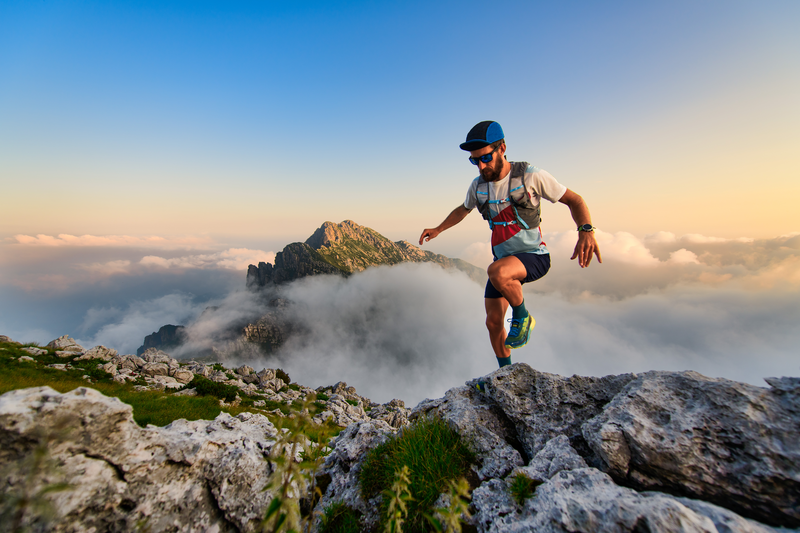
94% of researchers rate our articles as excellent or good
Learn more about the work of our research integrity team to safeguard the quality of each article we publish.
Find out more
ORIGINAL RESEARCH article
Front. Agron.
Sec. Agroecological Cropping Systems
Volume 7 - 2025 | doi: 10.3389/fagro.2025.1534962
This article is part of the Research Topic Agroecological Practices To Enhance Resilience Of Farming Systems View all 9 articles
The final, formatted version of the article will be published soon.
You have multiple emails registered with Frontiers:
Please enter your email address:
If you already have an account, please login
You don't have a Frontiers account ? You can register here
Intermediate wheatgrass [Thinopyrum intermedium (Host) Barkworth & D.R. Dewey] (IWG) is a novel perennial grain crop with the potential for dual use (DU) in a system that includes the harvest of summer grain and straw as well as the grazing of crop regrowth. This could diversify grower income streams but impacts on productivity and profitability of DU systems need evaluation. A 4-year on-farm trial was conducted in Minnesota, USA comparing yields and net revenue of a grain+straw production system (GP) vs. a DU system. For both the GP and DU systems, the grain and straw yields from the summer harvest were evaluated, the subsequent IWG regrowth was measured in the fall and again in spring to quantify forage production and nutritive value, and the economic value of grain, straw, and forage were calculated. In the DU system, the herbage intake and forage utilization were also studied. The GP system produced 42% more grain and 41% more straw than the DU system in year 2 but both systems produced similar grain and straw yields in year 3. The DU system produced greater grain yields than the GP in year 4. Across systems, the forage yield peaked in year 3. Both agronomic systems generally displayed similar forage yields of comparable nutritive value. Crude protein (CP) in fall and spring forage averaged 140 to 150 g kg-1 whereas CP was 30 g kg-1 in the summer straw, comparable to common annual small grains. The relative feed value of IWG forage in the fall was 100 and 127 in spring compared with 80 in the summer. The sale of higher year 2 grain yields in the GP system led to this system earning a net return to the enterprise of $721 ha-1 yr-1 with the DU system producing $608 ha-1 yr-1. In conclusion, grazing IWG can take advantage of on-farm forage resources to generate revenue but waiting to begin grazing until after the second-year grain harvest may reduce the risk of grain and straw yield losses to enhance net returns to the enterprise.
Keywords: Thinopyrum intermedium, Perennial grain, Nutritive Value, enterprise budget, Forage yield
Received: 26 Nov 2024; Accepted: 12 Mar 2025.
Copyright: © 2025 Rusch, Hunter, Kraus, Tautges and Jungers. This is an open-access article distributed under the terms of the Creative Commons Attribution License (CC BY). The use, distribution or reproduction in other forums is permitted, provided the original author(s) or licensor are credited and that the original publication in this journal is cited, in accordance with accepted academic practice. No use, distribution or reproduction is permitted which does not comply with these terms.
* Correspondence:
Hannah L Rusch, University of Minnesota Twin Cities, St. Paul, United States
Disclaimer: All claims expressed in this article are solely those of the authors and do not necessarily represent those of their affiliated organizations, or those of the publisher, the editors and the reviewers. Any product that may be evaluated in this article or claim that may be made by its manufacturer is not guaranteed or endorsed by the publisher.
Research integrity at Frontiers
Learn more about the work of our research integrity team to safeguard the quality of each article we publish.