- 1Departamento de Geología, Universidad de Sonora, Hermosillo, Mexico
- 2Environment and Climate Change Canada, National Wildlife Research Centre, Ottawa, ON, Canada
- 3Centro de Ciencias Genómicas, Universidad Nacional Autónoma de México, Cuernavaca, Morelos, Mexico
- 4Department of Biology, University of Nevada Reno, Reno, NV, United States
- 5Faculltad de Ciencias, Universidad Nacional Autónoma de México, Ciudad Universitaria, Mexico City, Mexico
- 6Departamento de Ciencias del Agua y Medio Ambiente, Instituto Tecnológico de Sonora, Ciudad Obregón, Sonora, Mexico
- 7Departamento de Investigación en Física, Universidad de Sonora, Hermosillo, Mexico
- 8Colección Nacional de Ácaros, Instituto de Biología, Universidad Nacional Autónoma de México, Mexico City, Mexico
The rapid transformation and pollution of ecosystems have severely impacted biodiversity. Specifically, anthropogenic activities have imposed adverse effects on amphibians, with evidence suggesting that these activities alter parasite and pathogen interactions within their hosts. To investigate these interactions in areas affected by different anthropogenic activities, our study focused on analyzing a pathogen and a parasite known to interact within the amphibian skin (spongy epidermis layer) and both compromising amphibian health: Batrachochytrium dendrobatidis (Bd), a fungus responsible for chytridiomycosis, a disease associated with massive population declines in amphibians and the Hannemania sp. mite in Mexico. Four sampling areas along the Sonora River were selected, representing different human activities: mining, livestock, wastewater discharge, agriculture, and one in an urban zone. We analyzed 135 amphibians across 10 anuran species. Among these, the most abundant species (Lithobates yavapaiensis) exhibited the highest prevalence of both pathogen and parasite (90.1% and 27.3%, respectively) and was significantly associated with the intensity of Bd-infection. The prevalence of Hannemania mites varied significantly across sampling sites as did Bd prevalence and infection load, with the highest Bd load found at the wastewater discharge site. A significant association between the intensity of Bd-infection and both mite abundance and amphibian species was observed when the sampling site was considered. Additionally, sites with Bd-positive individuals and Hannemania parasitism coincide with refractory elements characterized by mechanical or corrosion resistance. The persistence of these elements in the environment, along with the small particle size (<850 nm) found in sediments, poses a potential risk of internalization, bioaccumulation (e.g., Fe, Co, and Ti), and their transfer through the food chain. It is thus essential to consider monitoring environmental and biotic factors that modulate the relationships between parasites, pathogens, and amphibians if we are to propose conservation strategies adapted to disturbed environments.
1 Introduction
Anthropogenic activities have had detrimental effects on amphibians (Da Rocha et al., 2020; Yang et al., 2022), and there is compelling evidence that anthropogenic factors drive disease dynamics for these taxa (Becker et al., 2016; Bienentreu and Lesbarrères, 2020; De Andrade Serrano et al., 2022; Haver et al., 2022). Land use changes due to anthropogenic activities (e.g., urbanization, mining, industrialization, and agriculture) have intensified, leading to the over-exploitation, deterioration, and pollution of ecosystems (Archer and Stokes, 2000; Rashid and Romshoo, 2013; Kija et al., 2020). These anthropogenic threats have affected many species and biological processes (Thushari and Senevirathna, 2017; Ukaogo et al., 2020). Among vertebrates, amphibians present the greatest population decline and the greatest risk of extinction (Green et al., 2020; Button and Borzée, 2021). With permeable skin through which they exchange gases, pollutants, or substances, amphibians are more susceptible to changes or diseases than other vertebrate groups (Kaufmann and Dohmen, 2016) and are considered indicators of ecosystem health.
For instance, the pathogen Batrachochytrium dendrobatidis (Bd), the main causative agent of the disease chytridiomycosis, has resulted in mass mortalities among amphibians globally (Fisher and Garner, 2020). Additionally, the incidence of parasites like Hannemania mites has been acknowledged for their impact on amphibians, leading to deformities, loss of chemosensory function, reduced foraging capacity, diminished survival, and decreased reproduction (Anthony et al., 1994; Maksimowich and Mathis, 2000; Jacinto-Maldonado et al., 2016). It is recognized that anthropogenic activities, land-use changes, habitat loss, synergistic effects with pollutants (e.g., high concentrations of heavy metals), and other environmental factors (e.g., climate, altitude) may alter parasite and Bd infection dynamics, affecting occurrence rates, spread, transmission, prevalence, infection intensity of Bd, and host mortality (DeAlto, 2020; Siddons et al., 2020; Deknock et al., 2022). Both Bd and Hannemania affect amphibians by persisting and developing within the spongy epidermis layer (Duszynski and Jones, 1973; Stice and Briggs, 2010). During the larval instar of Hannemania, mites might transport toxic nanoparticles to the amphibian body (e.g., cerium oxide nanoparticles), potentially altering the parasite-host relationship and the pathogen-host dynamic, thereby increasing mortality, inducing behavioral changes, and inhibiting amphibians’ growth (Jacinto-Maldonado et al., 2022).
Mexico is a hotspot of diversity and endemism of amphibian species (Ochoa-Ochoa et al., 2014), and the presence of Bd and Hannemania parasites has been reported throughout the country including the Sonora state (Hoffmann, 1965; Loomis and Welbourn, 1969; Goldberg et al., 2002; Basanta et al., 2021; Jacinto-Maldonado et al., 2022). The Sonora state is situated at the northern edge of the country, hosting thirty-eight amphibian species (Lemos-Espinal et al., 2015, 2019). In 2014, a spill of 40,000 m3 of copper sulfate occurred in the Sonora River, and the consequences for wildlife remain unknown (León-García et al., 2018; Molina-Freaner and Martínez-Rodríguez, 2022). Additionally, the Sonora River receives waste such as garbage and wastewater, alongside ongoing anthropogenic activities like livestock rearing, agriculture, and urbanization. The impacts of these activities on the presence and dynamics of pathogens and parasites in amphibians are still poorly understood. Both the fungus and the mite can potentially interact in the spongy stratum of the amphibians; however, the impact of each one may be different and this can be influenced by the biotic and abiotic variables that characterize various types of disturbance. We aimed to analyze parasite and pathogen presence in amphibians (Hannemania mites and Bd) and their potential interaction with environmental variables where different anthropogenic activities are being developed in this region. We hypothesized that the presence and infection levels of the fungal pathogen Bd and Hannemania mites will vary depending on host species, the type of disturbance and the environmental variables associated with each study area.
2 Materials and methods
2.1 Study area
Our sampling was carried out in March, April, and July of 2021. Five sampling sites were selected for their anthropogenic activity. Three of them were affected by the copper sulfate spill that occurred in 2014 in Sonora state, Mexico (Figure 1): Bacanuchi (Mining site, closest to the spill site), Bacoachi (Extensive Livestock farming site, the spill did not reach this site), Aconchi (Wastewater discharge site, site affected by the spill), Ures (Agriculture site affected by the spill), and Hermosillo (Urban site, the spill did not reach this site). Sediment and water quality were analyzed at each sampling site.
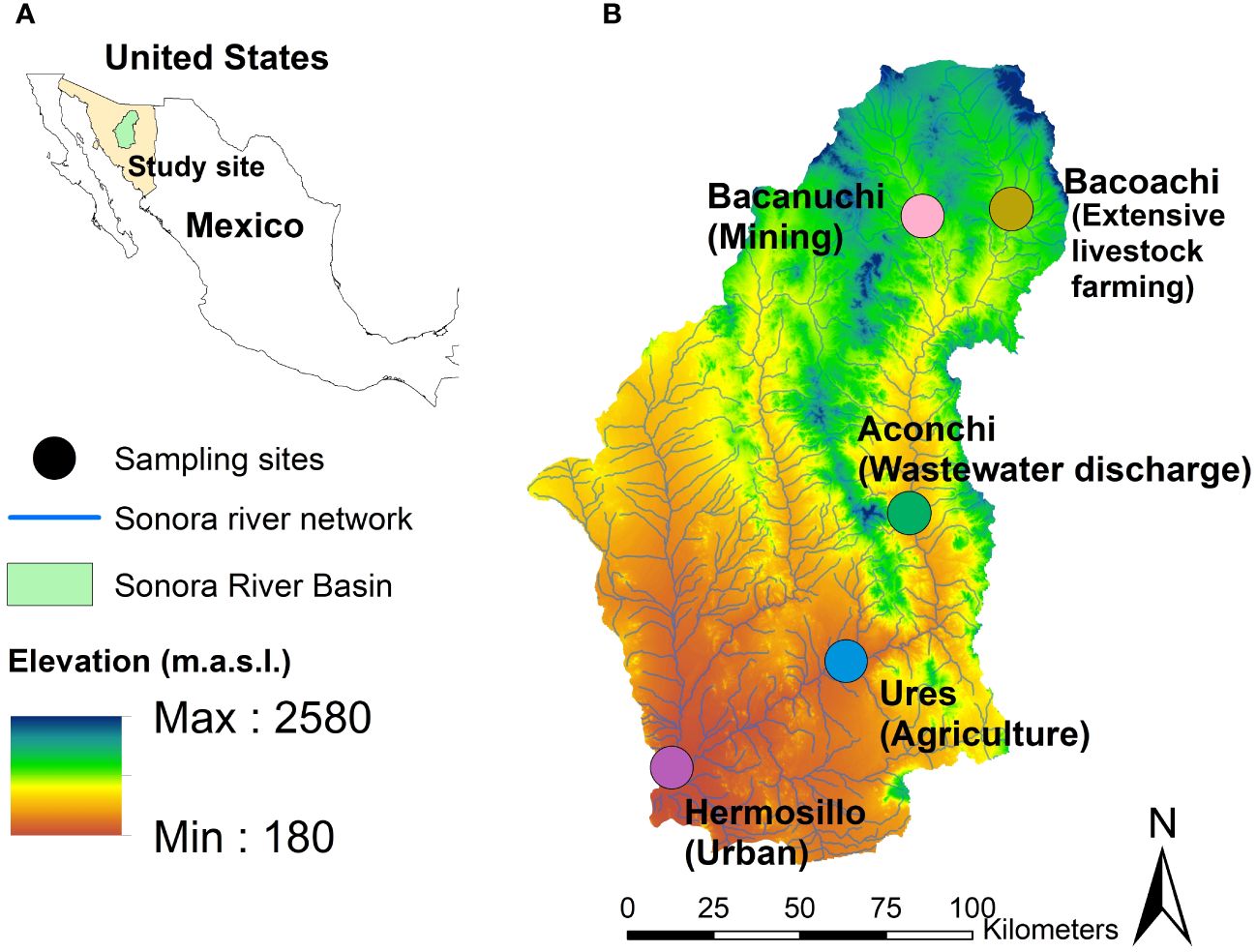
Figure 1 (A) Sonora state, Mexico. (B) Sampling sites where different anthropogenic activities are developing.
2.2 Environmental samples
At each site, soil samples were collected in three areas (two in the flood zone and one in the river). Sediments were analyzed using a portableX-rayfluorescence (PXRF) Niton FXL analyzer (ThermoScientificInc, MA, USA) and PXRF analyses were performed according to the procedures described in US EPA Method 6200. Three measures were implemented to ensure quality control and precision of PXRF measurements (Supplementary Material 1).
Water physicochemical characteristics were analyzed with a multi-parameter (Oakton PCSTestr 35 Impermeable) and the following variables were registered: pH, conductivity, total dissolved solids, and salinity (Supplementary Material 1).
2.3 Amphibian species sampling
The sampling effort for each site equaled 18 person-hours. The amphibians were manually collected wearing new vinyl gloves per each individual. Each specimen was swabbed, weighed, measured, and individuals were identified to species (Lemos-Espinal et al., 2015; Rorabaugh and Lemos-Espinal, 2016). Swabs were taken following Hyatt et al. (2007) protocol, the drink path, thighs, and toes were swabbed (5 times), and then the swab was preserved in liquid nitrogen and stored at −80° in the laboratory. Morphological variables such as malformations, injuries, or erythema (skin reddening) were also recorded (Supplementary Material 2). All individuals were released after sampling. Amphibians were collected under a scientific collector permit (SPARN/DGVS/02985/23) from the Secretaría de Medio Ambiente y Recursos Naturales.
2.4 Bd detection
DNA extraction was conducted with a Qiagen DNA extraction kit and real-time PCR was conducted as described in Boyle et al. (2004). All samples were analyzed in duplicate. Standards of DNA synthetic fragments (gBlocks, Integrated DNA Technologies) of 1, 10, 100, and 10,000 internal transcribed spacer (ITS) Bd equivalent copies were estimated to know ITS copies of Bd in each swab. Samples were considered positive if an exponential amplification curve was generated in both replicates. When one replicate was negative, a third replicate was run to determine the infection status of the sample.
2.5 Mite detection and taxonomic identification
After amphibian sampling (swab, weight, identification), amphibians with mites were anesthetized using an immersion bath of isoflurane (100%) (Doss et al., 2021) before mite removal. Subsequently, the area was disinfected with an hyper-oxidase solution, and individuals remained in disinfected containers until release (max. 5 mins). No individual died during this procedure. Once removed, mites were counted and preserved in 70% and 100% ethanol. Mites were cleared with lactophenol and then mounted them with PVA medium in semi-permanent microscope slides. Using the keys by Brennan and Goff (1977) and Hoffmann (1990), taxonomic identification was made. The mites were collected under a scientific collector permit (SGPA/DGVS/05384/22) from the Secretaría de Medio Ambiente y Recursos Naturales (SEMARNAT) and were deposited at the Colección Nacional de Ácaros (CNAC), Instituto de Biología, Universidad Nacional Autónoma de México, Mexico City, Mexico.
2.6 Statistical analysis
The amount of sediment composition variance within and among sampling sites was determined using boxplots and a linear Discriminant Analysis (LDA) to model the environmental data (sediment composition and water quality). Five classes based on the environmental variables of each sampling site were delimitated and 20% of samples in each category were chosen to conduct a cross-validation test (Balakrishnama and Ganapathiraju, 1998; Izenman, 2013).
A Canonical Correspondence Analysis (CCA) was used to investigate the relationships among matrices of amphibian species, amphibians positive for Bd, and amphibians parasitized by Hannemania mites and a set of concomitant sediment and water variables. The CCA provided a direct gradient analysis of amphibian species, amphibians parasitized by Hannemania mites, and amphibians positive for Bd relative to the underlying gradients within the measured environmental variables. The derived axes are linear combinations of environmental variables so that amphibian species, amphibian species parasitized or Bd positive were directly related to these axes under the assumption of unimodal amphibian species, amphibian parasitized or Bd positive response to environmental variables. The significance of the relationships between the parameters and the canonical axes was tested by permutational multivariate analysis of variance (PermANOVA).
To estimate and analyze the differences in Bd and Hannemania prevalences (as the proportion of infected individuals per population with 95% confidence intervals) among sampling sites, the prop.test function was used. Additionally, a Kruskal-Wallis test was carried out to analyze the differences in Bd-infection load (log10 transformation was made) among sampling sites. Both analyses were done through RStudio version 4.1.3 and in the R Stats Package (R Core Team, 2021; Basanta et al., 2022) (www.R-project.org). Additionally, a multiple regression analysis was used to identify associations between the intensity of Bd-infection (average of ITS copies of Bd in each swab of each individual analyzed), and the sampling site, the abundance of mites, and amphibian species. All analyses were done in the statistical environment R version 4.1.3 (www.R-project.org) using the vegan package (Oksanen et al., 2019).
3 Results
3.1 Amphibian species found in the sampling sites
In total, 135 amphibians across 10 species and 7 families were sampled: Anaxyrus woodhousii, A. punctatus, Gastrophryne mazatlanensis, Lithobates magnaocularis, L. yavapaiensis, Incilius alvarius, I. mazatlanensis, Spea multiplicata, Scaphiopus couchii, and Smilisca fodiens. Scaphiopus couchii was observed in all sampling sites. L. yavapaiensis and Smilisca fodiens were present in three out of five sites with more individuals found in Aconchi. L. magnaocularis was present in three out of five sites. Four amphibian species were observed in only one sampling site: A. woodhousii, A. punctatus, I. alvarius, and S. multiplicate (Figure 2; Supplementary Material 2).
3.2 Bd prevalence and infection intensity
Three amphibian species were positive for Bd: L. yavapaiensis, S. couchii, and G. mazatlanensis, the former showing the highest prevalence of 90.1% among all individuals. Bd was recorded at three sites with the highest number of Bd-positive individuals observed in Aconchi where wastewater discharges were constant (Table 1). Three Bd-positive individuals of L. yavapaiensis Bd-positives had erythema in Aconchi while two negative individuals of Anaxyrus woodhousii had erythema in Bacoachi (Supplementary Material 2).
The prevalence of Bd among sampling sites was significantly different (X2 = 30.937, df = 4, p-value = 3.154e-06) as was Bd-infection load (Kruskal-Wallis X2 = 9.867, df = 2, p-value = 0.007). A higher number of individuals with high Bd-infection load were observed in Aconchi and Bacoachi. At Aconchi 75% of individuals had a higher Bd-infection load range as compared to Bacoachi where just a few individuals showed a high Bd-infection load. No infected individuals were observed at Ures and Hermosillo and only 2 individuals were Bd-positive at Bacanuchi (Figure 3).
3.3 Hannemania mites’ presence and prevalence
Three amphibian species were positive for Hannemania mites: L. yavapaiensis, S. couchii, and S. fodiens with the former having the highest prevalence (Table 2). The prevalence of Hannemania mites was different among sampling sites (X2 = 16.682, df = 4, p = 0.002) with the highest prevalence at Bacoachi (Table 2).
3.4 Environmental variation
Bacanuchi and Aconchi showed the lowest water quality levels (higher conductivity values, salinity, and total dissolved solids). Additionally, the lowest pH value (<6.5) was recorded in Aconchi while the highest value was found in Ures (>8; Figure 4). There were also differences among sampling sites in sediment composition. Except for a few outliers, Sr, Cu, As, Zn, Mn, Sb, and Ca had the highest values in Bacanuchi (mining area). The highest concentrations of Cl, Rb, Fe, K, Nb, Y, Co, and Ti were found in Bacoachi (livestock area). In Aconchi (wastewater discharge area), V and Pb had the highest concentrations. By contrast, all elements showed low values In Ures as compared to other sites (Figure 4).
The LDA indicated a good fit of the data (accuracy, sensitivity, and specificity); 96% (n= 169) of all our environmental data analyzed (n= 176) were classified correctly in one of the five classes based on the environmental variables of each sampling site. Bacanuchi and Bacoachi were well segregated (sampling sites with less environmental similarities) while Hermosillo, Ures, and Aconchi showed some overlap (Figure 5).
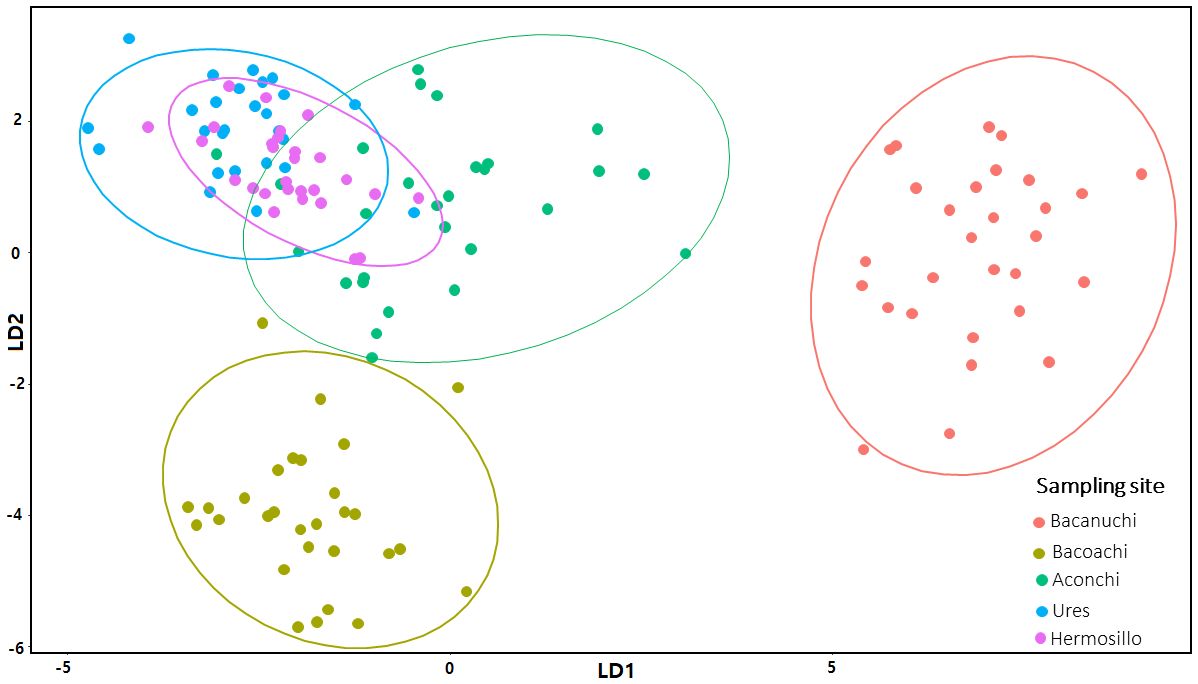
Figure 5 Linear Discriminant Analysis (LDA) of environmental variables at sampling sites. LDA1 = 39.5% and LDA2 = 26.4%.
3.5 Interaction among the intensity of Bd-infection versus the abundance of mites, the amphibian species, and the sampling site
While sampling site was not a significant predictor of Bd-intensity (r2 = 0.06, p = 0.99, df = 4), we found a significant interaction between Bd-infection and the abundance of mites (r2 = 2.63, p = 0.03, df = 4), and the amphibian species (r2 = 21.64, p = 0.01, df = 3) with our model explaining 63.71% of the variation in the multiple regression analysis. In particular, the intensity of Bd-infection was associated with the presence of L. yavapaiensis (p = 0.01).
3.6 Association of Bd and Hannemania mites with environmental variables
Both axes of the CCA explained 65.9% of the variance of environmental variables recorded in sediments and water (26.4% and 39.5% for axes 1 and 2 respectively). Environmental variables had significant effects on amphibian species, amphibians Bd-positive, and amphibians parasitized by Hannemania mites (all p < 0.05). In particular, both Bd-positive and Hannemania-parasitized amphibians were associated with two sampling sites (Bacoachi and Aconchi), the distribution of four amphibian species (L. yavapaiensis, G. mazatlanensis, A. woodhousii, and A. punctatus) and the signature of Ti, V, Y, Nb, Zr, Rb, Fe and Co (Figure 6).
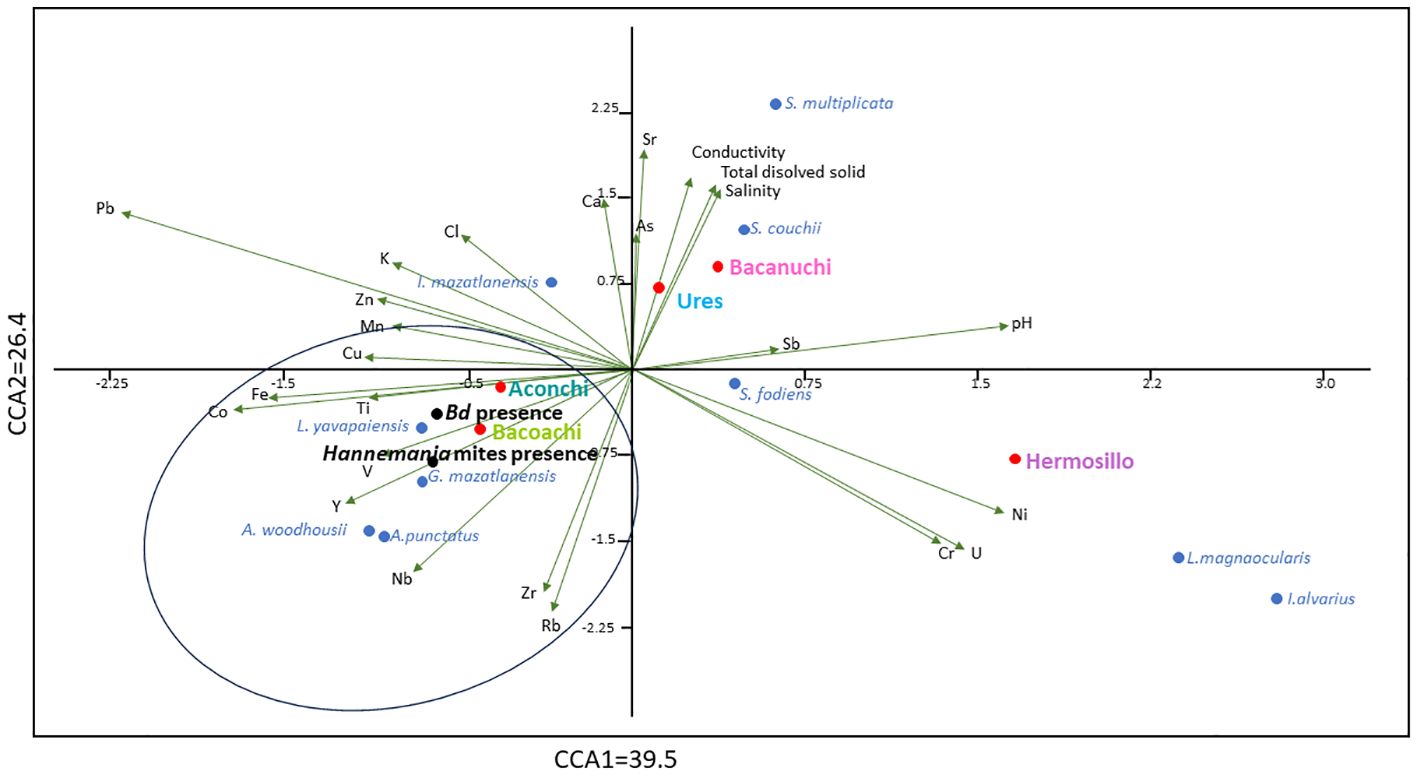
Figure 6 Canonical correspondence analysis of the variance of biotic variables (amphibian species, amphibians Bd-positive, and amphibians parasitized by Hannemania mites) due to environmental variables recorded in sediments and water. Sediments and water quality variables are in black, sampling site names are in color, and amphibian species are in blue. Amphibians positive for Bd and parasitized by Hannemania mites are in bold letters.
4 Discussion
To our knowledge, this is the first investigation of the presence and interaction of the fungal pathogen Bd and the Hannemania mites in disturbed environments in amphibians. Among sites with different types of perturbation, our results suggest that the prevalence of Bd and Hannemania mites were associated with the presence of four amphibian species and coincided with refractory elements characterized by mechanical or corrosion resistance. Moreover, we observed a positive association between the intensity of Bd-infection and the abundance of Hannemania mites in L. yavapaiensis. Such co-infection highlights the importance of monitoring environmental and biotic factors that modulate the relationship between parasites, pathogens, and hosts in transformed and polluted environments for future conservation strategies.
Aconchi exhibited the highest Bd infection load, coinciding with the presence of wastewater discharge at that site, aligning with previous studies that highlighted the association between low water quality or water pollution, particularly wastewater discharges, and Bd presence, prevalence, and infection load (Battaglin et al., 2016; Congram et al., 2022; Jacinto-Maldonado et al., 2023). By contrast, Hale et al. (2005) did not detect Bd in specimens collected in Aconchi in 2000, suggesting that Bd arrived recently or that changes in environmental conditions, such as water pollution, might have influenced the presence and Bd infection load in the region. Moreover, L. yavapaiensis showed the highest intensity of infection of Bd among amphibian species, with 23.08% of positive individuals showing erythema. The lowland leopard frog is listed as special protection with a declining population (NOM-059-SEMARNAT, 2010; IUCN, 2023) and further investigation should test the hypothesis that L. yavapaiensis acts as a reservoir or carrier of Bd in these sites and a potential threat to other susceptible amphibians (Miaud et al., 2016).
Among all species, Bd was also observed in G. mazatlanensis and S. couchii for the first time. In Sonora state, Bd has been previously reported in seven amphibian species (L. yavapaiensis, L. magnaocularis; Leptodactylus melanonotus; Agalychnis dacnicolor; Lithobates tarahumarae; Smilisca fodiens; Lithobates pustulosa) based on museum (Hale et al., 2005; Basanta et al., 2021) and live specimens from the Northern Jaguar Reserve and the locality of Tesopaco (Zamora-Bárcenas et al., 2012). Previous reports also indicated the presence of Bd in L. yavapaiensis in nearby Arizona, United States. While we observed a prevalence of 90.1% in this species, other studies reported higher (93%) and lower (43% and 1.6%) prevalences in Sonora (Schlaepfer et al., 2007; Savage et al., 2011). In addition, there are studies of L. yavapaiensis in Sonora with no information on prevalence (Bradley et al., 2002; Hale et al., 2005). Variations in prevalence, susceptibility, and vulnerability of amphibians to Bd infection could be linked to skin microbiome richness and composition, host life-history traits, phylogeny, morphology, physiology, gene expression, immune response, and resistance (Ortiz-Santaliestra et al., 2013; Eskew et al., 2018; Varela et al., 2018; Zamudio et al., 2020). Yet chytridiomycosis-related symptoms were only observed in three Bd-positive lowland leopard frogs (erythema in the ventral region) so more fieldwork, and experimental studies are imperative to better understand infection in this species.
The lowland leopard frog also showed the highest prevalence of Hannemania mites (27.27%), followed by S. fodiens (6.67%) and S. couchii (2.13%). The susceptibility of amphibians to Hannemania mites and their infestation rates have been associated with host size, behavior, microhabitat use, sex, the exposure time to the chiggers as well as environmental variables such as high humidity, high air temperature, proximity to water bodies, neutral and alkaline-pH water and areas with low canopy cover (Rankin, 1937; Jung et al., 2001; Wohltmann et al., 2006; Hatano et al., 2007; Alvarado-Rybak et al., 2018; Jacinto-Maldonado et al., 2020). Previous studies reported a higher prevalence of mites in L. yavapaiensis in the area (71.42%; Jacinto-Maldonado et al., 2022) suggesting interannual variation associated with environmental factors such as precipitation or temperature. This species was also the only amphibian species coinfected with Bd and Hannemania mites, and the only species with a significant relationship with the intensity of Bd-infection, suggesting a possible association between parasite-pathogen in this amphibian species. For instance, Hannemania mites might more readily infiltrate the stratum corneum and granulosum of the skin of Bd-positive amphibians due to skin damage, shedding, or ulcerations (Pessier, 2002; Berger et al., 2005). We also present the first report of Hannemania mites in S. fodiens. S. couchii has been recorded as the host of Hannemania hylae in Alamos, Sonora in 1943, but no information about its prevalence is available (Hoffmann, 1990). Among sites, Bacoachi presented the highest Hannemania prevalence, a cause for concern due to prior studies reporting toxic particles in sediments in this area. Hannemania mites have been identified as vectors of CeO2 and TiO2 particles (Jacinto-Maldonado et al., 2022). In amphibians, CeO2 particles can result in high mortality, growth inhibition, and genotoxic effects, while TiO2 particles may induce hormone disruption (thyroxine and triiodothyronine), cellular stress, decreased survival, altered growth, and cellular metabolism, as well as tissue damage (Zhang, 2011; Zhang et al., 2012; Hammond et al., 2013; Bour et al., 2015; Galdiero et al., 2017; Vijayaraj et al., 2018).
Our results also highlight that sites with a high prevalence of Bd or Hannemania mites, exhibit elevated levels of Fe, Ti, Co, Zr, V, Rb, Y, and Nb in sediments, particularly if L. yavapaiensis, G. mazatlanensis, A. woodhousii, and A. punctatus are present at these sites. These aforementioned elements, characterized as refractory elements renowned for their mechanical or corrosion resistance, find application in various sectors like alloys, ceramics, paints, and coatings (Balazic et al., 2007; Lodhi et al., 2008; Karimzadeh et al., 2019; Meza-Figueroa et al., 2020). Their extended persistence in the environment and the observed particle size (e.g. <850 nm) increase the risk of internalization, bioaccumulation (e.g. Fe, Co, and Ti), and potential transfer through the food chain, thus impacting aquatic and terrestrial ecosystems, thereby necessitating a more detailed analysis of particles (e.g. chemical composition, charge, surface structure, aerodynamic size, morphology) as well as periodic studies in these areas (Gál et al., 2008; Fabrega et al., 2011; Hammond et al., 2013; Jacinto-Maldonado et al., 2022; Esteves-Aguilar et al., 2023). With 38 amphibian species reported in Sonora and 21 amphibian species, including six endemics and three under special protection in Aconchi and Bacoachi, the impact of pollution and its association with the disease should be considered (NOM-059-SEMARNAT, 2010; Lemos-Espinal et al., 2019; IUCN, 2023; Naturalista, 2023).
Anthropogenic activities negatively impact and put at risk ecosystems and the species that live in them. Given the richness of amphibian diversity and the impact of anthropogenic activities in Sonora state and specifically in our study sites, continuous monitoring of environmental conditions, particularly water and sediment pollution should be pursued to understand better parasite-pathogen coinfections as well as better protect amphibian diversity.
Data availability statement
The original contributions presented in the study are included in the article/Supplementary Material. Further inquiries can be directed to the corresponding authors.
Ethics statement
The animal study was approved by Secretaría de Medio Ambiente y Recursos Naturales. The study was conducted in accordance with the local legislation and institutional requirements.
Author contributions
MJ-M: Conceptualization, Formal analysis, Investigation, Methodology, Writing – original draft, Writing – review & editing. DL: Conceptualization, Investigation, Resources, Writing – review & editing. ER: Data curation, Formal analysis, Methodology, Writing – review & editing, Resources. MB: Conceptualization, Methodology, Resources, Writing – review & editing. BG-G: Data curation, Formal analysis, Resources, Writing – review & editing. AR-M: Formal analysis, Methodology, Resources, Writing – review & editing, Data curation. OÁ-B: Formal analysis, Methodology, Writing – review & editing. EV-J: Methodology, Writing – review & editing, Data curation, Investigation. RP-L: Writing – review & editing. DM-F: Conceptualization, Funding acquisition, Investigation, Project administration, Resources, Supervision, Writing – review & editing.
Funding
The author(s) declare financial support was received for the research, authorship, and/or publication of this article. This research was funded by the National Council for Science and Technology in Mexico (CONAHCYT) Grant 309959 PRONACES and Grant A1-S-29697 to DM-F and a postdoctoral scholarship to MJ-M I1200/320/2022.
Acknowledgments
We gratefully acknowledge Miguel Ernesto Rosas Morales, Lourdes Gabriela Canizalez Juárez, and Iván Guillermo Souffle Lamphar from the Herpetology Club at the University of Sonora for field assistance and discussions. We also thank Alberto H. Orta and Griselda Montiel Parra for their valuable comments and technical support.
Conflict of interest
The authors declare that the research was conducted in the absence of any commercial or financial relationships that could be construed as a potential conflict of interest.
The author(s) declared that they were an editorial board member of Frontiers, at the time of submission. This had no impact on the peer review process and the final decision.
Publisher’s note
All claims expressed in this article are solely those of the authors and do not necessarily represent those of their affiliated organizations, or those of the publisher, the editors and the reviewers. Any product that may be evaluated in this article, or claim that may be made by its manufacturer, is not guaranteed or endorsed by the publisher.
Supplementary material
The Supplementary Material for this article can be found online at: https://www.frontiersin.org/articles/10.3389/famrs.2024.1372993/full#supplementary-material
References
Alvarado-Rybak M., Valenzuela-Sánchez A., Cevidanes A., Peñafiel-Ricaurte A., Uribe-Rivera D. E., Flores E., et al. (2018). High prevalence of chigger mite infection in a forest-specialist frog with evidence of parasite-related granulomatous myositis. Parasitol. Res. 117, 1643–1646. doi: 10.1007/S00436-018-5822-X/FIGURES/2
Anthony C. D., Mendelson J. R. I. I., Simons R. R. (1994). Differential parasitism by sex on plethodontid salamanders and histological evidence for structural damage to the nasolabial groove. Am. Midland Nat. 132, 302–307. doi: 10.2307/2426586
Archer S., Stokes C. (2000). Stress, disturbance and change in rangeland ecosystems. Rangeland Desertification 19, 17–38. doi: 10.1007/978-94-015-9602-2_3
Balakrishnama S., Ganapathiraju A. (1998). Linear discriminant analysis-a brief tutorial. Institute Signal Inf. Process. 18, 1–8.
Balazic M., Kopac J., Jackson M. J., Ahmed W. (2007). Review: titanium and titanium alloy applications in medicine. Int. J. Nano Biomaterials 1, 3–34. doi: 10.1504/IJNBM.2007.016517
Basanta M. D., Byrne A. Q., Rosenblum E. B., Piovia-Scott J., Parra-Olea G. (2021). Early presence of Batrachochytrium dendrobatidis in Mexico with a contemporary dominance of the global panzootic lineage. Mol. Ecol. 2, 424–437. doi: 10.1111/mec.15733
Basanta M. D., Rebollar E. A., García-Castillo M. G., Parra Olea G. (2022). Comparative analysis of skin bacterial diversity and its potential antifungal function between desert and pine forest populations of boreal toads Anaxyrus boreas. Microb. Ecol. 84, 257–266. doi: 10.1007/S00248-021-01845-1/METRICS
Battaglin W. A., Smalling K. L., Anderson C., Calhoun D., Chestnut T., Muths E. (2016). Potential interactions among disease, pesticides, water quality and adjacent land cover in amphibian habitats in the United States. Sci. Total Environ. 566–567, 320–332. doi: 10.1016/j.scitotenv.2016.05.062
Becker C. G., Rodriguez D., Longo A. V., Toledo L. F., Lambertini C., Leite D. S., et al. (2016). Deforestation, host community structure, and amphibian disease risk. Basic Appl. Ecol. 17, 72–80. doi: 10.1016/J.BAAE.2015.08.004
Berger L., Speare R., Skerratt L. F. (2005). Distribution of Batrachochytrium dendrobatidis and pathology in the skin of green tree frogs Litoria caerulea with severe chytridiomycosis. Dis. Aquat Organ 68, 65–70. doi: 10.3354/DAO068065
Bienentreu J. F., Lesbarrères D. (2020). Amphibian disease ecology: are we just scratching the surface? Herpetologica 76, 153–166. doi: 10.1655/0018-0831-76.2.153
Bour A., Mouchet F., Verneuil L., Evariste L., Silvestre J., Pinelli E., et al. (2015). Toxicity of ceO2 nanoparticles at different trophic levels - effects on diatoms, chironomids and amphibians. Chemosphere 120, 230–236. doi: 10.1016/j.chemosphere.2014.07.012
Boyle D. G., Boyle D. B., Olsen V., Morgan J. A. T., Hyatt A. D. (2004). Rapid quantitative detection of chytridiomycosis (Batrachochytrium dendrobatidis) in amphibian samples using real-time Taqman PCR assay. Dis. Aquat Organ 60, 141–148. doi: 10.3354/DAO060141
Bradley G. A., Rosen P. C., Sredl M. J., Jones T. R., Longcore J. E. (2002). Chytridiomycosis in native Arizona frogs. J. wildlife Dis. 38, 206–212. doi: 10.7589/0090-3558-38.1.206
Brennan J. M., Goff M. L. (1977). Keys to the genera of chiggers of the western hemisphere (Acarina: Trombiculidae). J. Parasitol. 63, 554–566. doi: 10.2307/3280021
Button S., Borzée A. (2021). An integrative synthesis to global amphibian conservation priorities. Glob Chang Biol. 27, 4516–4529. doi: 10.1111/GCB.15734
Congram M., Torres V. S., Wilson C. C., Kyle C. J., Lesbarrères D., Wikston M. J. H., et al. (2022). Tracking the prevalence of a fungal pathogen, Batrachochytrium dendrobatidis (chytrid fungus), using environmental DNA. Environ. DNA 4, 1–13. doi: 10.1002/EDN3.283
Da Rocha M. C., dos Santos M. B., Zanella R., Prestes O. D., Gonçalves A. S., Schuch A. P. (2020). Preserved riparian forest protects endangered forest-specialists amphibian species against the genotoxic impact of sunlight and agrochemicals. Biol. Conserv. 249, 108746. doi: 10.1016/J.BIOCON.2020.108746
DeAlto E. (2020) The current state of research on the global amphibian epidemic, chytridiomycosis: A systematic literature review and view into the future of the field (Independent Study Project (ISP) Collection). Available online at: https://digitalcollections.sit.edu/isp_collection/3344 (Accessed September 15, 2023).
De Andrade Serrano J., Toledo L. F., Sales L. P. (2022). Human impact modulates chytrid fungus occurrence in amphibians in the Brazilian Atlantic Forest. Perspect. Ecol. Conserv. 20, 256–262. doi: 10.1016/J.PECON.2022.05.002
Deknock A., Pasmans F., Van Leeuwenberg R., Van Praet S., De Troyer N., Goessens T., et al. (2022). Impact of heavy metal exposure on biological control of a deadly amphibian pathogen by zooplankton. Sci. Total Environ. 823, 153800. doi: 10.1016/J.SCITOTENV.2022.153800
Doss G. A., Mans C., Sladky K. K. (2021). “Analgesia, Anesthesia, and Monitoring,” in Exotic Animal Emergency and Critical Care Medicine (Oxford, United Kingdom: John Wiley & Sons), 746–757. doi: 10.1002/9781119149262.ch45
Duszynski D. W., Jones K. L. (1973). The occurrence of intradermal mites, Hannemania spp. (Acarina: Trombiculidae), in anurans in New Mexico with a histological description of the tissue capsule. Int. J. Parasitol. 3, 531–538. doi: 10.1016/0020-7519(73)90050-7
Eskew E. A., Shock B. C., Ladouceur E. E. B., Keel K., Miller M. R., Foley J. E., et al. (2018). Gene expression differs in susceptible and resistant amphibians exposed to Batrachochytrium dendrobatidis. R. Soc. Open Sci. 5, 1–23. doi: 10.1098/RSOS.170910
Esteves-Aguilar J., Mussali-Galante P., Valencia-Cuevas L., García-Cigarrero A. A., Rodríguez A., Castrejón-Godínez M. L., et al. (2023). Ecotoxicological effects of heavy metal bioaccumulation in two trophic levels. Environ. Sci. pollut. Res. 30, 49840–49855. doi: 10.1007/S11356-023-25804-0/METRICS
Fabrega J., Luoma S., Tyler C., Galloway T., Lead J. (2011). Silver nanoparticles: behaviour and effects in the aquatic environment. Environ. Int. 37, 517–531. doi: 10.1016/j.envint.2010.10.012
Fisher M. C., Garner T. W. J. (2020). Chytrid fungi and global amphibian declines. Nat. Rev. Microbiol. 18, 332–343. doi: 10.1038/s41579-020-0335-x
Gál J., Hursthouse A., Tatner P., Stewart F., Welton R. (2008). Cobalt and secondary poisoning in the terrestrial food chain: Data review and research gaps to support risk assessment. Environ. Int. 34, 821–838. doi: 10.1016/J.ENVINT.2007.10.006
Galdiero E., Falanga A., Siciliano A., Maselli V., Guida M., Carotenuto R., et al. (2017). Daphnia Magna and Xenopus laevis as In Vivo Models to Probe Toxicity and Uptake of Quantum Dots Functionalized with gH625. Int. J. Nanomedicine 12, 2717–2731. doi: 10.2147/ijn.s127226
Goldberg S. R., Wrenn W. J., Bursey C. R. (2002). Bufo mazatlanensis (Sinaloa toad), Rana tarahumarae (Tarahumara frog). Ectoparasites. Herp. Rev. 33, 301–302.
Green D. M., Lannoo M. J., Lesbarrères D., Muths E. (2020). Amphibian population declines: 30 years of progress in confronting a complex problem. Herpetologica 76, 97–100. doi: 10.1655/0018-0831-76.2.97
Hale S., Rosen P., Jarchow J., Bradley G. (2005). Effects of the chytrid fungus on the Tarahumara frog (Rana tarahumarae) in Arizona and Sonora, Mexico. Available online at: https://www.fs.usda.gov/rm/pubs/rmrs_p036.pdf#page=425 (Accessed December 24, 2023).
Hammond S. A., Carew A. C., Helbing C. C. (2013). Evaluation of the effects of titanium dioxide nanoparticles on cultured Rana catesbeiana tailfin tissue. Front. Genet. 4. doi: 10.3389/fgene.2013.00251
Hatano F. H., Gettinger D., Van Sluys M., Rocha C. F. D. (2007). Parasitism of Hylodes phyllodes (Anura: Cycloramphidae) by Hannemania sp. (Acari: Trombiculidae) in an area of Atlantic Forest, Ilha Grande, Southeastern Brazil. Parasite 14, 107–112. doi: 10.1051/PARASITE/2007142107
Haver M., Le Roux G., Friesen J., Loyau A., Vredenburg V. T., Schmeller D. S. (2022). The role of abiotic variables in an emerging global amphibian fungal disease in mountains. Sci. Total Environ. 815, 152735. doi: 10.1016/J.SCITOTENV.2021.152735
Hoffmann A. (1965). Contribuciones al conocimiento de los trombicúlidos mexicanos (Acarina: Trombiculidae). 11ªparte (Mexico City, México: Folia Entomológica Mexicana Press).
Hoffmann A. (1990). Los trombicúlidos de México (Acarida: Trombiculidae): Parte taxonómica (Universidad Nacional Autónoma de México Press).
Hyatt A. D., Boyle D. G., Olsen V., Boyle D. B., Berger L., Obendorf D., et al. (2007). Diagnostic assays and sampling protocols for the detection of Batrachochytrium dendrobatidis. Dis. Aquat Organ 73, 175–192. doi: 10.3354/DAO073175
IUCN (2023) The IUCN Red List of Threatened Species. Version 2023-1. Available online at: https://www.iucnredlist.org (Accessed 12 08 2023).
Izenman A. J. (2013). Linear Discriminant analysis. In: Modern Multivariate Statistical Techniques. Springer Texts in Statistics. Springer, New York, NY, 237–280. doi: 10.1007/978-0-387-78189-1_8
Jacinto-Maldonado M., García-Peña G. E., Paredes-León R., Saucedo B., Sarmiento-Silva R. E., García A., et al. (2020). Chiggers (Acariformes: Trombiculoidea) do not increase rates of infection by Batrachochytrium dendrobatidis fungus in the endemic Dwarf Mexican Treefrog Tlalocohyla smithii (Anura: Hylidae). Int. J. Parasitology: Parasites Wildlife 11, 163–173. doi: 10.1016/J.IJPPAW.2019.12.005
Jacinto-Maldonado M., González-Salazar C., Basanta M. D., García-Peña G. E., Saucedo B., Lesbarrères D., et al. (2023). Water pollution increases the risk of chytridiomycosis in mexican amphibians. EcoHealth 20, 74–83. doi: 10.1007/s10393-023-01631-0
Jacinto-Maldonado M., Meza-Figueroa D., Pedroza-Montero M., Lesbarrères D., Robles-Morúa A., Navarro-Espinoza S., et al. (2022). Mites as a potential path for ce-ti exposure of amphibians. Front. Environ. Sci. 10. doi: 10.3389/FENVS.2022.870645/BIBTEX
Jacinto-Maldonado M., Paredes-Leon R., Salgado-Maldonado G., Garcia A., Suzan G. (2016). New records of amphibians parasitized by chiggers in Los Tuxtlas Biosphere Reserve, Mexico, and taxonomic notes on Hannemania mexicana (Acariformes: Prostigmata: Leeuwenhoekiidae). Systematic Appl. Acarology 21, 13–20. doi: 10.11158/SAA.21.1.2
Jung R. E., Claeson S., Wallace J. E., Welbourn J. W.C. (2001). Eleutherodactylus guttilatus (Spotted Chirping Frog), Bufo punctatus (Red-spotted Toad), Hyla arenicolor (Canyon Tree Frog), and Rana berlandieri (Rio Grande Leopard Frog). Mite Infestation. Herpetological Rev. 32, 33–34.
Karimzadeh A., Aliofkhazraei M., Walsh F. C. (2019). ). A review of electrodeposited Ni-Co alloy and composite coatings: Microstructure, properties and applications. Surf Coat Technol. 372, 463–498. doi: 10.1016/J.SURFCOAT.2019.04.079
Kaufmann K., Dohmen P. (2016). Adaption of a dermal in vitro method to investigate the uptake of chemicals across amphibian skin. Environ. Sci. Eur. 28, 1–13. doi: 10.1186/S12302-016-0080-Y/FIGURES/3
Kija H. K., Ogutu J. O., Mangewa L. J., Bukombe J., Verones F., Graae B. J., et al. (2020). Spatio-temporal changes in wildlife habitat quality in the greater serengeti ecosystem. Sustainability 12, 2440. doi: 10.3390/SU12062440
Lemos-Espinal J. A., Smith H., Dixon J., Cruz A. (2015). Claves para los anfibios y reptiles de Sonora, Chihuahua y Coahuila, México. Amphibians and reptiles of Sonora, Chihuahua, and Coahuila, Mexico (Mexico City, México: CONABIO Press).
Lemos-Espinal J. A., Smith G. R., Rorabaugh J. C. (2019). A conservation checklist of the amphibians and reptiles of Sonora, Mexico, with updated species lists. Zookeys 829, 131. doi: 10.3897/ZOOKEYS.829.32146
León-García G. J., Meza-Figueroa D., Leobardo Valenzuela J., Encinas-Romero M. A., Villalba-Atondo A. I., Encinas-Soto K. K., et al. (2018). Study of Heavy Metal Pollution in Arid and Semi-Arid Regions Due to Mining Activity: Sonora and Bacanuchi Rivers Biomedical imaging with nanoplatforms for theranostics View project Participant in FAMA: Strengthening artisanal mining. P.I. Elizabeth Araux View project Study of Heavy Metal Pollution in Arid and Semi-Arid Regions Due to Mining Activity: Sonora and Bacanuchi Rivers. Int. J. Environ. Sci. Natural Resour. 11, 555804. doi: 10.19080/IJESNR.2018.10.555804
Lodhi Z. F., Mol J. M. C., Hovestad A., ‘t Hoen-Velterop L., Terryn H., De Wit J. H. W. (2008). Corrosion resistance of Zn-Co-Fe alloy coatings on high strength steel. Surface Coatings Technol. 203, 1415–1422. doi: 10.1016/j.surfcoat.2008.11.019
Loomis R. B., Welbourn W. C. (1969). A new species of Hannemania (Acarina, Trombiculidae) from Bufo punctatus of western north America, with comments on Hannemania hylae (Ewing). Bull. South. California Acad. Sci. 68, 160–168. doi: 10.3160/0038-3872-68.3.160
Maksimowich D. S., Mathis A. (2000). Parasitized salamanders are inferior competitors for territories and food resources. Ethology 106, 319–329. doi: 10.1046/J.1439-0310.2000.00526.X
Meza-Figueroa D., Pedroza-Montero M., Barboza-Flores M., Navarro-Espinoza S., Ruiz-Torres R., Robles-Morúa A., et al. (2020). Identification of refractory zirconia from catalytic converters in dust: An emerging pollutant in urban environments. Sci. Total Environ. 760, 1–11. doi: 10.1016/j.scitotenv.2020.143384
Miaud C., Dejean T., Savard K., Millery-Vigues A., Valentini A., Curt Grand Gaudin N., et al. (2016). Invasive North American bullfrogs transmit lethal fungus Batrachochytrium dendrobatidis infections to native amphibian host species. Biol. Invasions 18, 2299–2308. doi: 10.1007/S10530-016-1161-Y/TABLES/2
Molina-Freaner F., Martínez-Rodríguez J. (2022). Patterns of plant mortality caused by a copper mine spill. Anthropocene 39, 100344. doi: 10.1016/J.ANCENE.2022.100344
Naturalista (2023). Available online at: https://www.naturalist.mx.
NOM-059-SEMARNAT-2010 Secretaría de Medio Ambiente y Recursos Naturales (2010). Norma Oficial Mexicana. Protección ambiental. Especies nativas de México de flora y fauna silvestres. Categorías de riesgo y especificaciones para su inclusión, exclusión o cambio. Lista de especies en riesgo (Mexico City, México: Diario Oficial de la Federación, Instituto Nacional de Ecología, México).
Ochoa-Ochoa L. M., Campbell J. A., Flores-Villela O. A. (2014). Patterns of richness and endemism of the Mexican herpetofauna, a matter of spatial scale? Biol. J. Linn. Soc. 111, 305–316. doi: 10.1111/BIJ.12201/SUPPINFO
Oksanen J., Blanchet F. G., Friendly M., Kindt R., Legendre P., Mcglinn D., et al. (2019). Package “vegan” Title Community Ecology Package. Available at: https://cran.r-project.org/web/packages/vegan/vegan.pdf [Accessed December 1, 2023].
Ortiz-Santaliestra M. E., Rittenhouse T. A. G., Cary T. L., Karasov W. H. (2013). Interspecific and postmetamorphic variation in susceptibility of three north american anurans to Batrachochytrium dendrobatidis. J. Herpetology 47, 286–292. doi: 10.1670/11-134
Pessier A. P. (2002). An overview of amphibian skin disease. Semin. Avian Exotic Pet Med. 11, 162–174. doi: 10.1053/SAEP.2002.123980
Rankin J. S. (1937). An ecological study of parasites of some north carolina salamanders. Ecol. Monogr. 7, 169–269. doi: 10.2307/1943289
Rashid I., Romshoo S. A. (2013). Impact of anthropogenic activities on water quality of Lidder River in Kashmir Himalayas. Environ. Monit Assess. 185, 4705–4719. doi: 10.1007/S10661-012-2898-0/FIGURES/5
R Core Team (2021). R: A language and environment for statistical computing (Vienna, Austria: R Foundation for Statistical Computing). Available at: https://www.R-project.org/.
Rorabaugh J. C., Lemos-Espinal J. A. (2016). A field guide to the amphibians and reptiles of Sonora (Rodeo, New Mexico: ECO Wear & Publishing. United States Press).
Savage A. E., Sredl M. J., Zamudio K. R. (2011). Disease dynamics vary spatially and temporally in a North American amphibian. Biol. Conserv. 144, 1910–1915. doi: 10.1016/J.BIOCON.2011.03.018
Schlaepfer M. A., Sredl M. J., Rosen P. C., Ryan M. J. (2007). High prevalence of Batrachochytrium dendrobatidis in wild populations of lowland leopard frogs Rana yavapaiensis in Arizona. Ecohealth 4, 421–427. doi: 10.1007/S10393-007-0136-Y/FIGURES/2
Siddons S. R., Bray M. C., Searle C. L. (2020). Higher infection prevalence in amphibians inhabiting human-made compared to natural wetlands. J. Wildl Dis. 56, 823–836. doi: 10.7589/2019-09-220
Stice M. J., Briggs C. J. (2010). Immunization is ineffective at preventing infection and mortality due to the amphibian chytrid fungus Batrachochytrium dendrobatidis. J. Wildl Dis. 46, 70–77. doi: 10.7589/0090-3558-46.1.70
Thushari G. G. N., Senevirathna J. D. M. (2017). Plastic pollution in the marine environment. Heliyon 2020, e04709. doi: 10.1016/j.heliyon.2020.e04709
Ukaogo P. O., Ewuzie U., Onwuka C. V. (2020). Environmental pollution: causes, effects, and the remedies. Microorganisms Sustain. Environ. Health, 419–429. doi: 10.1016/B978-0-12-819001-2.00021-8
Varela B. J., Lesbarrères D., Ibáñez R., Green D. M. (2018). Abiotic factors and host-effects shape bacterial skin community structure and predicted function in three tropical frog species. Front. Microbiol. 9. doi: 10.3389/fmicb.2018.00298
Vijayaraj V., Liné C., Cadarsi S., Salvagnac C., Baqué D., Elger A., et al. (2018). Transfer and ecotoxicity of titanium dioxide nanoparticles in terrestrial and aquatic ecosystems: A microcosm study. Environ. Sci. Technol. 52, 12757–12764. doi: 10.1021/acs.est.8b02970
Wohltmann A., Köhler J., Martin P. (2006). Endoparasitic mite infections of anuran amphibians from Bolivian montane rain forests, with descriptions of two new Hannemania species (Acari: Parasitengona: Trombiculidae: Leeuwenhoekiinae). Organisms Diversity Evol. 6, 141–150. doi: 10.1016/J.ODE.2005.07.003
Yang L., Zhao S., Liu S. (2022). A global analysis of urbanization effects on amphibian richness: Patterns and drivers. Global Environ. Change 73, 102476. doi: 10.1016/J.GLOENVCHA.2022.102476
Zamora-Bárcenas D., Rorabaugh J. C., González C. L. (2012). Preliminary report: occurrence of the fungal skin disease organism, Batrachochytrium dendrobatidis, in amphibians of northwestern and central Mexico. Sonoran Herpetologist 25, 83–88.
Zamudio K. R., McDonald C. A., Belasen A. M. (2020). High variability in infection mechanisms and host responses: A review of functional genomic studies of amphibian chytridiomycosis. Herpetologica 76, 189–200. doi: 10.1655/0018-0831-76.2.189
Zhang J., Wages M., Cox S. B., Maul J. D., Li Y., Barnes M., et al. (2012). Effect of titanium dioxide nanomaterials and ultraviolet light coexposure on african clawed frogs (Xenopus laevis). Environ. Toxicol. Chem. 31, 176–183. doi: 10.1002/etc.718
Keywords: Batrachochytrium dendrobatidis, Hannemania mites, amphibians, anthropogenic activities, land use change, pollution
Citation: Jacinto-Maldonado M, Lesbarrères D, Rebollar EA, Basanta MD, González-Grijalva B, Robles-Morúa A, Álvarez-Bajo O, Vizuete-Jaramillo E, Paredes-León R and Meza-Figueroa D (2024) Batrachochytrium dendrobatidis and Hannemania mite’s relationships with Mexican amphibians in disturbed environments. Front. Amphib. Reptile Sci. 2:1372993. doi: 10.3389/famrs.2024.1372993
Received: 19 January 2024; Accepted: 19 March 2024;
Published: 03 April 2024.
Edited by:
Natalie Elizabeth Wildermann, King Abdullah University of Science and Technology, Saudi ArabiaReviewed by:
Raquel Fernanda Salla, Federal University of Sao Carlos, BrazilAlain Pagano, Université d’Angers, France
Copyright © 2024 Jacinto-Maldonado, Lesbarrères, Rebollar, Basanta, González-Grijalva, Robles-Morúa, Álvarez-Bajo, Vizuete-Jaramillo, Paredes-León and Meza-Figueroa. This is an open-access article distributed under the terms of the Creative Commons Attribution License (CC BY). The use, distribution or reproduction in other forums is permitted, provided the original author(s) and the copyright owner(s) are credited and that the original publication in this journal is cited, in accordance with accepted academic practice. No use, distribution or reproduction is permitted which does not comply with these terms.
*Correspondence: Mónica Jacinto-Maldonado, monica.jacinto@unison.mx
†These authors have contributed equally to this work and share first authorship