng/mL detection limits (Yu et al., 2017). Researchers observed that a long sample stacking time resulted in analyte loss. In contrast, insufficient stacking time resulted in the partial elimination of the sample matrix. Thus, optimization of the LVSS stacking and injection times were critical factors to monitor (Wang et al., 2021). Honegr et al. (Honegr and Pospisilova, 2013) coupled LVSS with a polarity-switching approach to improve sensitivity. The sample injection was 50% of the capillary volume, and the polarity was swapped after 1.6 min of analysis. An average 90-fold increase in the absorbance signal of the analytes was achieved under optimal conditions. A similar concept of LVSS with polarity switching mode was used based on the electric field strength disparity between the sample and the BGE zones. The researchers noted that at a reversed polarity of −20 kV, the peak shapes of analytes broadened with reduced peak height. In addition, Yu et al. proposed a CE separation method to evaluate and detect active constituents (chlorogenic acid, rutin, and hyperoside) in Flos Farfarae using an online LVSS method. The authors controlled the analyte volume as low as possible after stacking, which resulted in improved detection limits (Yu et al., 2017).
3.7 Field-amplified sample stacking (FASS) and field-amplified sample injection (FASI)
FASS and FASI are well-known online sample preconcentration techniques in CE and microchip electrophoresis (MCE). FASS is a simple and effective preconcentration approach that requires a BGE with higher conductivity than the hydrodynamically injected sample zone. As a result, analytes penetrate the BGE zones of a low electric field, slow, and condense into narrow bands for increased sensitivity. Deng et al. developed a fast and intuitive CE approach and FASI to obtain a 120-fold increase in detection sensitivity over conventional hydrodynamic sample injection without sacrificing resolution in a poor conductivity solvent plug (Deng et al., 2014). Moreover, to isolate organic acids in various TCMs (such as Portulaca oleracea L., Crataegus pinnatifida, and Aloe vera L.), a field enhancement sample stacking (FESS) and CE separation method was devised. A reverse electrode polarity stacking mode (REPSM) was used as an online preconcentration method in FASS (Zhu et al., 2012). In another study, an online concentration method coupled with FASS with an EOF pump was employed to extract and detect flavonoids in Goji berries (Wang et al., 2018). The FASS approach improves sensitivity and resolution when combined with an online concentration mechanism in CE-MS (Duan et al., 2012).
3.8 Miscellaneous extraction and preconcentration methods
Matrix solid-phase dispersion microextraction (MSPD) is a promising method that involves homogenizing, disrupting, extracting, and cleaning the target analytes from solid, semi-solid, and viscous samples in a single step. Excellent sorbent separation is critical in MSPD because they act as an abrasive substance and a bound sorbent. A practical method based on the MSPD coupled with CE-TOF-MS was developed to extract alkaloid compounds in Fritillariae Thunbergii Bulbus (Wang et al., 2019).
Microwave energy has recently been used to improve the extraction yield of TCMs for analytical purposes. MAE has numerous advantages over traditional extraction procedures, including reduced extraction solvent volume and time and improved extraction competency and sample throughput. Certain active ingredients tend to degrade at higher temperatures. As a result, temperature optimization is a critical factor in MAE. Li et al. developed an MAE technique with an organic solvent to extract Uncaria alkaloids from the stem of Uncaria rhynchophylla (Li et al., 2017). To concurrently quantitate seven alkaloids in Corydalis decumbens, a technique based on MAE followed by CE was developed (Mao et al., 2017).
A simple reflux extraction method combined with CE analysis has tremendous application for the preparation of TCM samples such as Sanhuang (Wang et al., 2014), Uncaria rhynchophylla (Li et al., 2017), Swertia mussotii Franch (Gao et al., 2015), Yinqiaojiedu (Ma et al., 2017), Rhizoma Chuanxiong tea (Zhang et al., 2016), Ginkgo biloba, rutin tablet (Wang et al., 2012), Lamiophlomis rotata, and Cistanche (Dong et al., 2014).
PLE is an excellent extraction process in which increased temperature and pressure improve component solubility and solvent mass transfer, resulting in reduced analysis time and solvent consumption. However, the elevated temperature used in PLE causes some active components in TCMs to degrade. Thus, their thermal stability must be considered (Jin et al., 2019). Lao and others devised a PLE and short-end injection MEKC for the simultaneous detection of seven hydrophilic bioactive compounds in a water extract of He-Shou-Wu. The LOD and LOQ were ≤2.0 μg/mL and ≤5.4 μg/mL, respectively (Lao et al., 2013).
LLE is premised on the idea that an analyte is partitioned between two immiscible aqueous and organic solvents in a specific proportion. The analyte of interest is extracted from interfering substances using a mixture of aqueous and organic solvents. To allow analyte transfer from the aqueous to the organic phase, a small quantity of the aqueous sample containing analytes is mixed with water-insoluble solvents in a centrifuge tube, typically by vortex or sonication. Chen et al. reported this simple technique to extract anthraquinones (emodin, chrysophanol, rhein, aloe-emodin, and physcion) from Rhubarb Enema using chloroform as an extracting solvent (Chen et al., 2015).
MSS is a new online sample preconcentration technique in CE based on the reversal of electrophoretic mobility of micelle-bound analytes at the boundary to isolate the sample and separation solution modified by organic solvent (Quirino, 2009). Micelles in the sample zone transfer the analytes to the MSS zone, which contains an organic solvent, reversing the electrophoretic mobility. Compared to the standard CZE method, MSS achieved a nearly 60-fold increase in concentration sensitivity for strychnine and brucine (Yin et al., 2011).
4 Applications of CZE, MEKC, and CEC for TCM analysis
An extensive literature review of all recently published research and review articles reported that CZE with UV detection is the most frequently utilized approach for separating various active constituents in TCMs and their natural sources. CZE-UV offers only moderate sensitivity, accuracy, low cost, and convenient instrument handling. As only the modest capillary diameter is used in CZE, only a tiny amount of the sample can be introduced to prevent column and detector saturation, resulting in an imprecise peak area. Limited sample quantities can significantly affect the UV sensitivity and precision of CE analysis. Therefore, selecting an alternative detection method is crucial in CE analysis. Although most analytes were measured by UV detector, additional detection methods, such as conductometry, fluorescence, CL, AD, and MS, could enhance the detection sensitivity of highly complex TCMs. CZE, with all the aforementioned detection techniques discussed in this review, has been reported in the analysis of the active components of various TCMs.
4.1 CZE analysis of alkaloids
A key finding of the current systematic review is that CZE with UV detection has the highest number (i.e., 14 out of a total of 19) of articles on the separation and quantitation of alkaloids in TCM (Table 2). Optimized experimental parameters, such as BGE type, concentration, composition, pH, capillary length, and voltage, are crucial for good separation and quantitation of various charged alkaloids. At least five applications in Table 2 reported good separation and quantitation of alkaloids in CZE in 25–50 mM borate buffer in the pH range of 9.5–10.1. Several other buffers such as carbonate, phosphate, acetate, citric acid, boric acid, and a mixture of two or more buffers (e.g., borate and phosphate or borate mixed with citric acid and boric acid) have been used in several applications; however, basic pH conditions for CZE are the most common. Supplementing the running buffer with organic modifiers such as acetonitrile or methanol or combining the aforementioned two organic solvents is a simple and effective way to improve separation efficiency in CE. Additionally, native, derivatized, or charged β-cyclodextrin (CD) has been reported. The number of completely resolved enantiomeric and diastereomeric peaks of ephedrine and pseudoephedrine in Ephedra sinica indicates that β-CD as a chiral modifier improves separation efficiency (Deng et al., 2014). Moreover, Li et al. developed a CZE method for the separation and determination of Uncaria alkaloids from Uncaria rhynchophylla using phosphate buffer with 2-hydroxypropyl (HP)-β-CD) and mono-(6-ethylenediamine-6-deoxy) (ED)-β-CD) as BGE modifiers (Li et al., 2017). This optimized buffer allowed component analysis with detection limits, with quantitation limits ranging from 0.63 to 0.98 μg/mL and from 2.08 to 3.28 μg/mL, respectively. However, Roda et al. used 25 mM Tris phosphate buffer (pH 2.5), which provided an optimal separation of all the cathinones from the leaves and stems of Catha edulis within 6 min of analysis time (Roda et al., 2013).
CE fingerprinting coupled with chemometrics has attracted attention for characterization and quality consistency assessment (Wang et al., 2014). CZE combined with chemometric data processing is an essential tool for exploring the bitterness of various TCM (Yaroshenko et al., 2016). A novel approach of molecularly imprinted solid-phase microextraction coupled to CE (SPME-CE) was devised to discriminate and precisely detect protoberberines in herbs, Cortex Phellodendri Chinensis. Initially, the authors tried the running buffer (20 mM Na2HPO4, pH 7) with an aqueous medium. However, a significant peak tailing was observed. Hence, 10% (v/v) methanol as an organic modifier in the BGE allowed separation within 8.5 min. The detection limit of 0.1 μg/mL was lower than that in a previous CE analysis of protoberberines (Zhang et al., 2015).
For the investigation of berberine alkaloids in Yansuan Xiaobojian Pian tablets, a CE technique with laser-induced fluorescence (LIF) detection was proposed. The fluorescence intensities of berberine were considerably enhanced by the use of 2-HP-β-CD as a complexing agent (Uzaşçı and Erim, 2014). The coupling of MCE and LIF detection not only provided ultrafast separation within 120 s, but the use of LIF detection improved the limit of detection; i.e., 5.0 ng/mL and 2.0 ng/mL for chelerythrine and sanguinarine in Macleaya cordata and Chelidonium majus, respectively (Sun et al., 2015).
Several studies have shown that the CE combined with CL detection can be a valuable tool for the trace analysis of numerous active components in complicated TCM materials, with alkaloid detection limits as low as 10–9 M (Bao et al., 2011; Liu et al., 2012; Sun et al., 2017). Using a unique MSS methodology for online sample preconcentration, this method detected strychnine and brucine at concentrations of 0.02 and 0.05 mg/mL, respectively, in the TCM preparations Feng shi an tai pian and Shen jin huo luo wan (Yin et al., 2011). Chen et al. reported LVSS as an influential online preconcentration method for separating and analyzing camptothecin alkaloids in camptothecin bark and fruit, with detection limits between 0.20 and 0.78 μg/L (Chen et al., 2018b). Graphene and graphene-based materials have recently been used in CE due to their excellent sensing performance. A capillary covered with graphene oxide was used to detect various tropane alkaloids (atropine, scopolamine, and anisodamine) in Flos Daturae, with a detection limit of 0.5 μg/mL (Ye et al., 2013a). The rhizome alkaloids (protopine, corypalmine, palmatine, jatrorrhizine, allocryptopine, tetrahydropalmatine, and bicuculline) of Corydalis decumbens were extracted using a simple and cost-effective MAE method. Using a 500 mM Tris buffer containing 45 %v/v methanol, electrophoretic separation was achieved in 15 min (Mao et al., 2017). Comparably, harmala alkaloids in Peganum harmala seed infusions were separated in <10 min (Tascón et al., 2017). In recent years, CZE fingerprints, in combination with linear quantitative profiling methods, have attracted interest in the quality assessment of highly complex TCM samples (Hou et al., 2019).
4.2 CZE analysis of flavonoids, anthraquinones, and terpenoids
As shown in Table 2, substantial research has been carried out on flavonoids, anthraquinones, and terpenoids by CZE in the last decade. CZE has become a progressively important method for the fingerprint characterization and biomarker determination of complex medicine and natural medicinal materials. For example, CZE is used for quality control and the quantitation of flavonoids in Rhizoma Smilacis Glabrae (Zhang and Cheung, 2011), Pollen Typhae (Han et al., 2012), and Coreopsis tinctoria (Deng et al., 2017) with simple UV detection. CZE-UV provides high specificity for the selective determination of flavanones and phenolic acids such as scutellarein and caffeic acid in Abelia triflora leaves using a simple SLE and 40 mM borate buffer (Alzoman et al., 2018). Other studies have also reported the efficient analysis of flavonoids and isoflavones by CZE-UV analysis using sodium borate as a BGE (Zhang and Cheung, 2011; Deng et al., 2017; Alzoman et al., 2018; Wang et al., 2018).
A rapid and straightforward CE-UV method also has applications for the chiral separation of isomers of triterpene acids (oleanolic acid, ursolic acid, and betulinic acid) by the addition of β-CD (Ren and Xu, 2018). Another literature report noted that CZE-UV with BGE containing 5 mM β-CD as a modifier showed enhanced selectivity for analyzing flavonoids and triterpenes in TCMs (Gao et al., 2015). Recently, a CZE approach coupling ultrasound extraction with FASS was used to separate five flavonoids in reverse polarity mode using only 30 mM borate buffer (Wang et al., 2018). Based on a dual CD system of 30 mM α-CD and 9 mM sulfobutylether β-CD, isoflavonoids were analyzed in several herbal tablets and capsules (Guo et al., 2019) of TCMs with enhanced sensitivity.
Because of the excellent sensitivity, selectivity, and miniaturization of the AD system, researchers have great expectations for its application as a detector for CE. A combination of CE-AD, with FIAE, SLE, and ultrasonic extraction was evaluated by Gan et al. (2012), Sheng et al. (2013), and Wang et al. (2020) for the rapid extraction of rutin and quercetin and other flavonoids with a short analysis time. Li et al. proposed a CE-AD method to inherently exemplify the content of each electrochemically active ingredient (acacetin, diosmetin, chlorogenic acid, kaempferol, apigenin, luteolin, p-hydroxy benzoic, and caffeic acids) in Valeriana plant samples, with detection limits ranging from 0.01 to 0.12 μg/mL (Li et al., 2014). However, Mao et al. showed further improvement in CE-AD by using carbon nanotubes and polylactic acid composite electrodes constructed by melt compounding to detect tectoridin and irigenin in the commonly used Belamcandae Rhizoma TCM (Mao and Chen, 2019). This advanced CE-AD method showed enhanced sensitivity, with LODs of 0.24 and 0.21 µM for tectoridin and irigenin, respectively.
In recent years, more emphasis has been placed on new, superior alternatives utilizing lab-on-a-chip microfluidic technologies. MCE combined with LIF detection showed improved detection limits compared to UV detection. Xiao et al. showed a rapid estimation of two anthraquinones (aloin A and B) in a variety of aloe plants in 40 s with cross-channel microchip CE paired with LIF detection (Xiao et al., 2018).
4.3 CZE analysis of phenolic acids/polyphenols, lignans, and carbohydrates
The detailed experimental conditions for CZE-UV of phenolic acids, phenols, lignans, and carbohydrates in TCMs are listed in Table 3. The efficient CZE-UV detection of polyphenolic compounds such as chlorogenic acid in honeysuckle (dried flower) with 50 mmol/L borate buffer at pH 8.7 allowed the analysis of Lonicera japonica Thunb with a detection limit of 0.05 μg/mL (Tang et al., 2012). However, changes in BGE conditions (37.5% methanol, 0.001% hexadimethrine bromide, and 15 mM 2-hydroxypropyl-β-CD) and negative polarity offered lower detection limits for phenolic acids to the ng/mL level (Honegr and Pospíšilová, 2013). Combining chemical fingerprinting and chemometric techniques is a valuable tool for assessing contents extracted using the heat reflux method from Yinqiaojiedu tablets (Ma et al., 2017). Facile separation was achieved because of the stable complex formed between the borate buffer and the hydroxyl moieties of the phenolic molecules (liquiritin, glycyrrhizic acid, and chlorogenic acid). Diop et al. compared capillaries with different diameters to analyze punicalagin phenolic in Combretum aculeatum (Diop et al., 2019) by CZE-UV with 25 mM phosphate buffer at a pH of 7.4 (with 1 M Tris) as an optimized BGE. A comparison of punicalagin content among the leaves, seeds, and stems using this approach revealed that the leaves had the highest punicalagin content (Diop et al., 2019).
Two studies analyzed lignans after ultrasonic extraction in various types of TCMs. In the first study, two selected lignans (honokiol and magnolol) were quantitated in Magnolia officinalis and Huoxiang Zhengqi by CZE-UV and BGE containing sodium tetraborate with 11% methanol adjusted to pH 10.0 as a modifier within 6 min (Han et al., 2012). In the second report, Liang et al. used 25 mM borate buffer with 2 mM β-CD and 5% methanol as modifiers for the simultaneous determination of eight lignans (phillyrin, lariciresinol, phillygenin, pinoresinol-4-O-β-glucoside, epipinoresinol-4-O-β-glucoside, pinoresinol, isolariciresinol, and vladinol D) in Forsythia suspensa within 15 min (Liang et al., 2018).
Five studies reported the combination of CZE-AD to analyze phenolic acids, glucosides and phenolics, flavonoids and phenolics, and phenolics only in various TCMs. A total of five phenolic constituents (vanillin, vanillic acid, ferulic acid, protocatechuic acid, and caffeic acid) present in Rhizoma Chuanxiong were well separated within 13 min using CZE-AD with a carbon nanotube-polydimethylsiloxane composite electrode (Zhang et al., 2016). The fabrication and use of a unique graphene/poly (methyl methacrylate) composite electrode as a precise CZE-AD detector are gaining popularity because of its unique nanostructure and characteristics. Chen et al. (2011) used this composite electrode to separate a mixture of salidroside and tyrosol within 6 min in Rhodiola, with an improved signal-to-noise ratio. CZE with AD using borate buffer at pH 9.2 and SLE to separate rutin, isoquercitrin, quercitrin, and chlorogenic acid in Herba houttuyniae was reported by Lu et al. (Lu et al., 2011). Wang et al. (2011a; 2011b) separated rutin, protocatechuic acid, geranin, hyperin, kaempferol, corilagin, and gallic acid from Herba Geranii. The efficient separation of four bioactive carbohydrate constituents such as glucose, mannitol, sucrose, and fructose within 18 min in a commonly used TCM, Folium Lysium Chinensis, was reported by Fu et al. (Fu et al., 2011). Using 50 mM NaOH as an electrophoretic separation medium allowed analysis with reduced detection limits ranging from 0.66 to 1.15 μM for all carbohydrate components in the CE-AD.
An online CL detection with CZE provided the selective, ultrasensitive, and rapid detection of polyphenolic acids in Ginkgo biloba extract (Wang et al., 2012). Another CZE-CL study showed detection limits ranging from 0.014 to 0.300 μg/mL for chlorogenic, ferulic, vanillic, and caffeic acids in Lycium chinense Miller, Shuanghuanglian oral liquid, and Taraxacum mongolicum granules (Yang et al., 2021).
4.4 CZE analysis of glycoside, saponins, and organic and amino acids
Several studies have proposed that CZE coupled with UV or AD in alkaline pH is a desirable method for analyzing glycoside, saponins, and organic and amino acids in TCMs and natural medicinal plant materials used as TCMs (Table 3). A BGE mixture of borate and phosphate with 5 mM β-CD as a complexing agent enhanced the separation of glycosides and aglycones (homovanillyl alcohol, caffeic acid, hydroxytyrosol, and 3,4-dimethoxycinnamic acid) in two TCM (Lamiophlomis rotata and Cistanche) (Dong et al., 2014). Saponin β escin from Aesculus hippocastanum was quantified without any matrix interference with high accuracy ranging from 98% to 105% within 6 min using an optimized CZE-UV method (Dutra et al., 2013). A mixture of organic acid and amino acids was separated into three TCM extracts obtained by ultrasonication. To improve the CE-UV detection sensitivity, a suitable FESS method with a reverse electrode polarity-stacking was used for sample preconcentration before separating organic acids to achieve detection limits at the ng/mL level (Zhu et al., 2012).
To achieve favorable sensitivity by CE, Zhang et al. reported a novel approach using a carbon nanotube (CNT)-epoxy composite electrode as an AD for the CE analysis of two glycosides (arbutin and bergenin) in the Bergeniae Rhizoma TCM (Zhang et al., 2015). Both a CNT-epoxy composite and graphite-epoxy composite electrodes were used to assess their effects on the electropherograms of glycosides under optimum conditions. The advantages of the fabricated CNT-epoxy composite electrode included lower detection limits with well-resolved peaks and rapid analysis within 10 min. Compared to the graphite-based electrode, the CNT-based detector provided lower detection limits (0.21 vs 0.77 μM for arbutin and 0.23 vs 0.83 μM for bergenin), respectively. CNT is a promising electrochemical sensing material. In recent decades, miniaturized chemical analysis systems have garnered tremendous interest in TCM analysis due to their unique qualities, including low reagent and sample demand, short analysis time, low power consumption, and portability for in situ applications (Zhao and Chen, 2013; Zhao and Chen, 2014; Tang et al., 2015; Xiao et al., 2018). Shih et al. analyzed guanosine, methionine, glycine, 3,4-dihydroxybenzaldehyde, and homogentisic acid within 5 min in a well-known TCM (Pinellia ternate) by MCE with AD using 20 mM borate buffer as BGE (Shih et al., 2018).
4.5 MEKC analysis of various TCMs
Many active components of TCMs are neutral, and separating elements in TCM with identical structural and physicochemical characteristics is challenging. The traditional CZE method, which focuses on disparities in analyte electrophoretic mobilities, is ineffective for separating neutral components in TCM, as these components move toward the detector at the same rate as the EOF. MEKC is an electrophoretic technique that expands the application of CE to neutral components that cannot be analyzed by free solution CE (Hancu et al., 2013). As discussed in Section 2, MEKC differs from CZE because it uses an ionic micellar solution instead of a simple buffer salt solution. The MEKC mode may differentiate ionic and neutral molecules, whereas CZE is often used to separate ionic components alone. Consequently, when separating compounds, including both ionic and neutral analytes, MEKC has a significant advantage over CZE. The results of the present review showed that MEKC combined with UV detection is the most common detection method for the CE analysis of TCMs compared to other detectors mentioned for CZE. Like CZE, AD and LIF detection interfaced to MEKC are expected to achieve an LLOD for separating analytes in TCM. However, one study has reported TCM evaluation by MEKC with AD, and one study applied the LIF detection method compared to twenty-four reports for MEKC with UV detection (Table 4).
The holistic analysis in Table 4 reveals several vital features. First, sodium dodecyl sulfate (SDS) as a micelle-forming surfactant was reported in most of the MEKC applications of TCM analysis, followed by a few studies using sodium deoxycholate (SDC). Other miscellaneous reagents include ionic liquids (ILs) and non-ionic surfactants used only as additives. The dissolved organic carbon (DOC), which is not an IL, is reported as a PSP but only in one TCM application. Second, in most studies, alkaline buffers (sodium borate, and sodium dihydrogen phosphate), were used in pH ranges of 7.4–10.4. Third, ultrasonication was the predominant method for extracting analytes from various TCM matrices, and hydrodynamic (pressure) injection was used in most MEKC applications, with few reports of electrokinetic injection. Finally, as mentioned previously, UV detection was the most used detector for MEKC of TCMs without any significant preconcentration method reported in this review period.
Three main types of anionic surfactants forming micelles were used for the analysis of TCMs, including SDS, SDC, and sodium cholate (SC). In contrast, only a few reports used cationic butylimmidazolium (BMIM)-IL and only in combination with non-ionic Triton X-100 or Tween 20 surfactant without their single use as a separation media. Additionally, dissolved organic carbon is an interesting application as a PSP. At least four reports described CD modified with MEKC (aka. CD-MEKC); however, in all applications, different types of neutral and derivatized CDs were exclusively combined with SDS.
Four studies reported the use of bile salts such as SDC or SC as PSP for MEKC of TCM. A mixture comprising glycoside, saponin, and flavonoid separated from the Yangwei granule TCM preparation was obtained using MEKC-UV. The electrophoretic buffer system contained 20 mM borate and 80 mM SDC, 5% methanol, and 0.5% ammonia to resolve peaks for all four compounds (albiflorin, paeoniflorin, liquiritin, and glycyrrhizic acid) within 25 min (Wang et al., 2011). The simultaneous separation of six coumarins (coumarin, scoparone, isoscopoletin, esculin, esculetin, and umbelliferone) and six furanocoumarins (xanthotoxin, byakangelicin, isopimpinellin, bergapten, phellopterin, and xanthotoxol) was achieved using 40 mM SC micelle in 50 mM borate buffer, 20% methanol at pH 9.0 (Figure 5A) (Dresler et al., 2018). Typical electropherograms of two types of extracted TCMs (Heracleum sphondylium herb and Aesculus hippocastanum cortex) and standards are shown in Figure 5A–C. Due to the 99.9% similarity of the isoabsorbance plots and comparison of retention times between the compounds contained in the samples (Figures 5B1, C1) and coumarin standards (Figure 5A1), isopimpinellin, bergapten, and phellopterin were identified in the Heracleum sphondylium, while esculin and esculetin were detected in the A. hippocastanum cortex. The MEKC method ensured adequate repeatability (6% RSD for peak area and 2% RSD for migration time), detection limits of 1.8 μg/mL, and excellent resolution in nearly 30 min. Another study reported the separation of five phenolic acids and anthraquinones (gallic acid, sennoside A, sennoside B, physcion, and aloe-emodin) from the Rhubarb plant using a relatively lower concentration of 30 mM SDC in 15 mM Na2B4O7/15 mM NaH2PO4 and 30% ACN v/v at pH 8.3 (Tseng et al., 2013). The metabolite signatures of anthraquinones in the Rhubarb plant were aligned and corrected before chemometric analysis. In addition, the mixed micelles as a pseudophase comprising SDS and SC were introduced for the simultaneous estimation and separation of coumarins such as praeruptorin A, B, and C in Peucedani Radix dried roots using MEKC-UV (Chen et al., 2018a).
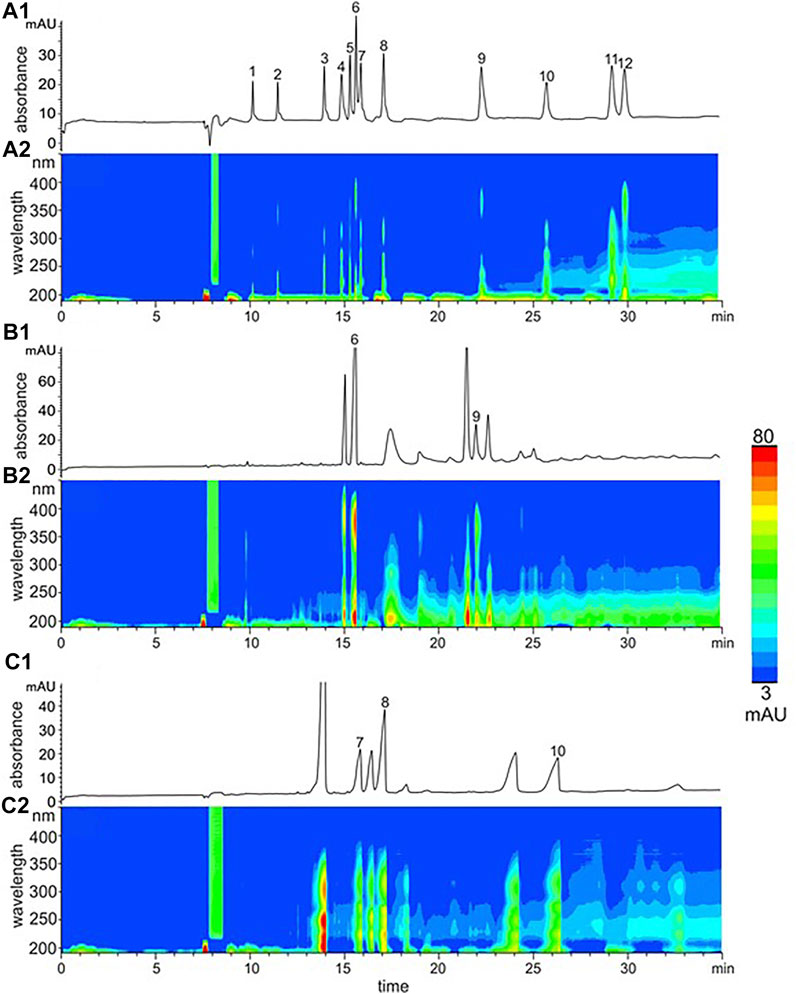
FIGURE 5. Electropherogram at 214 nm and isoabsorbance plot of (A) 12 standard coumarins, (B) a methanolic extract of Aesculus hippocastanum L. cortex, and (C) a methanolic extract of Heracleum sphondylium L. herb. 1-coumarin, 2-scoparone, 3-xanthotoxin, 4-byakangelicin, 5-isoscopoletin, 6-esculin, 7-isopimpinellin, 8-bergapten, 9-esculetin, 10-phellopterin, 11-xanthotoxol, and 12-umbelliferone. Conditions: 50 µm i. d. capillaries with a total length of 64.5 cm (56 cm effective length), capillary temperature of 27°C, voltage of 30 kV, and BGE at pH 9.00 consisting of 50 mM sodium tetraborate, 65 mM SC, and 20% of methanol (v/v). Figure and its caption are reproduced from Dresler et al. (2018), with permission from Elsevier.
The application of PSP in the form of SDS micelles, which is the most used surfactant in MEKC, has provided excellent repeatability of peak areas and retention times of TCMs in both acidic and basic buffers. For example, at least 20 of the 26 applications reported in Table 4 used SDS micelles, with significantly more studies under basic pH buffers compared to acidic pH buffers. Three applications reported the dissolution of the SDS micelles in an acidic phosphate buffer at pH 2.5. In the first application, Peng et al. separated four neutral flavonoids (naringenin, erianin, dendrophenol, and scoparone) from the Dendrobium officinale Kimura herb (Peng et al., 2020) using CD-assisted sweeping MEKC in 50 mM phosphoric acid. The authors screened several types of CDs, including α-CD, methyl-β-CD, 2-HP-β-CD, and γ-CD. Compared to conventional sweeping-MEKC, the 2-HP-γ-CD-modified MEKC showed improved sensitivity, with a detection limit ranging from 13 to 40 ng/mL. Rabanes et al. used SDS (without CD) in the acidic buffer of 50 mM phosphoric acid for the estimation of flavonoid glucoside (casticin, luteolin, isoorientin, vitexin, and negundoside) in Vitex negundo, with LODs of 0.17 μg/mL–0.33 μg/mL, which was sufficient for detection without the need for online or offline sample preconcentration (Rabanes et al., 2014). Several phenolics were separated in the dried root of Polygonum multiflorum Thunb by CD-MEKC using 0.2% 2-HP-β-CD with 90 mM SDS and 50 mM phosphate buffer at pH 2.5 (Lao et al., 2013).
At least twelve recipes for using SDS-only micelles in combination with a basic pH buffer system have been reported for the analysis of TCMs (Table 4). Basic pH buffers such as borate, sodium dihydrogen phosphate, and disodium phosphate are the most popular BGE for SDS dissolution. Flavonoids or mixtures of flavonoids and polyphenols were separated using borate buffer in the pH range of 9.1–10.2. A pH of 10.2, combined with 30 mM borate, 10% acetonitrile (ACN), and only 10 mM SDS was used to extract and characterize apigenin from six different TCM herbs by SLE (Głowacki et al., 2016). Additionally, mixtures of flavanones and phenolics were separated in three separate reports using SDS only. In the first study, Zhou et al. systematically optimized the separation of seven main constituents of TCMs containing flavones and phenolics (baicalein, baicalin, chlorogenic acid, wogonin, scutellarin, forsythin, and hyperin) after studying the effects of buffer pH, electrolyte concentration, organic modifier, and applied voltage (Zhou et al., 2015). The best separation was obtained for 12.5 mM borate–10 mM sodium dihydrogen phosphate–10 mM SDS at pH 9.1 containing 10% (v/v) ACN under 15 kV. The second study applied a triangle method to optimize the concentrations of boric acid, sodium borate, and SDS using the information index, I, as an objective function (Chen et al., 2017). The MEKC conditions of 30 mM sodium borate, 100 mM boric acid, and 95 mM SDS showed the maximum I value of 12.8 and were selected to fingerprint 28 batches of TCM samples. Five marker compounds (glycyrrhizic acid, wogonoside, genistein, caffeic acid, and rhein) were simultaneously quantitatively determined. Principal component analysis revealed that the 28 batches of samples could be clustered according to the TCM manufacturer. Orthogonal partial least-squares regression was used to explore the relationship between the fingerprint and the antioxidant activity. The authors concluded that fingerprint determination by the limited ratio quantified finger method was reliable for monitoring the quality of TCM components in Lianqiao Baidu pills. In a final study, an FIAE system was used to extract two flavanones (rutin and farrerol) and three phenolic acids (vanillic acid, 4-hydroxybenzoic acid, and syringic acid) from the leaves of the Rhododendron mucronulatum Turcz. TCM (Fu et al., 2012). MEKC with AD separated the five compounds in 8 min using 50 mM borate buffer and 50 mM SDS. The LOD was as low as 0.20 μM with a thousand-fold linear range. The results indicated that the FIAE method significantly enhanced the extraction efficiency with a much shorter extraction time of 6 min compared to 3 h for traditional solvent extraction.
Recently, Gomez et al. conducted a comparative study for the simultaneous estimation of three separate classes of metabolites (iridoids, phenylpropanoids, and flavonoids) in three different extracts of Lippia alba leaves between MEKC and HPLC (Gomes et al., 2018). The authors reported that 50 mM borax containing 75 mM SDS and 5% isopropanol were vital for effective metabolite separation by MEKC in 25 min, whereas HPLC separation required at least 60 min (Gomes et al., 2018). Thus, CE was superior to HPLC for the separation and quantitation of polar compounds in plant extracts.
To assess the quality of Guan-Xin-Ning (GXN) injection, MEKC-UV enabled the simultaneous separation and quantitation of seven bioactive quinones, diterpenoids, and phenolics (Xu et al., 2017). A baseline separation of all seven standard components was achieved in 35 min using 45 mM borate and 35 mM SDS (pH 9.3). Good linearity with LOD and a limit of quantitation (LOQ) of 0.40 and 4.90 μg/mL, respectively, were reported. Suitable recovery in the range of 99%–104% suggests that MEKC can be used for quality control of GXN injectables. For example, ten batches of samples were studied, and the fingerprint electropherograms showed 34 common peaks in 20 min.
Two separate studies evaluated polyphenols and a mixture of polyphenols and alkaloids in TCM using SDS micelles exclusively at 300 mM (pH 8.4) and 25 mM (pH 7.0), respectively. In the first study, Ji et al. (2016) showed that 27 peaks could be collected with satisfactory resolution in 33 min. The authors used the optimum BGE of 100 mM sodium borate, 300 mM SDS, 300 mM sodium dihydrogen phosphate, and 150 mM boric acid (5:5:2:12, v/v/v/v) in 22 repeated batches of Fufang Danshen pills, a TCM used to treat heart disease. The second report by Yaroshenko et al. (2016) explored TCM bitterness by comparing MEKC-UV to HPLC-UV and a potentiometric multisensory system for polyphenols and alkaloids. A mixture of four polyphenols and caffeine was separated in 9 min using 25 mM phosphate buffer and 25 mM SDS. All three methods provided satisfactory results in estimating bitterness, with a root mean squared error prediction of 0.9. To optimize the separation of saponins and flavonoids in Weibizhi tablets, Liu et al. (2015) applied MEKC combined with a multivariate optimization approach such as the Box–Behnken design using the resolution index as an integrated response. Additionally, a ratio fingerprint method was established for the comprehensive quality discrimination of TCMs for qualitative and quantitative analysis. The optimized conditions were 57 mM sodium borate, 21 mM SDS and 100 mM NaOH. The quality of 27 samples from the same manufacturer was well differentiated and the relationship between fingerprints and antioxidant activities was established using partial least squares regression.
The efficient separation of lignans in different parts of the medicinal plant Schisandra sphenanthera by MEKC-UV was developed and validated. In optimized experimental conditions containing 35 mM SDS, 10 mM phosphate buffer, and 35% ACN, the LOD and LOQ for the analytes were within the range of 0.4–1.2 μg/L and 1.5–4.0 μg/L, respectively (Yuan et al., 2011). However, it was challenging to screen and measure trace elements in TCMs by MEKC-UV. Furthermore, Stege et al. analyzed nordihydroguaiaretic acid (lignan) in Larrea divaricata Cav. extracts by MEKC-UV using phosphate buffer containing SDS and ACN at pH 7.5 (Stege et al., 2011). In contrast to the HPLC method, CE had a shorter migration time (Ramadoss and Oruganti 2016).
Several studies reported the CD-MEKC method for TCM analysis. A unique combination of LVSS with reversed-electrode polarity stacking mode offered a 10 to 31-fold enhancement in the detection sensitivity of five flavonoids (chlorogenic acid, hyperin, isoquercitrin, p-coumaric acid, rutin, and astragalin) using 15 mM β-CD, 250 mM SDS in phosphate buffer, and 5% ACN (Liu et al., 2016). In another report, anthraquinones in compound Rhubarb Enema (CRE) were extracted and separated using 25 mM Na2B4O7 (pH 10.1), 4 mM SBE-β-CD, 20 mM SDS, and 2% methanol in CD-MEKC. The HPLC and CD-MEKC were compared, with LODs of 0.04–0.08 µgmL−1 and 0.4–0.8 μg mL−1, respectively. The methods were compared for repeatability, precision, calibration range, and recovery and were equally suitable for determining anthraquinones in CRE (Chen et al., 2015). Lao et al. described a unique approach to PLE and short-end injection in CD-MEKC (Lao et al., 2013). Several polar bioactive phenolics (proanthocyanidin B1, proanthocyanidin B2, 2,3,5,4′-tetrahydroxy-stilbene, 2-O-β-D-glucoside, gallic acid, and epicatechin, catechin) were determined in water extracts of the dried roots of Polygonum multiflorum. The lack of time in the short-end injection approach provided poor phenolic separation. However, optimal separation was achieved within 14 min using 50 mM phosphate buffer containing 90 mM SDS and 2% (w/v) 2-HP-β-CD (pH 2.5), 15 kV, and 20°C. Good linearity (R2 = 0.9978) with LOD and LOD <2.0 μg/mL and 5.5 μg/mL, respectively, were reported. Additionally, the authors observed intraday and interday repeatability of 3% and 5%, respectively, with recoveries ranging from 97% to 104%. Ma et al. recently proposed an in-capillary–2,2-azinobis (3-ethylbenzo-thiazoline-6-sulfonic acid)–sweeping MEKC–UV detection method for screening and quantifying seven antioxidants (lignans) in trace amounts from the natural fruit Schisandra chinensis (Ma et al., 2019). A sweeping preconcentration of antioxidants and optimized experimental conditions allowed the trace determination of seven lignans (schizandrin, schisandrol B, schisantherin B, schisantherin A, schisanhenol, deoxyschizandrin, and schisandrin B) under the optimized conditions of 35 mM phosphate buffer (pH 8.0) containing 30 mM SDS, 10 mM β-CD, and 10% v/v can at 28 kV. Compared to previously reported MEKC, the sensitivity enhancement ranged from 17 to 167, with LODs as low as 6 ng/mL, and satisfactory analytical validation parameters (linearity, repeatability, accuracy, and recovery).
ILs, particularly long-chain IL-type surfactants, are recently emerging PSPs for both chiral (Rizvi and Shamsi, 2006) and achiral (Schnee et al., 2006) separations in MEKC. However, short-chain ILs have shown improved selectivity when used in combination with other reagents in CZE. For example, Liu et al. optimized the separation of phenolic acids in Danshen dripping pills with UV detection (Liu et al., 2016). A micelle system containing aqueous mixtures of IL such as BMIM-tetrafluoroborate (BF4) was used in combination with a non-ionic surfactant (Tween 20) in borate buffer. To achieve better resolution, higher peak response, and shorter retention time, an optimization index, RA, was proposed for the comprehensive assessment of resolution. The multivariate optimization indicated that adding [BMIM]BF4 in BGE enhanced the RA for several phenolic acids. In a separate study, Cao et al. could not separate all seven hydrophilic and four lipophilic bioactive component compounds (Table 4, row 19) in three Salvia species using MEKC with SDS (Cao et al., 2015). However, when [BMIM]BF4 and β-CD were added to SDS, all eleven analytes (protocatechuic aldehyde, salvianolic acid, rosmarinic acid, 9″-methyl lithospermate B, danshensu, salvianolic acid B, protocatechuic acid, tanshinones, dihydrotanshinone, cryptotanshinone, and tanshinone II A) achieved baseline separation in 34 min.
DOC is another class of unique PSP used in the analysis of TCMs. A BGE comprising SDS as a surfactant failed to separate glycosides in different Plantago species. DOC is chiral, with micellar properties different from those of SDS. Therefore, a combination of 15 mM borate with 20 mM (N-[tris(hydroxymethyl)-methyl]-3-aminopropanesulfonic acid (TAPS) as a buffer and 250 mM DOC was an effective BGE for the repeatable separation and quantitation of metabolites (glycosides aucubin, catalpol, phenylethanoid glycosides, acteoside (verbascoside), and plantamajoside) in water extracts of different Plantago samples (Gonda et al., 2013).
Recently, the most sensitive MEKC-UV with LVSS method was developed and validated for the simultaneous separation of five polyphenolic compounds (protocatechuic acid, protocatechuic aldehyde, caffeic acid, syringetin, and vanillin) in Cibotium barometz. The detection limits ranged from 32 to 65 pg, which were 12–27 times lower than those for MEKC and 500 times lower than previously reported (Wang et al., 2021).
In addition to UV detection as the most common in MEKC applications of TCM, one report each has described the use of AD and LIF detection, respectively (Table 4, rows 8 and 18, respectively). A unique and sensitive MEKC-AD detection reported the promising separation and analysis of active constituents in TCMs. To isolate phenolic acids (syringic acid, vanillic acid, and 4-hydroxybenzoic acid) and flavones (rutin and farrerol) from the commonly used TCM Rhododendron mucronulatum Turcz, far IR-assisted extraction was applied in combination with MEKC-AD detection (Fu et al., 2012). In recent years, researchers have focused on a novel and unique concept of mixed micelles to enhance the separation efficiency of the analytes. Wu et al. proposed a unique approach combining MEKC with LIF detection to increase the sensitivity of turmeric and its liniment preparation containing phenolic constituents such as curcumin, bisdemethoxy curcumin, and dimethoxy curcumin. The mixed micelles of non-ionic (TX100) and anionic (SDS) micelles enhanced the separation efficiency by sensitizing the native fluorescence of curcuminoids. Satisfactory separation of all three curcuminoids was achieved in 10 min with LOD values ranging from 0.4 to 4.1 ng/mL (Wu et al., 2018).
4.6 CEC analysis of TCM
The development of column technology, including packed, monolithic, and open-tubular columns (OTC), is a promising area of continuous growth in CEC. Over the past several years, the monolithic and OTC have become more prevalent in CEC due to their intrinsic advantages of ease of production and fritless design. OTC allows the use of cutting-edge microporous materials, nanoparticles, and biomaterials as stationary phase elements, providing a wide range of analytical alternatives. However, compared to OTC, monolithic columns have higher efficiency and resolution and have attracted increased attention in recent years (Mao and Chen, 2019).
In the past decade, the application of CEC was reported for only two classes of TCM compounds (alkaloids and coumarins). The CEC research studies for coumarins and alkaloids are described in Table 4 (rows 27–31). A methacrylate-based monolithic column using acrylamido-2-methyl-propane sulfonic acid (AMPS) monomer was prepared by UV photopolymerization to separate three aromatic compounds (tyramine, synephrine, and octopamine) in Citrus aurantium L. var. amara orange peel (Chizzali et al., 2011). The column allowed the separation of these alkaloids in <15 min using an optimized mobile phase mixture comprising 70% isopropanol: ACN (1:3) and 30% aqueous ammonia at pH 9.0. A positively charged monolithic CEC column such as 2-(methacryloyl-oxy)ethyl] trimethylammonium (META) was used in combination with butyl methacrylate (BMA) and ethylene dimethacrylate (EDMA) to rapidly separate five coumarins (byakangelicin, oxypeucedanin hydrate, xanthotoxol, 5-hydroxy-8-methoxypsoralen, and bergapten) in Angelica dahurica extract within 6 min (Chen et al., 2012). An improved separation selectivity of coumarin was obtained when only 0.25 mM SDC surfactant was added to the mobile phase containing phosphate buffer and ACN. Figures 6A–D show the comparison of the separation of five coumarin derivatives using the monolithic CEC column (A), MEKC (B), a pressurized CEC column packed with 3 um ODS (C), and HPLC (D). Among all four chromatographic methods, monolithic CEC provided the fastest run, with baseline resolution, improved peak shapes, and the highest sensitivity.
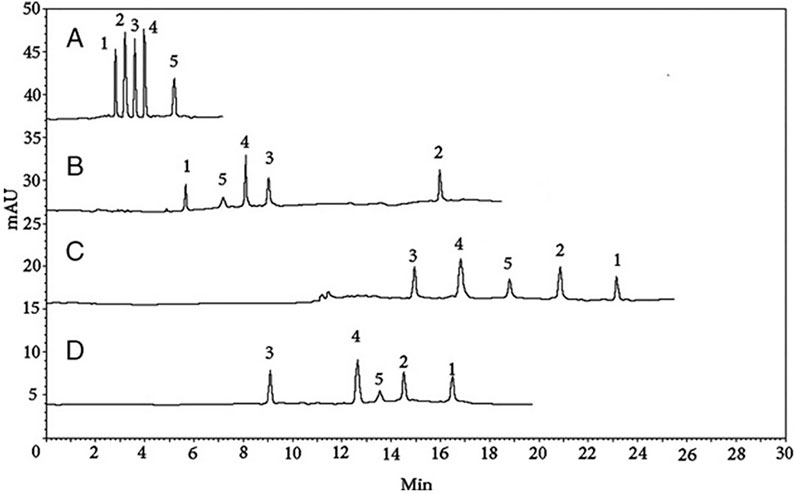
FIGURE 6. Separation by (A) monolithic CEC, (B) MEKC, (C) pCEC, and (D) HPLC. Conditions: (A) as in Figure 4. (B) Mobile phase, ACN/15 mM borax (Na2B4O7)−15 mM NaH2PO4–0.25 mM SDC5: 95, pH 8.59; applied voltage, 20 kV; injection, 10 kV 5 s; wavelength, 210 nm; capillary length, 60 cm (50 cm effective length); column temperature, 25°C. (C) Mobile phase, methanol–ACN–phosphate buffer (pH 4.8; 15 mM) (22.5:15:62.5, v/v/v); flow rate, 50 L/min; voltage, 1 kV; back pressure, 13.8 MPa; injection, 4 nL; detection wavelength, 210 nm; and temperature, 20°C. (D) Column, C18 (200 mm × 4.6 mm, 5 m); mobile phase, ACN-methanol–water–acetic acid (20:15:65:2, v/v/v/v); flow rate of 1.0 mL/min; wavelength, 210 nm. Figure and its caption are reproduced from Chen et al. (2012), with permission from Wiley and Sons.
Nanoparticle-based monoliths have been introduced for the chromatographic separation of various solutes using CEC and HPLC (Chambers et al., 2011; Mayadunne and El Rassi, 2014). Nanoparticles can be included in monolith supports by attaching them to the pore surface or copolymerizing them with the monomers (Pan et al., 2019). However, the latter is the more accessible and more convenient technique. Hence, Pan et al. developed the unique approach of using an epinephrine-functionalized polymethacrylate monolithic column with fumed silica nanoparticles for pressurized CEC-UV detection. The preparation of epinephrine-modified monoliths has been optimized for EOF and column performance. The proposed method showed satisfactory results in separating aromatic compounds and alkaloids (palmatine, jatrorrhizine, and berberine) in Huanglian Shangqing capsules (Pan et al., 2019). A novel and sensitive CEC approach for stereoisomer separation were developed by Ma et al. (2016). The authors suggested that the covalent bonding of homochiral metal–organic framework (MOF) in the capillaries facilitated the quantitative assessment of ephedra alkaloids (ephedrine and pseudoephedrine) in Ephedra. Additionally, this MOF column enantioseparated phenylalanine and penicillamine isomers with partial and baseline resolutions, respectively. This method provided adequate sensitivity, LOD, and LOQs of analytes at 0.1 and 0.25 μg/mL, respectively. Therefore, MOFs can be used as a stationary phase in this approach, expanding the scope of CEC separation in stereoisomer separation and chiral analysis (Ma et al., 2016).
CEC-UV detection can also be replaced by AD. Combining CEC and an AD improved the LOD compared to the UV detector. One recently published study (Wang et al., 2020) demonstrated the extensive application of this eco-friendly technique, including a chiral open-tubular column with bovine zeolite imidazolate framework-8 and serum albumin, for the analysis of ephedrine isomers in the herb Ephedra. Compared to previous approaches for CEC-based determination of ephedra alkaloids (ephedrine and pseudoephedrine) employing OTCs (Ye et al., 2013b; Pan et al., 2016; Zhao et al., 2020), this approach used only 10 mM borate running buffer without the need for extensive buffer additives and a relatively short (19.5 cm) OTC column that provided high detection sensitivity (LOD = 1.5–2.0 ng/mL) in the sample matrix.
5 Capillary electrophoresis-mass spectrometry (CE-MS) for the analysis of TCMs
CE-MS is a novel hyphenated technique that integrates the high-resolution separation of CE with highly sensitive and selective MS detection. It is a powerful method for detecting trace quantities of analytes ranging from micro to macro molecule size (Zhou et al., 2019). A volatile buffer solution is used in this approach to prevent salts from interfering with ionization. The charged analytes can be separated by electrophoretic mobility in the CE column depending on the strength of the applied electric field to the column and the rate of analyte migration. Positively charged analytes move freely toward the anode, while negatively charged analytes move freely toward the cathode. The rates of migration depend on the buffer pH. The capillary is inserted through the nebulizer (sprayer) in the ESI-MS interface, where the analyte is sprayed orthogonal to the electrospray ionization (ESI) source to form gas-phase ions before they enter the mass spectrometer. A schematic of solute migration in CZE-MS under positive polarity using a co-axial sheath flow system is depicted in Figure 7. As shown, under neutral to basic pH conditions, highly mobile cations with a small mass (or largest charge-to-mass ratio, z/m) elute first, followed by low-mobility cations with a small charge-to-mass ratio as they move in the same direction as the EOF. While the neutrals in CZE-UV cannot be distinguished, a CZE-MS electropherogram can be used to detect them. In the CZE buffer solution, neutral compounds will stay unionized; however, if they have an ionizable functional group in the gaseous state, they may ionize in the electrospray. The migratory order of cations, neutrals, and anions based on the mass-to-charge ratio is as follows: small cations < large cations < neutrals < large anions < small anions (Patel et al., 2021). The separation capillary is surrounded by the sheath liquid, which is surrounded by the nebulizer gas (nitrogen). In the co-axial delivery of sheath liquid to the end of the CE column, the separation capillary serves as an outlet electrolyte reservoir to prevent current drop during CE-MS runs without an outlet vial. For CZE with UV detection, buffers such as borate and phosphate are often used. These buffers are non-volatile and cannot be ionized in an ESI source. Therefore, the buffers utilized in CE-ESI-MS must be volatile to be compatible with the ESI procedure and to reduce background noise. Combinations of ammonium acetate (NH4COOH)/formic acid (HCOOH), ammonium formate (NH4COOH)/formic acid (HCOOH) (NH4CH3COO)/acetic acid (CH3COOH), and ammonium hydroxide/-NH4CH3COO are frequently used buffers, which cover the total acidic, neutral, and basic pH range, respectively. Because of its ease of use and ability to ionize many components in complicated matrices, ESI is the most employed ionization source for online CE-MS coupling in TCM analysis. CE-ESI-MS uses two commercially available interfaces: sheath flow and sheathless.
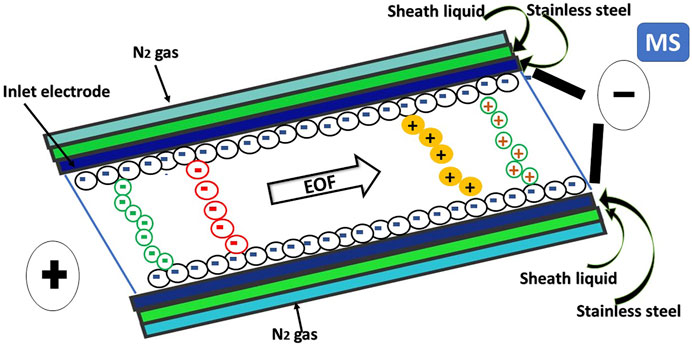
FIGURE 7. Schematic of solute migration in CZE-mass spectrometry (MS) under positive polarity. Reproduced from Patel et al. (2021), with permission from Elsevier.
5.1 Aqueous CE-MS
Compared to UV detection, employing an MS detector may improve the sensitivity and selectivity of TCM analysis (Liu et al., 2019). ESI is the most extensively used ionization source among the many MS ion sources due to its high specificity. To date, at least nine applications of aqueous CE-MS have been reported for TCM analysis (Table 5), eight of which reported CZE-MS, with only one application using CEC-MS and none using MEKC-MS. Furthermore, most of the CE-MS studies used a sheath liquid interface, ESI ionization source, and ion-trap mass spectrometer (IT-MS).
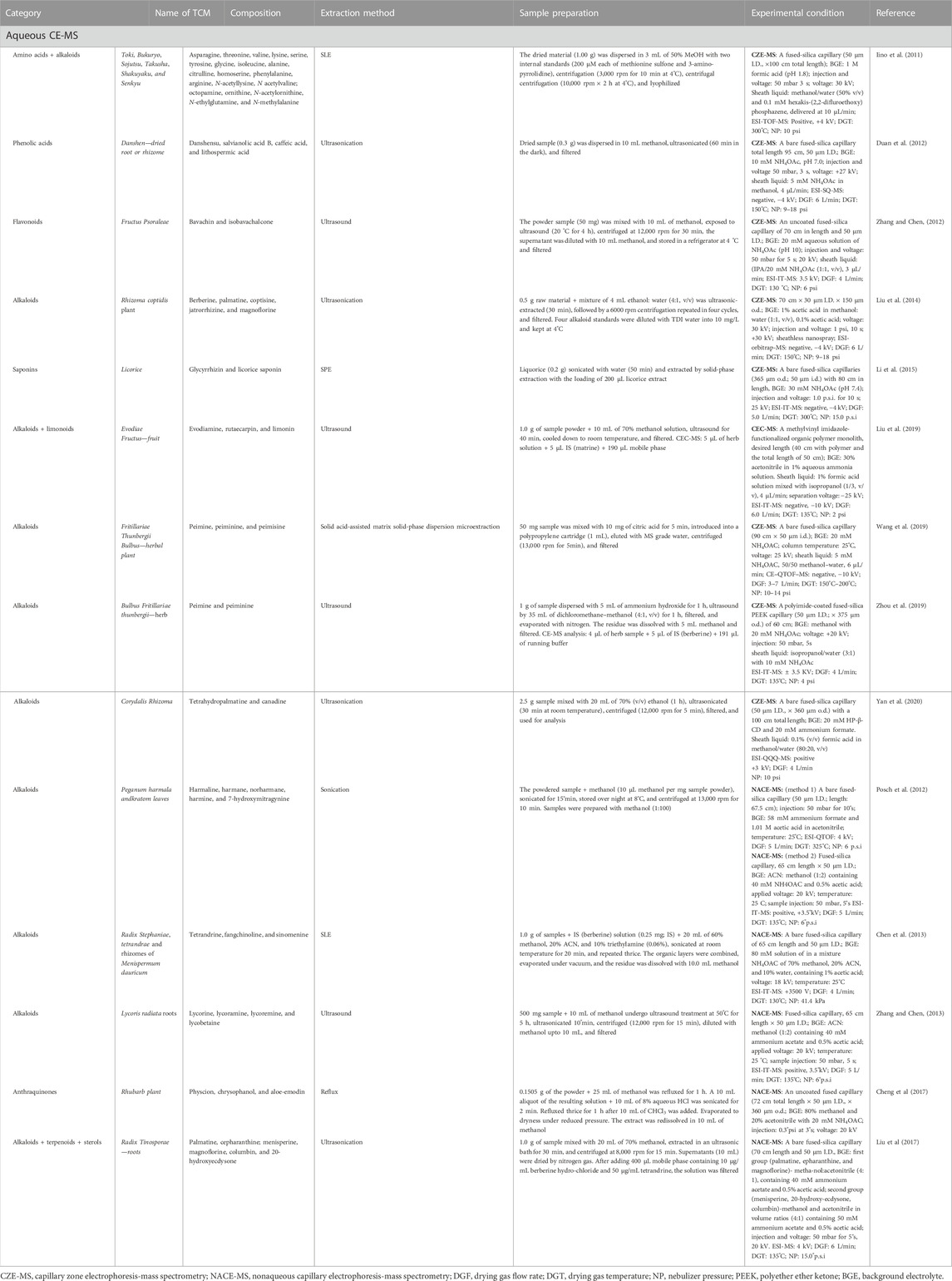
TABLE 5. Application of aqueous and non-aqueous capillary electrophoresis-mass spectrometry (CE-MS) for the analysis of TCM.
The experimental conditions of all the developed CE-MS methods for various TCM herbs analyses are detailed in Table 5 (rows 1–9). Toki-Shakuyaku-San is a TCM comprising several herbal medicines. To elucidate the composition of these herbs, non-targeted profiling of the amino acid and alkaloids metabolite and quantitation of extracts of these herbs was achieved using a non-targeted CE-TOF-MS (Iino, 2011). The loading plots demonstrated that amino acids and alkaloids are metabolite constituents. Several clusters overlapped in the tested herbs using the large-scale metabolomic approach, which is complementary to traditional quality control methods. Four hydrophilic phenolic acids (danshensu, salvianolic acid B, caffeic acid, and lithospermic acid) in plant-derived samples were evaluated for their antioxidant activity in the negative ion mode of ESI-MS (Duan et al., 2012). The spray chamber parameters (drying gas flow rate, drying gas temperature, and nebulizer pressure) and sheath liquid parameters (composition and flow rate of the sheath liquid) in combination with FAS injection increased the sensitivity by 50-fold without any matrix interference. CE-MS was used to analyze phenolic acids in the Danshen TCM. The isomers of bavachin (BC) and isobavachalcone (IBC) had similar mass spectra and fragmentation pathways when negative ESI-MS was used in combination with IT-MS (Zhang and Chen, 2012). The product ions at m/z 221 for each were further subjected to MS3 analysis to distinguish the two isomers. The formation of a product ion at m/z 203 occurred due to the neutral loss of H2O, whereas the formation of a fragment ion at m/z 159 from the parent ion at m/z 203 occurred due to the neutral loss of C3H8. However, the intensities of the BC dimer and its product ions were higher than those of the deprotonated molecule ion of the IBC dimer and its product ions. This abundance difference was the basis for discriminating BC from IBC in IT-MS.
A nanospray interface for CE-MS was applied to identify four alkaloids in the TCM Rhizoma coptidis. In response to the need to develop a stable and reliable sheathless interface, Liu et al. performed detailed optimization of emitter geometry, emitter position, emitter cleanliness, spray voltage, and buffer composition (Liu et al., 2014). The absolute (mass) LOD of four alkaloids ranged from 16 to 24 fg, which was 1000-fold lower than that for ultra-high-pressure HPLC-MS. CE-MS was combined with affinity CE to analyze the active components in Licorice extract for anti-HIV activity (Li et al., 2015). The interaction between the licorice extract and R15 K (core sequence of V3 loop in the R15K envelope protein) demonstrated its potential for the development of an anti-HIV-1 ingredient in this TCM extract.
CE coupled to quadrupole time-of-flight (QTOF)-MS and SPME was developed for the analysis of three alkaloids (peimine, peiminine, and peimisine) in Fritillariae Thunbergii Bulbus (Wang et al., 2019). The developed CE-MS method was superior due to the lower consumption of sample and dispersant, shorter grinding time, and greener elution solvent (water). The results demonstrated the satisfactory LODs (1.32–1.59 ng/mL), recoveries (86.63%–98.12%), and repeatability (peak areas RSDs<0.87%) of the CE-MS method. Two of the alkaloids (peimine and peiminine) were also analyzed in another TCM, Bulbus Frittillariae thunbergii using a robust polyetheretherketone (PEEK) capillary column (Zhou et al., 2019). The PEEK column cannot tolerate the high content of organic solvents but also ensures high mechanical and chemical stability to stabilize ESI. Utilizing 85% (v/v) methanol and 60 mM NH4OAc in the running buffer enhanced the resolution of the two hydrophobic alkaloids mentioned previously with a total analysis time of <17 min.
Recently, an enantioselective CE-MS method was developed for the simultaneous analysis of d/l-tetrahydropalmatine and R/S-canadine in Corydalis Rhizoma extract using 20 mM 2HP-β-CD as chiral selector and 20 mM NH4COOH (Yan et al., 2020). Because HP-β-CD is a non-volatile chiral reagent, a partial filling mode was used to avoid contamination of the chiral selector in ESI-MS. Furthermore, to facilitate ionization, a microvial CE-MS interface allowed a low flow rate (2 μL/min) for the post-column modifier (e.g., 0.1% (v/v) of HCOOH in 80/20 methanol–water) without any drying (sheath) gas, eliminating the suction effect that lowers the resolution of closely eluting enantiomers. Figure 8 shows the electropherograms for the analysis of Corydalis Rhizoma extracts, with well-separated enantiomers of d/l-tetrahydropalmatine (Figure 8A) by tandem MS/MS. However, the (R)-canadine peak was relatively small compared to that of (S)-canadine (Figure 8B). An internal standard (IS) (papaverine hydrochloride) was added to the extract due to its structural similarity to canadine and hydropalmatine (electropherogram not shown). The use of IS improves the analysis accuracy and repeatability. Furthermore, the analysis showed no interfering compound for papaverine in the Corydalis Rhizoma extract.
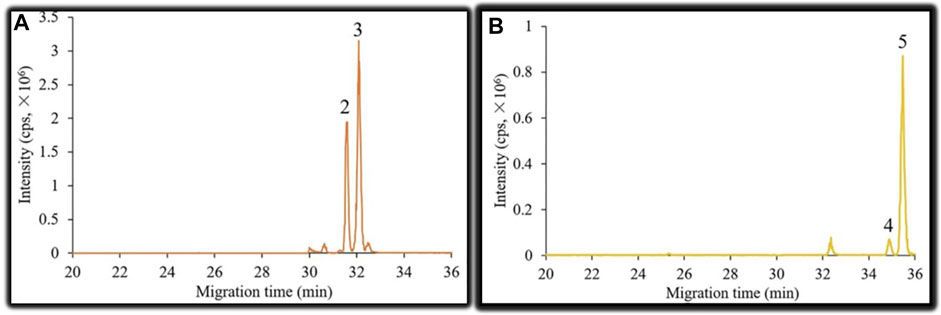
FIGURE 8. Electropherograms of (A) the mixed standard solution and (B) Corydalis Rhizoma extract peaks: 2. L-tetrahydropalmatine; 3. d-tetrahydropalmatine; 4 (R)-canadine; 5 (S)-canadine. Analytical conditions: 100 cm capillary length, 1.68 min partial filling time (at 10 psi), 20 mM HP-β-CD and 20 mM ammonium formate in 10% (v/v) FA solution as BGE, +24 kV effective separation voltage, and 0.1% (v/v) FA in methanol/water (80:20, v/v) as a chemical modifier with 2 μL min−1 flow rate. The figure and its caption are reproduced from Yan et al. (2020), with permission from Elsevier.
As mentioned previously in this section, only one reported study has described CEC-MS for TCM. The lower number of reports is mainly due to the lack of suitable and rugged CEC columns that interface with MS instruments. The column must also be compatible with commonly used volatile BGEs and have strong enough EOF to reasonably elute compounds. Liu et al. developed a CEC-MS (Liu et al., 2019) for evodiamine, rutaecarpin, and limonin in Evodiae Fructus fruit using homemade monolithic columns such as methyl-vinyl imidazole functionalized organic polymer monolith as stationary phases. The monolithic column offered extreme efficiency and outstanding separation selectivity by CEC-MS to analyze Evodiae Fructus. The authors observed at least 4–16-fold improvements in sensitivity for MS detection (LOD = 0.15–3.1 μg/mL) compared to UV detection (LOD = 2–12.5 μg mL−1).
5.2 Non-aqueous CE-MS
A significant obstacle in developing a CE-MS system is the possible contamination of the MS ionization source by non-volatile reagents such as borate and phosphate in the running buffer. Non-aqueous capillary electrophoresis (NACE) is a powerful tool for improving the selectivity of hydrophobic compounds that are hard to separate by electrophoresis in aqueous buffers (Chen et al., 2013). The detailed experimental conditions for NACE-MS methods in TCM analysis are listed in Table 5 (rows 10–14). Due to the comparatively high volatility and low surface tension of most organic solvents, NACE is well suited for online coupling with MS compared to traditional CE. The separation buffer in NACE comprises an organic solvent or a combination of two or more organic solvents containing dissolved electrolytes. Organic solvents offer a higher separation efficiency, lower electric current, and lower joule heat, enabling higher voltage and shorter analysis time (Kenndler, 2014).
A NACE-MS method with a carefully optimized experimental design was applied to many alkaloids in different plant extracts (Posch et al., 2012) to characterize the pattern of the psychoactive alkaloids in several plant alkaloids. The method successfully separated diastereomers and other isobaric compounds among alkaloid classes in the same run for high throughput. A comparison of techniques presented in the literature demonstrated the appropriateness of a near-generic NACE-MS method for the fast profiling of alkaloids in forensically relevant plants. For example, comparing the proposed method to existing methods for assessing indole alkaloid type allowed better separation and shorter analysis time. A subsequent study developed a non-aqueous CZE-IT MS with a nanospray ionization sheath liquid interface for the separation, identification, and quantitation of three alkaloids [tetrandrine (TET), fang-chinoline (FAN), and sinomenine] using berberine as internal standard (Chen et al., 2013] in radix Stephaniae tetrandrae and rhizomes of Menispermum dauricum. The method was optimized to separate all three alkaloids in 15 min using 80 mM NH4OAC in a mixture of 70/20% (v/v) methanol–acetonitrile with only 10% water in 1% acetic acid using a sheath liquid containing 50% (v/v) of aqueous methanol in 2% acetic acid. The two alkaloids, TET and FAN, were identified in radix Stephaniae tetrandrae, whereas TET and SIN were the alkaloids found in the rhizomes of Menispermum dauricum. The alkaloid extraction recoveries using SLE ranged from 95% to 99% with good precision of 2% and 4.6% RSD for migration time and peak areas, respectively. The LODs were 0.05 μg/mL, 0.08 μg/mL, and 0.15 μg/mL for TET, FAN, and SIN, respectively, which were lower than the previously published values for aqueous MEKC (Liu et al., 2015).
Lycorine, lycoramine, lycoremine, and lycobetaine are the four main alkaloids, which have similar structures and are difficult to separate in an aqueous CZE buffer. Therefore, the use of organic solvents in NACE with unique chemical and physical properties offers a different separation mechanism in CZE. A non-aqueous CZE coupled to ESI-IT-MS was optimized for the separation, identification, and quantitation of the Amaryllidaceae alkaloids (Zhang and Chen., 2013). The effects of composition and concentration of buffer applied voltage and sheath liquid flow rate, as well as nebulizing gas pressure, drying gas flow rate, and drying gas temperature, were optimized. Although lycoramine and lycoremine coeluted in the TIC mode, the additional selectivity offered in extract ion mode allowed the identification of all four alkaloids in <15 min. The IT-MS2 spectra in positive ion ESI-MS mode and the fragmentation pathways of primary fragment ions have been proposed to identify alkaloids in Lycoris radiata roots. The NACE-MS method shows high sensitivity and efficiency, as well as good linearity, recovery, and precision. Six active components (palmatine, cepharanthine, menisperine, magnoflorine, columbin, and 20-hydroxyecdysone) in Radix Tinosporae were analyzed by NACE-ESI-MS (Liu et al., 2017). All ingredients were separated into two groups and studied separately due to the disparity in ionization efficiencies of these six compounds. Following method validation, the results showed that this method has good linearity, precision, and recovery. This method has been successfully applied to determine the six bioactive components in Radix. Tinosporae. Cepharanthine could not be detected, whereas the levels of menisperine were too low to be qualified in the herb. The recoveries were performed by adding certain amounts of standard mixtures to real samples and ranged from 90% to 110%, indicating the good accuracy of this method.
Finally, a NACE-MS/MS was applied to separate, identify, and quantitate three anthraquinones (chrysophanol, physcion, and aloe-emodin) in the Rhubarb (Cheng et al., 2017). The separation parameters were optimized, including pH, BGE composition, flow-through microvial chemical modifier (sheath solution) composition, and flow rate. Using 20% ACN with 20 mM NH4OAC and 80% methanol as BGE, a mixture of ethanol in 0.2 mM NH4OAC showed strong signals and was considered an environmentally friendly modifier solution to use in the sheath liquid. The benefits of the developed NACE-MS were practically straightforward and required no further additive. Additionally, the improved sensitivity (low LODs), fast run times (analyses completed within 20 min), and high precision (based on low inter- and intraday RSD values) enabled the successful quantitation of closely related Rhubarb.
6 Conclusion
This review evaluated single and multicomponent mixtures of herbs present in TCM. After briefly describing the fundamentals of CE separation modes, we discussed the sample preparation and preconcentration techniques used for TCM. Next, we reviewed the applications regarding various modes of CE (CZE, MEKC, and CEC) and hyphenated CE (CE-MS and NACE-MS) for the analysis of TCM herbs from 2011 to 2021. The review reveals that short execution time, high efficiency, variety in separation modes, minimal solvent, and sample consumption make CE a cost-effective and environmentally friendly analytical tool for the quality control of TCM and raw plant material without compromising sensitivity.
Many contemporary methods for effectively extracting and preconcentrating target chemicals in TCMs have been developed recently. Conventional TCM sample preparation strategies, including UAE and MAE, are less sensitive and merely extract crude components, resulting in significant matrix interference. Furthermore, commercially accessible synergistic extraction devices (UAE combined with SPE and UAE combined with MAE) and online sample preparation methods for the quick and effective extraction of TCMs remain scarce. In future years, substantial attention should be devoted to designing and implementing proper instruments for TCM analysis. We particularly recommend increased consideration to developing eco-friendly methods with low expenditure on lethal organic solvents.
CE’s adaptability derives from its multidisciplinary approaches and various separation modes. The most commonly used CE mode is CZE with UV detection, which allows the rapid evaluation of a variety of active TCM compounds in dried raw with an LLOD. However, the resolution and sensitivity of active ingredients of TCM from its impurities are limited using the CZE-UV detection method in some cases, especially for trace impurity analysis. Additionally, among other electrophoretic techniques, including MEKC and CEC, are well-established CE modes that are beginning to be applied to simultaneously analyze ionic and neutral components in complex TCM samples. MEKC provides high separation efficiency with a short run time and lower sample volumes. Numerous applications have been developed in the past decade for TCM analysis based on this technique. In contrast, one application using monolithic CEC has been reported; however. this may change as CEC techniques become established in the scientific community. Both MEKC and CEC techniques are powerful alternatives to HPLC. Nevertheless, low sensitivity remains an issue. Alternative detection methods have been successfully applied, including conductometric, AD, CL, LIF, and MS. Other strategies to overcome this problem include the optimization of analytical parameters, including exotic modifiers to the BGE, and introducing sophisticated extraction and online preconcentration processes. In addition, miniaturized analytical approaches such as MCE for TCM analysis appeared to be effective, but only a few applications are reported. This review anticipates that CZE with UV detection will continue to be a mainstream analytical technique to identify and quantify complex medicinal plants and natural TCM products over the next 5 years.
Recently, CE with MS detection has attracted attention for the analysis of complex TCMs. The coupling of CE with MS can be used to measure molecular weights and provide structural information on the separated compounds in complex TCMs. Although in some circumstances, the findings were equivalent to those obtained by UV detection, the higher sensitivity and efficiency imply that CE-MS is going in the right direction and is considered a “green approach” for TCM analysis. MCE-MS and monolithic CEC-MS can reduce the amounts of reagents used, making these two technologies rapid, economical, and ecologically benign. Unlike HPLC-MS, CE-MS has a small number of dedicated commercial instruments, which limits the development and implementation of this potential hyphenated technique. Thus, the development of reliable, cost-effective CE-MS systems, ideally with thermostat cooling, solvent gradient capability, efficient and robust capillary columns, and an autosampler, is critical. As CE-MS has recently attracted the attention of researchers for the enantioselective separation of chiral pharmaceuticals, it can be an exceptional area for advancing research perspectives for the enantioselective screening of many types of herbs found in TCM.
Based on the current approaches and CE characteristics, establishing novel procedures for screening, identifying, and quantifying the active TCM components is a future CE trend with significant promise. Moreover, innovative materials, such as metal nanomaterial and nanoclusters, ILs, and graphene incorporated in the CE column, will help the development of more rugged stationary phases for reliable and repeatable separation by CEC-MS and microchip CEC-MS. The transfer of the unique selectivity and high sensitivity of HPLC-MS, to CEC-MS will enable it to contribute significantly to advancing the scientific analysis of TCM material. Furthermore, online coupling techniques based on MS-compatible micelles and chiral selectors used in MEKC will increase the sensitivity, accuracy, and efficiency of analytical techniques, which are a priority in TCM analysis. In the future, nanoparticles can be used in CE column fabrication to improve sensitivity and resolution, especially for trace analytes in complicated mixtures. In summary, CE is a powerful analytical technique and with further refinement to CE-MS or various modes of CE-MS such as CEC-MS, MEKC-MS, and microchip CEC-MS, this method could be a suitable replacement for costly methods for the analysis of neutral TCM and herbal raw materials.
Author contributions
JP wrote the draft and organized all the references and tables. SS reviewed and edited the manuscript.
Conflict of interest
The authors declare that the research was conducted in the absence of any commercial or financial relationships that could be construed as a potential conflict of interest.
Publisher’s note
All claims expressed in this article are solely those of the authors and do not necessarily represent those of their affiliated organizations, or those of the publisher, the editors, and the reviewers. Any product that may be evaluated in this article, or claim that may be made by its manufacturer, is not guaranteed or endorsed by the publisher.
Supplementary material
The Supplementary Material for this article can be found online at: https://www.frontiersin.org/articles/10.3389/frans.2023.1059884/full#supplementary-material
References
Acosta, G., Arce, S., Ortega, C., Martinez, L., and Gomez, M. (2014). On-line preconcentration preconcentration method using normal stacking mode and dynamic pH junction for the quality control of herbal medicines containing related phenolic compounds. Curr. Anal. Chem. 10, 197–204. doi:10.2174/15734110113090990018
Alves, R. C., Fernandes, R. P., Eloy, J. O., Salgado, H. R. N., and Chorilli, M. M. (2018). Characteristics, properties and analytical methods of paclitaxel: A review. Crit. Rev. Anal. Chem. 48, 110–118. doi:10.1080/10408347.2017.1416283
Alzoman, N. Z., Maher, H. M., Al-Showman, H., Fawzy, G. A., Al-Taweel, A. M., Perveen, S., et al. (2018). CE-dad determination of scutellarein and caffeic acid in abelia triflora crude extract. J. Chromatogr. Sci. 56, 746–752. doi:10.1093/chromsci/bmy042
Bao, Y., Yang, F., and Yang, X. (2011). CE-electrochemiluminescence with ionic liquid for the facile separation and determination of diester-diterpenoid aconitum alkaloids in traditional Chinese herbal medicine. Electrophoresis 32, 1515–1521. doi:10.1002/elps.201100040
Cao, J., Hu, J., Wei, J., Li, B., Zhang, M., Xiang, C., et al. (2015). Optimization of micellar electrokineticchromatography method for the simultaneous determination of seven hydrophilic and four lipophilic bioactive components in three salvia species. Molecules 20, 15304–15318. doi:10.3390/molecules200815304
Chambers, S. D., Svec, F., and Frechet, J. M. (2011). Incorporation of carbon nanotubes in porous polymer monolithic capillary columns to enhance the chromatographic separation of small molecules. J. Chromatogr. A 1218, 2546–2552. doi:10.1016/j.chroma.2011.02.055
Chen, B., Zhang, L., and Chen, G. (2011a). Determination of salidroside and tyrosol in rhodiola by capillary electrophoresis with graphene poly(urea-formaldehyde) composite modified electrode. Electrophoresis 32, 870–876. doi:10.1002/elps.201000435
Chen, C. Y., Wang, C. H., and Chen, A-H. (2011b). Recognition of molecularly imprinted polymers for a quaternary alkaloid of berberine. Talanta 84, 1038–1046. doi:10.1016/j.talanta.2011.03.009
Chen, J., Zhu, H., Chu, V. M., Jang, Y. S., Son, J. Y., Kim, Y. H., et al. (2011c). Quality control of a herbal medicinal preparation using high-performance liquid chromatographic and capillary electrophoretic methods. J. Pharm. Biomed. Anal. 55, 206–210. doi:10.1016/j.jpba.2010.12.022
Chen, Z., Wang, J., Chen, D., Fan, G., and Wu, Y. (2012). Sodium desoxycholate-assisted capillary electrochromatography with methacrylate ester-based monolithic column on fast separation and determination of coumarin analogs in Angelica dahurica extract. Electrophoresis 33, 2884–2891. doi:10.1002/elps.201200120
Chen, M., Chang, R., Xu, L., Huang, Y., Zhang, H., and Chen, A. (2018a). Simultaneous separation and determination of praeruptorin A, B, and C by micellar electrokinetic chromatography using sodium dodecyl sulphate and sodium cholate as mixed micelles. Phytochem. Anal. 29, 425–431. doi:10.1002/pca.2746
Chen, M., Huang, Y., Xu, L., Zhang, H., Zhang, G., and Chen, A. (2018b). Simultaneous separation and analysis of camptothecin alkaloids in real samples by large volume sample stacking in capillary electrophoresis. Biomed. Chromatogr. 32, e4125. doi:10.1002/bmc.4125
Chen, Q. H., Yu, F., Li, P., Wei, Y., and Huang, H. M. (2015). Comparative validations of capillary electrophoresis and high-performance liquid chromatography methods for the simultaneous determination of five anthraquinones in compound rhubarb enema. J. Liq. Chromatogr. Relat. Technol. 38, 942–947. doi:10.1080/10826076.2014.991873
Chen, Q., Li, N., Zhang, W., Chen, J., and Chen, Z. (2011d). Simultaneous determination of vinblastine and its monomeric precursors vindoline and catharanthine in Catharanthus roseus by capillary electrophoresis–mass spectrometry. J. Sep. Sci. 34, 2885–2892. doi:10.1002/jssc.201100359
Chen, Q., Zhang, J., Zhang, W., and Chen, Z. (2013). Analysis of active alkaloids in the menispermaceae family by nonaqueous capillary electrophoresis-ion trap mass spectrometry. J. Sep. Sci. 36, 341–349. doi:10.1002/jssc.201200678
Chen, S., Sun, G., Yang, L., and Zhang, J. (2017). Micellar electrokinetic chromatography fingerprinting combined with chemometrics as an efficient strategy for evaluating the quality consistency and predicting the antioxidant activity of Lianqiao Baidu pills. J. Sep. Sci. 40, 2838–2848. doi:10.1002/jssc.201700088
Chen, Y., Lu, W., Chen, X., and Teng, M. (2012a). Review of recent developments of on-line sample stacking techniques and their application in capillary electrophoresis. Cent. Eur. J. Chem. 10, 611–638. doi:10.2478/s11532-012-0007-4
Chen, Z., Wang, J., Chen, D., Fan, G., and Wu, Y. (2012b). Sodium deoxycholate-assisted capillary electrochromatography with methacrylate ester-based monolithic column on fast separation and determination of coumarin analogs in angelica dahurica extract. Electrophoresis 33, 2884–2891. doi:10.1002/elps.201200120
Cheng, J., Wang, L., Liu, W., and Chen, D. D. (2017). Quantitative nonaqueous capillary electrophoresis-mass spectrometry method for determining active ingredients in plant extracts. Anal. Chem. 89, 1411–1415. doi:10.1021/acs.analchem.6b04944
Chizzalli, E., Nischang, I., and Ganzera, M. (2011). Separation of adrenergic amines in citrus aurantium L. var. amara by capillary electrochromatography using a novel monolithic stationary phase. J. Sep. Sci. 34, 2301–2304. doi:10.1002/jssc.201100186
Deng, D., Deng, H., Zhang, L., and Su, Y. (2014). Determination of ephedrine and pseudoephedrine by field-amplified sample injection capillary electrophoresis. J. Chromatogr. Sci. 52, 357–362. doi:10.1093/chromsci/bmt039
Deng, Y., Lam, S. C., Zhao, J., and Li, S. P. (2017). Quantitative analysis of flavonoids and phenolic acid in coreopsis tinctoria nutt. by capillary zone electrophoresis. Electrophoresis 38, 2654–2661. doi:10.1002/elps.201700129
Diop, E. A., Jacquat, J., Drouin, N., Queiroz, E. F., Wolfender, J. L., Diop, T., et al. (2019). Quantitative CE analysis of punicalagin in combretum aculeatum extracts traditionally used in Senegal for the treatment of tuberculosis. Electrophoresis 40, 2820–2827. doi:10.1002/elps.201900240
Dong, S., Gao, R., Yang, Y., Guo, M., Ni, J., and Zhao, L. (2014). Simultaneous determination of phenylethanoid glycosides and aglycones by capillary zone electrophoresis with running buffer modifier. Anal. Biochem. 449, 158–163. doi:10.1016/j.ab.2013.11.014
Dresler, S., Bogucka-Koocka, A., Kovacik, J., Kubrak, T., Strzemski, M., Wojciak, K., et al. (2018). Separation and determination of coumarins including furanocoumarins using micellar electrokinetic capillary chromatography. Talanta 187, 120–124. doi:10.1016/j.talanta.2018.05.024
Duan, Q., Cao, J., and Zhang, J. (2012). Analysis of phenolic acids and their antioxidant activity by capillary electrophoresis-mass spectrometry with field-amplified sample injection. Anal. Methods. 4, 3027–3032. doi:10.1039/c2ay25437a
Dugheri, S., Marrubini, G., Mucci, N., Cappelli, G., Bonari, A., Pompilio, I., et al. (2021). A review of micro-solid phase extraction techniques and devices applied in sample pretreatment coupled with chromatographic analysis. Acta Chromatogr. 33, 99–111. doi:10.1556/1326.2020.00790
Dutra, L. S., Leite, M. N., Brandao, M. A., De Almeida, P. A., Vaz, F. A., and De Oliveira, M. A. (2013). A rapid method for total β-escin analysis in dry, hydroalcoholic and hydroglycol extracts of aesculus hippocastanum l. by capillary zone electrophoresis. Phytochem. Anal. 24, 513–519. doi:10.1002/pca.2425
Fang, S., Dong, L., Liu, L., Guo, J., Zhao, L., Zhang, J., et al. (2020). Herb: A high throughput experiment-and reference-guided database of traditional Chinese medicine. Nucl. Acids. Res. 49, D1197–D1206. doi:10.1093/nar/gkaa1063
Fu, Y., Zhang, L., and Chen, G. (2011). Determination of carbohydrates in folium lysium chinensis using capillary electrophoresis combined with far-infrared light irradiation-assisted extraction. J. Sep. Sci. 34, 3272–3278. doi:10.1002/jssc.201100649
Fu, Y., Zhang, L., and Chen, G. (2012). Far infrared-assisted extraction followed by MEKC for the simultaneous determination of flavones and phenolic acids in the leaves of rhododendron mucronulatum Turcz. J. Sep. Sci. 35, 468–475. doi:10.1002/jssc.201100816
Gackowski, M., Przyblska, A., Kruszewski, S., Koba, M., Madra-Gackowska, K., and Bogacz, A. (2021). Recent applications of capillary electrophoresis in the determination of active compounds in medicinal plants and pharmaceutical formulations. Molecules 26, 4141. doi:10.3390/molecules26144141
Gan, Z., Chen, Q., Fu, Y., and Chen, G. (2012). Determination of bioactive constituents in flossophorae immaturus and cortex fraxini by capillary electrophoresis in combination with far infrared-assisted solvent extraction. Food Chem. 130, 1122–1126. doi:10.1016/j.foodchem.2011.08.018
Gao, R., Wang, L., Yang, Y., Ni, J., Zhao, L., Dong, S., et al. (2015). Simultaneous determination of oleanolic acid, ursolic acid, quercetin and apigenin in Swertia mussotii franch by capillary zone electrophoresis with running buffer modifier. Biomed. Chromatogr. 29, 402–409. doi:10.1002/bmc.3290
Glowacki, R., Furmaniak, P., Kubalczyk, P., and Borowczyk, K. (2016). Determination of totalapigenin in herbs by micellar electrokinetic chromatography with UV Detection. J. Anal. Methods Chem. 2016, 3827832. doi:10.1155/2016/3827832
Gomes, A. F., Ganzera, M., Schwaiger, S., Stuppner, H., Halabalaki, M., Almeida, M. P., et al. (2018). Simultaneous determination of iridoids, phenylpropanoids and flavonoids in lippiaalba extracts by micellar electrokinetic capillary chromatography. Microchem. J. 138, 494–500. doi:10.1016/j.microc.2018.02.003
Gonda, S., Nguyen, N. M., Batta, G., Gyemant, G., Mathe, C., and Vasas, G. (2013). Determination of phenylethanoid glycosides and iridoid glycosides from therapeutically used plantago species by CE-MEKC. Electrophoresis 34, 2577–2584. doi:10.1002/elps.201300121
Guo, J., Wang, M., Guo, H., Chang, R., Yu, H., Zhang, G., et al. (2019). Simultaneous separation and determination of seven isoflavones in Radix Puerariae by capillary electrophoresis with a dual cyclodextrin system. Biomed. Chromatogr. 33, e4646. doi:10.1002/bmc.4646
Han, L., Liu, X., Yang, N., Li, J., Cai, B., and Cheng, S. (2012a). Simultaneous chromatographic fingerprinting and quantitative analysis of flavonoids in Pollen Typhae by high-performance capillary electrophoresis. Acta Pharm. Sin. B 2, 602–609. doi:10.1016/j.apsb.2012.10.003
Han, P., Luan, F., Yan, X., Gao, Y., and Liu, H. (2012b). Separation and determination of honokiol and magnolol in Chinese traditional medicines by capillary electrophoresis with the application of response surface methodology and radial basis function neural network. J.Chromatogr. Sci. 50, 71–75. doi:10.1093/chromsci/bmr010
Hancu, G., Simon, B., Rusu, A., Mircia, E., and Gyeresi, A. (2013). Principles of micellar electrokinetic capillary chromatography applied in pharmaceutical analysis. Adv. Pharm. Bull. 3, 1–8. doi:10.5681/apb.2013.001
Harvey, A. L., Edrada-Ebel, R., and Quinn, R. J. (2015). The re-emergence of natural products or drug discovery in the genomics era. Nat. Rev. Drug Discov. 14, 111–129. doi:10.1038/nrd4510
Honegr, J., and Pospisilova, M. (2013). Determination of phenolic acids in plant extracts using CZE with on-line transient isotachophoretic preconcentration. J.Sep. Sci. 36, 729–735. doi:10.1002/jssc.201200806
Hou, X., Sun, M., Bao, T., Xie, X., Wei, F., and Wang, S. (2020). Recent advances in screening active components from natural products based on bioaffinity techniques. Acta Pharm. Sin. B 10, 1800–1813. doi:10.1016/j.apsb.2020.04.016
Hou, Z., Sun, G., Guo, Y., Yang, F., and Gong, D. (2019). Capillary electrophoresis fingerprints combined with linear quantitative profiling method to monitor the quality consistency and predict the antioxidant activity of Alkaloids of Sophora flavescens. J.Chromatogr. B 1133, 121827. doi:10.1016/j.jchromb.2019.121827
Iino, K., Sugimoto, M., Soga, T., and Tomita, M. (2011). Profiling of the charged metabolites of traditional herbal medicines using capillary electrophoresis time-of-flight mass spectrometry. Metabolomics 8, 99–108. doi:10.1007/s11306-011-0290-7
Ji, Z., Sun, W., Sun, G., and Zhang, J. (2016). Monitoring the quality consistency of Fufang Danshen Pills using micellar electrokinetic chromatography fingerprint coupled with prediction of antioxidant activity and chemometrics. J. Sep. Sci. 39, 3019–3027. doi:10.1002/jssc.201600334
Jin, H., Tang, G., Li, J., Ma, L., Li, Y., and Chang, Y. X. (2020). Simultaneous determination of phenolic acids, anthraquinones, flavonoids, and triterpenes of cynomorii herba in different harvest times by LC-MS/MS. J. Anal. Methods Chem. 2020, 8861765. doi:10.1155/2020/8861765
Jin, W., Zhou, T., and Li, G. (2019). Recent advances of modern sample preparation techniques for traditional Chinese medicine. J. Chromatogr. A 1606, 460377. doi:10.1016/j.chroma.2019.460377
Kawai, T. (2017). Recent studies on online sample preconcentration methods in capillary electrophoresis coupled with mass spectrometry. Chromatogr 38, 1–8. doi:10.1002/elps.1150191317
Kenndler, E. (2014). A critical overview of non-aqueous capillary electrophoresis. Part II:Separation efficiency and analysis time. J.Chromatogr. A 1335, 31–41. doi:10.1016/j.chroma.2014.01.030
Kitagawa, F., Ishiguro, T., Tateyama, M., Nukatsuka, I., Sueyoshi, K., Kawai, T., et al. (2017). Combination of large volume sample stacking with an electroosmotic flow pump with field amplified sample injection on cross channel chips. Electrophoresis 38, 2075–2080. doi:10.1002/elps.201700155
Kitagawa, F., Kawai, T., and Otsuka, K. (2013). On-line sample preconcentration by large volume sample stacking with an electroosmotic flow pump (LVSEP) in microscale electrophoresis. Anal. Sci. 29, 1129–1139. doi:10.2116/analsci.29.1129
Kitagawa, F., and Otsuka, K. (2014). Recent applications of on-line sample preconcentration techniques in capillary electrophoresis. J.Chromatogr. A 1335, 43–60. doi:10.1016/j.chroma.2013.10.066
Kong, Y., Yang, G., Kong, L., Hou, Z., Yang, G., Li, H., et al. (2017). New application of pH-mediated acid stacking technique for amphoteric compounds in capillary electrophoresis: Example assay of blood glutathiones. J.Chromatogr. Sci. 55, 477–483. doi:10.1093/chromsci/bmw205
Lao, K. M., Han, D. Q., Chen, X. J., Zhao, J., Wang, T. J., and Li, S. P. (2013). Simultaneous determination of seven hydrophilic bioactive compounds in water extract of polygonum multiflorum using pressurized liquid extraction and short-end injection micellar electrokinetic chromatography. Chem. Cent. J. 7, 45. doi:10.1186/1752-153X-7-45
Leong, F., Hua, X., Wang, M., Chen, T., Song, Y., and Tu, P. (2020). Quality standard of traditional Chinese medicines: comparison between European Pharmacopoeia and Chinese Pharmacopoeia and recent advances. Chin. Med. 15 (76), 1–20.
Li, L., Xu, L., Chen, M., Zhang, G., Zhang, H., and Chen, A. (2017). Simultaneous separation and determination of four uncaria alkaloids by capillary electrophoresis using dual cyclodextrin system. J. Pharm. Biomed. Anal. 141, 39–45. doi:10.1016/j.jpba.2017.04.001
Li, W. L., Li, M. J., Pan, Y. L., Huang, B. K., Chu, Q. C., and Ye, J. N. (2014). Study on electrochemical profiles of valeriana medicinal plants by capillary electrophoresis. J. Anal. Chem. 69, 179–186. doi:10.1134/s1061934814020099
Li, Z., Zhao, Y., Lin, W., Ye, M., and Ling, X. (2015). Rapid screening and identification of active ingredients in licorice extract interacting with V3 loop region of HIV-1 gp120 using ACE and CE-MS. J. Pharm. Biomed. Anal. 111, 28–35. doi:10.1016/j.jpba.2015.02.021
Liang, J., Gong, F. Q., and Sun, H. M. (2018). Simultaneous separation of eight lignans in forsythia suspensa by β-cyclodextrin-modified capillary zone electrophoresis. Molecules 23, 514. doi:10.3390/molecules23030514
Liu, J., Tian, J., Li, J., Azietaku, J. T., Zhang, B. L., Gao, X. M., et al. (2016a). The in-capillary DPPH-capillary electrophoresis-the diode array detector combined with reversed electrode polarity stacking mode for screening and quantifying major antioxidants in cuscuta chinensis Lam. Electrophoresis 37, 1632–1639. doi:10.1002/elps.201500426
Liu, J. X., Zhang, Y. W., Yuan, F., Chen, H. X., and Zhang, X. X. (2014). Differential detection of rhizoma coptidis by capillary electrophoresis electrospray ionization mass spectrometry with a nanospray interface. Electrophoresis 35, 3258–3263. doi:10.1002/elps.201400334
Liu, L., Wu, B., Liu, K., Li, C. R., Zhou, X., Li, P., et al. (2016b). Multivariable optimization of the micellar system for the ionic liquid modified MEKC separation of phenolic acids. J. Pharm. Biomed. Anal. 126, 1–8. doi:10.1016/j.jpba.2016.04.027
Liu, Y. C., Sun, G. X., Wang, Y., Yang, L. P., and Yang, F. L. (2015). Monitoring the quality consistency of weibizhi tablets by micellar electrokinetic chromatography fingerprints combined with multivariate statistical analyses, the simple quantified ratio fingerprintmethod, and the fingerprint-efficacy relationship. J. Sep. Sci. 38, 2174–2181. doi:10.1002/jssc.201500145
Liu, Y. M., Li, J., Yang, Y., Du, J. J., and Mei, L. (2012). Separation and determination of ephedra alkaloids in traditional Chinese medicine and human urines by capillary electrophoresis coupled with electrochemiluminescence detection. Can. J. Chem. 90, 180–185. doi:10.1139/v11-143
Liu, Y., Zhou, W., Mao, Z., and Chen, Z. (2019). Analysis of Evodiae Fructus by capillary electrochromatography-mass spectrometry with methylvinylimidazole functionalized organic polymer monolilth as stationary phases. J.Chromatogr. A 1602, 474–480. doi:10.1016/j.chroma.2019.06.011
Liu, Y., Zhou, W., Mao, Z., Liao, X., and Chen, Z. (2017). Analysis of six active components in radix tinosporae by nonaqueous capillary electrophoresis with mass spectrometry. J. Sep. Sci. 40, 4628–4635. doi:10.1002/jssc.201700815
Lu, Y., Wang, X., Chen, D., and Chen, G. (2011). Polystyrene/graphene composite electrodefabricated by in situ polymerization for capillary electrophoretic determination of bioactive constituents in herba houttuyniae. Electrophoresis 32, 1906–1912. doi:10.1002/elps.201100162
Ma, D. D., Yang, L. P., Yan, B., and Sun, G. X. (2017). Capillary electrophoresis fingerprints combined with chemometric methods to evaluate the quality consistency and predict the antioxidant activity of Yinqiaojiedu tablet. J. Sep. Sci. 40, 1796–1804. doi:10.1002/jssc.201601155
Ma, H., Bai, Y., Li, J., and Chang, Y. X. (2018). Screening bioactive compounds from natural product and its preparations using capillary electrophoresis. Electrophoresis 39, 260–274. doi:10.1002/elps.201700239
Ma, H., Liu, T., Li, J., Ding, M., Gao, X. M., and Chang, Y. X. (2019). The in-capillary- 2,2-azinobis-(3-ethylbenzothiazoline-6-sulfonic acid) sweeping micellar electrokinetic chromatography diode array detector method for screening and quantifying trace natural antioxidants from schisandra chinensis. J. Chromatogr. A 1593, 147–155. doi:10.1016/j.chroma.2019.01.050
Ma, J., Ye, N., and Li, J. (2016). Covalent bonding of homochiral metal-organic framework in capillaries for stereoisomer separation by capillary electrochromatography. Electrophoresis 37, 601–608. doi:10.1002/elps.201500342
Maoe, H., Ye, X., Chen, W., Geng, W., and Chen, G. (2019). Fabrication of carbon nanotube polylactic acid composite electrode by melt compounding for capillary electrophoretic determination of tectoridin and irigenin in Belamcandae Rhizoma. J. Pharm. Biomed. Anal. 175, 112769. doi:10.1016/j.jpba.2019.07.017
Mao, Z., and Chen, Z. (2019). Advances in capillary electrochromatography. J. Pharm. Anal. 9, 227–237. doi:10.1016/j.jpha.2019.05.002
Mao, Z., Di, X., Zhang, J., Wang, X., Liu, Y., and Di, X. (2017). Rapid and cost-effective method for the simultaneous quantification of seven alkaloids in Corydalis decumbens by microwave assisted extraction and capillary electrophoresis. J. Sep. Sci. 40, 3008–3014. doi:10.1002/jssc.201700051
Mayadunne, E., and El Rassi, Z. (2014). Facile preparation of octadecyl monoliths with in corporated carbon nanotubes and neutral monoliths with coated carbon nanotubes stationary phases for HPLC of small and large molecules by hydrophobic and π–π interactions. Talanta 129, 565–574. doi:10.1016/j.talanta.2014.06.032
Pan, C., Wang, W., and Chen, X. (2016). In situ rapid preparation of homochiral metal-organicframework coated column for open tubular capillary electrochromatography. J. Chromatogr.A 1427, 125–133. doi:10.1016/j.chroma.2015.12.020
Pan, H., Liu, R., Cheddah, S., Zheng, Y., Hu, J., Wang, W., et al. (2019). Epinephrine-modified methacrylate monolithic column with fumed silica nanoparticle for pressurized capillary electrochromatography. Electrophoresis 40, 2469–2477. doi:10.1002/elps.201900040
Pang, H., Wu, L., Tang, Y., Zhou, G., Qu, C., and Duan, J. A. (2016). Chemical analysis of the herbal medicine salviae miltiorrhizae radix et rhizoma (danshen). Molecules 21, 51. doi:10.3390/molecules21010051
Patel, V. D., Shamsi, S. A., and Sutherland, K. (2021). Capillary electromigration techniques coupled to mass spectrometry: Applications to food analysis. Trends Anal. Chem. 139, 116240. doi:10.1016/j.trac.2021.116240
Peng, L. Q., Dong, X., Zhen, X. T., Yang, J., Chen, Y., Wang, S. L., et al. (2020). Simultaneous separation and concentration of neutral analytes by cyclodextrin-assisted sweeping-micellar electrokinetic chromatography. Anal. Chim. Acta 1105, 224–230. doi:10.1016/j.aca.2020.01.037
Posch, T. N., Martin, N., Pȕposch, T. N., Martin, N., Pȕtz, M., and Huhn, C. (2012). Nonaqueous capillary electrophoresis–mass spectrometry: A versatile, straightforward tool for the analysis of alkaloids from psychoactive plant extracts. Electrophoresis 33, 1557–1566. doi:10.1002/elps.201100682
Przybylska, A. A., Gackowski, M., and Koba, A. M. (2021). Application of capillary electrophoresis to the analysis of bioactive compounds in herbal raw materials. Molecules 26, 2135. doi:10.3390/molecules26082135
Quirino, J. P. (2009). Micelle to solvent stacking of organic cations in capillary zone electrophoresis with electrospray ionization mass spectrometry. J.Chromatogr.A. 1216, 294–299. doi:10.1016/j.chroma.2008.11.062
Rabanes, H. R., Guidote, A. M., and Quirino, J. P. (2014). Micellar electrokinetic chromatography of the constituents in philippine lagundi (vitex negundo) herbal products. Microchem. J. 112, 153–158. doi:10.1016/j.microc.2013.10.006
Ramadoss, K., and Oruganti, S. K. (2016). Isolation, characterization and determination of nordihydroguaiaretic acid in nutrition supplement by using reversed phase-high performance liquid chromatography. Eurasian J. Anal. Chem. 11, 225–232. doi:10.12973/ejac.2016.134a
Ramautar, R., Somsen, G. W., and De Jong, G. J. (2016). Developments in coupled solid phase extraction–capillary electrophoresis 2013–2015. Electrophoresis 37, 35–44. doi:10.1002/elps.201500401
Ren, T., and Xu, Z. (2018). Study of isomeric pentacyclic triterpene acids in traditional Chinese medicine of forsythiae fructus and their binding constants with β-cyclodextrin by capillary electrophoresis. Electrophoresis 39, 1006–1013. doi:10.1002/elps.201700408
Reyes, G., Gionfriddo, E., Gomez-Rioz, G. A., Alam, M. N., Gionfriddo, E., Gomez-Rios, G. A., et al. (2018). Advances in solid phase microextraction and perspective on future directions. Anal. Chem. 90, 302–360. doi:10.1021/acs.analchem.7b04502
Rizvi, S. A. A., and Shamsi, S. A. (2006). Synthesis, characterization, and application of chiral ionic liquids and their polymers in micellar electrokinetic chromatography. Anal. Chem. 78, 7061–7069. doi:10.1021/ac060878u
Roda, G., Liberti, V., Arnoldi, S., Argo, A., Rusconi, C., Suardi, S., et al. (2013). Capillary electrophoretic and extraction conditions for the analysis of catha edulis forks active principles. Forensic Sci. Int. 228, 154–159. doi:10.1016/j.forsciint.2013.02.034
Schnee, V. P., Baker, G. A., Rauk, E., and Palmer, C. P. (2006). Electrokinetic chromatographic characterization of novel pseudophases based on N-alkyl-N-methylpyrrolidinium ionic liquid type surfactants. Electrophoresis 27, 4141–4148. doi:10.1002/elps.200600268
Sheng, S., Liu, S., Zhang, L., and Chen, G. (2013). Graphene/poly(ethylene-co-vinyl acetate) composite electrode fabricated by melt compounding for capillary electrophoretic determination of flavones in Cacumen platycladi. J. Sep. Sci. 36, 721–728. doi:10.1002/jssc.201200856
Shih, T. T., Lee, H. L., Chen, S. C., Kang, C. Y., Shen, R. S., and Su, Y. A. (2018). Rapid analysis of traditional Chinese medicine pinellia ternata by microchip electrophoresis with electrochemical detection. J.Sep. Sci. 41, 740–746. doi:10.1002/jssc.201700901
Šlampova, A., Mala, Z., and Gebauer, P. (2018). Recent progress of sample stacking in capillary electrophoresis (2016-2018). Electrophoresis 40, 40–54. doi:10.1002/elps.201800261
Song, X. Y., Li, Y. D., Shi, Y. P., Jin, L., and Chen, J. (2013). Quality control of traditional Chinese medicine: A review. Chin. J. Nat. Med. 11, 596–607. doi:10.1016/S1875-5364(13)60069-2
Stege, P. W., S, L. L., Davicino, R. C., and Olsina, R. A. (2011). Analysis of nordihydroguaiaretic acid in larrea divaricata Cav. extracts by micellar electrokinetic chromatography. Phytochem. Anal. 22, 74–79. doi:10.1002/pca.1255
Sun, S., Wei, Y., Cao, Y., and Deng, B. (2017). Simultaneous electrochemiluminescence determination of galanthamine, homolycorine, lycorenine, and tazettine in lycoris radiata by capillary electrophoresis with ultrasonic-assisted extraction. J. Chromatogr. B. Anal. Technol. Biomed. Life Sci. 1055-1056, 15–19. doi:10.1016/j.jchromb.2017.04.020
Sun, Y., Li, Y., Zeng, J., Lu, Q., and Li, P. C. (2015). Microchip electrophoretic separation and fluorescence detection of chelerythrine and sanguinarine in medicinal plants. Talanta 142, 90–96. doi:10.1016/j.talanta.2015.04.008
Tang, L., Zhang, W., Zhao, H., and Chen, Z. (2015). Tyrosinase inhibitor screening in traditional Chinese medicine by electrophoretically mediated microanalysis. J. Sep. Sci. 38, 2887–2892. doi:10.1002/jssc.201500371
Tang, Z., Zang, S., and Zhang, X. (2012). Detection of chlorogenic acid in honeysuckle using infrared-assisted extraction followed by capillary electrophoresis with UV detector. J. Chromatogr. Sci. 50, 76–80. doi:10.1093/chromsci/bmr003
Tascon, M., Benavente, F., Vizioli, N. M., and Gagliardi, L. G. (2017). A rapid and simple method for the determination of psychoactive alkaloids by CE-UV: Application to peganum harmala seed infusions. Drug Test. Anal. 9, 596–602. doi:10.1002/dta.1989
Truus, K., Vaher, M., Borissova, M., Robai, M., Levandi, T., Tuvikene, R., et al. (2012). Characterization of yew tree (taxus) varieties by fingerprint and principal component analyses. Nat. Prod. Commun. 7, 1934578X1200700–1146. doi:10.1177/1934578x1200700908
Tseng, Y. J., Kuo, C. T., Wang, S. Y., Liao, H. W., Chen, G. Y., Ku, Y. L., et al. (2013). Metabolomic characterization of rhubarb species by capillary electrophoresis and ultra-highpressure liquid chromatography. Electrophoresis 34, 2918–2927. doi:10.1002/elps.201200580
Tu, Y., Li, L., Wand, Z., and Yang, L. (2021). Advances in analytical techniques and quality control of traditional Chinese medicine injections. J. Pharm. Biomed. Anal. 206, 114353. doi:10.1016/j.jpba.2021.114353
Tu, Y. (2011). The discovery of artemisinin (qinghaosu) and gifts from Chinese medicine. Nat. Med. 17, 1217–1220. doi:10.1038/nm.2471
Uzasci, S., and Erim, F. B. (2014). Enhancement of native fluorescence intensity of berberine by (2-hydroxypropyl)-β-cyclodextrin in capillary electrophoresis coupled by laser-induced fluorescence detection: Application to quality control of medicinal plants. J. Chromatogr. A 1338, 184–187. doi:10.1016/j.chroma.2014.02.068
Vinatoru, M., Mason, T. J., Calinescu, I., and Alinescu, I. (2017). Ultrasonically assisted extraction (UAE) and microwave assisted extraction (MAE) of functional compounds from plant materials. Trends. Anal. Chem. 97, 159–178. doi:10.1016/j.trac.2017.09.002
Wang, J., Wang, H., and Han, S. (2012). Ultrasensitive determination of epicatechin, rutin, and quercetin by capillary electrophoresis chemiluminescence. Acta Chromatogr. 24, 679–688. doi:10.1556/achrom.24.2012.4.13
Wang, L., Xu, H., Yu, L., Zhu, Z., Ye, H., Liu, L., et al. (2021). Simultaneous separation and analysis of five compounds in cibotium barometz by micellar electrokinetic chromatography with large volume sample stacking. Separations 8, 147–156. doi:10.3390/separations8090147
Wang, Q. Y., Dong, X., Yang, J., Zhen, X. T., Ye, L. H., Chu, C., et al. (2019). Solid acids assisted matrix solid-phase dispersion microextraction of alkaloids by capillary electrophoresis coupled with quadrupole time-of-flight mass spectrometry. J. Sep. Sci. 42, 3579–3588. doi:10.1002/jssc.201900647
Wang, S. F., Fang, H. Y., and Qu, H. B. (2011a). Optimization of micellar electrokinetic capillary chromatography method using central composite design for the analysis of components in yangwei granule. J. Zhejiang Univ. Sci. B 12, 193–200. doi:10.1631/jzus.B1000135
Wang, T., Wang, Y., Zhang, Y., Cheng, Y., Ye, J., Chu, Q., et al. (2020). Rapid preparation and evaluation of chiral open-tubular columns supported with bovine serum album and zeolite imidazolate framework-8 for mini-capillary electrochromatography. J. Chromatogr. A 1625, 461284. doi:10.1016/j.chroma.2020.461284
Wang, W. F., Yang, J. L., and Shi, Y. P. (2018). Quality evaluation of six bioactive constituents in goji berry based on capillary electrophoresis field amplified sample stacking. Electrophoresis 39, 2117–2124. doi:10.1002/elps.201800102
Wang, W., Lin, P., Ma, L., Xu, K., and Lin, X. (2016). Separation and determination of flavonoids in three traditional Chinese medicine by capillary electrophoresis with amperometric detection. J. Sep. Sci. 39, 1357–1362. doi:10.1002/jssc.201501287
Wang, X., Li, J., Qu, W., and Chen, G. (2011b). Fabrication of graphene/poly(methylmethacrylate) composite electrode for capillary electrophoretic determination of bioactive constituents in herba geranii. J.Chromatogr. A 1218, 5542–5548. doi:10.1016/j.chroma.2011.06.034
Wang, Y., Sun, G., Liu, Z., Liu, Y., Gao, Y., Zhang, J., et al. (2014). Capillary electrophoresis fingerprinting coupled with chemometrics to evaluate the quality consistency and predict the antioxidant activity of Sanhuang tablet as part of its quality control. J. Sep. Sci. 37, 3571–3578. doi:10.1002/jssc.201400765
Wu, C., Wang, W., Quan, F., Chen, P., Qian, J., Zhou, L., et al. (2018). Sensitive analysis of curcuminoids via micellar electrokinetic chromatography with laser-induced native fluorescence detection and mixed micelles-induced fluorescence synergism. J. Chromatogr. A 1564, 207–213. doi:10.1016/j.chroma.2018.06.012
Xiao, M. W., Bai, X. L., Liu, Y. M., Yang, L., Hu, Y. D., and Liao, X. (2018). Rapid quantification of aloin A and B in aloe plants and aloe containing beverages, and pharmaceutical preparations by microchip capillary electrophoresis with laser induced fluorescence detection. J. Sep. Sci. 41, 3772–3781. doi:10.1002/jssc.201800338
Xu, H. Y., Zhang, Y. Q., Liu, Z. M., Chen, T., Ly, C. Y., Tang, S. H., et al. (2019). Etcm: An encyclopaedia of traditional Chinese medicine. Nucleic Acids Res. 47, D976–D982. doi:10.1093/nar/gky987
Xu, L., Chang, R. C-M., Li, L., Huang, Y., Zhang, H., Chen, A., et al. (2017). Quality evaluation of guanxinning injection based on fingerprint analysis and simultaneous separation and determination of seven bioactive constituents by capillary electrophoresis. Electrophoresis 38, 3168–3176. doi:10.1002/elps.201700256
Xu, M., Zhang, H., Tang, T., Zhou, J., Zhou, W., Tan, S., et al. (2021). Potential and applications of capillary electrophoresis for analyzing traditional Chinese medicine: A critical review. Analyst 146, 4724–4736. doi:10.1039/d1an00767j
Yan, B. J., Huang, Z. A., Yahhaya, N., and Chen, D. D. (2020). Enantioselective analysis in complex matrices using capillary electrophoresis mass spectrometry: A case study of the botanical drug Corydalis rhizoma. J. Chromatogr. B Anal. Technol. Biomed. Life Sci. 1152, 122216. doi:10.1016/j.jchromb.2020.122216
Yang, S. P., Han, Y. Z., Wang, K. R., Wang, Y., Li, L. P., Li, N., et al. (2021). Simultaneous determination of four phenolic acids in traditional Chinese medicine by capillary electrophoresis-chemiluminescence. RSC Adv. 11, 33996–34003. doi:10.1039/d1ra06608k
Yaroshenko, I., Aroshenko, I., Roshenko, I., Kirsanov, D., Kartsova, L., Sidorova, A., et al. (2016). Exploring bitterness of traditional Chinese medicine samples by potentiometric electronic tongue and by capillary electrophoresis and liquid chromatography coupled to UV detection. Talanta 152, 105–111. doi:10.1016/j.talanta.2016.01.058
Ye, N., Li, J., Gao, C., and Xie, Y. (2013a). Simultaneous determination of atropine,scopolamine, and anisodamine in Flos daturae by capillary electrophoresis using a capillary coated by graphene oxide. J.Sep. Sci. 36, 2698–2702. doi:10.1002/jssc.201300304
Ye, N., Li, J., Xie, Y., and Liu, C. (2013b). Graphene oxide coated capillary for chiral separation by CE. Electrophoresis 34, 841–845. doi:10.1002/elps.201200516
Yin, X. F., Li, Z., Zhang, S. H., Wu, C. X., Wang, C., and Wang, Z. (2011). Determination of strychnine and brucine in traditional Chinese medicine preparations by capillary zone electrophoresis with micelle to solvent stacking. Chin. Chem. Lett. 22, 330–333. doi:10.1016/j.cclet.2010.10.044
Yu, H. X., Hao, Z. Y., Li, L., Huang, Y. Y., Zhang, H. F., and Chen, A. J. (2017). Analysis of three compounds in flos farfarae by capillary electrophoresis with large volume sample stacking. Int. J. Anal. Chem. 2017, 3813879. doi:10.1155/2017/3813879
Yuan, G., Liu, Y., Li, T., Wang, Y., Sheng, Y., and Guan, M. (2011). Simultaneous and rapid determination of main lignans in different parts of schisandra sphenanthera by micellar electrokinetic capillary chromatography. Molecules 16, 3713–3722. doi:10.3390/molecules16053713
Zahari, N. A. A. R., Chong, G. H., Abdullah, L. C., and Chua, B. L. (2020). Ultrasonic-assisted extraction (UAE) process on thymol concentration from plectranthus amboinicus leaves: Kinetic modeling and optimization. Processes 8 (8322), 1–17. doi:10.3390/pr8030322
Zhang, C., Xing, H., Yang, L., Fei, P., and Liu, H. (2022). Development trend and prospect of solid phase extraction technology. Chin. J. Chem. Eng. 42, 245–255. doi:10.1016/j.cjche.2021.05.031
Zhang, L., Zhang, W., and Chen, G. (2015a). Determination of arbutin and bergenin in Bergeniae Rhizoma by capillary electrophoresis with a carbon nanotube-epoxy composite electrode. J. Pharm. Biomed. Anal. 115, 323–329. doi:10.1016/j.jpba.2015.07.044
Zhang, L., Zhang, W., Chen, W., and Chen, G. (2016). Simultaneous determination of five bioactive constituents in rhizoma chuanxiong by capillary electrophoresis with a carbon nanotube-polydimethylsiloxane composite electrode. J. Pharm. Biomed. Anal. 131, 107–112. doi:10.1016/j.jpba.2016.08.031
Zhang, Q. F., and Cheung, H. Y. (2011). Development of capillary electrophoresis fingerprint for quality control of rhizoma smilacis glabrae. Phytochem. Anal. 22, 18–25. doi:10.1002/pca.1245
Zhang, W., Li, Y., and Chen, Z. (2015b). Selective and sensitive determination of protoberberines by capillary electrophoresis coupled with molecularly imprinted microextraction. J.Sep. Sci. 38, 3969–3975. doi:10.1002/jssc.201500864
Zhang, Y., and Chen, Z. (2013). Nonaqueous CE ESI-IT-MS analysis of amaryllidaceae alkaloids. J. Sep. Sci. 36, 1078–1084. doi:10.1002/jssc.201201083
Zhang, Y., and Chen, Z. (2012). Separation of isomeric bavachin and isobavachalcone in thefructus psoraleae by capillary electrophoresis–mass spectrometry. J. Sep. Sci. 35, 1644–1650. doi:10.1002/jssc.201200173
Zhao, H., and Chen, Z. (2013). Screening of aromatase inhibitors in traditional Chinese medicines by electrophoretically mediated microanalysisin a partially filled capillary. J.Sep. Sci. 36, 2691–2697. doi:10.1002/jssc.201300474
Zhao, H., and Chen, Z. (2014). Screening of neuraminidase inhibitors from traditional Chinese medicines by integrating capillary electrophoresis with immobilized enzyme microreactor. J. Chromatogr. A 1340, 139–145. doi:10.1016/j.chroma.2014.03.028
Zhao, T., Tang, H., Xei, L., Zheng, Y., Ma, Z., Sun, Q., et al. (2019). Scutellaria baicalensis georgi. (lamiaceae): A review of its traditional uses, botany, phytochemistry, pharmacology and toxicology. J. Pharm. Pharmacol. 71, 1353–1369. doi:10.1111/jphp.13129
Zhao, X., Liu, L., Li, N., Wang, T., Chai, Y., Yang, Z., et al. (2020). Zeolite silica nanoparticles-supported open-tubular columns for isomer and chiral separation using capillary electrochromatography coupled with amperometric detection. New J. Chem. 44, 1028–1035. doi:10.1039/c9nj04859f
Zhou, W., Liu, Y., Liao, X., and Chen, Z. (2019). Capillary electrophoresis-mass spectrometryusing robust poly(ether ether ketone) capillary for tolerance to high content of organic solvents. J. Chromatogr. A 1593, 156–163. doi:10.1016/j.chroma.2019.01.070
Zhou, X. J., Chen, J., Li, Y. D., Jin, L., and Shi, Y. P. (2015). Holistic analysis of seven active ingredients by micellar electrokinetic chromatography from three medicinal herbs composing Shuanghuanglian. J.Chromatogr Sci. 53, 1786–1793. doi:10.1093/chromsci/bmv067
Keywords: capillary electrophoresis, traditional Chinese medicine, characterization of active components in TCM, CE-MS for Chinese herbs, sample preparation and preconcentration
Citation: Shamsi SA and Patel J (2023) Advances and strategies for capillary electrophoresis in the characterization of traditional Chinese medicine: A review of the past decade (2011–2021). Front. Anal. Sci. 3:1059884. doi: 10.3389/frans.2023.1059884
Received: 02 October 2022; Accepted: 12 January 2023;
Published: 22 February 2023.
Edited by:
Marianne Fillet, University of Liège, BelgiumReviewed by:
Roman Řemínek, Institute of Analytical Chemistry (ASCR), CzechiaTomasz Baczek, Medical University of Gdansk, Poland
Copyright © 2023 Shamsi and Patel. This is an open-access article distributed under the terms of the Creative Commons Attribution License (CC BY). The use, distribution or reproduction in other forums is permitted, provided the original author(s) and the copyright owner(s) are credited and that the original publication in this journal is cited, in accordance with accepted academic practice. No use, distribution or reproduction is permitted which does not comply with these terms.
*Correspondence: Shahab A. Shamsi, c3NoYW1zaUBnc3UuZWR1