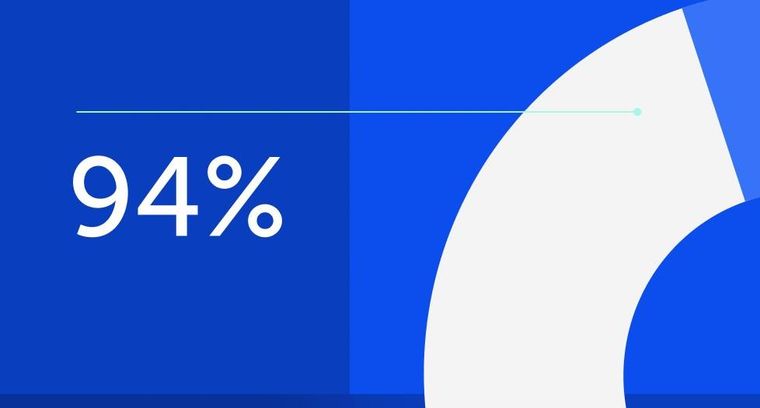
94% of researchers rate our articles as excellent or good
Learn more about the work of our research integrity team to safeguard the quality of each article we publish.
Find out more
ORIGINAL RESEARCH article
Front. Agron., 07 March 2025
Sec. Plant-Soil Interactions
Volume 7 - 2025 | https://doi.org/10.3389/fagro.2025.1546989
This article is part of the Research TopicSoil Tillage and Nitrogen Fertilization Methods for Sustainable Productivity of Industrial CropsView all 3 articles
In a context of climate change, barley can play a key role in cereal cropping systems, but the competitiveness of the crop requires the introduction of system innovations to increase its yield potential and to make the use of agronomic inputs more efficient. A hybrid genotype was compared with a conventional genotype in a three-year study. The treatments involved a factorial comparison of four combinations of genotype per seed density (medium vs high seed rate for each genotype), two soil tillages (conventional CT vs minimum tillage MT) and two N fertilization (equally split between the tillering and stem elongation growth stages vs a higher rate at the stem elongation stage). The hybrid genotype showed a higher grain yield (+8%) than the conventional one, as a consequence of a higher stay green. The lower ear density of the hybrid (-20%) was compensated by a higher number of kernels per ear (+16%) and a higher grain weight (+14%). A higher seeding rate did not increase the ear density and the grain yield; furthermore, CT and a higher N fertilization at the tillering stage led to a more rapid crop establishment. An increase in the seeding rate and the more balanced N application between the proposed timings resulted in a higher test weight for the hybrid (+0.9 kg hL-1). The hybrid genotype had a lower grain protein content than the conventional one, although this parameter increased on average by 0.4% for CT and a higher N application at stem elongation; moreover, the hybrid genotype showed a higher susceptibility to deoxynivalenol contamination (+51%). As regard the modelled global warming potential, the higher grain yield of the hybrid led to lower greenhouse gases emissions (-9%), compared to the conventional genotype. The adoption of MT, which did not have any impact on barley productivity, compared to CT, resulted in a further reduction of the environmental impact (-21%). The introduction of hybrids combined with a correct adaptation of other crop practices could be a way of improving both the grain yield and sustainability of the cereal cropping system.
According to the FAO prospects, the current and future requests for food, feeds and energy to satisfy the growing population represents a great challenge for the agriculture sector and leads to a strong pressure being exerted on land use and input production. Agriculture is responsible for different potential environmental impacts, due to its dependence on several productive inputs (e.g. fossil fuel, fertilizers, pesticides) and, at the same time, the higher and more frequent environmental stresses related to climate change represent a further risk for the stability of crop yields. In this context, the development of more efficient cropping systems, which would be able to reduce agronomic inputs without reducing the grain yield or its quality and which would be able to increase the sustainability of agricultural systems, is therefore necessary (Alexandratos and Bruinsma, 2012; Tricase et al., 2018a). Barley (Hordeum vulgare L.) is the world’s fourth most cultivated cereal crop after wheat (Triticum aestivum L.), maize (Zea mays L.) and rice (Oryza sativa L.), with a harvested area of about 47 million ha and an average grain yield of 3.3 t ha-1 (FAOSTAT, 2022). The crop is cultivated over a wide environmental range in temperate growing areas, thanks to its high ecological adaptation and lower production costs compared to other cereal crops. Furthermore, the achievable grain yield can be compared to that of cereals with higher agronomic requirements, such as wheat (Tricase et al., 2018a). Barley grains are used for multiple purposes and, in addition to the feed supply chain (around 70%), industrial end-uses, such as malting, brewing and distilling industries (21%), food (flour, pearled and puffed grain; 6%) and energy (biofuel production) are becoming increasingly important (Griffey et al., 2010; Tricase et al., 2018b). Industrial end-use of barley requires a higher attention to reach the quality requirements of the supply chains, in particular the respect of sanitary constraints (mycotoxin contamination) and the satisfaction of technological traits (test weight and grain protein content).
In a context of climate change, barley can play an important role in temperate growing areas, due to its potentially higher ability to avoid crop stress due to drought than other cereals. In addition, the early harvesting offers the opportunity to introduce an intercrop with longer duration and higher yield potential, such as maize, taking advantage of the greater number of growing degree days (GDDs) available due to the Global Warming Potential (GWP) (Maresma et al., 2019; Blandino et al., 2023). Furthermore, the competitiveness of the crop requires the introduction of agronomic and system innovations that would be able to increase its yield potential and, at the same time, reduce the use of agronomic inputs and make them more efficient, through the application of simpler agronomic techniques, such as minimum tillage.
In the last 15 years, the main genetic innovation has involved the development of hybrid cultivars, instead of conventional pure lines (Mühleisen et al., 2013). Hybrids obtained by crossing different genetic lines, thanks to the phenomenon of heterosis, which leads to an increase of heterozygosity, have a greater vigor and a greater development than conventional genotypes (Longin et al., 2012). The obtained advantages are due to certain desirable attributes, such as a better root penetrance, a potential better response to fertilizers, a longer duration of grain filling and the possibility of combining the characteristics of the parent lines, which leads to a greater adaptability of the hybrid to different cultivation environments and a potentially higher tolerance to abiotic and biotic factors (Gupta et al., 2019). The use of hybrids for allogamous species, such as maize, sorghum, rye or sunflower, is an established reality (Coors and Pandey, 1999). However, although hybrid breeding programs have been carried out for many decades, the diffusion of autogamous cereal hybrids on the market is still very limited (wheat) or has only occurred recently (barley), due to the drawbacks associated with their breeding. The first field comparisons reported that barley and wheat hybrids could have a higher biomass and grain yield than conventional ones (Maresma et al., 2019). Furthermore, lower levels of heterosis have generally been reported for autogamous cereals than for allogamous species. Moreover, small grain cereals require an overall higher plant density, which, combined with a lower cost-efficient seed production, because of their biological constraints, results in a possible lower return of investment for farmers (Mühleisen et al., 2013).
Since they have a higher seed cost, barley hybrid genotypes are generally sown at a lower seed rate than conventional genotypes. Thus, it is necessary to adapt the cropping system in order to enhance the overall profitability of the cultivation of hybrids, paying particular attention to agronomic practices that are able to recover the disadvantage of a lower seeding rate by promoting tillering and to exploit their high vigor.
In addition to the seeding rate, soil tillage, which has a direct effect on the soil temperature, and nitrogen (N) fertilization, can also influence barley tillering. A minimum tillage, as an alternative to conventional ploughing, could physically restrict crop emergence and root development, as a consequence of a higher soil compaction and the presence of crop residues on the soil surface. Moreover, if a lower soil porosity occurs, in the absence of the conventional ploughing, plant growth could slow down, because of a slower soil drying up and warming after the winter seasons, a lower root penetration and also a lower N availability (Peigné et al., 2007). The application of N fertilization, in temperate growing areas, is generally split between tillering (growth stage GS 23 (Zadoks et al., 1974)) and the beginning of stem elongation (GS 31), with a higher nutrient rate for this second timing, in order to support the higher plant uptake at this GS. Furthermore, there is a direct relationship between the N rate at the end of the winter season and the number of tillers (Otteson et al., 2008), which could be of interest for hybrids sown at a lower density.
In addition to crop yield, soil tillage and N fertilization also play key roles in the sustainability of cereal cultivation, since, among the different agronomic practices in the cropping system, they make the highest contribution to the indicators related to the GWP and nutrient loss (leaching, run-off, volatilization) (Sørensen et al., 2014; Campbell et al., 2017). In order to enhance the environmental sustainability of a cropping system, it is necessary to focus on both increasing the crop yield and the input use efficiency and on reducing the input applications.
The introduction of hybrid barley, combined with a correct adaptation of crop practices, could be one way of significantly improving the cultivation of the cereal and of reducing the GWP of the cropping system. Furthermore, hybrid barley breeding has generally targeted northern European regions, which have a high yield potential (Fernández-Calleja et al., 2023), while information about the agronomic performances of these genotypes in growing areas in southern Europe, which have a short vernalization period, as well as early flowering and maturation, has not yet been fully assessed. The aim of the present study has been to verify the agronomic potential of hybrid barley, considering different cropping systems related to the seeding rate, soil tillage and N fertilization strategies. For this purpose, we compared a hybrid barley genotype with a conventional genotype in a three-year study carried out in a growing area where barley has frequently been inserted into the crop sequences of cereal farms.
The study was carried out in the North-West of Italy over three growing seasons (2017-18, 2018-19 and 2019-20) in Chivasso (45°12’ N 07°58’ E, 183 m of altitude), in an area where maize, wheat and barley are the main crops. The compared treatments were factorial combinations of:
Four combination of barley genotype per seed rate:
● H150, a combination of a hybrid genotype (cv. Tektoo, Syngenta Italia) and a sowing rate of 150 seeds m-2 (approximately 70 kg seeds ha-1),
● H300, a combination of the hybrid genotype (cv. Tektoo) and a sowing rate of 300 seeds m-2 (approximately 140 kg seeds ha-1),
● C350, a combination of a conventional pure line genotype (cv. Ketos, Limagrain Italia) and a sowing rate of 350 seeds m-2 (approximately 140 kg seeds ha-1),
● C500, a combination of the conventional pure line genotype (cv. Ketos) and a sowing rate of 500 seeds m-2 (approximately 210 kg seeds ha-1);
Two soil tillages:
● CT, conventional tillage (ploughing to a depth of 25 cm and double harrowing with a disk and rotary harrow),
● MT, short-term minimum tillage (double harrowing to a depth of 10 cm using a disk harrow as an occasional tillage system);
Two N fertilization strategies:
● 60 + 60, 120 kg N ha-1 split between GS 23 (60 kg N ha-1) and GS 31 (60 kg N ha-1), respectively,
● 30 + 90, 120 kg N ha-1 split between GS 23 (30 kg N ha-1) and GS 31 (90 kg N ha-1), respectively.
The experiment was carried out according to a slit-plot design, with the combination of soil tillage and genotype per seed rate as the main-plot treatments and the N fertilization as the sub-plot treatment. Each treatment was replicated 4 times, the main-plot area was 125 m2 and the sub-plot area was 12 m2.
The experimental field was characterized by a superficial and fertile loamy soil (52% sand, 41% silt, 8% clay), typic Hapludalfs (USDA classification), with a high content of organic matter (2.55%) and a balanced C/N ratio (10.1). The soil was sub-acid (pH 6.2) and the Cation Exchange Capacity was medium (12.1 Cmol(+) kg−1). The soil was sampled at a depth of 0-30 cm each year at the end of the winter season and before N fertilization at tillering. The total N content was considered medium in each growing season, with 1.47, 1.37 and 1.27 g kg-1 in 2018, 2019 and 2020, respectively. The available phosphorus content (P Olsen, on average 42 mg kg-1) and the exchangeable potassium content (99 mg kg-1) were both high. The meteorological data were obtained from a weather station located near the experimental field.
The conventional crop practices of the growing areas were applied in each experiment. Briefly, the previous crop was grain maize each year. The conventional genotype (cv. Ketos) is the reference variety in the area of the study for its the high productivity, while the hybrid genotype (cv. Tektoo) was chosen among the commercial genotypes available on the market for its precocity, which is adequate for the considered environmental conditions.
The sowing density applied in the H150 and C350 treatments are those ordinary used in the growing area for the sowing of hybrid and conventional barley genotypes, respectively, with a comparable sowing cost. The treatments H300 and C500 allow to compare for both genotypes a higher sowing density, which could be necessary with late sowing, poor seedbed conditions and more generally production situations with an expected lower crop emergence. N fertilization was carried out by hand, according to application timing, using granular ammonium nitrate (27%). Sowing was carried out at the end of October in each experimental growing season, while harvesting was at the end of June (Table 1). Sowing was conducted in 12 cm wide rows. A chemical weed control was carried out with Pinoxaden 4.43% + Florasulam 0.49% + Cloquintocet-mexyl 1.11% (Axial One®, Syngenta Crop Protection S.p.A.) at the end of tillering. No fungicides were applied to protect the crop from foliar or head diseases.
A hand-held optical sensing device, GreenSeekerTM® (Trimble©, USA), was used to measure the normalized difference vegetation index (NDVI) during the vegetative stage, and the assessment was performed on all the plots approximately every 7 days. The instrument was held at a height of approximately 1 m above the ground, and its effective spatial resolution was 0.75 m per the entire length of the plot (6 m). The NDVI values were expressed as the area under the canopy development curve (AUCDC) using the following formula:
where R is the NDVI value, t is the time of observation, and n is the number of observations. This method was used to quantify the vigor of the compared treatments, in terms of crop canopy development. The AUCDC value was calculated for the entire crop cycle (GS 21-91) and for the vegetative (GS 21-61) and ripening (GS 61-91) periods. The AUCDC value pertaining to barley ripening, from flowering (GS 61) to complete senescence (GS 91), is a clear indicator of crop stay green, while the index could be influenced during the vegetative stage mainly by the ability of the canopy to cover the ground (Capo and Blandino, 2021).
The incidence and severity of foliar diseases were recorded for each plot by making a visual assessment of the symptoms at milk growth stage (GS 75). Incidence was calculated as the percentage of leaves showing symptoms, taking into account the two upper leaves of 20 stems per plot. Severity was calculated as the percentage of the leaf area with symptoms, using a standard area diagram (Capo and Blandino, 2021). In all the considered growing seasons and for both genotypes almost all the detected symptoms were attributable to Net Blotch disease (Pyrenophora teres f. teres).
Ear density assessments (ears per m2) were carried out, during the dough stage (GS 85), by counting the number of spikes within a 0.0625 m2 area of a metal square, twice per plot. The tillering index was calculated by dividing the number of ears per m2 by the number of plants per m2. The average number of kernels per ear was determined from 30 ears randomly selected from each plot.
The grain yield was obtained by harvesting the whole plot using a cereal plot combine harvester (Walter Wintersteiger, Austria). The test weight (TW) and grain moisture were analyzed using a GAC® 2100 Grain Analyzer (Dickens-John Corp, USA) and the previously weighed grain yield was adjusted to a 13% moisture content. The thousand kernel weight (TKW) was determined on two 200-kernel repetitions for each sample, using a precision balance.
A representative aliquot of barley grains was ground using a ZM 200 Ultra Centrifugal Mill (Retsch, Germany), and the grain protein content (GPC) was determined by means of near-infrared reflectance spectroscopy, using an NIR Systems 6500 monochromator instrument (Foss, Denmark).
The grains harvested from each plot were mixed thoroughly, and a representative aliquot of 4 kg of grains was milled. A representative sub-sample of the milled material was analyzed for the mycotoxin concentration. Deoxynivalenol (DON) contamination was analyzed using the ELISA method, by means of direct competitive immunoassays (Ridascreen® DON, R-Biopharm, Germany), according to the manufacturer’s instructions (Blandino et al., 2022).
The environmental impact of the compared cropping systems was quantified by means of the LCA method, in accordance with the ISO 14040 and ISO 14044 standards (ISO, 2006a, b). A functional unit of 1 t of dry-matter grains was assumed. The system boundaries were defined “from the cradle to the farm gate” in order to include all the agricultural practices and inputs required for barley production. The attributional approach was applied, and a mass allocation was performed. SimaPro v.9.5 (PRé Sustainability, The Netherlands), and the Ecoinvent v.3.9.1 database were used to model the studied systems. The environmental impact of the barley cropping systems was estimated as GWP (kg CO2 eq.), using the Intergovernmental Panel on Climate Change methodology (100 y, v 1.02) (IPCC, 2021).
The data analyses were performed in the R environment (R Core Team, 2016). A graphical method was used to verify the basic assumptions (Onofri et al., 2016). The experimental data were analyzed using a linear mixed effects model (lme () function from the nlme package) represented by the following equation:
Where yijkdlm is the response variable; µ is the overall mean, αi is the effect of the i-th genotype per seed rate fixed factor; Bj is the effect of the j-th soil tillage fixed factor; γk is the effect of the k-th N fertilization fixed factor; αβij, αγik, βγjk and αβγijk are the interaction effects between factors; δl is the effect of the l-th year random factor; λdl is the effect of the dl-th block random factor; ρm is the effect of the m-th main-plot random factor; ϵijkdlm is the random error term.
Similarly, the GWP was analyzed by considering the combination of genotype per seed rate and soil tillage as fixed effects, while the year and block were considered as random factors. The N fertilization was not considered as a factor, because the total N rate did not change between treatments. When single factors or their interaction determined a significant effect, the means were compared using the Bonferroni post hoc test, with p-value ≤ 0.05.
The three growing seasons experienced different meteorological trends, as far as both rainfall, temperature and GDDs are concerned (Table 2). The 2017-18 growing season had moderate rainfalls, and it was characterized by a cooler temperature, especially during the winter season, and fewer GDDs than in the other seasons. In addition, high rainfall was recorded in May, during the flowering, resulting in the lowest grain yield (on average 5.2 t ha-1) and the highest DON contamination (9252 µg kg-1). Overall, the 2018-19 growing season had the lowest amount of rainfall during the crop cycle, in particular from December to March and in June, resulting in the highest grain yield (on average 7.5 t ha-1) and intermediate values of DON (on average 3292 µg kg-1). Otherwise, the 2019-20 growing season showed the highest total rainfall, which was mainly concentrated in November, the highest number of total GDDs, and a warmer winter period. This condition resulted in a low grain yield (on average 5.3 t ha-1), due to a low number of kernels per ear, while the low rainfall at flowering, led to a low DON contamination (313 µg kg-1). In each growing season, the flowering time of compared genotypes occurred on the same days.
Table 2. Monthly rainfall, average temperature and growing degree days (GDDs) during the field experiment carried out in Chivasso in 2018-2020.
The AUCDC values during the whole crop cycle (GS 21-91), in the vegetative period, until flowering (GS 21-61) and in the ripening period (GS 61-91) are shown in Table 3. No significant differences were found in AUCDC GS 21-91, regarding the combination of genotype and seed rate. During the vegetative stage (GS 21-61), only the hybrid sown at the lower seed rate (H150) showed statistically lower values than C500 (-5%) due to the initial low number of plants, while no differences were found between the hybrid and the conventional genotype sown at comparable seed rates (H300 and C350), which showed intermediate values compared to the other treatments. Instead, the hybrid (H150 and H300) showed higher cumulative NDVI values during ripening (GS 61-91) than the conventional genotype (+13% compared to the average of C350 and C500 treatments), due to a more prolonged canopy stay green.
Table 3. Effect of the combination of barley genotype and seed rate, soil tillage and N fertilization on the area under the canopy development curve (AUCDC) values during the whole crop cycle (GS 21-91), as well as the vegetative (GS 21-61) and the ripening (GS 61-91) growth stages for the field experiment carried out in Chivasso in 2018-2020.
The hybrid genotype (H150 and H300) showed a significantly lower leaf disease incidence (16%) and severity (76%) than the conventional genotype (data not shown), explaining the higher reported stay green.
Overall, CT significantly increased the AUCDC values (+9%) of barley, compared to MT, throughout the growing season, and also when considering the vegetative or ripening period separately, although a lower leaf disease severity (40%) was observed in MT compared to CT. The N fertilization strategy directly influenced the AUCDC values, according to the growth stage: the 60 + 60 treatment reported a higher AUCDC 21-61 value (+6%), which refers to the vegetative stage, while the 30 + 90 treatment, with a later N rate application, reported a higher AUCDC 61-91 value during ripening (+2%). A higher cumulative value of NDVI was reported for the 60 + 60 treatment (+3%) when considering the entire crop cycle (AUCDC 21-91).
The interactions between G x T and G x N were significant for AUCDC during the vegetative development (GS 21-61) and the whole crop cycle (GS 21-91). As far as the genotypes are concerned, the hybrid sown at 150 seeds m-2 resulted in a higher NDVI value for CT than for MT (Figure 1). Differences between the tillage conditions for the cultivation of a conventional genotype were only reported for 350 seeds m-2, while the benefits, in terms of plant vigor and development of the ploughing, were always significant and more marked in the hybrid genotype than for the MT conditions (+19%). H300 and C350 showed intermediate values, while C500 showed no significant differences between the soil tillage systems.
Figure 1. Effect of the combination of genotype per seed rate and soil tillage on the normalize difference vegetation index (NDVI) values expressed as area under the canopy development curve (AUCDC) from tillering (GS21) to flowering (GS 61) for the field experiment carried out in Chivasso in 2018-2020. The reported data refer to the average of 3 growing seasons and 4 replications. Different letters between treatments indicate significant differences (p-value<0.05). Error bars represent the standard error of the mean. Genotype per seed rate: H150, hybrid barley sown at 150 seeds per m2; H300, hybrid barley sown at 300 seeds per m2; C350, conventional barley sown at 350 seeds per m2; C500, conventional barley sown at 500 seeds per m2. Soil tillage: CT, conventional tillage, with ploughing; MT, minimum tillage.
Regarding the N fertilization (Figure 2), the 60 + 60 treatment always showed higher AUCDC GS 21-61 values than the 30 + 90 treatment (+6%), thanks to the availability of the higher N dose during the tillering stage. Moreover, the AUCDC of the 60 + 60 treatment was significantly higher for C500 than for H150, while intermediate values were observed for H300 and C350. Otherwise, no difference was reported between genotypes for the 30 + 90 treatment, thus highlighting the importance of suppling a sufficient N rate in the early stages to support crop tillering. Similar trends between G x T and G x N were observed for AUCDC GS 21-91 (data not shown).
Figure 2. Effect of the combination of genotype per seed rate and N fertilization on the normalized difference vegetation index (NDVI) values expressed as the area under the canopy development curve (AUCDC) from tillering (GS21) to flowering (GS 61) for the field experiment carried out in Chivasso in 2018-2020. The reported data refer to the average of 3 growing seasons and 4 replications. Different letters between treatments indicate significant differences (p-value<0.05). Error bars represent the standard error of the mean. Genotype per seed rate: H150, hybrid barley sown at 150 seeds per m2; H300, hybrid barley sown at 300 seeds per m2; C350, conventional barley sown at 350 seeds per m2; C500, conventional barley sown at 500 seeds per m2. N fertilization: 60 + 60, 60 and 60 kg N ha-1 during GS 23 and GS 31, respectively; 30 + 90, 30 and 90 kg N ha-1 during GS 23 and GS 31, respectively.
The genotype per seed rate factor significantly influenced the grain yield and yield components (Table 4). The hybrid (H150 and H300) generally had a higher grain yield (on average +8%), kernels per ear (+16%) and TKW (+14%) than the conventional genotype (C350 and C500), which showed a higher ear density (+20%). H150 had the highest tillering index value, due to the lower seed density, and C500 had the lowest one, while no difference was found between H300 and C350. On average, no yield difference was found between the compared soil tillage practices. Instead, 60 + 60 N fertilization showed an overall higher grain yield (+3%), but a lower number of kernels per ear than the 30 + 90 treatment (3%), while no differences were found for the other yield components (ear density and TKW), although both were higher in the 60 + 60 treatment.
Table 4. Effect of the combination of barley genotypes and seeding rate, soil tillage and N fertilization on the grain yield, ear density, tillering index, kernels per ear and thousand kernel weight (TKW) for the field experiment carried out in Chivasso in 2018-2020.
Significant interactions between G x T were found for the grain yield, kernels per ear and TKW (Figure 3) and between T x N for the grain yield (data not shown). Regardless of the seeding rate, when comparing the soil tillage systems, the hybrid barley generally showed a higher grain yield (+10% for CT and +5% for MT), although the value was only statistically significant compared to C500 for CT. Moreover, the 60 + 60 N fertilization treatment resulted in a higher grain yield than the 30 + 90 treatment for the CT condition (+5%), while intermediate values were observed for both of the N fertilizations for MT.
Figure 3. Effect of the combination of genotype per seed rate and soil tillage on the grain yield, kernels per ear and thousand kernel weight (TKW) for the field experiment carried out in Chivasso in 2018-2020. The reported data refer to the average of 3 growing seasons and 4 replications. Different letters between treatments indicate significant differences (p-value<0.05). Error bars represent the standard error of the mean. Genotype per seed rate: H150, hybrid barley sown at 150 seeds per m2; H300, hybrid barley sown at 300 seeds per m2; C350, conventional barley sown at 350 seeds per m2; C500, conventional barley sown at 500 seeds per m2. Soil tillage: CT, conventional tillage, with ploughing; MT, minimum tillage.
Regarding the main yield components, H150 showed a higher number of kernels per ear for CT than for MT (+15%), but without any statistical differences from H300 for either of the soil tillages. Instead, the conventional genotype showed a lower number of kernels per ear, especially for the high seeding rate (C500). TKW was always significantly higher for the hybrid barley. Moreover, the lowest seed density (H150) resulted in a significantly higher seed weight for the MT condition than for the CT condition (+6%), while no differences between the soil tillages were found for the other treatments (H300, C350, C500).
As far as the genotype per seed rate is concerned, H300 had a higher TW than the other treatments (+1.2 Kg hL-1) (Table 5). The GPC was higher for C500 than for H300 (+0.5%), while H150 and C350 reported intermediate values. The DON content was higher for the hybrid (H150 and H300, on average +51%) than for the conventional genotype (C350 and C500), thus showing a higher susceptibility of the considered hybrid genotype to mycotoxin contamination.
Table 5. Effect of the combination of barley genotype and seeding rate, soil tillage and N fertilization on the test weight (TW), grain protein content (GPC) and deoxynivalenol (DON) contamination for the field experiment carried out in Chivasso in 2018-2020.
The soil tillage significantly affected the GPC and DON contents: CT resulted in a higher protein content (+0.4%) and lower contamination of mycotoxin (55%) than MT, while no differences were found for TW. The 60 + 60 treatment showed a higher TW (+0.3 kg hL-1) and lower GPC (-0.4%) than the 30 + 90 treatment, while N fertilization did not affect DON contamination to any great extent. The interactions between the compared agronomic factors were never significant for any of the compared qualitative parameters.
The crop practices applied to produce barley on average resulted in a GWP of 229 CO2 eq. per t of grain. By analyzing the relative contribution of each practice, it emerged that fertilization accounted for about 42% of the total GWP, and this was followed by grain harvesting (25%). The contribution of soil tillage (on average 23%) varied between 31% of the total for CT and 13% for MT. A lower contribution was reported for sowing (8%), with slight difference according to the seed density, as well as for the chemical weed control (1%).
Figure 4 shows the GWP of the different combinations of genotype per seed density and soil tillage. In accordance with the grain yield results, the hybrid genotype had lower greenhouse gas (GHG) emissions (9%) than the conventional one. The combinations of hybrid per seed rate (H150 and H300) showed a statistically lower GWP than C500 for the CT condition, due to the higher grain yield and the smaller number of seeds per ha, while no difference was found from C350. No differences were found between the combinations of genotype per seed rate for the MT condition. As far as soil tillage is concerned, as expected, MT had a significantly lower impact on GWP (202 kg CO2 eq. t-1, -21%) than CT (256 kg CO2 eq. t-1).
Figure 4. Effect of the combination of genotype per seed rate and soil tillage on the global warming potential (GWP, kg CO2 eq. t-1), considering 1 t of grain yield as the functional unit, for the field experiment carried out in Chivasso in 2018-2020. The reported data refer to the average of 3 growing seasons and 4 replications. Different letters between treatments indicate significant differences (p-value<0.05). Genotype per seed rate: H150, hybrid barley sown at 150 seeds per m2; H300, hybrid barley sown at 300 seeds per m2; C350, conventional barley sown at 350 seeds per m2; C500, conventional barley sown at 500 seeds per m2. Soil tillage: CT, conventional tillage, with ploughing; MT, minimum tillage.
Climate change is already affecting crop production, and abiotic stresses, such as higher temperatures, drought, extreme events and changing seasonal climate patterns, are expected to have an even greater impact on agriculture in the future (IPCC, 2014). It is therefore necessary to develop more resilient cropping systems by defining different combinations of agronomic practices that can satisfy this need. In this context, the cultivation of hybrid barley could represent an innovation that would be able to guarantee a higher resilience of cereal cropping system. As reported in previous studies (Mühleisen et al., 2014a, b; Philipp et al., 2016; Preiti et al., 2021; Blandino et al., 2023), but also in the present experiment, the hybrid showed a generally higher grain yield potential (on average +8%) than the conventional genotype under different agronomic conditions. The higher yield capacity of the considered hybrid genotype is clearly related to the higher ear dimension (i.e. number of kernels per ear) and the higher seed weight. In particular, the advantage, in term of TKW, is a consequence of the higher stay green, as detected by the higher NDVI during the ripening stages. The weak point of the hybrid, on the other hand, is related to the lower plant density, which, in turn, is related to the initial lower seed rate, and which always resulted in a lower ear density at harvest than the system based on the cultivation of a conventional genotype. Since the higher seed cost of a hybrid does not allow the same seed rate to be achieved as a conventional genotype, it is necessary to design cultivation systems that will be capable of minimizing the agronomic weakness of such genotypes. Compared to previous articles that compared the yield capacity of hybrid barley with that of a conventional one, the analysis of the yield performance has also been carried out in this study considering a series of cropping systems, based on combinations of different seed rates, soil tillage management practices and N fertilization, which are all crop practices that have a direct influence on the tillering capacity and ear density of barley. The compared seed rates did not significantly affect the final ear density of the different genotypes, thus confirming the plasticity of winter cereal for this trait that has been reported to be more plastic than the kernel per ear or kernel weight (Sadras and Slafer, 2012; Slafer et al., 2014). Indeed, the tillering index was inversely proportional to the seed density, with a significant decrease from the lowest density to the highest one, while no differences were found for the intermediate values, regardless of the genotype. This is likely to be related to an increased intra-row competition between neighboring plants for space and nutrients, as found in previously studies (O’Donovan et al., 2011). A different type of behavior was observed for the two compared genotypes concerning the grain yield, although the difference was not statistically significant, and it depended on the seeding rate, although a clear interaction with the soil tillage treatment was also observed. The highest initial investment of the conventional genotype (C500) under the MT conditions led to a higher grain yield, while the lower one (C350) led to a higher production under the CT conditions. Conversely, the hybrid genotype did not show such a difference between the two seeding rate treatments (H150 and H300), thus suggesting that reducing the seed quantity had less impact on the yield performances of this genotype, as already observed by Preiti et al. (2021).
Soil tillage influenced the vegetative development of the crop to a great extent throughout the early phases (GS 21-61), since MT reduced the emergence rate, but also the growth speed in the first stages (Peigné et al., 2007; Ingraffia et al., 2023). It was observed, when comparing the different combinations of genotype per seed rate, that the higher initial investment of the plants was a key factor in achieving a high vegetative crop development and an optimal soil coverage for MT. However, CT showed a more marked effect of the genotype, regardless of the seed rate. The hybrid, due to its greater vigor, which is mainly manifested in a high biomass development (Preiti et al., 2021; Fernández-Calleja et al., 2023), and because of the better seedbed preparation, was able to quickly overcome the initial disadvantage of a lower plant investment, and no statistically significant differences were observed between the H150 and C500 treatments, while slightly higher average soil cover values were detected. The more unfavorable conditions of MT, associated with a lower soil porosity, lower soil warming, and lower N availability (Melaj et al., 2003), could instead have limited the potential growth of the hybrid in the first steps of the cultural cycle. Indeed, the hybrid, with its greater plant development under CT conditions, had a larger number of kernels per spike than the conventional genotype. Conversely, the difference between the compared genotypes for this yield component was significantly less pronounced under the MT conditions.
It was also observed, for both genotypes, that a higher N availability at the beginning of spring (60 + 60) allowed the crop to grow faster, as a result of a higher tillering capacity and biomass development. N fertilization, considering the combination of the N rate and timing of application at different growth stages, has been shown to influence the plasticity of the yield components in winter cereal to a great extent (Benincasa et al., 2022). An early N application leads to a higher number of tillers per plant, and thus to a better survival rate (Tehulie and Eskezia, 2021), thereby ensuring a high early vigor and crop establishment. Preiti et al. (2021) also found that higher N levels at tillering resulted in a higher number of spikes. In addition, compared to other winter cereals, such as wheat, barley may have less need of N during the stem elongation phase, due to a shorter crop cycle, and the practice of anticipating a higher rate of the nutrient at tillering could probably be applied with greater agronomic benefits. Indeed, the application of a higher N fertilization at tillering (60 + 60) resulted in a higher yield, although the effect was influenced by the soil tillage: the effect of the compared N fertilization treatments was more pronounced under the CT conditions, whereas MT seems to mitigate the impact of N fertilization on the grain yield, probably because of a lower soil N availability (Melaj et al., 2003). Overall, the combination of CT and a higher dose of N at the beginning of tillering (60 + 60) led to a more rapid barley canopy closure, thereby maximizing light and nutrient use efficiency (Schmitz and Ransom, 2021), and led to a better crop establishment, which could be an important advantage for hybrid genotypes due to their lower seeding rates. Furthermore, although both soil tillage and the N fertilization timing showed a similar impact on barley canopy development, in function of the considered genotypes and seeding rate, only the N application led to a significant effect on grain yield. Instead, the hybrid showed a prolonged canopy stay green during the maturation phase, mainly due to the lower susceptibility to foliar disease, which was superior to the compared conventional genotype, regardless of the seeding density, soil tillage and N fertilization. A prolonged canopy stay green is closely related to the duration of the photosynthetic activity of the leaf area, which has a positive effect on grain yield and late yield components (fully developed grain per ear and grain weight) for a longer grain filling period. Therefore, the use of hybrid genotypes could be an alternative to the use of other agronomic strategies that are able to prolong the canopy stay green of barley, such as the application of fungicides or foliar fertilizers (Marinaccio et al., 2015). The longer stay green of the hybrid genotypes is another agronomic trait that could lead to a higher N fertilization rate at the tillering growth stage instead of at stem elongation, without the risk of incurring a too rapid senescence, which could negatively affect the targeted late yield components (grain per ear and grain weight). This agronomic behavior could also be supported by a deeper and more efficient root system (Kempe and Gils, 2011), which could be able to increase the plant uptake of N during the later phases of the crop cycle.
The hybrid and conventional barley genotypes also resulted in differences in the grain quality traits, which were influenced to a great extent by the adoption of the specific agronomic practices within the cropping system, as already reported for the yield traits. The increase in the seeding rate of the hybrid genotype resulted in a higher TW, due to a lower tillering index and, consequently, a lower number of late tillers, which were characterized by an incomplete starch accumulation and less dense grain filling than the main stems (Wang and Fu, 2020). No difference was found between the seeding rates for the conventional genotype. Moreover, a more balanced N fertilization between the tillering and the stem elongation increased the TW, probably thanks to a more contemporary tiller development.
As regard the nutritional composition, the hybrid genotype generally showed a lower GPC than the conventional one, as a result of the greater stay green and grain weight, thereby resulting in a higher starch-to-protein ratio. Moreover, considering the superior grain yield, the protein yield per unit area of the hybrid genotype was higher (+6%) than that of the conventional genotype. Regarding the agronomic practices, both soil tillage and the N fertilization timing influenced the GPC: CT resulted in higher values than MT, probably due to a higher availability of N and better root functionality, which led to a higher plant uptake (Peigné et al., 2007). The N fertilization carried out at a higher rate at stem elongation also led to a significant increase in GPC, due to both a higher N availability in the last stages of the crop cycle and to a lower grain yield than the 60 + 60 treatment.
From a sanitary point of view, the compared hybrid genotype showed a higher susceptibility to Fusarium head blight and resulted in a higher accumulation of DON mycotoxins. Although the higher sanitary risk associated with the cultivation of the hybrid genotype than with the conventional genotype should be evaluated as part of a larger pool of cultivars in order to obtain more reliable results, a possible explanation for this higher susceptibility could be the increased time required for ovary pollination and, consequently, the wider temporal window for the onset of infection by the pathogen, as has already been hypothesized for a rye hybrid regarding ergot alkaloid contamination (Carbonell-Rozas et al., 2023). The potential higher susceptibility of hybrid genotype needs to be minimized by adopting an appropriate cropping system, and, in particular, it is necessary to avoid the application of MT for high-risk precessions, such as grain maize (Blandino et al., 2012). Interestingly, the increase in the seed rate led to a reduction in the DON content in both genotypes, probably due to the reduced tillering and consequently to a lower number of late tillers, which prolonged the flowering and maturation, and resulted in an overall higher risk of infection and accumulation of mycotoxins (Beres et al., 2018).
As is well known, a lower environmental impact can be achieved for the mitigation of the GWP either by implementing less polluting agronomic techniques, such as reduced tillage practices and a lower rate of N fertilization, or by increasing the crop yield per unit area (Tricase et al., 2018a; Lovarelli et al., 2020). Therefore, the cultivation of barley hybrids also needs to consider the environmental sustainability requirements through an appropriate choice of the other crop practices, in order to maximize their yield efficiency (Vinci et al., 2022). In this study, the higher grain yield of the hybrid genotype led to a reduction of the GWP, compared to that of the conventional genotype, although this was only statistically significant compared to C500 under the CT condition. Furthermore, the adoption of MT, which did not impact barley productivity, compared to CT, resulted in a greater reduction of the barley GWP, due to the lower number of direct and indirect GHG emissions. The present experiment has highlighted that hybrid genotypes, in the same way as conventional ones, could be cultivated with the application of reduced tillage, without any clear reduction of productivity, although special care must be taken due to the higher risk of mycotoxin accumulation. Although the N rate was not considered in this experiment, it is the first factor that contributes to the GWP of winter cereal (Fantin et al., 2017; Del Hierro et al., 2021; Vinci et al., 2022). In order to enhance the environmental sustainability of a barley cropping system, it is necessary to verify whether the hybrid genotypes exert a higher N use efficiency than conventional ones, and, if so, an overall reduction of the N rate could be introduced, without any negative impact on the agronomic traits.
Despite the drawbacks of their breeding, related to the high seeding density coupled with a low cost-effective production system and, consequently, high seed costs, the selection gain of hybrids could be competitive, compared to conventional genotypes, and the demand for a higher yield per unit area and a lower use of agronomic input, could support the cultivation of autogamous cereal hybrids. This research has highlighted that the introduction of hybrid barley into the cropping system could maximize the grain yield even when low input cultivation strategies are adopted. Furthermore, the study has further underlined that genetic innovation requires an appropriate agronomic adaptation of the cultivation management practices in order to fully express its potential. Indeed, it is necessary to correctly address the crop practices of a barley hybrid that allow the effects of a lower seeding rate to be recovered, by stimulating crop tillering. The management of N fertilization plays a key role in such a context. Further investigations are needed to enlarge the comparison of a higher number of hybrid and conventional genotypes in order to better validate and generalize these findings, and to define adapted field programs for the cultivation of these genotypes in different production situations. Furthermore, since the adaptation of crop practices determines significant effects not only on the cereal yield, but also leads to relevant changes of the sanitary and quality traits, as well as of the intangible qualitative parameters, such as the GWP, the future breeding of barley hybrids and their contribution to re-designing cereal cropping systems should be addressed by carefully considering these additional drivers through a holistic approach.
The raw data supporting the conclusions of this article will be made available by the authors, without undue reservation.
MS: Data curation, Formal analysis, Investigation, Writing – original draft. RM: Formal analysis, Writing – review & editing. MB: Conceptualization, Formal analysis, Funding acquisition, Investigation, Methodology, Supervision, Writing – original draft.
The author(s) declare that no financial support was received for the research, authorship, and/or publication of this article.
The authors would like to thank all the field and lab technicians who made valuable contributions to the study and Marguerite Jones for the English and technical editing.
The authors declare that the research was conducted in the absence of any commercial or financial relationships that could be construed as a potential conflict of interest.
The author(s) declare that no Generative AI was used in the creation of this manuscript.
All claims expressed in this article are solely those of the authors and do not necessarily represent those of their affiliated organizations, or those of the publisher, the editors and the reviewers. Any product that may be evaluated in this article, or claim that may be made by its manufacturer, is not guaranteed or endorsed by the publisher.
AUCDC, area under canopy development curve; CT, conventional tillage; DON, deoxynivalenol; GDDs, Growing degree days; GHG, greenhouse gas; GPC, grain protein content; GS, growth stage; GWP, global warming potential; H150, hybrid barley genotype per 150 seeds m-2; H300, hybrid barley genotype per 300 seeds m-2; C350, conventional barley genotype per 350 seeds m-2; C500, conventional barley genotype per 500 seeds m-2; MT, short-term minimum tillage; N, nitrogen; NDVI, normalized difference vegetation index; TKW, thousand kernel weight; TW, test weight.
Alexandratos N., Bruinsma J. (2012). “World agriculture towards 2030/2050: the 2012 revision,” in ESA Working Paper No. 12-03 (ESA, Rome, Italy). Available at: https://www.fao.org/agrifood-economics/publications/detail/en/c/147899 (Accessed December 12, 2023).
Benincasa P., Reale L., Cerri M., Tedeschini E., Tosti G., Falcinelli B., et al. (2022). Nitrogen fertilization levels and timing affect the plasticity of yield components in bread wheat (Triticum aestivum L.). Field Crops Res. 289, 108734. doi: 10.1016/j.fcr.2022.108734
Beres B. L., Brûlé-Babel A. L., Ye Z., Graf R. J., Turkington T. K., Harding M. W., et al. (2018). Exploring Genotype × Environment × Management synergies to manage Fusarium head blight in wheat. Can. J. Plant Pathol. 40, 179–188. doi: 10.1080/07060661.2018.1445661
Blandino M., Battisti M., Vanara F., Reyneri A. (2022). The synergistic effect of nitrogen and phosphorus starter fertilization sub-surface banded at sowing on the early vigor, grain yield and quality of maize. Eur. J. Agron. 137, 126509. doi: 10.1016/j.eja.2022.126509
Blandino M., Haidukowski M., Pascale M., Plizzari L., Scudellari D., Reyneri A. (2012). Integrated strategies for the control of Fusarium head blight and deoxynivalenol contamination in winter wheat. Field Crops Res. 133, 139–149. doi: 10.1016/j.fcr.2012.04.004
Blandino M., Scapino M., Rollè L., Dinuccio E., Reyneri A. (2023). Biomass and methane production in double cereal cropping systems with different winter cereal and maize plant densities. Agronomy 13, 536. doi: 10.3390/agronomy13020536
Campbell B. M., Beare D. J., Bennett E. M., Hall-Spencer J. M., Ingram J. S. I., Jaramillo F., et al. (2017). Agriculture production as a major driver of the Earth system exceeding planetary boundaries. Ecol. Soc 22, 8. doi: 10.5751/ES-09595-220408
Capo L., Blandino M. (2021). Minimizing yield losses and sanitary risks through an appropriate combination of fungicide seed and foliar treatments on wheat in different production situations. Agronomy 11, 725. doi: 10.3390/agronomy11040725
Carbonell-Rozas L., Alabrese A., Meloni R., Righetti L., Blandino M., Dall’Asta C. (2023). Occurrence of ergot alkaloids in major and minor cereals from northern Italy: A three harvesting years scenario. J. Agric. Food Chem. 71, 15821–15828. doi: 10.1021/acs.jafc.3c05612
Coors J. G., Pandey S. (1999). The genetics and exploitation of heterosis in crops. (Madison, WI, USA: American Society of Agronomy, Crop Science Society of America, and Soil Science Society of America.
Del Hierro Ó., Gallejones P., Besga G., Artetxe A., Garbisu C. (2021). A comparison of IPCC guidelines and allocation methods to estimate the environmental impact of barley production in the basque country through life cycle assessment (LCA). Agriculture 11, 1005. doi: 10.3390/agriculture11101005
Fantin V., Righi S., Rondini I., Masoni P. (2017). Environmental assessment of wheat and maize production in an Italian farmers’ cooperative. J. Clean. Prod. 140, 631–643. doi: 10.1016/j.jclepro.2016.06.136
Fernández-Calleja M., Boutin C., Dyrszka E., Manès Y., Reif J. C., Zhao Y., et al. (2023). Identification of adapted breeding lines to improve barley hybrids for Spain. Crop Sci. 63, 186–203. doi: 10.1002/csc2.20858
FAOSTAT. (2022). Food and Agriculture Organization of the United Nations. Available online at: https://www.fao.org/faostat/en/home (Accessed December 11, 2023).
Griffey C., Brooks W., Kurantz M., Thomason W., Taylor F., Obert D., et al. (2010). Grain composition of Virginia winter barley and implications for use in feed, food, and biofuels production. J. Cereal Sci. 51, 41–49. doi: 10.1016/j.jcs.2009.09.004
Gupta P. K., Balyan H. S., Gahlaut V., Saripalli G., Pal B., Basnet B. R., et al. (2019). Hybrid wheat: past, present and future. Theor. Appl. Genet. 132, 2463–2483. doi: 10.1007/s00122-019-03397-y
Ingraffia R., Lo Porto A., Ruisi P., Amato G., Giambalvo D., Frenda A. S. (2023). Conventional tillage versus no-tillage: Nitrogen use efficiency component analysis of contrasting durum wheat genotypes grown in a Mediterranean environment. Field Crops Res. 296, 108904. doi: 10.1016/j.fcr.2023.108904
IPCC (2014). “Climate change 2014: synthesis report,” in Contribution of Working Groups I, II and III to the Fifth Assessment Report of the Intergovernmental Panel on Climate Change. Eds. Pachauri R. K., Meyer L. A. (IPCC, Geneva, Switzerland), 151 pp. Core Writing Team.
IPCC (2021). “Climate change 2021: the physical science basis,” in Contribution of Working Group I to the Sixth Assessment Report of the Intergovernmental Panel on Climate Change. Eds. Masson-Delmotte V., Zhai P., Pirani A., Connors S. L., Péan C., Berger S., Caud N., Chen Y., Goldfarb L., Gomis M. I., Huang M., Leitzell K., Lonnoy E., Matthews J. B. R., Maycock T. K., Waterfield T., Yelekçi O., Yu R., Zhou B. (Cambridge University Press, Cambridge, United Kingdom and New York, NY, USA), 2391 pp.
ISO (2006a). ISO 14040: Environmental management - Life cycle assessment - Principles and framework. International Organization for Standardization (Geneva, Switzerland).
ISO (2006b). ISO 14044: Environmental management - Life cycle assessment - Requirements and Guidelines. International Organization for Standardization (Geneva, Switzerland).
Kempe K., Gils M. (2011). Pollination control technologies for hybrid breeding. Mol. Breed. 27, 417–437. doi: 10.1007/s11032-011-9555-0
Longin C. F. H., Mühleisen J., Maurer H. P., Zhang H., Gowda M., Reif J. C. (2012). Hybrid breeding in autogamous cereals. Theor. Appl. Genet. 125, 1087–1096. doi: 10.1007/s00122-012-1967-7
Lovarelli D., Garcia L. R., Sánchez-Girón V., Bacenetti J. (2020). Barley production in Spain and Italy: Environmental comparison between different cultivation practices. Sci. Total Environ. 707, 135982. doi: 10.1016/j.scitotenv.2019.135982
Maresma Á., Martínez-Casasnovas J. A., Santiveri F., Lloveras J. (2019). Nitrogen management in double-annual cropping system (barley-maize) under irrigated Mediterranean environments. Eur. J. Agron. 103, 98–107. doi: 10.1016/j.eja.2018.12.002
Marinaccio F., Reyneri A., Blandino M. (2015). Enhancing grain yield and quality of winter barley through agronomic strategies to prolong canopy greenness. Field Crops Res. 170, 109–118. doi: 10.1016/j.fcr.2014.10.002
Melaj M. A., Echeverría H. E., López S. C., Studdert G., Andrade F., Bárbaro N. O. (2003). Timing of nitrogen fertilization in wheat under conventional and no-tillage system. Agron. J. 95, 1525–1531. doi: 10.2134/agronj2003.1525
Mühleisen J., Maurer H.-P., Stiewe G., Bury P., Reif J. C. (2013). Hybrid breeding in barley. Crop Sci. 53, 819–824. doi: 10.2135/cropsci2012.07.0411
Mühleisen J., Piepho H.-P., Maurer H. P., Longin C. F. H., Reif J. C. (2014a). Yield stability of hybrids versus lines in wheat, barley, and triticale. Theor. Appl. Genet. 127, 309–316. doi: 10.1007/s00122-013-2219-1
Mühleisen J., Piepho H.-P., Maurer H. P., Zhao Y., Reif J. C. (2014b). Exploitation of yield stability in barley. Theor. Appl. Genet. 127, 1949–1962. doi: 10.1007/s00122-014-2351-6
O’Donovan J. T., Turkington T. K., Edney M. J., Clayton G. W., McKenzie R. H., Juskiw P. E., et al. (2011). Seeding rate, nitrogen rate, and cultivar effects on malting barley production. Agron. J. 103, 709–716. doi: 10.2134/agronj2010.0490
Onofri A., Seddaiu G., Piepho H.-P. (2016). Long-Term Experiments with cropping systems: Case studies on data analysis. Eur. J. Agron. 77, 223–235. doi: 10.1016/j.eja.2016.02.005
Otteson B. N., Mergoum M., Ransom J. K., Schatz B. (2008). Tiller contribution to spring wheat yield under varying seeding and nitrogen management. Agron. J. 100, 406–413. doi: 10.2134/agronj2007.0109
Peigné J., Ball B. C., Roger-Estrade J., David C. (2007). Is conservation tillage suitable for organic farming? A review. Soil Use Manage. 23, 129–144. doi: 10.1111/j.1475-2743.2006.00082.x
Philipp N., Liu G., Zhao Y., He S., Spiller M., Stiewe G., et al. (2016). Genomic prediction of barley hybrid performance. Plant Genome 9, 1–8. doi: 10.3835/plantgenome2016.02.0016
Preiti G., Calvi A., Romeo M., Badagliacca G., Bacchi M. (2021). Seeding density and nitrogen fertilization effects on agronomic responses of some hybrid barley lines in a mediterranean environment. Agronomy 11, 1942. doi: 10.3390/agronomy11101942
R Core Team (2016). R: A Language and Environment for Statistical Computing (Vienna, Austria: R Foundation for Statistical Computing). Available at: http://www.R-project.org/.
Sadras V. O., Slafer G. A. (2012). Environmental modulation of yield components in cereals: Heritabilities reveal a hierarchy of phenotypic plasticities. Field Crops Res. 127, 215–224. doi: 10.1016/j.fcr.2011.11.014
Schmitz P. K., Ransom J. K. (2021). Seeding rate effects on hybrid spring wheat yield, yield components, and quality. Agronomy 11, 1240. doi: 10.3390/agronomy11061240
Slafer G. A., Savin R., Sadras V. O. (2014). Coarse and fine regulation of wheat yield components in response to genotype and environment. Field Crops Res. 157, 71–83. doi: 10.1016/j.fcr.2013.12.004
Sørensen C. G., Halberg N., Oudshoorn F. W., Petersen B. M., Dalgaard R. (2014). Energy inputs and GHG emissions of tillage systems. Biosyst. Eng. 120, 2–14. doi: 10.1016/j.biosystemseng.2014.01.004
Tehulie N. S., Eskezia H. (2021). Effects of nitrogen fertilizer rates on growth, yield components and yield of food Barley (Hordeum vulgare L.): A Review. J. Plant Sci. Agric. Res. 5, 46. Available at: http://www.imedpub.com/plant-sciences-and-agricultural-research/ (Accessed December 12, 2023).
Tricase C., Amicarelli V., Lamonaca E., Roberto Leonardo R. (2018b). “Economic analysis of the barley market and related uses,” in Grasses as Food and Feed (London, UK: IntechOpen). doi: 10.5772/intechopen.78967
Tricase C., Lamonaca E., Ingrao C., Bacenetti J., Lo Giudice A. (2018a). A comparative Life Cycle Assessment between organic and conventional barley cultivation for sustainable agriculture pathways. J. Clean. Prod. 172, 3747–3759. doi: 10.1016/j.jclepro.2017.07.008
Vinci G., Ruggieri R., Ruggeri M., Zaki M. G. (2022). “Application of Life Cycle Assessment (LCA) to cereal production: an overview,” in IOP Conference Series: Earth and Environmental Science, Vol. 1077. 012004 (IOP Publishing). doi: 10.1088/1755-1315/1077/1/012004
Wang K., Fu B. X. (2020). Inter-relationships between test weight, thousand kernel weight, kernel size distribution and their effects on durum wheat milling, semolina composition and pasta processing quality. Foods 9, 1308. doi: 10.3390/foods9091308
Keywords: Hordeum vulgare, hybrid, cropping system, grain yield, mycotoxins, sustainability
Citation: Scapino M, Meloni R and Blandino M (2025) A comparison of the agronomic management of a winter barley hybrid and a conventional genotype: effect of the seeding rate, soil tillage and nitrogen fertilization. Front. Agron. 7:1546989. doi: 10.3389/fagro.2025.1546989
Received: 17 December 2024; Accepted: 11 February 2025;
Published: 07 March 2025.
Edited by:
Ioannis Roussis, Agricultural University of Athens, GreeceReviewed by:
Giovanni Cabassi, Council for Agricultural Research and Agricultural Economy Analysis | CREA, ItalyCopyright © 2025 Scapino, Meloni and Blandino. This is an open-access article distributed under the terms of the Creative Commons Attribution License (CC BY). The use, distribution or reproduction in other forums is permitted, provided the original author(s) and the copyright owner(s) are credited and that the original publication in this journal is cited, in accordance with accepted academic practice. No use, distribution or reproduction is permitted which does not comply with these terms.
*Correspondence: Massimo Blandino, bWFzc2ltby5ibGFuZGlub0B1bml0by5pdA==
Disclaimer: All claims expressed in this article are solely those of the authors and do not necessarily represent those of their affiliated organizations, or those of the publisher, the editors and the reviewers. Any product that may be evaluated in this article or claim that may be made by its manufacturer is not guaranteed or endorsed by the publisher.
Research integrity at Frontiers
Learn more about the work of our research integrity team to safeguard the quality of each article we publish.