- 1Department of Biological Sciences, Darrin Freshwater Institute, Rensselaer Polytechnic Institute, Troy, NY, United States
- 2Biology Department, Skidmore College, Saratoga Springs, NY, United States
In the fight against emerging pathogens, an important management strategy is to identify host refuges to prevent extinctions. Fungal pathogens (Batrachochytrium) are decimating amphibians around the world, but researchers have discovered that coastal amphibians living in high-salt water bodies can experience a refuge because the pathogens have lower survival outside the host in these conditions. This has led to the recommendation that managers should create disease refuges for amphibians by adding salt to water bodies. We review this literature and highlight that while some populations of coastal amphibians have evolved increased salt tolerance, most amphibian populations around the world have not. The relatively high concentrations of salt that are needed to kill the pathogens would cause widespread direct and indirect harm on amphibians and would kill most other aquatic taxa. Thus, intentionally salinizing freshwater habitats is a well-intentioned management strategy, but it is not a well-advised strategy.
Introduction
Emerging pathogens are a growing concern as new diseases are appearing at a rate of nearly one per year since the 1970s (Relyea, 2021) and causing declines in biodiversity and losses of ecosystem function. Such diseases include avian influenza, wasting diseases in deer and sea stars, white-nose syndrome in bats, and chytridiomycosis in amphibians. These pathogens can be native or invasive, but as each new pathogen emerges and spreads, biologists have struggled to control their spread and mitigate their impact. The challenge in such management efforts is to assess the full suite of potential impacts to minimize harm to the species we wish to protect. Borrowing from the physician’s Hippocratic oath, we need to ensure that we “first, do no harm.”
A common approach to protect species from emerging pathogens is to identify habitat refuges where hosts experience reduced infections. Often such refuges are extreme environments where a host can persist but the pathogen or its vector cannot (Springer, 2009). Such extreme environments are not optimal for the host’s growth and survival (Gsell et al., 2023). For instance, oysters (Crassostrea virginica) living in low-salinity estuaries experience reduced growth and reproduction, but they experience fewer infections from two invasive marine parasites (Haplosporidium nelsoni and Perkinsus marinus) because parasite survival is reduced (Levinton et al., 2011). These refuges allow the host species to persist until we find ways to control the pathogen.
When refuges from pathogens are scarce, we can consider creating refuges through habitat modifications. Creating refuges from stressors has a long history, such as predator controls to protect vulnerable prey populations (Reside et al., 2019) or habitat manipulations to favor the enemies of crop pests (Landis et al., 2000). While refuges can be created through minimal change to the environment (e.g., artificial hotspot shelters in [Waddle et al., 2024]), modifying habitat has recently gained momentum for protecting hosts from emerging wildlife pathogens. However, habitat manipulations can have widespread, cascading effects on ecological communities, so we need a holistic understanding of how communities will respond. Unfortunately, such assessments have rarely been conducted (Langwig et al., 2015). Here, we review recent ideas and concerns regarding creating host refuges for amphibians using salt as a management tool.
The global impact of chytrid pathogens on amphibians
Amphibians face a number of pathogens including two species of chytrid fungus (Batrachochytrium dendrobatidis [Bd] and B. salamandrivorans [Bsal]), which cause the disease chytridiomycosis. The global pandemic lineage of Bd (GPL) and the only known lineage of Bsal are both thought to have originated in Asia and transported to other continents via the commercial pet trade (O’Hanlon et al., 2018).
Bd was first reported in Central America in 1998 (Berger et al., 1998) and has now been found on all six continents that contain amphibians. Given that amphibians in these other regions of the world have no evolutionary history with chytrid fungi, the amphibians commonly have no defenses. It has infected >500 species and may have contributed to the decline of hundreds of species, with dozens going extinct or presumably extinct (Scheele et al., 2019; but see Lambert et al., 2020).
Bsal was discovered in 2013 in Europe where it caused high mortality in fire salamanders (Salamandra salamandra; Martel et al., 2013; Spitzen-van der Sluijs et al., 2013; Richgels et al., 2016). As a result of the pet trade, there is a high probability that Bsal will spread globally (if not already present). This is a major concern in North America, which contains the highest diversity of salamander species in the world. Bsal appears to pose a major risk to this biodiversity hotspot, given recent evidence that Bsal can infect 25 of 35 North American species, spanning five salamander and four frog families, with salamanders exhibiting the highest mortality (Martel et al., 2014; Gray et al., 2023).
The need for refuges from Bd and Bsal
Given the many amphibian population declines likely caused by Batrachochytrium pathogens, there has been a growing effort to identify natural refuges. The main strategies proposed include reducing the abundance of environmental zoospores and reducing direct contact between uninfected and infected individuals (Courtois et al., 2017). Models suggest that host extinction dynamics are determined by the environmental zoospore pool, host resistance, and host tolerance (e.g., Wilber et al., 2017). Importantly, these models suggest that if refuges were less hospitable to zoospores, there would be a reduction of infection, but not an elimination because tolerant individuals can still transmit through direct contact (Kilpatrick et al., 2010). Thus, an ideal refuge would reduce the zoospore pool, tolerant host infections, and direct contact, while also increasing the resistance of susceptible hosts. The environmental conditions that favor these goals depend on the physiological optima of each species (consider the thermal mismatch hypothesis; Cohen et al., 2017). Finding the best refuge conditions for multiple cohabiting species will likely present trade-offs between these four processes that drive disease-induced declines.
The proposition for salinity refuges from Bd and Bsal
In recent years, researchers have proposed strategies to combat Bd and Bsal (Scheele et al., 2014 [see Table 1 ], Woodhams et al., 2018 [see Table 2 ]). These proposed strategies include eliminating reservoir species, intervening to clear infections, captive breeding, and using chemicals such as fungicides and salts to inhibit the fungal pathogens.
Salt acts as a fungal inhibitor by interfering with osmotic gradients (Blomberg and Adler, 1993), so it has been proposed as a management strategy by some amphibian biologists to make a water body inhospitable to Bd and Bsal. Stockwell et al. (2015) discovered that 3–4 ppt of NaCl slows Bd growth and zoospore motility in culture, but growth is not halted at 5 ppt. Concentrations above 5 ppt have not been tested in culture, except for confirming that 50 ppt of NaCl is lethal to Bd (Johnson et al., 2003). Bsal is more salt tolerant than Bd, maintaining growth from 0 to 6 ppt; growth drops to less than half at 8 ppt and is nearly zero at 14 ppt (Woodhams et al., 2018, Supplementary Figure 2, note: no statistics reported). For comparison, the salinity of the ocean is 35 ppt.
Based on these findings, researchers are promoting salinity as a management tool to mitigate impacts of Bd/Bsal, where it is feasible, well advised, and allowed by regulatory agencies (Stockwell et al., 2014; Clulow et al., 2018; Heard et al., 2018; Woodhams et al., 2018; Garner et al., 2016). The problem is that relatively few situations exist where these three criteria will be met. Given that most freshwater bodies around the world have salinities of < 0.1 ppt, the level of salinity required to inhibit or kill Bd and Bsal is much higher than observed in freshwater habitats.
The effectiveness of salt in reducing Bd infection and mortality
For amphibian populations and species that tolerate high-salinity water bodies, these habitats can serve as refuges from Bd. For example, field surveys of natterjack toad (Epidalea calamita) populations—which inhabit coastal wetlands and display high salinity tolerance (10 ppt; Gomez-Mestre and Tejedo, 2003)—found a negative correlation between conductivity (a measure of salinity) and Bd prevalence, though more research is warranted given the limited sample size (Bramwell, 2011). In Australia, most relict populations of Bd-susceptible Litoria aurea occur within 500 m of coast, so brackish waters have been suggested as a refuge (Pyke and White, 2001). Similarly, L. raniformis has experienced Bd-related declines, and Heard et al. (2014) found a negative correlation between conductivity and infection probability or intensity (although the 95% CIs overlapped with zero). Stockwell et al. (2014) showed further support by adding 0, 2, or 4 ppt of salt to constructed pools and stocking them with uninfected L. aurea tadpoles, allowing Bd infections to occur from local sources. After 1 year, frogs metamorphosing from the 0 ppt pools had the lowest survival and the highest infection prevalence (27%). There was improved survival and reduced infection prevalence in the 2 ppt pools (14%) and 4 ppt pools (3%). This suggests that a salty environment can serve as a Bd refuge for salt-tolerant amphibian species. Notably, these salt manipulations negatively impacted larval dragonflies and damselflies, which is a topic we explore in more detail below.
In some salt-tolerant populations, salty water does not always provide protection from Bd. For instance, when the salt-tolerant Crinia signifera was exposed to Bd and 0.75 to 5 ppt of salt for 4 wks, there were no difference in Bd infection intensity or prevalence (Besedin et al., 2022). Clulow et al. (2018) used outdoor mesocosms with either low (0.5 ppt) or high (3.5-4.5 ppt) salinity and then added exposed and unexposed adult frogs (L. aurea). After 23 weeks, exposed frogs had very low survival (1 to 4%) regardless of treatment, and prevalence did not differ, but survival of initially uninfected frogs was greater with high salt (34%) than low salt (20%).
Sometimes salinity effects can be non-linear. For example, Stockwell et al. (2012), exposed L. peronii to a range of salt concentrations and found that only intermediate concentrations reduced infection loads and mortality rates. At low (0 ppt) and high (5 ppt) salt concentrations, individuals experienced high infection loads and high mortality. Such non-linear responses suggest that management strategies must walk a tight line between helping and causing harm.
Salt-adapted populations also do not always prefer higher salinity pools. For example, Klop-Toker et al. (2017) assessed the salinity preference of L. aurea adults. In a laboratory behavioral experiment, Bd-exposed frogs exhibited no preference for high-salinity water. In outdoor pools, there was no relationship between Bd load or infection status with frog preference for low- or high-salt pools. The researchers also noted the difficulty in maintaining salt concentrations in the outdoor pools; their targeted concentration of 3 ppt fluctuated between 0.5 and 9 ppt.
The impacts of salinization on amphibians
When estimating impacts of elevated salinity on amphibians, we must consider a population’s evolutionary history; several genera contain populations inhabiting high salinity conditions, suggesting these osmoregulatory adaptations are likely a derived trait. Hopkins and Brodie (2015) reviewed the amphibian species living in naturally saline habitats—such as coastal wetlands, saline hot springs, and temporary desert ponds—and found that about 2% of more than 7,300 amphibian species had populations adapted to elevated salinity. Of the 42 salt-tolerant species that were categorized by the authors as “comprehensively studied” with measured tolerance, only 25 species could tolerate >8 ppt and only 5 species could tolerate >14 ppt salinity. Thus, many of these salt-adapted species are not able to survive proposed Bsal refuge conditions. Moreover, this is likely an overestimate of species for which this strategy could work, given that coastal populations can be locally adapted to higher salinity while inland freshwater populations display no such capacity and die at lower concentrations (e.g., Gomez-Mestre and Tejedo, 2003; Albecker and McCoy, 2017). Collectively, this suggests that chytrid refuges can be tolerated by only a small fraction of amphibian species and only a subset of their populations.
In an analysis of salt tolerance data for many aquatic species, Brady et al. (2017) examined 13 North American amphibian species that do not inhabit saline habitats. They found that all 13 species had LC50 values (i.e., the concentration required to kill 50% of a test population) below 6 ppt and one species had an LC50 of 0.2 ppt. One would predict that the salt would need to be 10% of this value (<0.6 ppt) to cause no death in these amphibians. In a meta-analysis of 39 studies of freshwater and brackish-adapted anuran species’ survivorship to salt exposure, Albecker and McCoy (2017) estimated the LC50 for anuran eggs at 4.2 ppt, larvae at 5.5 ppt, and adults at 9.0 ppt. Collectively, these data confirm that freshwater anurans are generally salt-sensitive and have a lower salt tolerance than Bd and Bsal.
Even small changes in salt concentrations can cause harmful sublethal effects for freshwater amphibian populations. So far, exposures at sublethal concentrations have demonstrated increased energy consumption, sex changes, stunted growth, sluggish behaviors, dysregulated stress responses, reduced immune function, altered gut microbiomes, and susceptibility to other pathogens (Hall et al., 2017; Lambert et al., 2017; Hughey et al., 2023; Buss and Hua, 2018; Tornabene et al., 2021). Depending on the species’ density dependent effects, these sublethal effects can have demographic consequences (e.g., Karraker et al., 2008). Given that exposure to high salinity caused more severe and more lethal ranavirus infections in Rana sylvatica tadpoles and salinity was positively correlated with the incidence of ranavirus mortality events (>95% tadpole mortality; Hall et al., 2020), creating refuges from one pathogen could cause increased mortality from another (Figure 1).
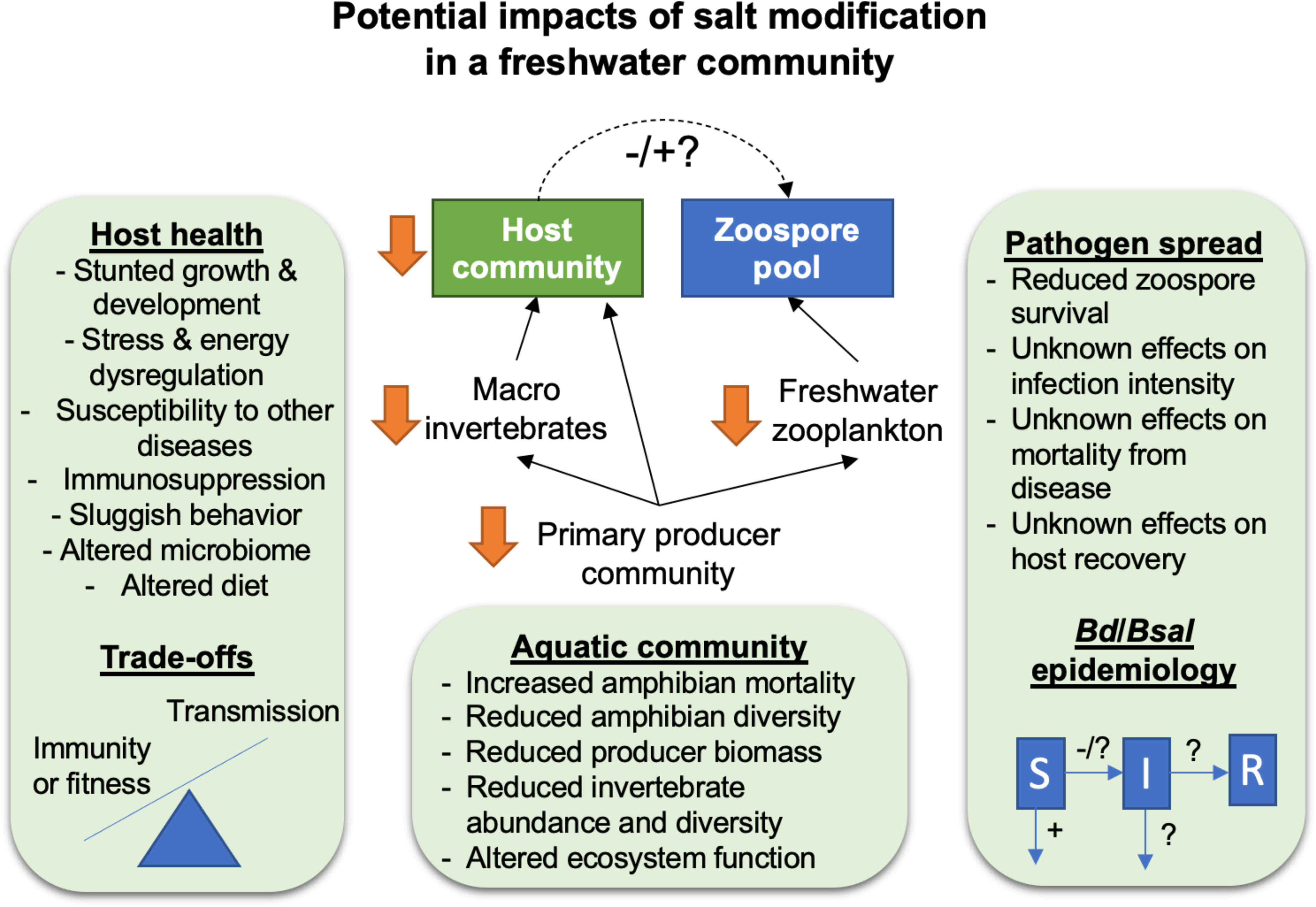
Figure 1. Impacts of salt additions to mitigate Bd and Bsal in a freshwater community. Using salt additions to reduce the abundance of zoospores has the unintended consequence of altering food webs, altering ecosystem function, negatively impacting amphibian host health, and unknown effects on disease dynamics and epidemiology. A simplified freshwater food web shows losses of primary producers, prey for amphibians, and predators of pathogen zoospores (freshwater zooplankton) with salt modification.
The impacts of salinization on freshwater ecosystems
While promoting the use of salinization against pathogens for amphibian conservation, there has been little discussion of the unintended impacts of salinization on the rest of the food web in freshwater ecosystems, other than suggesting caution about potential negative impacts. As Stockwell et al. (2015) concluded, “For the creation of salt refuges, this would involve confirmation that … this use will result in desired outcomes for survival and no detrimental effect on target and non-target organisms.” Similar cautionary notes were offered by Stockwell et al. (2014), Clulow et al. (2018), and Heard et al. (2018). When the above studies were written, concerns about biodiversity loss due to the issue of secondary salinization were starting to be raised across the globe (e.g., Kaushal et al., 2005; Karraker et al., 2008). Secondary salinization refers to recent elevated salinity in freshwater ecosystems from agricultural clearing, irrigation, mining, seawater intrusion, and the application of deicing salts in cold regions of the world. However, there has been an exponential increase in research on how secondary salinization affects individuals, populations, communities, and ecosystems (reviewed in Hintz and Relyea, 2019, Hintz et al., 2022b) and our current understanding is that the proposed salt concentrations can cause direct lethal effects, sublethal effects, and indirect effects through a food web.
While a few species of amphibians have exhibited modest levels of evolved tolerance to salt (Brady, 2012; Relyea et al., 2024), the lack of evolutionary pressures to tolerate high salinities is widespread in freshwater plants, animals, and algae, as they all perform quite poorly under high saline conditions (Hintz and Relyea, 2019 and references therein). A recent collaboration of 16 research groups around the world has found that LC50 values for zooplankton can be as low as 0.1 ppt Cl-, demonstrating that freshwater zooplankton can be exceptionally sensitive to salt (Hintz et al., 2022a). This is important because zooplankton and algae form the base of aquatic food webs, including serving as the food for larval anurans and salamanders. Among macroinvertebrates, LC50 values are quite variable, but many are below 1 ppt Cl-. With some evidence that invertebrates can be predators of Bd zoospores (Schmeller et al., 2014), elevated salt could reduce this benefit from a diverse food web.
In short, just as high concentrations of salt will make freshwater habitats inhospitable to Bd and Bsal, it can also make water bodies inhospitable to most freshwater species of plants and animals (Figure 2). This is why the U.S. EPA has set the acute threshold for salt exposure (i.e. short-term) at 0.86 ppt Cl- and the chronic threshold (i.e. long-term) at 0.23 ppt Cl- (Benoit and Stephan, 1988). In Canada, the guidelines are more conservative, with an acute threshold of 0.64 ppt CL- and a chronic threshold of 0.12 ppt Cl- (Canadian Council of Ministers of the Environment, 2011). As noted in a recent review, other regions of the world, including most European countries, have not set chloride thresholds (Schuler et al., 2019). However, these safety thresholds were developed before the research on salinization rapidly grew. We now appreciate that these thresholds need to be even lower to protect freshwater taxa. Further, even salt-adapted species experience consequences of living in salt pools such as reduced survival and growth (e.g., Gomez-Mestre and Tejedo, 2003; Wu and Kam, 2009). If managers seek to create a refuge from disease through adaptation, why not assist in the evolution of resistance to chytridiomycosis rather than hoping for the evolution of salt tolerance?
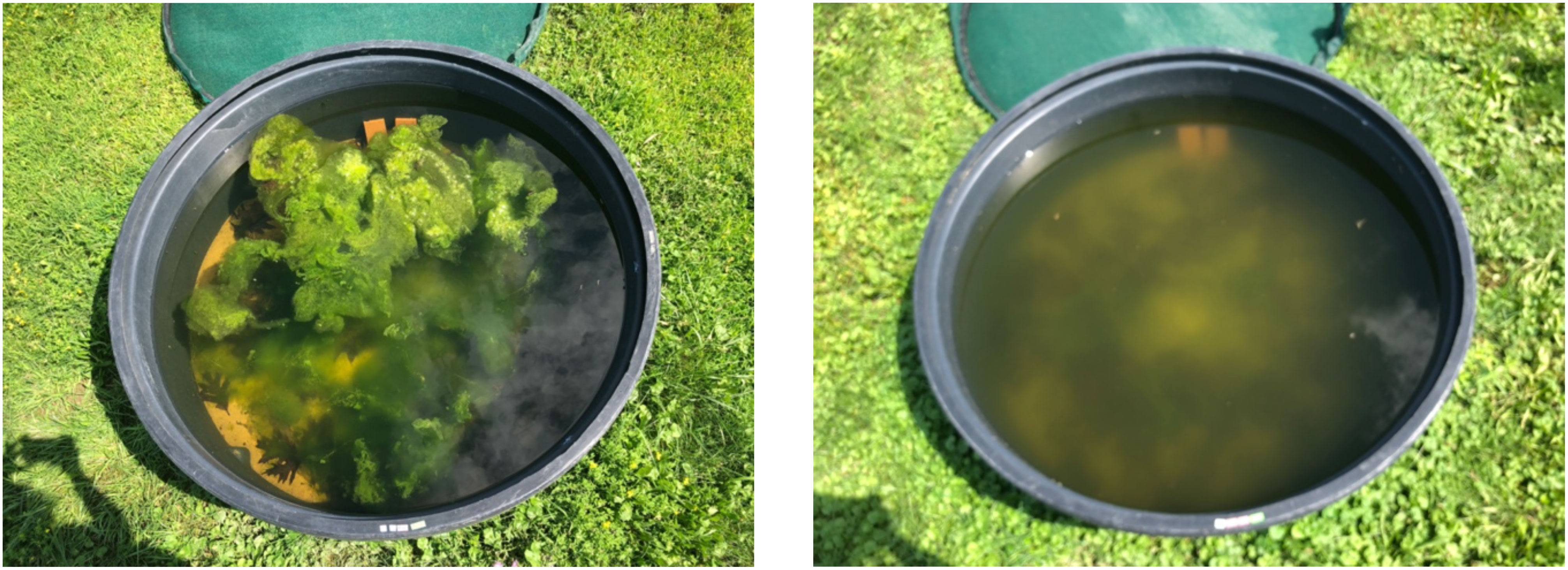
Figure 2. The impacts of adding salt to water bodies. The experimental mesocosm on the left is the control, containing 15 mg/L of chloride. The mesocosm on the right contains 1600 mg/L of chloride. The two photos illustrate the dramatic effects of adding salt to aquatic food webs. High salt concentrations eliminate filamentous algae, aquatic plants, and many of the zooplankton species that normally consume the floating phytoplankton. These changes have a multitude of cascading effects throughout the food web, including on amphibians and their pathogens. Photos by Kayla Coldsnow.
Conclusions
The search for management strategies to mitigate Bd and Bsal impacts is a high priority for amphibian conservation and all possible options should be explored. Based on a review of the latest literature, it seems clear that for the small percentage of salt-tolerant amphibian populations, a saline environment can often reduce Bd transmission and mortality from chytridiomycosis, supporting habitat modification as a feasible management strategy. However, for the 98% of amphibian species that do not inhabit saline environments, creating a Bd/Bsal refuge using 8-14 ppt NaCl should not be considered a viable option because it will result in direct lethal effects to the amphibians and many other aquatic taxa, indirect harmful effects throughout the food web, a wide range of sublethal effects with demographic consequences, and potentially increased disease incidence from other infectious agents. Note, we are not suggesting that treating salt-tolerant animals with a salt bath would be harmful, unless the salt was released into the freshwater environment, which could happen if salty aquatic habitats were created in the vicinity of low-salt aquatic habitats. As stated by Stockwell et al. (2014), “the benefits for host survival need to be weighed against the potentially negative effects on tadpole fitness, nontarget species abundance, and community dynamics.” With our rapidly growing understanding of how unintentional salinization harms freshwater ecosystems, any plans for intentional salinization of these water bodies seems ill-advised. Thus, when working to protect amphibians from Bd and Bsal, we have to remember to first, do no harm.
Data availability statement
The original contributions presented in the study are included in the article/supplementary material. Further inquiries can be directed to the corresponding author.
Author contributions
RR: Conceptualization, Funding acquisition, Writing – original draft, Writing – review & editing. EL: Conceptualization, Writing – original draft, Writing – review & editing.
Funding
The author(s) declare that financial support was received for the research, authorship, and/or publication of this article. RR would like to acknowledge the funding support of the National Science Foundation (DEB 22-43432).
Acknowledgments
EL would like to thank Mitch LeSage for thoughtful conversations that helped shape this review.
Conflict of interest
The authors declare that the research was conducted in the absence of any commercial or financial relationships that could be construed as a potential conflict of interest.
Publisher’s note
All claims expressed in this article are solely those of the authors and do not necessarily represent those of their affiliated organizations, or those of the publisher, the editors and the reviewers. Any product that may be evaluated in this article, or claim that may be made by its manufacturer, is not guaranteed or endorsed by the publisher.
References
Albecker M. A., McCoy W. M. (2017). Adaptive responses to salinity stress across multiple life stages in anuran amphibians. Front. Zool. 14, 40. doi: 10.1186/s12983-017-0222-0
Benoit D. A., Stephan C. E. (1988). Ambient Water Quality for Chloride (Duluth, MN: U.S. Environmental Protection Agency).
Berger L., Speare R., Daszak P., Green D. E., Goggin C. L., Slocombe R., et al. (1998). Chytridiomycosis causes amphibian mortality associated with population declines in the rain forests of Australia and Central America. PNAS 95, 9031–9036. doi: 10.1073/pnas.95.15.9031
Besedin D., Turner B. J., Deo P., Lopes M. D. B., Williams C. R. (2022). Effect of captivity and water salinity on culture-dependent frog skin microbiota and Batrachochytrium dendrobatidis (Bd) infection. Trans. R. Soc. South Aust. 146, 273–294. doi: 10.1080/03721426.2022.2086358
Brady S. P. (2012). Road to evolution? Local adaptation to road adjacency in an amphibian (Ambystoma maculatum). Sci. Rep. 2, 235. doi: 10.1038/srep00235
Brady S. P., Richardson J. L., Kunz B. K. (2017). Incorporating evolutionary insights to improve ecotoxicology for freshwater species. Evolutionary Appl. 10, 829–838. doi: 10.1111/eva.2017.10.issue-8
Bramwell R. (2011). Do salinity and pH help protect natterjack toads from chytridiomycosis, a disease caused by the amphibian fungus Batrachochytrium dendrobatidis (Bd)? (London: Diss. Department of Life Sciences, Silwood Park, Imperial College).
Buss N., Hua J. (2018). Parasite susceptibility in an amphibian host is modified by salinization and predators. Environ. pollut. 236, 754–763. doi: 10.1016/j.envpol.2018.01.060
Canadian Council of Ministers of the Environment (2011). Canadian Water Quality Guidelines for the Protection of Aquatic Life–Chloride Factsheet.
Clulow S., Gould J., James H., Stockwell M., Clulow J., Mahony M. (2018). Elevated salinity blocks pathogen transmission and improves host survival from the global amphibian chytrid pandemic: Implications for translocations. J. Appl. Ecol. 55, 830–840. doi: 10.1111/jpe.2018.55.issue-2
Cohen J. M., Venesky M. D., Sauer E. L., Civitello D. J., McMahon T. A., Roznik E. A., et al. (2017). The thermal mismatch hypothesis explains host susceptibility to an emerging infectious disease. Ecol. Lett. 20, 184–193. doi: 10.1111/ele.2017.20.issue-2
Courtois E. A., Loyau A., Bourgoin M., Schmeller D. S. (2017). Initiation of Batrachochytrium dendrobatidis infection in the absence of physical contact with infected hosts - a field study in a high altitude lake. Oikos 126, 843–851. doi: 10.1111/oik.03462
Garner T. W. J., Schmidt B. R., Martel A., Pasmans F., Muths E., Cunningham A. A., et al. (2016). Mitigating amphibian chytridiomycosis in nature. Philos. Trans. R. Soc. B 371, 20160207. doi: 10.1098/rstb.2016.0207
Gomez-Mestre I., Tejedo M. (2003). Local adaptation of an anuran amphibian to osmotically stressful environments. Evolution 57, 1889–1899. doi: 10.1111/j.0014-3820.2003.tb00596.x
Gray M. J., Carter E. D., Piovia-Scott J., Cusaac J. P. W., Peterson A. C., Whetstone R. D., et al. (2023). Broad host susceptibility of North American amphibian species to Batrachochytrium salamandrivorans suggests high invasion potential and biodiversity risk. Nat. Commun. 14, 3270. doi: 10.1038/s41467-023-38979-4
Gsell A. S., Biere A., de Boer W., de Bruijn I., Eichhorn G., Frenken T., et al. (2023). Environmental refuges from disease in host–parasite interactions under global change. Ecology 104, e4001. doi: 10.1002/ecy.v104.4
Hall E. M., Brady S. P., Mattheus N. M., Earley R. L., Diamond M., Crespi E. J. (2017). Physiological consequences of exposure to salinized roadside ponds on wood frog larvae and adults. Biol. Conserv. 209, 98–106. doi: 10.1016/j.biocon.2017.02.013
Hall E. M., Brunner J. L., Hutzenbiler B., Crespi E. J. (2020). Salinity stress increases the severity of ranavirus epidemics in amphibian populations. Proceedings of the Royal Society B. 287 (1926), 20200062. doi: 10.1098/rspb.2020.0062
Heard G. W., Scroggie M. P., Clemann N., Ramsey D. S. (2014). Wetland characteristics influence disease risk for a threatened amphibian. Ecol. Appl. 24, 650–662. doi: 10.1890/13-0389.1
Heard G. W., Scroggie M. P., Ramsey D. S. L., Clemann N., Hodgson J. A., Thomas C. D. (2018). Can habitat management mitigate disease impacts on threatened amphibians? Conserv. Lett. 11, e12375. doi: 10.1111/conl.12375
Hintz W. D., Arnott S. E., Symons C. C., Greco D. A., McClymont A., Brentrup J. A., et al. (2022a). Current water quality guidelines across North America and Europe do not protect lake food webs from freshwater salinization. PNAS 119, e2115033119. doi: 10.1073/pnas.2115033119
Hintz W. D., Fay L., Relyea R. A. (2022b). Road salts, human safety, and the rising salinity of our fresh waters. Front. Ecol. Environ. 20, 22–30. doi: 10.1002/fee.v20.1
Hintz W. D., Relyea R. A. (2019). A review of the species, community, and ecosystem impacts of road salt salinization in fresh waters. Freshw. Biol. 64, 1081–1097. doi: 10.1111/fwb.2019.64.issue-6
Hopkins G. R., Brodie E. D. Jr. (2015). Occurrence of amphibians in saline habitats: A review and evolutionary perspective. Herpetological Monogr. 29, 1–27. doi: 10.1655/HERPMONOGRAPHS-D-14-00006
Hughey M. C., Warne R., Dulmage A., Reeve R. E., Curtis G. H., Whitfield K., et al. (2023). Diet- and salinity-induced modifications of the gut microbiota are associated with differential physiological responses to ranavirus infection in Rana sylvatica. Philos. Trans. R. Soc. B 378, 20220121. doi: 10.1098/rstb.2022.0121
Johnson M. L., Berger L., Philips L., Speare R. (2003). Fungicidal effects of chemical disinfectants, UV light, desiccation and heat on the amphibian chytrid Batrachochytrium dendrobatidis. Dis. Aquat. Organisms 57, 255–260. doi: 10.3354/dao057255
Karraker N. E., Gibbs J. P., Vonesh J. R. (2008). Impacts of road deicing salt on the demography of vernal pool-breeding amphibians. Ecol. Appl. 18, 724–734. doi: 10.1890/07-1644.1
Kaushal S. S., Groffman P. M., Likens G. E., Belt K. T., Stack W. P., Kelly V. R., et al. (2005). Increased salinization of fresh water in the northeastern United States. PNAS 102, 13517–13520. doi: 10.1073/pnas.0506414102
Kilpatrick A. M., Briggs C. J., Daszak P. (2010). The ecology and impact of chytridiomycosis: an emerging disease of amphibians. Trends Ecol. Evol. 25, 109–118. doi: 10.1016/j.tree.2009.07.011
Klop-Toker K. L., Valdez J. W., Stockwell M. P., Edgar M. E., Fardell L., Clulow S., et al. (2017). Assessing host response to disease treatment: how chytrid-susceptible frogs react to increased water salinity. Wildlife Res. 44, 648–659. doi: 10.1071/WR16145
Lambert M. R., Stoler A. B., Smylie M. S., Relyea R. A., Skelly D. K. (2017). Interactive effects of road salt and leaf litter on wood frog sex ratios and sexual size dimorphism. Can. J. Fisheries Aquat. Sci. 74, 141–146. doi: 10.1139/cjfas-2016-0324
Lambert M. R., Womack M. C., Byrne A. Q., Hernández-Gómez O., Noss C. F., Balckburn A.P.R. D. C., et al. (2020). Comment on “Amphibian fungal panzootic causes catastrophic and ongoing loss of biodiversity. Science 367, eaay1838. doi: 10.1126/science.aay1838
Landis D. A., Wratten S. D., Gurr M. (2000). Habitat management to conserve natural enemies of arthropod pests in agriculture. Annu. Rev. Entomol. 45, 175–201. doi: 10.1146/annurev.ento.45.1.175
Langwig K. E., Voyles J., Wilber M. Q., Frick W. F., Murray K. A., Bolker B. M., et al. (2015). Context-dependent conservation responses to emerging wildlife diseases. Front. Ecol. Environ. 13, 195–202. doi: 10.1890/140241
Levinton J., Doall M., Ralston D., Starke A., Allam B. (2011). Climate change, precipitation and impacts on an estuarine refuge from disease. PloS One 6, e18849. doi: 10.1371/journal.pone.0018849
Martel A., Blooi M., Adriaensen C., Van Rooij P., Beukema W., Fisher M. C., et al. (2014). Recent introduction of a chytrid fungus endangers Western Palearctic salamanders. Science 346, 630–631. doi: 10.1126/science.1258268
Martel A., Spitzen-van der Sluijs A., Blooi M., Bert W., Ducatelle R., Fisher M. C., et al. (2013). Batrachochytrium salamandrivorans sp. nov. causes lethal chytridiomycosis in amphibians. PNAS 110, 15325–15329. doi: 10.1073/pnas.1307356110
O’Hanlon S. J., Rieux A., Farrer R. A., Rosa G. M., Waldman B., Bataille A., et al. (2018). Recent Asian origin of chytrid fungi causing global amphibian declines. Science 360, 621–627. doi: 10.1126/science.aar1965
Pyke G., White A. (2001). A review of the biology of the green and golden bell frog Litoria aurea. Aust. Zoologist 31, 563–598. doi: 10.7882/AZ.2001.003
Relyea R. A., Mattes B., Schermerhorn C. X., Shepard I. (2024). Freshwater salinization and the evolved tolerance of amphibians. Ecol. Evol. 14, e11069. doi: 10.1002/ece3.11069
Reside A. E., Briscoe N. J., Dickman C. R., Greenville A. C., Hradsky B. A., Kark S., et al. (2019). Persistence through tough times: fixed and shifting refuges in threatened species conservation. Biodiversity Conserv. 28, 1303–1330. doi: 10.1007/s10531-019-01734-7
Richgels K. L. D., Russell R. E., Adams M. J., White C. L., Grant E. H. C. (2016). Spatial variation in risk and consequence of Batrachochytrium salamandrivorans introduction in the USA. R. Soc. Open Sci. 3, 150616. doi: 10.1098/rsos.150616
Scheele B. C., Hunter D. A., Grogan L. F., Berger L., Kolby J. E., McFadden M. S., et al. (2014). Interventions for reducing extinction risk in chytridiomycosis-threatened amphibians. Conserv. Biol. 28, 1195–1205. doi: 10.1111/cobi.2014.28.issue-5
Scheele B. C., Pasmans F., Skerratt L. F., Berger L., Martel A., Beukema W., et al. (2019). Amphibian fungal panzootic causes catastrophic and ongoing loss of biodiversity. Science 363, 1459–1463. doi: 10.1126/science.aav0379
Schmeller D. S., Blooi M., Martel A., Garner T. W., Fisher M. C., Azemar F., et al. (2014). Microscopic aquatic predators strongly affect infection dynamics of a globally emerged pathogen. Curr. Biol. 24, 176–180. doi: 10.1016/j.cub.2013.11.032
Schuler M. S., Cañedo-Argüelles M., Hintz W. D., Dyack B., Birk S., Relyea R. A. (2019). Regulations are needed to protect freshwater ecosystems from salinization. Philos. Trans. R. Soc. B 374, 20180019. doi: 10.1098/rstb.2018.0019
Spitzen-van der Sluijs A., Spikmans F., Bosman W., de Zeeuw M., van der Meij T., Goverse E., et al. (2013). Rapid enigmatic decline drives the fire salamander (Salamandra salamandra) to the edge of extinction in the Netherlands. Amphibia-Reptilia 34, 233–239. doi: 10.1163/15685381-00002891
Springer Y. P. (2009). Do extreme environments provide a refuge from pathogens? A phylogenetic test using serpentine flax. Am. J. Bot. 96, 2010–2021. doi: 10.3732/ajb.0900047
Stockwell M. P., Clulow J., Mahony M. J. (2012). Sodium chloride inhibits the growth and infective capacity of the amphibian chytrid fungus and increases host survival rates. PloS One 7, e36942. doi: 10.1371/journal.pone.0036942
Stockwell M. P., Clulow J., Mahony M. J. (2015). Evidence of a salt refuge: chytrid infection loads are suppressed in hosts exposed to salt. Oecologia 177, 901–910. doi: 10.1007/s00442-014-3157-6
Stockwell M. P., Storrie L. J., Pollard C. J., Clulow J., Mahony M. J. (2014). Effects of pond salinization on survival rate of amphibian hosts infected with the chytrid fungus. Conserv. Biol. 29, 391–399. doi: 10.1111/cobi.2015.29.issue-2
Tornabene B. J., Hossack B. R., Crespi E. J., Breuner C. W. (2021). Corticosterone mediates a growth-survival tradeoff for an amphibian exposed to increased salinity. J. Exp. Zool. Part A: Ecol. Integr. Physiol. 335, 703–715. doi: 10.1002/jez.2535
Waddle A. W., Clulow S., Aquilina A., Sauer E. L., Kaiser S. W., Miller C., et al. (2024). Hotspot shelters stimulate frog resistance to chytridiomycosis. Nature 631, 344–249. doi: 10.1038/s41586-024-07582-y
Wilber M. Q., Knapp R. A., Toothman M., Briggs C. J. (2017). Resistance, tolerance and environmental transmission dynamics determine host extinction risk in a load-dependent amphibian disease. Ecol. Lett. 20, 1169–1181. doi: 10.1111/ele.2017.20.issue-9
Woodhams D. C., Barnhart K. L., Bletz M. C., Campos A. J., Ganem S. J., Hertz A., et al. (2018). Batrachochytrium: Biology and management of amphibian chytridiomycosis. eLS, 1–18. doi: 10.1002/9780470015902.a0027207
Keywords: disease ecology, parasite, salinization, frogs, salamanders
Citation: Relyea RA and Le Sage E (2024) Creating salt refuges to protect amphibians from chytridiomycosis: first, do no harm. Front. Amphib. Reptile Sci. 2:1465754. doi: 10.3389/famrs.2024.1465754
Received: 16 July 2024; Accepted: 16 October 2024;
Published: 01 November 2024.
Edited by:
Michael J. Jowers, University of Granada, SpainReviewed by:
Gary Bucciarelli, University of California, Davis, United StatesJudit Vörös, Eötvös Loránd Research Network, Hungary
Copyright © 2024 Relyea and Le Sage. This is an open-access article distributed under the terms of the Creative Commons Attribution License (CC BY). The use, distribution or reproduction in other forums is permitted, provided the original author(s) and the copyright owner(s) are credited and that the original publication in this journal is cited, in accordance with accepted academic practice. No use, distribution or reproduction is permitted which does not comply with these terms.
*Correspondence: Rick A. Relyea, cmVseWVyQHJwaS5lZHU=
†These authors have contributed equally to this work