- 1Laboratório de Ecologia de Vertebrados Terrestres, Universidade do Vale do Rio dos Sinos, São Leopoldo, Rio Grande do Sul, Brazil
- 2Laboratório de Metacomunidades, Instituto de Biociências, Universidade Federal do Rio Grande do Sul, Porto Alegre, Rio Grande do Sul, Brazil
- 3Laboratório de História Natural de Anfíbios Brasileiros (LaHNAB), Instituto de Biologia, Universidade Estadual de Campinas, Campinas, São Paulo, Brazil
- 4Vizlab/X-Reality and GeoInformatics Lab – Universidade do Vale do Rio dos Sinos, São Leopoldo, Rio Grande do Sul, Brazil
- 5Departamento de Zootecnia e Ciências Biológicas, Universidade Federal de Santa Maria, Palmeira das Missões, Rio Grande do Sul, Brazil
Introduction: Habitat loss, as well as infectious diseases, have been suggested as the main causes for the worldwide decline of amphibian populations. Thus, the search for biotic indicators is a useful tool for mapping priority demands in disease management.
Aims: Here we analyzed the prevalence of two pathogens, Batrachochytrium dendrobatidis (Bd) and Ranavirus (Rv), in anuran communities in southern Brazil. We explored the relationship between the prevalence of Bd and landscape class and used an indicator-species and indicator-community approach to identify which species and communities have the potential to indicate the presence of Bd.
Methods: We used histological analyses to access Bd and PCR for Rv in tadpole communities.
Key results: Our data, based on 26 anuran communities, revealed high Bd prevalence but absence of Rv. Three species, of the 26 communities studied, were found to be Bd indicators, including endemic species from southern Brazil and Argentina. Additionally, the analysis with an indicator-community approach revealed that communities from larger forest areas are Bd indicators.
Conclusions: Analyses at the community level, which relate landscape to pathogens, provide unprecedented and highly relevant information for fragmented Atlantic Forest landscapes. Based on our results, we argue that even communities in larger forest patches (apparently well-preserved) are susceptible to Bd and deserve attention regarding the possibility of incidence of chytridiomycosis. Implications. From our results, we consider that the use of Bd-indicator species and Bd-indicator communities is a useful approach for conservation and could be used to evaluate the threatened status of species and for proposals for landscape management.
1 Introduction
Human-induced changes in natural habitats can indirectly increase the susceptibility of wild species to infectious diseases by disturbing the natural host-pathogen dynamics (Daszak et al., 2001; Preuss et al., 2020; Silva et al., 2022) and promoting the emergence of new pathogens or more virulent forms of pre-existing pathogens (Jones et al., 2008; Giam, 2017; Silva et al., 2022). The impact and severity of diseases over native species depend on the speed at which pathogens spread, combined with host susceptibility to infections (Fisher and Garner, 2020; Bates et al., 2023). Habitat modifications, such as deforestation, pollution, and microclimatic changes, are known to be associated with a high prevalence of infection in amphibian host species (Gillespie et al., 2005; Kärvemo et al., 2019; McMillan et al., 2020). This scenario is of special concern in tropical regions, which harbor high biodiversity (Mittermeier et al., 2005, 2011) and where a continuous landscape change is noted, in particular driven by economic development in some countries. Therefore, identifying species that are most affected by these changes is relevant for conservation purposes and may serve as a powerful tool for landscape management.
Emerging infectious diseases have been reported as important threats to amphibians (Latney and Klaphake, 2020), particularly chytridiomycosis and ranaviruses due to their lethality potential. Chytridiomycosis is a disease caused by the fungi Batrachochytrium dendrobatidis (Bd) and B. salamandrivorans (Bsal) (Longcore et al., 1999; Martel et al., 2013), where Bd, in particular, has caused population declines and extinction in more than 500 amphibian species (Scheele et al., 2019; Fisher and Garner, 2020) and is considered a major threat for amphibians worldwide (O’Hanlon et al., 2018). The chytrid Bd parasites all amphibian life stages (Rachowicz and Vredenburg, 2004), causing epidermal hyperplasia and hyperkeratosis and/or death in adults (Pessier et al., 1999; Nichols et al., 2001; Voyles et al., 2009) and, in tadpoles, dekeratinization of the mouthparts, which can result in deformities (Knapp and Morgan, 2006), and sublethal (Hanlon et al., 2015).
The impact of Bd in amphibian populations may vary depending on several aspects of the hosts, such as their biology, reproductive behavior and skin microbiome (Ruggeri et al., 2018; Sapsford et al., 2018), and is associated with environmental factors, including habitat integrity (Becker et al., 2010; Haddad et al., 2015) and temperature (Piotrowski et al., 2004; Sonn et al., 2017). Studies showed that a high prevalence of Bd infection is observed even in undisturbed habitats (Valencia-Aguilar et al., 2016; Delazeri et al., 2021; Lambertini et al., 2021). Many landscape features are known to facilitate Bd infection, such as shading and low temperatures, which seem to favor the fungus’ development (Valencia-Aguilar et al., 2016; Delazeri et al., 2021). Thus, we hypothesize that subtropical forests, such as those in southern Brazil, would be potential hotspots for Bd in the Neotropics due to their suitability for the fungus survival (Jenkinson et al., 2016). In fact, high prevalences of Bd infection have been recorded in the southern (subtropical) domain of the Atlantic Forest (Ernetti et al., 2020; Forti et al., 2020; Delazeri et al., 2021).
The Atlantic Forest encompasses high diversity and endemism of anuran species (Mittermeier et al., 2011; Haddad et al., 2013) and is, therefore, a biome of great concern for extinction risks. Even though Bd records are well documented in the Atlantic Forest of Southern Brazil (Preuss et al., 2016; Ernetti et al., 2020; Forti et al., 2020; Santos et al., 2020; Delazeri et al., 2021; Ruggeri et al., 2024), other pathogens remain understudied. For example, viruses in the genus Ranavirus (Rv) represent an important threat to amphibians (Miller et al., 2011; Ruggeri et al., 2019) as they spread quickly and can cause high mortality (Daszak et al., 1999; Green et al., 2002). These viruses, which affect not only amphibians but also fishes and reptiles, deserve special attention since a recent study revealed that they are also widely distributed in this region and may even be more prevalent than Bd (Ruggeri et al., 2024). Therefore, we expected to find a wide distribution of this pathogen in our sample sites.
Mortality by Bd is rare in tadpoles (Garner et al., 2009; Gervasi et al., 2013), but mortalities associated with Rv have been reported in many parts of the world (Duffus et al., 2015), including in Brazil (Mazzoni et al., 2009; Ruggeri et al., 2019). Thus, Bd and Rv seem to vary in terms of lethality among tadpoles and adults, where Rv usually represents a threat to the survival of tadpoles while Bd represents the same for adults (Rachowicz and Vredenburg, 2004; Grayfer et al., 2015). However, tadpoles are suggested to act as a reservoir and vector of Bd (Daszak et al., 2003; Rachowicz and Vredenburg, 2004; Blaustein et al., 2005; Narayan et al., 2014; Valencia-Aguilar et al., 2016). For this reason, the association of Rv and Bd can be highly problematic to the maintenance of amphibian populations due to their combined/complementary impact over all stages of their lifespan. Given that host-pathogen dynamics are associated with intrinsic features of hosts, such as life stage (Garner et al., 2009), and extrinsic factors (Lambertini et al., 2021), we analyzed the prevalence of Bd and Rv in tadpole communities in the subtropical Atlantic Forest of southern Brazil by exploring the relationship of pathogen prevalence and landscape configuration. Due to the variety of responses to pathogen infection among different species (i.e., susceptibility, tolerance and resistance; Woodhams et al., 2007; Hoverman et al., 2011), we used an indicator-community and indicator-species approach to Bd and Rv infections.
2 Study area
We conducted this study in the subtropical Atlantic Forest, southern Brazil, between coordinates 22°30’ N to 33°45’ S and 48°02’ E to 57°40’ W, which encompasses the states of Paraná, Santa Catarina and Rio Grande do Sul (Figure 1). This region was originally covered by different phytophysiognomy of Atlantic Forest: Mixed Ombrophilous Forest (Araucaria Forest), Seasonal Forest, and High-Altitude Grasslands (Veloso and Goés-Filho, 1982; https://www.sosmatatlantica.org.br). Currently, remnants of those phytophysiognomy share space with urban, livestock-raising, and agricultural areas (Ribeiro et al., 2009; Pillar and Vélez, 2010). The climate is subtropical, with annual air temperatures varying from 16°C to 24°C and rainfall varies from 1,600–2,200 mm annually (Alvares et al., 2013).
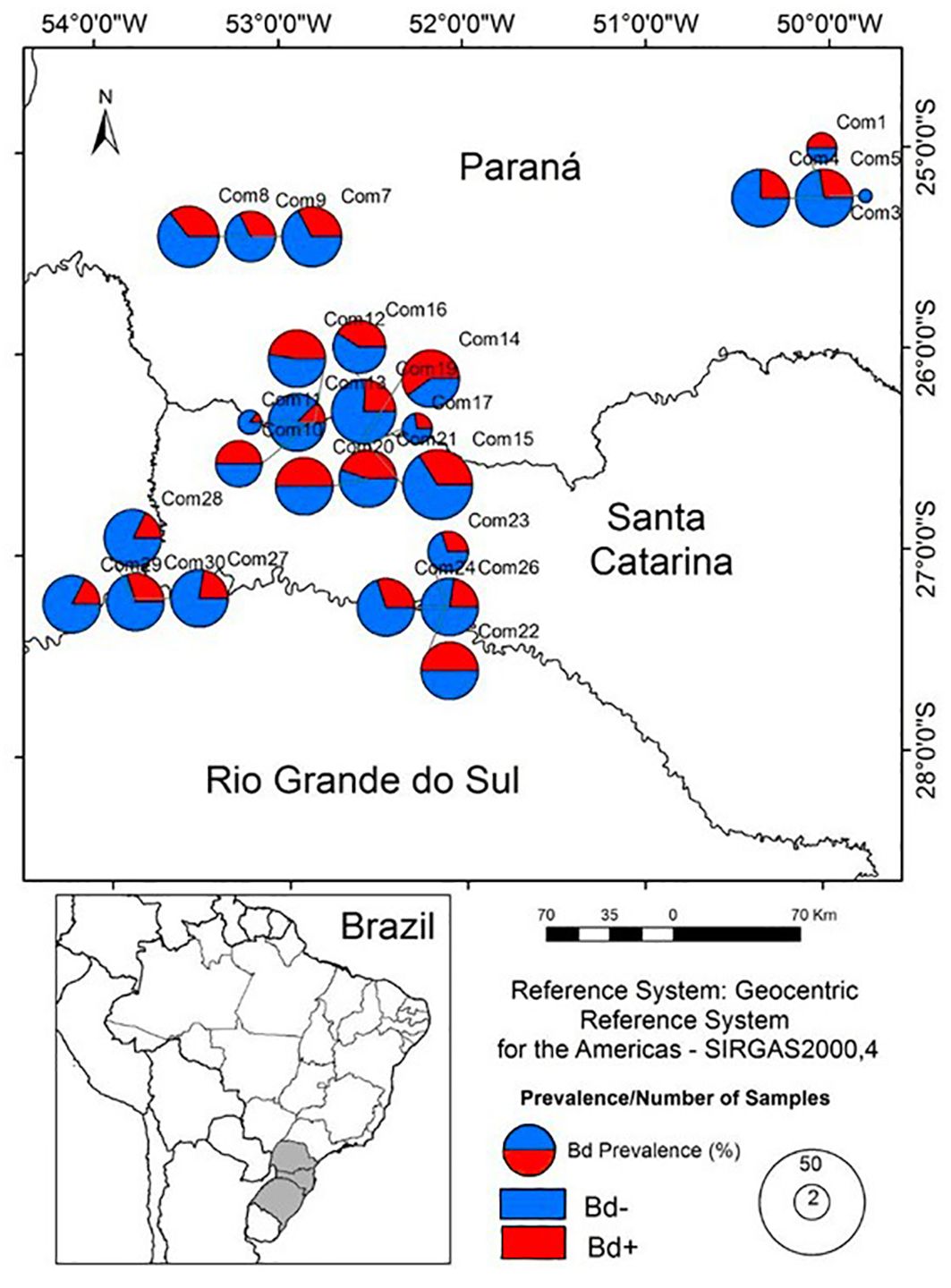
Figure 1. Location of the sampling areas and Bd prevalence in the tadpole communities in southern Brazil (More details in Supplementary Table S1). Blue color shows the proportion of infected (Bd+) and red color non-infected (Bd−) tadpoles. The size of the graphs shows the number of tadpoles sampled in each community range 2 to 50.
We selected seven sampling areas using satellite images, based on the following criteria: (1) presence of well-preserved native forest; (2) similar climatic conditions; (3) elevation between 300 m and 900 m above sea level; (4) similar topography; and (5) presence of lentic and lotic waterbodies used as breeding sites by anurans. Next, we selected “in loco” waterbodies in these areas, which were the sampling sites. We sampled at least one lentic and one lotic waterbody in each one of the seven areas to assess a wide variety of habitats and tadpole communities (Melo et al., 2017). We sampled a total of 30 waterbodies and selected 26 for analysis, 12 of which are ponds (permanent or temporary) and 14 streams associated with the forest, with stretches of up to 100 m in length (see Supplementary Table S1). As a selection criterion, we exclusively analyzed tadpoles species that were found in at least three waterbodies. So, the waterbodies “2, 6, 18 and 25” was excluded.
In order to characterize the waterbodies sampled, we measured their areas and depth. The area of the ponds was recorded with polygons drawn in Google Earth, and, for streams, it was determined by width x length in a 100-m stretch in each stream. Water depth was measured at three points for each waterbody at the same location where tadpoles were sampled using a measuring tape. The streams sampled were of low order (width of 1.99 ± 0.79 m) and the polygon area of the ponds was 386.45 ± 307.39 m². Streams had a mean depth of 0.45 ± 0.12 m and ponds of 0.8 ± 0.40 m. The vegetation surrounding the waterbodies consisted of grasses, shrubs, and trees, having distinct compositions (see Supplementary Table S1).
3 Methods
3.1 Tadpole collection
We sampled waterbodies from October 2018 to March 2019 to include the period of highest temperatures and breeding season of the majority of species in southern Brazil (Preuss et al., 2020; Santos et al., 2021). The extent of sampled area is approximately 10,500,000 ha, thus each waterbody could be sampled just once. We performed tadpole sampling from 08:00 a.m. to 06:00 p.m., using a 3-mm²-mesh dip-net (Heyer, 1976). We used nets of various sizes and all of them were immersed with 2.5% sodium hypochlorite for 10 minutes at the end of each sampling, and rinsed with local water before use. We did the same with the boots. Due to the large distance among waterbodies, each one was considered as a source of a unique and independent tadpole community (see more details in Data Analysis session).
For tadpole collection, two people screened all available habitats in the waterbody for one hour, using the dip-net. Sweepings were systematized, considering the whole perimeter of the ponds’ margins (Vasconcelos and Rossa-Feres, 2005; Santos et al., 2007; Both et al., 2009; Bolzan et al., 2016; Marques et al., 2018) and, for streams, we additionally used smaller dip nets that allowed us to screen smaller spaces between rocks (adapted from Jordani et al., 2017). During the sampling, we conducted a rigorous inspection of the presence of dead or lethargic tadpoles. Afterward, the tadpoles were euthanized by immersion in a solution of 2% lidocaine (according to the Conselho Nacional para o Controle de Experimentação Animal – CONCEA, 2018) and later transferred to absolute ethanol (see Lambertini et al., 2013). We conducted the taxonomic identification of the specimens under a stereomicroscope (Yaxum/IX-AK02) using identification keys (e.g., MaChado and Maltchik, 2007; Gonçalves, 2014). Concurrently, we classified the tadpoles according to the stage of ontogenetic development following Gosner (1960).
Analysis of the presence zoosporangia of the 26 waterbodies selected, we analyzed 899 tadpoles in the developmental stages 26 to 40 (following Altig and Johnston, 1986) for histological analysis of Bd. We sectioned the mouthparts of each tadpole in order to obtain tissue samples of about 2 mm. We have considered an individual positive for Bd when we detected zoosporangia in the tissue samples (according to Neves-Da-Silva et al., 2021), which were observed by a detailed inspection in an optical microscope with a magnification of up to 400x for about 30 min for each tissue sample (Vieira et al., 2013). All analyses were conducted by the same person for standardization.
3.2 Ranavirus analysis
Only waterbodies with >50 sampled tadpoles were selected to ensure a sample size that is compatible to what is suggested for studies of viral infections (see DiGiacomo and Koepsell, 1986), totalling 24 waterbodies out of 30. We extracted samples of liver and kidneys from 1440 tadpoles (60 specimens from each waterbody) to analyze for the presence of Ranavirus spp. For that, we separated each tadpole’s liver and kidneys using a surgical scalpel and dissecting needle under the stereomicroscope, cut the tissues (liver and kidneys) into small fragments, and stored the samples in individual 1.5-ml microtubes with absolute ethanol. We then performed a pool of tissues from 60 individuals per waterbody, totalling 24 samples, and put up to 25 mg of each pool of tissues in 1.5-ml microtubes with absolute ethanol to proceed with DNA extraction using the DNeasy® Blood & Tissue kit following the manufacturer’s protocol. After DNA extraction, we measured the concentration of DNA in the samples using NanoDrop Lite (Thermofisher) and diluted all samples to 20 ng/µl.
We used PCR analysis for detecting the presence of the virus in the pool of tissues of the waterbody. We used specific primers for the genes MCP and Pol II described in Mazzoni et al. (2009), and we determined the optimal annealing temperature for these primers by gradient PCR (from 50 to 65°C). For each reaction, we used 12.5 µl of GoTaq® Colorless Master Mix, 1 µl of each primer at 10 µM, 5.0 µl of DNA template (100 ng/µl) and 5.5 µl of ultrapure water to complete 25 µl for each reaction. We adapted the amplification conditions for each set of primers (Mazzoni et al., 2009). Therefore, we performed an initial 2-min cycle at 95°C, followed by 40 cycle of 60 seconds at 95°C (denaturation), 60 seconds at 60°C (annealing), and 90 seconds at 72°C (MCP extension) followed by a final extension of 5 min at 72°C. The products of the amplification were transferred to agarose gel at 1%, dipped into TAE buffer solution (tris-acetate 40 mM, EDTA 1 mM), stained with 0.5 µg/ml of ethidium bromide, and visualized with UV transilluminator.
3.3 Microhabitat and land use analysis
We evaluated the canopy cover surrounding each water body using a spherical lens (Universal clip lens; 180°) coupled to a cell phone (Xiaomi MI). The images were taken at the central point at the tadpole-collection and later treated in the GapLight program, version 2.0 (Frazer et al., 1999) and presented as a percentage. We made a single measurement per waterbody (see Supplementary Table S2). For a broader range of evaluation, we described the land use type in which waterbodies are inserted. Analyses were based on satellite images (Landsat 8 multispectral images, sensor Operational Land Imager from the U.S. Geological Survey; https://earthexplorer.usgs.gov) captured between January and July 2019. We selected images with minimal cloud cover and without significant radiometric noise. We performed the following pre-processing steps of the images: 1) geometric corrections, due to the inherent geometric distortions in images collected at different times, through the georeferencing of these images; 2) atmospheric corrections to reduce the interference of atmospheric scattering on the images (Soares et al., 2015); and 3) mosaic and enhancement of the different images in each season to reduce possible seasonal effects on the visual aspect of the images. Pre-processing was conducted using software ENVI, version 5.51 (L3Harris Geospatial, Boulder, Colorado, USA). After the pre-processing stages, we defined the classes of land use and occupation from observations in loco, considering the predominant use of the areas. The categories were established as Canopy cover (in percentage), Forest (native forest formations in intermittent and advanced successional process), Agriculture (cultivated areas, with soybean, corn, or wheat), Livestock farming (extensive livestock farming), Urban area (buildings) and Aquatic environment (streams, artificial and natural ponds) (Supplementary Table S2). We classified the images based on their vectorization in ArcGIS software version 10.3 (Environmental Systems Research Institute Esri, Redlands, California, USA), considering the buffer with a 500-m radius for each waterbody. The buffer size was based on previous studies that describe the average habitat size for amphibians, ranging from 290 m (Semlitsch and Bodie, 2003) to 500 m (Canessa and Parris, 2013). We consider the central point of the buffer to be the pond or stream (collection points). The polygons for each type of cover were reprojected to the Geocentric Reference System for the Americas SIRGAS 2000, Universal Transverse Mercator (UTM) projection, zone 22S, and we calculated the areas in km².
3.4 Data analysis
We used Generalized linear mixed models (GLMMs) to evaluate the influence of the landscape variables (land use class; Supplementary Table S2) on Bd prevalence in tadpoles of 26 communities (waterbodies). This analysis allows us to test the contribution of fixed and random effects in the distribution of observed data of Bd prevalence. We included the landscape variables (such as Canopy cover, Forest, Agriculture, Livestock farming, Urban area and Aquatic environment) as fixed effects (predictor variables) and as random effects, the tadpole communities. To avoid collinearity of variables, we used the vif function in “car” package and removed the variable Forest (vif = 6.77) from the model. We applied a multi model inference approach (Burnham and Anderson, 2002) using all possible combinations of predictors (i.e., Canopy cover, Agriculture, Livestock farming, Urban area and Aquatic environment), and we used Akaike’s Information Criterion to select the best model(s). GLMMs were built using the ‘nlme’, ‘MuMIn’ and ‘lme4’ packages (Bates et al., 2015) in R software. We analyzed models using the “lme” function of the “lme4” package, and we evaluated the significance of each predictor using the anova function in the package “stats”.
We conducted an indicator-species analysis (see Cáceres et al., 2010; Severns and Sykes, 2020) to identify anuran species and communities most sensitive to the presence of Bd. To list the anuran species with the potential to indicate the presence of Bd, we conducted an indicator species analysis using the “indicspecies” package in R (Cáceres and Legendre, 2009). As the first step, we used the function “pruneindicators” to determine the best set of indicator species. This analysis works in stages. First, it selects the species with positive values of prediction, sensitivity, and indication. Then, a “speciescomb” object with confidence intervals is produced, and the lower limits are used to select “indicator species”, excluding species that show an occurrence pattern that is nested with other species. Finally, the function evaluates the coverage of the remaining set of species until the maximum indication values are reached to discriminate species with real indication potential (Cáceres and Legendre, 2009). Following this step, we used the “predict.indicators” function to determine the existence of communities that would be considered indicators of the presence of Bd. This function calculates the probabilities corresponding to the communities to construct the indicator object. We used the function “pruneindicators” to find the communities with the highest indicator values. The groups are defined by species, which act as group indicators. The main indicators are those found in only one group (specificity) and, if all members of the group are positive for Bd (coverage), this species would have “1” as its indication value. The specificity of a species is calculated considering the prevalence of the fungus and based on the number of individuals, while coverage only considers the fungus’ presence or absence. Finally, we performed a principal component analysis (PCA) to observe the association between landscape classes and Bd prevalence in the tadpole communities using the “prcomp” function in the package vegan of R software. Rv was not detected in our sampling and therefore not included in any models.
4 Results
All tadpoles analyzed for the presence of Rv (N = 1440) tested negative. For Bd analyses, the 899 tadpoles selected belonged to 10 anuran species distributed within four families (Table 1): Hylidae (Boana curupi, B. faber, Dendropsophus microps, D. minutus, Scinax fuscovarius, S. granulatus, S. perereca), Hylodidae (Crossodactylus schmidti), Leptodactylidae (Physalaemus cuvieri), and Phyllomedusidae (Phyllomedusa tetraploidea). Of the 899 tadpoles, 298 had zoosporangia in the mouthparts, representing an overall Bd prevalence of 32.04% (Table 2). All species and 96% of communities were infected with Bd. The prevalence of Bd-infected tadpoles in communities ranged from 0 to 60% (Table 2). Tadpoles of Boana faber presented the highest Bd prevalence (47.69%) among species and Scinax granulatus the lowest (16.67%). We did not find dead or lethargic tadpoles in any of the 26 communities analysed.
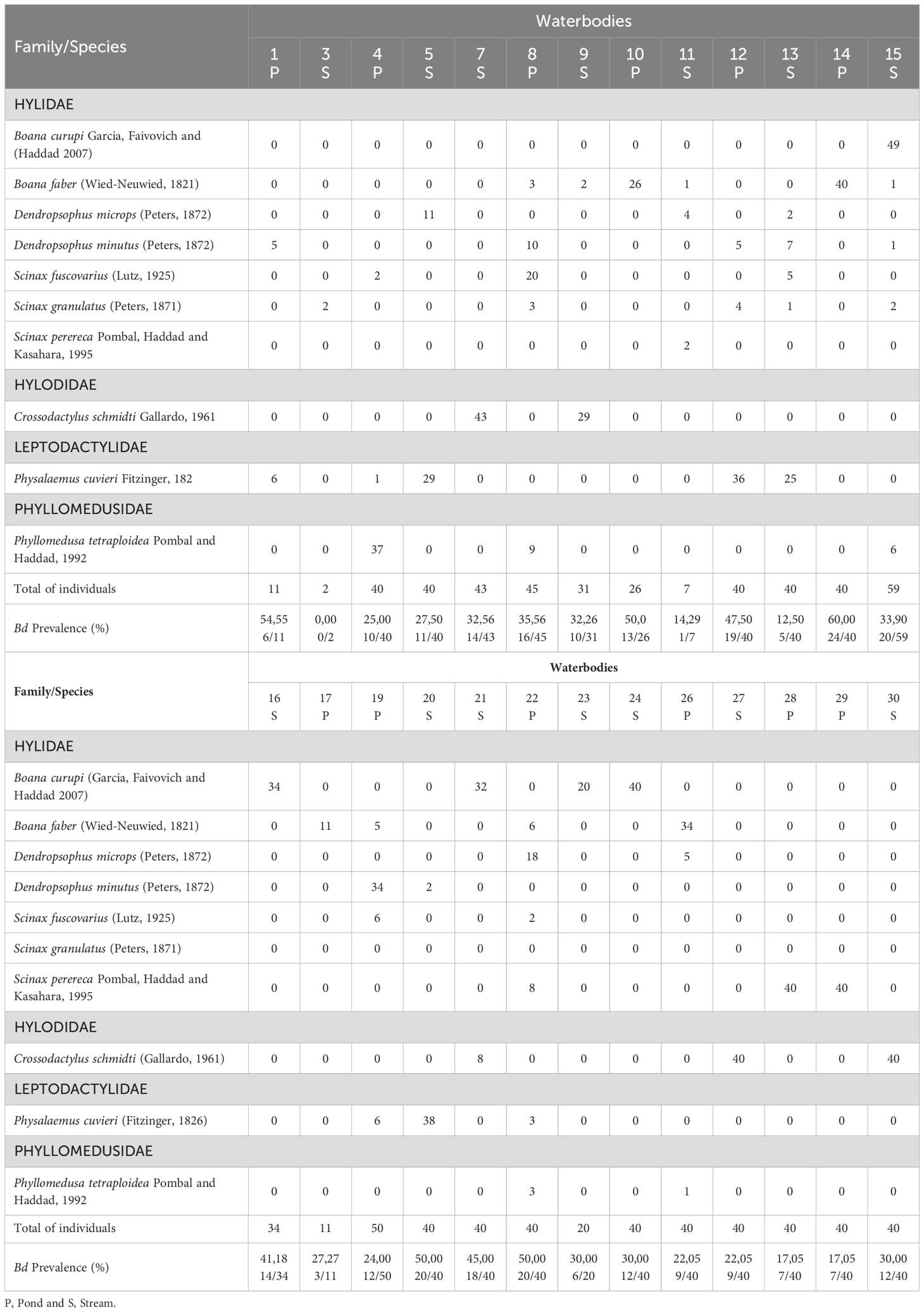
Table 1. Anuran species, number of tadpoles in developmental stages 26 to 40 (Gosner, 1960) and prevalence of Batrachochytrium dendrobatidis in 26 waterbodies (streams and ponds) in forest remnants from October 2018 to March 2019 in southern Brazil.
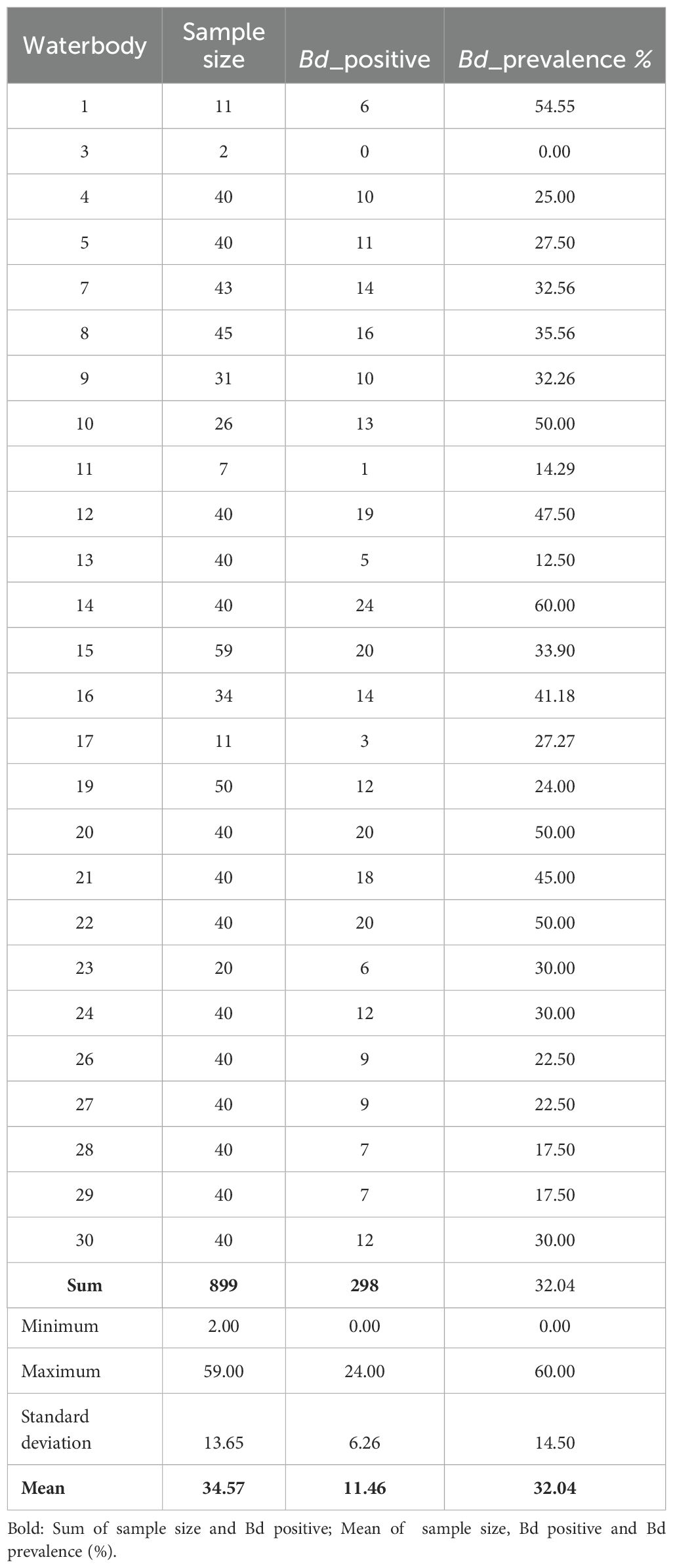
Table 2. Total number of tadpoles, number of tadpoles positive to Batrachochytrium dendrobatidis (Bd_positive) and prevalence of Batrachochytrium dendrobatidis prevalence per waterbody sampled (n = 26) from forest remnants in southern Brazil, from October 2018 to March 2019.
The GLMM showed that there is no significant influence of the land use on the Bd prevalence (contribution of fixed effects, R2m = 0.10; contribution of fixed effects + random effects, R2c = 0.89; see Supplementary Table S3). However, the analysis of indicator communities identified five tadpole communities as indicators of Bd presence (communities 15, 16, 21, 23, and 24; Table 3), these communities are formed mainly by Boana curupi species. The PCA showed that waterbodies from larger forested areas were the most associated with communities of infected tadpoles (Figure 2). Of the 10 species analysed, three functioned as indicators of the presence of Bd (Supplementary Table S4). Boana curupi (Forest Treefrog) and Crossodactylus schmidti (Schmidt’s Spinythumb Frog) had the greatest specificity values (close to 1) than Boana faber (Gladiator Treefrog; 0,86). Boana faber presented a greatest coverage value (0.33). Finally, the indication values were similar between the three species, ranging from 0.49 (C. schmidti) to 0.54 (B. faber).
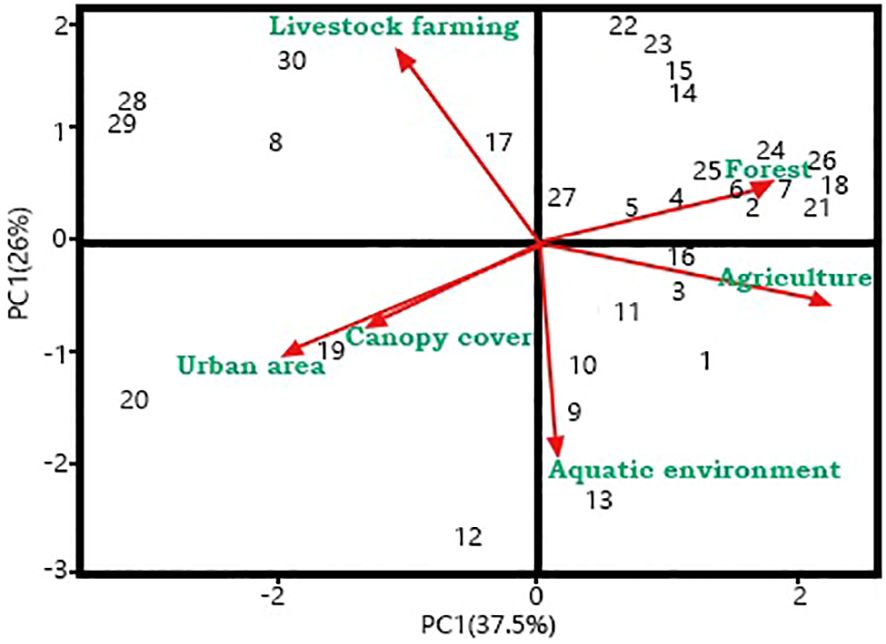
Figure 2. Principal component analysis (PCA) showing the association between landscape classes and Batrachochytrium dendrobatidis prevalence in the tadpole communities from waterbodies in reproductive sites of Atlantic Forest habitats in southern Brazil from October 2018 to March 2019.
5 Discussion
We did not detect the presence of Ranavirus spp. in the studied communities. However, Rv was recently reported for tadpoles from other communities in Southern Brazil (Ruggeri et al., 2024), including a site where a die-off of tadpoles of the invasive American bullfrog was observed (Ruggeri et al., 2019). As these viruses can persist in the environment without a host (Brunner and Yarber, 2018; Miaud et al., 2019), the non-detectability of Rv in the specimens sampled in this study could be due to spatial and temporal variation of the host-pathogen dynamics not yet investigated in the area. Further analysis using environmental DNA could be used as a tool to investigate the presence of Rv in these sites. Contrarily, if the virus is indeed absent in the sites investigated herein, it should still be regularly inspected as long-distance spread and Rv emergence are often associated with increased environmental stress and human activity (Duffus et al., 2015). As the region where we conducted our research is a highly fragmented landscape, these communities might as well be vulnerable to different pathogens, including Rv. We therefore argue that Rv is a potential threat to anuran species in this region and should be investigated regardless of our results having shown absence of the virus.
Differently from Rv, we detected high prevalence of Bd (32%) amongst tadpoles in the area and most communities (96%) were positive for the chytrid. This result is expected as different tadpole species and communities from the Southern portion of the Brazil’s Atlantic Forest are often found infected by Bd (Vieira et al., 2013; Carvalho et al., 2017; Ruggeri et al., 2018, 2020; Neves-Da-Silva et al., 2021). It is concerning that all species were infected. Because Bd infection is favored by colder climate regimes (Fernández-Loras et al., 2019), we suggest that such high Bd prevalence (this study) and infection loads observed in species from the Southern of Brazil (Preuss et al., 2016; Ernetti et al., 2020; Santos et al., 2020; Delazeri et al., 2021) is due to the subtropical climate in this region, indicating the southern portion of the Atlantic Forest as a hotspot for Bd. Future studies should investigate the anuran susceptibility to Bd infections considering the latitudinal components, such as environmental factors (i.e., temperature and rainfall) and the community diversity (e.g., Lambertini et al., 2021) as well as the existence of Bd-tolerant species in anuran communities from this region in order to detect reservoir species that would promote the survival and persistence of the fungus in these communities (see Valencia-Aguilar et al., 2016; Ruggeri et al., 2020; Neves-Da-Silva et al., 2021).
Although previous studies have found an association between the composition of anuran communities in the study area and the configuration of the landscape (Santos et al., 2021), we did not detect an association between landscape and Bd presence on the studied tadpole communities. Human changes of these environments seem not to affect the susceptibility of tadpoles to Bd infection, as we expected. In fact, based on our Bd-indicator communities evaluation, Bd was more associated with communities from larger forest areas. A possible explanation for this forest-Bd association is that there are more shading in forested habitats which likely generate microclimate conditions (as lower temperatures) that benefit Bd development (Beyer et al., 2015; Muletz-Wolz et al., 2019; Wanderley et al., 2019). In addition, the Bd suitability to infect anurans in forested areas has been associated with a genetic-driven decrease in immune resistance of forest specialist anurans when their habitats are disturbed (Belasen et al., 2022). We must consider that even the largest patches are remnants of a continuous forest and therefore, suffer or have already suffered some degree of disturbance. Hence, our data highlights the risks of Bd exposure for all tadpole communities in the area, but especially for those tadpoles inhabiting waterbodies from larger remnant forest patches. Finally, as the relationship between Bd infection and landscape features is complex, we insist that this theme must be investigated under a scenario of individual responses to landscape/habitat modifications (e.g., Silva et al., 2022).
The prevalence of Bd varied between communities, which could be explained by the differences in species composition among the sampled communities. For example, species would vary their response to Bd infection due to differences in aspects of their life history (Corey and Waite, 2008) and their reproductive mode (Lips et al., 2003). We have analyzed 10 different anuran species disproportionately distributed in the sampled communities. As a consequence of tadpole diversity in a community, we expected that the more species evaluated simultaneously in the same community, the higher variability of responses to infection the community would show, making it difficult to find a general pattern at a community level. For this reason, we have also analyzed responses to infection at a species level. Even so, our results corroborate that Bd-indicator communities are represented mainly by forest-specialist species (e.g., Belasen et al., 2022), such as Boana curupi and Crossodactylus schmidti.
We detected three Bd-indicator tadpole species: B. curupi, B. faber, and C. schmidti. This result is of high concern as B. curupi, and C. schmidti are endemic to the south of Brazil and Argentina (Frost, 2023), and are threatened are threatened in southern Brazil, in the states of Santa Catarina and Rio Grande do Sul (CONSEMA, 2011; FZRGS, 2014), and tadpoles of B. faber are suggested as reservoir for Bd (Ruggeri et al., 2020). Our findings corroborate previous studies in this region that have indicated adults of B. curupi and C. schmidti as species highly vulnerable to Bd infection, particularly B. curupi that showed high prevalence of infection and zoospore loads (Preuss et al., 2016; Delazeri et al., 2021). In addition, B. faber is a species of particular interest because females of B. faber lay their eggs on ponds in open areas, differently from the adults of B. curupi and C. schmidti, that lay their eggs in forested areas (Haddad et al., 2013; Oliveira et al., 2016; Frost, 2023). Thus, have a long period of larval development (8 to 12 months; Martins, 1993; Madalozzo et al., 2013), which can increase their time of exposure to Bd zoospores (Bradley et al., 2019; Ruggeri et al., 2020; Neves-Da-Silva et al., 2021) leading to a high prevalence of fungal infection in communities where the species is present (Neves-Da-Silva et al., 2021). Our results reinforce the relevance of using natural-history attributes to investigate the anuran suitability to Bd infection (Alvarado-Rybak et al., 2021).
The region where we conducted our study is a highly fragmented landscape, formed by forest patches of different sizes. Even the largest patches resulted from an intense fragmentation and deforestation process driven by human activities, such as agriculture, pastures, and urban areas (Wanderley et al., 2019; Ernetti et al., 2020; Preuss et al., 2020). Therefore, the association of landscape features (i.e. forest remnant patches, land use) and Bd that we found with this study, highlights that even communities from the last preserved forests (patches) are not Bd-free and deserve attention. We advocate for the use of Bd-indicator species and as a useful approach for conservation and landscape management proposals.
Data availability statement
The original contributions presented in the study are included in the article/Supplementary Material. Further inquiries can be directed to the corresponding author.
Ethics statement
The animal study was approved by Animal Ethics Committee of Universidade do Vale do Rio dos Sinos (CEUA-UNISINOS # PPECEUA08.2018). The study was conducted in accordance with the local legislation and institutional requirements.
Author contributions
RC: Formal analysis, Funding acquisition, Investigation, Writing – original draft, Writing – review & editing, Methodology, Conceptualization, Supervision. DD: Formal analysis, Writing – review & editing, Data curation. JR: Funding acquisition, Supervision, Writing – review & editing. DB: Formal analysis, Funding acquisition, Writing – review & editing. MV: Supervision, Writing – review & editing. EL: Conceptualization, Supervision, Writing – review & editing, Formal analysis. AT: Formal analysis, Project administration, Supervision, Writing – review & editing.
Funding
The author(s) declare financial support was received for the research, authorship, and/or publication of this article. This work was funded by a grant from the Coordenação de Aperfeiçoamento de Pessoal de Nível Superior (CAPES) – Finance Code 001, via a master’s degree fellowship to DB, the FAPESP via a Post-Doctorate fellowship to JR (2017/01917-6) and the Pe. Theobaldo Frantz Fund via a doctorate fellowship to RC.
Acknowledgments
We are grateful to all private owners that allowed us to access their properties and the state environmental agencies that authorized the research in the conservation units. The collection and manipulation of specimens were authorized by ICMBio (#64962), state organs (IAP/PR #33/2018, IMA/SC #01/2019, SEMA/RS #37/2018) and the Animal Ethics Committee of Universidade do Vale do Rio dos Sinos (CEUA-UNISINOS # PPECEUA08.2018). Piter Kehoma Boll helped with the English translation and editing of the manuscript.
Conflict of interest
The authors declare that the research was conducted in the absence of any commercial or financial relationships that could be construed as a potential conflict of interest.
Publisher’s note
All claims expressed in this article are solely those of the authors and do not necessarily represent those of their affiliated organizations, or those of the publisher, the editors and the reviewers. Any product that may be evaluated in this article, or claim that may be made by its manufacturer, is not guaranteed or endorsed by the publisher.
Supplementary material
The Supplementary Material for this article can be found online at: https://www.frontiersin.org/articles/10.3389/famrs.2024.1433502/full#supplementary-material
References
Altig R., Johnston G. F. (1986). Major characteristics of free-living anuran tadpoles. Smithson. Herpetol. Inf. Serv. 67, 1–75. doi: 10.5479/si.23317515.67.1
Alvarado-Rybak M., Lepe-Lopez M., Peñafiel-Ricaurte A., Valenzuela-Sánchez A., Valdivia C., Mardones F. O., et al. (2021). Bioclimatic and anthropogenic variables shape the occurrence of Batrachochytrium dendrobatidis over a large latitudinal gradient. Sci. Rep. 11, 17383. doi: 10.1038/s41598-021-96535-w
Alvares C. A., Stape J. L., Sentelhas P. C., Gonçalves J. L. M., Sparovek G. (2013). Köppen’s climate classification map for Brazil. Meteorol. Z. 22, 711–728. doi: 10.1127/0941-2948/2013/0507
Bates K. A., Friesen J., Loyau A., Butler H., Vredenbrug V. T., Laufer A., et al. (2023). Environmental and anthropogenic factors shape the skin bacterial communities of a semi-arid amphibian species. Microb. Ecol. 86, 1393–1404. doi: 10.1007/s00248-022-02130-5
Bates D., Maechler M., Bolker B., Walker S. (2015). Fitting linear mixed-effects models using lme4. J. Stat. Software 67, 1–48. doi: 10.18637/jss.v067.i01
Becker C. G., Fonseca C. R., Haddad C. F. B., Prado P. I. (2010). Habitat split as a cause of local population declines of amphibians with aquatic larvae. Cons. Biol. 24, 287–294. doi: 10.1111/j.1523-1739.2009.01324.xB
Belasen A. M., Amses K. R., Clemons R. A., Becker G., Toledo F., James T. (2022). Habitat fragmentation in the Brazilian Atlantic Forest is associated with erosion of frog immunogenetic diversity and increased fungal infections. Immunogenetics 74, 431–441. doi: 10.1007/s00251-022-01252-x
Beyer S. E., Phillips C. A., Schooley R. L. (2015). Canopy cover and drought influence the landscape epidemiology of an amphibian chytrid fungus. Ecosphere 6, 78. doi: 10.1890/ES14-00263.1
Blaustein A., Romansic J. M., Schessele E. A., Han B. A., Pessier A. P., Longcore J. E. (2005). Interspecific variation in susceptibility of frog tadpoles to the pathogenic fungus Batrachochytrium dendrobatidis. Conserv. Biol. 19, 1460–1468. doi: 10.1111/j.1523-1739.2005.00195.x
Bolzan A. M. R., Saccol S. A., Santos T. G. (2016). Composition and diversity of anurans in the largest conservation unit in Pampa biome, Brazil. Biot. Neotrop. 16, e20150113. doi: 10.1590/16760611-BN-2015-0113
Both C., Sole M., Santos T. G., Cechin S. Z. (2009). The role of spatial and temporal descriptors for neotropical tadpole communities in southern Brazil. Hydrobiologia 624, 125–138. doi: 10.1007/s10750-008-9685-5
Bradley P. W., Brawner M. D., Raffel T. R., Rohr J. R., Olson D. H., Blaustein A. R. (2019). Shifts in temperature influence how Batrachochytrium dendrobatidis infects amphibian larvae. PloS One 14, e0222237. doi: 10.1371/journal.pone.0222237
Brunner J. L., Yarber C. M. (2018). Evaluating the importance of environmental persistence for ranavirus transmission and epidemiology. Adv. Virus Res. 101, 129–148. doi: 10.1016/bs.aivir.2018.02.005
Burnham K. P., Anderson D. R. (Eds.) (2002). Model selection and multimodel inference: a practical information-theoretic approach (New York, NY: Springer New York).
Cáceres M., Legendre P. (2009). Associations between species and groups of sites: indices and statistical inference. Ecology 90, 3566–3574. doi: 10.1890/08-1823.1
Cáceres M., Legendre P., Moretti M. (2010). Improving indicator species analysis by combining groups of sites. Oikos 119, 1674–1684. doi: 10.1111/j.1600-0706.2010.18334.x
Canessa S., Parris K. M. (2013). Multi-scale, direct and indirect effects of the urban stream syndrome on amphibian communities in streams. PloS One 8, e70262. doi: 10.1371/journal.pone.0070262
Carvalho T., Becker C. G., Toledo L. F. (2017). Historical amphibian declines and extinctions in Brazil linked to chytridiomycosis. Proc. R. Soc London Ser. B: Biol. Sc. 28, 20162254. doi: 10.1098/rspb.2016.2254
Conselho Nacional para o Controle de Experimentação Animal (CONCEA) (2018). Normative Resolution n° 37 (Brasília: National Council for Animal Experimentation Euthanasia Practice Directive). Official Diary.
CONSEMA – Conselho Estadual do Meio Ambiente (2011). Lista de Espécies da Fauna Ameaçadas de Extinção no Estado de Santa Catarina. Available online at: https://www.ima.sc.gov.br/index.php/biodiversidade/biodiversidade/fauna (Accessed 30 January 2022).
Corey S. J., Waite T. A. (2008). Phylogenetic autocorrelation of extinction threat in globally imperilled amphibians. Divers. Distrib. 14, 614–629. doi: 10.1111/j.1472-4642.2007.00448.x
Daszak P., Berger L., Cunningham A. A., Hyatt A. D., Green D., Speare R. (1999). Emerging infectious diseases and amphibian population declines. Emerg. Infect. Dis. 5, 1–23. doi: 10.3201/eid0506.990601
Daszak P., Cunningham A. A., Hyatt A. D. (2001). Anthropogenic environmental change and the emergence of infectious diseases in wildlife. Acta Trop. 78, 103–116. doi: 10.1016/S0001-706X(00)00179-0
Daszak P., Cunningham A. A., Hyatt A. D. (2003). Infectious disease and amphibian population declines. Divers. Distrib. 9, 141–150. doi: 10.1046/j.1472-4642.2003.00016.x
Delazeri F., Ernetti J. R., De Bastiani V. I. M., Lingnau R., Toledo L. F., Lucas E. M. (2021). Forest cover influences chytrid infections in populations of Boana curupi, a threatened treefrog of south Brazil. Dis. Aquat. Org. 144, 133–142. doi: 10.3354/dao03585
DiGiacomo R. F., Koepsell T. D. (1986). Sampling for detection of infection or disease in animal populations. J. Am. Vet. Med. Assoc. 189, 22–23.
Duffus A. L. J., Waltzek T. B., Stöhr A. C., Allender M. C., Gotesman M., Whittington R. J., et al. (2015). “Distribution and host range of ranaviruses,” in Ranaviruses: lethal pathogens of ectothermic vertebrates, vol. 9-57 . Eds. Gray M. J., Chinchar V. G. (Springer, New York).
Ernetti J. R., Boschetti J. P., Delazeri F., De Bastiani V. I. M., Pontes M. R., Ribeiro L. P., et al. (2020). High temporal and individual variation in the prevalence and intensity of chytrid infection in the southernmost leaf frog of the genus Pithecopus (Anura, Phyllomedusidae). Hydrobiologia 847, 3355–3364. doi: 10.1007/s10750-020-04339-2
Fernández-Loras A., Boyero L., Correa-Araneda F., Tejedo M., Hettyey A., Bosch J. (2019). Infection with Batrachochytrium dendrobatidis lowers heat tolerance of tadpole hosts and cannot be cleared by brief exposure to CTmax. PloS One 14, e0216090. doi: 10.1371/journal.pone.0216090
Fisher M. C., Garner T. W. J. (2020). Chytrid fungi and global amphibian declines. Nat. Rev. Microbiol. 18, 332–343. doi: 10.1038/s41579-020-0335-x
Forti L. R., Pontes M. R., Alcantara E. P., Morais D. H., Da Silva R. J., Dodonov P., et al. (2020). Torrent frogs have fewer macroparasites but higher rates of chytrid infection in landscapes with smaller forest cover. Ecosphere 11, e03169. doi: 10.1002/ecs2.3169
Frazer G. W., Canham C. D., Lertzman K. P. (1999). Manual do Gap Light Analyzer (GLA), Version 2.0: Imaging software to extract canopy structure and gap light transmission indices from true-color fisheye photographs, users manual and program documentation (Millbrook, New York: Simon Fraser University, Burnaby, British Columbia, and the Institute of Ecosystem Studies).
Frost D. R. (2023). Amphibian Species of the World: an Online Reference. Version 6.1 (New York: American Museum of Natural History). doi: 10.5531/db.vz.0001
FZRGS – Fundação Zoobotânica do Rio Grande do Sul (2014). Lista de espécies da fauna gaúcha ameaçadas de extinção. Available online at: https://www.al.rs.gov.br/filerepository/replegis/arquivos/dec%2051.797.pdf (Accessed 20 January 2022).
Garner T. W. J., Garcia G., Carroll B., Fisher M. C. (2009). Using itraconazole to clear Batrachochytrium dendrobatidis infection, and subsequent depigmentation of Alytes muletensis tadpoles. Dis. Aquat. Org. 83, 257–260. doi: 10.3354/dao02008
Gervasi S. S., Urbina J., Hua J., Chestnut T., Relyea R. A., Blaustein A. R. (2013). Experimental evidence for American bullfrog (Lithobates catesbeianus) susceptibility to chytrid fungus (Batrachochytrium dendrobatidis). EcoHealth 10, 166–171. doi: 10.1007/s10393-013-0832-8
Giam X. (2017). Global biodiversity loss from tropical deforestation. PNAS 114, 5775–5777. doi: 10.1073/pnas.1706264114
Gillespie T. R., Chapman C. A., Greiner E. C. (2005). Effects of logging on gastrointestinal parasite infections and infection risk in African primates. J. Appl. Ecol. 42, 699–707. Available at: https://www.jstor.org/stable/3505903.
Gonçalves D. D. S. (2014). Diversidade e chave de identificação para girinos ocorrentes em áreas de floresta com araucária. Universidade Federal do Paraná, Curitiba.
Gosner K. L. (1960). A simplified table for staging anuran embryos and larvae with notes on identification. Herpetologica 16, 183–190. Available at: https://www.jstor.org/stable/3890061.
Grayfer L., Edholm E. S., Andino F. D. J., Chinchar V. G., Robert J. (2015). “Ranavirus host immunity and immune evasion,” in Ranaviruses. Eds. Gray M. J., Chinchar V. G. (Springer, New York), 141–170.
Green D. E., Converse K. A., Schrader A. K. (2002). Epizootiology of sixty-four amphibian morbidity and mortality events in the USA 1996–2001. Ann. N.Y. Acad. Sci. 969, 323–339. doi: 10.1111/j.1749-6632.2002.tb04400.x
Haddad N. M., Brudvig L. A., Clobert J., Davies K. F., Gonzalez A., Holt R. D., et al. (2015). Habitat fragmentation and its lasting impact on Earth’s ecosystems. Sci. Adv. 1, e1500052. doi: 10.1126/sciadv.1500052
Haddad C. F. B., Toledo L. F., Prado C. P. A., Loebmann D., Gasparini J. L., Sazima I. (2013). Guide to the Amphibians of the Atlantic Forest: Diversity and Biology (São Paulo: Anolis Books).
Hanlon S. M., Lynch K. J., Kerby J., Parris M. J. (2015). Batrachochytrium dendrobatidis exposure effects on foraging efficiencies and body size in anuran tadpoles. Dis. Aq. Org. 112, 237–242. doi: 10.3354/dao02810
Heyer W. R. (1976). Studies on larval amphibian habitat partitioning. Smithson. Contr. Zool. 242, 1–27. doi: 10.5479/si.00810282.242
Hoverman J. T., Gray M. J., Haislip J. T., Miller D. L. (2011). Phylogeny, life history, and ecology contribute to differences in amphibian susceptibility to ranaviruses. EcoHealth 8, 301–319. doi: 10.1007/s10393-011-0717-7
Jenkinson T. S., Betancourt Román C. M., Lambertini C., Valencia-Aguilar A., Rodriguez D., Nunes-de-Almeida C. H., et al. (2016). Amphibian-killing chytrid in Brazil comprises both locally endemic and globally expanding populations. Mol. Ecol. 25, 2978–2996. doi: 10.1111/mec.13599
Jones K. E., Patel N. G., Levy M. A., Storeygard A., Balk D., Gittleman J. L., et al. (2008). Global trends in emerging infectious diseases. Nature 451, 990–994. doi: 10.1038/nature06536
Jordani M. X., Ouchi-De-Melo L. S., Queiroz C. S., Rossa-Feres D. C., Garey M. V. (2017). Tadpole community structure in lentic and lotic habitats: richness and diversity in the Atlantic Rainforest lowland. Herpetol. J. 27, 299–306.
Kärvemo S., Laurila A., Höglund J. (2019). Urban environment and reservoir host species are associated with Batrachochytrium dendrobatidis infection prevalence in the common toad. Dis. Aquat. Organ. 134, 33–42. doi: 10.3354/dao03359
Knapp R. A., Morgan J. A. T. (2006). Tadpole mouthpart depigmentation as an accurate indicator of chytridiomycosis, an emerging disease of amphibians. Copeia 2, 188–197. doi: 10.1643/0045-8511
Lambertini C., Becker C. G., Belasen A., Valencia-Aguilar A., Nunes-de-Almeida C. H., Betancourt-Román C. M., et al. (2021). Biotic and abiotic determinants of Batrachochytrium dendrobatidis infections in amphibians of the Brazilian Atlantic Forest. Fungal Ecol. 49, 100995. doi: 10.1016/j.funeco.2020.100995
Lambertini C., Rodrigues D., Brito F. B., Leite D. S., Toledo L. F. (2013). Diagnóstico do fungo quitrídio: Batrachochytrium dendrobatidis. Herpetol. Bras. 2, 12–17.
Latney L. V., Klaphake E. (2020). Selected emerging infectious diseases of amphibians. Vet. Clin. North Am. Exot. Anim. Pract. 23, 397–412. doi: 10.1016/j.cvex.2020.01.003
Lips K. R., Reeve J. D., Witters L. R. (2003). Ecological traits predicting amphibian population declines in Central America. Conserv. Biol. 17, 1078–1088. doi: 10.1046/j.1523-1739.2003.01623.x
Longcore J. E., Pessier A. P., Nichols D. K. (1999). Batrachochytrium dendrobatidis gen. et sp. nov., a Chytrid Pathogenic to Amphibians. Mycologia 91, 219–227. doi: 10.1080/00275514.1999.12061011
MaChado I. F., Maltchik L. (2007). Check-list of diversity of anurans in Rio Grande do Sul (Brazil) and a classification propose for larval forms. Neotrop. Biol. Conserv. 2, 101–116.
Madalozzo B., Both C., Cechin S. (2013). Temporal distribution and age structure of tadpoles of Hypsiboas faber and H. leptolineatus in ponds: how do they coexist? J. Nat. Hist. 47, 2575–2581. doi: 10.1080/00222933.2013.773100
Marques N. C. S., Rattis L., Nomura F. (2018). Local environmental conditions affecting anuran tadpoles’ microhabitat choice and morphological adaptation. Mar. Freshw. Res. 70, 395–401. doi: 10.1071/MF18106
Martel A., Adriaensen C., Sharifian-Fard M., Slouijs A. S. V. D., Louette G., Baert K., et al. (2013). The absence of zoonotic agents in invasive bullfrogs (Lithobates catesbeianus) in Belgium and the Netherlands. EcoHealth 10, 344–347. doi: 10.1007/s10393-013-0864-0
Martins M. (1993). Observations on nest dynamics and embryonic and larval development in the nest building gladiator frog, Hyla faber. Amphibia-Reptilia 14, 411–421. doi: 10.1163/156853893X00101
Mazzoni R., de Mesquita A., Fleury L., Brito W. M. E. D., Nunes I. A., Robert J., et al. (2009). Mass mortality associated with a frog virus 3-like Ranavirus infection in farmed tadpoles Rana catesbeiana from Brazil. Dis. Aquat. Org. 86, 181–191. doi: 10.3354/dao02096
McMillan K. M., Lesbarrères D., Harrison X. A., Garner T. W. J. (2020). Spatiotemporal heterogeneity decouples infection parameters of amphibian chytridiomycosis. J. Anim. Ecol. 89, 1109–1121. doi: 10.1111/1365-2656.13170
Melo L. S. O., Gonçalves-Souza T., Garey M. V., Cerqueira D. (2017). Tadpole species richness within lentic and lotic microhabitats: an interactive influence of environmental and spatial factors. Herpetol. J. 27, 339–345.
Miaud C., Arnal V., Poulain M., Valentini A., Dejean T. (2019). eDNA increases the detectability of ranavirus infection in an alpine amphibian population. Viruses 11, e526. doi: 10.3390/v11060526
Miller D., Gray M., Storfer A. (2011). Ecopathology of ranaviruses infecting amphibians. Viruses 3, 2351–2373. doi: 10.3390/v3112351
Mittermeier R. A., Fonseca G. A. B., Rylands A. B., Brandon K. (2005). A brief history of biodiversity conservation in Brazil. Conserv. Biol. 19, 601–611. doi: 10.1111/j.1523-1739.2005.00709.x
Mittermeier R. A., Turner W. R., Larsen F. W., Brooks T. M., Gascon C. (2011). “Global biodiversity conservation: the critical role of hotspots,” in Biodiversity hotspots. Eds. Zachos F. E., Habel J. C. (Springer, Berlin, Heidelberg), 3–22. doi: 10.1007/978-3-642-20992-5_1
Muletz-Wolz C. R., Barnett S. E., DiRenzo G. V., Zamudio K. R., Toledo L. F., James T. Y., et al. (2019). Diverse genotypes of the amphibian-killing fungus produce distinct phenotypes through plastic responses to temperature. J. Evol. Biol. 32, 287–298. doi: 10.1111/jeb.13413
Narayan E. J., Graham C., McCallum H., Hero J. M. (2014). Over-Wintering Tadpoles of Mixophyes fasciolatus Act as Reservoir Host for Batrachochytrium dendrobatidis. PloS One 9, e92499. doi: 10.1371/journal.pone.0092499
Neves-Da-Silva D., Borges-Júnior V. N. T., Branco C. W. C., Carvalho-e-Silva A. M. P. T. (2021). Effects of intrinsic and extrinsic factors on the prevalence of the fungus Batrachochytrium dendrobatidis (Chytridiomycota) in stream tadpoles in the Atlantic Forest domain. Aquat. Ecol. 55, 891–902. doi: 10.1007/s10452-021-09869-y
Nichols D. K., Lamirande E. W., Pessier A. P., Longcore J. E. (2001). Experimental transmission of cutaneous chytridiomycosis in dendrobatid frogs. J. Wildl. Dis. 37, 1–11. doi: 10.7589/0090-3558-37.1.1
O’Hanlon S. J., Rieux A., Farrer R. A., Rosa G. M., Waldman B., Bataille A., et al. (2018). Recent Asian origin of chytrid fungi causing global amphibian declines. Science 360, 621–627. doi: 10.1126/science.aar1965
Oliveira M., Aver G. F., Moreira L. F. B., Colombo P., Tozetti A. M. (2016). Daily Movement and Microhabitat Use by the Blacksmith Treefrog Hypsiboas faber (Anura: Hylidae) during the Breeding Season in a Subtemperate Forest of Southern Brazil. S. Am. J. Herpetol. 11, 89–97. doi: 10.2994/SAJH-D-16-00017.1
Pessier A. P., Nichols D. K., Longcore J. E., Fuller M. S. (1999). Cutaneous Chytridiomycosis in Poison Dart Frogs (Dendrobates spp.) and White’s Tree Frogs (Litoria caerulea). J. Vet. Diag. Invest. 11, 194–199. doi: 10.1177/104063879901100219
Pillar V. P., Vélez E. (2010). Extinção dos Campos Sulinos em Unidades de Conservação: um Fenômeno Natural ou um Problema Ético? Nat. Conserv. 8, 84–86. doi: 10.4322/natcon.00801014
Piotrowski J. S., Annis S. L., Longcore J. E. (2004). Physiology of Batrachochytrium dendrobatidis, a chytrid pathogen of amphibians. Mycologia 96, 9–15.
Preuss J. F. P., Greenspan S. E., Rossi E. M., Gonsales E. M. G., Neely W. J., Valiati V. H., et al. (2020). Widespread pig farming practice linked to shifts in skin microbiomes and disease in pond-breeding amphibians. Environ. Sci. Technol. 54, 11301–11312. doi: 10.1021/acs.est.0c03219
Preuss J. F., Lambertini C., Leite D. S., Toledo L. F., Lucas E. M. (2016). Crossing the threshold: an amphibian assemblage highly infected with Batrachochytrium dendrobatidis in the southern Brazilian Atlantic Forest. Stud. Neotrop. Fauna Environ. 51, 68–77. doi: 10.1080/01650521.2016.1163857
Rachowicz L. J., Vredenburg V. T. (2004). Transmission of Batrachochytrium dendrobatidis within and between amphibian life stages. Dis. Aquat. Organ. 61, 75–83. doi: 10.3354/dao061075
Ribeiro M. C., Metzger J. P., Martensen A. C., Ponzoni F. J., Hirota M. M. (2009). The Brazilian Atlantic Forest: How much is left, and how is the remaining forest distributed? Implications for conservation. Biol. Conserv. 142, 1141–1153. doi: 10.1016/j.biocon.2009.02.021
Ruggeri J., Martins A. G. D. S., Domingos A. H. R., Santos I., Viroomal I. B., Toledo L. F. (2020). Seasonal prevalence of the amphibian chytrid in a tropical pond-dwelling tadpole species. Dis. Aquat. Org. 142, 171–176. doi: 10.3354/dao03539
Ruggeri J., Pontes M. R., Ribeiro L. P., Gendreau K. L., Sousa R. L. M., Toledo L. F. (2023). Predominant prevalence of Ranavirus in southern Brazil, a region with widespread occurrence of the amphibian chytrid. Anim. Conserv. 27, 338–349. doi: 10.1111/acv.12911
Ruggeri J., Ribeiro L. P., Pontes M. R., Toffolo C., Candido M., Carriero M. M., et al. (2019). Discovery of wild amphibians infected with ranavirus in Brazil. J. Wildl. Dis. 55, 897–902. doi: 10.7589/2018-09-224
Ruggeri J., Toledo L. F., Carvalho-e-Silva S. P. (2018). Stream tadpoles present high prevalence but low infection loads of Batrachochytrium dendrobatidis (Chytridiomycota). Hydrobiologia 806, 303–311. doi: 10.1007/s10750-017-3367-0
Santos R. C., Bastiani V. I. M., Medina D., Ribeiro L. P., Pontes M. R., Leite D. S., et al. (2020). High prevalence and low intensity of infection by Batrachochytrium dendrobatidis in rainforest bullfrog populations in southern Brazil. Herpetol. Conserv. Biol. 15, 118–130.
Santos R. C., Dalmolin D. A., Brum D., Veronez M. R., Lucas E. M., Tozetti A. M. (2021). Association between land use and composition of amphibian species in temperate Brazilian forest habitats. Herpetol. Conserv. Biol. 16, 612–623.
Santos T. G., Rossa-Feres D. C., Casatti L. (2007). Diversidade e distribuição espaço-temporal de anuros em região com pronunciada estação seca no sudeste do Brasil. Iheringia Série Zool. 97, 37–49. doi: 10.1590/S0073-47212007000100007
Sapsford S. J., Alford R. A., Schwarzkopf L. (2018). Disentangling causes of seasonal infection prevalence patterns: tropical tadpoles and chytridiomycosis as a model system. Dis. Aquat. Organ. 130, 83–93. doi: 10.3354/dao03269
Scheele B. C., Pasmans F., Skerratt L. F., Berger L., Martel A., Beukema W., et al. (2019). Amphibian fungal panzootic causes catastrophic and ongoing loss of biodiversity. Science 363, 1459–1463. doi: 10.1126/science.aav0379
Semlitsch R. D., Bodie J. R. (2003). Biological criteria for buffer zones around wetlands and riparian habitats for amphibians and reptiles. Conserv. Biol. 17, 1219–1228. doi: 10.1046/j.1523-1739.2003.02177.x
Severns P., Sykes E. M. (2020). Indicator Species Analysis: a useful tool for plant disease studies. Phytopathology 110, 1860–1862. doi: 10.1094/PHYTO-12-19-0462-LE
Silva A. A. P., Franquelino A. R., Teodoro P. E., Montanari R., Faria G. A., Ribeiro da Silva C. H., et al. (2022). The fewer, the better fare: Can the loss of vegetation in the Cerrado drive the increase in dengue fever cases infection? PloS One 17, e0262473. doi: 10.1371/journal.pone.0262473
Soares F. S., Almeida R. K., Rubim I. B., Barros R. S., Cruz C. B. M., Mello G. V., et al. (2015). “Análise comparativa da correção atmosférica de imagem do Landsat 8: o uso do 6S e do ATCOR2,” in Simpósio Brasileiro de Sensoriamento Remoto. Eds. Gherardi D. F. M., Aragão L. E. O. C. (Instituto Nacional de Pesquisas Espaciais, João Pessoa), 1821–1825.
Sonn J. M., Berman S., Richards-Zawacki C. L. (2017). Influence of temperature on chytridiomycosis in vivo. EcoHealth 14, 762–770. doi: 10.1007/s10393-017-1269-2
Valencia-Aguilar A., Toledo L. F., Vital M. V., Mott T. (2016). Seasonality, environmental factors, and host behavior linked to disease risk in stream-dwelling tadpoles. Herpetologica 72, 98–106. doi: 10.1655/HERPETOLOGICA-D-15-00013
Vasconcelos T. S., Rossa-Feres D. C. (2005). Diversidade, distribuição espacial e temporal de anfíbios anuros (Amphibia, Anura) na região noroeste do Estado de São Paulo. Biot. Neotrop. 5, 1–14. doi: 10.1590/S1676-06032005000300010
Veloso H. P., Goés-Filho L. (1982). Fitogeografia brasileira: classificação fisionômico-ecológica da vegetação neotropical (Salvador: Ministério das Minas e Energia-Secretaria Geral-Projeto RADAM BRASIL).
Vieira C. A., Toledo L. F., Longcore J. E., Longcore J. R. (2013). Body length of Hylodes cf. ornatus and Lithobates catesbeianus tadpoles, depigmentation of mouthparts, and presence of Batrachochytrium dendrobatidis are related. Braz. J. Biol. 73, 195–199. doi: 10.1590/S1519-69842013000100021
Voyles J., Young S., Berger L., Campbell C., Voyles W. F., Dinudom A., et al. (2009). Pathogenesis of chytridiomycosis, a cause of catastrophic amphibian declines. Science 326, 582–585. doi: 10.1126/science.1176765
Wanderley R. L. N., Domingues L. M., Joly C. A., Rocha H. R. (2019). Relationship between land surface temperature and fraction of anthropized area in the Atlantic forest region, Brazil. PloS One 14, e0225443. doi: 10.1371/journal.pone.0225443
Keywords: amphibians, pathogens, environmental changes, freshwater ecosystems, indicator species, community-indicator, forest remnants
Citation: Santos RCd, Dalmolin DA, Ruggeri J, Brum D, Veronez MR, Lucas EM and Tozetti AM (2024) Influence of landscape on the presence of Batrachochytrium dendrobatidis and Ranavirus in tadpoles in the southern Atlantic Forest of Brazil. Front. Amphib. Reptile Sci. 2:1433502. doi: 10.3389/famrs.2024.1433502
Received: 16 May 2024; Accepted: 30 July 2024;
Published: 15 August 2024.
Edited by:
Gabriela Parra-Olea, National Autonomous University of Mexico, MexicoReviewed by:
Adua Olvera, National Autonomous University of Mexico, MexicoRaluca Ioana Bancila, Romanian Academy, Romania
Copyright © 2024 Santos, Dalmolin, Ruggeri, Brum, Veronez, Lucas and Tozetti. This is an open-access article distributed under the terms of the Creative Commons Attribution License (CC BY). The use, distribution or reproduction in other forums is permitted, provided the original author(s) and the copyright owner(s) are credited and that the original publication in this journal is cited, in accordance with accepted academic practice. No use, distribution or reproduction is permitted which does not comply with these terms.
*Correspondence: Roseli Coelho dos Santos, cm9zZWxpY2Jpb0BnbWFpbC5jb20=
†Deceased