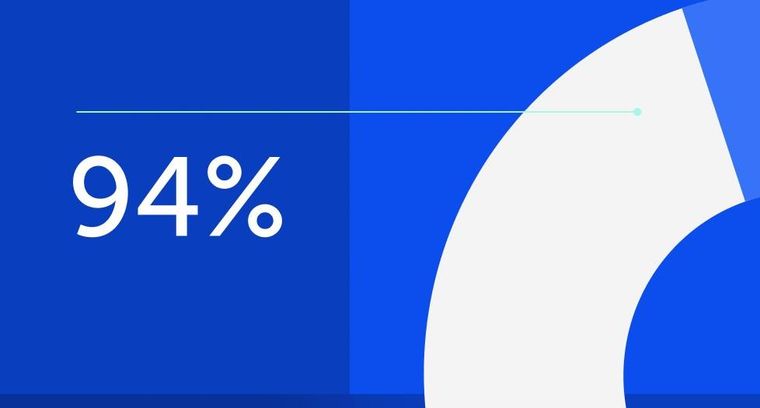
94% of researchers rate our articles as excellent or good
Learn more about the work of our research integrity team to safeguard the quality of each article we publish.
Find out more
ORIGINAL RESEARCH article
Front. Agron., 31 March 2025
Sec. Agroecological Cropping Systems
Volume 7 - 2025 | https://doi.org/10.3389/fagro.2025.1456015
This study investigated pedological characteristics of the soils in Sikonge, Uyui, and Tabora districts in Tabora Region of Tanzania, focusing on their suitability for tobacco (Nicotiana tabacum L.) cultivation. Soil profiles excavated in each district, yielding pedons named TUTUO P1, KIPERA P1, and ISIKIZYA P1, respectively. Standard laboratory methods used to analyze soil texture, bulk density, pH, macronutrients and micronutrients of the soil samples. Results indicated predominantly sandy texture in the studied soils, with Uyui exhibiting loamy sand texture, Sikonge featuring loamy sand and sandy loam, and Tabora comprising sandy clay loam at the surface transitioning to sandy loam and clay in the subsoil. Soil reaction ranged from extremely acid to strongly acid, with pH < 4.5 and pH 5.1-5.5 observed. Nitrogen was notably low across pedons (N< 0.05%). Bulk density exhibited varying trends with depth across districts, ranging from 0.02 to 1.78 g cm-3. Cation exchange capacity increased with soil depth in ISIKIZYA P1 but decreased in TUTUO P1 and KIPERA P1. Available P, varied from very low (0.01 mg kg-1 soil) to medium (15.93 mg kg-1 soil) with increasing depth. Micronutrient analysis revealed elevated copper and iron levels through pedons, zinc deficiency observed in ISIKIZYA P1. Soil classification identified Alfisols, Oxisols and Mollisols, in the respective study areas. The findings revealed notable soil variations, indicating both challenges and prospects for optimizing tobacco production. Tailored soil management is crucial to improve production efficiency.
Tobacco (Nicotiana tabacum L.) remains an important global cash crop, central to agricultural economies and international trade. Despite a global decline in demand due to health concerns and regulatory pressures, tobacco continues to play a major role in the economies of many countries. In particular, Africa remains a key producer, with countries such as Malawi, Zimbabwe, and Tanzania heavily involved in its cultivation (Shonhe et al., 2022; Mwalembe et al., 2024). However, the sector faces growing challenges from soil degradation, climate change, and shifting market dynamics. In this context, understanding soil properties that affect tobacco productivity is crucial for ensuring sustainable production (Chen et al., 2025).
Tobacco cultivation is essential to the livelihoods of many smallholder farmers, particularly in Southern and Eastern African countries (Hu and Lee, 2014; Prowse and Pérez Niño, 2022). While much research has been dedicated to soil fertility for staple crops like maize and rice, tobacco-specific pedological studies are limited. The region’s diverse agro-ecological conditions and soil types require detailed research to optimize tobacco production. Countries like Malawi and Zambia have undertaken some tobacco-specific soil assessments, but comprehensive studies, particularly those focusing on Tanzania remain scarce (Andersson Djurfeldt et al., 2019). This lack of region-specific data has hindered the development of tailored agronomic practices for tobacco farmers in Africa (Ouma et al., 2024).
Tanzania is one of the largest tobacco producers in Sub-Saharan Africa, with Tabora being the primary tobacco-growing region. Despite its economic importance, the region faces challenges such as soil fertility decline, soil erosion, and nutrient imbalances, which affect crop yield and quality. While general soil fertility assessments have been conducted for various crops in Tanzania, there is a notable gap in tobacco-specific soil studies, particularly in Tabora. The unique soil requirements of tobacco—such as its sensitivity to nutrient availability, soil texture, and structure—demand targeted research to optimize productivity and quality. This gap in knowledge makes it difficult to develop effective, sustainable soil management practices for tobacco farming in the region (Makoi and Mmbaga, 2018; Msanya et al., 2002).
Previous research, such as studies by Marzouk et al. (2023) and Makoi and Mmbaga (2018), has provided valuable insights into general soil conditions in Tanzania’s irrigation schemes. However, these studies have not specifically addressed the soil characteristics needed for tobacco production. Others like those of Funakawa et al. (2012) and Msanya et al. (2002) mapped major soil types across the country but did not focus on their suitability for tobacco. This study aims to provide detailed assessments of the physical and chemical properties of soils in tobacco-growing Tabora region, focusing on nutrient availability, cation-exchange capacity, and the role of chloride (Cl), which is critical for regulating osmotic pressure and enhancing tobacco leaf quality (Chen et al., 2025).
The findings from this study are expected to contribute to the understanding of soil properties that influence tobacco cultivation, providing targeted insights into the nutrient requirements and management practices essential for optimizing tobacco productivity and quality in Tabora region and similar areas. This research will also inform the development of sustainable soil management practices, ensuring long-term soil health while enhancing tobacco farming in Tanzania.
This research was conducted in three districts of Tabora region in Tanzania. The study area was selected due to its prominence in tobacco cultivation, accounting for a significant share of the country’s production. The region has six districts widely recognized for tobacco farming, namely Sikonge, Uyui, Tabora, Urambo, Kaliua, and Nzega (Kuzilwa et al., 2019; Mbotwa and Rweyemamu, 2024). To ensure a representative analysis of soil conditions, site selection considered variations in tobacco production intensity and geographic distribution within the region. Following a series of field experiences and consultations with agricultural experts, three districts were selected based on their production dominance and soil diversity.
The study villages were purposively chosen due to their long history of tobacco cultivation and relevance for soil characterization. Soil profile pits were dug at Tutuo village in Sikonge district (05°47’775” S, 032°65’686” E), Isikizya village in Uyui district (04°86’294” S, 033°10’862” E), and Kipera village in Tabora Municipal (05°14’055” S, 032°68’026” E). These sites are characterized by a unimodal rainfall pattern, which starts from November and ends in March (Mbotwa and Rweyemamu, 2024). The selected locations represent different soil types and production systems, ensuring a broad assessment of pedological characteristics in the region.
Although microbial activity and biological soil properties influence soil fertility, this study focused on pedological aspects, including physical and chemical properties. The exclusion of microbial activity analysis was due to resource constraints and the primary objective of soil classification and fertility evaluation. Future research should incorporate microbial assessments to provide a more holistic understanding of soil health in tobacco-growing areas.
Reconnaissance field survey using transects and observations conducted in the study sites. On each site, one representative soil profile pit of 120 cm width, 200 cm length and depth ranging from 90-200 cm, depending on limiting layer, was excavated (Figure 1). The profiles named as ISIKIZYA P1, TUTUO P1 and KIPERA P1 for Uyui, Sikonge and Tabora districts, respectively. These profiles named basing on the villages’ names where the study conducted. Data on landform, soil morphological features (color, texture, consistency, structure, porosity, and depth), parent material, natural vegetation, drainage, slope gradient; elevation, erosion, and land use recorded and filled in forms designed by the National Soil Service of Tanzania, adopted from the FAO Guidelines for Soil description. The representative study sites were recorded using international coordinates with the Global Positioning System (GPS).
Figure 1. Description of profiles: (a) TUTUO P1, (b) ISIKIZYA P1, (c) KIPERA P1 from the study sites in Sikonge, Uyui and Tabora districts, respectively.
Soil samples were collected from the designated pedogenic horizons, with four sections sampled from the TUTUO P1 and ISIKIZYA P1 profiles, and three sections from the KIPERA P1 profile for laboratory analysis. Composite soils samples (0-30 cm depth) collected from six sampling spots using zigzag sampling approach. The six subsamples collected from the sampling spots mixed and quartered to obtain representative composite surface soil samples of 1 kg for laboratory analysis. Physical and chemical properties were determined following standard procedures. Particle size analysis determined by the hydrometer method after dispersion with 5% sodium hexametaphosphate (Gee and Bauder, 1986). Soil bulk density was determined by drying soil samples at 105°C for 24 hrs (Okalebo et al., 2002). Soil color was determined by Munsell Soil Color Charts (Color, 2009). Soil pH, electrical conductivity and Chlorine (Cl) were determined Potentiometrically in soil-to-water suspension (1:2.5) (Lindsay and Norvell, 1978). Soil organic carbon was determined by the Walkley and Black wet oxidation method (Matus et al., 2009). Available P determined by a Bray and Kurtz P-1 (Okalebo et al., 2002). Total nitrogen was analyzed by the micro-Kjeldahl distillation method (Bremner and Mulvaney, 1982). Cation exchange capacity (CEC) was determined by the neutral ammonium acetate saturation method (NH4-Ac, pH 7.0) followed by Kjeldahl distillation (Mattigod and Zachara, 1996). Exchangeable bases (K+, Mg2+, Ca2+, and Na+) were determined by the 1 N NH4-Ac (pH 7.0) methods (Mattigod and Zachara, 1996). Mg and Ca read by the UV-Vis spectrophotometer and K and Na by flame emission photometer. Extractable micronutrients (Fe, Cu, Zn, and Mn) extracted by diethylenetriamine pentaacetic acid (DTPA) and determined using atomic absorption spectroscopy (AAS) (Lindsay and Norvell, 1978). Extractable sulfur was determined by turbidi-metric methods (Moberg, 2000). Base saturation percent (% BS) was obtained by dividing total exchangeable bases (TEB) by CEC and then multiplied by 100. Likewise, exchangeable sodium percentage (ESP) obtained by dividing total exchangeable sodium by CEC, and then multiplied by 100. The K: TEB ratio was obtained by dividing K by TEB; C: N ratio was obtained by dividing OC by Total N, Ca: Mg ratio obtained by dividing Exchangeable Ca by Exchangeable Mg, K:Mg obtained by dividing K by Exchangeable Mg, K:CEC obtained by dividing K by CEC.
Using both field and laboratory data the soils classified up to family level of the USDA Soil Taxonomy and to level tier-2 of the WRB Soil Classification (Schad, 2023). The soils in each pedon classified into their appropriate order, suborder, great group, and subgroup following the guideline of the USDA Soil Taxonomy and tier-2 of the FAO WRB for soil resources (Brevik et al., 2016; Soil Survey Staff, 2022; Schad, 2023).
The studied pedons showed slight variation in drainage patterns, but KIPERA P1 pedon, poorly drained and shallow (90 cm). The surface soil was characterized by gravel sand clay while the subsoil (90 cm) was very hard characterized with clay, while TUTUO P1 was well drained and very deep (>115 cm), in the 110 cm depth water was observed. The ISIKIZYA P1 topsoil was characterized by fine sand, while the subsoil ranged from friable to hard soils with many fine (m.f.) pores.The topsoil color of the TUTUO P1 pedon varied from orange (7.5YR6/8) when dry to brown (10YR4/3) when moist. The subsoil color varied from pink (7.5YR7/4) when dry to reddish yellow (7.5YR6/6) when moist with a predominant of few fine (f.f) mottles. At ISIKIZYA P1, the topsoil color varied from light yellowish brown (10YR6/4) when dry to yellowish brown (10YR3/6) when moist, with the subsoil varying from bright brown (7.5YR7/4) when dry to dark reddish brown (5YR5/8) when moist with no mottles. KIPERA P1 pedon, the top soil was characterized by brown color (7.5YR4/4) when dry to dark reddish brown (5YR3/3) when moist, the sub soil varied from reddish yellow (7.5YR6/8) when dry to yellowish red (5YR4/6) when moist while having no mottles.
The physical properties of the studied soils are summarized in Table 1. ISIKIZYA P1 and TUTUO P1 pedons had sandy loam soils at both topsoil and subsoil layers, whereas KIPERA P1 had sandy clay loam topsoil transitioning to clay in deeper horizons. The silt/clay ratio varied across sites, with ISIKIZYA P1 ranging from 1.29 to 4.6, TUTUO P1 from 0.14 to 5.8, and KIPERA P1 from 0.2 to 0.4. Bulk density values ranged from 1.02 to 1.28 g cm-3 at ISIKIZYA P1, 1.02 to 1.28 g cm-3 at TUTUO P1, and 1.51 to 1.78 g cm-3 at KIPERA P1.
The results for the chemical properties of the studied soils presented (Tables 2, 3). The soil reaction at ISIKIZYA P1 was very strongly acid (pH 4.67-4.85), but ranged from very strongly acid (pH 5.67-4.91) to strongly acid (pH 5.1-5.5) at TUTUO P1and KIPERA P1. Variations in EC were observed, with ISIKIZYA P1 displaying an EC of 0.049 dS m–1 in the topsoil and varying values ranging from 0.01 to 0.021 dS m–1 in the subsoil. In contrast, TUTUO P1 showed EC values overall, with the topsoil registering 0.026 dS m–1 and subsoil ranging from 0.017 to 0.032 dS m–1. Conversely, KIPERA P1 exhibited EC values, measuring 0.089 dS m–1 in the topsoil and ranging from 0.006 to 0.015 dS m–1 in the subsoil.
Exchangeable sodium percentage varied across locations, with ISIKIZYA P1 showing ESP values of 1.1% in the topsoil and ranging from 0.2% to 0.3% in the subsoil. TUTUO P1 and KIPERA P1 revealed consistent ESP values, maintaining around 0.1% in the topsoil and 0.2% in the subsoil. Organic carbon content varied significantly, with ISIKIZYA P1 having an OC content of 0.97% in the topsoil and ranging from 0.01% to 1.51% in the subsoil. TUTUO P1 and KIPERA P1 showed similar trends, with higher OC content in the subsoil compared to the topsoil. Total nitrogen and carbon to nitrogen ratio varied across locations, suggesting differences in nutrient availability and soil fertility. Phosphorus availability also varied, with ISIKIZYA P1 displaying higher P availability in both topsoil and subsoil compared to TUTUO P1 and KIPERA P1. The CEC exhibited location-specific patterns, with ISIKIZYA P1 and KIPERA P1 showing higher CEC values in the subsoil compared to the topsoil, while TUTUO P1 had notably higher CEC values in the topsoil compared to subsoils.
The ISIKIZYA P1 pedon exhibited topsoil Ca concentrations of 0.52 cmol(+) kg-1, with subsoil concentrations ranging from 0.33 to 0.92 cmol(+) kg-1. TUTUO P1 pedon showed topsoil calcium of 0.34 cmol(+) kg-1, with subsoil concentrations varying from 0.25 to 0.97 cmol(+) kg-1. KIPERA P1 pedon displayed topsoil Ca of 0.49 cmol(+) kg-1, with subsoil concentrations ranging from 0.29 to 0.37 cmol(+) kg-1. Exchangeable Magnesium concentrations at ISIKIZYA P1 pedon’s topsoil were 0.72 cmol(+) kg-1, with subsoil concentrations ranging from 0.5 to 2.34 cmol(+) kg-1. TUTUO P1 pedon exhibited topsoil Magnesium concentrations of 0.57 cmol(+) kg-1, with subsoil concentrations varying from 0.8 to 2.34 cmol(+) kg-1. KIPERA P1 pedon displayed topsoil Mg concentrations of 0.73 cmol(+) kg-1, with subsoil concentrations ranging from 0.69 to 0.85 cmol(+) kg-1. Potassium concentrations at ISIKIZYA P1 pedon’s topsoil were 0.1 cmol(+) kg-1, with subsoil concentrations ranging from 0 to 11 cmol(+) kg-1. TUTUO P1 pedon exhibited topsoil potassium concentrations of 0.1 cmol(+) kg-1, with subsoil concentrations varying from 8.49 to 17 cmol(+) kg-1. KIPERA P1 pedon displayed topsoil potassium concentrations of 0.1 cmol(+) kg-1, with subsoil concentrations ranging from 43 to 64 cmol(+) kg-1. Sodium concentrations at ISIKIZYA P1 pedon’s topsoil were 0.07 cmol(+) kg-1, with subsoil concentrations of 0.02 cmol(+) kg-1. TUTUO P1 pedon exhibited topsoil Na concentrations of 0.02 cmol(+) kg-1, with subsoil concentrations ranging from 0.02 to 0.03 cmol(+) kg-1. KIPERA P1 pedon displayed sodium concentrations of 0.02 cmol(+) kg-1 throughout the pedon.
The analysis of extractable micronutrients (Cu, Fe, Mn, and Zn) and extractable sulfur revealed varying concentrations across the studied profiles. At ISIKIZYA P1, the topsoil had a copper content of 2.06 mg kg-1, decreasing to 1.12-2.68 mg kg-1 in the subsoil. Iron was 196.67 mg kg-1 in the topsoil and ranged from 84.93 to 119.5 mg kg-1 in the subsoil. Interestingly, negligible Zn content was detected in the ISIKIZYA P1 pedon, while chlorine was present at very low concentrations throughout the entire pedon. For TUTUO P1, Cu ranged from 2.68 to 3.12 mg kg-1 in the topsoil and subsoil, while Fe levels varied from 58.83 to 287.17 mg kg-1. Zn was negligibly small in the topsoil but present in the subsoil at 0.37-0.63 mg kg-1. Similarly, KIPERA P1 exhibited Cu concentrations of 4.74-6.36 mg kg-1 in the topsoil and Fe levels ranging from 84.83 to 273.00 mg kg-1. Zn detected in the topsoil at 1.09-1.58 mg kg-1 and in the subsoil at 1.09-1.29 mg kg-1. Manganese contents ranged from 25.17 to 159.10 mg kg-1 in the ISIKIZYA P1 pedon, 11.40 to 162.39 mg kg-1 in TUTUO P1, and 125.17 to 175.31 mg kg-1 in KIPERA P1. Sulfur content varied across the sites, with ISIKIZYA P1 showing 5.69 mg kg-1 in the topsoil and 2.84-8.45 mg kg-1 in the subsoil. TUTUO P1 had sulfur 9.60 mg kg-1 in the topsoil and 6.32-20.2 mg kg-1 in the subsoil, while KIPERA P1 exhibited 8.26-8.68 mg kg-1 in the topsoil and negligible sulfur content was in the subsoil. Chlorine concentrations remained minimal throughout the KIPERA P1 and TUTUO P1 pedons.
The soil cation ratios were determined (Table 4) and the results were Ca/Mg ratio at ISIKIZYA P1 topsoil was 0.72 and the subsoil ranged from 0.39 to 0.68 while TUTUO P1 topsoil was 0.59 to subsoil of 0.31 to 0.41, and KIPERA P1 had topsoil of 0.67 to 0.42 subsoil. The K/Mg ratios in ISIKIZYA P1 topsoil was 0.14 to subsoil of 0.08 to 0.69 while TUTUO P1 had 0.14 topsoil to 0.08 to 4.69 subsoil, and KIPERA P1 had topsoil of 0.11 topsoil to subsoil of 12.46 and 0.6. The K/CEC ratio at ISIKIZYA P1 0.02 topsoil to subsoil of 0.004 to 0.73, TUTUO P1 was 0.001 topsoil to subsoil 0.004 to 0.73 and the KIPERA P1 had a top soil of 0.02 to subsoil of 0.04 and 0.77.
The results reveal significant variability in soil properties across the pedons studied in Sikonge, Tabora Mc, and Uyui districts, which is important for agricultural planning, particularly for tobacco cultivation in the Tabora region (Table 5; Table 6). Based on field and laboratory data, the pedons classified Chromic Luvisol (Coluvic, Humic, Ochric, Neocambic), featuring an umbric epipedon and a cambic horizon. The soil was characterized by gently sloping, moderately deep sandy loam to sandy clay loam with a strong to very strong acidity (pH 4.5 - 5.0) and was categorized by ustic, isohyperthermic. The KIPERA P1 pedon featured as Xanthic Geric Ferralsol (Aric, clayic, ochric), exhibited an ochric epipedon and an oxic horizon, with gently sloping, moderately deep sandy clay to silt clay soil and strong to very strong acidity (pH 4.2 - 4.8), also classified as ustic, isohyperthermic. The ISIKIZYA P1 pedon was classified as Gleyic, Fluvic, Duric Chernozem (Aric, Cambic), characterized by a mollic epipedon and a cambic horizon with very strong acidity (pH 4.3 - 4.9) and gently sloping, moderately deep sandy loam to sandy clay soil, classified as ustic, isohyperthermic. Soil properties such as texture, pH, and horizon depth were found to vary across the pedons: TUTUO P1 showed a sand content of 70%, silt content of 20%, and clay content of 10%, KIPERA P1 had sand content of 60%, silt content of 30%, and clay content of 10%, while ISIKIZYA P1 displayed sand content of 65%, silt content of 25%, and clay content of 10%. These findings provide essential information for understanding the variability in soil properties across the study areas and their implications for agricultural practices.
Soil color is a useful indicator of soil properties, such as drainage and fertility. Orange soils, rich in iron oxides, suggest good aeration and drainage (Lufega and Msanya, 2017; Marzouk et al., 2023), while brown soils, with higher organic matter, indicate better fertility due to enhanced nutrient retention (Baumann et al., 2016; Gerke, 2022). Soil color reflects processes like iron oxidation and organic matter decomposition, which affect soil fertility and moisture retention. In the ISIKIZYA P1 and KIPERA P1 profiles, color changes in the topsoil and subsoil highlight moisture retention and iron oxide activity, which are key for nutrient cycling and land management (Meng et al., 2023; Msanya et al., 2018; Mfaume et al., 2019; Merumba et al., 2020; Marzouk et al., 2023). Other studies have shown that darker soils often correlate with higher fertility and better moisture retention, essential for crop growth.
Generally, the soils for ISIKIZYA P1 pedons had loam sand at the top and subsoil. Loam sand offers a balance between the rapid drainage of sandy soils and the nutrient-retaining capacity. Its specific properties can vary depending on the exact composition of sand, silt, and clay in the soil, while it has good drainage properties, loam sand can retain some nutrients due to the presence of silt and clay particles (Msanya et al., 2003). This makes it suitable for some types of plants and crops including tobacco (Lisuma et al., 2023). Top sandy loam soils at TUTUO P1 have a significant importance, allows for excellent drainage, excess water drains away quickly, reducing the risk of waterlogged roots in plants. At KIPERA P1, the topsoil was sandy clay loam while the subsoil was clay soils. Sandy clay loamy soils contain a balanced mixture of sand, silt, and clay particles. However, in this soil type, the clay component is more dominant than in sandy loam soils. The balance between these particle sizes contributes to the soil’s overall texture. According to Miller (2014), sandy clay loam soils have good drainage properties due to the presence of sand particles. Water can penetrate through the soil relatively well reducing the risk of waterlogging, while these soils drain well, they also retain nutrients effectively due to the clay component (Manik et al., 2019). However, they may become compacted more easily than sandy or loamy soils, so proper soil management practices are important (Marzouk et al., 2023).
Clay particles are the smallest among soil particles and tend to stick together, resulting in fine-textured soil with notable poor drainage (Schjønning et al., 2017). Due to their ability to hold water tightly, clay particles lead to slow water infiltration and drainage, often causing waterlogged conditions during heavy rainfall. Despite this, clay soils have good nutrient retention because of its fine particles. However, they are prone to compaction under pressure or heavy machinery, which reduces pore spaces, limiting root growth and water infiltration. Proper soil management practices are crucial to mitigate compaction. Although challenging to work with when wet, clay subsoil can be productive and fertile with proper management, addressing its unique drainage and workability challenges (Lufega and Msanya, 2017). In the ISIKIZYA P1 and TUTUO P1 profiles, clay content was low, while in the KIPERA P1 pedon, clay content increased with depth, indicating some clay migration (Kebeney et al., 2015). All three profiles had low silt content. The silt-to-clay ratio was high at ISIKIZYA P1 but lower at KIPERA P1, with a lower silt/clay ratio indicating greater soil susceptibility to detachment and transport (Kalala et al., 2017). Soils with a high silt-to-clay ratio tend to have better drainage than those with higher clay content, as silt particles allow for better water infiltration and drainage, reducing waterlogging risks. Silt-rich soils are generally easier to work with than pure clay soils, offering good workability and suitability for cultivation, although they may also be prone to compaction when wet. Additionally, silt particles have moderate nutrient retention capabilities (Uwitonze et al., 2016).
Bulk density is a critical soil property influencing soil health and land use. High bulk density indicates soil compaction, where soil particles pressed closely together, reducing pore space and hindering air, water, and root penetration (Marzouk et al., 2023). Compacted soils suffer from limited aeration, negatively impacting soil microbial activity, root growth, and overall soil health. Poor aeration and reduced pore spaces also impede water infiltration, leading to surface runoff and potential waterlogging in lower areas (Merumba, 2022). Bulk density greater than 1.65 g cm-³ considered unfavorable for plant root growth. For instance, the KIPERA P1 subsoil exhibited a bulk density of 1.78 gcm-³, necessitating soil management practices to improve soil structure (Kalala et al., 2017). Methods such as tilling, subsoiling, or using aerators can alleviate compaction, and incorporating organic matter like compost or mulch can enhance soil structure and water-holding capacity (Chaudhari et al., 2013). At TUTUO P1, the topsoil had higher bulk density than the subsoil, likely due to water presence at 110 cm depth in the excavated profile. These variations underscore the importance of tailored soil management practices to address specific compaction issues and improve soil health.
Soil reaction, measured by pH, is a critical factor in determining soil suitability for tobacco cultivation. In the studied soils, all pedons were highly acidic, with pH values below 5.0, whereas the ideal pH range for tobacco cultivation is between 5.5 and 6.5 (Zeng et al., 2014). Acidic soils, with pH below 5.5, increase the solubility of toxic elements like aluminum and manganese, which hinder nutrient availability, particularly phosphorus, calcium, and magnesium, and reduce microbial activity (Lufega and Msanya, 2017; Massawe et al., 2017). Low pH levels can therefore limit tobacco growth, whereas pH levels above 6.5 can also cause nutrient imbalances (Lufega and Msanya, 2017; Massawe et al., 2017). Maintaining optimal pH levels is essential for nutrient cycling and microbial activity, which are critical for crop productivity.
Electrical conductivity measures soil’s ability to conduct electrical currents and is important for tobacco cultivation as it assesses soil salinity. High soil salinity can be detrimental to tobacco crops by affecting water uptake and nutrient availability (Lisuma et al., 2022). Although tobacco does not have specific EC requirements, high EC levels can limit nutrient uptake (Msanya et al., 2001). In the studied soils, ISIKIZYA P1 and TUTUO P1 exhibited varying EC levels within the pedons, likely due to rainwater percolating through the soil and carrying soluble ions such as calcium, magnesium, potassium, and sodium (Okalebo et al., 2002). As water moves through the soil profile, it dissolves and transports ions, causing fluctuations in EC within different horizons. Soils with an exchangeable sodium percentage of less than 5% are generally suitable for most crops. However, ESP levels above 5%, particularly those approaching or exceeding 15% indicate a risk of soil degradation due to high sodium content (AbdelRahman et al., 2022).
All study sites exhibited sodium below 5%, indicating negligible salinity effects. High sodium levels can compete with essential nutrients like calcium and magnesium, causing nutrient imbalances and reduced crop yields (Mfaume et al., 2019). Elevated sodium levels also negatively affect tobacco leaf quality, influencing flavor and curing characteristics. Soil organic carbon is a crucial component of the global carbon cycle, influencing soil properties such as water infiltration, water-holding capacity, nutrient availability, soil structure, bulk density, and microbial activity (Meng et al., 2023).
The total nitrogen in the studied pedons was very low (less than 0.1%), highlighting the need for appropriate nitrogenous fertilizer application. High N levels (above 2%) typically no need for additional nitrogenous fertilizers, avoiding over-fertilization and associated environmental concerns (Cui et al., 2010). The low total N observed might due to the very acidic soil pH, which affects nitrogen conversion into plant-usable forms like ammonium and nitrate, as well as to erosion, leaching, and nutrient depletion by plants (Marzouk et al., 2023).
The carbon-to-nitrogen ratio is a vital indicator of organic material quality and predicts organic matter mineralization (Tian et al., 2010). According to Yang et al., (2023), the C: N ratio influences soil health, nutrient cycling, and plant growth. The C: N ratio increased with depth in ISIKIZYA P1 and KIPERA P1 but decreased in TUTUO P1, likely due to the water table at 110 cm depth in the TUTUO profile. Optimal C: N ratios vary based on soil type, climate, and management practices. High C: N ratios, such as those found in crop residues or straw, indicate higher carbon relative to nitrogen, affecting decomposition rates (Van Sundert et al., 2020). Tobacco is a nutrient-demanding crop and nitrogen is a critical nutrient for its growth (Liu et al., 2024). Maintaining an appropriate C: N ratio in the soil can help ensure a steady supply of N to the tobacco plants throughout the growing season (Zhou et al., 2017).
The available P at ISIKIZYA P1 and KIPERA P1 varied with increased depth except at TUTUO P1 site where available P decreased with increase in depth probably due to availability of water found at the deep leading to flushing of P element. Phosphorus is an essential nutrient for plant growth and is particularly important for tobacco crop (Ziadi et al., 2013). The availability of phosphorus in soils for tobacco cultivation is crucial because it plays a vital role in various physiological processes within the plant (Khan et al., 2023). Soils with available P levels below 10-15 mg P kg-1 considered low in phosphorus (Tucker et al., 1999). In high-P soils, usually no immediate need for additional high rates of phosphorus fertilization, and high phosphorus can lead to environmental issues such as water pollution. P availability to plants strongly influenced by the pH of the soils and maximized when the soil pH is between 5.5 and 7.5 (Lufega and Msanya, 2017). The studied pedons have pH < 5.0, which could be the reasons for low to medium available P.
Low CEC in the soil is an indication of poor fertility and poor water infiltration (Lufega and Msanya, 2017). A higher CEC generally indicates a soil’s capacity to hold and supply more nutrients to plants. For tobacco crop a CEC of around 10 to 20 cmol(+) kg-1 is often considered suitable. The low CEC values (6 cmol(+) kg-1) in top soils at ISIKIZYA P1 pedon may be due to Sandy soils dominance which consist of large particles with fewer negatively charged sites for cation exchange.
Calcium was low through the pedons (< 1 cmol(+) kg-1, it varied with increase in depth at ISIKIZYA P1 and TUTUO P1, but decreased with depth increase at KIPERA P1, in this regard, the addition of calcium containing fertilizer such as CAN 27% N in a recommended rates required at all sites (Motsara and Roy, 2008). Magnesium is another critical nutrient for tobacco growth and development. A target range for magnesium in the soil for tobacco cultivation is generally between 0.5-2 cmol(+) kg-1). Exchangeable Mg for studied pedons was found in the sufficient range (<2.5 cmol(+) kg-1).
The study pedons had varying K within the specific pedons, therefore additional of potassium containing fertilizers such as NPK is very crucial for better growth and yield of tobacco regarding that Potassium is essential for overall tobacco plant health and leaf quality. Maintain sodium (Na) at low levels in soils for tobacco cultivation, typically less than 0.17 cmol (+) kg-1. The studied pedons had low sodium (<0.07 cmol(+) kg-1). High sodium level can lead to salinity of the soil, which is detrimental to crop growth and quality (Marzouk et al., 2023). Tobacco is a sensitive crop; soil pH and nutrient availability can significantly affect its growth and quality. According to Alloway (2013), tobacco cultivation would want the soil to have a base saturation percentage that favors the presence of calcium and magnesium that are essential nutrients for its growth.
A common target range for base saturation in soils for most crop cultivation should not exceed 80% (Rawal et al., 2019). Base saturation was seen to be very low in all the top soils for all sites it increased with increase in depth, probably was due low pH (acidic soils) that was found in all the soils, acidic conditions tend to displace basic cations (calcium, magnesium, potassium, and sodium). The subsoil for KIPERA P1 had a base saturation of 405% which is above the normal range (80%), this is probably due pedon being high in potassium within the subsurface and natural mineral deposits (Wang et al., 2019).
The recommended sufficiency range for Cu in the soil for tobacco crop is typically between 0.2 to 2.0 mg/kg. According to Xu et al. (2024), copper acts as a catalyzer in some metabolic reactions in plants. Soil with copper levels below 0.2 mg kg-1 is deficient. According to Zewide and Sherefu (2021), copper deficiency can lead to reduced growth and potential nutrient-related issues in plants (Alloway, 2013). However, the amount of copper in all the studied soils were above the recommended rate for tobacco production this is probably due to weathering of rocks and minerals containing copper, the use of copper-based pesticides and fungicides may have left a legacy of copper in the soils.
Iron (Fe) deficiency can lead to reduced growth and potential nutrient-related issues in tobacco plants. Soil with iron levels above 100 mg kg-1 is high (Blancquaert et al., 2017). According to Shukla et al. (2018), elevated Fe levels in the soil can lead to Fe toxicity in tobacco plants, which can cause growth problems and adversely affect crop health. All the studied pedons had soils with high Fe; this is probably due to weathering of Iron rocks that release Iron in the soils and continuous application of pesticides containing iron materials (Colombo et al., 2014).
The recommended sufficiency range for Zn in the soil for tobacco crop is typically between 0.5 to 2.0 mg kg-1. Zinc affects plant–pathogen interactions via its key role in the activation/stabilization of metalloenzymes in plants (Xu et al., 2024). According to Solanki (2021), soil with Zn levels below 0.5 mg kg-1 is deficient. Zinc above 2.0 mg kg-1 in the soil is high. Elevated Zn levels in the soil can lead to Zn toxicity in tobacco plants, which can cause growth problems and adversely affect crop health. Generally, the ISIKIZYA P1 and TUTUO P1 soils had Zn deficit while the KIPERA P1 had the sufficient range of Zn.
Manganese below 20 mg kg-1 may result in Mn deficiency in tobacco plants, leading to reduced growth and potential nutrient-related issues (Fageria et al., 2002). The recommended range of manganese for tobacco production in the soil is between 20 to 100 mg kg-1. Soil with Mn above 100 mg kg-1 can lead to Mn toxicity in tobacco plants, causing growth problems and potentially reducing yield and quality (Baker et al., 2000). The amount of Mn in top soil through the studied sites was above the sufficiency rate. The subsoil for ISIKIZYA P1 and TUTUO P1 site had the recommended range of Mn except for KIPERA P1, which had all top and sub soil with the amount above the recommended rate. This is probably due to weathering of Mn-rich rocks or minerals in the soil’s parent material can release Mn into the soil, leading to elevated levels over time (Uchida, 2000).
Sulfur is a key component of amino acids, particularly cysteine and methionine. Amino acids are the building blocks of proteins essential for various physiological processes in plants, including enzyme activity, photosynthesis, and cell structure (Bhat et al., 2020). In tobacco plants specifically, S contribute to the development of larger, healthier leaves with better curing and processing qualities (Tripathi et al., 2015). The high amount of S has negative effects on aroma, nicotine, combustibility, ash color and smoking characteristics for tobacco crop (Xu et al., 2024). All the studied sites had accepted sulfur contents except KIPERA P1 subsoil, which had negligible S contents. This is probably due volatilization as sulfur dioxide (SO2) gas S dioxide can escape into the atmosphere, slow rate of S mineralization in subsoil also can contribute to low S levels or due to the composition of parent materials (Coelho-Junior et al., 2020). Flue-cured tobacco is highly sensitive to chlorine. High levels can negatively affect the burning quality, flavor, and leaf texture. The recommended chlorine levels in soil are less than 30–50 kg ha-1 of available chloride (Tripathi et al., 2015).
Ca/Mg ratio in the soil of approximately 6:1 to 8:1 is desirable for most crops’ growth including tobacco (Xu et al., 2022). This means that there should be six to eight times more Ca in the soil compared to Mg, maintaining a balanced Ca/Mg ratio is important because both calcium and magnesium are essential nutrients for plant growth (Uchida et al., 2018). Calcium is important for cell wall structure and function, while Mg is a central component of chlorophyll, essential for photosynthesis (Nadeem et al., 2018). There are un-desirable ratios through the study sites, addition of fertilizer containing calcium and Mg is required to supplement these nutrients in the soils.
The K/Mg ratio indicates the balance between K and Mg in the soil. A balanced K/Mg ratio is important for crop health. The potassium-to-magnesium ratio in the soil for tobacco cultivation is an important factor to consider in maintaining soil fertility and promoting healthy plant (Tian et al., 2010). In many cases, a ratio close to 1:1 is sufficient, meaning that there should be approximately equal amounts of K and Mg in the soil (Vatansever et al., 2017). According to Knecht and Göransson (2004), higher K/Mg ratio can lead to Mg deficiency in plants, which can negatively affect plant health and development.
The potassium-to-cation exchange capacity (K/CEC) ratio in the soil for tobacco cultivation is an important parameter that reflects the balance between K and the soil’s cation exchange capacity. The cation exchange capacity represents the soil’s ability to retain and release cations, which include essential plant nutrients like K, Ca, Mg, and others. The K/CEC ratio is a useful indicator of K availability and nutrient management in the soil (Dhaliwal et al., 2022). A balanced ratio generally falls within the range of 2% to 5% K in relation to the cation exchange capacity. This means that K should make up around 2% to 5% of the total cations in the soil. Maintaining an appropriate K/CEC ratio ensures that there is an adequate supply of K available to tobacco plants (Kim et al., 2021). The K/CEC ratio for all the sites were below the recommended for top soils while varying with increase of depth, this is probably due to percolation of water leading to translocation of basic cations in the specific pedons.
The soils for TUTUO P1 pedons classified as Alfisols as per the USDA and Luvisols in FAO-WRB soil classifications with Umbric epipedon having Udic Paleustalfs features and Coluvic, Humic, and Neocambic properties, as a result of high organic matter. Alfisols (Luvisols), these soils have a horizon with a significant accumulation of clay, iron, and/or organic matter. The soils often exhibit a distinct horizon known as an “illuvial horizon” (Brevik et al., 2016; Schad, 2023). KIPERA P1 pedons classified as Oxisols in the USDA, Ferrasols in FAO-WRB soil classifications. Having Xanthic, Geric features and Aric, Clayic and Ochric properties, as the results of continuous ploughing at the depth of ≥20 cm, textural class of clay in ≥30 cm thick and having an organic carbon of ≥0.2% in the layer from the mineral soil surface at the depth of 10 cm from the mineral soil surface. Oxisols (Ferralsols) soil characterized by the accumulation of iron and aluminium oxides, as well as low cation exchange capacity (Schad, 2023). The pedon ISIKIZYA P1 classified as Mollisols in the USDA soil taxonomy and as Chernozems in the FAO-WRB soil classification having Gleyic, Fluvic and Duric features and Aric, Cambic properties, as the results of continuous ploughing and having the albic materials starting from ≤ 50 cm from the soil surface. Mollisols (Chernozems) are soils that are rich in organic matter, have a deep, dark, fertile topsoil (A horizon), and are associated with grassland ecosystems characterized by their high fertility and suitability for agriculture (Brevik et al., 2016; Soil Survey Staff, 2022; Schad, 2023).
The results provide the fertility status of the studied pedons (ISIKIZYA P1 - Uyui, TUTUO P1 - Sikonge, and KIPERA P1 - Tabora) that are under tobacco production. The ISIKIZYA P1 topsoil was characterized by fine sand, while the subsoil was friable to hard soils with many fine (m.f) pores. The data suggests that soil fertility indicators such as pH, total N, available P, K, OC, Zn, and S for ISIKIZYA P1 are the main constraints, and management strategies should target these components for sustainable crop production and system productivity. The fertility status for the studied pedons was generally rated as medium. The ISIKIZYA P1 pedon had features of Mollisols, TUTUO P1 had features of Alfisols, while the KIPERA P1 pedon had features of Cambisols.
However, this study has limitations that should be acknowledged. The lack of microbial analyses means that the role of soil microorganisms in nutrient cycling and fertility has not been explored, which could provide further insights into soil health. Additionally, the limited geographic scope of the study restricts its applicability to broader regions with different soil types or climatic conditions. Future research could focus on integrated soil management practices, incorporating both organic and inorganic inputs for improved soil fertility and sustainability. Long-term studies are needed to track soil fertility trends over time, considering the cumulative effects of various management practices on soil health and productivity.
Based on landform features and soil physico-chemical properties, the soils represented by the three studied profiles were recommended as suitable for tobacco production. Extra attention should be given to addressing possible deficiencies of some nutrient elements through the use of NPK and CAN fertilizers in recommended rates. The study sites will benefit if proper soil fertility management practices are employed with the following recommendations. The soil analysis suggests varying fertility and challenges across the sites. Very low pH (soil acidity), low organic matter, and nutrient imbalances require targeted interventions, including pH correction, organic matter addition, and nutrient-specific fertilization for optimal tobacco production. Proper soil management practices, such as avoiding compaction, incorporating organic matter, and adjusting nutrient levels, are crucial for sustainable agriculture in these sites.
The original contributions presented in the study are included in the article/supplementary material. Further inquiries can be directed to the corresponding author.
DM: Conceptualization, Data curation, Formal analysis, Funding acquisition, Investigation, Methodology, Project administration, Resources, Software, Validation, Visualization, Writing – original draft, Writing – review & editing. BM: Supervision, Validation, Writing – review & editing. EN: Supervision, Validation, Writing – review & editing.
The author(s) declare that no financial support was received for the research and/or publication of this article.
The authors declare that the research was conducted in the absence of any commercial or financial relationships that could be construed as a potential conflict of interest.
All claims expressed in this article are solely those of the authors and do not necessarily represent those of their affiliated organizations, or those of the publisher, the editors and the reviewers. Any product that may be evaluated in this article, or claim that may be made by its manufacturer, is not guaranteed or endorsed by the publisher.
AbdelRahman M. A., Metwaly M. M., Afifi A. A., Scopa A. (2022). Assessment of soil fertility status under soil degradation rate using geomatics in west nile delta. Land 11 (8), 1256. doi: 10.3390/land11081256
Alloway B. J. (2013). “Heavy metals and metalloids as micronutrients for plants and animals,” in Heavy metals in soils: Trace Metals and Metalloids in Soils and Their Bioavailability. (Dordrecht Heidelberg New York, London, UK: Springer), 195–209. doi: 10.1007/978-94-007-4470-7
Andersson Djurfeldt A., Djurfeldt G., Hillbom E., Isinika A. C., Joshua M. D. K., Kaleng’a W. C., et al. (2019). Is there such a thing as sustainable agricultural intensification in smallholder-based farming in sub-Saharan Africa? Understanding yield differences in relation to gender in Malawi, Tanzania and Zambia. Dev. Stud. Res. 6, 62–75. doi: 10.1080/21665095.2019.1593048
Baker W. H., Bell P. F., Campbell C. R., Cox F. R., Donohue S. J., Gascho G. J., et al. (2000). Reference Sufficiency Ranges for Plant Analysis in the Southern Region of the United States. (Raleigh, North Carolina: North Carolina Department of Agriculture and Consumer Services), 134pp.
Baumann K., Schöning I., Schrumpf M., Ellerbrock R. H., Leinweber P. (2016). Rapid assessment of soil organic matter: Soil colour analysis and Fourier transform infrared spectroscopy. Geoderma 278, 49–57. doi: 10.1016/j.geoderma.2016.05.012
Bhat B. A., Islam S. T., Ali A., Sheikh B. A., Tariq L., Islam S. U., et al. (2020). “Role of micronutrients in secondary metabolism of plants,” in Plant Micronutrients: Deficiency and Toxicity Management. (Switzerland: Springer Nature), 311–329. doi: 10.1007/978-3-030-49856-6
Blancquaert D., De Steur H., Gellynck X., Van Der Straeten D. (2017). Metabolic engineering of micronutrients in crop plants. Ann. New York Acad. Sci. 1390, 59–73. doi: 10.1111/nyas.2017.1390.issue-1
Bremner J. M., Mulvaney C. S. (1982). Nitrogen—total. Methods of soil analysis. Chem. Microbiological Properties 9, 595–624. doi: 10.2134/agronmonogr9.2.2ed.c31
Brevik E. C., Calzolari C., Miller B. A., Pereira P., Kabala C., Baumgarten A., et al. (2016). Soil mapping, classification, and pedologic modeling: History and future directions. Geoderma 264, 256–274. doi: 10.1016/j.geoderma.2015.05.017
Chaudhari P. R., Ahire D. V., Ahire V. D., Chkravarty M., Maity S. (2013). Soil bulk density as related to soil texture, organic matter content and available total nutrients of Coimbatore soil. Int. J. Sci. Res. Publications 3, 1–8.
Chen D., Zhou Y., Wang G., Dai K., Li J., Song X., et al. (2025). Biochar-based organic fertilizer application promotes the alleviation of tobacco (Nicotiana tabacum l.) continuous cropping obstacles by improving soil chemical properties and microbial community structure. BMC Plant Biol. 25 (1), 271. doi: 10.1186/s12870-025-06266-7
Coelho-Junior H. J., Marzetti E., Picca A., Cesari M., Uchida M. C., Calvani R. (2020). Protein intake and frailty: A matter of quantity, quality, and timing. Nutrients 12, 2915. doi: 10.3390/nu12102915
Colombo C., Palumbo G., He J. Z., Pinton R., Cesco S. (2014). Review on iron availability in soil: interaction of fe minerals, plants, and microbes. J. Soils Sediments. 14, 538–548. doi: 10.1007/s11368-013-0814-z
Color M. S. (2009). Munsell Soil Colour Chart (Baltimore, Maryland, USA: Macbeth Division of Kollmorgen Corporation).
Cui Z., Zhang F., Chen X., Dou Z., Li J. (2010). In-season nitrogen management strategy for winter wheat: Maximizing yields, minimizing environmental impact in an over-fertilization context. Field Crops Res. 116 (1-2), 140–146. doi: 10.1016/j.fcr.2009.12.004
Dhaliwal S. S., Sharma V., Shukla A. K. (2022). Impact of micronutrients in mitigation of abiotic stresses in soils and plants—A progressive step toward crop security and nutritional quality. Adv. Agron. 173, 1–78. doi: 10.1016/bs.agron.2022.02.001
Fageria N. K., Baligar V. C., Clark R. B. (2002). Micronutrients in crop production. Adv. Agron. 77, 185–268. doi: 10.1016/S0065-2113(02)77015-6
Funakawa S., Yoshida H., Watanabe T., Sugihara S., Kosaki T. (2012). “Soil fertility status and its determining factors in Tanzania,” in Soil Health and Land Use Management, Soriano M. C. H. (Ed.). (InTech Croatia and China). Available online at: http://www.intechopen.com/books/soil-health-and-land-use-management/soil-fertility-status-and-itsdetermining-factors-in-tanzania (Accessed April 20, 2024).
Gee G. W., Bauder J. W. (1986). Particle-size analysis. Methods Soil analysis: Part 1 Phys. Mineralogical Methods 5, 383–411. doi: 10.2136/sssabookser5.1.2ed.c15
Gerke J. (2022). The central role of soil organic matter in soil fertility and carbon storage. Soil Syst. 6, 1–33. doi: 10.3390/soilsystems6020033
Hu T.-W., Lee A. H. (2014). Tobacco control and tobacco farming in African countries. J. Public Health Policy 36, 41. doi: 10.1057/jphp.2014.47
IUSS Working Group WRB. (2022). World Reference Base for Soil Resources. International soil classification system for naming soils and creating legends for soil maps. 4th Edition. (Vienna, Austria: International Union of Soil Sciences (IUSS)). Available online at: https://www.isric.org/sites/default/files/WRB_fourth_edition_2022-12-18.pdf (Accessed April 20, 2024).
Kalala A. M., Msanya B. M., Amuri N. A., Semoka J. M. (2017). Pedological characterization of some typical alluvial soils of Kilombero District, Tanzania. American J. Agric. Forestry 5 (1), 1015011. doi: 10.11648/j.ajaf.20170501.11
Kebeney S. J., Msanya B. M., Ng’etich W. K., Semoka J. M. R., Serrem C. K. (2015). Pedological characterization of some typical soils of Busia County, Western Kenya: Soil morphology, physico-chemical properties, classification and fertility trends. Int. J. Plant Soil Sci. 4 (1), 29–44. doi: 10.9734/IJPSS/2015/11880
Khan F., Siddique A. B., Shabala S., Zhou M., Zhao C. (2023). Phosphorus plays key roles in regulating plants’ physiological responses to abiotic stresses. Plants 12 (15), 2861. doi: 10.3390/plants12152861
Kim H., Kojima N., Uchida R., Somekawa S., Inoue N., Kobayashi H., et al. (2021). The additive effects of exercise and essential amino acid on muscle mass and strength in community-dwelling older Japanese women with muscle mass decline, but not weakness and slowness: A randomized controlled and placebo trial. Aging Clin. Exp. Res. 33, 1841–1852. doi: 10.1007/s40520-020-01713-x
Knecht M. F., Göransson A. (2004). Terrestrial plants require nutrients in similar proportions. Tree Physiol. 24, 447–460. doi: 10.1093/treephys/24.4.447
Kuzilwa J., Ilembo B., Mpeta D., Coulson A. (2019). Contract farming in Tanzania: Experiences from tobacco and sunflower. Tanzanian Development: A Comp. Perspective 2019, 116–139.
Lindsay W. L., Norvell W. A. (1978). Development of a DTPA soil test for zinc, iron, manganese, and copper. Soil Sci. Soc. America J. 42, 421–428. doi: 10.2136/sssaj1978.03615995004200030009x
Lisuma J. B., Philip A. J., Ndakidemi P. A., Mbega E. R. (2022). Assessing residue effects of tobacco nicotine on the yields, nutrient concentrations and nicotine uptake of a subsequent maize crop. Field Crops Res. 277, 108401. doi: 10.1016/j.fcr.2021.108401
Lisuma J. B., Semoka J. M., Mbwambo A. F. (2023). Effect of Timing Fertilizer Application on leaf yield and quality of tobacco. Heliyon 9, e19670. doi: 10.1016/j.heliyon.2023.e19670
Liu M., Xue R., Han N., Yang S., Wang D., Hu Y., et al. (2024). The impact of different preceding crops on soil nitrogen structure and nitrogen cycling in tobacco-planting soil. Sci. Rep. 2024, 1–14. doi: 10.1038/s41598-024-52285-z
Lufega S., Msanya B. (2017). Pedological characterization and soil classification of selected soil units of Morogoro District, Tanzania. Int. J. Plant Soil Sci. 16, 1–12. doi: 10.9734/IJPSS/2017/32681
Makoi J. H., Mmbaga H. (2018). Soil fertility characterization in Mvumi and Mbogo-Komtonga Irrigation Schemes in Kilosa and Mvomero Districts, Morogoro Region, Tanzania. Int. J. Environment Agric. Biotechnol. 3 (3), 1088–1099. doi: 10.22161/ijeab/3.3.49
Manik S. N., Pengilley G., Dean G., Field B., Shabala S., Zhou M. (2019). Soil and crop management practices to minimize the impact of waterlogging on crop productivity. Front. Plant Sci. 10, 140. doi: 10.3389/fpls.2019.00140
Marzouk S. H., Tindwa H. J., Massawe B. H., Amuri N. A., Semoka J. M. (2023). Pedological characterization and soil fertility assessment of the selected rice irrigation schemes, Tanzania. Front. Soil Sci. 3, 117–1849. doi: 10.3389/fsoil.2023.1171849
Massawe I. K., Msanya B. M., Rwehumbiza F. B. (2017). Pedological characterization and fertility evaluation of paddy soils of Mvumi village, Kilosa District, Tanzania. Int. J. Curr. Res. Biosci. Plant Biol. 4 (2), 49–60. doi: 10.20546/ijcrbp.2017.404.009
Mattigod S. V., Zachara J. M. (1996). Methods of soil analysis PART 3 chemical methods soil science society of america book series (Madison WI, USA: JBD, Cheng SBHH, Westerman RL Methods of Soil Analysis).
Matus F. J., Escudey M., Förster J. E., Gutiérrez M., Chang A. C. (2009). Is the walkley–black method suitable for organic carbon determination in chilean volcanic soils? Commun. Soil Sci. Plant Anal. 40 (11-12), 1862–1872. doi: 10.1080/00103620902896746
Mbotwa C. H., Rweyemamu L. P. (2024). Tobacco use and associated factors among men in Tanzania: Further Analysis of the 2022 Tanzania demographic and health survey data. Tobacco Use Insights. 17, 1–8. doi: 10.1177/1179173X241259605
Meng X., Zhang X., Li Y., Jiao Y., Fan L., Jiang Y., et al. (2023). Nitrogen fertilizer builds soil organic carbon under straw return mainly via microbial necromass formation. Soil Biol. Biochem. 2023, 109–223. doi: 10.2139/ssrn.4427832
Merumba M. S. (2022). Effect of tillage, farmyard manure and potassium rates on the perfomance and profitability of cassava in selected districts of Kagera, Tanzania. Sokoine University of Agriculture, Tanzania. doi: 10.9734/IJPSS/2022/v34i1330972
Merumba M. S., Msanya B. M., Semu E., Semoka J. M. (2020). Pedological characterization and suitability assessment for cassava production in Bukoba, Missenyi and Biharamulo districts, Tanzania. Am. J. Agric. Forestry 8, 144–166. doi: 10.11648/j.ajaf.20200804.18
Mfaume D. P., Msanya B. M., Msaky J. J. (2019). Pedological Characterization and fertility, assessment of mbimba substation soils under coffee production in Mbozi District, Tanzania. Int. J. Adv. Sci. Res. Eng. 5, 221–236. doi: 10.31695/IJASRE.2019.33540
Moberg J. P. (2000). Soil and plant analysis manual (Copenhagen, Denmark: The Royal Veterinary and Agricultural University, Chemistry Department), 133.
Motsara M. R., Roy R. N. (2008). Guide to laboratory establishment for plant nutrient analysis (Vol. 19). (Rome: Food and Agriculture Organization of the United Nations), 101–122. Available online at: https://www.fao.org/4/i0131e/i0131e.pdf (Accessed April 4, 2024).
Msanya B. M., Kaaya A. K., Araki S., Otsuka H., Nyadzi G. I. (2003). Pedological characteristics, general fertility and classification of some benchmark soils of Morogoro District, Tanzania. Afr. J. Sci. Technol. 4, 101–111. doi: 10.18920/pedologist.46.2_79
Msanya B. M., Kimaro D. N., Kileo E. P., Kimbi G. G., Munisi A. I. M. (2001). “Land resources inventory and suitability assessment for the production of the major crops in the eastern part of morogoro rural district, Tanzania,” in Soils and Land Resources of Morogoro Rural and Urban Districts (Department of Soil Science, Faculty of Agriculture, Sokoine University of Agriculture, Morogoro, Tanzania).
Msanya B. M., Magoggo J. P., Otsuka H. (2002). Development of soil surveys in Tanzania. Pedologist 46, 79–88.
Msanya B. M., Mwasyika T. A., Amuri N., Semu E., Mhoro L. (2018). Pedological characterization of typical soils of Dodoma capital city district, Tanzania: soil morphology, physico-chemical properties, classification and soil fertility trends. Annuals Advanced Agric. Sci. 2, 59–71. doi: 10.22606/as.2018.24002
Mwalembe D. S., Massawe B. H. J., Nassary E. K. (2024). Impact of NPK and CAN fertilizers on tobacco performance and economic returns under three major soil types of Tabora, Tanzania. Int. J. Res. Agron. 7, 551–558. doi: 10.33545/2618060X.2024.v7.i12g.2209
Nadeem F., Hanif M. A., Majeed M. I., Mushtaq Z. (2018). Role of macronutrients and micronutrients in the growth and development of plants and prevention of deleterious plant diseases-a comprehensive review. Int. J. Chem. Biochem. 14, 1–22.
Okalebo J. R., Gathua K. W., Woomer P. L. (2002). Laboratory methods of soil and plant analysis: a working manual. Second edition. Sacred Africa Nairobi 21, 25–26.
Ouma J., Oino P. G., Mamboleo D. (2024). Evaluating tobacco farming practices and climate variability adaptation strategies in uriri sub-county: Mitigation and sustainable agricultural solutions. J. Res. Innov. Implic. Educ. 8 (3), 247–258. doi: 10.59765/seva9426h
Prowse M., Pérez Niño H. (2022). Tobacco farming and agrarian change in contemporary Southern Africa–an introduction. J. South. Afr. Stud. 48, 221–233. doi: 10.1080/03057070.2022.2096773
Rawal A., Chakraborty S., Li B., Lewis K., Godoy M., Paulette L., et al. (2019). Determination of base saturation percentage in agricultural soils via portable X-ray fluorescence spectrometer. Geoderma 338, 375–382. doi: 10.1016/j.geoderma.2018.12.032
Schad P. (2023). World Reference Base for Soil Resources—Its fourth edition and its history. J. Plant Nutr. Soil Sci. 186, 151–163. doi: 10.1002/jpln.202200417
Schjønning P., McBride R. A., Keller T., Obour P. B. (2017). Predicting soil particle density from clay and soil organic matter contents. Geoderma 286, 83–87. doi: 10.1016/j.geoderma.2016.10.020
Shonhe T., Scoones I., Mutyasira V., Murimbarimba F. (2022). Tobacco farming following land reform in Zimbabwe: A new dynamic of social differentiation and accumulation. J. South. Afr. Stud. 48, 251–271. doi: 10.1080/03057070.2022.2030954
Shukla A. K., Behera S. K., Pakhre A., Chaudhari S. K. (2018). Micronutrients in soils, plants, animals and humans. Indian J. Fertilisers 14, 30–54.
Soil Survey Staff. (2022). Keys to Soil Taxonomy. 13th edition (Kansa, US: USDA Natural Resources Conservation Service). Available online at: https://www.nrcs.usda.gov/sites/default/files/2022-09/Keys-to-Soil-Taxonomy.pdf (Accessed April 20, 2024).
Solanki M. (2021). The Zn as a vital micronutrient in plants. J. Microbiology Biotechnol. Food Sci. 11, 4026–4026. doi: 10.15414/jmbfs.4026
Tian H., Chen G., Zhang C., Melillo J. M., Hall C. A. (2010). Pattern and variation of C: N: P ratios in China’s soils: a synthesis of observational data. Biogeochemistry 98, 139–151. doi: 10.1007/s10533-009-9382-0
Tripathi D. K., Singh S., Singh S., Mishra S., Chauhan D. K., Dubey N. K. (2015). Micronutrients and their diverse role in agricultural crops: Advances and future prospective. Acta Physiologiae Plantarum 37, 1–14. doi: 10.1007/s11738-015-1870-3
Tucker K. L., Hannan M. T., Chen H., Cupples L. A., Wilson P. W., Kiel D. P. (1999). Potassium, magnesium, and fruit and vegetable intakes are associated with greater bone mineral density in elderly men and women. Am. J. Cin Nutr. 69, 727–736. doi: 10.1093/ajcn/69.4.727
Uchida R. (2000). Essential nutrients for plant growth: nutrient functions and deficiency symptoms. Plant Nutrient Manage. Hawaii’s Soils 4, 31–55.
Uchida D., Takaki A., Adachi T., Okada H. (2018). Beneficial and paradoxical roles of anti-oxidative nutritional support for non-alcoholic fatty liver disease. Nutrients 10, 977. doi: 10.3390/nu10080977
Uwitonze P., Msanya B. M., Mtakwa P. W., Uwingabire S., Sirikare S. (2016). Pedological characterization of soils developed from volcanic parent materials of Northern Province of Rwanda. Agriculture Forestry Fisheries 5, 225–236. doi: 10.11648/j.aff.20160506.13
Van Sundert K., Radujković D., Cools N., De Vos B., Etzold S., Fernández-Martínez M., et al. (2020). Towards comparable assessment of the soil nutrient status across scales—Review and development of nutrient metrics. Global Change Biol. 26, 392–409. doi: 10.1111/gcb.14802
Vatansever R., Ozyigit I. I., Filiz E. (2017). Essential and beneficial trace elements in plants, and their transport in roots: a review. Appl. Biochem. Biotechnol. 181, 464–482. doi: 10.1007/s12010-016-2224-3
Wang A., Ju B., Li D. (2019). Predicting base saturation percentage by pH—a case study of red soil series in South China. Agric. Sci. 10, 508–517. doi: 10.4236/as.2019.104040
Xu E., Liu Y., Gu D., Zhan X., Li J., Zhou K., et al. (2024). Molecular mechanisms of plant responses to copper: From deficiency to excess. Int. J. Mol. Sci. 25 (13), 6993. doi: 10.3390/ijms25136993
Xu Z., Wang Y., Sun D., Li H., Dong Y., Wang Z., et al. (2022). Soil nutrients and nutrient ratios influence the ratios of soil microbial biomass and metabolic nutrient limitations in mountain peatlands. Catena 218, 106–528. doi: 10.1016/j.catena.2022.106528
Yang Y., Ye C., Zhang W., Zhu X., Li H., Yang D., et al. (2023). Elucidating the impact of biochar with different carbon/nitrogen ratios on soil biochemical properties and rhizosphere bacterial communities of flue-cured tobacco plants. Front. Plant Sci. 14, 1250669. doi: 10.3389/fpls.2023.1250669
Zeng W., Zeng M., Zhou H., Li H., Xu Q., Fan L. (2014). The effects of soil pH on tobacco growth. J. Chem. Pharm. Res. 6 (3), 452–457.
Zewide I., Sherefu A. (2021). Review paper on effect of micronutrients for crop production. J. Nutr. Food Process 4, 1–8. doi: 10.31579/2637-8914/063
Zhou Z., Wang C., Jin Y. (2017). Stoichiometric responses of soil microflora to nutrient additions for two temperate forest soils. Biol. Fertility Soils 53, 397–406. doi: 10.1007/s00374-017-1188-y
Keywords: Alfisols, Oxisols, Mollisols, pedons, soil classification, tobacco enhancement, Tanzania
Citation: Mwalembe DS, Massawe BHJ and Nassary EK (2025) Pedological characterization of soils under tobacco cultivation: insights from Sikonge, Uyui and Tabora districts in Tabora Region, Tanzania. Front. Agron. 7:1456015. doi: 10.3389/fagro.2025.1456015
Received: 27 June 2024; Accepted: 10 March 2025;
Published: 31 March 2025.
Edited by:
Cathy Hawes, The James Hutton Institute, United KingdomReviewed by:
Tarek Alshaal, University of Debrecen, HungaryCopyright © 2025 Mwalembe, Massawe and Nassary. This is an open-access article distributed under the terms of the Creative Commons Attribution License (CC BY). The use, distribution or reproduction in other forums is permitted, provided the original author(s) and the copyright owner(s) are credited and that the original publication in this journal is cited, in accordance with accepted academic practice. No use, distribution or reproduction is permitted which does not comply with these terms.
*Correspondence: Dotto Simon Mwalembe, bXdhbGVtYmVkb3R0bzY3QGdtYWlsLmNvbQ==
Disclaimer: All claims expressed in this article are solely those of the authors and do not necessarily represent those of their affiliated organizations, or those of the publisher, the editors and the reviewers. Any product that may be evaluated in this article or claim that may be made by its manufacturer is not guaranteed or endorsed by the publisher.
Research integrity at Frontiers
Learn more about the work of our research integrity team to safeguard the quality of each article we publish.