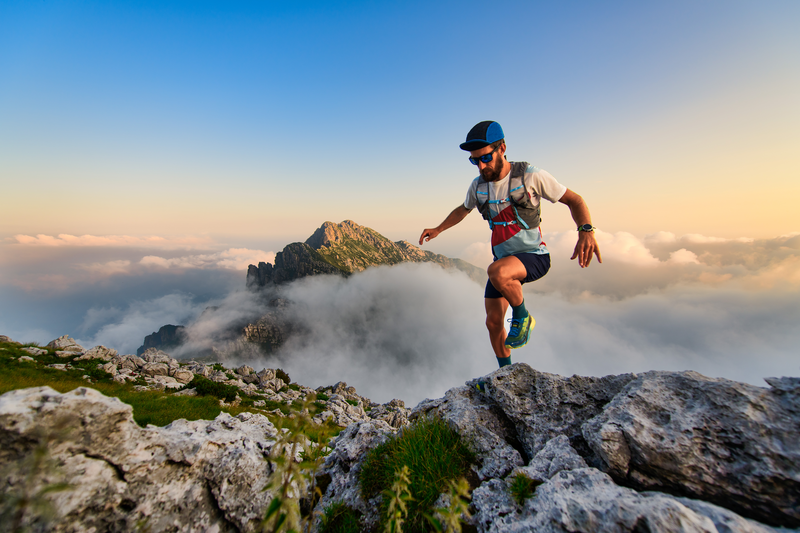
95% of researchers rate our articles as excellent or good
Learn more about the work of our research integrity team to safeguard the quality of each article we publish.
Find out more
ORIGINAL RESEARCH article
Front. Agron. , 25 March 2025
Sec. Weed Management
Volume 7 - 2025 | https://doi.org/10.3389/fagro.2025.1568894
Hazelnut (Corylus avellana L.) plays a crucial role in the agricultural landscape of Oregon's Willamette Valley, where weed and sucker management are labor-intensive and time-consuming endeavors. Current control strategies are either costly but effective, ineffective, or environmentally unfriendly. Tiafenacil, a relatively new herbicide, could be an effective solution. Field studies were conducted in commercial hazelnut orchards across the Willamette Valley to evaluate the efficacy of tiafenacil for weed and hazelnut sucker control. The results confirmed that tiafenacil applied three times per season up to 200 g ai ha-1 did not injure tree trunk or canopy and had no adverse effects on growth parameters, chlorophyll fluorescence, or yield. Tiafenacil at 50 g ai ha-1 outperformed carfentrazone 35 g ai ha-1 in controlling prostrate knotweed (Polygonum aviculare L.), wild carrot (Daucus carota L.), and Canada thistle (Cirsium arvense L. Scop). However, tiafenacil up to 50 g ai ha-1 was less effective than glufosinate 1,050 g ai ha-1 for weed control. Tiafenacil at 50 g ai ha-1 effectively managed suckers comparable to manual removal and with superior efficacy to carfentrazone. Tiafenacil at 50 g ai ha-1 combined with glufosinate or 2,4-D 1,060 g ai ha-1 improved sucker and weed control compared with tiafenacil alone at the same rate, suggesting that its efficacy is enhanced in mixtures. Importantly, tiafenacil exhibited excellent compatibility with 2,4-D and glufosinate, making it a practical option for improving weed and sucker control strategies. For growers, incorporating tiafenacil into their management programs—either as a standalone treatment or in combination with glufosinate or 2,4-D—offers an effective alternative to manual sucker removal while maintaining strong weed control. These findings support tiafenacil as a valuable addition to hazelnut management programs, especially when used in combination with other herbicides for enhanced sucker and weed control without compromising tree health. While no antagonistic effects were observed when tiafenacil was mixed with glufosinate or 2,4-D, further research is necessary to explore potential interactions with other herbicides. Additionally, the economic viability of herbicide combinations should be evaluated before broad adoption.
Oregon leads hazelnut (Corylus avellana L.) production in the United States of America (USA), with over 99% of the nation's orchards located in the Willamette Valley, situated in the western region of the state (Colburn et al., 2015). The cultivation of hazelnuts in Oregon's Willamette Valley is a century-long tradition that strongly emphasizes environmental stewardship and the well-being of the local community and producers (Oregon Hazelnut Industry, 2024). An essential feature of this stewardship is developing new weed management tools with reduced risks to humans and the environment. Paraquat, an important herbicide used for weed and sucker control in hazelnut production systems, has severe adverse impacts on human health (Peachey, 2022; Stuart et al., 2023). According to the most recently available hazelnut-specific pesticide usage data, 3,772 kg of paraquat was applied to hazelnuts in 1999 (at a rate of 648 g ai ha-1, with 2.3 applications per season) (USDA-NASS, 2023). Since then, the hazelnut industry has grown significantly in Oregon, expanding to 34,000 ha by 2020, marking a remarkable 5.9-fold increase (FAOSTAT, 2023). Likely, paraquat use demand for hazelnuts has increased accordingly over the years. Oregon hazelnut growers are committed to and interested in adopting more sustainable production practices (Oregon Hazelnut Industry, 2024), and finding alternatives to paraquat is a priority.
Tiafenacil is a nonselective contact herbicide that controls a wide range of grass and broadleaf weeds (Soltani et al., 2021). Tiafenacil inhibits protoporphyrinogen oxidase (PPO), an essential enzyme that catalyzes the conversion of protoporphyrinogen into protoporphyrin and leads to the production of Mg-proto and Fe-proto, the key precursors to chlorophyll and heme production, respectively (Cha et al., 2022; Park et al., 2018; Takano et al., 2020; Traxler et al., 2023). When PPO is inhibited, protogen leaks out of the chloroplast and accumulates into the cytoplasm, where protogen is rapidly oxidized to proto (Takano et al., 2020; Traxler et al., 2023). Herbicides targeting PPO are fast-acting because the unregulated accumulation of the photodynamic proto in the cytosol leads to the generation of reactive oxygen species (ROS) in the presence of light, causing lipid peroxidation and, ultimately, plant death (Cha et al., 2022; Takano et al., 2020; Traxler et al., 2023). Unlike paraquat and other PPO-inhibiting herbicides, tiafenacil has minimal soil activity, lower toxicity to non-target organisms, and a generally more favorable human health and environmental safety profile (Cha et al., 2022; Hu et al., 2020). Additionaly, currently availabe PPO-inhibiting herbicides have limitations in hazelnut production. For instance, carfentrazone and saflufenacil provide similar sucker control efficacy to glufosinate but are less effective than 2,4-D (de Souza and Moretti, 2020), and they do not control grass weeds.
Currently, herbicides remain the preferred control method among Oregon hazelnut farmers because of the absence of practical alternatives and also because herbicides are the most economically viable option for sucker and weed control. Suckers emerge from adventitious buds on the roots and tree base, requiring repeated control throughout the growing season (Pacchiarelli et al., 2022). In Oregon, growers typically apply herbicides four to eight times annually to suppress sucker growth (de Souza and Moretti, 2020; Hill et al., 2021). Compared to other tree nuts, fruit trees, and vines, hazelnuts produce a significantly higher volume of suckers, making chemical control an essential part of orchard management.
Weeds are undesirable in hazelnut orchards because they compete with trees for water, nutrients, and space, decreasing yield potential and harvest efficiency. According to a study by Kaya-Altop et al. (2016), hazelnut yield decreased by nearly 30% due to weed competition. Additionally, weed competition during critical growth stages increases the mortality of young trees (Mia et al., 2020). Common weeds in hazelnut orchards include Italian ryegrass (Lolium perenne ssp. multiflorum), annual bluegrass (Poa annua), field bindweed (Convolvulus arvensis), Canada thistle (Cirsium arvense), wild carrot (Daucus carota), prostrate knotweed (Polygonum aviculare), and yellow nutsedge (Cyperus esculentus). Many of these species also create management challenges in other perennial cropping systems, such as California orchards, where Italian ryegrass and annual bluegrass are especially difficult to control due to herbicide resistance. Hazelnut growers face the added challenge of balancing effective weed and sucker control while minimizing herbicide impact on tree health and nut yield. The increasing prevalence of herbicide-resistant weeds further complicates weed management in hazelnuts. Italian ryegrass and annual bluegrass have evolved resistance to multiple herbicide modes of action (Heap, 2024; Moretti, 2021). Although resistance to PPO-inhibiting herbicides has been reported in 15 species worldwide, no resistance to PPO-inhibiting herbicides in any weed across the western USA has been reported (Heap, 2024). The only known case of PPO-inhibitor (oxufluorfen) resistance in tree nut orchards was reported in Lolium rigidum in Spanish olive groves. This makes PPO-inhibiting herbicides, such as tiafenacil, an appealing weed control option for the hazelnut industry. Tiafenacil has demonstrated effective broadleaf and grass weed control in vineyards, where similar management challenges exist (Laguerre et al., 2024). Prior to this research, no information was available on tree nut tolerance to tiafenacil, its use in sucker management, or its broader weed control efficacy. The objectives of this research were (1) to evalute the effectiveness of tiafenacil for weed control; (2) to test its potential as an alternative or supplement for hazelnut sucker control compared to current benchmarks; and (3) to document hazelnut tolerance to repeated basal-directed applications of tiafenacil. This research helped support the registration fo tiafenacil for hazelnut.
Field studies were conducted to evaluate hazelnut tolerance to and weed and hazelnut sucker control by tiafenacil in commercial hazelnut orchards (Table 1). All studies were arranged as randomized complete block designs with four replicates. Herbicide treatments were applied using a CO2 pressurized backpack sprayer equipped with a spray boom containing three AI-11002 nozzles (TeeJet Technologies, Glendale Heights, IL) spaced at 50 cm and calibrated to deliver 187 L ha-1 at 275 kPa at 4.8 km h-1. Treatments were applied as a single pass on each side of the trees, covering a 1.5-m swath. This configuration ensured uniform coverage of weeds and suckers (Miranda and Moretti, 2025; de Souza and Moretti, 2020). Two rows were left as borders around each study area to avoid border effects.
Table 1. List of herbicides, rates, and adjuvants applied in studies evaluating weed control, short-term hazelnut sucker control, hazelnut tolerance and long-term sucker control.
Two field studies were conducted, one in 2022 near Monroe (44.29, -123.23) and one in 2023 near Corvallis (44.56, -123.22), OR (Table 1). The Monroe study was conducted in a commercial one-year-old rainfed hazelnut orchard planted with the cv. ‘PollyO’ at 6.1 by 6.1 m planting configuration. The soil type was a Chehalis silty clay loam (fine-silty, mixed, superactive, mesic Cumulic Ultic Haploxerolls) (USDA-NRCS, 2023). The Corvallis study was conducted in a fallow rainfed area on a Willamette silt loam soil (fine-silty, mixed, superactive, mesic Pachic Ultic Argixerolls) (USDA-NRCS, 2023). The Monroe site was infested with prostrate knotweed, whereas prostrate knotweed, wild carrot, and Canada thistle infested the Corvallis site. Weed growth stages were recorded using the BBCH scale. At the initiation of the 2022 study, prostrate knotweed was 20 to 25 cm tall (BBCH-60), while in the 2023 study, prostrate knotweed was 10 to 18 cm tall (BBCH 39), Canada thistle was 25 to35 cm tall (BBCH-19), and wild carrot was 8 to15 cm tall (BBCH-19). Plant growth stages were recorded following the BBCH scale for standardization (Hess et al., 1997). Areas with consistent weed density were selected, and each field was scouted beforehand to ensure high weed density within the experimental area.
Nine treatments were tested, including tiafenacil at 25, 50, and 75 g a.i. ha-1, carfentrazone at 35 g a.i. ha-1, glufosinate at 1,150 g a.i. ha-1, and 2,4-D at 1,060 g a.i. ha-1, and the combination of tiafenacil at 50 g a.i. ha-1 with glufosinate or 2,4-D (Table 1). A nontreated control was included for comparison with herbicide treatments. Plot size was 3 m by 12.2 m.
Assessments included visual estimates of weed control by weed species, aboveground green area coverage, and aboveground biomass, with species-specific separation. Weed control was rated on a scale from 0 to 100%, where 0% represents no control and 100% represents complete control. For aboveground green area coverage, two random photos were taken in each plot from a height of 1.5 meters using an iPhone 14 (Apple Inc., Cupertino, CA). These photos were processed using Canopeo software (Patrignani and Ochsner, 2015) to estimate green area coverage based on green pixels. In Canopeo, the settings included a foliage type of ‘cover crops,’ with a red-to-green ratio of 0.95, a blue-to-green ratio of 0.60, and a minimum excess green of 20. Higher weed aboveground green area coverage indicated greater weed density and healthier weeds. Weed control and weed aboveground green area coverage measurements were taken 14, 28, and 35 days after treatment (DAT). At 35 DAT, aboveground biomass was harvested from two randomly placed 0.25-m2 quadrats per plot. Weeds were separated by species, dried, and then weighed.
Two field studies were conducted in 2022 and 2023 in commercial hazelnut orchards near Keizer (45.06, -123.08) and Corvallis (44.63, -123.19), OR (Table 1). Both studies involved one-year-old drip-irrigated hazelnut orchards planted with cv. ‘PollyO’ at 3 m by 6.1 m planting configuration. The Keizer site had Amity silt loam soil (fine-silty, mixed, superactive, mesic Aquultic Haploxerolls) (USDA-NRCS, 2023), while the Corvallis site had Willamette silt loam soil (fine-silty, mixed, superactive, mesic Pachic Ultic Argixerolls) (USDA-NRCS, 2023). The study consisted of two herbicide applications, 28 d apart, directed to the base of the trees and targeting hazelnut suckers. Treatments were similar to those of the weed control study with the addition of a manual removal of suckers as a reference, resulting in ten treatments. Each plot included three to four trees that were treated as sub-replicates. At the start of the study, suckers were 25 to 40 cm long in Keizer and 15 to 25 cm long in Corvallis. Sucker control was visually assessed on a scale from 0% (no control) to 100% (complete control) at 14, 28, 42, and 56 days after the initial treatment (DAIT), based on the percentage reduction in sucker growth compared to the nontreated control. Sucker length was estimated by randomly selecting three suckers per tree and measuring their length from base to tip. Sucker fresh biomass and cross-sectional area at the base of the sucker were assessed at 56 DAIT. The cross-sectional area was determined by measuring the base diameter of five suckers per tree (15 to 20 per plot) with a digital caliper (Fisherbrand™ Traceable™ Digital Calipers; Thermo-Fischer Scientific, Waltham, WA) and applying the formula:
Where A is the cross-sectional area, r is the radius, and π is a constant (3.14159). All hazelnut suckers from each tree in each plot were hand-clipped at the soil surface, counted, and fresh weight was recorded. Reduction in sucker length, cross-sectional area, and fresh biomass was calculated using Equation 2 below:
where NT represents the mean sucker length, cross-sectional area, or fresh weight in the nontreated control, and Trt represents the corresponding mean values in the treated plants.
Two multi-year field studies were initiated in the spring of 2022. The first study was near Amity (45.14, -123.27), OR (Table 1). The site was planted with cv. ‘McDonald’ and ‘Wepster’ in January 2019 with a 3 m by 3 m spacing and irrigated via surface drip, following the grower’s irrigation schedule. The soil was an Amity silt loam soil (fine-silty, mixed, superactive, mesic Aquultic Haploxerolls) (USDA-NRCS, 2023). The second study was located near Hubbard (45.20, -122.85), OR, in a rainfed field planted in November 2020 with ‘PollyO’ cv. and a planting configuration of 3 m by 6.1 m. The soil was a Donald loam soil (fine, smectitic Abruptic Argicryolls) (USDA-NRCS, 2023). The study consisted of tiafenacil applied at three rates equivalent to the reference rate (50 g ai ha-1), two- and four-times the reference rate. A combination treatment of tiafenacil at 50 g ai ha-1 with glufosinate was included. Glufosinate and a nontreated control were included for comparison. Each treatment was applied four times to the same trees each year over two years. In the first year, applications began in April 2022, wiht the second and third application timings spaced 28-d apart. A fourth application was made in November 2022, during the dormant season, which is the time when growers typically apply postemergence herbicides in conjunction with preemergence herbicides to manage winter annual weeds. In the second year, applications resumed in May 2023, following the same 28-day interval for the second and third applications, and concluded with a fourth application in November 2023 during dormancy. The applications were directed to the base of the trees, targeting hazelnut suckers.
Hazelnut growth in response to tiafenacil basal applications was based on the main trunk cross-sectional area (TCSA) recorded at 0.5 m above ground, which was calculated by transforming the caliper diameter using Equation 1; and the volume of the canopy, measured as width at the widest points both parallel and perpendicular to the plant row, as well as its depth (Hill et al., 2021; Pedroso and Moretti, 2022a, b). All trees in each plot were measured similarly before the initiation of the studies and at 300 and 650 DAIT. Relative changes in TCSA and canopy volume were calculated to assess growth over the course of the study at each location. Visual estimates of injury to the trunk and canopy were recorded every 28 DAIT until the dormant period. Photosynthetic performance and stress were evaluated by measuring the maximum quantum yield of PS-II (Fv/Fm) in the trunk at 100 and 450 DAIT using a portable chlorophyll fluorometer (OS1p, Opti-Sciences Inc., NH) at 0.5 m above ground during the early morning hours (between 6:00-9:00 am local time) after a 20-min dark adaptation (Johnstone et al., 2012). Additionally, the quantum yield efficiency of PS-II electron transport in the presence of light, [Y(II)] (Murchie and Lawson, 2013), was measured on two sun-exposed, newly expanded leaves per tree at 100 and 450 DAIT, using the same portable chlorophyll fluorometer used for the Fv/Fm assessments.
Since the trees in the Hubbard study were in their juvenile stage, only the trees in the Amity study were evaluated for hazelnut yield. Nuts that had fallen from all trees in a plot were collected using a push-behind harvester (Bag-a-Nut, Jacksonville, FL). These hazelnuts were subsequently oven-dried at 56°C until they reached a consistent moisture content of 6% before being weighed. Yield per tree is reported and was calculated by dividing the total yield per plot, at 6% moisture, by the total number of trees harvested in the plot. In addition, 200 nuts from each plot in each year of the study were assessed for kernel percentage and kernel quality. Kernel percentage was determined by weighing the kernels obtained from the 200 nuts and dividing that by the weight of the 200 nuts in each plot. Kernel quality was evaluated by sorting kernels into classifications, including blanks (shells without kernels), brown stain (shells and kernels turning into a soft, brown, watery mass), poorly filled nuts, twins, moldy (contaminated with fungus), and kernels with black tips (USDA, 2023, 2016). Kernels were considered marketable if they did not exhibit any of these defects.
To evaluate the efficacy of tiafenacil for sucker control, we visually estimated sucker control and measured sucker length at three specific time points: 28, 56, and 84 DAIT during each year of the studies at each site. Additionally, at the 84 DAIT date, we determined the fresh biomass of the hazelnut suckers. Hazelnut sucker length and sucker control were estimated as previously described in the short-term hazelnut sucker control study. The determination of hazelnut sucker fresh biomass followed the same procedures as outlined in the short-term hazelnut sucker control study. For brevity, only data collected at 84 DAIT is presented; additional data is available as supplementary information.
All data were analyzed using R software version 4.2.2 (R Core Team, 2022) and underwent analyses of variance with a significance level set at alpha=0.05. Fixed effects included evaluation timing, study site, treatments, and interactions, while experimental blocks were treated as random effects. Percentage-based data, which included weed control and aboveground green area coverage, weed biomass reduction, hazelnut sucker control, hazelnut sucker length and fresh biomass reduction, hazelnut tree canopy and trunk injury, the change over time in tree growth parameters (TCSA and tree canopy volume), frequency of marketable kernel, and kernel percentage were transformed using beta-transformation, scaling data from 0 to 1. These beta-transformed data were then analyzed using the 'glmmTMB' package (version 1.1.7) with a generalized linear mixed model employing a beta error distribution (Brooks et al., 2017). Weed biomass reduction, sucker control, sucker length, and fresh biomass reduction for the nontreated control were not included in the analysis, given that values were zero. For Fv/Fm values, Y(II), and hazelnut yield, a linear mixed-effect model was applied with the ‘lmer’ function in the ‘lme4’ package (v.1.1–32) (Bates et al., 2020). When significant treatment and/or interaction effects were detected, post hoc comparisons of means were conducted using Tukey's HSD method with the assistance of the 'emmeans' package (version 1.8.5) and the 'cld' function (Lenth, 2020).
Each weed control study site was analyzed separately due to a significant (P<0.05) treatment-by-site interaction. Likewise, short-term hazelnut sucker control studies underwent separate analyses because of the significant (P<0.05) treatment-by-site interactions across most evaluation timings and to simplify interpretation. The hazelnut tolerance and long-term sucker control studies were also analyzed separately, as significant (P<0.05) treatment-by-site interactions were observed (considering also differences in cultivars and tree age). Additionally, evaluation timings within these studies were analyzed independently because the interaction between evaluation timing and treatment was statistically significant at the P<0.05 level.
In the Monroe study, tiafenacil applied at 12.5, 25, and 50 g ha-1 controlled prostrate knotweed by 48% to 69% at 35 DAT (Table 2). While the 50 g ha-1 rate provided greater prostrate knotweed control, it did not reduce weed green area coverage or biomass more effectively than the 12.5 or 25 g ha-1 rates. Tiafenacil at 50 g ha-1 (69% control) outperformed carfentrazone at 35 g ha-1 (37% control), reducing weed green area coverage and biomass by up to 25% and 6% over carfentrazone, respectively. Tiafenacil at 50 g ha-1 provided similar prostrate knotweed control and biomass reduction as 2,4-D alone at 1,060 g ha-1. Glufosinate at 1,150 g ha-1 and the combination of glufosinate + tiafenacil at 50 g ha-1 were the most effective, providing the greatest control and the greatest reductions in green area coverage and biomass.
Table 2. Prostrate knotweed control, aboveground green area coverage, and biomass reduction at 35 d after treatment at Monroe. 1, 2.
In the Corvallis study, glufosinate at 1,150 g ha-1 and the combination of glufosinate + tiafenacil at 50 g ha-1 provided the greatest control of prostrate knotweed, wild carrot, and Canada thistle (Table 3). Tiafenacil at 50 g ha-1 controlled prostrate knotweed (65%) and Canada thistle (46%) better than carfentrazone (15-26%). Prostrate knotweed biomass reductions ranged from 64 to 95% across treatments, with no significant difference among herbicides. Tiafenacil at 50 g ha-1 plus 2,4-D increased Canada thistle control by 27% compared to tiafenacil alone, but did not improve the performance of glufosinate or 2,4-D for the other weed species.
Table 3. Weed control, weed aboveground green area coverage, and weed biomass reduction at 35 d after treatment at Corvallis.1, 2.
Glufosinate and glufosinate plus tiafenacil at 50 g ha-1 provided greatest and most consistent control of hazelnut suckers across all the evaluation timings in the Keizer study (Supplementary Figure S1). Tiafenacil at 25 and 50 g ha-1 provided similar hazelnut sucker control as glufosinate alone at 56 DAIT (Figure 1A). Manual sucker removal was ineffective over time because of ample regrowth (Supplementary Figure S2). All herbicide treatments resulted in control levels comparable to manual removal. No significant treatment effects were observed in reducing sucker length or fresh biomass (Figures 1C, E). However, glufosinate alone and in combination with tiafenacil at 50 g ha-1 effectively reduced sucker cross-sectional area, performing similarly to most treatments except for carfentrazone and tiafenacil at 25 g ha-1.
Figure 1. Hazelnut sucker control (A, B), sucker length reduction (C, D), sucker biomass reduction (E, F), and cross-sectional area (G, H) reduction relative to the nontreated control (NTC) at 56 d after initial treatment in the short-term hazelnut sucker control studies conducted in Keizer (left column) and Corvallis (right column). Means sharing the same letter within plots are not statistically different at a significance level of P=0.05, as determined by Tukey's HSD test. Average sucker lengths in the NTC were 34.4 cm in Keizer and 38.9 cm in Corvallis. The average fresh biomass per sucker in the NTC was 68.4 g in Keizer and 47.8 g in Corvallis, while the average cross-sectional area averaged 0.35 cm2 in Keizer and 0.33 cm2 in Corvallis. All treatments included ammonium sulfate at 10 g L-1 and methylated seed oil at 8.9 g L-1, except for the manual removal and the NTC. ns, non-significant; NTC, nontreated control.
In the Corvallis study, tiafenacil at 50 g ha-1 plus glufosinate provided the greatest sucker control across all dates, consistently outperforming carfentrazone (Figure 1B; Supplementary Figure S1). Tiafenacil at this rate was as effective as glufosinate alone, 2,4-D, and manual sucker removal by 56 DAIT. Combining tiafenacil at 50 g ha-1 with 2,4-D enhanced control compared to 2,4-D alone at 42 DAIT only (Supplementary Figure S1). Visual estimates of control correlated with reductions in sucker length, biomass, and cross-sectional area, highlighting the effectiveness of these treatments (Figure 1). Tiafenacil at 12.5 g ha-1 rate also surpassed carfentrazone in reducing sucker length. Overall, herbicide treatments generally matched the performance of manual removal in reducing sucker length and biomass, except for carfentrazone.
None of the herbicide treatments caused injury to the trunk or canopy of the tree across all sites and years, even when tiafenacil was applied at 2–4 times the recommended rate (Supplementary Figure S3F). As a result, injury data were excluded due to a lack of variation. Tree growth, chlorophyll fluorescence, and hazelnut yield showed no significant differences among herbicide treatments, including high rates of tiafenacil (Table 4; Supplementary Figure S3).
Table 4. Growth parameters measured from the initiation of the hazelnut tolerance and long-term sucker control studies through 650 days after treatment, along with yield and quality parameters, conducted at Amity and Hubbard, OR.
In the Amity study, hazelnut canopy volume increased by 256% to 318% (9.3-11.1 m3) from the initiation of the study to 650 DAIT (Table 4). In the Hubbard study, where trees were younger, canopy volume grew by 23,000% to 39,300% (3.9-4.4 m3) at 650 DAIT. TCSA growth in the Hubbard study showed that nontreated controls had 17% to 29% less growth (9.9 cm2) compared to herbicide-treated trees (14 cm2) by 650 DAIT (Table 4). Chlorophyll fluorescence (Fv/Fm and Y(II) ratios) showed no stress effects from herbicides (Supplementary Figure S3). Hazelnut yields in 2022 ranged from 0.49 to 0.68 kg tree-1, with over 92% of kernels being marketable. In 2023, yields increased to 2.27–3.04 kg tree-1, with over 96% marketable kernels (Table 4). These results demonstrate that tiafenacil, even at higher rates, did not negatively impact hazelnut tree health, growth, or productivity.
In the Amity study, herbicide treatments achieved less than 80% hazelnut sucker control at 28 DAIT, showing that a single application is insufficient due to rapid regrowth (Supplementary Table S1). Tiafenacil at 50 g ha-1 provided 70-84% sucker control, similar to the 100 g ai ha-1 rate, but the 200 g ai ha-1 rate improved control by 84 DAIT (Table 5). Tiafenacil at 50 g ha-1 reduced sucker length by 64-65% and biomass by 86-90%, comparable to glufosinate alone. The combination of tiafenacil at 50 g ha-1 with glufosinate resulted in the greatest biomass reduction in 2023 (93%) but showed no added benefit over tiafenacil alone in 2022.
Table 5. Hazelnut sucker control, length and fresh biomass reduction at 84 d after initial treatment from the hazelnut tolerance and long-term sucker control studies conducted in Amity and Hubbard in 2022 and 2023.1, 2.
In the Hubbard study, herbicide treatments provided less than 66% sucker control at 28 DAIT, but control improved with additional applications (Supplementary Table S2). Tiafenacil at 50 g ha-1 (85-88%) was as effective as glufosinate alone in 2022 and 2023 (Table 5). By 84 DAIT in 2023, tiafenacil’s effectiveness was similar across all rates. Adding glufosinate to tiafenacil at 50 g ai ha-1 improved sucker control in 2022 (97%) but not in 2023 (92%) compared to tiafenacil alone. Tiafenacil at 50 g ha-1 reduced sucker length by 67-79%, and in 2023, combining it with glufosinate achieved the greatest reduction (72%). Biomass reduction was similar across all herbicide treatments in 2022, but in 2023, tiafenacil alone at 50 g ha-1 reduced biomass by 89%, increasing to 95% with glufosinate.
Tiafenacil at 50 g ha-1 outperformed carfentrazone in controlling prostrate knotweed and Canada thistle, effectively reducing weed green area coverage and biomass. Its performance was comparable to that of 2,4-D but fell short of glufosinate, which provided the most effective weed control. Similar findings were reported by Soltani et al. (2021) and Westerveld et al. (2021), who noted tiafenacil’s limited effectiveness when used alone to control glyphosate-resistant horseweed (Conyza canadensis L.). The combination of tiafenacil at 50 g ha-1 with glufosinate or 2,4-D did not consistently enhace the control of prostrate knotweed, wild carrot, or Canada thistle compared to individual herbicides. However, Miranda and Moretti (2024) suggested that combinations of tiafenacil (at rates of 50 or 75 g ha-1) with glufosinate at 1,150 g ha-1 or clethodim 135 g ha-1 could improve the control of herbicide-resistant Italian ryegrass in hazelnut. Their study showed that mixtures of tiafenacil and glufosinate increased Italian ryegrass control by 24–43% and reduced inflorescence weight by 15–34%, indicating synergistic effects. It is worth noting that we observed prostrate knotweed and Canada thistle regrowth during the final evaluation period across all herbicide treatments, underscoring the need for multiple, integrated control strategies.
While tiafenacil at 50 g ha-1 did not match glufosinate in overall weed control, it performed comparably to glufosinate and better than carfentrazone for short-term hazelnut sucker control. Lower rates of tiafenacil (12.5 and 25 g ha-1) also outperformed carfentrazone, though the 12.5 g rate should be avoided for sucker control, as the exposure of nearby weeds to sublethal doses could promote herbicide resistance, as reported by Benedetti et al. (2020). This is particularly concerning in areas closest to the trees, where repeated exposure is most likely. Diversifying herbicide modes of action and incorporating rotation strategies are essential to mitigate resistance risks, particularly in these zones closest to the trees, where repeated exposure is most likely. Tiafenacil at 50 g ha-1, either alone or combined with glufosinate or 2,4-D performed similarly to manual removal. Similar findings have been observed in other crops, like peach, where herbicides proved as effective as manual removal (Muro and Luri, 1990). However, in hazelnuts, de Souza and Moretti (2020) found that while glufosinate, 2,4-D, and paraquat were effective for sucker control, manual removal was more consistent in reducing sucker length. Despite its effectiveness, manual removal is labor-intensive and costly, making multiple herbicide applications a more economical alternative.
Tiafenacil at 50, 100, and 200 g ha-1 caused no injury to hazelnut trees and had no adverse effects on growth, chlorophyll fluorescence, or yields, meeting the Oregon hazelnut industry’s crop safety standards. Trees in the nontreated control group showed the lowest TCSA growth over 650 days, indicating that suckers hinder tree growth and emphasizing the need for effective sucker management. Similar findings were reported by Miranda and Moretti (2025), where young hazelnut trees in the nontreated control exhibited lower TCSA and canopy volume growth compared to herbicide-treated trees.
Tiafenacil at 50 g ha-1, applied in three consecutive treatments, was as effective as glufosinate in controlling hazelnut suckers. While the addition of glufosinate to tiafenacil at 50 g ha-1 did not consistently enhance sucker control, the combination of both herbicides has the potential to reduce the evolution of herbicide-resistant weeds and expand the spectrum of weed control (Norsworthy et al., 2012; Soltani et al., 2021). Growers often prefer combining herbicides that offer broad-spectrum control in a single application to reduce labor and fuel costs. Additionally, tiafenacil provides an opportunity to rotate herbicide modes of action, ensuring compliance with maximum allowable per-season application rates and mitigating the risk of herbicide-resistant weed evolution. This makes tiafenacil a valuable addition to integrated weed and sucker management in hazelnut orchards.
Since tiafenacil is less effective than glufosinate, glufosinate will likely remain the preferred choice for growers. However, tiafenacil can be effectively used in herbicide rotations or mixtures to reduce the risk of resistance selection. Applying tiafenacil with other herbicides and targeting younger weeds might improve its efficacy (Miranda and Moretti, 2024). However, repeated use of tiafenacil can select for resistant individuals, so growers should avoid overrelying on any single herbicide. While tiafenacil may offer certain advantages over paraquat, further research is needed to fully understand its long-term effects on crop safety and the environment before recommending it as a replacement for paraquat.
In summary, the results showed that: (1) tiafenacil applied alone may not be as effective as current benchmarks for weed control, like glufosinate, but it outperformed carfentrazone and could serve as a potential substitute for paraquat. (2) tiafenacil at 50 g ha-1 effectively controlled hazelnut suckers, performing similarly to the current standards, glufosinate and 2,4-D. Moreover, tiafenacil at 50 g ha-1 outperformed carfentrazone performance for sucker control. (3) tiafenacil is a safe herbicide for hazelnuts, causing no tree injury or negative impacts on growth parameters, chlorophyll fluorescence, or yield. Additionally, tiafenacil demonstrated excellent compatibility with both 2,4-D and glufosinate, suggesting it can be mixed with a variety of herbicides to enhance both weed and sucker control. This expands the weed control spectrum while ensuring the safety of hazelnut trees and growers. Furthermore, tiafenacil allows growers to rotate herbicide modes of action, ensuring compliance with maximum per-season application rates and reducing the risk of herbicide-resistant weed evolution. Future research should focus on evaluating tiafenacil’s full spectrum of weed control, its performance in various environmental conditions, and its integration with non-chemical management methods. Additionally, exploring its application in other tree orchards could help expand its use across different tree crop systems.
The raw data supporting the conclusions of this article will be made available by the authors, without undue reservation.
JM: Conceptualization, Data curation, Formal Analysis, Investigation, Methodology, Project administration, Software, Visualization, Writing – original draft, Writing – review & editing. MM: Conceptualization, Funding acquisition, Project administration, Resources, Supervision, Writing – review & editing.
The author(s) declare that financial support was received for the research and/or publication of this article. This study received financial support from the Oregon Hazelnut Commission, the Inter-Regional Project 4, and the Ferrero Hazelnut Company. The funders were not involved in the study design, data collection, analysis, interpretation, writing of the article, or decision to submit it for publication.
We acknowledge the growers who graciously provided space within their orchards to conduct this research.
The authors declare that the research was conducted in the absence of any commercial or financial relationships that could be construed as a potential conflict of interest.
The author(s) declare that no Generative AI was used in the creation of this manuscript.
All claims expressed in this article are solely those of the authors and do not necessarily represent those of their affiliated organizations, or those of the publisher, the editors and the reviewers. Any product that may be evaluated in this article, or claim that may be made by its manufacturer, is not guaranteed or endorsed by the publisher.
The Supplementary Material for this article can be found online at: https://www.frontiersin.org/articles/10.3389/fagro.2025.1568894/full#supplementary-material
Bates D., Maechler M., Bolker B., Walker S., Christensen R., Singmann H., et al. (2020). Package “lme4”: Linear Mixed-Effects Models using “Eigen” and S4, version 1.1-26. Available online at: https://CRAN.R-project.org/package=lme4 (Accessed March 21, 2024).
Benedetti L., Rangani G., Ebeling Viana V., Carvalho-Moore P., Merotto A., Rabaioli Camargo E., et al. (2020). Rapid reduction of herbicide susceptibility in junglerice by recurrent selection with sublethal doses of herbicides and heat stress. Agronomy 10, 1761. doi: 10.3390/agronomy10111761
Brooks M. E., Kristensen K., van Benthem K. J., Magnusson A., Berg C. W., Nielsen A., et al. (2017). glmmTMB balances speed and flexibility among packages for zero-inflated generalized linear mixed modeling. R J. 9, 378–400. doi: 10.32614/RJ-2017-066
Cha J. Y., Shin G. I., Ahn G., Jeong S. Y., Ji M. G., Alimzhan A., et al. (2022). Loss-of-function in GIGANTEA confers resistance to PPO-inhibiting herbicide tiafenacil through transcriptional activation of antioxidant genes in Arabidopsis. Appl. Biol. Chem. 65, 66. doi: 10.1186/s13765-022-00734-6
Colburn B. C., Mehlenbacher S. A., Sathuvalli V. R., Smith D. C. (2015). Eastern filbert blight resistance in hazelnut accessions ‘Culplà’, ‘Crvenje’, and OSU 495.072. J. Am. Soc Hortic. Sci. 140, 191–200. doi: 10.21273/JASHS.140.2.191
de Souza L. L., Moretti M. L. (2020). Chemical control of suckers in hazelnut orchards of western Oregon. Weed Technol. 34, 863–869. doi: 10.1017/wet.2020.78
FAOSTAT (2023). Crops and livestock products. Available online at: https://www.fao.org/faostat/en/data/QCL (Accessed March 21, 2024).
Heap I. (2024). The international herbicide-resistant weed database. Available online at: http://www.weedscience.org/ (Accessed March 21, 2024).
Hess M., Barralis G., Bleiholder H., Buhr L., Eggers Th., Hack H., et al. (1997). Use of the extended BBCH scale—general for the description of growth stages of monocotyledonous and dicotyledonous weed species. Weed Res. 37, 433–441. doi: 10.1046/j.1365-3180.1997.d01-57.x
Hill R. J., King D. R., Zollinger R., Moretti M. L. (2021). 1-Naphthaleneacetic acid (NAA) reduces sucker growth in European hazelnut (Corylus avellana L.). HortScience 56, 1594–1598. doi: 10.21273/HORTSCI16176-21
Hu M., Tan H., Li Y., Qiu J., Liu L., Zeng D. (2020). Simultaneous determination of tiafenacil and its six metabolites in fruits using ultra-high-performance liquid chromatography/tandem mass spectrometry. Food Chem. 327, 127015. doi: 10.1016/j.foodchem.2020.127015
Johnstone D., Tausz M., Moore G., Nicolas M. (2012). Chlorophyll fluorescence of the trunk rather than leaves indicates visual vitality in Eucalyptus saligna. Trees 26, 1565–1576. doi: 10.1007/s00468-012-0730-7
Kaya-Altop E., Haghnama K., Sarıaslan D., Phillippo C. J., Mennan H., Zandstra B. H. (2016). Long-term perennial weed control strategies: economic analyses and yield effect in hazelnut (Corylus avellana). Crop Prot. 80, 7–14. doi: 10.1016/j.cropro.2015.10.022
Laguerre G., Contreras A., Hanson B. D. (2024). Evaluation of weed control efficacy and crop safety of the PPO-inhibiting herbicide tiafenacil in California orchard cropping systems. Weed Technol. 38, e46. doi: 10.1017/wet.2024.46
Lenth R. (2020). emmeans: Estimated marginal means, aka least-squares means. R package version 1.5.1. Available online at: https://CRAN.R-project.org/package=emmeans (Accessed March 21, 2024).
Mia M. J., Massetani F., Murri G., Facchi J., Monaci E., Amadio L., et al. (2020). Integrated weed management in high-density fruit orchards. Agronomy 10, 1492. doi: 10.3390/agronomy10101492
Miranda J. W. A., Moretti M. L. (2024). Improved management of Italian ryegrass (Lolium perenne ssp. multiflorum) with mixtures of tiafenacil and ACCase or glutamine synthetase inhibitors. Weed Sci. 73, e11. doi: 10.1017/wsc.2024.93
Miranda J. W. A., Moretti M. L. (2025). Limited efficacy of florpyrauxifen-benzyl alone for Lolium perenne L. ssp. multiflorum and Poa annua L. control: the need for herbicide combinations in hazelnut (Corylus avellana L.) orchard management. Weed Res. 65, e70001. doi: 10.1111/wre.70001
Moretti M. L. (2021). POST control of Italian ryegrass in hazelnut orchards. Weed Technol. 35, 638–643. doi: 10.1017/wet.2021.41
Murchie E. H., Lawson T. (2013). Chlorophyll fluorescence analysis: a guide to good practice and understanding some new applications. J. Exp. Bot. 64, 3983–3998. doi: 10.1093/jxb/ert208
Muro J., Luri E. (1990). “Initial control and subsequent regrowth inhibition of plum suckers in a peach orchard,” in Proceedings of la Reunion de la Sociedad Espanola de Malherbología (Sociedad Espannola de Malherbología, Madrid, Spain), 179–187.
Norsworthy J. K., Ward S. M., Shaw D. R., Llewellyn R. S., Nichols R. L., Webster T. M., et al. (2012). Reducing the risks of herbicide resistance: best management practices and recommendations. Weed Sc. 60, 31–62. doi: 10.1614/WS-D-11-00155.1
Oregon Hazelnut Industry (2024). Oregon Hazelnut Stewardship Program. Available online at: https://www.hazelnutstewardship.org/ (Accessed March 21, 2024).
Pacchiarelli A., Silvestri C., Cristofori V. (2022). Advances in sucker control for sustainable European hazelnut (Corylus avellana L.) cultivation. Plants 11, 3416. doi: 10.3390/plants11243416
Park J., Ahn Y. O., Nam J. W., Hong M. K., Song N., Kim T., et al. (2018). Biochemical and physiological mode of action of tiafenacil, a new protoporphyrinogen IX oxidase-inhibiting herbicide. Pestic. Biochem. Physiol. 152, 38–44. doi: 10.1016/j.pestbp.2018.08.010
Patrignani A., Ochsner T. E. (2015). Canopeo: A powerful new tool for measuring fractional green canopy cover. Agron. J. 107, 2312–2320. doi: 10.2134/agronj15.0150
Peachey E. Ed. (2022). Pacific Northwest Weed Management Handbook (Corvallis, OR: Oregon State University). Available online at: https://pnwhandbooks.org/weed (Accessed March 21, 2024).
Pedroso R. M., Moretti M. L. (2022a). Hazelnut tolerance to basal-directed applications of clopyralid and quinclorac. Weed Technol. 36, 576–582. doi: 10.1017/wet.2022.60
Pedroso R. M., Moretti M. L. (2022b). Hazelnut growth and weed control in response to selected preemergence herbicides. Weed Technol. 36, 570–575. doi: 10.1017/wet.2022.58
R Core Team (2022). R: A Language and Environment for Statistical Computing (Vienna, Austria: R Foundation for Statistical Computing).
Soltani N., Shropshire C., Sikkema P. H. (2021). Control of glyphosate-resistant horseweed (Conyza canadensis) with tiafenacil mixes in corn. Weed Technol. 35, 908–911. doi: 10.1017/wet.2021.44
Stuart A. M., Merfield C. N., Horgan F. G., Willis S., Watts M. A., Ramírez-Muñoz F., et al. (2023). Agriculture without paraquat is feasible without loss of productivity: Lessons learned from phasing out a highly hazardous herbicide. Environ. Sci. pollut. Res. 30, 16984–17008. doi: 10.1007/s11356-022-24951-0
Takano H. K., Beffa R., Preston C., Westra P., Dayan F. E. (2020). Glufosinate enhances the activity of protoporphyrinogen oxidase inhibitors. Weed Sci. 68, 324–332. doi: 10.1017/wsc.2020.39
Traxler C., Gaines T. A., Küpper A., Luemmen P., Dayan F. E. (2023). The nexus between reactive oxygen species and the mechanism of action of herbicides. J. Biol. Chem. 299, 105267. doi: 10.1016/j.jbc.2023.105267
USDA (2016). Filbert/Hazelnut kernels and filberts in the shell-inspection instruction. Available online at: https://www.ams.usda.gov/sites/default/files/media/FilbertInspectionInstructions.pdf (Accessed March 21, 2024).
USDA (2023). Filberts in the shell grades and standards. Available online at: https://www.ams.usda.gov/grades-standards/filberts-shellgrades-and-standards (Accessed March 21, 2024).
USDA-NASS (2023). Quick stats. Available online at: https://quickstats.nass.usda.gov/ (Accessed March 21, 2024).
USDA-NRCS (2023). Soil survey staff, natural resources conservation service (United States Department of Agriculture). Available online at: http://websoilsurvey.sc.egov.usda.gov/ (Accessed March 21, 2024).
Keywords: crop safety, filbert, herbicide mixture, protoporphyrinogen oxidase (PPO)inhibiting herbicide, resistance management
Citation: Miranda JWA and Moretti ML (2025) Optimizing weed and sucker control in hazelnut orchards with tiafenacil. Front. Agron. 7:1568894. doi: 10.3389/fagro.2025.1568894
Received: 30 January 2025; Accepted: 05 March 2025;
Published: 25 March 2025.
Edited by:
Simerjeet Kaur, Punjab Agricultural University, IndiaReviewed by:
Qaiser Javed, Jiangsu University, ChinaCopyright © 2025 Miranda and Moretti. This is an open-access article distributed under the terms of the Creative Commons Attribution License (CC BY). The use, distribution or reproduction in other forums is permitted, provided the original author(s) and the copyright owner(s) are credited and that the original publication in this journal is cited, in accordance with accepted academic practice. No use, distribution or reproduction is permitted which does not comply with these terms.
*Correspondence: Joshua W. A. Miranda, am9zaC5taXJhbmRhQG9yZWdvbnN0YXRlLmVkdQ==
†ORCID: Joshua W. A. Miranda, orcid.org/0000-0003-3591-5190
Marcelo L. Moretti, orcid.org/0000-0001-8313-9195
Disclaimer: All claims expressed in this article are solely those of the authors and do not necessarily represent those of their affiliated organizations, or those of the publisher, the editors and the reviewers. Any product that may be evaluated in this article or claim that may be made by its manufacturer is not guaranteed or endorsed by the publisher.
Research integrity at Frontiers
Learn more about the work of our research integrity team to safeguard the quality of each article we publish.