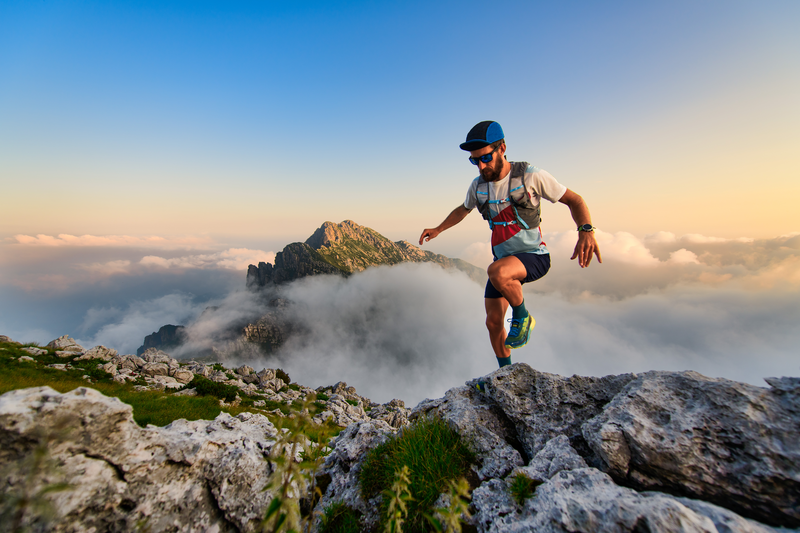
94% of researchers rate our articles as excellent or good
Learn more about the work of our research integrity team to safeguard the quality of each article we publish.
Find out more
ORIGINAL RESEARCH article
Front. Agron.
Sec. Plant-Soil Interactions
Volume 7 - 2025 | doi: 10.3389/fagro.2025.1535871
The final, formatted version of the article will be published soon.
You have multiple emails registered with Frontiers:
Please enter your email address:
If you already have an account, please login
You don't have a Frontiers account ? You can register here
Intercropping and phosphorus application are effective ways to increase crop yield and improve cultivated land quality. This study took the soil under the maize-peanut intercropping system which has been planted for 12 years as the research object, the physical, chemical, and electrochemical properties of soil, and crop yield under different planting patterns (sole-crop maize (SM), sole-crop peanut (SP), and maize-peanut intercropping (M/P)) and phosphorus application rates (P application (180 kg P2O5 ha−1) and no P application (0 kg P2O5 ha−1)) were studied. The results showed that intercropping increased soil aggregate stability, clay content, and gas phase ratio, P application further optimized the soil physical properties. At the same time, intercropping decreased soil pH and EC, increased soil organic carbon (SOC), total nitrogen (TN), and total phosphorus (TP) contents. P application increased soil pH, SOC, TN, TP, and EC. Compared with monoculture, intercropping increased the surface charge number (SCN) by 19.98%, specific surface area (SSA) by 44.34%, surface charge density (σ0) by 38.08, electric field strength (E0) by 38.22, and Zeta potential by 46.85%. P application further increased the SCN by 20.75%, SSA by 23.43%, σ0 by 67.82%, E0 by 67.13%, and Zeta potential by 15.51%. Maize-peanut intercropping increased the total crop yield of the intercropping system, the application of phosphate fertilizer further increased the yield of maize and peanut. The aggregate stability and nitrogen were significantly positively correlated with crop yield, and the carbon, phosphorus, and electrochemical properties were extremely significantly positively correlated with crop yield.
Keywords: Intercropping system, Phosphatic fertilizer, Surface charge number, specific surface area, Zeta Potential
Received: 28 Nov 2024; Accepted: 11 Mar 2025.
Copyright: © 2025 Ma, Yu, Zhao, Kou and Jiao. This is an open-access article distributed under the terms of the Creative Commons Attribution License (CC BY). The use, distribution or reproduction in other forums is permitted, provided the original author(s) or licensor are credited and that the original publication in this journal is cited, in accordance with accepted academic practice. No use, distribution or reproduction is permitted which does not comply with these terms.
* Correspondence:
Rentian Ma, College of Agriculture, Henan University of Science and Technology, Luoyang, China
Disclaimer: All claims expressed in this article are solely those of the authors and do not necessarily represent those of their affiliated organizations, or those of the publisher, the editors and the reviewers. Any product that may be evaluated in this article or claim that may be made by its manufacturer is not guaranteed or endorsed by the publisher.
Research integrity at Frontiers
Learn more about the work of our research integrity team to safeguard the quality of each article we publish.