- 1Department of Crop, Soil, and Environmental Sciences, University of Arkansas, Fayetteville, AR, United States
- 2Univeristy of Arkansas Division of Agriculture, Lonoke, AR, United States
Florpyrauxifen-benzyl is a new synthetic auxin herbicide labeled in rice with a broad spectrum of control, typically more potent on broadleaf weeds. It has garnered some interest as a potential broadleaf weed control option for use in corn at low rates. Field experiments were conducted in Fayetteville, Arkansas, from 2019 through 2021 to examine the response of corn to postemergence applications of florpyrauxifen-benzyl at three rates (7.5, 15, and 30 g ae ha-1), as well as mixtures of the herbicide with atrazine (at half or full rate), mesotrione, or a combination of atrazine (at half rate) and mesotrione. Injury and yield response varied among years, possibly attributed to temperature and solar radiation variations following treatment application. Three weeks after application (WAA), stand-alone florpyrauxifen-benzyl or mixtures caused incrementally greater injury with increasing rates (5% to 76% injury). The levels of early injury were largely sustained at 7 WAA, with a few instances of recovery, particularly with a mixture of florpyrauxifen-benzyl at 7.5 g ae ha-1 with the full rate of atrazine. Corn yield loss generally surpassed the respective injury levels. The yield loss was overall the least in 2020 (8 to 46%) and most severe in 2021 (26 to 93%), largely depending on florpyrauxifen-benzyl rates. Averaged across years, the full rate of atrazine mixed with florpyrauxifen-benzyl at 7.5 g ae ha-1 caused less yield loss (12%) compared to the stand-alone application (28% yield loss). These results suggest that using florpyrauxifen-benzyl in corn, even at low rates and/or in a mixture with atrazine/mesotrione, can cause immediate and sustained injury, often leading to greater than 10% yield reduction. Further research could explore in-crop, in-chemistry, or in-application technology opportunities for mitigating this inadequate safety to render this novel tool a viable option for use in corn.
1 Introduction
The rapid emergence of weed resistance issues has spurred innovative research in several weed management domains, to a great extent, compelling the imperative for an expanded repertoire of herbicidal diversity in cropping systems. Such efforts include exploring new possibilities and evaluating the potential of existing herbicides for application in novel crops. Florpyrauxifen-benzyl, a member of the synthetic auxins (WSSA Group 4), pyridine carboxylic acids (6-arylpicolinates) class, has been commercialized as a postemergence rice (Oryza sativa) herbicide by Dow AgroSciences, LLC (now known as Corteva AgriscienceTM) (Yerkes et al., 2014). Studies have already established the weed control benefits of florpyrauxifen-benzyl. Florpyrauxifen-benzyl controls both dicot and monocot plant species (Busi et al., 2018; Tresch and Grossmann, 2003) but offers natural tolerance to rice. According to Barber et al. (2022), assuming the weed densities are uniformly distributed in a rice field in the mid-southern U.S., florpyrauxifen-benzyl would offer the greatest value among available herbicide options. Studies have reported that monocot crops, as well as weeds, are relatively less sensitive to florpyrauxifen-benzyl compared to dicot species (Miller and Norsworthy, 2018a; Wang et al., 2021). In the context of corn [Zea mays (L.)], greenhouse research found that florpyrauxifen-benzyl at 3 g ae ha-1 did not result in any growth effect (Miller and Norsworthy, 2018b). It is worth investigating if such differential sensitivity to florpyrauxifen-benzyl could be exploited for targeted control of highly sensitive broadleaf weeds in corn, especially rates as low as 7.5 g ae ha-1. Additionally, understanding the tolerance level of corn to florpyrauxifen-benzyl could provide baseline information for future endeavors such as structural modifications of the herbicide, genetic improvement of the crop, or the implementation of other crop-safening measures to enhance its selectivity in the crop.
While other auxin herbicides such as 2,4-D and dicamba can be used in corn, a high affinity for the auxin F-Box 5 (AFB5) indole-3-acetic acid (IAA) co-receptor, sets florpyrauxifen-benzyl apart (Lee et al., 2014; Prusinska et al., 2022; Walsh et al., 2006). The typical use rate in various crops ranges sixfold (5 to 30 g ae ha-1), suggesting its potential utility at very low rates for targeted species (Herrera et al., 2021). Given its safety on rice and the need to control relatively more tolerant Echinochloa species, the use rate of florpyrauxifen-benzyl in rice typically ranges from 15-30 g ae ha-¹. In rice systems, grasses are problematic. Additionally, when applied to less than 10-cm tall Plamer amaranth plants in rice, sequential applications at 8 g ae ha−1 were found to be as effective as the sequential applications at 30 g ae ha-1 (Beesinger et al., 2022). Although there is an explicit need for research to evaluate its weed control efficacy in corn at low rates for broadleaf control, the primary concern is the safety on corn. Broadleaf weeds, particularly Palmer amaranth, have evolved resistance to a wide array of herbicide chemical classes, requiring broader chemical interventions in corn systems to mitigate ongoing herbicide selection pressure. The available knowledge on Palmer amaranth sensitivity, combined with corn being a monocot crop and the need for greater herbicide diversity, warrants research to investigate whether differential sensitivity manifests in the utility of florpyrauxifen-benzyl.
The distinct chemical structure of florpyrauxifen-benzyl not only enables it to provide a novel site of action and undergo a different metabolic pathway compared to other synthetic auxin herbicides, such as 2,4-D and dicamba, but also allows for the control of weeds that have evolved target-site resistance to these herbicides and potentially non-target site resistance as well. As a result, florpyrauxifen-benzyl can play a pivotal role as a herbicide in corn cropping systems, providing an additional tool for weed management. Despite the existence of a diversified portfolio of herbicides (Barber et al., 2022) and herbicide resistance traits in corn, either naturally present, conventionally bred, or developed through extensive genetic engineering efforts (Green and Owen, 2011), the available options are not yet considered adequate for effective resistance management (Gaines et al., 2021). According to the United States Department of Agriculture (USDA, 2023), a two-year rotation of corn and soybean [Glycine max (L.) Merr.] is one of the most adopted crop rotations, covering most of the acreage of these two crops. Total area under cultivation for corn and soybean in 2023 was 38.1 and 33.8 million ha, respectively. However, the herbicide-resistant technologies for these crops overlap in traits, limiting the full scope of herbicide rotation. Both crops often utilize similar herbicide-resistant traits, such as resistance to glyphosate and glufosinate. This overlap means that the same herbicides are frequently used in both crops, reducing the diversity of herbicide modes of action employed in the rotation. Consequently, this can lead to increased selection pressure for herbicide-resistant weed populations, as the weeds are exposed to the same herbicides year after year. To effectively manage herbicide resistance, it is critical to incorporate herbicides with different modes of action and to explore new herbicidal options to diversify weed management strategies in these systems. While there may still be considerable rotational flexibility among herbicides within this cropping system, the overlap in the herbicide spectrum coupled with the widespread presence of weed resistance to herbicides constrains the significance of herbicide diversity employed.
Atrazine and mesotrione are common corn herbicides and are often applied together. Atrazine is a photosystem II (PSII)-inhibitor (WSSA Group 5) and mesotrione is a hydroxyphenyl pyruvate dioxygenase (HPPD)-inhibitor (WSSA Group 27), both causing the production of reactive oxygen species (ROS) under light in the plant system. Studies have shown that the interactions of PSII and HPPD inhibitors can result in synergistic outcomes for phytotoxicity in sensitive weeds (Abendroth et al., 2006; Armel et al., 2005; Kim et al., 1999). Corn, however, has excellent tolerance to atrazine and mesotrione, as they are rapidly converted into nontoxic forms via hydroxylation and/or N-dealkylation pathways (Ma et al., 2021; Shimabukuro, 1968). In rice, florpyrauxifen-benzyl exhibits excellent flexibility in various herbicide mixtures, including propanil, a PSII-inhibitor (Miller and Norsworthy, 2018a). The objectives of this study were to explore the potential utility of florpyrauxifen-benzyl in corn and investigate how the plant-herbicide interaction manifests in corn growth and yield when florpyrauxifen-benzyl, a systemic herbicide with low dissociation constant, is applied in combinations with specific light-dependent, and ROS-generating corn herbicides such as atrazine and/or mesotrione. This study focuses on the injury and yield response of corn to florpyrauxifen-benzyl, with an emphasis on the need for further research to evaluate its weed control efficacy at reduced rates in this crop.
2 Materials and methods
Field experiments were carried out in Fayetteville, Arkansas, during the corn growing season in 2019, 2020, and 2021 on a Captina silt loam soil under irrigated conventional till conditions. Corn planting, herbicide application dates, cumulative precipitation, and general weather conditions (seasonal and following treatment applications) for each year are presented in Table 1 and Figure 1. The experiment was established using a factorial arrangement of florpyrauxifen-benzyl rates and mix partners in a randomized complete block design with four replications with plot sizes of four rows wide and 9 m long. A glyphosate- and glufosinate-resistant corn hybrid, DKC 62-52 RIB (Dekalb brand, Bayer Crop Sciences), was planted 2.5- to 3-cm deep at a seeding rate of 69,000 seeds ha−1 with a 91-cm-wide row spacing.
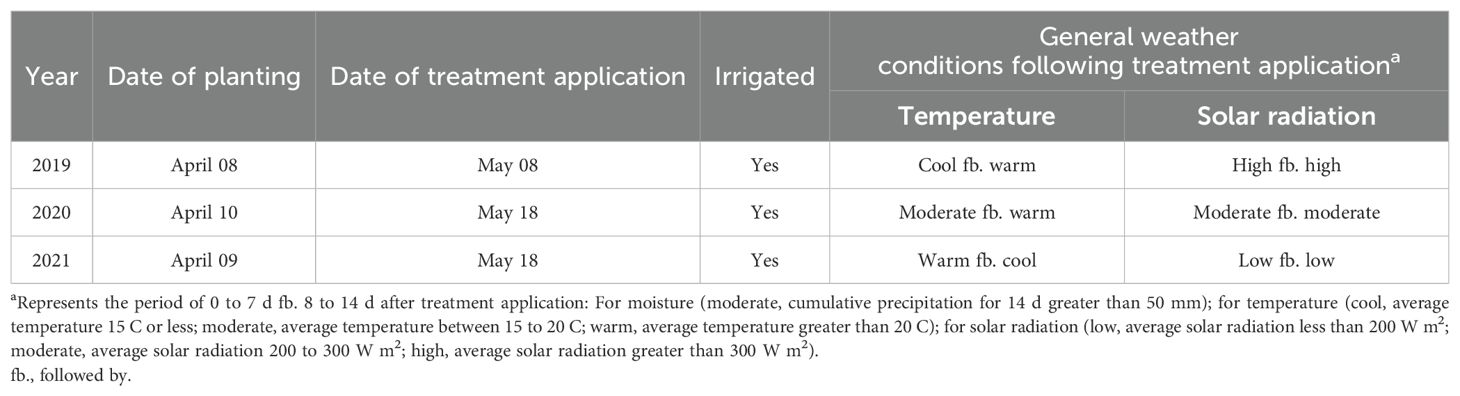
Table 1. Corn planting dates, treatment application dates, cumulative precipitation, and weather conditions for each year of experiment in Fayetteville, AR.
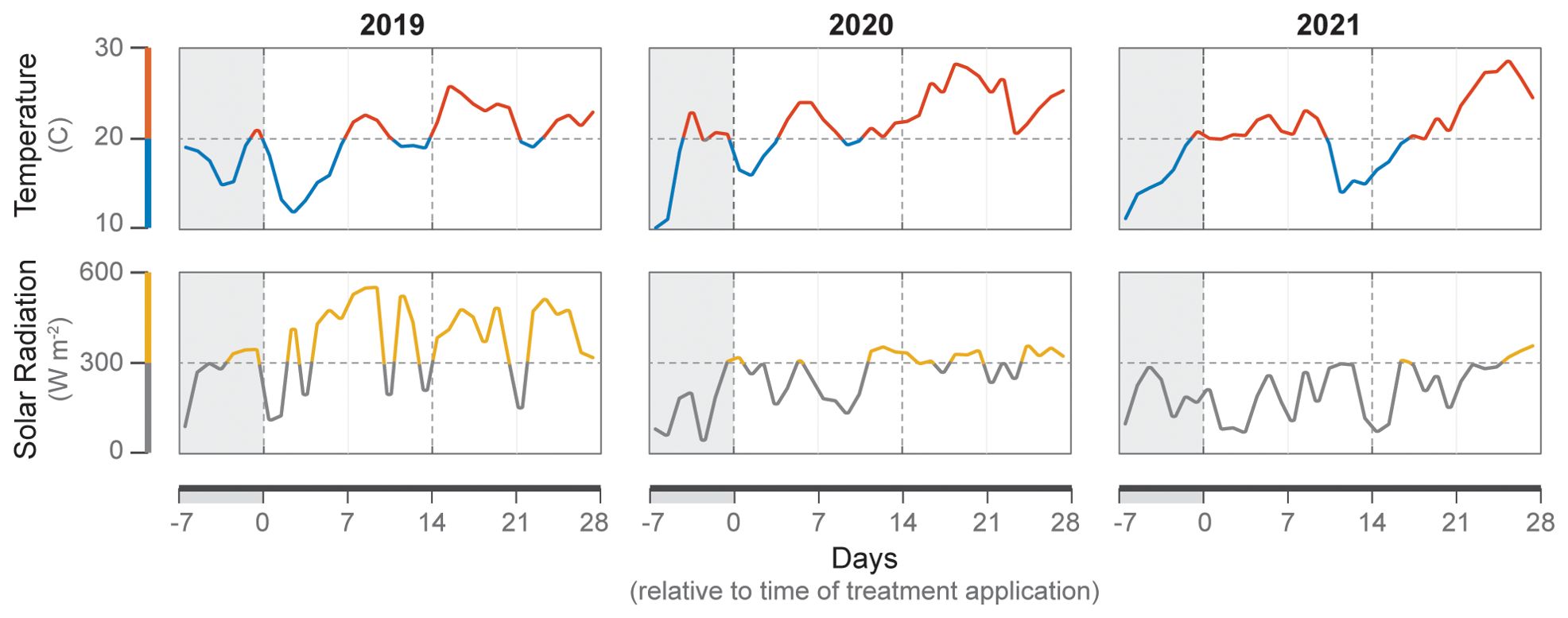
Figure 1. Air temperature and solar radiation before 7 d and following 28 d after treatment application in 2019, 2020, and 2021 in Fayetteville, AR.
Treatments consisted of three rates of florpyrauxifen-benzyl, 7.5, 15, or 30 g ae ha-1, alone or in mixture with atrazine at 0.5X or 1X (1,120 g ai ha-1), or mesotrione (105 g ai ha-1) or combination of the 0.5X rate of atrazine and mesotrione. A nontreated check was included to compare the assessments. Treatments were applied to two center rows of corn in four-row plots at the three- to four-leaf stage (V3-V4). Treatments were applied using a CO2-pressurized backpack sprayer calibrated to deliver 140 L ha-1 at 166 kPa fitted with AIXR 110015 flat-fan nozzles (TeeJet®; Spraying Systems Co., Wheaton, IL). As the evaluation of weed control efficacy was not the objective of the study and to avoid compound effect of weeds on corn beyond the effect of florpyrauxifen-benzyl, other herbicides were used as part of the standard weed management practices. The entire experimental area was applied with atrazine, S-metolachlor, and glyphosate at planting and a premix of S-metolachlor + glyphosate + mesotrione at V6 to V8 stage of corn to keep research plots nearly weed-free. Information on herbicides used in treatments, as well as other herbicides used for in-crop weed management, is presented in Table 2. Fertility and pest management were maintained in all sites following the University of Arkansas System Division of Agriculture Cooperative Extension Service recommendations (Faske et al., 2022; Studebaker et al., 2022).
Visible injury ratings were based on a composite assessment of chlorosis, necrosis, malformation, leaf rolling, and stunting on the aboveground plant parts and were performed 1, 2, 3, 5, 6, and 7 wk after application (WAA). The injury ratings were based on a scale of 0% (no injury, which is equivalent to the nontreated control) to 100% (complete plant death). Two center rows of corn were harvested at crop maturity using a plot combine, and grain yield was adjusted to 15% moisture. Yield data were converted to a percentage of the nontreated control.
Statistical analysis was performed using R statistical software version 4.3.3 (R Core Team, 2024). Corn injury and yield loss data were fit to generalized linear mixed-effect models (GLMM) using glmmTMB package (function glmmTMB) with beta (link = “identity”) error distributions (Brooks et al., 2017; Stroup, 2015). First, the data were analyzed to evaluate the interactions between treatments (each combination of florpyrauxifen-benzyl rate and mix partner) and year for injury and yield data. If significant, the data were analyzed separately for each year. For evaluation timings (all six), treatments and evaluation timings, and their interaction were considered fixed effects, while block was a random effect in the models with a first-order autoregressive structure on the evaluation timings (repeated measure). For injury at 3 WAA and 7 WAA, and yield loss data, the levels of florpyrauxifen-benzyl rate, mix partner, and their interaction were considered fixed effects, while block was a random effect in the models. ANOVA was performed on fitted models using CAR package (Fox and Weisberg, 2019) with Type III Wald chi-square tests. Estimated marginal means (EMMs) (Searle et al., 1980) for the treatments were obtained using EMMEANS package (Lenth, 2022), with separate EMMs for each rate of florpyrauxifen-benzyl. The Sidak method was used to adjust for multiple comparisons. A compact letter display (CLD) was generated using MULTCOMP package (Hothorn et al., 2008) to visually represent which groups were significantly different from each other based on the results of multiple comparison tests. Asymptotic lower and upper limits at the confidence level of 95% were also generated for the EMMs.
In addition to the repeated measure analysis, ternary plots were generated to provide a clear means of visualizing the data from injury evaluation timings and to elucidate patterns in the injury progression or recovery. Similarly, the proportional relationships among injury at 3 WAA, injury at 7 WAA, and corn yield loss were also visualized using a ternary plot.
The relationship between the florpyrauxifen-benzyl rate (as a numerical variable) and yield loss was modeled using linear regression. The nls function in R was used to fit the models, which uses an iterative algorithm to estimate the coefficients by minimizing the sum of squared errors. The logarithmic model was specified as yield loss ~ a * log (florpyrauxifen-benzyl rate) + b, where a represents the slope of the line, while b represents the y-intercept. Initial values for the coefficients were provided as start = list (a = 1, b = 1), which are required by the nls function. A separate model was fitted for each year to account for variations in the relationship across years.
3 Results and discussion
Temperature and amount of solar radiation for the period of 7 d before and 28 d following the application of the treatments are shown in Figure 1. Irrigation, either furrow or overhead, was employed weekly when rainfall was <2.5 cm to prevent moisture stress during the growing season. The potential role of these covariates is acknowledged while interpreting treatment effects on corn injury and yield loss.
3.1 Overview of injury development
The repeated measure analysis (6 evaluation timings) for each year revealed a significant effect of treatments (P < 0.001) and evaluation timings (P < 0.001); however, there was also a two-way interaction (P < 0.01), complicating interpretations. Therefore, ternary plots encompassing plot-level injury data were generated to elucidate the dynamic course of injury development (Figure 2). These insights went beyond the scope of numerical results alone. Subsequent sections provide further statistical analysis and context tailored to the injury evaluation timings at 3 and 7 WAA.
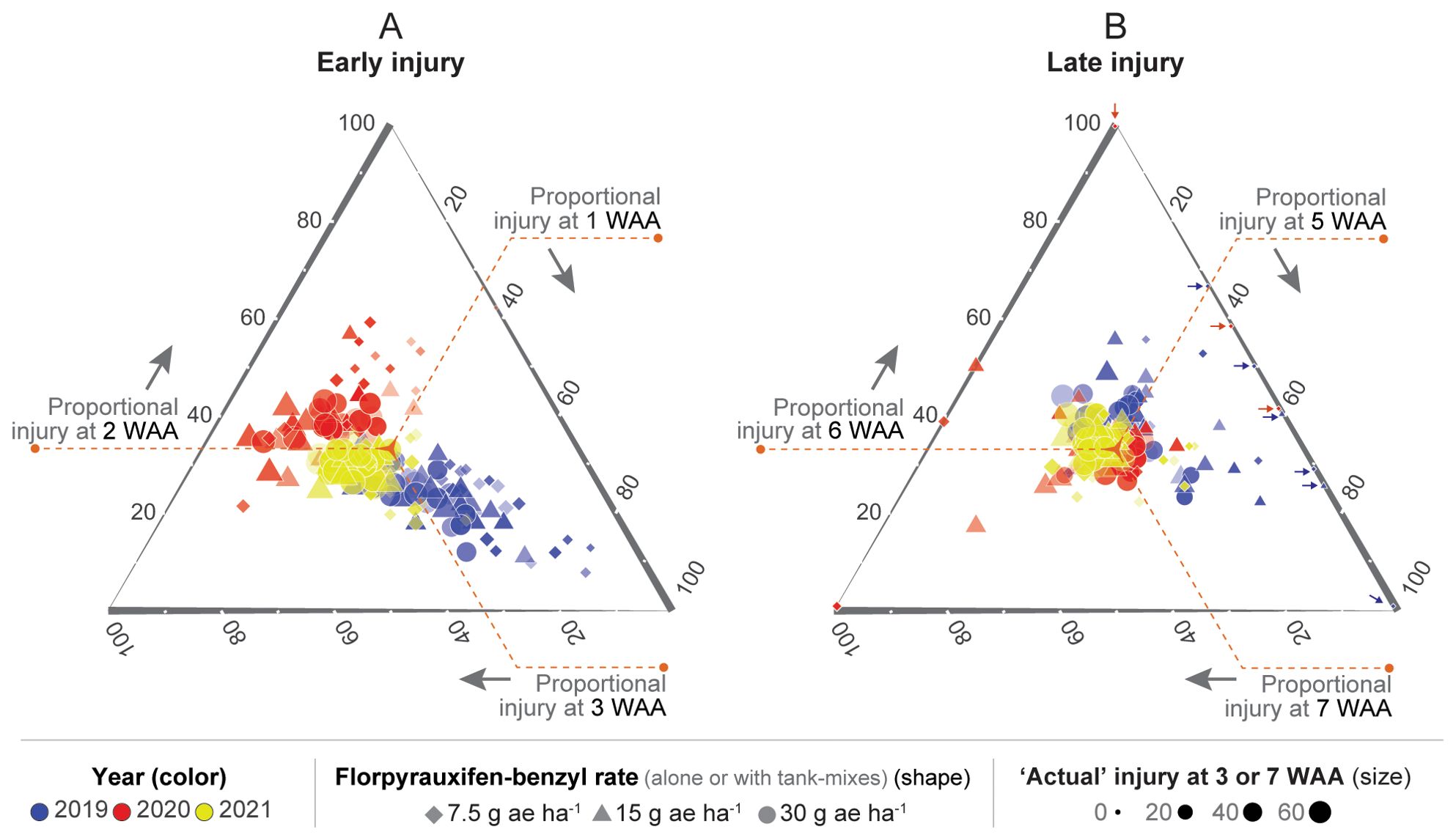
Figure 2. Ternary plots illustrating relationships among (A) early injury evaluation timings at 1, 2, or 3 WAA and (B) late injury evaluation timings at 5, 6, or 7 WAA. Points within the triangle represent proportion of the injury by florpyrauxifen-benzyl rates at three evaluation timings (totaling 100) and are color-coded by year.
In the ternary plot, the ‘early injury’ involves evaluations at 1, 2, and 3 WAA (Figure 2A), where a clear injury pattern emerged across different years. In 2019, most treatments caused proportionally higher injury 1 WAA, suggesting rapid recovery, particularly notable with florpyrauxifen-benzyl at 7.5 g ae ha-1. In contrast, injury ratings were generally greater for the later evaluation timings compared to 1 WAA in 2020. There was only a minimal disparity in injury among the evaluation timings in 2021.
The ‘late injury’ comprises evaluations at 5, 6, and 7 WAA (Figure 2B). Injury ratings in most plots recovered only subtly, represented by the size of the data points for 7 WAA. In a few instances in 2019 and 2020, particularly with florpyrauxifen-benzyl at 7.5 or 15 g ae ha-1, injury recovered by 7 WAA.
3.2 Injury and crop yield
There was a three-way interaction among year, florpyrauxifen-benzyl rate, and its mix partner for corn injury (at 3 WAA or 7 WAA) and yield data (Table 3). Therefore, injury and yield data were analyzed separately by year. Except for the yield in 2020, there was a two-way interaction for florpyrauxifen-benzyl rate and the mix partner. Although the presence of an interaction precludes a concrete interpretation, the main effects of florpyrauxifen-benzyl and the mix partner are shown in Table 4 primarily to offer insights into the relative importance of these two factors. The impact of the florpyrauxifen-benzyl rate was greater with the increasing rate of the herbicide, and the effect was prominent over the effect of the mix partner. Mixtures with florpyrauxifen-benzyl compared to the stand-alone application resulted in inconsistent effects across year and/or evaluation timings, especially on corn injury. To simplify further analysis, the effect of mixtures is compared within a specific florpyrauxifen-benzyl rate.
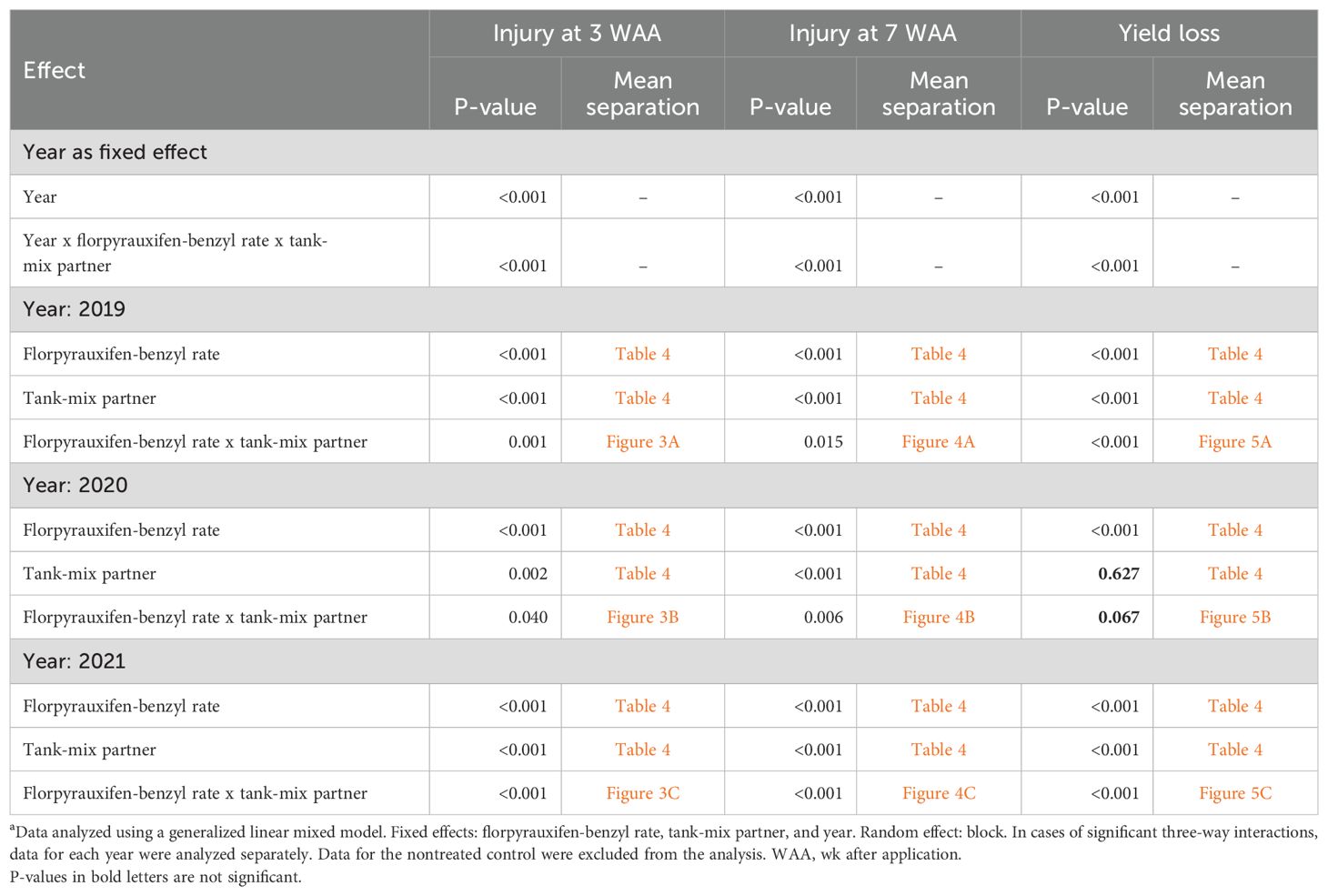
Table 3. Results of ANOVA (Type III Wald chi-square tests) for the effects of year, florpyrauxifen-benzyl rate, tank-mix partner, and their interactions on corn injury at 3 and 7 WAA, and yield loss data using a generalized linear mixed modela.
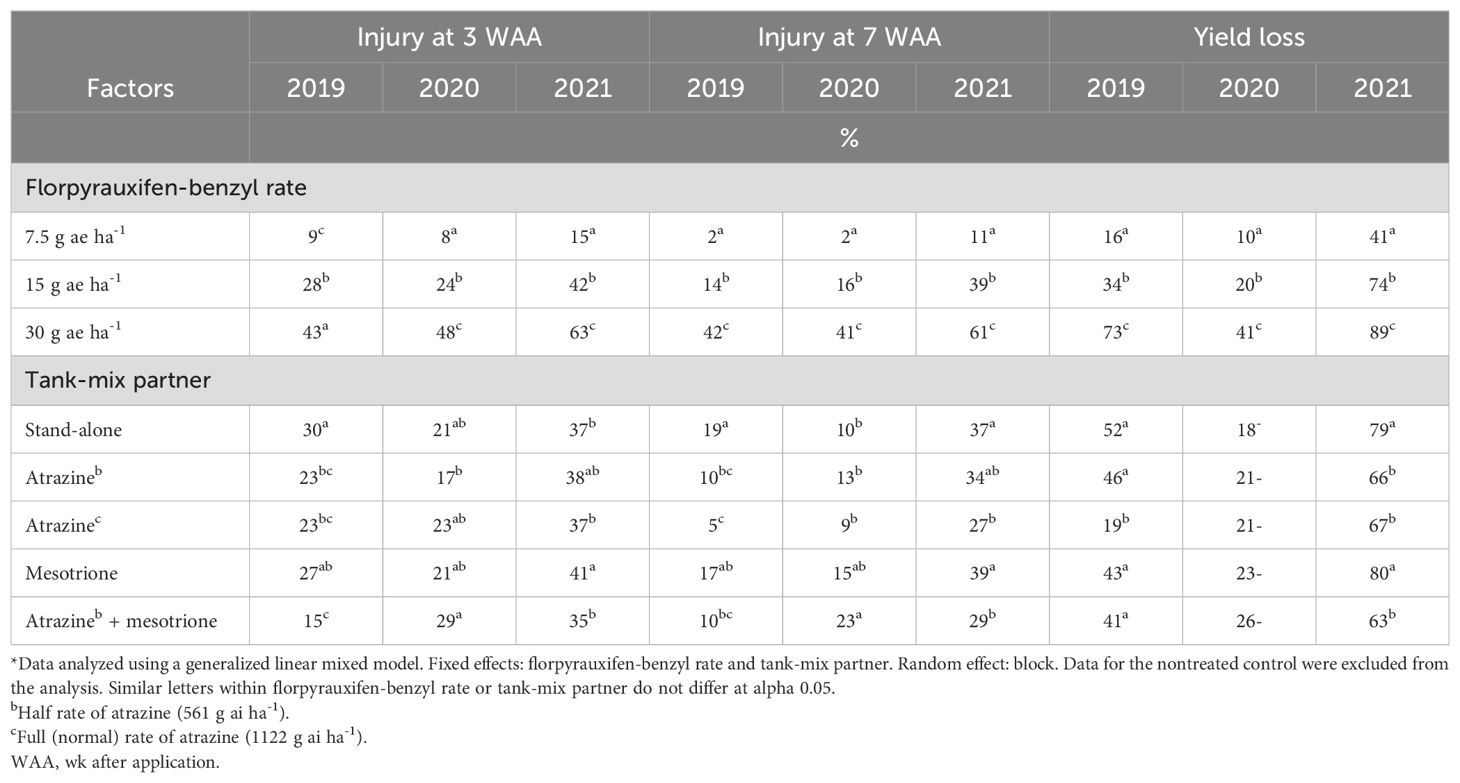
Table 4. Mean separation of the main effects of florpyrauxifen-benzyl rate and tank-mix partner on corn injury at 3 and 7 WAA, and yield loss data for 2019, 2020, and 2021.
3.2.1 Injury at 3 WAA
The effect of tank-mix partners varied only for florpyrauxifen-benzyl at 7.5 and 15 g ae ha-1 (P < 0.001). With the 7.5 g ae ha-1 rate, atrazine and mesotrione mixtures caused slightly less injury (2% injury) compared to florpyrauxifen-benzyl alone or its other mixtures (11 to 15% injury) (Figure 3A). The stand-alone florpyrauxifen-benzyl at 15 g ae ha-1 caused greater injury (35% injury) compared to its mixture with the full rate of atrazine (21% injury), but not compared to other mixtures (24 to 33% injury). On average, florpyrauxifen-benzyl at 30 g ae ha-1 caused 43% corn injury.
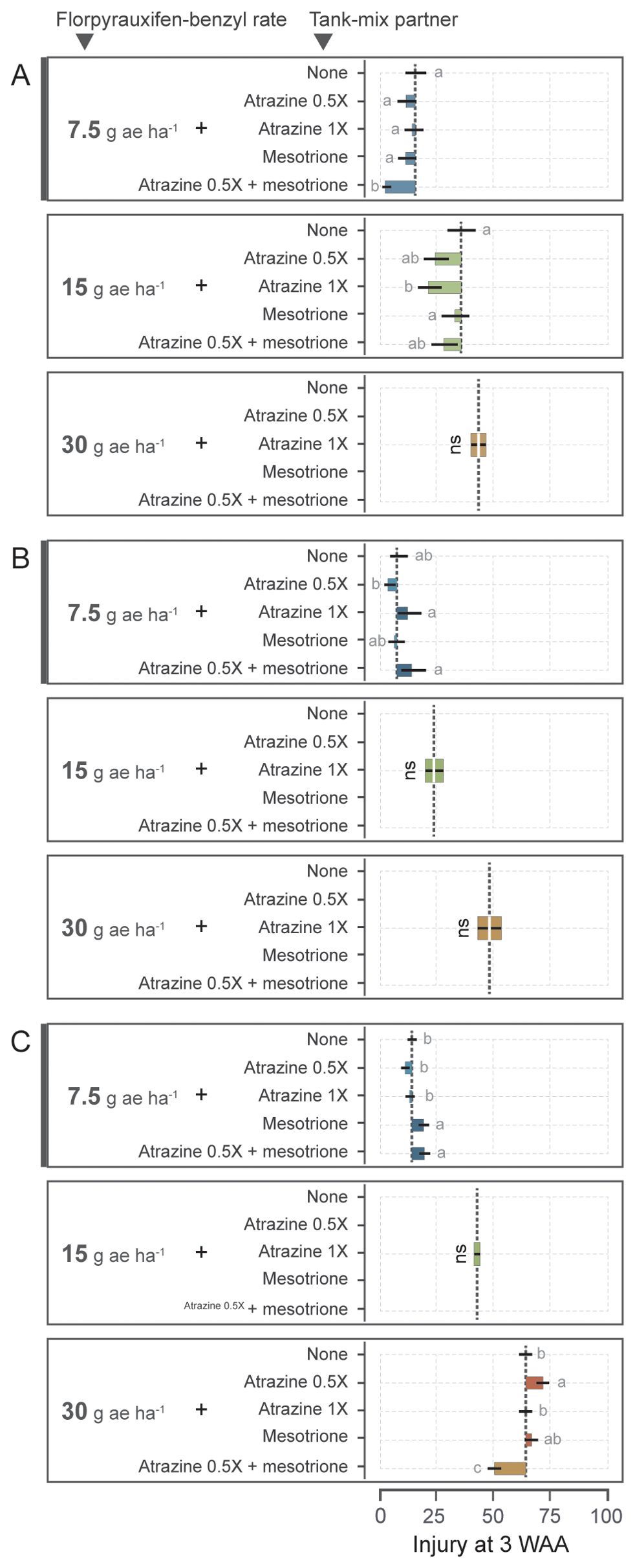
Figure 3. Visible injury of corn to florpyrauxifen-benzyl rates and mixtures 3 WAA in (A) 2019, (B) 2020, (C) 2021 in Fayetteville, AR. The end of the bars represents the estimated marginal means of the injury data with an asymptotic 95% confidence interval (represented by lines) for the means. Means with similar letters within florpyrauxifen-benzyl rates do not differ. Atrazine 0.5X = 560 g ai ha-1; Atrazine 1X = 1120 g ai ha-1. WAA, wk after application.
The effects of tank-mix partners varied only for florpyrauxifen-benzyl at 7.5 g ae ha-1 in 2020 (Figure 3B, P = 0.002). None of the tank-mix applications caused injury levels different from the stand-alone florpyrauxifen-benzyl application (3 to 14% injury). Injury with the half rate of atrazine tank-mix was less (3% injury) than its full rate or combination with mesotrione (12 to 14% injury). On average, florpyrauxifen-benzyl at 15 and 30 g ae ha-1 caused 24 and 48% injury, respectively.
In 2021, corn injury varied among tank-mix partners for florpyrauxifen-benzyl at 7.5 and 30 g ae ha-1 (Figure 3C, P < 0.002). At the 7.5 g ae ha-1 rate, mesotrione-containing mixtures caused greater injury (19% injury) than the stand-alone florpyrauxifen-benzyl or the other mixtures (11 to 14% injury). On average, the 15 g ae ha-1 rate caused 42% injury to corn. Atrazine and mesotrione in combination with florpyrauxifen-benzyl at 30 g ha-1 caused 50% injury, and the injury was less compared to the stand-alone florpyrauxifen-benzyl or other tank-mix applications (64 to 71% injury).
Discrepancies in early corn injury among years might be partially attributed to prevailing temperature and/or solar radiation after treatment application, as discussed earlier. Velásquez et al. (2021) and Wright et al. (2021) observed greater injuries in rice under conditions of cooler temperature surrounding the florpyrauxifen-benzyl application. Greater injury in rice was also found to be associated with low solar radiation (Velásquez et al., 2021). Moreover, florpyrauxifen-benzyl (Loyant™) use in rice is associated with label recommendations that advise against usage under extreme cold or heat conditions (Anonymous, 2023). As a result, it is reasonable to infer that similar temperature-dependent effects might extend to corn. Regarding moisture, the average precipitation over the 28-d period following treatment application remained consistent across years (data not shown), and the crop was grown under irrigated conditions. Hence, its effect is assumed to be minimal. The interplay of processes thought to confer florpyrauxifen-benzyl selectivity in rice, such as esterase-facilitated bio-activation, herbicide degradation pathways, and receptor affinity at the site of action (Lee et al., 2014), and their potential manifestations in corn, is not known.
3.2.2 Injury at 7 WAA
In 2019, the injury was similar among the stand-alone application and the mixtures for florpyrauxifen-benzyl at 7.5 g ae ha-1 (1 to 3% injury) (Figure 4A). However, injury levels varied across the tank-mix partners for the 15 and 30 g ae ha-1 rates. At the 15 g ae ha-1 rate, the stand-alone application caused similar injury (15 to 30% injury) to its mixtures with mesotrione-containing tank-mix partners, but greater injury when compared to the full or the half rate of atrazine-containing mixtures (2 and 13% injury, respectively). The stand-alone application at the 30 g ae ha-1 rate caused greater injury only when compared to the tank-mix of the full rate of atrazine (55 vs 35% injury), and all tank-mix applications caused similar corn injury.
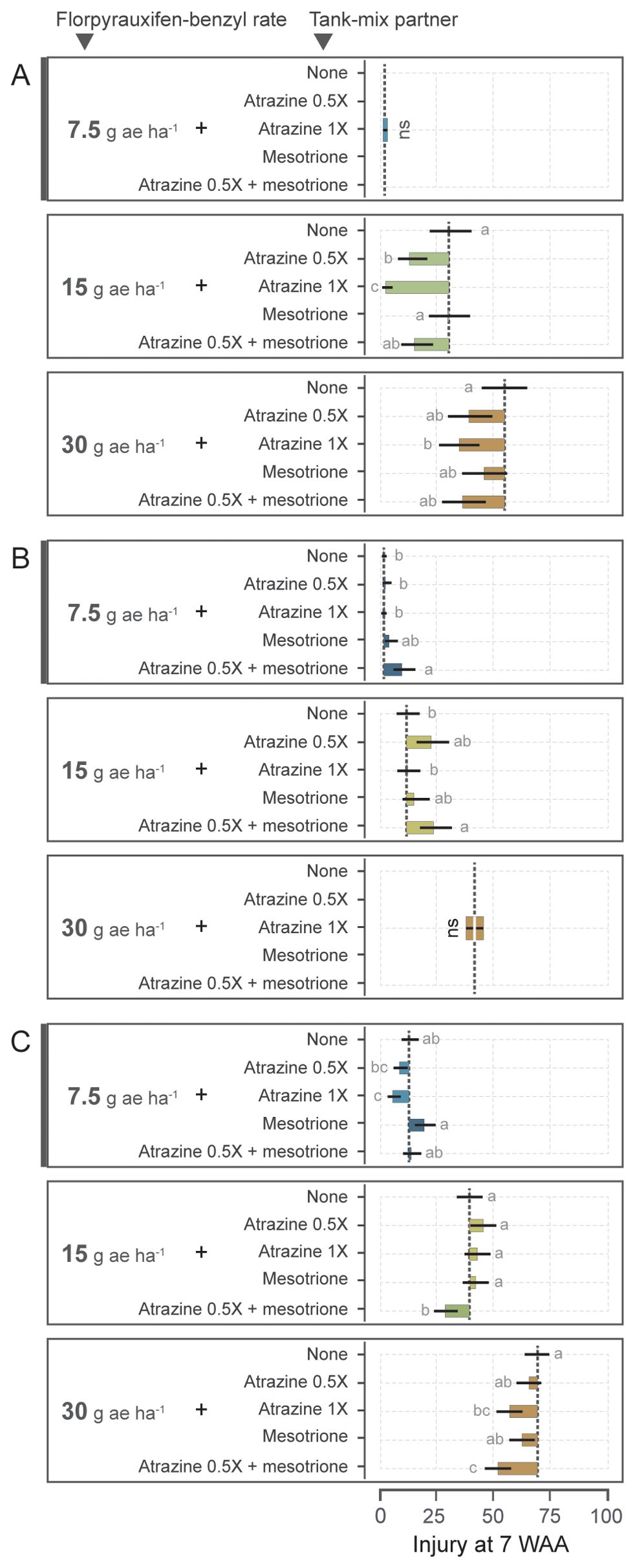
Figure 4. Visible injury of corn to florpyrauxifen-benzyl rates and mixtures 7 WAA in (A) 2019, (B) 2020, (C) 2021 in Fayetteville, AR. The end of the bars represents the estimated marginal means of the injury data with an asymptotic 95% confidence interval (represented by lines) for the means. Means with similar letters within florpyrauxifen-benzyl rates do not differ. Atrazine 0.5X = 560 g ai ha-1; Atrazine 1X = 1120 g ai ha-1. WAA, wk after application.
In 2020, the stand-alone florpyrauxifen-benzyl at 7.5 g ae ha-1 caused similar injury to its mixtures (1 to 4% injury), except when mixed with combinations of atrazine and mesotrione (9% injury) (Figure 4B). Similarly, the injury was greater (23% injury) with this tank-mix at the 15 g ae ha-1 rate, compared to the stand-alone florpyrauxifen-benzyl or its tank-mix with the full rate of atrazine (11 to 12% injury). On average, florpyrauxifen-benzyl at 30 g ae ha-1 caused 41% injury.
In 2021, injury levels varied across the tank-mix partners for all the rates of florpyrauxifen-benzyl (Figure 4C). At the 7.5 g ae ha-1 rate, injury with the stand-alone application of florpyrauxifen-benzyl or mesotrione-containing mixtures were greater (12 to 19% injury) compared to a tank mix with the full rate of atrazine (5% injury). However, injury with only the tank mix of the atrazine-mesotrione combination was less (28% injury) than with the stand-alone or the other mixtures (39 to 45% injury). At the 30 g ae ha-1 rate, the injury was greater with stand-alone florpyrauxifen-benzyl (69% injury) compared to its mixtures with the full rate of atrazine or atrazine-mesotrione combination (52 to 57% injury).
Overall, the effect of florpyrauxifen-benzyl rate and the year was prominent at 7 WAA as at 3 WAA. The effect of the tank-mix partner was more significant in 2019. Generally, the initial injury was sustained for most of the treatment combinations, except for the injuries that were initially very low. In sunflower, which is a highly sensitive crop, injuries observed within the first few weeks of application generally increased as time progressed after the application of florpyrauxifen-benzyl (Serim and Patterson, 2024). However, full recovery in rice, and subsequently no yield reduction, has been reported for initial injuries of less than 20% (Wells and Taylor, 2016; Wright et al., 2021). These results indicate that low initial injuries have the potential for complete recovery. In this study, florpyrauxifen-benzyl at a low rate caused relatively less injury, and instances of recovery were more probable when mixed with the full rate of atrazine. Generalized over three years, it becomes subtly apparent that when the full rate of atrazine is mixed with a low rate of florpyrauxifen-benzyl, the resulting effect on corn injury is antagonistic.
3.2.3 Crop yield
In 2019, yield loss with a stand-alone application of florpyrauxifen-benzyl at 7.5 g ha-1 did not differ from any tank mix applications (7 to 35% yield loss) (Figure 5A). However, yield loss with a tank-mix of atrazine or mesotrione (7 to 8% yield loss) was less than that of the tank-mix of the half rate of atrazine (35% yield loss). At the 15 g ae ha-1 rate, the effect of tank-mix partners was more pronounced, with less yield loss with the full rate of atrazine tank-mix (7% yield loss) or atrazine-containing mixtures (32 to 34% yield loss) compared to its stand-alone application (54% yield loss). The 30 g ae ha-1 rate, on average, caused a 67 to 82% yield loss. The effects of the tank-mix partner did not vary within any of the levels of florpyrauxifen-benzyl rate in 2020. Injury with florpyrauxifen-benzyl was 10%, 20%, and 41% for the rates of 7.5, 15, and 30 g ae ha-1, respectively (Figure 5B).
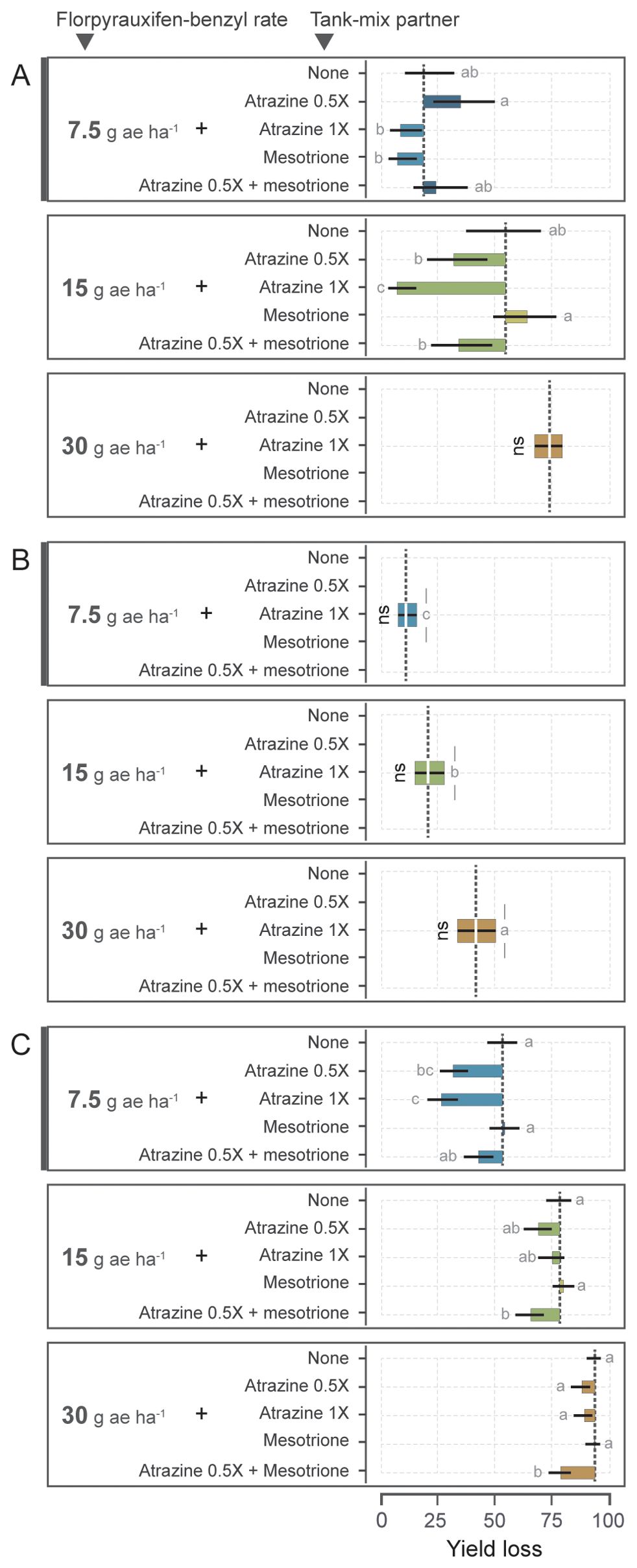
Figure 5. Yield response of corn to florpyrauxifen-benzyl rates and mixtures in (A) 2019, (B) 2020, (C) 2021, in Fayetteville, AR. Corn yield loss is expressed as a percentage of nontreated corn. The end of the bars represents the estimated marginal means of the injury data with an asymptotic 95% confidence interval (CI) for the means. Means with similar letters within florpyrauxifen-benzyl rates do not differ. Atrazine 0.5X = 560 g ai ha-1; Atrazine 1X = 1120 g ai ha-1.
In 2021, yield loss varied among tank-mix partners for all rates of florpyrauxifen-benzyl (Figure 5C). At the 7.5 g ae ha-1 rate, the half or full rate of the atrazine tank-mix caused less yield loss (26 to 31% yield loss) compared to its stand-alone application or when compared to mesotrione or atrazine-mesotrione tank-mix (42 to 54% yield loss). At the 15 g ae ha-1 rate, the stand-alone application caused similar yield loss to tank-mix applications (69 to 80% yield loss), except with the atrazine-mesotrione tank mix (65% yield loss). Similarly, with an overall greater yield loss compared to the 15 g ae ha-1 rate, the yield loss pattern was similar to the 30 g ae ha-1 rate that ranged from 88 to 93% for the stand-alone or tank mixes. The tank mix of atrazine-mesotrione combination caused only 78% yield loss.
Generally, florpyrauxifen-benzyl alone or its mixtures caused greater than 10% yield loss, and varied by year, the rate and tank-mix partner. Florpyrauxifen-benzyl can cause injury in rice, with severity depending on the cultivar, rate, and frequency of application (Wright et al., 2021). Such injury often leads to yield loss. Similarly, a yield loss proportional to the level of injury occurred in broadleaf crops such as soybean and sunflower (Miller and Norsworthy, 2018b; Serim and Patterson, 2024). Analyzed by year, there were instances of yield loss magnitude exceeding the injury level observed at both the early and late evaluation timings, and other instances of some recovery. The subsequent sections leverage visual representations of the data averaged across years to clarify these findings and concretize the conclusion.
3.3 Yield loss in relation to injury observed
The relationships among the levels of early injury (at 3 WAA), late injury (at 7 WAA), and yield loss for each combination of florpyrauxifen-benzyl rates and tank-mix partner averaged over years were visualized in a ternary plot (Figure 6). The plot reinforced the fact from Figure 2 that there was little or no difference between early and late injury for most treatments, indicating limited recovery. The proportion of yield loss was consistently higher across the treatments compared to the individual proportions of the injury evaluation timings, indicating a greater yield loss relative to the observed injury. When early injury, late injury, and yield loss were proportionally close, the ‘actual’ yield loss was predominantly greater, indicating no recovery and subsequent yield loss. These treatments consisted particularly of 15 or 30 g ae ha-1 rates of florpyrauxifen-benzyl, regardless of the tank-mix partners used. Additionally, florpyrauxifen-benzyl at 7.5 g ae ha-1 alone or most of its tank mixes caused the highest proportional corn yield loss, suggesting that despite lower injury with this rate of florpyrauxifen-benzyl, yield loss manifested with a proportionally greater amount. The only treatment for which corn recovered from the injury and resulted in a lesser amount of yield loss was the tank-mix of the full rate of atrazine. Overall, the results indicated that the prospects for recovery from injury in corn after exposure to florpyrauxifen-benzyl were limited. Previous research has shown that the yield response in corn was stronger than the initial injury observed at 2 WAA, and florpyrauxifen-benzyl at 30 g ae ha-1 caused greater than 70% yield loss (Godar et al., 2023). Any discernible recovery in this study manifested exclusively only with the low rate and, more prominently, when the full rate of atrazine was the tank-mix partner.
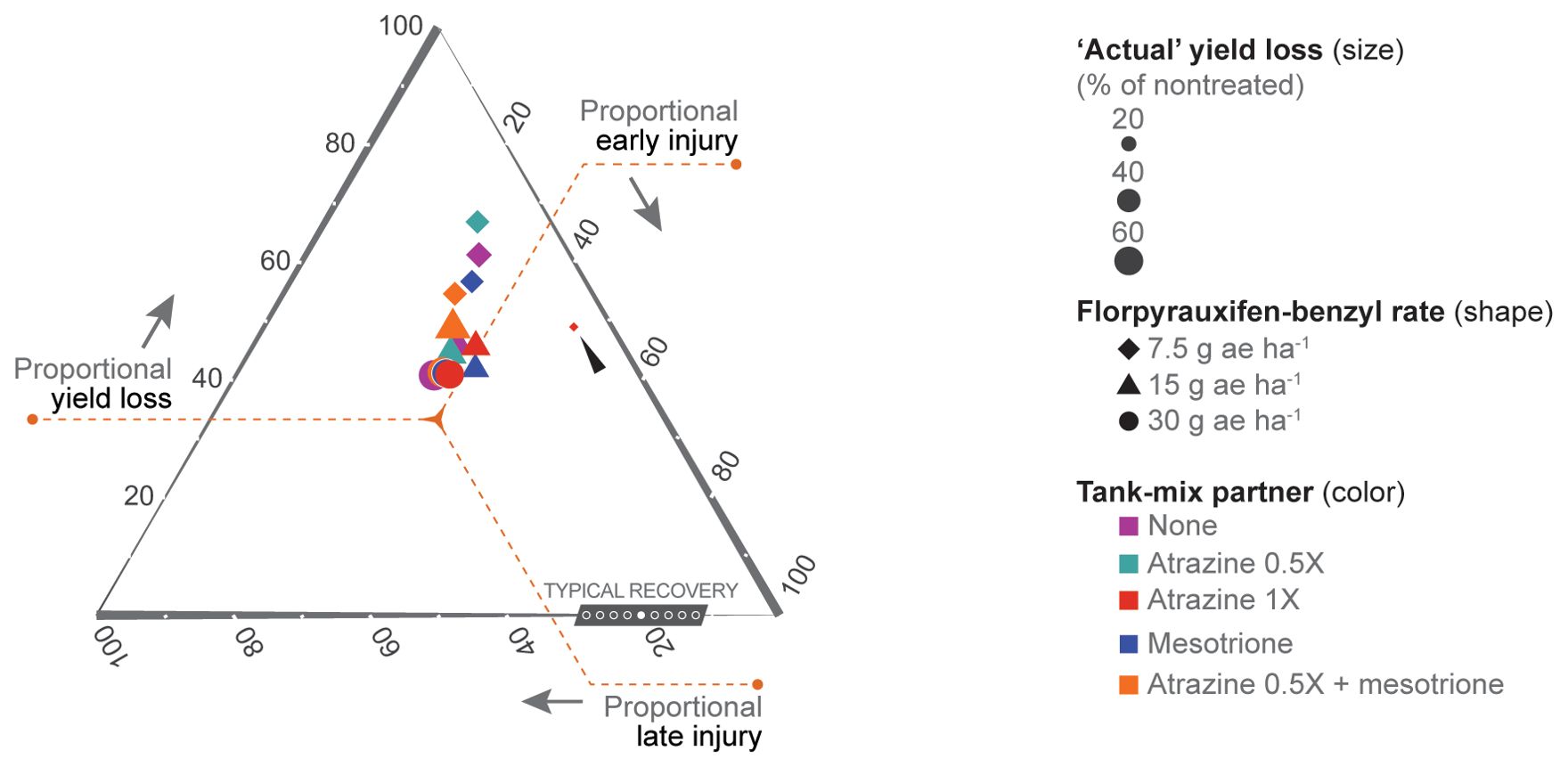
Figure 6. A ternary plot illustrating the relationships of early injury, late injury and yield loss of corn as affected by florpyrauxifen-benzyl rates and its mixtures in Fayetteville, AR. Data points are positioned based on the relative proportions of each variable (unique combination of florpyrauxifen-benzyl rates and its mixtures averaged over three years), with the point size indicating ‘actual’ yield loss. Atrazine 0.5X = 560 g ai ha-1; Atrazine 1X = 1120 g ai ha-1.
3.4 Potential rate range in relation to yield response
In the previous analysis, the effects of both the factors, florpyrauxifen-benzyl rates, and tank-mix partner, as well as their interactions, were examined on yield loss. In this analysis (Figure 7), the focus is on the main effect of florpyrauxifen-benzyl rates, and the analysis excludes those levels of tank-mix partner that caused differential response than the stand-alone florpyrauxifen-benzyl. As the year effect was prominent from the previous analyses, the relationship was explored separately for each year. While complementing the previous analysis, this analysis provides additional insights into how the relationship between the yield loss and florpyrauxifen-benzyl rates varied among years, and also helps draw a broader picture of how close the corn response is from the potential utility range of florpyrauxifen-benzyl rate. Based on the results of the fitted regression models, there are differences in the strength and intercept of this relationship across years, as indicated by the varying values of the slope a and b coefficients. Extrapolating the predicted response shows that the null effects of florpyrauxifen-benzyl rates range from 1.3 to 4.6 g ae ha-1, which is lower than its potential utility rate range (5 to 10 g ae ha-1) for use in corn.
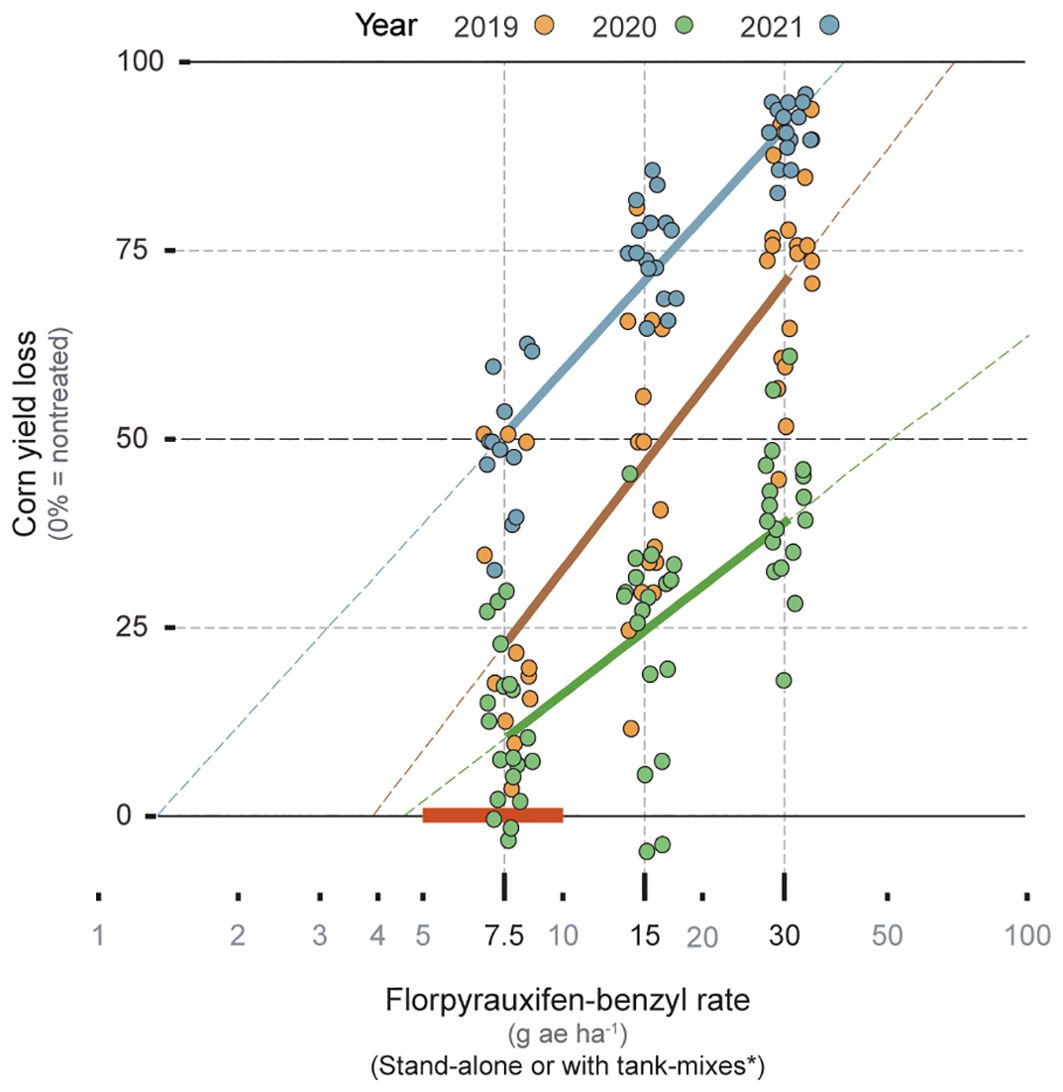
Figure 7. Corn yield response as a function of florpyrauxifen-benzyl rate and year. Model equations were as follows. For 2019: y = 34.88 ln(x) - 46.39, R2 = 0.6; for 2020: y = 20.81 ln(x) - 30.74, R2 = 0.53; and for 2021: y = 29.54 ln(x) - 7.84, R2 = 0.85, where x signifies the florpyrauxifen-benzyl rate, and y represents yield loss. The dashed lines, beyond the range of measured data, represent an extrapolation of the trend established by the model, facilitating useful inferences. However, they do not account for model fitting and may not precisely define the relationship between variables beyond the range of measured data. Each data point denotes plot-level yield loss for those treatments.
The year variable, a proxy for environmental conditions encompassing temperature and solar radiation, potentially caused differences in corn yield across years. The expected effect of the florpyrauxifen-benzyl rates (ranging from 7 to 30 g ae ha-1) was evident in the results; however, the magnitude of the year effect was unexpected. Additionally, there were instances of significant impact of the tank-mix partners on these outcomes. However, their effect size was comparatively minor compared to the year and the rates of the primary herbicide. Future research is needed to isolate and quantify the specific contributions of temperature and solar radiation in manifesting corn injury and yield. Understanding these interaction dynamics is crucial for any future efforts that target enhancing the selectivity of corn to florpyrauxifen-benzyl if it truly exists.
Future research could explore possibilities around the utility of florpyrauxifen-benzyl in corn may include in the crop itself, in the chemistry of this compound, or in application methods. The results from the study indicate that an elevated tolerance by a factor of 3- to 5-fold would suffice its utility in corn. While more tolerant varieties may exist as they do for rice (Wright et al., 2021) or potentially be developed, there have been successful instances of structural modification of existing herbicides to enhance their herbicidal activity and selectivity in crops. One such case involves the alteration of picloram, where Yang et al. (2021) achieved the synthesis of 3-chloro-6-pyrazolyl-2-picolinic acids and their ester derivatives by introducing substituted pyrazole rings to modify clopyralid. This adjustment led to enhanced selectivity in specific crops. Another example pertains to the advancement of florpyrauxifen-benzyl itself. Epp et al. (2016) strategically replaced the chlorine atom with a phenyl group at position 6 of 2-picolinic acid herbicides, developing 6-aryl-2-picolinates. This innovation led to the discovery of halauxifen-methyl and florpyrauxifen-benzyl. Exploring the potential for structural modifications to refine the properties of florpyrauxifen-benzyl or its analogs, thereby enhancing safety in corn, can be a complex endeavor.
The exigent need for diversified herbicides to combat the alarming escalation of herbicide resistance draws attention to the significance of this research. In this framework, this study evaluated the effects of florpyrauxifen-benzyl rates, and tank-mix partner, as well as the interactions between these factors in details, and drew a broader inference about the results. This study furthered our understanding of the effects of florpyrauxifen-benzyl on corn while serving as a basis for future research to explore new possibilities; in-crop, in-chemistry, or in-application methods, for elevating the selectivity of florpyrauxifen-benzyl or its derivatives in corn. Additionally, while this study did not evaluate weed control, the potential utility of florpyrauxifen-benzyl for controlling Palmer amaranth and other broadleaf weeds, inferred from available information, warrants empirical validation as research progresses.
Data availability statement
The raw data supporting the conclusions of this article will be made available by the authors, without undue reservation.
Author contributions
AG: Formal analysis, Visualization, Writing – original draft, Writing – review & editing. JN: Conceptualization, Data curation, Funding acquisition, Investigation, Methodology, Project administration, Resources, Supervision, Validation, Writing – review & editing. LB: Conceptualization, Funding acquisition, Methodology, Validation, Writing – review & editing.
Funding
The author(s) declare that financial support was received for the research, authorship, and/or publication of this article. Arkansas Corn and Grain Sorghum Promotion Board
Acknowledgments
This publication is a contribution of the University of Arkansas System Division of Agriculture.
Conflict of interest
The authors declare that the research was conducted in the absence of any commercial or financial relationships that could be construed as a potential conflict of interest.
Generative AI statement
The author(s) declare that no Generative AI was used in the creation of this manuscript.
Publisher’s note
All claims expressed in this article are solely those of the authors and do not necessarily represent those of their affiliated organizations, or those of the publisher, the editors and the reviewers. Any product that may be evaluated in this article, or claim that may be made by its manufacturer, is not guaranteed or endorsed by the publisher.
References
Abendroth J. A., Martin A. R., Roeth F. W. (2006). Plant response to combinations of mesotrione and photosystem II inhibitors. Weed Technol. 20, 267–274. doi: 10.1614/WT-05-020R.1
Anonymous (2023). Specimen label loyant. Available online at: https://www.corteva.us/products-and-solutions/crop-protection/loyant.html (Accessed October 10, 2024).
Armel G. R., Hall G. J., Wilson H. P., Cullen N. (2005). Mesotrione plus atrazine mixtures for control of Canada thistle (Cirsium arvense). Weed Sci. 53, 202–211. doi: 10.1614/WS-04-039R
Barber L. T., Boyd J. W., Selden G., Norsworthy J. K., Burgos N. R., Bertucci M. (2022). MP44 2022, Recommended chemicals for weed and brush control (Little Rock, Arkansas, USA: University of Arkansas System Division of Agriculture, Research and Extension), 198.
Beesinger J. W., Norsworthy J. K., Butts T. R., Roberts T. L. (2022). Palmer amaranth control in furrow-irrigated rice with florpyrauxifen-benzyl. Weed Technol. 36, 490–496. doi: 10.1017/wet.2022.42
Brooks M. E., Kristensen K., van Benthem K. J., Magnusson A., Berg C. W., Nielsen A., et al. (2017). glmmTMB balances speed and flexibility among packages for zero-inflated generalized linear mixed modeling. R. J. 9, 378–400. doi: 10.32614/RJ-2017-066
Busi R., Goggin D. E., Heap I. M., Horak M. J., Jugulam M., Masters R. A., et al. (2018). Weed resistance to synthetic auxin herbicides. Pest Manage. Sci. 74, 2264–2276. doi: 10.1002/ps.2018.74.issue-10
Epp J. B., Alexander A. L., Balko T. W., Buysse A. M., Brewster W. K., Bryan K., et al. (2016). The discovery of Arylex™ active and Rinskor™ active: Two novel auxin herbicides. Bioorg. Med. Chem. 24, 362–371. doi: 10.1016/j.bmc.2015.08.011
Faske T., Supurlock T., Cato A., Smith S., Urrea-Morawicki K., Wamishe Y. (2022). MP154, Arkansas plant disease control products guide (Little Rock, Arkansas, USA: University of Arkansas System Division of Agriculture, Research and Extension), 158.
Fox J., Weisberg S. (2019). An R companion to applied regression (Thousand Oaks CA: Sage). Available online at: https://socialsciences.mcmaster.ca/jfox/Books/Companion/ (Accessed October 3, 2024).
Gaines T. A., Busi R., Küpper A. (2021). Can new herbicide discovery allow weed management to outpace resistance evolution? Pest Manage. Sci. 77, 3036–3041.
Godar A. S., Norsworthy J. K., Barber T. L. (2023). Enlist™ corn tolerance to preemergence and postemergence applications of synthetic auxin and ACCase-inhibiting herbicides. Weed Technol. 37, 147–155. doi: 10.1017/wet.2023.25
Green J. M., Owen M. D. K. (2011). Herbicide-resistant crops: utilities and limitations for herbicide-resistant weed management. J. Agric. Food Chem. 59, 5819–5829. doi: 10.1021/jf101286h
Herrera R., Weimer M. R., Morell M., Havens P. L., Meregalli G., Papineni S., et al. (2021). “Rinskor active herbicide-A new environment-friendly tool for weed management in rice and aquatic environments,” in Recent highlights in the discovery and optimization of crop protection products (Academic Press, London, UK), 511–523.
Hothorn T., Bretz F., Westfall P. (2008). multcomp: Simultaneous inference in general parametric models. Available online at: https://CRAN.R-project.org/package=multcomp (Accessed April 3, 2024).
Kim J., Jung S., Hwang I. T., Cho K. Y. (1999). Characteristics of chlorophyll a fluorescence induction in cucumber cotyledons treated with diuron, norflurazon, and sulcotrione. Pestic. Biochem. Physiol. 65, 73–81. doi: 10.1006/pest.1999.2428
Lee S., Sundaram S., Armitage L., Evans J. P., Hawkes T., Kepinski S., et al. (2014). Defining binding efficiency and specificity of auxins for SCFTIR1/AFB_ AUX/IAA co-receptor complex formation. ACS Chem. Biol. 9, 673–682. doi: 10.1021/cb400618m
Lenth R. V. (2022). emmeans: Estimated marginal means, aka least-squares means. Available online at: https://CRAN.R-project.org/package=emmeans (Accessed April 3, 2024).
Ma L. Y., Zhang A. P., Liu J. T., Zhang N., Chen M., Yang H. (2021). Minimized atrazine risks to crop security and its residue in the environment by a rice methyltransferase as a regulation factor. J. Agric. Food Chem. 70, 87–98. doi: 10.1021/acs.jafc.1c04172
Miller M. R., Norsworthy J. K. (2018a). Florpyrauxifen-benzyl weed control spectrum and tank-mix compatibility with other commonly applied herbicides in rice. Weed Technol. 32, 319–325. doi: 10.1017/wet.2017.107
Miller M. R., Norsworthy J. K. (2018b). Row crop sensitivity to low rates of foliar-applied florpyrauxifen-benzyl. Weed Technol. 32, 398–403. doi: 10.1017/wet.2017.114
Prusinska J., Uzunova V., Schmitzer P., Weimer M., Bell J., Napier R. M. (2022). The differential binding and biological efficacy of auxin herbicides. Pest Manage. Sci. 79, 1305–1315. doi: 10.1002/ps.v79.4
R Core Team (2024). R: A language and environment for statistical computing (Vienna, Austria: R Foundation for Statistical Computing). Available online at: https://www.R-project.org/ (Accessed October 3, 2024).
Searle S. R., Speed F. M., Milliken G. A. (1980). Population marginal means in the linear model: An alternative to least squares means. Am. Stat. 34, 216–221. doi: 10.1080/00031305.1980.10483031
Serim A. T., Patterson E. L. (2024). Response of conventional sunflower cultivars to drift rates of synthetic auxin herbicides. PeerJ 12, e16729. doi: 10.7717/peerj.16729
Shimabukuro R. H. (1968). Atrazine metabolism in resistant corn and sorghum. Plant Physiol. 43, 1925–1930. doi: 10.1104/pp.43.12.1925
Stroup W. W. (2015). Rethinking the analysis of non-normal data in plant and soil science. Agron. J. 107, 811–827. doi: 10.2134/agronj2013.0342
Studebaker G., Bateman N., Davis J., Cato A., Thrash B., Lee J., et al. (2022). MP144 2022, insecticide recommendation for arkansas (Little Rock, Arkansas, USA: University of Arkansas System Division of Agriculture, Research and Extension), 332.
Tresch S., Grossmann K. (2003). Quinclorac does not inhibit cellulose (cell wall) biosynthesis in sensitive barnyard grass and maize roots. Pestic. Biochem. Physiol. 75, 73–78. doi: 10.1016/S0048-3575(03)00013-0
USDA-NASS Cropland Data Layer [U.S. Department of Agriculture–National Agricultural Statistics Service] (2023). Published crop-specific data layer [Online]. Available online at: https://nassgeodata.gmu.edu/CropScape/ (Accessed October 2, 2024).
Velásquez J. C., Bundt A. D. C., Camargo E. R., Andres A., Viana V. E., Hoyos V., et al. (2021). Florpyrauxifen-benzyl selectivity to rice. Agric. 11, 1270. doi: 10.3390/agriculture11121270
Walsh T. A., Nela R., Merlo A. O., Honma M., Hicks G. R., Wolff K., et al. (2006). Mutations in an auxin receptor homolog AFB5 and in SGT1b confer resistance to synthetic picolinate auxins and not to 2,4-dichlorophenoxyacetic acid or indole-3-acetic acid in Arabidopsis. Plant Physiol. 142, 542–552. doi: 10.1104/pp.106.085969
Wang H., Sun X., Yu J., Li J., Dong L. (2021). The phytotoxicity mechanism of florpyrauxifen-benzyl to Echinochloa crus-galli (L.) P. Beauv and weed control effect. Pestic. Bichem. Physiol. 179, 104978. doi: 10.1016/j.pestbp.2021.104978
Wells G. S., Taylor M. (2016). “Rinskor™ Active-A new herbicide for rice weed control in Australia,” in Proceedings of the twentieth australasian weeds conference. Eds. Randall R., Lloyd S., Borger C. (Weeds Society of Western Australia, Perth, Australia), 269–271.
Wright H. E., Norsworthy J. K., Roberts T. L., Scott R., Hardke J., Gbur E. E. (2021). Characterization of rice cultivar response to florpyrauxifen-benzyl. Weed Technol. 35, 82–92. doi: 10.1017/wet.2020.80
Yang Z., Li Q., Yin J., Liu R., Tian H., Duan L., et al. (2021). Design, synthesis and mode of action of novel 3-chloro-6-pyrazolyl picolinate derivatives as herbicide candidates. Pest Manage. Sci. 77, 2252–2263. doi: 10.1002/ps.v77.5
Yerkes C. N., Lowe C. T., Eckelbarger J. D., Epp J. B., Guenthenspberger K. A., Siddall T. L., et al. (2014). Arylalkyl esters of 4-amino-6-(substituted phenyl)-picolinates and 6-amino-2-(substituted phenyl)-pyrimidinecarboxylates and their use as selective herbicides for crops. US Patent, US8883688B2.
Keywords: crop tolerance, herbicide diversity, novel herbicide, Palmer amaranth, herbicide injury
Citation: Godar AS, Norsworthy JK and Barber LT (2025) Corn tolerance to florpyrauxifen-benzyl rates and its mixture with atrazine and mesotrione. Front. Agron. 7:1535148. doi: 10.3389/fagro.2025.1535148
Received: 26 November 2024; Accepted: 20 January 2025;
Published: 07 February 2025.
Edited by:
Simerjeet Kaur, Punjab Agricultural University, IndiaReviewed by:
Sachin Dhanda, University of Minnesota Twin Cities, United StatesNavjot Brar, Guru Angad Dev Veterinary and Animal Sciences University, India
Copyright © 2025 Godar, Norsworthy and Barber. This is an open-access article distributed under the terms of the Creative Commons Attribution License (CC BY). The use, distribution or reproduction in other forums is permitted, provided the original author(s) and the copyright owner(s) are credited and that the original publication in this journal is cited, in accordance with accepted academic practice. No use, distribution or reproduction is permitted which does not comply with these terms.
*Correspondence: Amar S. Godar, YWdvZGFyQHVhcmsuZWR1