- 1Centro de Museos, Museo de Historia Natural, Universidad de Caldas, Manizales, Colombia
- 2Centro de Colecciones y Gestión de Especies, Instituto de Investigación de Recursos Biológicos Alexander von Humboldt, Villa de Leyva, Colombia
- 3Grupo de Investigación en Biología Matemática y Computacional (BIOMAC), Departamento de Ingeniería Biomédica, Universidad de los Andes, Bogotá, Colombia
- 4Universidad de los Andes, Centro de Investigaciones en Microbiología y Parasitología Tropical, Bogotá, Colombia
- 5Departamento de Zoologia, Instituto de Ciências Biológicas Universidade Federal de Minas Gerais Av. Antônio Carlos, Belo Horizonte, MG, Brazil
- 6Department of Herpetology, Division of Vertebrate Zoology, American Museum of Natural History, New York, NY, United States
Snakes have diverse and unique sensory systems that make them extremely efficient at moving through the environment while detecting prey and predators and performing courtship behaviors. In recent years, the number of studies on the mechanoreception of aquatic snakes has increased, principally focusing on the ecological and sexual roles of cephalic mechanoreceptors or sensilla. However, few studies have focused on the presence and role of corporal mechanoreceptors sensilla in freshwater snakes. This study describes the morphology of dome-shaped corporal scale sensilla for the first time in three species of aquatic Neotropical snakes (Helicops angulatus, Helicops danieli, and Helicops pastazae), using histological sections and Scanning Electron Microscopy (SEM). Histological sections revealed that the corporal sensilla resemble the previously described cephalic sensilla with a thinner beta keratin layer above the sensillum, and a group of central cells. Further, SEM images show dome-shaped protuberant organs with concentric rings. To infer possible ecological and sexual roles in corporal sensilla, we employed mixed ANOVA permutation tests to assess for differences in the number and area of sensilla between the dorsal and lateral position of the scales, and the anterior and posterior corporal region, as well as among species and sexes. Our results show that individuals across all species consistently exhibited a higher number of mechanoreceptors sensilla in the anterior and lateral region when compared to the posterior or midbody dorsal region. We qualitatively identified that scale sensilla are usually restricted to the keeled portion of the scale in the dorsal region, but are spread out throughout the scale in the lateral region. We also found differences in the average sensillum area between species, with H. angulatus exhibiting larger sensilla than H. danieli. Our results showed no evidence of sexual dimorphism in the number or area of corporal sensilla. These findings are the first to report corporal dome shaped mechanoreceptors in freshwater snakes and contribute to the understanding of mechanosensory systems in these organisms by elucidating the morphology, quantity, distribution and possible function of these corporal scale sensilla.
Introduction
Sensory systems play a crucial role in how animals interact with their environment, allowing them to perceive external stimuli such as the location of prey, mates, or predators (Schraft and Clark, 2019). Snakes particularly stand out for having extraordinary sensory systems that are unique and efficient. For example, the ability to detect infrared or thermal radiation and form a clear three-dimensional image with the help of the visual system is unique for some groups of pythons, boas, and vipers (Lillywhite, 2014; Schraft and Clark, 2019). Most snakes are known to efficiently detect chemical stimuli through vomeronasal chemoreception. For instance, male rattlesnakes can detect a potential mate from distances of up to one kilometer (Duvall et al., 1992). Snakes also display great diversity in ocular anatomy from fossorial species with small and underdeveloped eyes compared to well-developed binocular vision in many terrestrial or arboreal species (Simões et al., 2016; Segall et al., 2021). Other cutaneous sensory systems used to detect vibrations such as mechanoreception have previously been described in fossorial snakes (Jackson, 1977), and have recently received more attention in aquatic snakes, such as sea snakes (Crowe-Riddell et al., 2016, Crowe-Riddell et al., 2019), and freshwater species (Van Der Kooij and Povel, 1996; Catania et al., 2010; García-Cobos et al., 2021; García-Cobos and Vásquez-Restrepo, 2022).
Colonization of aquatic habitats changes snakes’ abilities to perceive external stimuli due to the contrasting physical properties between terrestrial and aquatic environments. For instance, water influences light transmission through scattering and absorption, resulting in a broader visual spectrum in shallow waters compared to deeper ones where shorter waves of around 475 nanometers predominate (Seiko et al., 2020). Thus, as depth increases, the availability of light diminishes. Consequently, sea snakes have experienced accelerated diversification of their visual pigments to evolve higher sensitivity towards the wavelengths that predominate below the sea surface (Seiko et al., 2020; Simões et al., 2020). Chemoreception in snakes also undergoes significant changes when transitioning from terrestrial to aquatic environments. For example, Kishida et al. (2019) reported that sea snakes exhibit fewer olfactory receptor (OR) genes than their terrestrial relatives. This may be explained by the slower chemical diffusion experienced underwater, whereas air molecules are transported faster due to their volatile properties (Segall et al., 2021). Conversely, given that sound travels faster and more effectively in water than in air (Segall et al., 2021), it is reasonable to assume that aquatic snakes possess highly developed and sensitive mechanoreceptive sensory systems.
Mechanoreceptors have primarily been described in the cephalic region of aquatic snakes. An extraordinary case of specialized mechanoreception occurs in the tentacled snake Erpeton tentaculatum, which has a unique pair of tentacles that detect water movements with remarkable sensitivity, perceiving forces as minimal as 0.008 g generated by potential prey in the turbid waters they inhabit (Catania et al., 2010). Also, Hydrophiinae sea snakes (Crowe-Riddell et al., 2016, Crowe-Riddell et al., 2019) and freshwater snakes from the genus Helicops (García-Cobos et al., 2021) have “dome-shaped’’ cephalic mechanosensory sensilla concentrated around the mouth, suggesting that these sensory organs may improve their underwater hunting ability. A third type of cephalic mechanoreceptor sensilla is described in the aquatic Wart Snakes from the Acrochordus genus, characterized by filiform-shaped organs (Van Der Kooij and Povel, 1996).
In addition to cephalic sensilla, some aquatic snakes have corporal mechanoreceptive organs throughout their body. The hair-like or filiform sensilla observed in the cephalic region of Wart snakes are also present in corporal scales around the neck and dorsum region of the body (Van Der Kooij and Povel, 1996). Males of the sea snake Emydocephalus annulatus have a second type of corporal mechanoreceptor known as anal knobs near their cloaca that may function as tactile organs for coordination and intromission of the hemipenes during mating (Crowe-Riddell et al., 2021). Tail sensilla have also been described in the sea snake Aipysurus laevis, where the central cells displace the stratum germinativum but do not result in a protuberance. This may indicate a less specialized mechanoreceptor that exhibits differences in mechanosensitivity compared to cephalic sensilla (Crowe-Riddell et al., 2019).
Helicops freshwater snakes from South America, also commonly known as “Mapaná de agua”, are nocturnal species that inhabit a variety of aquatic habitats ranging from lentic to lotic bodies of water (Schöneberg and Köhler, 2022). In Colombia H. angulatus, H. danieli, and H. pastazae are abundant species with distinct habitat occupations and feeding ecologies. For example, H. angulatus and H. danieli inhabit temporary or slow-flowing waters, such as swamps (Carvajal-Cogollo et al., 2007; Rocha and López-Baucells, 2014). Due to their generalist habitats, both species can also be found in disturbed areas such as fish production ponds or flooded pastures (Acosta-Ortiz and Vos, 2023), and may feed on a variety of prey such as fish, tadpoles, adult anurans, lizards, and invertebrates (Ford and Ford, 2002; Acosta-Ortiz and Vos, 2023). On the contrary, H. pastazae only inhabits mountain rivers, typically characterized by strong currents, and has a restricted diet to aquatic prey like fish and tadpoles (Almendáriz et al., 2017; García-Cobos et al., 2020). Interestingly, these three species exhibit sexual dimorphism with females larger in size than males (Ford and Ford, 2002; García-Cobos et al., 2020; Citeli et al., 2022).
The present study describes the morphology of corporal mechanoreceptors sensilla in H. angulatus, H. danieli, and H. pastazae by using scanning microscopy (SEM) and histological techniques. We further quantify the interspecific and sexual variation of corporal scale sensilla in these species accounting for sex and body region (dorsal vs lateral, and anterior to posterior corporal regions). We first hypothesize that scale sensilla will not be evenly distributed across the body, predicting that they will be more abundant in the anterior and lateral regions of the body, compared to the posterior and dorsal regions, due to the role that mechanoreceptors may play in enhancing foraging and predator detection. Second, we hypothesize that corporal sensilla will differ between species, predicting that the most aquatic and fish-specialized species, H. pastazae, will have larger and more corporal sensilla compared to H. angulatus and H. danieli, due to the difficulty of catching prey and detecting predators in mountain rivers. Third, we hypothesize that traits of corporal mechanoreceptors would differ between sexes and predict that males will have a greater number of sensilla compared to females, as males tend to be more active in searching for food and mates, as has been demonstrated in other snake species (King and Duvall, 1990).
Materials and methods
Specimens
We quantified corporal mechanoreceptors in 111 specimens of males and females of Helicops angulatus, H. danieli, and H. pastazae, deposited at the Reptile Collection of the Instituto de Investigación de Recursos Biológicos Alexander von Humboldt (IAvH-R) (Supplementary Table 1). Individuals were sexed through a subcaudal incision to detect the presence or absence of hemipenis and retractor muscles. We measured the snout-vent length (SVL) and tail length (TL) with a non-elastic string along the ventral region which was then measured with a ruler. These measurements were taken three times and then averaged to acquire a more precise size of the individuals. To avoid ontogeny effects, we selected only adults based on the minimum size of sexual maturity reported in the literature for H. pastazae (276 mm SVL in males, and 400 mm SVL in females, García-Cobos et al., 2021) and H. angulatus (228 mm SVL in males, and 336 mm SVL in females, dos Santos Costa et al., 2022). In the case of H. danieli, this information has not been recorded, thus we selected males and females larger than the minimum size of sexual maturity recorded for the other two species.
Histology
To describe the internal morphology of the corporal scale sensilla, we performed histological sections of five anterior dorsal scales (Figure 1) from individuals of each sex and species (H. pastazae, H. angulatus, and H. danieli) including both dermal and epidermal tissue. We included five scales per individual to increase the probability of obtaining a sensillum in the histological sections. The scales were fixed in 10% formalin and were subsequently dehydrated for 30 minutes in ethanol dilutions (70%, 80%, 90%, 96%, and 100%), rinsed with xylene, and embedded in paraffin. Then, paraffin blocks were cut transversely into 5-μm slides using a rotatory microtome from the Laboratory of Veterinary Pathology and Biology at the Universidad Nacional de Colombia. Slices were then stained with hematoxylin and eosin and photographed using light optical microscopy Leica DM750 with a camera Leica ICC 50W.
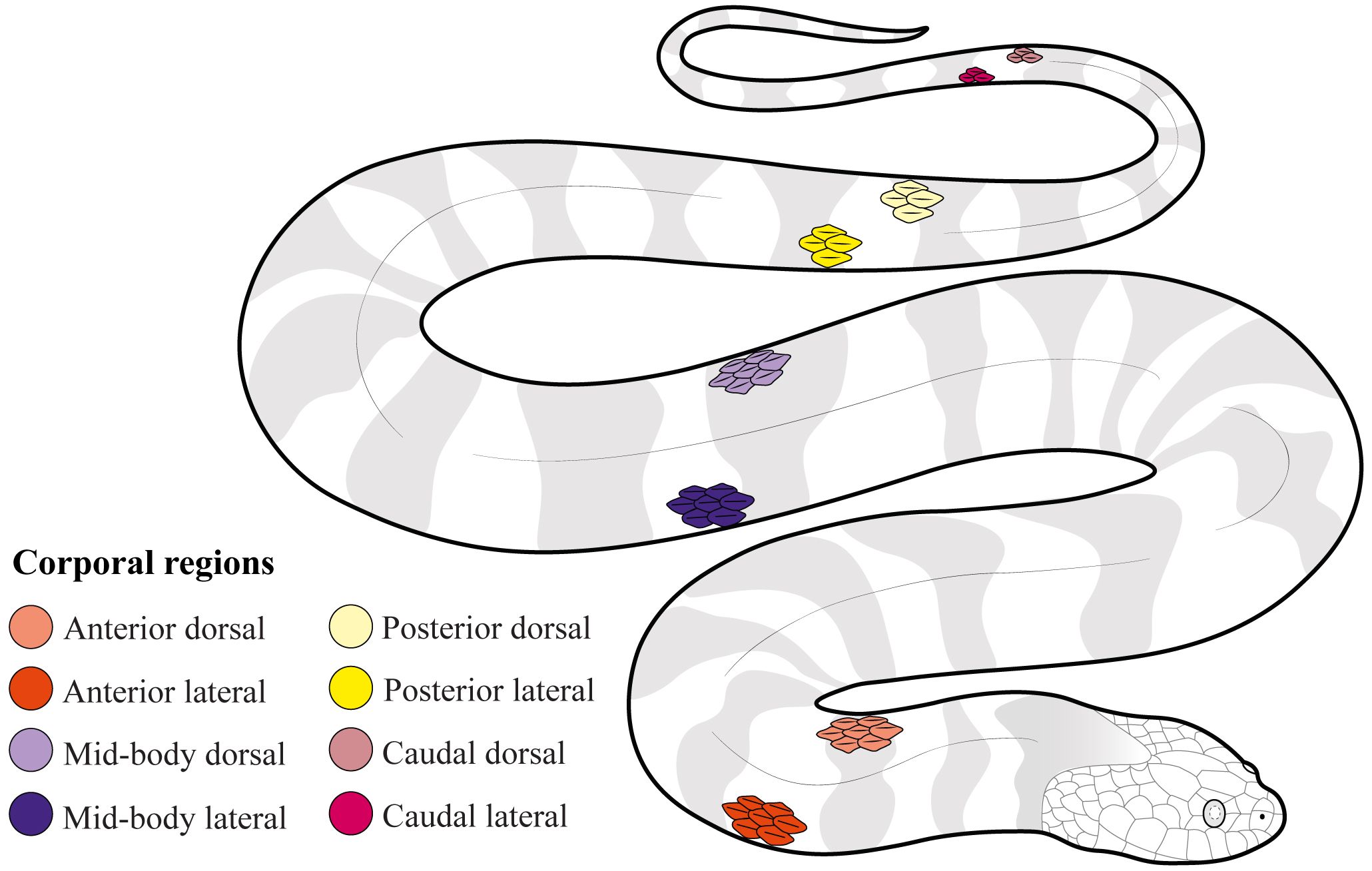
Figure 1 Distribution of the corporal scales used in this study to quantify differences across corporal regions (anterior, mid-body, posterior, and caudal), position of scale (dorsal vs lateral), species, and sexes in Helicops.
Scanning electron microscopy
To characterize the external morphology of the sensillum, anterior dorsal scales (Figure 1) of one male and one female of each species were analyzed, using a Tescan Lyra 3 scanning electron microscope. Scales were dissected from specimens previously fixed in 10% formaldehyde and preserved in 70% ethanol. The last layer of beta-keratin was removed with fine forceps to control for possible differences in the morphology of the scale sensilla due to different shedding stages of individuals. Scales were then fixed in 2.5% glutaraldehyde for 24 hours at room temperature (18°C) and dehydrated by serial dilution of ethanol (30%, 50%, 70%, 96%, and 100%), for 30 minutes each. Posteriorly, scales were dried to the critical point (SAMDR-795) for 1 hour and coated with gold for 45 seconds in a sputter-coating machine (DentomVacuumDesk IV). Finally, the scales were imaged at 5 kV with a magnification of 3.00 kx- 4.00 kx for images of a single sensillum and 245x for images of the entire scale showing multiple scale sensilla.
Quantification of corporal scale sensilla
To quantify the number of sensilla and sensillum average area in corporal mechanoreceptors, we extracted the outer epidermal layer of the snake’s skin (beta keratin layer) with fine forceps or entomological needles and placed it on a microscope slide. Mechanoreceptors were seen as translucent circles when exposed to light under the stereoscope due to the reduction of the keratin layer just above the sensillum (Jackson and Sharawy, 1980; García-Cobos et al., 2021; Riedel and Schwarzkopf, 2022). To evaluate differences in the number of sensilla and sensillum average area between the anterior and posterior corporal regions and between the dorsal and lateral positions of the scales, we extracted, when possible, one scale from each one of eight regions for each individual (Figure 1). These regions were standardized as follows: 1) Anterior dorsal region (AD), located at the tenth ventral scale on a dorsal position; 2) Anterior lateral region (AL), located at the tenth ventral scale on a lateral position; 3) Mid-body dorsal region (MD), located at the middle part of the body between the head and the cloaca, on a dorsal position; 4) Mid-body lateral region (ML), located at the middle of the body between the head and cloaca on a lateral position; 5) Posterior dorsal region (PD), located ten ventral scales before the cloaca on a dorsal position; 6) Posterior lateral region (PL), located ten ventral scales before the cloaca on a lateral position; 7) Caudal dorsal region (CD), located at the eight subcaudal scale on a dorsal position; and 8) Caudal lateral region (CL), located at the eight subcaudal scale on a lateral position. We extracted scales from 14 males and 20 females of H. danieli, 15 males and 21 females of H. angulatus, and 20 males and 21 females of H. pastazae, for a total of 111 individuals and 839 scales. However, we were not able to include all specimens in the statistical analysis due to the unbalanced data (see statistical analysis below).
Photographic images were taken for each one of the scales using a LEICA S8APO stereoscope and a LEICA DM190HD camera with a 1 mm scale bar. These images were used to estimate the scale area, number of sensilla, and sensillum average area using the Image-J V.1.53 software. In addition to counting and estimating their size, we also describe qualitatively the distribution of mechanoreceptors within the scale, specifying if the sensilla were found above the keel of the scale or dispersed around the scale.
Allometry effects
Sexual dimorphism is evident within the Helicops genus, with females attaining larger sizes than males (García-Cobos et al., 2021; Citeli et al., 2022). Thus, to account for allometric effects on the number of sensilla and sensillum average area we tested for the effect of scale area by conducting a mixed-effect permutation ANCOVA using the ‘permuco’ package (version 1.1.2; Frossard and Renaud, 2021) in R (version 4.3.1), given the non-parametric and non-homoscedastic nature of the dataset. This mixed-effect framework controls for pseudo-replication issues arising from examining factors regarding scale location in the same individual by using a random effect based on within-group factors (position of scale and corporal region), and factors between individuals defined as scale area, sex, and species. We found that only the average sensillum area was positively correlated to scale area, and therefore we size-corrected this variable by obtaining residuals from a normal linear regression between these two variables (Supplementary Table 2). These residuals are hereafter used in the analysis of the sensilla area.
Statistical analysis
The dataset of 111 individuals and 839 scales was unbalanced, meaning that we did not have complete and equally distributed data for all the scales in males and females of the three species (Supplementary Table 1). Given the unbalance in the study design, permutation analyses may present bias as a consequence of different permuted probabilities between the combinations of levels (Kerr, 2009). To correct this, we excluded individuals with incomplete data, specifically those lacking data for all eight scales, resulting in the removal of 22 individuals, leaving a total of 89 individuals. After balancing the data to contain all the scales, we proceeded to balance the dataset to an even number of samples for each species and sex, resulting in 11 individuals per combination of categories. Then, we conducted 100 resamplings of our data by randomly choosing the minimum number of individuals per species and sex, resulting in 100 balanced datasets for 66 individuals. We then performed a mixed-effect permutation ANOVA for each balanced dataset using the ‘permuco’ package (version 1.1.2; Frossard and Renaud, 2021) in R (version 4.3.1) to test and determine differences in the number of sensilla and sensillum average area between species, sex, corporal region, and position of scale. We defined position of scale and corporal region as within-group factors controlling for specimen ID, whereas sex and species were defined as between-group factors. We then tabulated the number of resamples where each factor or interaction between factors reached significance (P-value < 0.01). Employing the same significance threshold, we identified factors or interactions as significant if at least 99% of the bootstrap iterations were significant. We performed post-hoc pairwise comparisons by bootstrapping the differences of the marginal means between the levels of the factors determined as significant in the previous mixed-effect permutation ANOVA using the ‘emmeans’ R package (version 1.10.0; Lenth, 2021).
Results
Histology
Overall, the transverse histological sections of the anterior dorsal scales in Helicops reveal a structure in the dermis and epidermis similar to that of other squamates. The dermis is composed of fibrous connective tissue, irrigated with blood vessels, as well as pigmentary cells or melanophores (Figure 2). The epidermis consists of the stratum germinativum characterized by cuboidal dividing cells or keratinocytes, along with keratinized layers of alpha keratin, followed by the beta keratin layer in the more external region of the scale. In H. angulatus (Figure 2A) and H. pastazae (Figure 2C), the corporal scale sensilla detected in these histological sections were located above the keel, while in H. danieli (Figure 2B), the sensilla were situated outside of the keel.
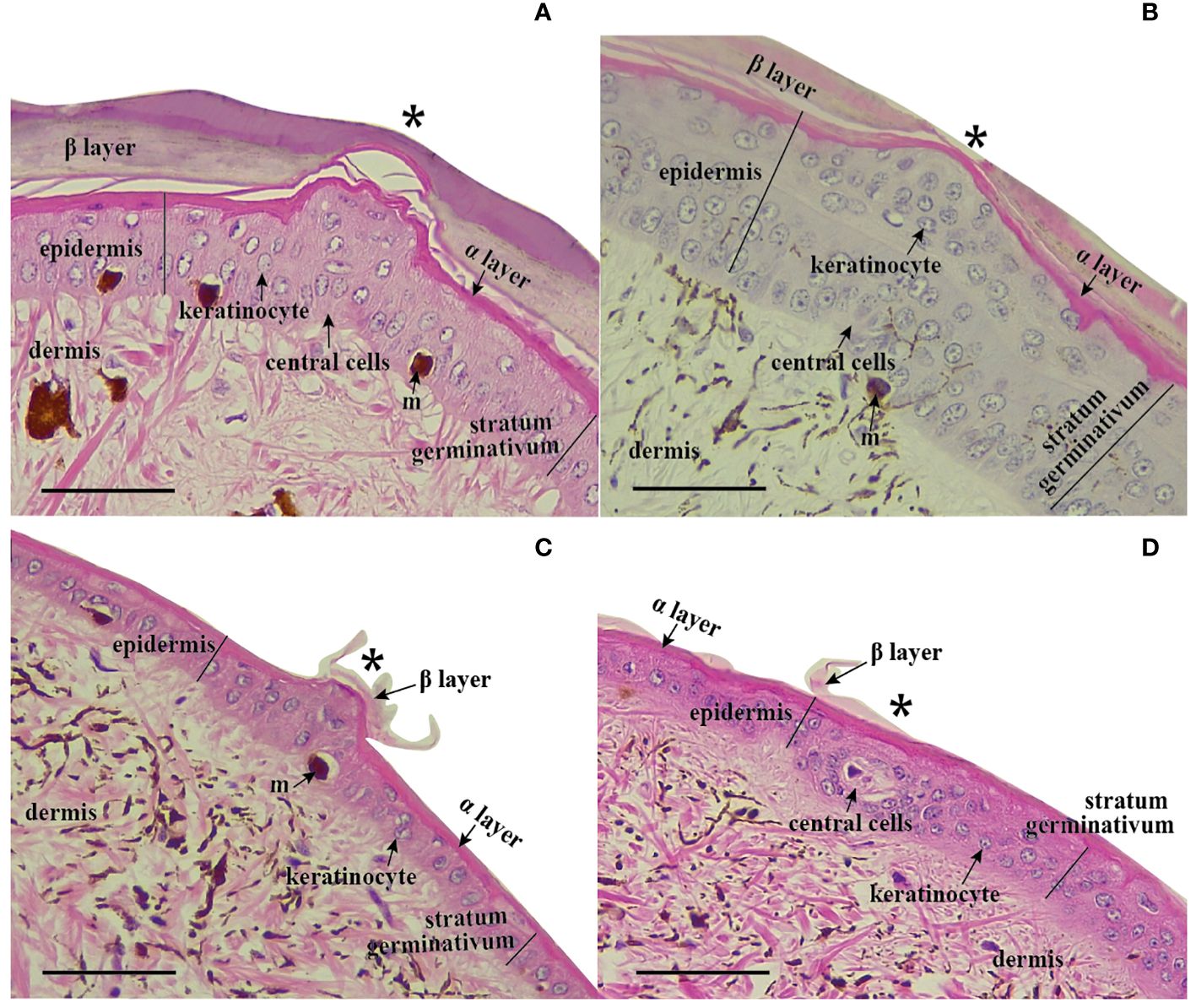
Figure 2 Histological sections of corporal scale sensilla in Helicops. Scale sensilla are denoted with a (*). Protuberant scale sensilla in (A) H. angulatus, (B) H. pastazae, and (C) H. danieli. (D) Non-protuberant scale sensilla in the corporal scales of H. danieli. All photographs were taken at 40X magnitude. Scale bar = 0.05 mm.
Scale sensilla are characterized by a group of central cells originating from the dermis, creating a dermal papilla by displacing outward the stratum germinativum and keratin layers of the epidermis (Figure 2A). Central cells were not evident in the histological sections of protuberant scale sensilla in H. danieli, likely due to artifacts of the tissue sectioning process (Figure 2C). The keratinized epidermis consisting of the alpha and beta layers was notably thinner in the region just above the sensillum for all species except H. danieli. The shape of the corporal scale sensilla in H. danieli and H. pastazae is characterized by a uniform round shape. In contrast, the corporal scale sensilla of H. angulatus appears to have a noticeable invagination around the central region of the sensillum (Figure 2A). A second type of dermal corporal sensilla was identified in H. danieli. These scale sensilla are not observed as protuberant bumps in the skin of the individuals since they consist of a group of central cells that do not displace outward the stratum germinativum or keratin layers of the epidermis (Figure 2D).
Scanning electron microscopy
Scanning electron microscopy revealed that corporal scale sensilla on the anterior dorsal region of the three species of Helicops are externally dome-shaped with concentric rings around them. The number and conspicuousness of concentric rings may vary between species. For example, H. angulatus shows a clear external concentric ring with a smaller central ring, whereas H. danieli and H. pastazae show heterogeneous, less defined rings around the scale sensilla (Figure 3). Scale sensilla were principally found aligned above the keeled region of the scale for the dorsal position, whereas they appeared more scattered in the lateral position (Figures 3A, 4). We also observed a distribution pattern within the scale, where the sensilla for both the lateral and dorsal regions were concentrated in the posterior region of the scale (Figure 4).
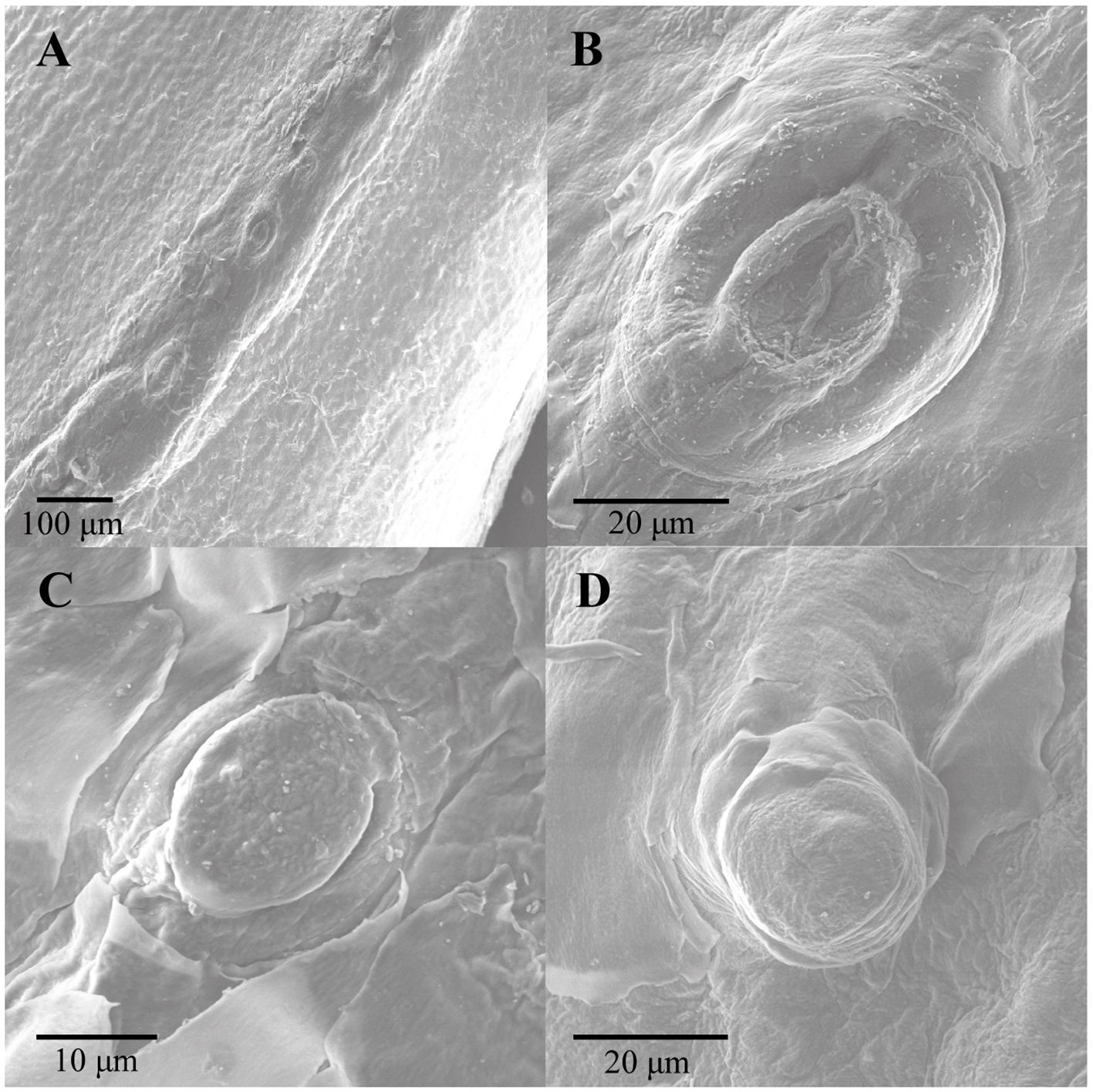
Figure 3 External morphology of corporal scale sensilla mechanoreceptors in the three species of Helicops studied. (A) row of mechanoreceptors distributed above the keel of an anterior dorsal scale in H. angulatus, (B) H. angulatus male, (C) H. danieli male, and (D) H. pastazae female.
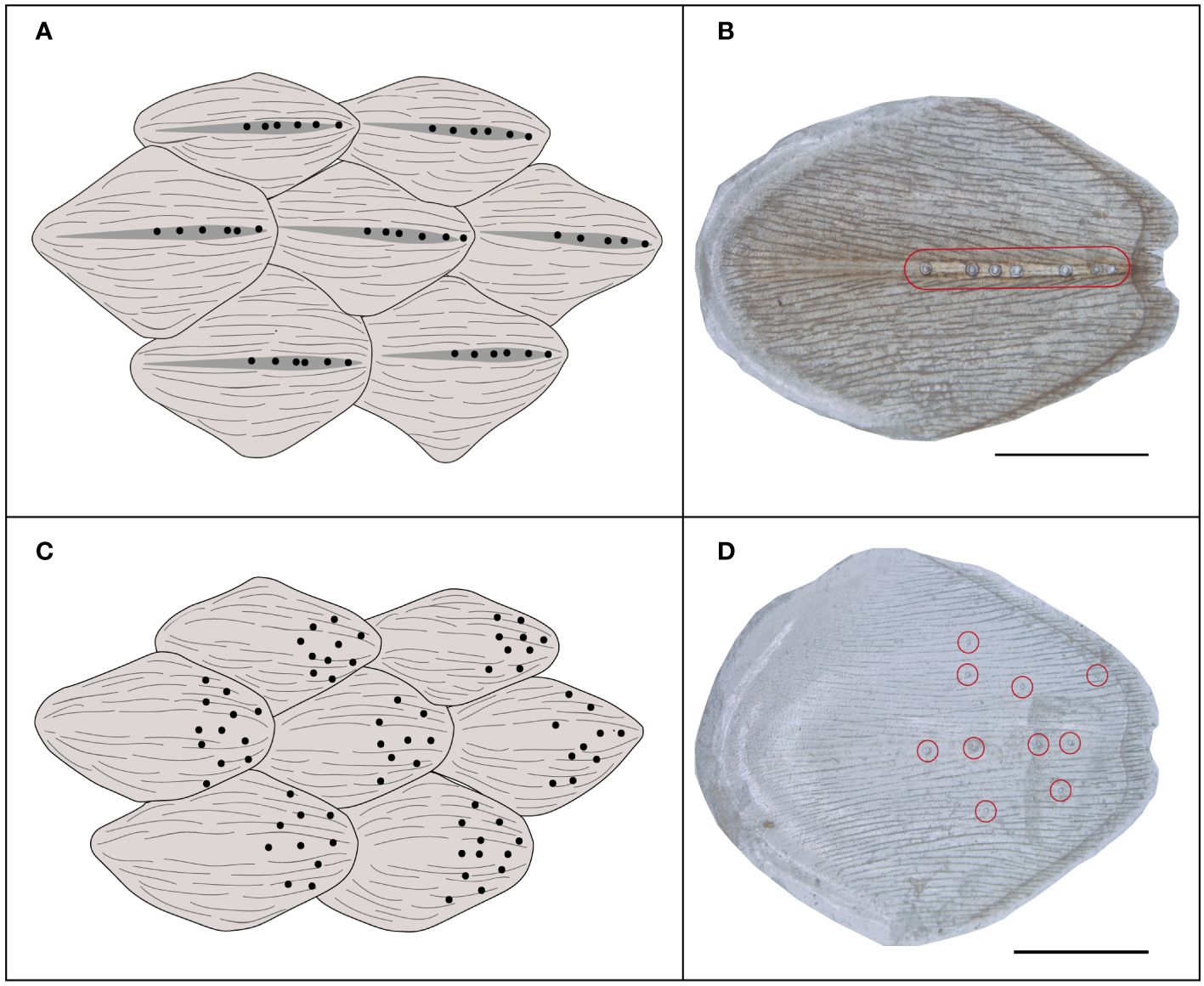
Figure 4 Distribution of corporal scale sensilla within the dorsal and lateral scales of Helicops. (A) scheme diagram showing that scale sensilla are restricted to the keel in dorsal scales, (B) light microscopy photographs of the beta-keratin layer mounted on a glass slide of a dorsal scale (C) scheme diagram showing dispersed sensilla distribution on the lateral scales, and (D) light microscopy photographs of the beta-keratin layer mounted on a glass slide of a lateral scale. Red circles show the presence of scale sensilla. Scale bar = 1 mm. In all cases anterior region of the scale is to the left.
Differences in corporal sensilla across the body
We found significant differences in the number of sensilla across the corporal regions and the position of the corporal scale, as well as the interaction between these two factors (Figure 5). Across the lateral positioned scales, the anterior corporal region had significantly higher numbers of mechanoreceptors sensilla compared to the mid-body, posterior, and caudal regions (Figure 6; Table 1). Scales located at the dorsal position have significantly more sensilla in the anterior and caudal regions compared to the midbody and posterior regions (Figure 6; Table 1). In general, the number of sensilla between dorsal and laterally positioned scales do not show evident differences. However, the three species evaluated have significantly more scale sensilla in the lateral positioned scales of the anterior and midbody corporal regions compared to the dorsal scales within these same regions (Table 1).
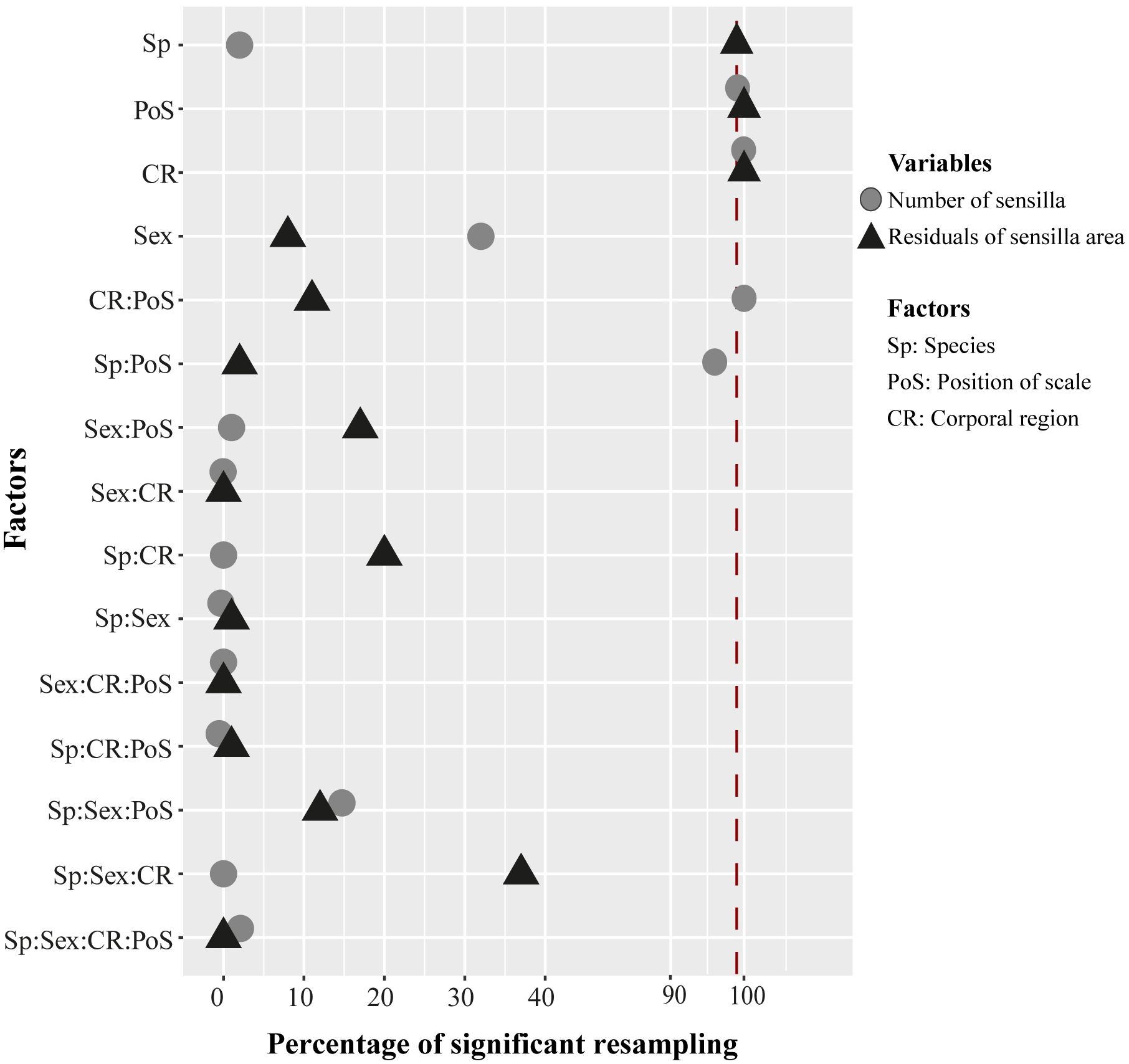
Figure 5 Percentage results of 100 resamplings running a mixed-effect permutation ANOVA test to evaluate significant differences in the number of sensilla (circles) and area of sensillum (triangles) across species, sex, position of scale, and corporal regions. Interactions between factors are represented by “:”. The vertical red line represents 99% or 0.01 of significance across the bootstrap tests.
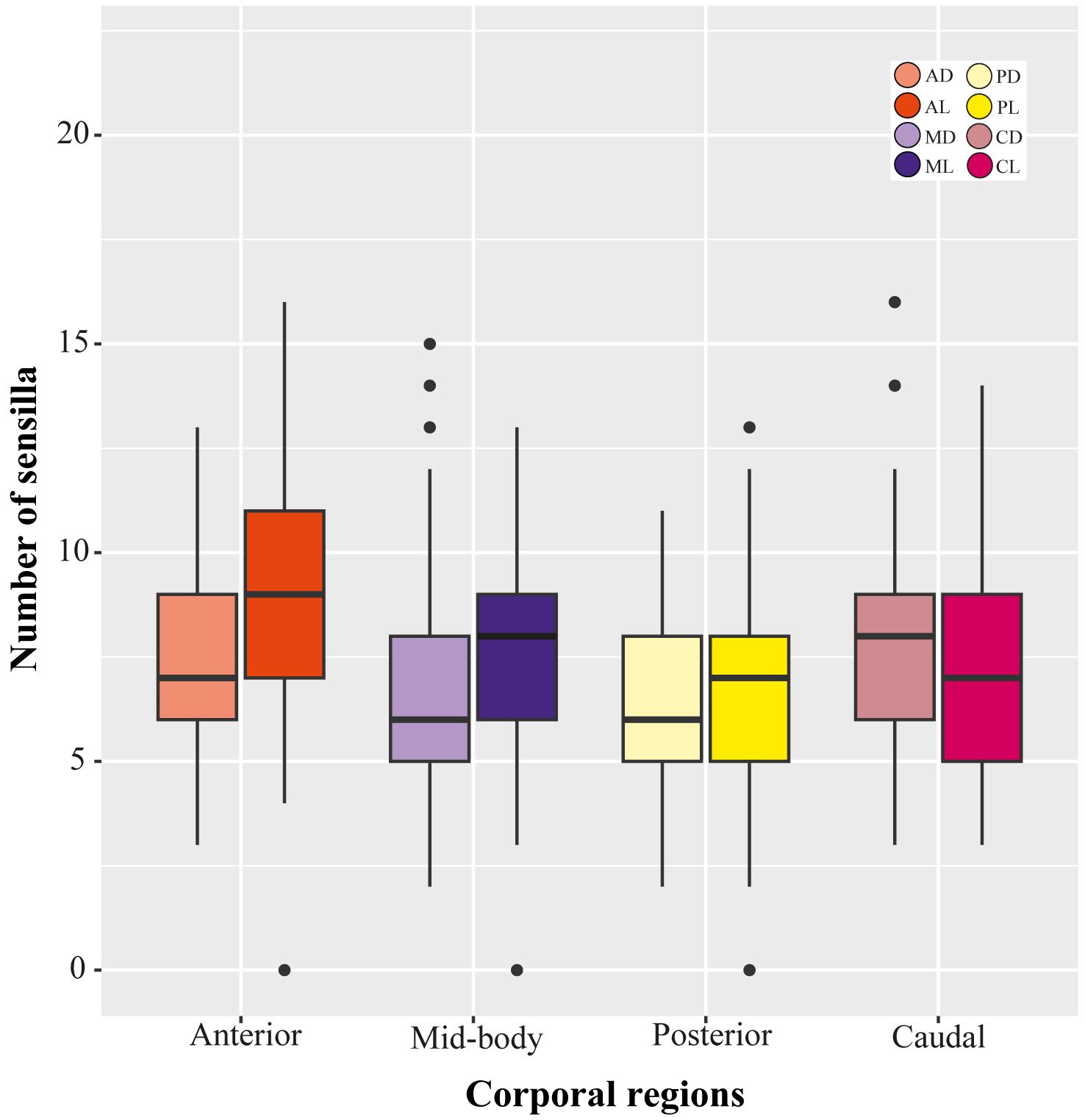
Figure 6 Variation in the number of sensilla across corporal regions and position of scale in Helicops. AD, anterior dorsal; AL, anterior lateral; MD, mid-body dorsal; ML, mid-body lateral; PD, posterior dorsal; PL, posterior lateral; CD, caudal dorsal; CL, caudal lateral.
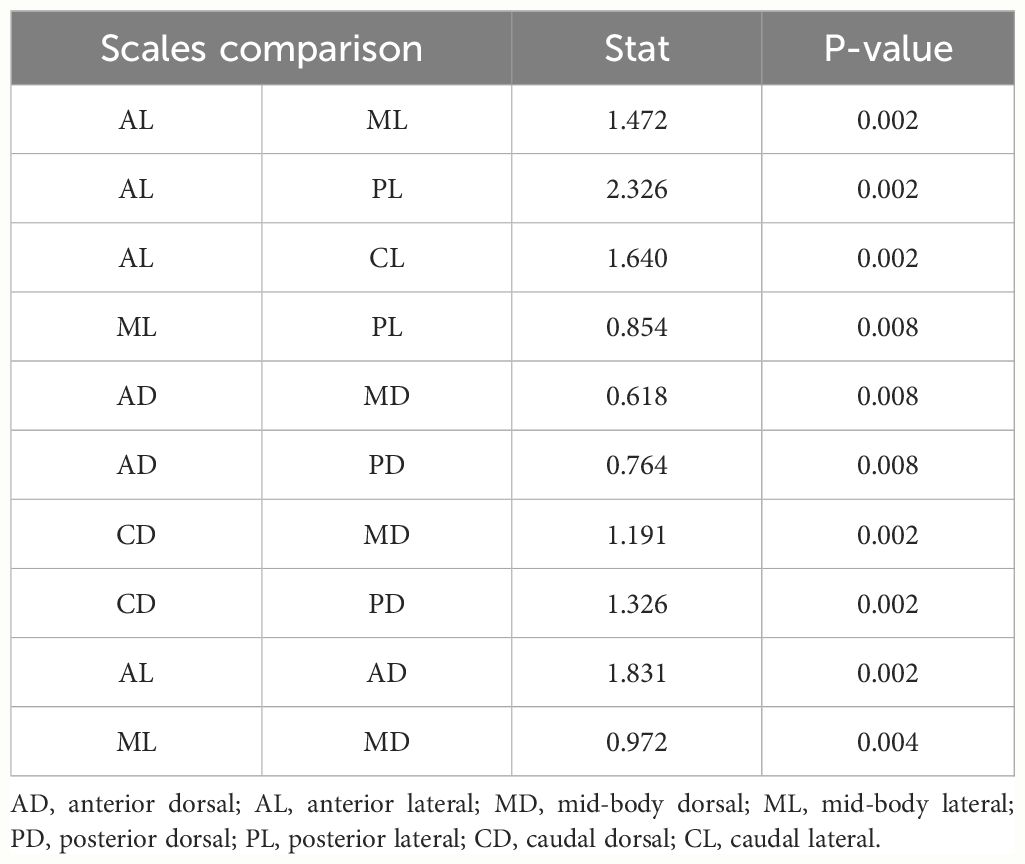
Table 1 Significant results (P-value <0.01) obtained from post-hoc pairwise comparisons of the number of sensilla between corporal regions and position of scale in Helicops.
The average area of sensilla was significantly different among the species, across the corporal regions and position of scale (Figure 5). Differences across corporal regions did not show a general trend since the average sensillum area varied in degree depending on the species (Figure 7; Table 2). Post hoc comparisons in H. angulatus show that this species has larger scale sensilla area in the posterior and caudal dorsal scales compared to anterior and midbody dorsal scales. It further shows higher scale sensilla area values in the caudal lateral scales than the anterior and mid-body scales. H. danieli shows the lowest values of scale sensilla area in the mid-body region for both dorsal and lateral positioned scales compared to the anterior, posterior, and caudal regions (Table 2). H. pastazae seems to have uniform scale sensilla areas across the body, except for the caudal lateral scales that have significantly larger scale sensilla than the midbody lateral scales. The scale sensilla area differs between dorsal and lateral positioned scales, being larger on the dorsal scales in all the corporal regions of H. danieli, in two corporal regions in H. angulatus (mid-body and posterior regions), and in one in H. pastazae (mid-body region).
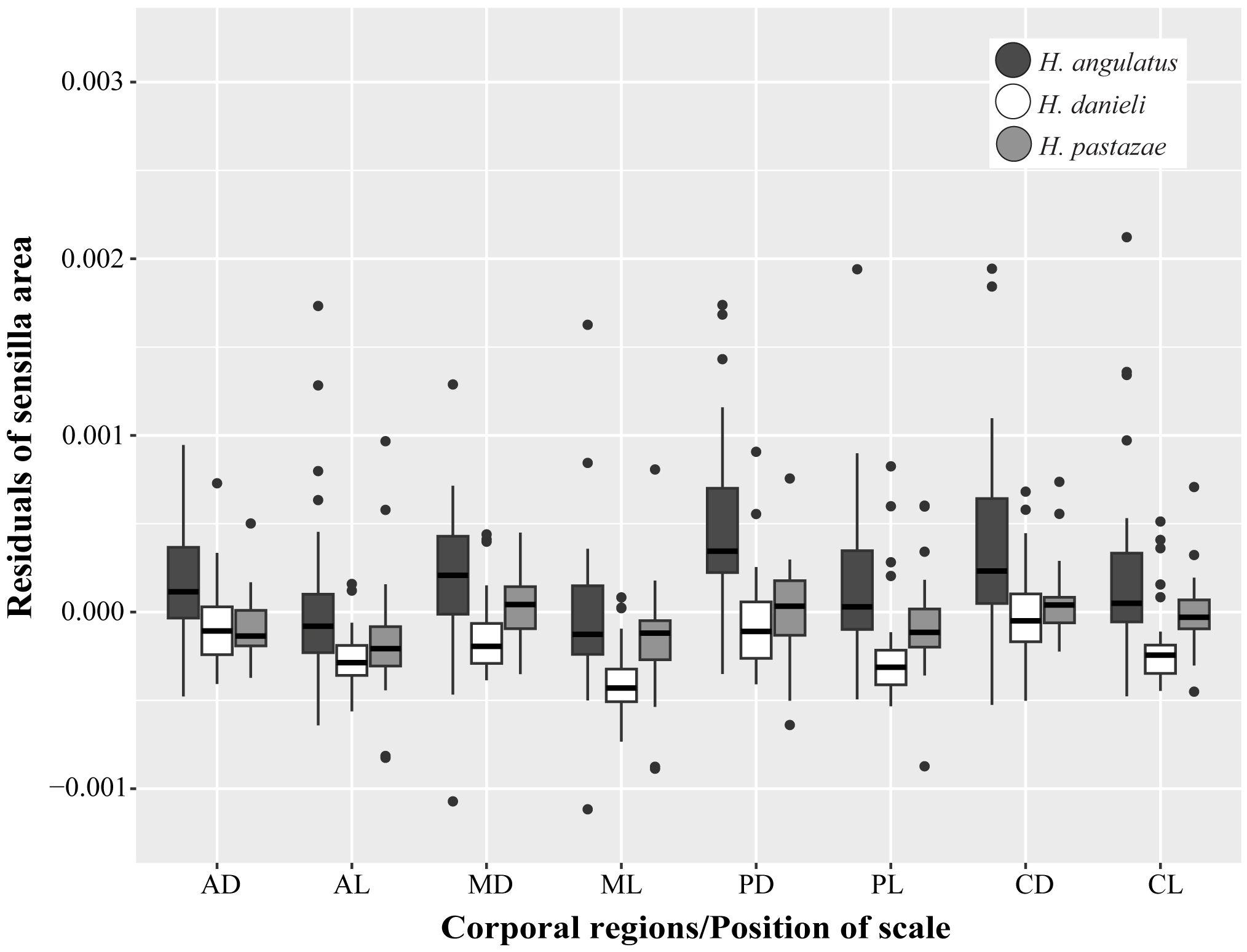
Figure 7 Interspecific, corporal regions and position of scales differences in the sensillum average area (corrected by size using residuals). AD, anterior dorsal; AL, anterior lateral; MD, mid-body dorsal; ML, mid-body lateral; PD, posterior dorsal; PL, posterior lateral; CD, caudal dorsal; CL, caudal lateral.
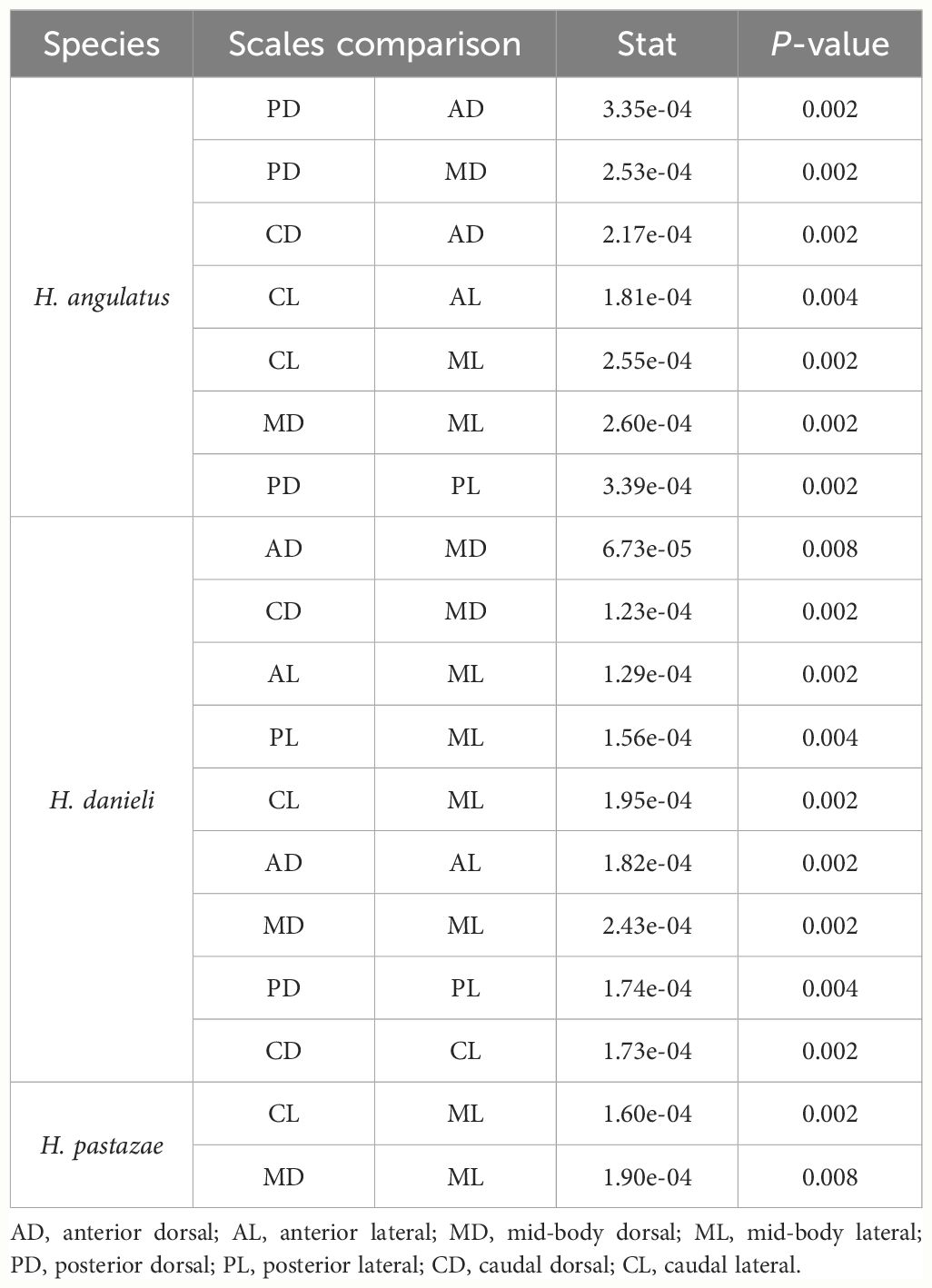
Table 2 Significant results (P-value <0.01) obtained from post-hoc pairwise comparisons of the residuals of sensilla area between corporal regions and position of scale of each species of Helicops.
Interspecific and sexual variation of corporal scale sensilla
We did not find significant differences in the number of sensilla between species (Figure 5). However, the sensillum average area varied between species, with H. angulatus having larger scale sensilla area than H. danieli for all the scales analyzed (Figure 7; Table 3). Similarly, H. angulatus showed larger scale sensilla than H. pastazae in the dorsally positioned scales of the anterior, posterior, and caudal corporal regions. Finally, H. pastazae showed significantly larger scale sensilla than H. danieli in the mid-body dorsal and caudal lateral scales. Our results did not show significant differences in the number or average area of scale sensilla between males and females of Helicops (Figure 5).
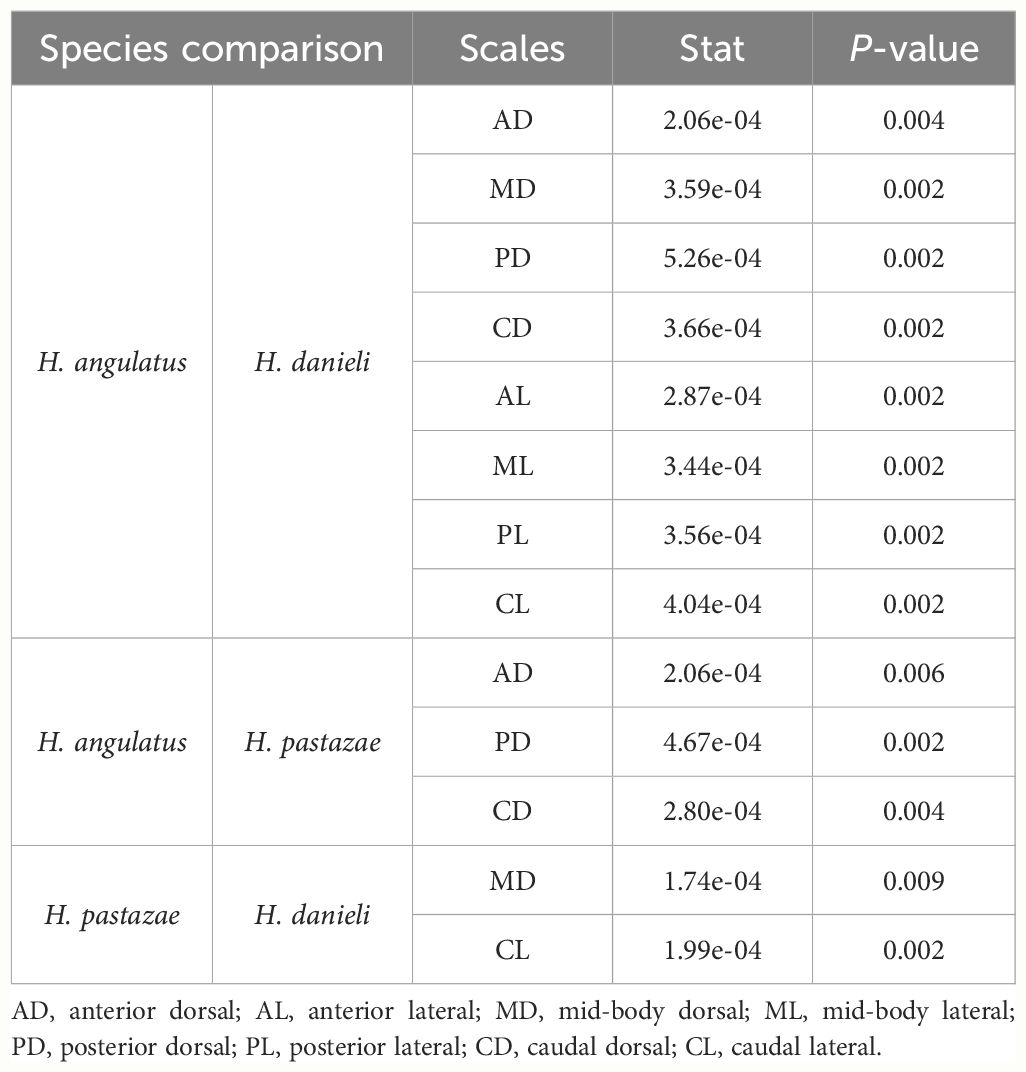
Table 3 Significant results (P-value <0.01) obtained from post-hoc pairwise comparisons of the residuals of sensilla area to evaluate interspecific differences between corporal regions and position of scale in Helicops.
Discussion
This study describes and quantifies the corporal dome-shaped mechanoreceptors sensilla in Neotropical freshwater snakes for the first time. Consistent with our predictions, we found that corporal sensilla are not uniformly distributed along the body since individuals from all species presented a higher number of sensilla in the anterior lateral region compared to the rest of the body. We expected to find larger and more scale sensilla in the river specialist snake H. pastazae compared to the generalist snakes H. angulatus and H. danieli. However, contrary to our prediction, we found that H. angulatus presented a significantly larger scale sensilla area in all scales than H. danieli, as well as when compared to the dorsally positioned scales in H. pastazae. Finally, we predicted that males would have a higher number and area in corporal sensilla, but our results did not show significant differences between sexes.
Histological results of the corporal scale sensilla of H. angulatus, H. pastazae, and H. danieli exhibit a similar morphology to the cephalic scale sensilla, genial knobs, and anal knobs previously found in aquatic snakes (Crowe-Riddell et al., 2019, Crowe-Riddell et al., 2021; García-Cobos et al., 2021). We suggest that corporal sensilla have a mechanoreceptor function based on the presence of a dermal papilla consisting of central cells and a reduced keratin layer above the organ, as has been previously described for other reptilian mechanoreceptors (Jackson et al., 1996; Crowe-Riddell et al., 2019). However, the central cells within the dermal papilla in the corporal scale sensilla are smaller and less conspicuous than in cephalic sensilla. While this might suggest that corporal sensilla contain a smaller bundle of central cells, it could also be due to the inability to capture the complete structure in the histological sections. Immunohistochemistry studies searching for neuronal markers in the cephalic skin of the sea snakes Aipysurus laevis and Hydrophis stokesii, found the presence of discoid receptors above the dermal papilla, suggesting that central cells have a functional role in traducing mechanical stimuli (Crowe-Riddell et al., 2019). Furthermore, a thinner layer of keratin above the sensillum may indicate a greater sensitivity to detect mechanical stimuli in this region (García-Cobos et al., 2021).
We also found a second type of dermal corporal sensilla in the skin of H. danieli characterized by a group of central cells that do not displace outward the keratinocytes and stratum germinativum, thus not forming an external bump. Although we only found these mechanoreceptors in H. danieli, further studies including more histological sections could reveal these structures in the skin of H. pastazae and H. angulatus. Similar organs were initially described in the tails of sea snakes and are considered simplified mechanoreceptors with less specialization compared to the protuberant scale sensilla (Crowe-Riddell et al., 2019). Unfortunately, histological sections stained with hematoxylin and eosin do not reveal nerves, myelin, and unmyelinated axons located at the base of the dermal papilla, as suggested in previous reptile studies (Avolio et al., 2006; Leitch and Catania, 2012; Crowe-Riddell et al., 2019). Thus, we suggest that future morphological studies on scale sensilla in Helicops should include immunohistochemical techniques and specialized staining (e.g. Bodian silver staining) to account for direct evidence of neuronal components.
SEM images revealed dome-shaped corporal sensilla for all three species of Helicops. This shape is likely advantageous in aquatic environments as it enables individuals to capture stimuli from different directions (Crowe-Riddell et al., 2016). However, dome-shaped mechanoreceptors are not unique to aquatic snakes, as they have also been reported in other reptiles including terrestrial snakes (Jackson, 1977; Jackson and Sharawy, 1980), fossorial snakes (Young and Wallach, 1998), lizards (Landmann, 1975; von Düring and Miller, 1979; Sherbrooke and Nagle, 1996) and crocodiles (Jackson et al., 1996; Leitch and Catania, 2012). The corporal sensilla in H. angulatus present an invagination surrounding the base of the dome (Figure 3). This crater is hypothesized to have a protective function, acting as a filter that modulates the effects of mechanical stimuli on the surface of the scale sensilla (Jackson and Doetsch, 1977a). Hair-like corporal mechanoreceptors have previously been described in the file snakes Acrochordus (Van Der Kooij and Povel, 1996) and geckos (Riedel et al., 2019). These mechanoreceptors have a more complex morphology since each sensillum can have single or multiple bristles that can split into setules (Riedel and Schwarzkopf, 2022). Similar to the dome-shaped sensilla, the small bristles present in these corporal mechanoreceptors are suggested to cause a neuronal response through the dermal papilla upon movement (Van Der Kooij and Povel, 1996; Riedel and Schwarzkopf, 2022).
Within an individual scale, we qualitatively observed that corporal scale sensilla are mainly located in the posterior and central regions for both dorsal and lateral scales. The arrangement of the corporal scales in Helicops is imbricated, meaning that they have an overlapping pattern in which the posterior region of a scale covers the anterior part of the scale immediately behind it. Therefore, it is likely that having mechanoreceptors in the anterior region of the scale would not be optimal for sensing external movement signals, since they would not be in direct contact with the environment.
The three species of Helicops evaluated in this study present a strong pronounced longitudinal ridge, also known as a keel, on the dorsal scales compared to a weaker and less protuberant keel in the lateral region (Costa et al., 2016). Although the function of the keel present in some snakes is unclear, previous studies have suggested that this thickened longitudinal ridge may protect the scales from wear, considering the constant friction snakes experience during movement (Liu et al., 2023). An alternative hypothesis suggests that the keeled surface structure in marine snakes might also play a hydrodynamic role, potentially reducing drag while swimming (Lillywhite, 2014). Interestingly, we observed that corporal sensilla were mainly distributed above the keel of the dorsal scales, compared to the widely dispersed distribution observed on the lateral scales. We suggest that the distribution of scale sensilla on the strongly keeled dorsal scales in Helicops may be attributed to the keel’s prominent exposure to external stimuli in an aquatic environment. However, future studies quantifying our qualitative observation of the uneven distribution of scale sensilla within the scales are needed for a more robust conclusion regarding this observed pattern.
All the species examined showed an uneven distribution in the number of sensilla across the different regions of the body, with a higher concentration at the anterior lateral region. The presence of a higher number of mechanoreceptors on a specific scale could be suggesting an increase in sensitivity of that region, since physiological studies in other species such as the Texas Rat Snake (Elaphe obsoleta) reported that stimulation of several mechanoreceptors produces a greater discharge than stimulation of a single organ (Jackson and Doetsch, 1977a). Further, from an electrophysiological point of view, scales sensilla in terrestrial snakes have shown similar physical displacement thresholds as Meissner corpuscles in mammalian skin (Jackson and Doetsch, 1977a, b; Proske, 1969); and due to this mechanical sensitivity, scale sensilla are used by snakes to explore and navigate through the environment, discriminate different types of preys, and get involved in courtship behaviors (Crowe-Riddell et al., 2019). Additionally, for marine snakes, scale sensilla have been suggested to be involved in hydrodynamic reception of water motion caused by low-amplitude water motions (Westhoff et al., 2005; Crowe-Riddell et al., 2019). Therefore, the increased sensitivity in the anterior lateral region of these Helicops species could be related to the need to explore in an aquatic environment and improve their perception when capturing and manipulating prey.
Although there are no studies describing the foraging behavior of Helicops, studies on other freshwater snakes such as Thamnophis (Alfaro, 2002, 2003), Nerodia (Alfaro, 2003; Herrel et al., 2008), and Erpeton tentaculatum (Smith et al., 2002), posit that these snakes may use a frontal or lateral strike to capture their prey. In the frontal strike, the snake visually orients its prey before projecting the head forward to capture it, whereas a lateral strike is initiated by tactile cues, and is achieved by moving the head sideways, usually with the mouth open (Drummond, 1983; Bilcke et al., 2006). Moreover, the frontal striking feeding strategy has been suggested to be common in piscivorous specialist-feeding snakes that live in bodies of water with low densities of prey, whereas lateral strikes are hypothesized to be common in dietary generalists living in environments with high densities of prey (Bilcke et al., 2006).
According to these previously proposed hypotheses, we would have expected to find evidence of lateral strike foraging behavior in the generalist snakes H. angulatus and H. danieli, and frontal strike in the piscivorous specialist H. pastazae. However, our findings regarding an overall higher number of scale sensilla in the anterior lateral region may indicate that these three species of Helicops may forage by performing lateral strikes, with tactile cues around the neck playing an important role in detecting and manipulating prey. Further studies evaluating foraging behavior and prey abundance in the sites where Helicops inhabit will help resolve the relationship between the presence and number of mechanoreceptors in the neck and the lateral strike behavior in this species. Other studies in reptiles have also reported uneven distribution of mechanoreceptor organs associated with foraging behaviors. For instance, crocodiles (Leitch and Catania, 2012), geckos (Riedel and Schwarzkopf, 2022) terrestrial snakes (Jackson and Sharawy, 1980), and freshwater snakes (García-Cobos et al., 2021) have more mechanoreceptors around their mouths, which has been associated to the need for greater sensitivity to detect and capture prey.
The size of sensory organs has also been suggested to be a useful indicator of the sensory capability of a particular sensorial mode (Ruiz-Monachesi et al., 2020). For instance, haplorhine primates present extreme ocular hypertrophy as an adaptation to improve visual acuity and sensitivity at night (Kay and Kirk, 2000). Similarly, the size of the olfactory bulbs in sharks is larger in deep-sea species that live in habitats with low light, where chemoreception plays an important role (Yopak et al., 2015). In this study, differences in average sensillum area, an approximation of sensillum size, were observed across corporal regions of some species and between species. The presence of larger scale sensilla was particularly evident in the posterior corporal regions of H. angulatus. We suggest that higher sensitivity in the caudal region may be important for detecting predators arriving from behind. Lizards that present caudal autotomy detach their tail as an anti-predation behavior induced by the mechanical stimuli detected by sensilla located at this region (Bradley et al., 2021). Additionally, larger scale sensilla in the caudal region may facilitate body placement and monitoring of the environment. For example, geckos of the genus Nephrurus have sensilla on their tails that have been associated with a behavior of repeatedly touching the substrate with their tail to sense the environment (Riedel and Schwarzkopf, 2022).
Contrary to our predictions, interspecific differences in sensillum size reveal that the generalist snake H. angulatus presents the largest corporal scale sensilla. Previous studies on the cephalic sensilla of Helicops showed that the river specialist snake H. pastazae have larger scale sensilla than the generalist snake H. angulatus, likely due to differences in ecological preferences such as foraging behavior, diet, and habitat (García-Cobos et al., 2021). The size difference in corporal sensilla between species may be related to variation in sensilla shape, considering that H. pastazae and H. danieli appear to have protuberant organs compared to the flattened sensilla characterized by an interior and external concentric ring in H. angulatus. Unfortunately, the methodology we used to quantify the scale sensilla size only considers the transversal area of the mechanoreceptors in a 2D image taken from above. Protuberance in height of scale sensilla was a variable we did not consider in this study, and would require other techniques that involve a 3D surface analysis to address with more precision (for instance, Atomic Force Microscopy). Furthermore, we cannot disregard that our results on sensilla size may involve confounding variables such as phylogenetic signals, indicating that more closely related species share similar morphology due to common ancestry, as has been observed in other studies (Baeckens et al., 2017; Riedel et al., 2019). However, studies elucidating the evolutionary relationships of the Hydropsini tribe, currently lack information on two of the focal species of this study, H. danieli and H. pastazae, in their phylogenetic trees.
Although sexual dimorphism in sensory systems is common among snakes, our results did not reveal significant differences in the number and area of corporal sensilla between males and females of the three species. Our initial predictions followed the study of García-Cobos et al. (2021) which observed that males of H. angulatus and H. pastazae, presented higher values in the number and size of cephalic mechanoreceptors sensilla compared to females. This sexual dimorphism has been suggested to be related to differences in foraging behavior or mate searching in these species. Variation in mechanoreception has also been observed in sea snakes where only males have genial and anal knobs that help them orient toward females and ensure cloacal alignment during mating (Crowe-Riddell et al., 2021). In the North American Pitviper (Agkistrodon contortrix), males exhibit longer and deeper bifurcated tongues than females, enhancing their chemosensory ability to search for mates (Smith et al., 2008). Furthermore, females have smaller eye sizes than males in the Aipysurine snakes from the genera Emydocephalus and Aipysurus, indicating a possible sexual selection pressure on mate-finding in males, or sex differences in foraging habitats (Shine et al., 2023).
Overall, this study contributes to a better understanding of the mechanosensory world of Neotropical freshwater snakes. We suggest that the uneven distribution in number and size of scale sensilla may have an important role in foraging modes, highlighting the anterior lateral region as an important sensory area for prey detection and manipulation. However, other factors not considered in this study, such as phylogenetic signals, may also influence and explain the observed differences between species and corporal regions. We also highlight that our results show differences in the distribution and size patterns with cephalic sensilla which may be partly explained as a result of the stronger selective pressure in sensory modes on the head compared to corporal regions. We further emphasize the importance of conducting physiological and behavioral studies that may allow a deeper knowledge of the role that corporal scale sensilla plays in these aquatic organisms.
Data availability statement
The original contributions presented in the study are included in the article/Supplementary Material. Further inquiries can be directed to the corresponding author.
Ethics statement
Ethical approval was not required for the study involving animals in accordance with the local legislation and institutional requirements because we used specimens previously deposited in biological collections. This work did not use live animals.
Author contributions
VV: Conceptualization, Data curation, Formal analysis, Investigation, Methodology, Validation, Visualization, Writing – original draft, Writing – review & editing. CB: Conceptualization, Data curation, Formal analysis, Methodology, Validation, Visualization, Writing – review & editing. SG: Conceptualization, Resources, Validation, Writing – review & editing. JM: Conceptualization, Resources, Validation, Writing – review & editing. AS: Conceptualization, Methodology, Validation, Visualization, Writing – original draft, Writing – review & editing. DG: Conceptualization, Formal analysis, Investigation, Methodology, Supervision, Validation, Visualization, Writing – original draft, Writing – review & editing.
Funding
The author(s) declare financial support was received for the research, authorship, and/or publication of this article. JM wants to thanks the Faculty of Sciences-Universidad de los Andes for funding through the research Program INV-2023-176-2970, and also its fund to support publications.
Acknowledgments
We are very grateful to Diego A. Gómez-Sánchez for noticing the possibility of finding corporal sensilla in Helicops and for deep conversations on the natural history of these species. We thank the Instituto de Investigación de Recursos Biológicos Alexander von Humboldt for the internship opportunity given to the first author and for providing us with access to the Reptile Collection (IAvH-R) specimens and the required equipment for analyzing and photographing the scales and histological sections. We also thank the Center of Microscopy at the Universidad de Los Andes for their assistance with taking SEM images, and the Laboratory of Veterinary Pathology and Biology at the Universidad Nacional de Colombia for performing histological sections. Many thanks to Amanda Markee for her revision and feedback on the English text, to Julián Clavijo-Bustos for his collaboration in taking some photos for this article, and to Julián Andrés Rojas for his assistance in structuring the initial idea of this work.
Conflict of interest
The authors declare that the research was conducted in the absence of any commercial or financial relationships that could be construed as a potential conflict of interest.
Publisher’s note
All claims expressed in this article are solely those of the authors and do not necessarily represent those of their affiliated organizations, or those of the publisher, the editors and the reviewers. Any product that may be evaluated in this article, or claim that may be made by its manufacturer, is not guaranteed or endorsed by the publisher.
Supplementary material
The Supplementary Material for this article can be found online at: https://www.frontiersin.org/articles/10.3389/famrs.2024.1412004/full#supplementary-material
References
Acosta-Ortiz J. M., Vos V. A. (2023). Anurophagy by the Brown-banded Watersnake, Helicops angulatus (Serpentes: Colubridae): a review with new records. Reptiles Amphibians. 30, e18435–e18435. doi: 10.17161/randa.v30i1.18435
Alfaro M. E. (2002). Forward attack modes of aquatic feeding garter snakes. Funct. Ecology. 16, 204–215. doi: 10.1046/j.1365-2435.2002.00620.x
Alfaro M. E. (2003). Sweeping and striking: a kinematic study of the trunk during prey capture in three thamnophiine snakes. J. Exp. Biol. 206, 2381–2392. doi: 10.1242/jeb.00424
Almendáriz A., Barriga R., Rivadeneira D. (2017). Feeding behavior of Helicops pastazae Shreve 1934 (Serpentes, Colubridae, Dipsadinae) in the Ecuadorian Amazon. Herpetology Notes 10, 449–451.
Avolio C., Shine R., Pile A. J. (2006). The adaptive significance of sexually dimorphic scale rugosity in sea snakes. Am. Naturalist. 167, 728–738. doi: 10.1086/503386
Baeckens S., Van Damme R., Cooper W. E. Jr. (2017). How phylogeny and foraging ecology drive the level of chemosensory exploration in lizards and snakes. J. Evolutionary Biol. 30, 627–640. doi: 10.1111/jeb.13032
Bilcke J., Herrel A., Van Damme R. (2006). Correlated evolution of aquatic prey-capture strategies in European and American natricine snakes. Biol. J. Linn. Society. 88, 73–83. doi: 10.1111/bij.2006.88.issue-1
Bradley S. S., Howe E., Bent L. R., Vickaryous M. K. (2021). Cutaneous tactile sensitivity before and after tail loss and regeneration in the leopard gecko (Eublepharis macularius). J. Exp. Biol. 224, jeb234054. doi: 10.1242/jeb.234054
Carvajal-Cogollo J. E., Castaño-Mora O. V., Cárdenas-Arévalo G., Urbina-Cardona J. N. (2007). Reptiles of associated areas to wetlands from the plain of the department of Córdoba, Colombia. Caldasia 29, 427–438.
Catania K. C., Leitch D. B., Gauthier D. (2010). Function of the appendages in tentacled snakes (Erpeton tentaculatus). J. Exp. Biol. 213, 359–367. doi: 10.1242/jeb.039685
Citeli N., Klaczko J., De-Lima A. K. S., De-Carvalho M., Nunes P. M. S., Passos P., et al. (2022). Taxonomy, allometry, sexual dimorphism, and conservation of the trans-Andean watersnake Helicops danieli Amaral 1937 (Serpentes: dipsadidae: hydropsini). Can. J. Zoology. 100, 184–196. doi: 10.1139/cjz-2021-0073
Costa H. C., Santana D. J., Leal F., Koroiva R., García P. C. (2016). A new species of Helicops (Serpentes: Dipsadidae: Hydropsini) from southeastern Brazil. Herpetologica 72, 157–166. doi: 10.1655/HERPETOLOGICA-D-15-00059
Crowe-Riddell J. M., Jolly C. J., Goiran C., Sanders K. L. (2021). The sex life aquatic: sexually dimorphic scale mechanoreceptors and tactile courtship in a sea snake Emydocephalus annulatus (Elapidae: Hydrophiinae). Biol. J. Linn. Society. 134, 154–164. doi: 10.1163/156853983X00543
Crowe-Riddell J. M., Snelling E. P., Watson A. P., Suh A. K., Partridge J. C., Sanders K. L. (2016). The evolution of scale sensilla in the transition from land to sea in elapid snakes. Open Biol. 6, 160054. doi: 10.1098/rsob.160054
Crowe-Riddell J. M., Williams R., Chapuis L., Sanders K. L. (2019). Ultrastructural evidence of a mechanosensory function of scale organs (sensilla) in sea snakes (Hydrophiinae). R. Soc. Open science. 6, 182022. doi: 10.1098/rsos.182022
dos Santos Costa M. C., Abe P. S., Albarelli L. P. P., da Costa Prudente A. L., Pinheiro L. C., da Cunha Bitar Y. O., et al. (2022). Sexual dimorphism, reproduction and feeding biology of Helicops angulatus (Linnaeus 1758) (Colubridae: Hydropsini) in eastern Amazon, Brazil. Boletín la Sociedad Zoológica del Uruguay. 31, e31–e31. doi: 10.26462/31.1.1
Drummond H. (1983). Aquatic foraging in garter snakes: a comparison of specialists and generalists. Behaviour 86, 1–30. doi: 10.1163/156853983X00543
Duvall D., Arnold S. J., Schuett G. W. (1992). “Pitviper mating systems: ecological potential, sexual selection, and microevolution,” in Biology of the pitvipers. Eds. Campbell J. A., Brodie E. D. (Selva Publishing, Tyler, TX), 321–336.
Ford N. B., Ford D. F. (2002). Notes on the ecology of the South American water snake Helicops angulatus (Squamata: Colubridae) in Nariva Swamp, Trinidad. Caribbean J. Science. 38, 129–131.
Frossard J., Renaud O. (2021). Permutation tests for regression, ANOVA, and comparison of signals: the permuco package. J. Stat. Software 99, 1–32. doi: 10.18637/jss.v099.i15
García-Cobos D., Crawford A. J., Ramírez-Pinilla M. P. (2020). Reproductive phenology in a Neotropical aquatic snake shows marked seasonality influenced by rainfall patterns. J. Natural History. 54, 1845–1862. doi: 10.1080/00222933.2020.1829724
García-Cobos D., Gómez-Sánchez D. A., Crowe-Riddell J. M., Sanders K. L., Molina J. (2021). Ecological and sexual roles of scale mechanoreceptors in two species of Neotropical freshwater snake (Dipsadinae: Helicops). Biol. J. Linn. Society. 134, 958–974. doi: 10.1093/biolinnean/blab129
García-Cobos D., Vásquez-Restrepo J. D. (2022). Notes on the distribution, morphological variation, and mechanoreceptors of Tretanorhinus nigroluteus (Serpentes: Dipsadidae) in Colombia. Biota Colombiana. 23, 1–11. doi: 10.21068/2539200X.943
Herrel A., Vincent S. E., Alfaro M. E., Van Wassenbergh S., Vanhooydonck B., Irschick D. J. (2008). Morphological convergence as a consequence of extreme functional demands: examples from the feeding system of natricine snakes. J. Evolutionary Biol. 21, 1438–1448. doi: 10.1111/j.1420-9101.2008.01552.x
Jackson M. K. (1977). Histology and distribution of cutaneous touch corpuscles in some Leptotyphlopid and Colubrid Snakes (Reptilia, Serpentes). J. Herpetology. 11, 7–15. doi: 10.2307/1563285
Jackson K., Butler D. G., Youson J. H. (1996). Morphology and ultrastructure of possible integumentary sense organs in the estuarine crocodile (Crocodylus porosus). J. Morphology. 229, 315–324. doi: 10.1002/(ISSN)1097-4687
Jackson M. K., Doetsch G. S. (1977a). Functional properties of nerve fibers innervating cutaneous corpuscles within cephalic skin of the Texas rat snake. Exp. Neurology. 56, 63–77. doi: 10.1016/0014-4886(77)90139-X
Jackson M. K., Doetsch G. S. (1977b). Response properties of mechanosensitive nerve fibers innervating cephalic skin of the Texas rat snake. Exp. Neurology. 56, 78–90. doi: 10.1016/0014-4886(77)90140-6
Jackson M. K., Sharawy M. (1980). Scanning electron microscopy and distribution of specialized mechanoreceptors in the Texas rat snake, Elaphe obsoleta lindheimeri (Baird and Girard). J. Morphology. 163, 59–67. doi: 10.1002/jmor.1051630108
Kay R. F., Kirk E. C. (2000). Osteological evidence for the evolution of activity pattern and visual acuity in primates. Am. J. Biol. Anthropology. 113, 235–262. doi: 10.1002/(ISSN)1096-8644
Kerr K. F. (2009). Comments on the analysis of unbalanced microarray data. Bioinformatics 25, 2035–2041. doi: 10.1093/bioinformatics/btp363
King M. B., Duvall D. (1990). Prairie rattlesnake seasonal migrations: episodes of movement, vernal foraging and sex differences. Anim. Behaviour. 39, 924–935. doi: 10.1016/S0003-3472(05)80957-1
Kishida T., Go Y., Tatsumoto S., Tatsumi K., Kuraku S., Toda M. (2019). Loss of olfaction in sea snakes provides new perspectives on the aquatic adaptation of amniotes. Proc. R. Soc. B. 286, 20191828. doi: 10.1098/rspb.2019.1828
Landmann L. (1975). The sense organs in the skin of the head of Squamata (Reptilia). Israel J. Ecol. Evolution. 24, 99–135. doi: 10.1080/00212210.1975.10688416
Leitch D. B., Catania K. C. (2012). Structure, innervation and response properties of integumentary sensory organs in crocodilians. J. Exp. Biol. 215, 4217–4230. doi: 10.1242/jeb.076836
Lenth R. V. (2021). emmeans: Estimated Marginal Means, aka Least-Squares Means. R package version 1.6.2. Available online at: https://CRAN.R-project.org/package=emmeans.
Lillywhite H. B. (2014). “Perceiving the snake’s world: structure and function of sense organs,” in How snakes work: structure, function and behavior of the world’s snakes. Ed. Lillywhite H. B. (Oxford University Press, Oxford), 163–180.
Liu C., Chen Y., Zheng Y., Bo J., Yang C., Xu S., et al. (2023). Wear resistance improvement of keeled structure and overlapped distribution of snake scales. J. Bionic Engineering. 20, 1121–1131. doi: 10.1007/s42235-022-00300-2
Proske U. (1969). An electrophysiological analysis of cutaneous mechanoreceptors in a snake. Comp. Biochem. Physiol. 29, 1039–1046. doi: 10.1016/0010-406X(69)91006-8
Riedel J., Schwarzkopf L. (2022). Variation in density, but not morphology, of cutaneous sensilla among body regions in nine species of Australian geckos. J. Morphology. 283, 637–652. doi: 10.1002/jmor.21462
Riedel J., Vucko M. J., Blomberg S. P., Robson S. K., Schwarzkopf L. (2019). Ecological associations among epidermal microstructure and scale characteristics of Australian geckos (Squamata: Carphodactylidae and Diplodactylidae). J. Anatomy. 234, 853–874. doi: 10.1111/joa.12969
Rocha R., López-Baucells A. (2014). Predation attempt of Hypsiboas boans (Anura: Hylidae) by Helicops angulatus (Squamata: Dipsadidae) with notes on defensive behavior. Alytes 30, 78–81.
Ruiz-Monachesi M. R., Cruz F. B., Valdecantos S., Labra A. (2020). Unravelling associations among chemosensory system components in Liolaemus lizards. J. Zoology. 312, 148–157. doi: 10.1111/jzo.12819
Schöneberg Y., Köhler G. (2022). Distribution and identification of the species in the genus Helicops Wagler 1830 (Serpentes, Colubridae, Xenodontinae). Biodiversity Data J. 10, e69234. doi: 10.3897/BDJ.10.e69234
Schraft H. A., Clark R. W. (2019). Sensory basis of navigation in snakes: the relative importance of eyes and pit organs. Anim. Behaviour. 147, 77–82. doi: 10.1016/j.anbehav.2018.11.004
Segall M., Cornette R., Rasmussen A. R., Raxworthy C. J. (2021). Inside the head of snakes: influence of size, phylogeny, and sensory ecology on endocranium morphology. Brain Structure Funct. 226, 2401–2415. doi: 10.1007/s00429-021-02340-6
Seiko T., Kishida T., Toyama M., Hariyama T., Okitsu T., Wada A., et al. (2020). Visual adaptation of opsin genes to the aquatic environment in sea snakes. BMC Evolutionary Biol. 20, 1–13. doi: 10.1186/s12862-020-01725-1
Sherbrooke W. C., Nagle R. B. (1996). Phrynosoma intraepidermal receptor: a dorsal intraepidermal mechanoreceptor in horned lizards (Phrynosoma; Phrynosomatidae; Reptilia). J. morphology. 228, 145–154. doi: 10.1002/(ISSN)1097-4687
Shine R., Shine T. G., Brown G. P., Udyawer V. (2023). Sexual dimorphism in aipysurine sea snakes (Elapidae, Hydrophiinae). R. Soc. Open Science. 10, 231261. doi: 10.1098/rsos.231261
Simões B. F., Gower D. J., Rasmussen A. R., Sarker M. A., Fry G. C., Casewell N. R., et al. (2020). Spectral diversification and trans-species allelic polymorphism during the land-to-sea transition in snakes. Curr. Biol. 30, 2608–2615. doi: 10.1016/j.cub.2020.04.061
Simões B. F., Sampaio F. L., Douglas R. H., Kodandaramaiah U., Casewell N. R., Harrison R. A., et al. (2016). Visual pigments, ocular filters and the evolution of snake vision. Mol. Biol. evolution. 33, 2483–2495. doi: 10.1093/molbev/msw148
Smith T. L., Povel G. D. E., Kardong K. V. (2002). Predatory strike of the tentacled snake (Erpeton tentaculatum). J. Zoology. 256, 233–242. doi: 10.1017/S0952836902000274
Smith C. F., Schwenk K., Earley R. L., Schuett G. W. (2008). Sexual size dimorphism of the tongue in a North American pitviper. J. Zoology. 274, 367–374. doi: 10.1111/j.1469-7998.2007.00396.x
Van Der Kooij J., Povel D. (1996). Scale sensillae of the file snake (Serpentes: Acrochordidae) and some other aquatic and burrowing snakes. Netherlands J. Zoology. 47, 443–456. doi: 10.1163/156854297X00111
von Düring M., Miller M. R. (1979). “Sensory nerve endings of the skin and deeper structures,” in Biology of the reptilia, Volume 9, Neurology A. Eds. Northcutt R. G., Ulinsky P. S. (Academic Press, New York), 407–441.
Westhoff G., Fry B. G., Bleckmann H. (2005). Sea snakes (Lapemis curtus) are sensitive to low-amplitude water motions. Zoology 108, 195–200. doi: 10.1016/j.zool.2005.07.001
Yopak K. E., Lisney T. J., Collin S. P. (2015). Not all sharks are “swimming noses”: variation in olfactory bulb size in cartilaginous fishes. Brain Structure Funct. 220, 1127–1143. doi: 10.1007/s00429-014-0705-0
Keywords: Helicops, cutaneous mechanoreceptive organs, histology, SEM, interspecific differences
Citation: Velasquez-Cañon V, Bravo-Vega C, Galeano SP, Molina J, Salazar-Guzmán AM and García-Cobos D (2024) The mechanosensory world in aquatic snakes: corporal scale sensilla in three species of Neotropical freshwater dipsadine. Front. Amphib. Reptile Sci. 2:1412004. doi: 10.3389/famrs.2024.1412004
Received: 03 April 2024; Accepted: 26 June 2024;
Published: 16 July 2024.
Edited by:
Jenna M. Crowe-Riddell, La Trobe University, AustraliaReviewed by:
Natalia Ananjeva, Zoological Institute (RAS), RussiaDuminda Dissanayake, University of Canberra, Australia
Copyright © 2024 Velasquez-Cañon, Bravo-Vega, Galeano, Molina, Salazar-Guzmán and García-Cobos. This is an open-access article distributed under the terms of the Creative Commons Attribution License (CC BY). The use, distribution or reproduction in other forums is permitted, provided the original author(s) and the copyright owner(s) are credited and that the original publication in this journal is cited, in accordance with accepted academic practice. No use, distribution or reproduction is permitted which does not comply with these terms.
*Correspondence: Daniela García-Cobos, daniela.garcia.helicops@gmail.com
†ORCID: Valeria Velasquez-Cañon, orcid.org/0009-0004-4391-3464
Carlos Bravo-Vega, orcid.org/0000-0002-5588-7396
Jorge Molina, orcid.org/0000-0003-3018-6726
Daniela García-Cobos, orcid.org/0000-0002-7085-1113