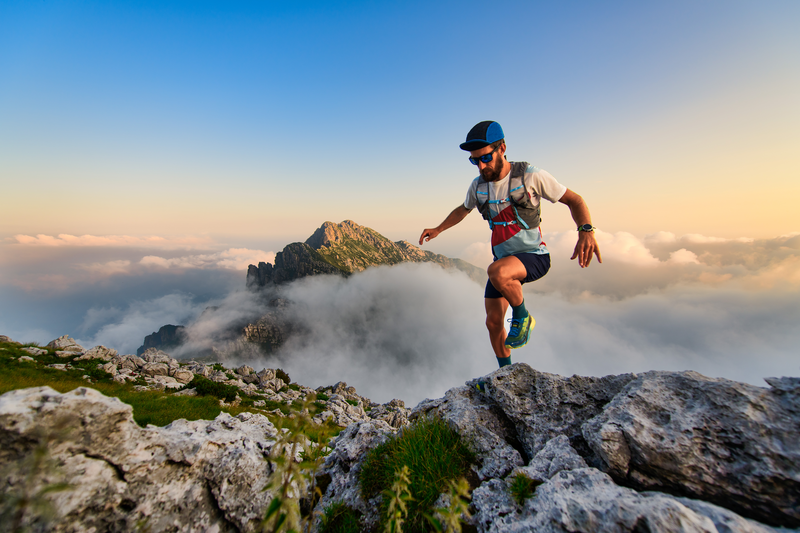
94% of researchers rate our articles as excellent or good
Learn more about the work of our research integrity team to safeguard the quality of each article we publish.
Find out more
ORIGINAL RESEARCH article
Front. Amphib. Reptile Sci. , 10 March 2025
Sec. Physiology and Health
Volume 3 - 2025 | https://doi.org/10.3389/famrs.2025.1500598
This article is part of the Research Topic Effects of Urbanization and Climate Change on the Physiology of Amphibians and Reptiles View all 3 articles
Introduction: Anthropogenic conversion of natural habitats is one of the most significant threats to biodiversity, and it is also partially responsible for the largest recorded decline in amphibians. Urbanization exposes wildlife to increased disturbance by proximity to humans, artificial light at night (ALAN), and noise pollution, all of which can be harmful to wild animals. Glucocorticoid hormones may play an important role in coping with environmental challenges. A growing body of evidence indicates in various vertebrate species that more efficient regulation of the glucocorticoid stress response may be favored by natural selection under stressful environmental conditions. In several species, baseline levels of glucocorticoids and also their response to acute stressors are repeatable, heritable, and related to individual fitness. However, in these latter respects, our knowledge is scarce for other aspects of glucocorticoid flexibility, such as the ability to recover from acute stress by negative feedback.
Methods: To address this knowledge gap, we measured the repeatability of four aspects of the corticosterone (CORT) profile (baseline release rate, strength of stress response to agitation, rate of recovery by negative feedback, and a recently proposed measure of sequential variability) over the ontogeny of tadpoles and juveniles of the common toad (Bufo bufo). Thereafter we measured the toadlets’ behavioral responses to three anthropogenic stressors, specifically their hop performance while fleeing from a human, and foraging rate under ALAN and during daytime noise pollution, to test whether the hormonal characteristics explain the behavioral differences between individuals.
Results: We found that the CORT profile changed during ontogeny, and all its measured aspects had low repeatability. Foraging rate increased in response to both ALAN and noise, and the two responses were correlated within individuals, but not with fleeing performance. None of the behavioral variables correlated with the CORT variables.
Discussion: Our results suggest that this widespread species, found in anthropogenic environments, has limited individual consistency in tolerance to anthropogenic stressors during early development, pointing to the importance of phenotypic plasticity in coping with the challenges of urban environments.
Biodiversity loss is one of the most pressing environmental issues today caused by habitat alteration, endangering ecosystem services and human well-being (Ceballos et al., 2017). A major facet of the human-induced rapid environmental changes is urbanization, which alters the physical, chemical, and biological environment in a multitude of ways. Depending on the species and circumstances, some of these changes may be beneficial, e.g. by providing novel resources, while others may be detrimental, e.g. by exposing wildlife to persistent stressors that negatively impact their health or fitness. How these changes affect wildlife has become a hot topic in evolutionary ecology, with a plethora of case studies documenting changes in various phenotypic traits of urban populations (e.g. Seress and Liker, 2015; French et al., 2018; Merckx et al., 2018; Sepp et al., 2018; Vincze and Kovács, 2022). For example, urban animals are frequently exposed to disturbance by humans, to which they consistently respond by altering their flight behavior (Samia et al., 2015). Urbanization is also accompanied by various forms of environmental pollution, including chemical contaminants and sensory pollutants such as anthropogenic light and noise (Kunc and Schmidt, 2019; Sanders et al., 2020; Sordello et al., 2020). These anthropogenic stressors also have wide-ranging ecological effects; for example, noise pollution can disrupt intraspecific communication and interspecific interactions (Samia et al., 2015; Kunc and Schmidt, 2019; Sordello et al., 2020), whereas artificial light at night (ALAN) affects diurnal cycles and the timing of seasonal processes (Owens and Lewis, 2018; Falcón et al., 2020; Parkinson et al., 2020; Sanders et al., 2020). Wild populations may cope with these challenges by physiological and behavioral adjustments, but which adjustment is adaptive depends on the stage of the urbanization process (Sol et al., 2013; Sanders et al., 2020; Hölker et al., 2021). For example, a trait that facilitates moving into urban habitats may be no longer advantageous for persisting there, and vice versa (Sol et al., 2013).
Animals can quickly adapt to new environmental conditions by changing their behavior, which is largely controlled by endocrine systems. The glucocorticoid (GC) hormones of vertebrates, secreted by the activation of the hypothalamus–pituitary–adrenal/interrenal (HPA/I) axis are particularly important in responding to stressors (Sapolsky et al., 2000; Romero, 2004; Romero et al., 2009). At baseline concentrations, these hormones regulate metabolic functions, but their circulating levels rise quickly when facing an acute stressor, and this endocrine stress response governs physiological and behavioral adjustments to escape or endure the stressor. After that, negative feedback along the HPA/I axis returns GC levels to their baseline to protect the organism from the negative effects of sustained high GC levels. How individuals regulate their GC profiles can influence their fitness; for example, reproductive success in a bird species is highest in individuals that exhibit certain combinations of high or low baseline, stress-induced, and recovery GC levels (Vitousek et al., 2018, 2019). Thus, if individuals differ consistently in aspects of their HPA/I axis regulation by heritable variation, natural selection can act on these traits, favoring the hormonal (and thereby behavioral) phenotypes that are adaptive in their environment. In line with this, both baseline and stress-induced GC levels are heritable in several species (reviewed by Hau et al., 2016; Guindre-Parker, 2018) and probably heritable in many more because they are often repeatable, i.e. vary consistently between individuals (Schoenemann and Bonier, 2018; Taff et al., 2018). Repeatability has been rarely investigated for the flexibility (i.e. reaction norm) of the HPA/I axis; however, the few studies that did so tended to find consistent individual variation in the magnitude of change in GC levels during stress response (Narayan et al., 2013; Lendvai et al., 2014; Fürtbauer et al., 2015) or in GC levels after negative feedback (Vitousek et al., 2019).
Urban environments may select for individuals with particular GC profiles. One hypothesis is that dampening of the stress response or “resistance to stress” may be adaptive in cities because too frequent or chronic elevation of GCs can be harmful (Partecke et al., 2006). Alternatively, the “on again, off again” hypothesis Zimmer et al. (2019) predicts that urban animals should maintain or even enhance their GC responsiveness for dealing with frequent stressors, but they should avoid the harmful effects by shutting down their stress responses rapidly via more efficient negative feedback. However, when researchers have compared baseline or stress-induced GC levels or negative feedback between urban and non-urban populations, the results were highly heterogeneous, with no clear consensus on an “urban GC profile” (Fokidis and Deviche, 2011; Bonier, 2012; French et al., 2018; Iglesias-Carrasco et al., 2020; Injaian et al., 2020; Bókony et al., 2021; Kolonin et al., 2022). It is possible that this heterogeneity is partly due to the fact that some traits may be beneficial during the colonization of urban habitats while some others may be preferred for establishment and yet others for coping with the challenges of a dense, long-urbanized population (Partecke et al., 2006; Sol et al., 2013).
In this study, we took a different approach to infer the role of GC regulation in coping with the challenges posed by urban environments. Rather than comparing conspecifics living in urban and non-urban habitats, we investigated what happens to an individual when it first encounters an anthropogenic stressor, as if it had just dispersed into an urban habitat. We aimed to answer the following questions: 1) Do individuals vary consistently in various aspects of their GC profile over their ontogeny? 2) Do the hormonal traits explain the individual differences in behavior when faced with novel anthropogenic stressors? If the answer to both questions is yes, then natural selection can act on the GC phenotype that yields the behaviors most beneficial for colonizing the urban habitat. To answer our questions, we raised common toads (Bufo bufo) in captivity throughout their larval development and for some more time after metamorphosis. We used a non-invasive sampling method to measure water-borne GC release rates before, during, and after an acute stressor (agitation) for all individuals three times as tadpoles and three times as juveniles. At the end of the study, we tested the toadlets’ behavior in three novel situations, in which we exposed them to ALAN, anthropogenic noise, and prodding by a human, respectively. The common toad is a suitable study species because it is widespread across much of Eurasia and often occurs in anthropogenically modified habitats (Cogălniceanu et al., 2023). Although the toads tend to be philopatric, they can disperse to large distances and thus colonize new habitats (Smith and Green, 2005).
Every procedure in this study followed animal ethics rules and were approved by the Ethics Committee of the Plant Protection Institute. The Environment Protection and Nature Conservation Department of the Pest County Bureau of the Hungarian Government granted permission for the study (PE06/KTF/8060-3/2018, PE-06/KTF/8060-1/2018, PE/EA/295-7/2018).
We collected common toad eggs from a Hungarian pond “Bajdázó-tó” (coordinates: N47.903437, E18.978268) surrounded by natural woodlands. We collected ca. 30 freshly spawned eggs from each of six different egg strings and took them into captivity in a room with mean temperature 22°C ± 2.04 and natural outdoors photoperiod (via a window) during the entire investigation. Throughout the study, we used oxygenated aged tap water. The tadpole siblings were kept together until they reached developmental stage 25 (Gosner, 1960), at which point (day 1 of the study) we randomly selected five tadpoles from each sibling group, yielding a total sample size of 30 animals. We released the remaining tadpoles at the egg-collection site.
We kept the 30 tadpoles individually in 2-L white plastic boxes filled with 1 L water. The boxes were placed on four shelves in a random order, and the individuals’ order was changed every week until the experiment ended. We changed the tadpoles’ water twice a week and fed them ad libitum with finely chopped spinach. When the individuals started the climax of metamorphosis and forelimbs appeared (developmental stage 42), we reduced the water to one deciliter, and we angled the boxes to create a dry region within the box. When metamorphosis was complete (at developmental stage 46), we placed a wet paper towel in the bottom of the box to keep the animals moist, as well as an egg carton piece to serve as shelter for the toadlets. Twice a week, we fed the toadlets with ant-sized house crickets (Acheta domesticus) powdered with a mixture of vitamins and minerals. The top of each toadlet’s box was covered with a perforated, transparent lid to allow light in but prevent escape.
We took water-borne CORT samples from each individual on six occasions: one, two, and three weeks after the study began (days 8, 15, and 22), and three, four, and five weeks after the last individual had completed metamorphosis (days 57, 64, and 71). Then each toadlet participated in three behavioral assays to test their foraging performance under ALAN (day 84) and daytime noise (day 90) and their locomotor performance while fleeing from a human (day 93). We did not sample CORT around the expected time of metamorphosis because CORT levels change during that time as part of mediating that process (Jaffe, 1981; Kikuyama et al., 1993; Glennemeier and Denver, 2002), so individual differences in the timing of metamorphosis may have masked repeatability. Furthermore, freshly metamorphosed toadlets are very small and fragile, and we did not want to risk their drowning during water-borne CORT sampling.
After collecting the third CORT sample on each sampling occasion, we took photographs of the tadpoles to estimate their body size, and we measured the body mass of the toadlets (± 0.01 g) after gently patting them dry with a paper towel. We did not directly measure body mass in tadpoles to avoid the stress of drying them. Instead, we followed the methods of Davis et al. (2008), who showed that the dorsal surface area of the tadpoles correlates with their body mass. The tadpoles were photographed from above individually in a small plastic dish with an Olympus TG4 camera fixed on a tripod, under natural light, and including millimeter paper for size reference in the picture. We took several JPG images of each tadpole in macro mode and burst mode, and we chose one in which the tadpole was not moving and its dorsal surface was fully in view. We used the ImageJ software (Schneider et al., 2012) to measure the total surface area of the tadpole’s body (including the tail). We took a similar photograph of each individual when they reached developmental stage 42, upon which we measured their body mass as we did with toadlets, to estimate the relationship between mass and surface area. Using standardized major axis regression, we obtained the following formula: body mass (mg) = 50.1 + (1.8 × surface area in mm2); the relationship was highly significant (P < 0.001). We used this formula to estimate tadpole body mass at CORT sampling.
During the study, 4 out of the 30 animals died for unknown reasons, all belonging to a single sibling group that showed stunted growth. Throughout the paper we present data for the remaining 26 individuals. After the last behavioral assay, the animals were returned to their habitat of origin.
We took a baseline, stressed, and recovery sample of CORT released by individuals into the surrounding water (Figure 1) using a non-invasive method of water-borne hormone sampling (Gabor et al., 2013b, a; Forsburg et al., 2019). This approach gives a time-integrated CORT measurement that is repeatable within individuals, correlates with plasma levels, and responds to an ACTH challenge (Gabor et al., 2013a, b; Forsburg et al., 2019). Earlier studies with various toad species showed that this approach is appropriate for sampling the CORT profile in both tadpoles and juveniles (Bókony et al., 2021; Monroe et al., 2024). Upon each sampling occasion, we placed each tadpole in a clean plastic insert (a perforated cup, to facilitate removal of tadpoles from the other cup) using a hand-made small net into a 250 ml plastic cup containing 100 ml water. We left the animals undisturbed for an hour to measure baseline CORT release rates. Then we moved them (with the perforated insert) into another plastic cup of 100 ml water, and we agitated them for an hour by gently shaking their cups for 1 minute every 3 minutes to measure stressed corticosterone release rates (Forsburg et al., 2019). We then transferred the tadpoles into a third plastic cup with 100 ml water and left them undisturbed for an hour to measure recovery CORT release rates. After metamorphosis we took CORT samples from the toadlets in a similar way, except that we put them into 50 ml water in a 750 ml plastic box and inserted another, identical box on top to prevent escaping from the water (Figure 1). Each toadlet was moved using a separate mosquito net piece.
Figure 1. Schematic illustration of the waterborne hormone sampling protocol used to measure corticosterone profile across time. The profile represents the baseline, response to agitation (stressed), and recovery samples of CORT release rate. Source: Created with BioRender.com.
Each water-borne hormone sample was filtered using coffee filters (equivalent to grade 4 filter paper) immediately after collection and stored at −20°C. We extracted hormones from the water samples using C18 solid phase extraction (SPE) columns (SepPak Vac3 cc/500 mg; Waters, Inc., Milford, MA, USA) primed with 100% HPLC-grade methanol (4 ml) and distilled water (4 ml). Then we stored the columns at −20°C until analysis. After defrosting, we washed the columns with 2 ml distilled water and eluted them with 4 ml methanol into borosilicate vials, followed by nitrogen gas evaporation. After drying, we re-suspended the residue in a total volume of 500 μl consisting of 25 µl ethanol (95% lab grade) and 475 µl enzyme-immunoassay (EIA) buffer (Cayman Chemicals Inc., Ann Arbor, MI, USA). We measured CORT concentration in duplicates for all samples using Corticosterone EIA kits (№ 501320, Cayman Chemical Company, Inc.; assay has a range of 8.2–5000 pg/ml and a sensitivity (80% B/B0) of approximately 30 pg/ml). Sample absorbance was read on a spectrophotometer plate reader at 405 nm (BioTek 800XS). Inter-plate coefficient of variation of the concentration standards was 12.8% across 19 plates; intra-assay coefficient of variation (CV) averaged 9.5% (range: 6.7–16.1%). Six plates had CV > 10%; samples with high intra-assay CV were re-run and we used the measurement with the lowest CV for each individual.
We calculated the amount of CORT in each sample by multiplying CORT concentration (pg/ml) by the resuspension volume (0.5 ml). We quantified corticosterone release rates (pg/g/h) as the amount of water-borne corticosterone measured divided by the duration of sample collection and body mass. To quantify the magnitude of stress response, we calculated the relative change of CORT release rate in response to stress (stress-induced change) as: 100 × (stressed − baseline)/baseline. Similarly, we quantified the rate of negative feedback as the relative change from stressed to recovery levels as: 100 × (stressed − recovery)/stressed (Lattin and Kelly, 2020). We also calculated a recently proposed measure of HPA/I flexibility (Zimmer et al., 2021): the square root of the mean squared differences (RMSSD) of corticosterone concentrations between successive sampling occasions. We calculated RMSSD for all CORT release rates (3 × 6 = 18 values per individual), and also separately for tadpoles and for toadlets.
The two assays of foraging behavior were performed in the same order for all individuals. Each assay consisted of an acclimation period, an 8-hours control trial, and an 8-hours disturbance trial, always in this order (Figure 2). For acclimation, we placed the animal-containing boxes in a circle on the floor two days before the control trial, we removed the shelters from the boxes (to prevent the toadlets from hiding) and we left a less damp paper towel in each box (to prevent the crickets from drowning). We expected that the acclimation period would allow the animals to habituate to the slightly altered circumstances of the control trial, and thereby minimize any carry-over effect to the disturbance trial. Because we had no capacity for keeping a control group to quantify the sequential effect, we preferred to expose all individuals to each trial in the same order so that their behavioral data would be directly comparable to each other as well as to the GC measurements.
Figure 2. Schematic illustration of the ALAN and noise tests. Source: Created with BioRender.com.
To investigate how ALAN affects feeding success, we measured the change in the number of crickets ingested, corrected for toadlet body mass. The control trial started at sunrise (0500h), when we took all the crickets from the boxes and measured the toadlets’ mass. The animals spent the day without food to standardize hunger levels. At sunset (2100h), we put twenty crickets into each toadlet’s box and left the animals undisturbed in the dark until the next sunrise. At 0500h, we removed all the remaining crickets from the boxes, counted them, and left the toadlets without food for the day. We began the disturbance trial at 2100h by putting twenty crickets into each box, but this time we left a light on until 0500h (Figure 2). We used an EMOS ZQ5130 (LED-A60-PISW-WW) light bulb (230 V, 50Hz/70mA, −5~+40°C/8W/E27/2700K), which was 193 cm above the ground in the center of the area surrounded by the boxes. Light intensity was 66 LUX directly under the lamp, and 39 LUX in the boxes, as measured with Lux Light Meter Pro application. We counted the remaining crickets at sunrise, and after weighing the toadlets, we placed their boxes back on the rearing shelves.
We performed a similar assay to test how daytime noise affects feeding success. After two days of acclimation, we removed all crickets from the boxes for the night and weighed the toadlets. The next day at 0900h, we started the control trial by placing twenty crickets in each toadlet’s box. We left the animals forage without disturbance until 1700h, when we removed all the remaining crickets from the boxes and counted them. After a night without food, at 0900h we placed twenty crickets in each toadlet’s box again to begin the disturbance trial, during which we continuously played back urban sounds and noises until 1700h, using the SONYC Urban Sound Tagging Database (Cartwright et al., 2019) (Figure 2). The decibel X PRO: Sound Metre application was used to measure the strength of the various noise levels at the boxes, which ranged from 45 to 98 dB.
To control for individual differences in body size, we divided the number of crickets eaten with body mass measured at the start of the control trial of the respective assay (i.e., ALAN and noise test). We are referring to this variable as consumption rate (i.e. number of crickets eaten per each gram of body mass). To express the toadlets’ foraging response to each disturbance with a single variable for each assay, we subtracted the consumption rate during the control trial from the consumption rate during the disturbance trial. This latter variable will be referred to as the change in consumption rate. One toadlet had to be excluded from the analysis of the ALAN test because the crickets hid under the paper towel on bottom of the box.
Locomotor performance is often key in animal fitness. To quantify the toadlets’ performance in a situation mimicking a chase by a human, we conducted a trial that is often used with amphibians (Goater et al., 1993; Sanabria et al., 2013; Zamora-Camacho, 2018; Hudson et al., 2020; Park and Do, 2023). The test was conducted two days following the noise pollution trial. The animals were fed crickets ad libitum before the test, and they had access to shelter as well. We made an oval-shaped arena (110 cm × 70 cm) out of a flexible PVC slab and drew a 5 cm × 5 cm rectangle on the bottom of the arena. To start the trial, we placed a toadlet into the rectangle, and gently prodded on the urostyle with a wooden stick to induce hopping. The trials were recorded using a Canon HD Legria HF R66 type camera (advanced zoom was 57×, optical zoom was 32×, and we utilized optical image stabilizer) into MP4 files. Each trial lasted a maximum duration of 6 minutes or ended if the toadlet stopped hopping when poked twice (Figure 3). After each trial, we cleaned the bottom of the arena with 96% ethanol and waited 2 minutes for the alcohol to evaporate before proceeding to the next animal. The trials were performed between 1100h and 1500h in a random order of individuals.
Figure 3. Testing arena for locomotor performance trial of toadlets. We started toadlets in a rectangle at the bottom of the arena and prodded the urostyle with a stick to induce hopping for 6 min while recording the behavior. The yellow line is the total track hopped and the numbered black tags mark the consecutive landing spots. Source: Created with BioRender.com.
We used Boris video analysis software (Friard and Gamba, 2016) to clip the images from the video recordings for measuring the hopping distances as follows. We selected the frames when the animal landed after each hop and saved them to PNG files. Then, by overlaying the photos (Figure 3), we calculated the distance (in cm) between the consecutive landing spots using the ROI management tools (Dobretsov et al., 2017) of the ImageJ program. For each toadlet, we calculated the total number, total distance, and average distance of all hops, and the average distance of the first four hops (Goater et al., 1993). To control for individual differences in body size, we divided each of these variables with the last body mass measurement (taken at the end of the noise trial).
All analyses were run in R4.3.2 (R Core Team, 2023). To analyze how CORT release rates varied between the three consecutive GC profile samples (“sample type”) within occasion and between occasions (“age”), we used a linear mixed-effects (LME) model (function “lme” of package “nlme”). The dependent variable was log10-transformed CORT release rate (18 values per individual), the explanatory variables were sample type and age and their interaction, and the random factors were individual identity nested in sibling group. We allowed for heterogeneous variances among the combinations of sample type and age by the compound symmetry autocorrelation structure (function “varIdent” of package “nlme”). Using a likelihood ratio test, we compared this model to a similar model without the sibling group variable to test if this random effect was significant. Because it was not (see Results), we omitted it. From the resulting model, we calculated pairwise comparisons (function “emmeans” from package “emmeans”) and corrected their p-values for multiple testing by the false-discovery rate (FDR) method (Pike, 2011).
To test the repeatability of the CORT profile, we estimated the intra-class correlation coefficient (ICC) using function “rpt” from package “rptR” (Nakagawa and Schielzeth, 2010). First, we quantified ICC for all CORT release rates, adjusted for the interaction between sample type and age. Then we calculated ICC for various aspects of the CORT profile as follows, adjusting to age (the number of sampling occasion, treated as a categorical variable). Within each sampling occasion, let us designate the three consecutive samples as B, D, and F, short for before, during, and following agitation. For tadpoles, we tested the repeatability of the following variables across the first 3 sampling occasions: baseline CORT release rate (B levels measured in the first hour; log-transformed), stressed CORT release rate (D levels measured in the second hour; log-transformed), recovery CORT release rate (F levels measured in the third hour; log-transformed), the magnitude of stress response quantified as 100 × (D − B)/B and transformed as square-root(x+65) following Bókony et al. (2021), and the rate of negative feedback quantified as 100 × (D − F)/D and transformed as (x+239)3 following Bókony et al. (2021). Because the shape of the CORT profile changed from the second to the third tadpole sampling occasion (see Results), we also tested the above repeatabilities across the first two sampling occasions only. For toadlets, we tested the repeatability of CORT release rates in the 3 samples (B, D, and F) similarly as in tadpoles. However, due to the unexpected shape of the CORT profile of toadlets (see Results) we could not quantify the magnitude of the stress response in toadlets, and we estimated the repeatability of their rate of negative feedback calculated as 100 × (B − average(D,F))/B (this variable had several outliers so it was rank-transformed). To test repeatability for the aspects of the CORT profile over all 6 sampling occasions, as stressed CORT release rates we used the second samples for tadpoles (D) and the first samples for toadlets (B), whereas as negative feedback we used the respective calculation for tadpoles and toadlets based on the shape of their CORT profiles, as explained above. We also tested the correlation between tadpole RMSSD and toadlet RMSSD using Pearson correlation. Note that we could not use the reaction-norm approach to quantify CORT profile repeatability because the reaction-norm shape was non-linear and changed with ontogeny; furthermore, our sample size would not have permitted good power for estimating repeatability of reaction-norm slopes (Martin et al., 2011; van de Pol, 2012).
To test the effects of ALAN and noise on foraging success, we used an LME model in which the dependent variable was consumption rate (4 values per individual), the explanatory variables were trial type (disturbance or control) and time of day (night for ALAN, day for noise) and their interaction, and the random factors were individual identity nested in sibling group. Because the random effect of sibling group was non-significant (p > 0.999) in a likelihood ratio test, we omitted it. Then, to test if any aspect of the CORT profile explained the changes in consumption, we added each CORT variable (i.e. those that were tested for repeatability) into the model, one at a time, in a three-way interaction with the other two explanatory variables. To test if the toadlets’ responses to ALAN and noise were correlated, we performed a Spearman rank-correlation test between the two foraging assays using the change in consumption rate (from control to disturbance) for each assay. To test if measures of fleeing performance were correlated with changes in consumption rate in the two foraging assays, and with CORT variables, we performed Spearman rank-correlation tests, and we corrected the p-values for multiple testing by the FDR method.
We report the results of the LME models with type-2 analysis-of-deviance tables (function “Anova” from package “car”). In all analyses, we used 95% confidence levels. However, when graphically presenting the results, we provide 84% confidence intervals (CI) to facilitate visual comparisons between groups, because non-overlap between two 84% CIs is equivalent to a significant difference between them (i.e. the 95% CI of their difference excludes zero).
Individual corticosterone release rates varied significantly with age and sampling type (sample type: χ2 = 13.32, df = 2, p = 0.001; age: χ2 = 1227.72, df = 5, p < 0.001, sample type × age: χ2 = 112.7, df = 10, p < 0.001). At the same time, the random effect of sibling group was practically zero (likelihood-ratio test, p = 0.999). The pairwise comparisons revealed a significant stress response at all three tadpole sampling occasions (Figure 4), as well as significant recovery following agitation at the first two (but not at the third) tadpole sampling occasions (Figure 4). Also, the stressed CORT release rate of agitated tadpoles decreased from the second to the third sampling occasion (Figure 4). In toadlets, however, CORT release rates at all sampling occasions were higher in the first sample (before agitation) than in the second and third samples (i.e. during and following agitation; Figure 4). Between occasions, CORT release rate of the first sample decreased from the second to the third toadlet sampling occasion (Figure 4), and the CORT release rate of the third sample increased from the first to the third toadlet sampling occasion (Figure 4).
Figure 4. Corticosterone (pg/g/h) release rate profiles (baseline, stressed, and recovery) of tadpoles and toadlets across six sampling occasions. Boxplots present medians, interquartile ranges, and full data ranges (middle line, box, and whiskers, respectively); error bars represent means and 84% confidence intervals estimated by a linear mixed model. Below each plot, letters “a” and “b” mark differences with p ≤ 0.03 after FDR correction; the asterisk means p = 0.057 (samples marked with different letters are significantly different from each other within sampling occasions). Dashed lines marked with two asterisks denote significant differences between sampling occasions (p ≤ 0.03 after FDR correction).
All investigated aspects of the CORT profile had low repeatability that did not differ significantly from zero both within tadpoles and within toadlets, as well as when we included all six sampling occasions (Table 1). There were two exceptions to this general trend. First, the repeatability of CORT release rates across all sampling occasions and all sample types was significantly higher than zero, although its value was still very low (ICC = 0.05; Table 1). Second, early tadpole stress response had relatively high repeatability (ICC = 0.30; Figure 5A) compared to all other CORT variables, although estimation uncertainty was high and thus the p-value fell just above the traditional significance threshold (Table 1). RMSSD over the tadpole stage did not correlate significantly with RMSSD over the toadlet stage (r = 0.28, p = 0.163, n = 26).
Table 1. Repeatability estimated as intraclass correlation coefficient (ICC) with standard error (SE) and 95% confidence interval (CI) for various aspects of the CORT profile in tadpoles and toadlets.
Figure 5. Correlations between various aspects of the CORT profile and behavioral responses to anthropogenic disturbances. Note that the variables are presented here without transformations; the outlying data points were dealt with in the statistical analyses by appropriate transformations as detailed in the Methods.
Consumption rate was significantly greater in the disturbance trials than in the control trials (main effect of trial type: χ2 = 41.59, df = 1, p < 0.001; Figure 6) and smaller during the day than during the night (main effect of time of day: χ2 = 61.2, df = 1, p < 0.001; Figure 6), but the interaction was not significant (trial type × time of day: χ2 = 2.46, df = 1, p = 0.117). Thus, toadlets responded to both ALAN and daytime noise by a similar increase in consumption rate (Figure 6). None of the CORT variables affected this response, as the three-way interaction between trial type, time of day, and each CORT variable was always non-significant (p > 0.117). The change in consumption rate from control to disturbance trials was correlated between the ALAN test and the noise test (rs = 0.42, p = 0.034, n = 25; Figure 5B).
Figure 6. Feeding success in the foraging trials of the ALAN (artificial light at night) test and the daytime noise test. Boxplots present medians, interquartile ranges, and full data ranges (middle line, box, and whiskers, respectively); error bars represent means and 84% confidence intervals estimated by a linear mixed model. Below each plot, letters mark differences with p ≤ 0.01 after FDR correction (samples marked with different letters are significantly different from each other).
All variables measured in the fleeing performance test were significantly correlated with each other (rs > 0.50, p ≤ 0.010, n = 26), except for mean distance with hop count (rs = 0.28, p = 0.170, n = 26) and with total time of hops (rs =0.26, p = 0.200, n = 26). However, none of these variables correlated significantly with the change of consumption rate in response to ALAN (r ≤ 0.22, p > 0.696, n = 25) or noise (r ≤ 0.19, p > 0.962, n = 26). Hop performance variables were uncorrelated with all CORT variables, with two exceptions. Longer average distances over the first four hops correlated significantly with lower magnitude of stress response at the second tadpole sampling occasion (rs = −0.42, p = 0.034, n = 26; Figure 5C) and with higher HPI flexibility measured as RMSSD over the tadpole stage (rs = 0.42, p = 0.033, n = 26; Figure 5D). However, neither of these relationships were significant after FDR correction for multiple testing (p > 0.806).
In this study, by measuring CORT release rates repeatedly in common toads as tadpoles and after they had metamorphosed, we investigated if individuals vary consistently in the ways they regulate their HPI axis, and whether these hormonal differences explain how they respond to their first encounter with novel anthropogenic challenges. We found low CORT repeatability and little correlation between hormonal and behavioral variables; however, several facets of our results were unexpected both for CORT profiles and for behavioral responses. While these unexpected results complicate the interpretation of our findings, they also point to some important lessons, as discussed below.
To characterize glucocorticoid regulation, we used a well-established non-invasive protocol for quantifying baseline CORT levels, the CORT response to an acute stressor, and the speed of post-stress recovery by negative feedback (Narayan, 2013; Narayan et al., 2019; Bókony et al., 2021). This approach yielded the expected CORT profile for tadpoles but not for toadlets. In the latter, CORT release rates were highest during the first sampling hour and were as low during the agitation procedure as afterwards. It is possible that the toadlets experienced greater stress at the start of sampling, when they were captured from their relatively dry home boxes and placed into shallow water. For tadpoles, this process was probably not stressful as they were swiftly scooped up from their rearing water into the sampling water. Furthermore, the second hour of sampling may have been more stressful for tadpoles than for toadlets, because the latter were better able to withstand agitation by bracing themselves to the box walls with their limbs. These considerations suggest that, in toadlets, the CORT sample of the first hour might reflect stress-induced levels whereas the second hour might reflect recovery levels. Similar problems with water-borne hormone sampling have sometimes been reported; for example, the supposedly “baseline” sample appearing to reflect stress-induced levels (Boulton et al., 2015) or water-borne measure correlating with whole-body CORT in tadpoles but not in post-metamorphic frogs (Ruthsatz et al., 2023). Altogether, these findings highlight the importance of validating the CORT-profile sampling protocol (including the technique used for eliciting acute stress) not only for each species but also for each age group or life stage (e.g. aquatic versus terrestrial). Interestingly, in another species of toad (Incilius nebulifer) and using wild-caught juveniles, Monroe et al. (2024) found the expected CORT profile in response to the same protocol that we used here, i.e. those toadlets showed a stress response to agitation and recovery thereafter. This indicates that differences between species and/or rearing environments (e.g. wild vs. captivity) can have major impact on how individuals perceive and respond to the same stressors.
Bearing these caveats in mind, we found low repeatability in all aspects of the CORT profile that we investigated, both within tadpoles and within toadlets, as well as between the two life stages. The early tadpole stress response had the highest repeatability (r = 0.3); this value is similar to the repeatability of CORT release rates found by Forsburg et al. (2019) for tadpoles measured 6 times following agitation (over an hour) using the same water-borne methods. It is also similar to the average repeatability of GC concentrations across vertebrates (Schoenemann and Bonier, 2018; Taff et al., 2018). These results mirror the conclusions from meta-analyses of vertebrate GC levels that repeatability is higher for stress responses than for baseline levels (Schoenemann and Bonier, 2018; Taff et al., 2018; Fanson and Biro, 2019). In contrast, we found the lowest repeatabilites when we included both tadpoles and toadlets. This again mirrors earlier findings that GC repeatability is lower for longer sampling intervals and higher within than across life-history stages (Schoenemann and Bonier, 2018; Taff et al., 2018; Fanson and Biro, 2019). In our case, this might have been caused by the transition from aquatic to terrestrial life stage affecting hormone secretion and/or skin permeability to steroids. The meta-analyses found that baseline and stress-induced glucocorticoid levels are often repeatable in vertebrates, although with a great deal of heterogeneity (Schoenemann and Bonier, 2018; Taff et al., 2018; Fanson and Biro, 2019), and lower repeatability is more often found in less well-known non-model organisms (Fanson and Biro, 2019). The lack of consistent individual differences may be due to the fact that all animals in our study were raised in the same environment. Developing in different environments is known to induce differences in CORT regulation between individuals (Ouyang et al., 2019; Bókony et al., 2021); lack of environmental heterogeneity could have resulted in low variance across individuals and thus in low repeatability measures. Furthermore, the environment our animals experienced was benign, with relatively few disturbances or challenges. Previous findings indicate that individuals develop consistent behavioral differences only if they experienced relevant environmental stimuli such as predation risk or sociality (Urszán et al., 2015); the same might be true for hormonal differences. In the absence of environmental variance, individuals may still express consistent differences due to heritable (genetic or transgenerational) variation. However, in our study, there was no sign of such variation between the different sibling groups, perhaps because we had accidentally sampled genetically similar families from the same pond. Thus, for future studies aiming to understand how the repeatability of various aspects of HPA/I flexibility emerges, it would be most enlightening to sample individuals from different environments and/or to expose them to different rearing conditions. Alternatively, or additionally, it is possible that the animals in our study habituated towards our regime for measuring GC profile across time, and this may have caused (or contributed to) high within-individual variation (i.e. change with age) in CORT variables, resulting in low repeatability measures. Habituation may explain the overall lower CORT release rates of toadlets compared to tadpoles, the lower stressed levels of tadpoles in the third than in the second sampling, and of toadlets in the fifth than in the sixth sampling. GC levels can habituate to repeated homotypic stressors but less so to heterotypic stressors (Dickens and Romero, 2013), so it would be worthwhile to investigate repeatability across situations with heterotypic stressors, to disentangle the effects of habituation from other effects that vary with age.
With our behavioral assays, we mimicked situations where animals experience urban noise and light pollution for the first time, as when dispersing into an urban habitat from the natural surroundings. Based on the multitude of studies documenting negative effects of these pollution forms on wildlife (Gaston et al., 2015; Owens and Lewis, 2018; Kunc and Schmidt, 2019; Sanders et al., 2020), we expected that short-term exposure to both ALAN and noise would be stressful for the toadlets, reducing their foraging success e.g. by making them try to escape or find a hiding place. However, we found just the opposite, as the toadlets consumed significantly more crickets both under ALAN and noise than in the absence of these disturbances. It is possible that the first exposure to these disturbances invoked predictive uncertainty about the environment and that might lead to high foraging rates. Another potential explanation could be that the crickets might have been more negatively affected than the toadlets, rendering them easier to capture. While young toadlets forage during both day and night, the house cricket is a nocturnal species, and cricket behavior can be disrupted by ALAN as well as by noise (Classen-Rodríguez et al., 2021; Levy et al., 2024). We cannot test this idea because we could not document the behavior of crickets in our study. However, this unexpected result underscores the importance of paying attention to predator–prey interactions and other inter-specific relationships when studying the effects of urbanization (Siemers and Schaub, 2011; Parkinson et al., 2020; Secondi et al., 2020). For example, it is possible that the common toad is relatively successful in colonizing urban habitats partly because it is more tolerant to acute sensory pollution than its prey; however, this difference might disappear or even reverse when the animals become chronically exposed to pollution. Future studies are needed to test these possibilities, and also to explore other aspects of behavioral stress response, such as to desiccation or heat shock that are particularly relevant in urban heat islands.
Interestingly, the toadlets’ behavioral responses were correlated between the ALAN and noise assays, but not with their locomotor performance when they were fleeing from a human prodding them for the first time. This result fits with the above idea that the foraging assays were probably not stressful for the toadlets, or at least not in the way they experienced being chased by a predator-like entity. If we accept this speculation that the only stressful behavioral assay was the fleeing test, and we assume that individuals’ differences in CORT regulation explain how they behave in stressful situations, then we should expect the strongest hormone–behavior correlation between fleeing performance and the most repeatable aspects of the hormonal stress response. This is indeed what we found, as longer average hop distance over the first 4 hops correlated with lower magnitude of stress response at the second tadpole sampling occasion. This correlation was mirrored by a significant positive correlation between HPI flexibility measured as RMSSD in the tadpole stage and average hop distance over the first 4 hops (although neither of these correlations were significant after correcting for the large number of correlation tests). In agreement with the latter result, the study that introduced RMSSD as a measure of HPA/I flexibility indirectly suggested that this variable correlated positively with bolder behavior (Zimmer et al., 2021). Our findings tentatively suggest that individual variation in behavioral responses to stressful anthropogenic challenges might be linked to certain aspects of glucocorticoid regulation, but the chances of detecting these links may have been hampered in our study by the low inter-individual variation of CORT profiles and the putative lack of behavioral stress in the foraging assays. Therefore, further studies are needed on the roles of endocrine and behavioral variation in the various phases of the urbanization process.
The original contributions presented in the study are included in the article/Supplementary Material. Further inquiries can be directed to the corresponding author.
The animal study was approved by Environment Protection and Nature Conservation Department of the Pest County Bureau of the Hungarian Government. The study was conducted in accordance with the local legislation and institutional requirements.
NU: Conceptualization, Data curation, Formal analysis, Investigation, Methodology, Project administration, Visualization, Writing – original draft. BB: Investigation, Methodology, Visualization, Writing – review & editing. CG: Conceptualization, Methodology, Resources, Validation, Writing – review & editing. VB: Conceptualization, Data curation, Formal analysis, Funding acquisition, Methodology, Project administration, Supervision, Visualization, Writing – review & editing.
The author(s) declare financial support was received for the research, authorship, and/or publication of this article. The study was supported by the National Research, Development and Innovation Office of Hungary (NKFIH K‐135016 and 2019‐2.1.11‐ TÉT‐2019‐00026 to VB), and NU was supported by the EKÖP-MATE/2024/25/K university research Scolarship Programme of the Ministry for Culture and Innovation from the source of the National Research, Development and Innovation Fund.
We thank Andrea Kásler and Dr. Zsanett Mikó for their help in solid phase extraction, and Dr. Ágnes Móricz for letting us use the vacuum manifold. We are grateful to Saeid Panahi Hassan Barough for help with preparing the samples for EIA. We used BioRender.com to create Figures 1–3.
The authors declare that the research was conducted in the absence of any commercial or financial relationships that could be construed as a potential conflict of interest.
All claims expressed in this article are solely those of the authors and do not necessarily represent those of their affiliated organizations, or those of the publisher, the editors and the reviewers. Any product that may be evaluated in this article, or claim that may be made by its manufacturer, is not guaranteed or endorsed by the publisher.
The Supplementary Material for this article can be found online at: https://www.frontiersin.org/articles/10.3389/famrs.2025.1500598/full#supplementary-material
Bókony V., Ujhegyi N., Hamow K., Bosch J., Thumsová B., Vörös J., et al. (2021). Stressed tadpoles mount more efficient glucocorticoid negative feedback in anthropogenic habitats due to phenotypic plasticity. Sci. Total. Environ. 753, 141896. doi: 10.1016/j.scitotenv.2020.141896
Bonier F. (2012). Hormones in the city: Endocrine ecology of urban birds. Horm. Behav. 61, 763–772. doi: 10.1016/j.yhbeh.2012.03.016
Boulton K., Couto E., Grimmer A. J., Earley R. L., Canario A. V. M., Wilson A. J., et al. (2015). How integrated are behavioral and endocrine stress response traits? A repeated measures approach to testing the stress-coping style model. Ecol. Evol. 5, 618–633. doi: 10.1002/ece3.1395
Cartwright M., Mendez A. E. M., Cramer J., Lostanlen V., Dove G., Wu H.-H., et al. (2019). SONYC Urban Sound Tagging (SONYC-UST): A Multilabel Dataset from an Urban Acoustic Sensor Network (New York, USA: New York University), 35–39. doi: 10.33682/j5zw-2t88
Ceballos G., Ehrlich P. R., Dirzo R. (2017). Biological annihilation via the ongoing sixth mass extinction signaled by vertebrate population losses and declines. Proc. Natl. Acad. Sci. U.S.A. 114, E6089–E6096. doi: 10.1073/pnas.1704949114
Classen-Rodríguez L., Tinghitella R., Fowler-Finn K. (2021). Anthropogenic noise affects insect and arachnid behavior, thus changing interactions within and between species. Curr. Opin. Insect Sci. 47, 142–153. doi: 10.1016/j.cois.2021.06.005
Cogălniceanu D., Crnobrnja-Isailović J., Ficetola F., Halpern B., Kornilev Y., Krása A., et al (2024). Bufo bufo (Europe assessment). The IUCN Red List of Threatened Species 2024: e.T88316072A200188858. doi: 10.2305/IUCN.UK.2024-2.RLTS.T88316072A200188858.en
Davis A. K., Connell L. L., Grosse A., Maerz J. C. (2008). A fast, non-invasive method of measuring growth in tadpoles using image analysis. Herpetol. Rev. 39, 56–58.
Dickens M. J., Romero L. M. (2013). A consensus endocrine profile for chronically stressed wild animals does not exist. Gen. Comp. Endocrinol. 191, 177–189. doi: 10.1016/j.ygcen.2013.06.014
Dobretsov M., Petkau G., Hayar A., Petkau E. (2017). Clock scan protocol for image analysis: ImageJ plugins. J. Visualized. Experiments. 2017 (124), e55819. doi: 10.3791/55819
Falcón J., Torriglia A., Attia D., Viénot F., Gronfier C., Behar-Cohen F., et al. (2020). Exposure to artificial light at night and the consequences for flora, fauna, and ecosystems. Front. Neurosci. 14. doi: 10.3389/fnins.2020.602796
Fanson K. V., Biro P. A. (2019). Meta-analytic insights into factors influencing the repeatability of hormone levels in agricultural, ecological, and medical fields. Am. J. Physiol. Regul. Integr. Comp. Physiol. 316, 101–109. doi: 10.1152/ajp
Fokidis H. B., Deviche P. (2011). Plasma corticosterone of city and desert Curve-billed Thrashers, Toxostoma curvirostre, in response to stress-related peptide administration. Comp. Biochem. Physiol. - A. Mol. Integr. Physiol. 159, 32–38. doi: 10.1016/j.cbpa.2011.01.011
Forsburg Z. R., Goff C. B., Perkins H. R., Robicheaux J. A., Almond G. F., Gabor C. R. (2019). Validation of water-borne cortisol and corticosterone in tadpoles: Recovery rate from an acute stressor, repeatability, and evaluating rearing methods. Gen. Comp. Endocrinol. 281, 145–152. doi: 10.1016/j.ygcen.2019.06.007
French S. S., Webb A. C., Hudson S. B., Virgin E. E. (2018). Town and country reptiles: A review of reptilian responses to urbanization. Integr. Comp. Biol. 58, 948–966. doi: 10.1093/icb/icy052
Friard O., Gamba M. (2016). BORIS: a free, versatile open-source event-logging software for video/audio coding and live observations. Methods Ecol. Evol. 7, 1325–1330. doi: 10.1111/2041-210X.12584
Fürtbauer I., Pond A., Heistermann M., King A. J. (2015). Personality, plasticity and predation: Linking endocrine and behavioural reaction norms in stickleback fish. Funct. Ecol. 29, 931–940. doi: 10.1111/1365-2435.12400
Gabor C. R., Bosch J., Fries J. N., Davis D. R. (2013a). A non-invasive water-borne hormone assay for amphibians. Amphibia. Reptilia. 34, 151–162. doi: 10.1163/15685381-00002877
Gabor C. R., Fisher M. C., Bosch J. (2013b). A non-invasive stress assay shows that tadpole populations infected with batrachochytrium dendrobatidis have elevated corticosterone levels. PloS One 8, 1–5. doi: 10.1371/journal.pone.0056054
Gaston K. J., Visser M. E., Ho F., Gaston K. J. (2015). The biological impacts of artificial light at night: the research challenge. Philos. Trans. R. Soc. B.: Biol. Sci. 370, 20140133. doi: 10.1098/rstb.2014.0133
Glennemeier K. A., Denver R. J. (2002). Developmental Changes in Interrenal Responsiveness in Anuran Amphibians. Integrative and Comparative Biology 42, 565–573. doi: 10.1093/icb/42.3.565
Goater C. P., Semlitsch R. D., Bernasconi M. V. (1993). Effects of body size and parasite infection on the locomotory performance of juvenile toads, bufo bufo. Oikos 66, 129. doi: 10.2307/3545205
Gosner K. L. (1960). A simplified table for staging anuran embryos larvae with notes on identification. Herpetologica 16, 183–190. doi: 10.2307/3890061
Guindre-Parker S. (2018). The evolutionary endocrinology of circulating glucocorticoids in free-living vertebrates: Recent advances and future directions across scales of study. Integr. Comp. Biol. 58, 814–825. doi: 10.1093/icb/icy048
Hau M., Casagrande S., Ouyang J. Q., Baugh A. T. (2016). Glucocorticoid-mediated phenotypes in vertebrates: multilevel variation and evolution. Adv. Study. Behav. 48, 41–115. doi: 10.1016/bs.asb.2016.01.002
Hölker F., Bolliger J., Davies T. W., Giavi S., Jechow A., Kalinkat G., et al. (2021). 11 pressing research questions on how light pollution affects biodiversity. Front. Ecol. Evol. 9. doi: 10.3389/fevo.2021.767177
Hudson C. M., Vidal-García M., Murray T. G., Shine R. (2020). The accelerating anuran: evolution of locomotor performance in cane toads (Rhinella marina. Proc. R. Soc. B.: Biol. Sci. 287, 20201964. doi: 10.1098/rspb.2020.1964
Iglesias-Carrasco M., Aich U., Jennions M. D., Head M. L. (2020). Stress in the city: meta-analysis indicates no overall evidence for stress in urban vertebrates. Proc. R. Soc. B.: Biol. Sci. 287, 20201754. doi: 10.1098/rspb.2020.1754
Injaian A. S., Francis C. D., Ouyang J. Q., Dominoni D. M., Donald J. W., Fuxjager M. J., et al. (2020). Baseline and stress-induced corticosterone levels across birds and reptiles do not reflect urbanization levels. Conserv. Physiol. 8 (1), coz110. doi: 10.1093/conphys/coz110
Jaffe R. C. (1981). Plasma Concentration of Corticosterone during Rana catesbeiana Tadpole Metamorphosis. 44 (3), 314–318. doi: 10.1016/0016-6480(81)90007-1
Kikuyama S., Kawamura K., Tanaka S., Yamamoto K. (1993). Aspects of amphibian metamorphosis: hormonal control. 145, 105–148. doi: 10.1016/S0074-7696(08)60426-X
Kolonin A. M., Bókony V., Bonner T. H., Zúñiga-Vega J. J., Aspbury A. S., Guzman A., et al. (2022). Coping with urban habitats via glucocorticoid regulation: physiology, behavior, and life history in stream fishes. Integr. Comp. Biol. 62, 90–103. doi: 10.1093/icb/icac002
Kunc H. P., Schmidt R. (2019). The effects of anthropogenic noise on animals: A meta-analysis. Biol. Lett. 15, 20190649. doi: 10.1098/rsbl.2019.0649
Lattin C. R., Kelly T. R. (2020). Glucocorticoid negative feedback as a potential mediator of trade-offs between reproduction and survival. Gen. Comp. Endocrinol. 283, 113301. doi: 10.1016/j.ygcen.2019.113301
Lendvai Á.Z., Ouyang J. Q., Schoenle L. A., Fasanello V., Haussmann M. F., Bonier F., et al. (2014). Experimental food restriction reveals individual differences in corticosterone reaction norms with no oxidative costs. PloS One 9 (11), e110564. doi: 10.1371/journal.pone.0110564
Levy K., Barnea A., Tauber E., Ayali A. (2024). Crickets in the spotlight: exploring the impact of light on circadian behavior. J. Comp. Physiol. A. Neuroethol. Sens. Neural Behav. Physiol. 210, 267–279. doi: 10.1007/s00359-023-01686-y
Martin J. G. A., Nussey D. H., Wilson A. J., Réale D. (2011). Measuring individual differences in reaction norms in field and experimental studies: A power analysis of random regression models. Methods Ecol. Evol. 2, 362–374. doi: 10.1111/j.2041-210X.2010.00084.x
Merckx T., Souffreau C., Kaiser A., Baardsen L. F., Backeljau T., Bonte D., et al. (2018). Body-size shifts in aquatic and terrestrial urban communities. Nature 558, 113–116. doi: 10.1038/s41586-018-0140-0
Monroe D. J., Bókony V., Gabor C. R. (2024). Physiological and behavioral variation by urbanization and climate in an urban-tolerant toad. Front. Amphibian. Reptile. Sci. 2, 1444580. doi: 10.3389/famrs.2024.1444580
Nakagawa S., Schielzeth H. (2010). Repeatability for Gaussian and non-Gaussian data: A practical guide for biologists. Biol. Rev. 85, 935–956. doi: 10.1111/j.1469-185X.2010.00141.x
Narayan E. J. (2013). Non-invasive reproductive and stress endocrinology in amphibian conservation physiology. Conserv. Physiol. 1, 1–16. doi: 10.1093/;conphys/cot011
Narayan E. J., Cockrem J. F., Hero J. M. (2013). Repeatability of baseline corticosterone and short-term corticosterone stress responses, and their correlation with testosterone and body condition in a terrestrial breeding anuran (Platymantis vitiana). Comp. Biochem. Physiol. - A. Mol. Integr. Physiol. 165, 304–312. doi: 10.1016/j.cbpa.2013.03.033
Narayan E. J., Forsburg Z. R., Davis D. R., Gabor C. R. (2019). Non-invasive methods for measuring and monitoring stress physiology in imperiled amphibians. Front. Ecol. Evol. 7. doi: 10.3389/fevo.2019.00431
Ouyang J. Q., Baldan D., Munguia C., Davies S. (2019). Genetic inheritance and environment determine endocrine plasticity to urban living. Proc. R. Soc. B.: Biol. Sci. 286, 20191215. doi: 10.1098/rspb.2019.1215
Owens A. C. S., Lewis S. M. (2018). The impact of artificial light at night on nocturnal insects: A review and synthesis. Ecol. Evol. 8, 11337–11358. doi: 10.1002/ece3.4557
Park J., Do Y. (2023). Phenotypic plasticity in juvenile frogs that experienced predation pressure as tadpoles does not alter their locomotory performance. Biol. (Basel). 12 (3), 341. doi: 10.3390/biology12030341
Parkinson E., Lawson J., Tiegs S. D. (2020). Artificial light at night at the terrestrial-aquatic interface: Effects on predators and fluxes of insect prey. PloS One 15 (10), e0240138. doi: 10.1371/journal.pone.0240138
Partecke J., Schwabl I., Gwinner E. (2006). Stress and the city: urbanization and its effects on the stress physiology in european blackbirds. Ecology 87, 1945–1952. doi: 10.1890/0012-9658(2006)87[1945:SATCUA]2.0.CO;2
Pike N. (2011). Using false discovery rates for multiple comparisons in ecology and evolution. Methods Ecol. Evol. 2, 278–282. doi: 10.1111/j.2041-210X.2010.00061.x
R Core Team. (2023). R: A language and environment for statistical computing. R Foundation for Statistical Computing, Vienna, Austria. Available online at: http://www.r-project.org.
Romero L. M. (2004). Physiological stress in ecology: Lessons from biomedical research. Trends Ecol. Evol. 19, 249–255. doi: 10.1016/j.tree.2004.03.008
Romero L. M., Dickens M. J., Cyr N. E. (2009). The reactive scope model - A new model integrating homeostasis, allostasis, and stress. Horm. Behav. 55, 375–389. doi: 10.1016/j.yhbeh.2008.12.009
Ruthsatz K., Rico-Millan R., Eterovick P. C., Gomez-Mestre I. (2023). Exploring water-borne corticosterone collection as a non-invasive tool in amphibian conservation physiology: benefits, limitations and future perspectives. Conserv. Physiol. 11 (1), coad070. doi: 10.1093/conphys/coad070
Samia D. S. M., Nakagawa S., Nomura F., Rangel T. F., Blumstein D. T. (2015). Increased tolerance to humans among disturbed wildlife. Nat. Commun. 6, 8877. doi: 10.1038/ncomms9877
Sanabria E. A., Quiroga L. B., González E., Moreno D., Cataldo A. (2013). Thermal parameters and locomotor performance in juvenile of Pleurodema nebulosum (Anura: Leptodactylidae) from the Monte Desert. J. Therm. Biol. 38, 390–395. doi: 10.1016/j.jtherbio.2013.05.005
Sanders D., Frago E., Kehoe R., Patterson C., Gaston K. J. (2021). A meta-analysis of biological impacts of artificial light at night. Nat. Ecol. Evol. 5, 74–81. doi: 10.1038/s41559-020-01322-x
Sapolsky R. M., Romero L. M., Munck A. U. (2000). How do glucocorticoids influence stress responses? Integrating permissive, suppressive, stimulatory, and preparative actions. Endocr. Rev. 21, 55–89. doi: 10.1210/er.21.1.55
Schneider C. A., Rasband W. S., Eliceiri K. W. (2012). NIH Image to ImageJ: 25 years of image analysis. Nature methods 9, 671–675. doi: 10.1038/nmeth.2089
Schoenemann K. L., Bonier F. (2018). Repeatability of glucocorticoid hormones in vertebrates: A meta-analysis. PeerJ 6, e4398. doi: 10.7717/peerj.4398
Secondi J., Davranche A., Théry M., Mondy N., Lengagne T. (2020). Assessing the effects of artificial light at night on biodiversity across latitude – Current knowledge gaps. Global Ecol. Biogeogr. 29, 404–419. doi: 10.1111/geb.13037
Sepp T., McGraw K. J., Kaasik A., Giraudeau M. (2018). A review of urban impacts on avian life-history evolution: Does city living lead to slower pace of life? Glob. Chang. Biol. 24, 1452–1469. doi: 10.1111/gcb.13969
Seress G., Liker A. (2015). Habitat urbanization and its effects on birds. Acta Zool. Acad. Scientiarum. Hungaricae. 61, 373–408. doi: 10.17109/AZH.61.4.373.2015
Siemers B. M., Schaub A. (2011). Hunting at the highway: Traffic noise reduces foraging efficiency in acoustic predators. Proc. R. Soc. B.: Biol. Sci. 278, 1646–1652. doi: 10.1098/rspb.2010.2262
Smith M. A., Green D. M. (2005). Dispersal and the metapopulation paradigm in amphibian ecology and conservation: Are all amphibian populations metapopulations? Ecography 28, 110–128. doi: 10.1111/j.0906-7590.2005.04042.x
Sol D., Lapiedra O., González-Lagos C. (2013). Behavioural adjustments for a life in the city. Anim. Behav. 85, 1101–1112. doi: 10.1016/j.anbehav.2013.01.023
Sordello R., Ratel O., de Lachapelle F. F., Leger C., Dambry A., Vanpeene S. (2020). Evidence of the impact of noise pollution on biodiversity: A systematic map. Environ. Evid. 9, 1–27. doi: 10.1186/s13750-020-00202-y
Taff C. C., Schoenle L. A., Vitousek M. N. (2018). The repeatability of glucocorticoids: A review and meta-analysis. Gen. Comp. Endocrinol. 260, 136–145. doi: 10.1016/j.ygcen.2018.01.011
Urszán T. J., Garamszegi L. Z., Nagy G., Hettyey A., Török J., Herczeg G. (2015). No personality without experience? A test on Rana dalmatina tadpoles. Ecol. Evol. 5, 5847–5856. doi: 10.1002/ece3.1804
van de Pol M. (2012). Quantifying individual variation in reaction norms: How study design affects the accuracy, precision and power of random regression models. Methods Ecol. Evol. 3, 268–280. doi: 10.1111/j.2041-210X.2011.00160.x
Vincze E., Kovács B. (2022). Urbanization’s effects on problem solving abilities: A meta-analysis. Front. Ecol. Evol. 10. doi: 10.3389/fevo.2022.834436
Vitousek M. N., Taff C. C., Hallinger K. K., Zimmer C., Winkler D. W. (2018). Hormones and fitness: Evidence for trade-offs in glucocorticoid regulation across contexts. Front. Ecol. Evol. 6, 59. doi: 10.3389/fevo.2018.00042
Vitousek M. N., Taff C. C., Ryan T. A., Zimmer C. (2019). Stress resilience and the dynamic regulation of glucocorticoids. Integr. Comp. Biol. 59, 251–263. doi: 10.1093/icb/icz087
Zamora-Camacho F. J. (2018). Locomotor performance in a running toad: Roles of morphology, sex and agrosystem versus natural habitat. Biol. J. Linn. Soc. 123, 411–421. doi: 10.1093/biolinnean/blx147
Zimmer C., Hanson H. E., Martin L. B. (2021). FKBP5 expression is related to HPA flexibility and the capacity to cope with stressors in female and male house sparrows. Horm. Behav. 135, 105038. doi: 10.1016/j.yhbeh.2021.105038
Keywords: ALAN, corticosterone, stress response, coping capacity, escaping behavior
Citation: Ujhegyi N, Bombay B, Gabor CR and Bókony V (2025) Unexpected hormonal and behavioral responses to anthropogenic stressors in young common toads. Front. Amphib. Reptile Sci. 3:1500598. doi: 10.3389/famrs.2025.1500598
Received: 23 September 2024; Accepted: 18 February 2025;
Published: 10 March 2025.
Edited by:
Duminda S. B. Dissanayake, University of Canberra, AustraliaCopyright © 2025 Ujhegyi, Bombay, Gabor and Bókony. This is an open-access article distributed under the terms of the Creative Commons Attribution License (CC BY). The use, distribution or reproduction in other forums is permitted, provided the original author(s) and the copyright owner(s) are credited and that the original publication in this journal is cited, in accordance with accepted academic practice. No use, distribution or reproduction is permitted which does not comply with these terms.
*Correspondence: Nikolett Ujhegyi, dWpoZWd5aS5uaWtvbGV0dEBnbWFpbC5jb20=
Disclaimer: All claims expressed in this article are solely those of the authors and do not necessarily represent those of their affiliated organizations, or those of the publisher, the editors and the reviewers. Any product that may be evaluated in this article or claim that may be made by its manufacturer is not guaranteed or endorsed by the publisher.
Research integrity at Frontiers
Learn more about the work of our research integrity team to safeguard the quality of each article we publish.