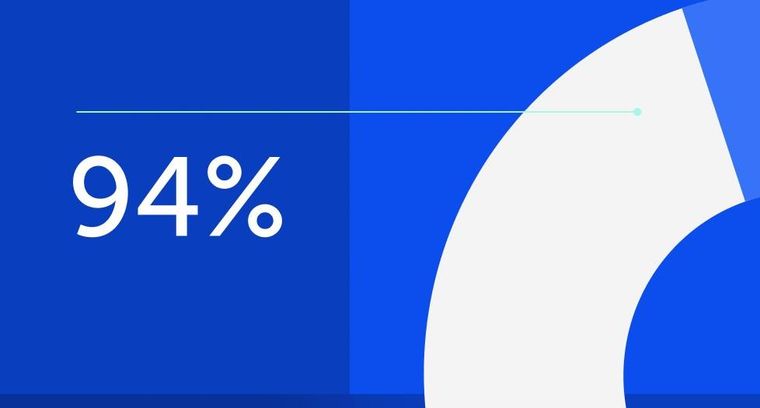
94% of researchers rate our articles as excellent or good
Learn more about the work of our research integrity team to safeguard the quality of each article we publish.
Find out more
ORIGINAL RESEARCH article
Front. Agron., 09 April 2025
Sec. Weed Management
Volume 7 - 2025 | https://doi.org/10.3389/fagro.2025.1576547
This article is part of the Research TopicMethods in Weed ManagementView all 5 articles
In February 2024, the United States Environmental Protection Agency (EPA) vacated the registrations of dicamba products for over-the-top applications on dicamba-resistant cotton and soybean following a court ruling. This decision has raised significant concerns among United States farmers, who now have limited chemical options to manage tough-to-control weeds. However, the risk of off-target dicamba movement to sensitive plants remains a critical issue. If permitted in the future, applying dicamba as a preemergence (PRE) treatment, tank-mixed with other soil residual herbicides, could help reduce off-target movement while preserving its utility for managing problem weeds. Field experiments were conducted in 2022 and 2023 in Minnesota and North Dakota, and in 2021 and 2022 in Wisconsin, to evaluate the effectiveness of dicamba-based PRE herbicide mixtures in soybean. Across all site-years, dicamba tank mixed with other soil residual herbicides provided better control of targeted weed species at 21 d after treatment (DAT) compared to applying the residual herbicides alone. In Minnesota, dicamba-based herbicide tank mixes provided an average waterhemp control of 72%, compared to 59% for treatments without dicamba at 21 DAT. Similarly, in North Dakota, waterhemp control at 21 DAT improved from 74% with residual herbicides alone to 97% when tank mixed with dicamba. In Wisconsin, dicamba-based tank mixes resulted in 96% control of common ragweed and 83% of velvetleaf, versus 83% and 73% for those species, respectively, without dicamba. At the Minnesota site, adding dicamba to residual herbicides improved common lambsquarters and giant ragweed control by 17% and 20%, respectively, and their densities were reduced by at least 50%. At the North Dakota site, kochia control was improved by 23% with dicamba PRE. The results from this research outlined the effectiveness of PRE application of dicamba tank mixed with other residual herbicides for effective weed management in the Upper Midwest.
Soybean [Glycine max (L.) Merr.] is an important crop in the United States, with an estimated planted area of 34.84 million hectares in 2024 (Anonymous, 2024a). Weeds can potentially cause soybean yield losses worth US $16.2 billion in the United States when no control measures are applied (Soltani et al., 2017). With the widespread distribution of glyphosate-resistant (GR) weeds such as Palmer amaranth (Amaranthus palmeri S. Watson), waterhemp [Amaranthus tuberculatus (Moq.) J. D. Sauer], common ragweed (Ambrosia artemisiifolia L.), giant ragweed (Ambrosia trifida L.), kochia [Bassia scoparia (L.) A. J. Scott], and horseweed (Erigeron canadensis L.), there is an increasing trend in the use of preemergence (PRE) herbicides in the United States (Beckie et al., 2019).
Dicamba is an auxin mimic herbicide [Weed Science Society of America’s (WSSA’s) herbicide site of action Group 4] commonly used for postemergence (POST) control of broadleaf weeds in pastures, turf, and cereal crops (Shaner, 2014). It is highly soluble in water (4,500 mg L−1 at 25°C) and has low soil binding properties (soil sorption coefficient normalized to organic carbon, Koc = 2 g mL−1), therefore, can be used in limited rainfall environments to achieve greater residual activity (Cojocaru et al., 2013; Shaner, 2014; Silva et al., 2023). However, it is important to note that higher water solubility and low sorption potential of dicamba can increase the risk of leaching under higher rainfall conditions (Gazola et al., 2022). The commercialization of dicamba-resistant soybean and cotton (Gossypium hirsutum L.) allowed farmers to use dicamba POST in these crops to control GR weeds. Following commercialization, soybean farmers in the United States rapidly adopted dicamba-resistant traits (Roundup Ready2 Xtend®: glyphosate and dicamba resistant; XtendFlex®: glyphosate, dicamba, and glufosinate resistant). Dicamba use in soybean increased nearly 97-fold ranging from 0.078 to 7.6 million kg of active ingredients between 2015 and 2020 due to the adoption of dicamba-resistant traits and the use of over-the-top dicamba in the United States (Mortensen et al., 2024).
Physical drift of herbicide can injure nearby sensitive plants when sprayed using improper nozzles or under high wind speed and gusts. Additionally, dicamba’s high vapor pressure makes it highly volatile, allowing it to vaporize easily. The vaporized form of dicamba, along with finer spray particles, can be carried by the wind under certain conditions, often referred to as “temperature inversions” and “atmospheric loading” (Hammer et al., 2024; Oseland et al., 2024; Sarangi et al., 2021; Soltani et al., 2020). In 2021, the United States Environmental Protection Agency (EPA) received nearly 3,500 dicamba injury complaints caused by off-target movement of dicamba to sensitive crops including row crops, orchards, and vegetables (Anonymous, 2021). This volatility has led to widespread damage to dicamba-susceptible soybean and other broadleaf plants, prompting the EPA to implement stricter application restrictions (Anonymous, 2020). Some states, including Minnesota, imposed additional state-specific restrictions for dicamba use like date and temperature cut-off limits (Anonymous, 2022). Following a recent court ruling in February 2024, the EPA issued an order for the limited sale and distribution of dicamba products in the United States for 2024, with the future of dicamba application in soybean and cotton remaining uncertain (Anonymous, 2024b). In addition to off-target movement concerns, the heavy reliance on dicamba POST for controlling GR weeds has exerted strong selection pressure, leading to the evolution of dicamba resistance in weeds such as kochia, Palmer amaranth, and waterhemp (Dhanda et al., 2024; Foster and Steckel, 2022; Heap, 2024; Kumar et al., 2019; Singh et al., 2024).
Applying dicamba PRE can reduce the potential of volatilization compared to POST applications (Striegel et al., 2021). Since PRE applications are made to bareground before the emergence of both soybean and weeds, temperature inversions are less frequent earlier in the season, which reduces the risk of dicamba volatilization. The application of PRE herbicides can reduce overreliance on POST herbicides (Knezevic et al., 2019). Additionally, PRE herbicides can extend the window before POST applications are needed, helping to manage early-season weed competition (Knezevic et al., 2013). A previous greenhouse experiment showed that control of dicamba-resistant kochia increased from 10% with dicamba (at 560 g ae ha−1) applied POST to 97% with dicamba (420 g ae ha−1) applied PRE, indicating the significance of PRE application of dicamba (Ou et al., 2018). Johnson et al. (2010) reported that PRE application of dicamba provided 97% control of common lambsquarters (Chenopodium album L.) and horseweed at 21 days after treatment (DAT). However, limited information is available on the effect of PRE application of dicamba when tank mixed with other soil residual herbicides on weed control in dicamba-resistant soybean. The objective of this research was to compare the efficacy of commonly used PRE herbicides, with or without the addition of dicamba at 560 g ae ha−1 in tank mixes, for weed control in dicamba-resistant soybean in the Upper midwestern United States.
Field experiments were conducted at three locations in Minnesota, North Dakota, and Wisconsin: the University of Minnesota’s Rosemount Research and Outreach Center in Rosemount, MN (44°42′ N; 93°04′ W), North Dakota State University’s Research Farm in Fargo, ND (46°55′ N; 96°51′ W), and the University of Wisconsin-Madison’s Arlington Agricultural Research Station near Arlington, WI (43°30′ N; 89°33′ W). Experiments were carried out in 2022 and 2023 in Minnesota and North Dakota and in 2021 and 2022 in Wisconsin. Additionally, a separate experiment for kochia control was conducted in 2023 at the North Dakota site, totaling seven site-years. The soil type at the Minnesota site was silty clay loam (8.7% sand, 57.6% silt, and 33.7% clay) with 4.5% organic matter and 6.0 pH. The soil at the North Dakota site was silty clay (2.2% sand, 41.1% silt, and 56.7% clay) with 5.3% organic matter and a pH of 8.0. At the Wisconsin site, the soil was Plano silt loam (7% sand, 68% silt, and 25% clay) with 3.4% organic matter and a pH of 6.4 in 2021 and 5.9 in 2022. All sites were under conventional tillage [two-pass tillage in the fall and spring (2–3 days before soybean planting)], except for the North Dakota site in 2023, which was under a no-till system. All tillage-based site-years were weed-free at the time of soybean planting and PRE application. Paraquat (Gramoxone® SL 3.0; Syngenta Crop Protection, Greensboro, NC, 27409) at 420 g ai ha−1 plus non-ionic surfactant (NIS) at 0.25% were applied 1 day before planting to control weeds at the North Dakota sites in 2023. Treatments were laid out in a randomized complete block design with four replications with a plot size of 9- by 3-m length and width. Soybean was planted in 76.2-cm row spacing across all site-years. Soybean planting and harvesting dates, variety and seeding rate information, and herbicide application dates are provided in Table 1, and the PRE herbicide treatment details are outlined in Table 2. Acetochlor and fomesafen premix treatment was not included in the North Dakota site, as this herbicide cannot be applied in this region due to geographic restrictions (Anonymous, 2024c). Dicamba-only PRE treatment was not included in Wisconsin in 2021; however, it was included in 2022. Herbicides were applied using a CO2-pressurized backpack sprayer equipped with TTI 110015 nozzles (Teejet, Springfield, IL, 62703) at the Minnesota and Wisconsin sites, and TTI 11002 nozzles at the North Dakota site, calibrated to deliver 140 L ha−1 of spray solution at 248 kPa. Daily air temperature (°C) and precipitation (mm) data for each growing season for all site-years were collected from nearby weather stations. Percent weed control was estimated visually at 21 DAT on a scale of 0%–100%, where 0% means no control and 100% means complete control. This research utilized naturally occurring weed populations at respective sites, with data collected only on the dominant species. At the Minnesota site, waterhemp and common lambsquarters control were evaluated in both 2022 and 2023, while giant ragweed control was estimated only in 2023, along with the other two species. At the North Dakota site, waterhemp control was measured in 2022 and 2023, and kochia control was evaluated in 2023 at a separate experiment. In Wisconsin, common ragweed and velvetleaf (Abutilon theophrasti Medik.) control were estimated in both 2021 and 2022. Weed density at the Minnesota site was recorded at 21 DAT by placing two 0.5- by 0.5-m quadrats randomly between the middle two soybean rows in each plot, and the data were converted to plants m−2. Soybean injury data were visually recorded at 21 DAT across all site-years using a 0%–100% scale, where 0% means no injury and 100% means soybean plant death. The soybean stand was recorded only at the Minnesota site by counting the number of plants from 1 m of the two center rows of each plot at 21 DAT.
Table 1. Details for the field experiments conducted from 2021 to 2023 in Minnesota, North Dakota, and Wisconsin.
Table 2. List of preemergence herbicide treatments, rates, trade names, manufacturers, and adjuvants used in the field experiments for weed control in dicamba-resistant soybean from 2021 to 2023 in Minnesota, North Dakota, and Wisconsin.
The data were analyzed using R statistical software (R Core Team, 2024). Given the variability and abundance of weed species across the experiment sites, data on each weed species from each site were analyzed separately. Percent weed control and soybean injury data were subjected to generalized linear mixed models using “beta” distribution with a “log” link function in glmmTMB package (Brooks et al., 2017). Weed density and soybean stand count data were subjected to generalized linear mixed models but using a “negative binomial” distribution with a “log” link function. The PRE herbicide treatment was considered a fixed effect, and replications nested within years (or replication alone in the single-year data) were considered random effects. Model assumptions were verified by plotting simulated residuals and estimating goodness-of-fit using DHARMa package (Hartig, 2024). Analysis of variance (ANOVA) and Type III Wald chi-square tests were performed followed by mean separation using Fisher’s protected LSD at α = 0.05. A priori orthogonal contrast analysis was performed to compare the efficacy of PRE herbicide programs with and without dicamba addition in the tank mixture.
The average temperature at the Minnesota site was 18°C and 19°C, and the total precipitation (from May to June) was 130 and 101 mm in 2022 and 2023, respectively (Figure 1). At the North Dakota site, the average temperature was 17°C and 20°C and the total precipitation (from May to June) was 154 and 169 mm in 2022 and 2023, respectively. The average temperature at the Wisconsin site was 18°C and 17°C and the total precipitation (from May to June) was 182 and 207 mm in 2021 and 2022, respectively (Figure 1). Soybean injury from PRE herbicide treatments was minimal (<10%) at Minnesota and Wisconsin sites at 21 DAT, and no soybean injury was observed at the North Dakota site (data not shown). In addition, no soybean stand reduction was observed at the Minnesota site (data not shown).
Figure 1. Daily average air temperature (°C) and precipitation (mm) in May and June: Arlington, Wisconsin (2021–2022), Fargo, North Dakota (2022–2023), and Rosemount, Minnesota (2022–2023). The “PRE” indicates the date of preemergence herbicide application.
In Minnesota, all PRE herbicide treatments tank mixed with dicamba provided 69%–76% residual control of waterhemp at 21 DAT, which was comparable to acetochlor and fomesafen (69%), acetochlor plus metribuzin (67%), and dicamba-only (60%) treatments (Table 3). Acetochlor, when tank mixed with dicamba, reduced waterhemp density to 1 plant m−2, which was similar to acetochlor and fomesafen plus dicamba, and acetochlor plus metribuzin treatments (2 plants m−2). Dicamba-only PRE treatment reduced waterhemp density by 72% compared to the non-treated control at 21 DAT. Benoit et al. (2019) reported 54% control and 56% density reduction of waterhemp resistant to WSSA Groups 2, 5, and 9 with PRE application of dicamba. Hedges et al. (2018) reported that PRE application of pyroxasulfone plus premix of glyphosate and dicamba resulted in 98% control of GR waterhemp at 28 DAT. In this research in Minnesota, adding dicamba to a premix of metribuzin and sulfentrazone reduced waterhemp density by 55% and 88% compared to metribuzin and sulfentrazone only and non-treated control treatments, respectively, indicating the significance of adding dicamba as PRE in a mixture with other residual herbicides (Table 3). When averaging across the treatments, contrast analysis indicated that waterhemp control increased from 59% to 72% and density reduced from 7 to 3 plants m−2 with dicamba-based mixtures compared to non-dicamba treatments (Figures 2, 3). The relatively lower waterhemp control with all the PRE herbicides at the Minnesota site was likely due to lower rainfall after herbicide application (Figure 1).
Table 3. The effect of preemergence herbicide treatments on the control and density of waterhemp, common lambsquarters, and giant ragweed at 21 days after treatment in field experiments conducted in Rosemount, Minnesota, in 2022 and 2023a,b.
Figure 2. Percent weed control at 21 days after treatment with and without dicamba in combination with residual herbicides applied preemergence. Triangles in the boxplot represent replicated data points, the cross (x) represents the estimated mean, gray circles represent boxplot outliers, and the solid black line within each box represents the median. Mean weed control comparisons are presented as significant at p < 0.01 (**) and p < 0.001 (***). Common lambsquarters, giant ragweed, and waterhemp were present at the Minnesota (MN) site; waterhemp and kochia were present at the North Dakota (ND) site; and common ragweed and velvetleaf were present at the Wisconsin (WI) site.
Figure 3. Weed density at 21 days after treatment at the Minnesota site with and without dicamba in combination with residual herbicides applied preemergence. Triangles in the boxplot represent replicated data points, the cross (x) represents the estimated mean, gray circles represent boxplot outliers, and the solid black line within each box represents the median. Mean weed density comparisons are presented as significant at p < 0.001 (***).
In North Dakota, all dicamba-based PRE herbicide mixtures, except for flumioxazin and pyroxasulfone premix plus dicamba, provided 98% waterhemp control at 21 DAT (Table 4). Waterhemp control was lower (≤ 65%) with flumioxazin alone or flumioxazin and pyroxasulfone; however, when dicamba was tank-mixed with these herbicides, control increased to ≥94%. Averaging across the treatments, tank mixing dicamba with soil residual herbicides provided 97% waterhemp control compared to 74% control with herbicide treatments without dicamba (Figure 2). A recent study conducted in Ontario, Canada reported 59% and 89% control of waterhemp with a separate PRE application of dicamba and acetochlor, respectively; however, the control increased to 94% when dicamba was tank mixed with acetochlor (Symington et al., 2024). The same study also reported that combined PRE application of dicamba plus acetochlor reduced waterhemp density by 98% compared to non-treated control.
Table 4. The effect of preemergence herbicide treatments on waterhemp and kochia control at 21 days after treatment in field experiments conducted in Fargo, North Dakota, in 2022 and 2023a,b,c.
Dicamba applied alone or in a tank mix with other PRE herbicides resulted in 56%–67% control of common lambsquarters at 21 DAT in Minnesota, which was similar to flumioxazin alone (61%) (Table 3). The contrast analysis showed that dicamba-based PRE herbicide mixtures provided an average common lambsquarters control of 62%, compared to only 45% control achieved by non-dicamba treatments (Figure 2). All dicamba-based mixtures reduced common lambsquarters density by ≥77% compared to non-treated control in Minnesota. Non-treated control had a common lambsquarters density of 52 plants m−2, which was similar to PRE application of acetochlor-alone (23 plants m−2) and metribuzin and sulfentrazone premix (34 plants m−2) treatments; however, the density reduced to ≤9 plants m−2 when dicamba was mixed to these herbicides (Table 3). Jha et al. (2015) also reported relatively lower control of common lambsquarters (51%–65%) with PRE application of acetochlor in corn (Zea mays L.). The contrast analysis also showed that adding dicamba in the tank mixture reduced common lambsquarters density substantially (9 plants m−2) compared to non-dicamba tank mixes (20 plants m−2) (Figure 3).
In Minnesota at 21 DAT, tank mixing dicamba with soil residual herbicides (except for acetochlor and fomesafen premix) improved giant ragweed control compared to the herbicides applied alone (Table 3). Dicamba-alone treatment applied PRE resulted in 38% control of giant ragweed at that time. Johnson et al. (2010) also reported <10% giant ragweed control with PRE application of dicamba. When averaged across the treatments, dicamba-based PRE herbicide mixtures resulted in 34% giant ragweed control compared to only 14% control achieved by non-dicamba treatments (Figure 2). Similarly, dicamba in tank mixtures reduced average giant ragweed density to 4 plants m−2 compared to herbicide treatments without dicamba (8 plants m−2) (Figure 3). Soltani et al. (2011) reported that PRE application of dicamba in corn resulted in 60%–80% giant ragweed control and reduced density by 45% compared to non-treated control. Giant ragweed is an early-emerging weed and continues to germinate later in the growing season (Schutte et al., 2012); therefore, the PRE application of dicamba would be effective when tank-mixed with another effective PRE herbicide. Residual control of giant ragweed is challenging because of its larger seed size. Silva et al. (2023) reported greater giant ragweed control with PRE application of dicamba in corn with 21 mm of rainfall received within 15 d of application. However, in the present study, the amount of rainfall received within 15 days of PRE application at the site was only 8 and 2 mm during 2022 and 2023, respectively, which resulted in lower control (Figure 1).
Common ragweed and velvetleaf were the two predominant weed species at the Wisconsin research site in 2021 and 2022. Dicamba applied alone or in a tank mixture with other PRE herbicides resulted in ≥94% control of common ragweed, which was better than any of the PRE treatments without dicamba at 21 DAT (Table 5). The contrast analysis also showed that dicamba-based PRE herbicide mixtures provided an average common ragweed control of 96% compared to 83% control with non-dicamba herbicides (Figure 2). Jha et al. (2022) also reported that adding dicamba to a premix of metribuzin and sulfentrazone increased common ragweed control from 80% to 99% compared to metribuzin and sulfentrazone only. In a field experiment conducted in Ontario, Canada, Soltani et al. (2018) reported that PRE application of dicamba at 600 g ae ha−1 resulted in 100% control and density reduction in GR common ragweed in corn at 28 DAT.
Table 5. The effect of preemergence herbicide treatments on common ragweed and velvetleaf control at 21 days after treatment in field experiments conducted in Arlington, Wisconsin, in 2021 and 2022a.
In Wisconsin, flumioxazin-based PRE herbicide treatments provided ≥90% velvetleaf control, regardless of the dicamba tank mix. This was comparable to dicamba only (82%) and all dicamba-based tank-mix PRE treatments (≥ 83%) at 21 DAT (Table 5). Sarangi and Jhala (2019) also reported 98% velvetleaf control with PRE application of flumioxazin and pyroxasulfone in soybean at 28 DAT. Similarly, Mahoney et al. (2014) also reported that PRE application of flumioxazin and pyroxasulfone resulted in 94% control of velvetleaf at 28 DAT. When averaged across the treatments, dicamba-based PRE herbicide mixtures provided 83% velvetleaf control compared to 73% control obtained with herbicide treatments without dicamba (Figure 2). Jha et al. (2022) reported 47% and 70% velvetleaf control with acetochlor and a premix of metribuzin and sulfentrazone, respectively; however, control increased to 83% and 91% with the addition of dicamba.
In Fargo, North Dakota, dicamba-based herbicide mixtures resulted in 85%–94% kochia control, which was similar to dicamba alone (86%) or metribuzin and sulfentrazone premix (87%) at 21 DAT (Table 4). Ou et al. (2018) reported that PRE application of dicamba at 280–420 g ae ha−1 resulted in 75%–97% control of dicamba-resistant kochia, whereas POST application of dicamba at 560 g ae ha−1 provided only 10% control. The same research also reported that PRE application of dicamba at 560 g ae ha−1 could provide consistent kochia control in fields where seed densities range from 1 to 1,200 viable seeds m−2 and no dicamba resistance observed. Acetochlor alone provided 23% kochia control in North Dakota, but the control improved substantially to 85% when dicamba was tank mixed with acetochlor (Table 4). The contrast analysis indicated that dicamba-based herbicide mixtures resulted in an average of 84% kochia control compared to only 61% control achieved with non-dicamba treatments (Figure 2).
This research was conducted over seven site-years across diverse environmental and soil conditions in the Upper Midwest, focusing on the six most dominant weed species in soybean. The findings demonstrate that applying dicamba-based herbicide mixtures as PRE treatments can be an effective option to control problematic weeds in dicamba-resistant soybean compared to using soil residual herbicides alone (Figure 4). Dicamba, known for its high water solubility and low soil binding property (Cojocaru et al., 2013; Shaner, 2014), can be beneficial when used as a PRE herbicide in tank mixtures with other residual herbicides. This combination helps mitigate the performance risks typically associated with the reduced efficacy of soil residual herbicides under drought conditions, where low soil moisture availability can limit their effectiveness. Applying dicamba PRE is expected to minimize its off-target movement to sensitive plants compared to its POST application. Weeds resistant to dicamba have been confirmed in the United States (Foster and Steckel, 2022; Heap, 2024; Kumar et al., 2019; Singh et al., 2024). However, applying dicamba as a PRE treatment along with other soil residual herbicides can diversify the herbicide sites of action and reduce the selection pressure exerted by a single herbicide. In some cases, PRE application of dicamba achieved satisfactory control of dicamba-resistant weeds, whereas dicamba applied POST at the same dose did not provide comparable control (Ou et al., 2018). However, it is important to note that applying dicamba as a PRE treatment in non-dicamba-resistant soybean should be avoided to prevent crop injury. This research highlights the new use of dicamba as PRE treatment in dicamba-resistant soybean, offering effective weed control while reducing the risk of off-target movement. The findings provide valuable insights for registrants, legislators, and policymakers, helping them make informed decisions about the future use of dicamba in dicamba-resistant soybean.
Figure 4. Weed control at 21 days after treatment as affected by the tank mixtures of dicamba with soil residual herbicides applied preemergence in dicamba-resistant soybean in Rosemount, Minnesota in 2022. Treatments are (A) non-treated control, (B) flumioxazin and pyroxasulfone, (C) flumioxazin and pyroxasulfone + dicamba, and (D) dicamba only.
The raw data supporting the conclusions of this article will be made available by the authors, without undue reservation.
SD: Data curation, Writing – original draft, Writing – review & editing. NS: Formal analysis, Investigation, Writing – review & editing. JI: Conceptualization, Data curation, Funding acquisition, Investigation, Methodology, Project administration, Resources, Supervision, Validation, Writing – review & editing. RD: Data curation, Investigation, Methodology, Validation, Writing – review & editing. RW: Conceptualization, Funding acquisition, Project administration, Resources, Supervision, Validation, Writing – review & editing. DS: Conceptualization, Funding acquisition, Investigation, Methodology, Project administration, Resources, Supervision, Validation, Writing – review & editing.
The author(s) declare that financial support was received for the research and/or publication of this article. The authors would like to acknowledge the funding provided by Bayer Crop Science to conduct this research.
The assistance from the team members of the University of Minnesota Weed Science Lab, North Dakota State University Extension Weed Science Lab, and University of Wisconsin-Madison Cropping Systems Weed Science Lab in this project are greatly appreciated. The authors also acknowledge the help from the University of Minnesota’s Rosemount Research and Outreach Center staff members and Mr. Dave Nicolai in this project.
The authors declare that this study received funding from Bayer Crop Science. While the funder was involved in the study design, they had no role in data collection, analysis, interpretation of data, the writing of the article, or the decision to submit it for publication.
The author(s) declared that they were an editorial board member of Frontiers, at the time of submission. This had no impact on the peer review process and the final decision.
The author(s) declare that no Generative AI was used in the creation of this manuscript.
All claims expressed in this article are solely those of the authors and do not necessarily represent those of their affiliated organizations, or those of the publisher, the editors and the reviewers. Any product that may be evaluated in this article, or claim that may be made by its manufacturer, is not guaranteed or endorsed by the publisher.
Anonymous (2020). U.S. Environmental Protection Agency. Dicamba use on genetically modified dicamba-tolerant (DT) cotton and soybean: Incidents and impacts to users and non-users from proposed registrations. Available online at: https://www.regulations.gov/document/EPA-HQ-OPP-2020-0492-0003 (Accessed August 5, 2024). PC100094, 128931.
Anonymous (2021). U.S. Environmental Protection Agency. Status of over-the-top dicamba: Summary of 2021 usage, incidents and consequences of off-target movement, and impacts of stakeholder-suggested mitigations. Available online at: https://www.regulations.gov/document/EPA-HQ-OPP-2020-0492-0021 (Accessed August 5, 2024). PC128931.
Anonymous (2022). EPA Approves 2022 Minnesota-specific restrictions for dicamba herbicide (Minnesota Crop News. University of Minnesota Extension). Available online at: https://blog-crop-news.extension.umn.edu/2022/03/epa-approves-2022-minnesota-specific.html (Accessed October 20, 2024).
Anonymous (2024a). National Agricultural Statistics Service (NASS), Agricultural Statistics Board, United States Department of Agriculture (USDA). Available online at: https://downloads.usda.library.cornell.edu/usda-esmis/files/j098zb09z/b2775k600/dv141h86n/acrg0624.pdf (Accessed August 5, 2024).
Anonymous (2024b). US Environmental Protection Agency. Revision to February 14, 2024. Existing Stocks Order for Dicamba Products Previously Registered for Overthe-Top Use on Dicamba-Tolerant Cotton and Soybean. Available online at: https://www.epa.gov/system/files/documents/2024-03/revised-dicamba-notice-existing-stocks-order.pdf (Accessed August 5, 2024).
Anonymous (2024c). Warrant Ultra label. Available online at: https://www.cdms.net/ldat/ld0U5004.pdf (Accessed October 20, 2024).
Beckie H. J., Ashworth M. B., Flower K. C. (2019). Herbicide resistance management: Recent developments and trends. Plants 8, 161. doi: 10.3390/plants8060161
Benoit L., Soltani N., Hooker D. C., Robinson D. E., Sikkema P. H. (2019). Control of multiple-resistant waterhemp [Amaranthus tuberculatus (Moq.) Sauer] with preemergence and postemergence herbicides in corn in Ontario. Can. J. Plant Sci. 99, 364–370. doi: 10.1139/cjps-2018-0087
Brooks M. E., Kristensen K., Van Benthem K. J., Magnusson A., Berg C. W., Nielsen A., et al. (2017). glmmTMB balances speed and flexibility among packages for zero-inflated generalized linear mixed modeling. R J. 9, 378–400. doi: 10.32614/RJ-2017-066
Cojocaru O. A., Shamshina J. L., Gurau G., Syguda A., Praczyk T., Pernak J., et al. (2013). Ionic liquid forms of the herbicide dicamba with increased efficacy and reduced volatility. Green Chem. 15, 2110–2120. doi: 10.1039/c3gc37143c
Dhanda S., Kumar V., Manuchehri M., Bagavathiannan M., Dotray P. A., Dille J. A., et al. (2024). Multiple herbicide resistance among kochia (Bassia scoparia) populations in southcentral Great Plains. Weed Sci. 73 (e16), 1–10. doi: 10.1017/wsc.2024.88
Foster D. C., Steckel L. E. (2022). Confirmation of dicamba-resistant Palmer amaranth in Tennessee. Weed Technol. 36, 777–780. doi: 10.1017/wet.2022.87
Gazola T., Costa R. N., Carbonari C. A., Velini E. D. (2022). Dynamics of 2, 4-D and dicamba applied to corn straw and their residual action in weeds. Plants 11, 2800. doi: 10.3390/plants11202800
Hammer C. R., Griffis T. J., Baker J. M., Rice P. J., Frankson L. E., Gunsolus J. L., et al. (2024). Reformulation of dicamba herbicide: Impacts on offsite transport and soybean damage. Agron. J. 116, 2200–2216. doi: 10.1002/agj2.v116.5
Hartig F. (2024). DHARMa: Residual diagnostics for hierarchical (multi-level/mixed) regression models. Available online at: https://CRAN.R-project.org/package=DHARMa (Accessed June 5, 2024).
Heap I. (2024). The International Herbicide-resistant weed database. Available online at: http://www.weedscience.org (Accessed June 10, 2024).
Hedges B. K., Soltani N., Hooker D. C., Robinson D. E., Sikkema P. H. (2018). Control of glyphosate-resistant waterhemp with preemergence herbicides in glyphosate-and dicamba-resistant soybean. Can. J. Plant Sci. 99, 34–39. doi: 10.1139/cjps-2018-0046
Jha P., Franzenburg D., Macvilay I. (2022). Addition of dicamba to preemergence programs for weed control in soybean Vol. 5 (Ames, IA, USA: Iowa State University Research and Demonstration Farms Progress Reports), 13–15.
Jha P., Kumar V., Garcia J., Reichard N. (2015). Tank mixing pendimethalin with pyroxasulfone and chloroacetamide herbicides enhances in-season residual weed control in corn. Weed Technol. 29, 198–206. doi: 10.1614/WT-D-14-00095.1
Johnson B., Young B., Matthews J., Marquardt P., Slack C., Bradley K., et al. (2010). Weed control in dicamba-resistant soybeans. Crop Manage. 9, 1–23. doi: 10.1094/CM-2010-0920-01-RS
Knezevic S. Z., Elezovic I., Datta A., Vrbnicanin S., Glamoclija D., Simic M., et al. (2013). Delay in the critical time for weed removal in imidazolinone-resistant sunflower (Helianthus annuus) caused by application of pre-emergence herbicide. Int. J. Pest Manage. 59, 229–235. doi: 10.1080/09670874.2013.830797
Knezevic S. Z., Pavlovic P., Osipitan O. A., Barnes E. R., Beiermann C., Oliveira M. C., et al. (2019). Critical time for weed removal in glyphosate-resistant soybean as influenced by preemergence herbicides. Weed Technol. 33, 393–399. doi: 10.1017/wet.2019.18
Kumar V., Engel R. P., Currie R., Jha P., Stahlman P. W., Thompson C. (2019). Dicamba-resistant kochia (Bassia scoparia) in Kansas: characterization and management with fall-or spring-applied PRE herbicides. Weed Technol. 33, 342–348. doi: 10.1017/wet.2019.4
Mahoney K. J., Shropshire C., Sikkema P. H. (2014). Weed management in conventional- and no-till soybean using flumioxazin/pyroxasulfone. Weed Technol. 28, 298–306. doi: 10.1614/WT-D-13-00128.1
Mortensen D. A., Ryan M. R., Smith R. G. (2024). Another step on the transgene-facilitated herbicide treadmill. Pest Manage. Sci. 80, 4145–4149. doi: 10.1002/ps.v80.9
Oseland E., Bish M., Lerch R., Bradley K. W. (2024). Atmospheric deposition of dicamba herbicide can cause injury to sensitive soybean. Weed Sci. 72, 422–431. doi: 10.1017/wsc.2024.9
Ou J., Thompson C. R., Stahlman P. W., Jugulam M. (2018). Preemergence application of dicamba to manage dicamba-resistant kochia (Kochia scoparia). Weed Technol. 32, 309–313. doi: 10.1017/wet.2018.1
R Core Team (2024). R: A language and environment for statistical computing (Vienna, Austria: R Foundation for Statistical Computing). Available online at: https://www.R-project.org/ (Accessed June 15, 2024).
Sarangi D., Goplen J., Nicolai D., Peters T., Naeve S., Miller R. (2021). Growth regulator herbicide injury on soybean (Minnesota Crops News, University of Minnesota Extension). Available online at: https://blog-crop-news.extension.umn.edu/2021/08/growth-regulator-herbicide-injury-on.html (Accessed February 10, 2025).
Sarangi D., Jhala A. J. (2019). Palmer amaranth (Amaranthus palmeri) and velvetleaf (Abutilon theophrasti) control in no-tillage conventional (non– genetically engineered) soybean using overlapping residual herbicide programs. Weed Technol. 33, 95–105. doi: 10.1017/wet.2018.78
Schutte B. J., Regnier E. E., Harrison S. K. (2012). Seed dormancy and adaptive seedling emergence timing in giant ragweed (Ambrosia trifida). Weed Sci. 60, 19–26. doi: 10.1614/WS-D-11-00049.1
Shaner D. L. (Ed.) (2014). Herbicide Handbook. 10th ed (Lawrence, KS, USA: Weed Science Society of America).
Silva T. S., Arneson N. J., DeWerff R. P., Smith D. H., Silva D. V., Werle R. (2023). Preemergence herbicide premixes reduce the risk of soil residual weed control failure in corn. Weed Technol. 37, 410–421. doi: 10.1017/wet.2023.45
Singh N., Peters T. J., Miller R. P., Naeve S. L., Sarangi D. (2024). Profile and extent of herbicide-resistant waterhemp (Amaranthus tuberculatus) in Minnesota. Weed Sci. 72, 673–682. doi: 10.1017/wsc.2024.64
Soltani N., Brown L., Sikkema P. H. (2018). Control of glyphosate-resistant common ragweed in corn with preemergence herbicides. Can. J. Plant Sci. 98, 959–962. doi: 10.1139/cjps-2017-0325
Soltani N., Dille J. A., Burke I. C., Everman W. J., VanGessel M. J., Davis V. M., et al. (2017). Perspectives on potential soybean yield losses from weeds in North America. Weed Technol. 31, 148–154. doi: 10.1017/wet.2016.2
Soltani N., Oliveira M. C., Alves G. S., Werle R., Norsworthy J. K., Sprague C. L., et al. (2020). Off-target movement assessment of dicamba in North America. Weed Technol. 34, 318–330. doi: 10.1017/wet.2020.17
Soltani N., Shropshire C., Sikkema P. (2011). Giant ragweed (Ambrosia trifida L.) control in corn. Can. J. Plant Sci. 91, 577–581. doi: 10.4141/cjps2010-004
Striegel S., Oliveira M. C., Arneson N., Conley S. P., Stoltenberg D. E., Werle R. (2021). Spray solution pH and soybean injury as influenced by synthetic auxin formulation and spray additives. Weed Technol. 35, 113–127. doi: 10.1017/wet.2020.89
Keywords: dicamba ruling, herbicide mixture, premix, residual herbicide, resistance management
Citation: Dhanda S, Singh N, Ikley JT, DeWerff RP, Werle R and Sarangi D (2025) Dicamba-based preemergence herbicide tank mixtures improved residual weed control in dicamba-resistant soybean. Front. Agron. 7:1576547. doi: 10.3389/fagro.2025.1576547
Received: 14 February 2025; Accepted: 19 March 2025;
Published: 09 April 2025.
Edited by:
Baruch Rubin, Hebrew University of Jerusalem, IsraelReviewed by:
Sheikh Muhammad Masum, Sher-e-Bangla Agricultural University, BangladeshCopyright © 2025 Dhanda, Singh, Ikley, DeWerff, Werle and Sarangi. This is an open-access article distributed under the terms of the Creative Commons Attribution License (CC BY). The use, distribution or reproduction in other forums is permitted, provided the original author(s) and the copyright owner(s) are credited and that the original publication in this journal is cited, in accordance with accepted academic practice. No use, distribution or reproduction is permitted which does not comply with these terms.
*Correspondence: Debalin Sarangi, ZHNhcmFuZ2lAdW1uLmVkdQ==
Disclaimer: All claims expressed in this article are solely those of the authors and do not necessarily represent those of their affiliated organizations, or those of the publisher, the editors and the reviewers. Any product that may be evaluated in this article or claim that may be made by its manufacturer is not guaranteed or endorsed by the publisher.
Research integrity at Frontiers
Learn more about the work of our research integrity team to safeguard the quality of each article we publish.