- 1Center for Environment and Development Studies, Addis Ababa University, Addis Ababa, Ethiopia
- 2Water and Land Resource Center (WLRC), Addis Ababa University, Addis Ababa, Ethiopia
- 3School of Earth Sciences, Addis Ababa University, Addis Ababa, Ethiopia
- 4Department of Geography and Environmental Studies, Addis Ababa University, Addis Ababa, Ethiopia
- 5Australia River Institute and School of Engineering, Griffith University, Brisbane, QLD, Australia
- 6School of Engineering, Newcastle University, Newcastle upon Tyne, United Kingdom
It is widely acknowledged that the world is currently experiencing an unprecedented water shortage, with agriculture being a crucial contributor. This paper presents a synthesis of available evidence, identify knowledge gaps, and make a state-of-the-art synthesis on green water management in Ethiopia. A systematic review methodology was implemented, encompassing the compilation and analysis of peer-reviewed and gray literature. The paper demonstrates that rainfed agriculture, which relies on “green water” (soil moisture from rainfall), accounts for 80% of cultivated land and 60-70% of global crop production. However, green water management has not received adequate attention in water policy and land rehabilitation programs in Ethiopia, where irrigation is limited. The analysis reveals a large yield gap and water productivity gap for major crops like maize, sorghum, and wheat in Ethiopia’s rainfed agriculture. Increasing crop yields through better soil, water, and crop management practices can significantly improve water productivity, offering “windows of opportunity” to enhance food and water security. Thus, a paradigm shift from the traditional narrow focus on soil erosion control towards an integrated green-blue water management approach in water and agricultural policies and programs is urgently required. Increased investments and expertise in green water management at the government level are crucial. Optimizing the use of green water resources in rainfed farming can also unlock Ethiopia’s export potential while improving domestic water and food security through strategic virtual water trade. In conclusion, the review highlights unlocking the potential of green water resources through targeted investments and policy support for rainfed agriculture can significantly contribute to Ethiopia’s water and food security objectives in a cost-effective and environmentally sustainable manner.
1 Introduction
The world is facing an unprecedented water crisis. Among the key factors influencing this situation are agricultural water management problems. The current global water demand for all uses is approximately 4,600 km3 per year. However, this demand is expected to increase by 20% to 30% by the year 2050, reaching between 5,500 and 6,000 km3 per year. This increase will be driven by factors such as economic development, population growth, and changing consumption patterns (Burek et al., 2016; WWAP, 2018). Globally, water use for agriculture currently accounts for 70% of the total freshwater withdrawals. With rising water demands for food production, intensifying competition for water resources, and the projected impacts of climate change, there is an urgent need to find new approaches to water management (WWAP, 2016). Since agriculture is the largest consumer of water, enhancing agricultural water productivity is viewed as one of the most crucial strategies for addressing current and future water scarcity issues (Rockström et al., 2009; Molden et al., 2010).
Agriculture serves as the primary engine driving economic growth and ensuring food security in Ethiopia. In 2010, agriculture contributed significantly, approximately 46%, to the country’s gross domestic product (GDP) (MoFED, 2010). In recent years, this sector has accounted for approximately 34% of the GDP. The agricultural and forestry sectors engage a significant labor force of 35.5 million individuals, accounting for 63.7% of total employment. Ethiopia’s agricultural landscape comprises nearly 18.6 million hectares of cropland, with the per capita cropland area averaging a modest 0.15 hectares in 2021. The country’s cereal production reached 30.1 million tonnes in 2021. However, Ethiopia remains heavily reliant on cereal imports, having imported approximately 3.3 million tonnes of cereals in 2021. Rainfed agricultural land covers 59% of Ethiopia’s total land area, 33% of which is currently cultivated (Kassawmar et al., 2018). The term “rainfed areas” refers to regions where reliable rainfall is adequate to sustain crop cultivation and crop-livestock mixed farming systems. These production systems are dominated by smallholder farmers practicing rainfed agriculture with a high degree of land degradation and climate variability (Teferi et al., 2023). The levels of food insecurity and undernourishment in the nation are substantial. Between 2020 and 2022, an estimated 26.4 million individuals faced undernourishment, with over one-fifth (21.1%) of the population experiencing severe levels of food insecurity (FAO, 2023). Given the significant contribution of agriculture to the economy, employment, and food security, developing this sector through investments, technological advancements, infrastructure improvements, and policy support is critical for Ethiopia’s overall development and the well-being of its population.
The productivity of cereals in Ethiopia is very low, with average cereal yields ranging from 1.7 to 3.7 t/ha (Central Statistics Agency of Ethiopia (CSA), 2018). There is a yield gap between on-station yield and actual farm yield (Mann and Warner, 2017). For example, in Ethiopia, the national average yield gaps for maize, wheat, and sorghum were 10.25, 6.06, and 4.85 t/ha, respectively (http://www.yieldgap.org/ethiopia). Such large yield gaps suggest untapped potential for yield increases. As reported by Rockström (2003), in the low-yield range, there is great potential to improve water productivity (up to fivefold). Water productivity increases dramatically, from ~ 2 kg grain/mm at ~ 0.5 t/ha yield to ~ 10 kg grain/mm of evapotranspiration flow at ~ 3 t/ha. Despite the great potential for upgrading rainfed agriculture, investments to reduce yield gaps and increase water productivity are lacking. Such an improvement in water productivity by increasing crop yield offers ‘windows of opportunity’ for countries such as Ethiopia, as more frequent dry spells and droughts are occurring due to the changing climate.
Studies indicate that various factors contribute to the gaps between actual and potential crop yields. Van Dijk et al. (2017, 2020) outlined four components of the total yield gap: (1) the technical efficiency yield gap, (2) the economic yield gap, (3) the allocative yield gap, and (4) the technology yield gap. The major contributor to the yield gap was identified as the technology yield gap, which was partly caused by farmers’ limited adoption and use of fertilizers and improved seed varieties. Although factors related to technology adoption, such as in-creased input use and the adoption of new agricultural technologies, account for the largest portion of the total yield gap (Van Dijk et al., 2020; Assefa et al., 2020), socioeconomic factors (e.g., profitability) are also important for reducing yield gaps. Reducing the technology yield gap also requires consideration of biophysical characteristics such as soil erosion, rainfall variability, low soil water holding capacity, low soil infiltration, and poor water and nutrient uptake by crops (Rockström and Falkenmark, 2000). Crucially, land degradation (Teferi et al., 2023) and increasing rainfall variability driven by climate change pose a significant threat to national food security in Ethiopia. Projected increases in temperature and rainfall variability due to climate change, leading to recurrent droughts and dry spells (IPCC, 2007), have been reported as causes of crop failure or yield reductions in semiarid and dry subhumid tropical regions in general (Barron et al., 2003). For regions in the equatorial tropics, an increase of 1°C in the mean temperature is linked to a 10% reduction in crop yields (Sova et al., 2019). Additionally, soil erosion, resulting in soil losses of 1.5 to 2 billion tonnes annually (35 t/ha), directly impacts food production in the Ethiopian highlands, estimated at a monetary value of US$1 to 2 billion per year (Sonneveld, 2002). Traditional agricultural practices on croplands are considered major factors affecting the high level of soil erosion (Sonneveld and Keyzer, 2003). For example, conventional tillage practices in the Ethiopian highlands have been frequently reported as major contributors to soil erosion, low infiltration and low agricultural productivity (Hurni et al., 2005; Temesgen et al., 2009).
If agriculture is to meet the challenge of feeding more than 9 billion people by 2050 while still leaving sufficient water available for other uses, it will be necessary to protect and optimize the management of all the different forms or “colors” of water (e.g., blue, green, ultraviolet, white, and gray) represented in the hydrologic cycle (Savenije, 2000; Schneider, 2013). This type of classification provides a comprehensive framework to account for all components of the water cycle and human interactions with water resources. This holistic view is crucial for integrated water resource management. Within this framework, green water, represents the rainwater stored in unsaturated soil, which is utilized for biomass growth (e.g., Savenije, 1999; Hoekstra, 2019; Falkenmark and Rockström, 2006), as opposed to blue water which denotes liquid water in lakes, rivers, wetlands, and aquifer systems (Rockström, 1997; Rockström and Falkenmark, 2000). In addition to green water and blue water, there is also white water, gray water, and ultraviolet water. White water refers to the portion of rainfall that is intercepted and immediately evaporates back into the atmosphere, as well as nonproductive evaporation from open water bodies and soil surfaces (Savenije, 2000). Gray water is the return flow, such as sewage from cities and industries, that flows back into rivers or percolates into aquifers. Virtual water is the water consumed in the production of traded goods and products (Allan, 1993). Ultraviolet water is a term first introduced by Savenije (2000) to refer to this concept of virtual water contained in traded products.
Green water in Ethiopia, where rainfed agriculture dominates is very important and crucial for agricultural expansion and increased productivity (Hoekstra and Mekonnen, 2012). Unfortunately, the country’s agricultural sector faces severe issues in the efficient management of green water resources. Through the optimization of green water resources, Ethiopia has the potential to improve crop yields, enhance water productivity, and enhance food security (Rockström and Falkenmark, 2000; Rockström, 2003). This will require a shift from conventional blue water focused practice to comprehensive practices which accounts for green water as a key component in the system’s inherent water cycle.
Improving water productivity in the agricultural sector is crucial for enhancing the livelihoods of smallholder farmers in Ethiopia. To this end, the Government of Ethiopia (GoE), in collaboration with multiple development partners, has implemented various interventions in agriculture. These include the ongoing efforts of the Agricultural Transformation Institute (since 2010). These programs aim to boost agricultural productivity and support sustainable water management practices among smallholder farmers. It is worth noting that these interventions were concentrated on rainfed agriculture until 1995, after which the focus shifted.
Although these efforts have resulted in many ecological benefits, they have fallen short in addressing the pressing issue of closing the yield gap. Notably, the primary focus of nearly all projects has been on mitigating soil erosion and reversing land degradation, with limited attention devoted to boosting crop productivity and bridging the yield gap. While rainwater is a major contributor, by enabling agricultural production, to the livelihoods of smallholder farmers, it has not received adequate attention in water policy and in land rehabilitation programs (Hagos et al., 2011; FDRE, 2013; Planning and Development Commission, 2020). The past and present focus of Ethiopia’s water management has been on constructing irrigation infrastructures and overseeing blue water resources, which can be costly endeavors. The current practice of not giving proper attention to rainfed agriculture aligns with Ethiopia’s Ten Years Development Plan (Planning and Development Commission, 2020). The development plan aims to shift away from a reliance on rainfed, green water-based agriculture toward increasing irrigation (blue water) infrastructure and expanding large-scale, mechanized farming by smallholders acquiring more land. Little focus has been given to improving the productivity and management of existing rainfed, green water agricultural systems.
The concept of green water management is not new to Ethiopia. What is new is the need to increase awareness among all stakeholders about its potential large-scale impacts on agricultural productivity. Despite its importance, green water is likely the most undervalued resource of all water resources and is often not featured on the water agenda. There is no state-of-the-art synthesis that presents research results, gaps and future directions on green water management in the country. The relative im-portance of green water use in relation to food security and water security has been noted by many researchers (Falkenmark and Rockström, 2004; Keys and Falkenmark, 2018). Dile et al. (2018) reviewed research conducted in Ethiopia with an emphasis on advances in blue water resources research. They high-lighted the different types of hydrological models applied in Ethiopia and the availability of runoff, groundwater recharge, sediment transport, and tracer data. Asmamaw (2017) reviewed the impacts of conservation tillage on water balance and crop yield in Ethiopia. The review, however, did not cover all aspects of green water management; instead, it focused on one part of green water management, especially conservation tillage. The studies mentioned previously did not concentrate on or address the management of green water resources. Therefore, this review focuses on two closely related topics: the yield gap in rainfed agriculture and the management of green water resources, highlighting the importance of green water management in addressing the yield gap in rainfed agriculture. Using a systematic review methodology, this study examines the current state of knowledge on green water management and its impact on addressing the yield gap in rainfed agriculture. The findings of this review are relevant to policymakers, agricultural water management researchers, and practitioners seeking to improve crop yields and promote sustainable agriculture in rainfed areas, particularly in Ethiopia.
2 Methods
To gain a comprehensive understanding of research on green water (referring to rainwater stored in the soil), a systematic review process was undertaken. Systematic reviews are valuable for synthesizing trends and extracting key findings from large bodies of information (Petticrew and Roberts, 2008). The review concentrated on peer-reviewed journal publications as well as other relevant technical and programmatic publications (known as “gray” literature) related to green water management. During the initial screening stage, three inclusion criteria were applied: (1) the search criteria are indicated in the search string in Table 1; (2) the publication was written in the English language; and (3) the publication focused on rainfed agriculture.
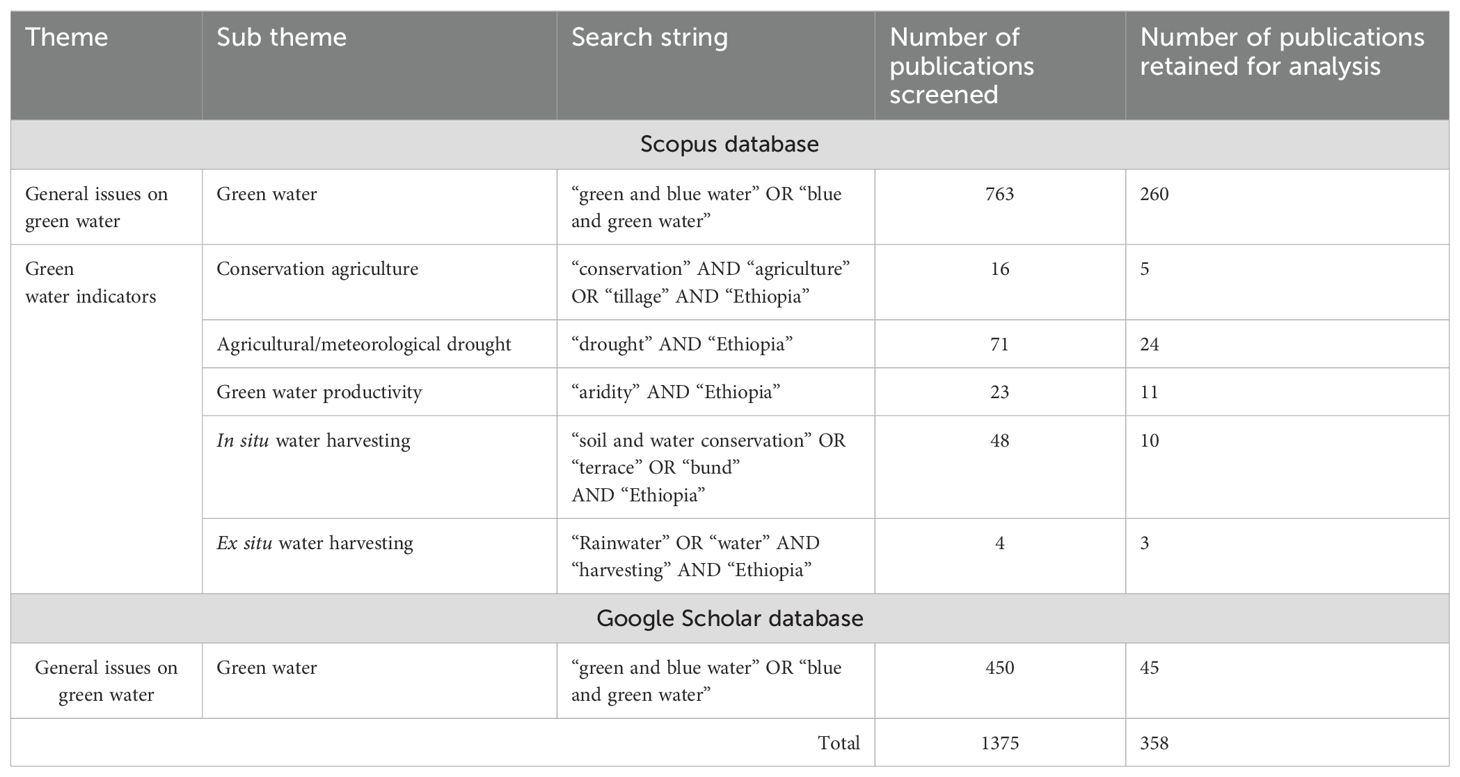
Table 1. Search string used in Scopus and the number of publications and grey literature included for the review.
In the data collection stage, these criteria were converted into a search query that was designed to identify and retrieve publications focused on green water concepts and related indicators. Both scientific publications and relevant technical and programmatic publications (gray literature) were targeted. The search string summarized in Table 1 was used to search peer-reviewed literature (i.e., scientific journals) from the Scopus database. Scopus (https://www.elsevier.com/solutions/scopus) was selected because of its large archival of rainfed agriculture journals. The search returned 763, 16, 71, 23, 48, and 4 publications on green water, conservation agriculture, drought, water productivity, in situ water harvesting, and ex situ water harvesting, respectively, that met the four inclusion criteria. The literature search was restricted to publications from January 2000 through December 2019. We chose this search period because the concept of green water management has gained significant attention in the past two decades (Cosgrove and Rijsberman, 2000). To capture “gray” literature, we searched, among others, the International Water Management Institute Library Catalog (https://www.iwmi.cgiar.org/publications/library-catalog/), the FDRE Ministry of Agriculture website, the World Bank e-library (https://elibrary.worldbank.org/) and the United Nations (http://www.unilibrary.org) repositories. A total of 45 gray literature samples were obtained from Google Scholar, which is widely known as a good source of gray literature (Giustini and Boulos, 2013).
After a thorough screening process, a total of 358 publications were retained for this review, consisting of 313 publications from Scopus and 45 from Google Scholar. Bibliographic data from these 358 publications were compiled using the Mendeley Desktop Reference Manager software for in-depth analysis. Additionally, to answer research questions related to virtual water and water footprints, data were obtained and analyzed from http://www.yieldgap.org/ and https://www.trademap.org. The first author and the second author of this paper independently assessed each study against the predefined eligibility criteria. This dual-assessment approach aimed to minimize errors and reduce the potential for individual biases to influence the selection process. Inclusion decisions were based on a thorough evaluation of the full texts of potentially eligible studies.
We employed a systematic and iterative process to synthesize trends and extract key findings. Firstly, we conducted a descriptive analysis of the extracted data to identify patterns and themes. We then used a thematic analysis approach to categorize and code the data, using a set of predetermined codes and themes related to green water management. To ensure consistency and accuracy, we used a data extraction form to collect and organize the data from each publication. The form included fields for publication characteristics, interventions, outcomes, and conclusions. We also used a coding scheme to classify the publications according to their focus on green water management, conservation agriculture, drought, water productivity, and in situ and ex situ water harvesting. We then used a narrative synthesis approach to synthesize the findings across the publications. This involved identifying and describing the main trends, patterns, and relationships in the data, as well as highlighting any inconsistencies or gaps in the existing literature. A PRISMA flow diagram to illustrate the overall process of literature search and screening is presented in Figure 1.
3 Results and discussion
The following sections present the findings and insights from the analysis, which are organized into three key themes. Firstly, we examine the significance of green water to water and food security, reviewing the current state of knowledge in academic literature and its relevance in water resource assessment. Secondly, we provide an overview of the current state of rainfed agriculture intervention in Ethiopia, highlighting the challenges and opportunities in this context. Finally, we explore pathways to improving green water management in rainfed agriculture, with a focus on strategies to enhance water productivity, harness virtual green water, invest in green water management, and implement water harvesting and supplemental irrigation techniques. These findings and discussions provide a comprehensive understanding of the role of green water in addressing water and food security challenges in rainfed agriculture.
3.1 Green water in the academic literature
An analysis of the number of publications on the topic of ‘green water’ over the last two decades (2000-2019) revealed a steady increase since 2010 (Figure 2). As publications were identified through a systematic process, this increasing trend suggests a growing scholarly interest in the concept of green water. Overall, approximately 53% of all the publications reviewed were published in the past four years, i.e., between 2015 and 2019. Most of the studies (45%) were conducted at the local level, followed by the global scale (36%) and the national level (19%).
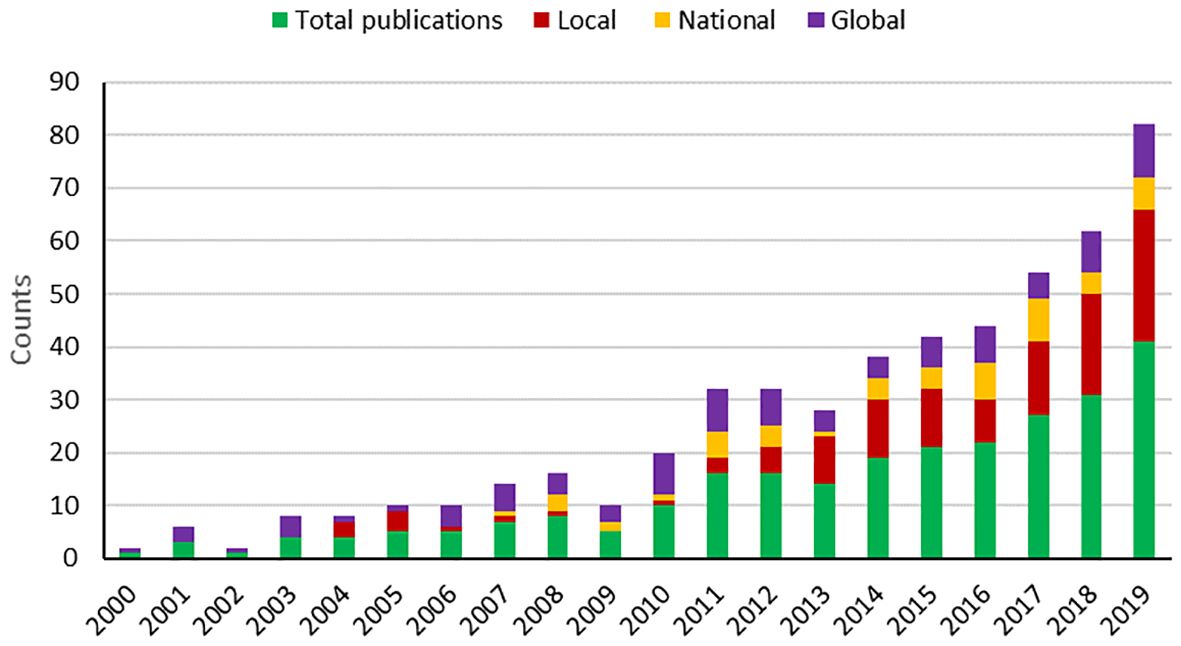
Figure 2. Peer-reviewed articles on green water by spatial scale: A summary of the literature reviewed, illustrating the relative emphasis on local, regional, and global scales in green water research.
A closer look at the most highly cited publications on green water in the Scopus database revealed that the majority of these impactful works were published in journals focused on hydrology, water resources, or ecological economics during the period from 2006 to 2011 (Table 2). The first publication that stands out in terms of the number of citations (Mekonnen and Hoekstra, 2011) is a study on the water footprint of crops.
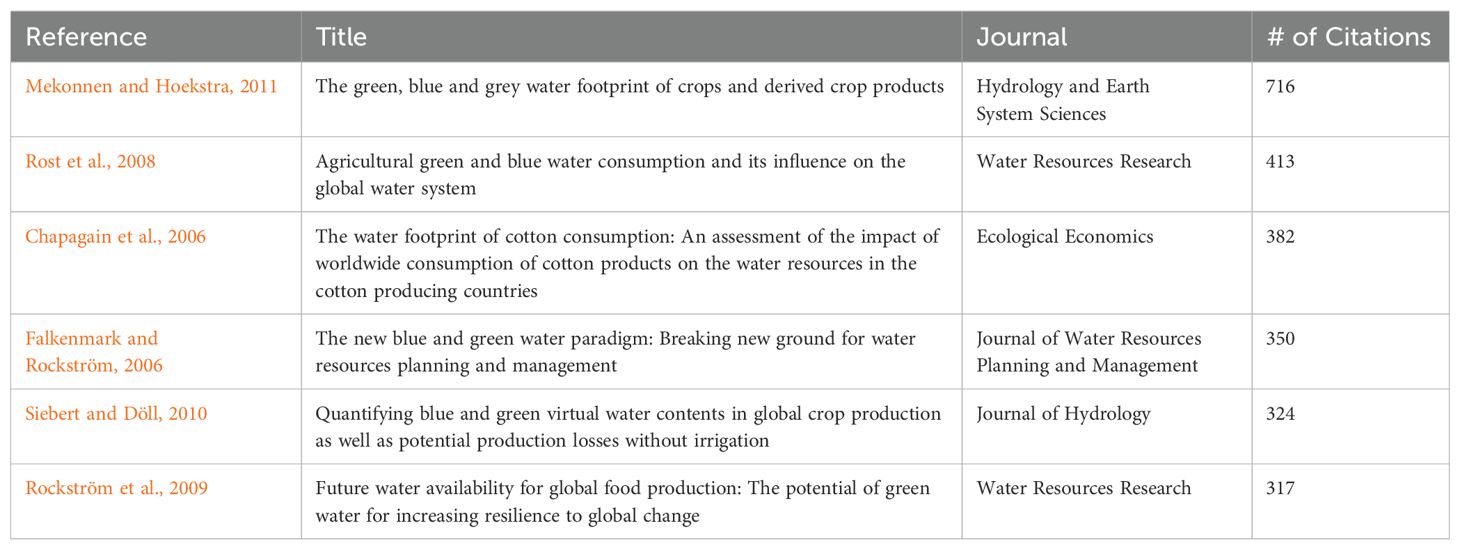
Table 2. Overview of the most cited peer reviewed articles about green water, receiving > 300 citations in Scopus until June 2020.
3.2 The relevance of green water in water resource assessment
Estimates based solely on blue water (surface and groundwater) suggest that a large portion of the world’s population will face absolute water scarcity in the coming decades (IWMI, 2000; Siebert and Döll, 2010). However, these estimates fail to account for green water (soil moisture from rainfall), which constitutes the majority of the water consumed in agriculture. Projections indicate a 60% increase in green water use and a 14% increase in blue water use for agriculture in sub-Saharan Africa by 2050 (Rockström et al., 2009; Falkenmark and Rockström, 2006; Hoff et al., 2010). Several researchers analyzing both green and blue water availability have found that many countries previously assessed as severely water stressed can actually produce sufficient food for their populations if green water resources are appropriately accounted for and managed effectively (Hoff et al., 2010; Mekonnen and Hoekstra, 2011; Gerten et al., 2011). Solely relying on blue water underestimates the total fresh-water resources contributing to food production. While more than 3 billion people were estimated to face chronic shortages of blue water resources in 2000, this number decreased dramatically to less than 300 million (or 4.5% of the population) when green water resources from rainfall were also considered (Rockström et al., 2009).
3.3 Green water in water footprint analysis
The “water footprint” is a concept initially introduced by Hoekstra and Hung (2002) and further developed by Hoekstra and Chapagain (2008) to quantify the total volume of freshwater utilized in producing the goods and services consumed by a country. Water footprint analysis provides a framework for assessing water requirements for production and identifying water saving opportunities, contributing to better water resource management (e.g., Vanham et al., 2013; Ercin and Hoekstra, 2014). The water footprint consists of three components: the green water footprint, which is the rainwater consumed and is especially relevant for crop production; the blue water footprint, which is the consumption of surface and groundwater resources; and the grey water footprint, an indicator of freshwater pollution calculated as the volume needed to assimilate pollutant loads based on water quality standards. Water footprint analysis offers a comprehensive way to evaluate total freshwater appropriation and identify more sustainable water use across the green, blue and grey components.
3.4 Overview of rainfed agricultural interventions in Ethiopia
Table 3 shows that the percentage of agricultural land increased from around 30.5% in 1995 to 34.09% in 2020 in Ethiopia. Similarly, the arable land percentage and arable land area have also increased over the years, while the arable land per person has decreased slightly. The increasing trend in agricultural land and arable land suggests that more land is being utilized for agricultural purposes over time in Ethiopia.
Historically, agricultural investments in developing countries have prioritized irrigated lands and high-rainfall areas with the goal of boosting food production. Even in high-rainfall regions, the main focus has been on using improved seed varieties and fertilizers instead of regenerative agriculture practices. This approach of concentrating investments in irrigated and high-potential areas has been driven by donor agencies and organizations providing development aid and financing for agriculture in these countries.
Over the last five decades, the Ethiopian government and various development partners have implemented several initiatives to boost agricultural productivity in the country (Figure 3). These initiatives include the Comprehensive Integrated Package Projects (CIPP, 1967-1975), the Minimum Package Program (MPP-I and II, 1971-1985), the Peasant Agricultural Development Programme (PADEP, 1986-1995), the Participatory Small-scale Irrigation Development Programme (PASIDP I and II, 2008-2024), the Agricultural Growth Programs (AGP I and II, 2011-2020), the Small and Micro Irrigation Support (SMIS) Project (2015-2019), and the Agricultural Transformation Institute (ATI) Projects (2010-to date).
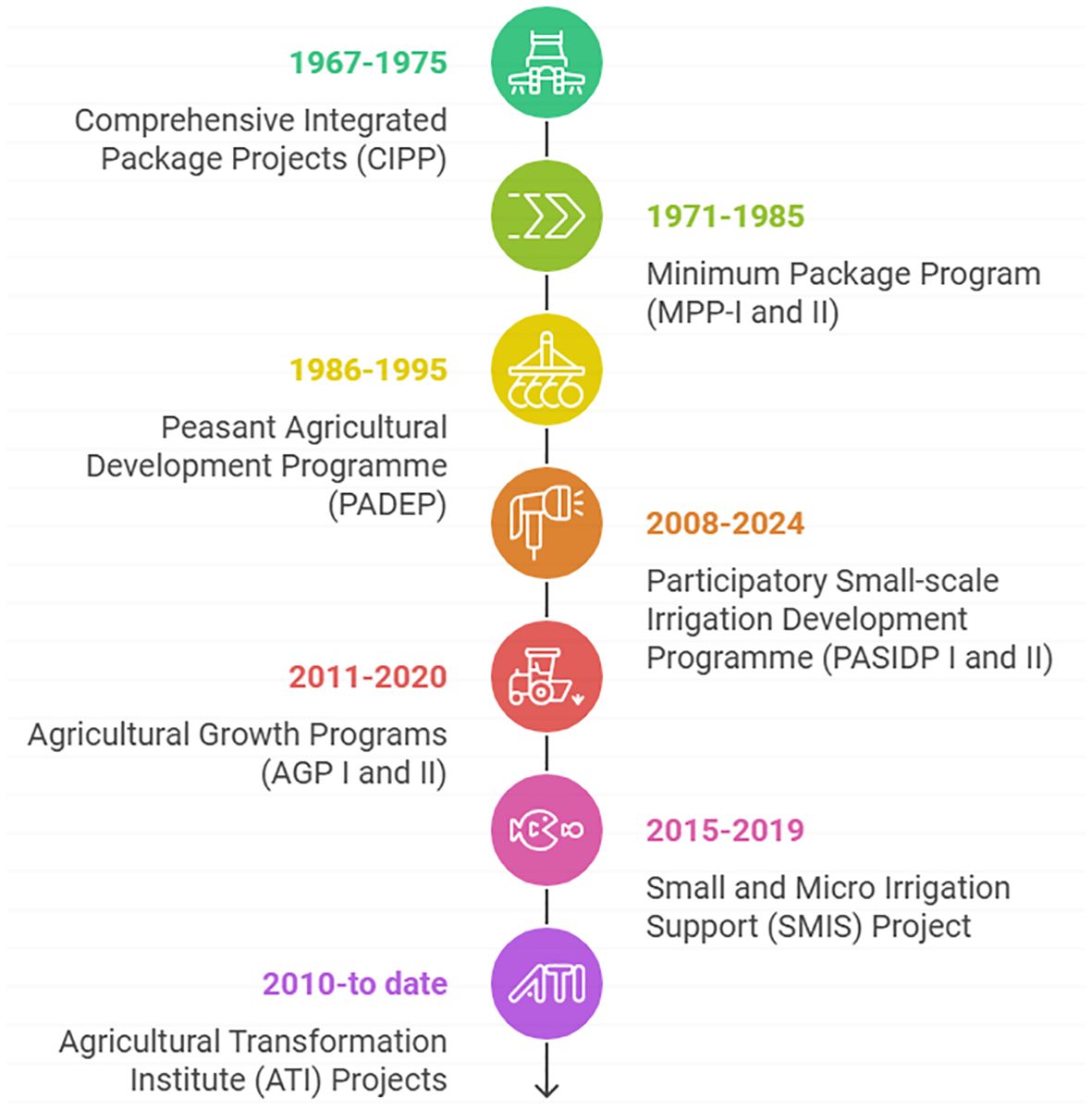
Figure 3. Evolution of agricultural interventions in Ethiopia: a visual representation of the country's progress, from traditional practices to modern innovations. Created with the help of https://www.napkin.ai/.
3.4.1 Comprehensive integrated package projects, 1967-1975
Several comprehensive pilot package projects have been implemented since 1967 (Tessema, 2000): the Chillalo Agricultural Development Unit (CADU), which later became the Arsi Rural Development Unit (ARDU); the Wolayita Agricultural Development Unit (WADU); and the Ada’a Woreda Development Project (ADDP). The comprehensive package approach focused on high potential areas and relied on external donor inputs to improve agricultural productivity of rainfed agriculture. Prior to the early 1970s, the Ethiopian government’s strategy to boost agricultural production focused on promoting large commercial farms and the use of capital-intensive machinery. The implementation of the comprehensive package approach proved to be excessively costly, making it financially impractical to replicate this model of integrated rural development in other regions of the country. The experience from a comprehensive package approach implemented through projects such as CADU had (1) generated evidence that small-scale farmers and tenants could contribute to national growth if they were supported with technologies; (2) promoted a shift in agricultural policies from large-scale commercial farming to peasant agriculture; (3) provided the experience and insight underlying the design of the country-wide, smallholder-focused Minimum Package Project that began in 1971; and (4) influenced the reorganization of the Ministry of Agriculture.
3.4.2 The minimum package program
The minimum package program (MPP) was established in 1971 to provide a basic package of fertilizer, seeds, credit and advice to smallholder farmers of rainfed agriculture within 5 km of all-weather roads, as the earlier CADU-ARDU projects were too costly. Approximately 10% of the farmers in the MPP I group (1971-1978) lived along main roads. It was implemented by the Extension and Project Implementation Department (EPID) under the then Ministry of Agriculture and Settlement (World Bank, 1979). The basic unit was the Minimum Package Project Area (MPPA), which typically covers approximately 10,000 farmers within a 5 km radius on either side of a 75 km all-weather road stretch (with one extension worker for every 2,000 farmers). Five such units constituted the MPP area. The MPPAs varied considerably in factors such as ecological conditions, land tenure, market access, and population density. By 1974, EPID had reached 50,000 out of Ethiopia’s total 5 million farmers at the time (less than 10% of farmers lived along major roads). By 1974, the EPID had extended MPP projects to all provinces of the country, with 48 MPPAs and 347 marketing centers. In this way, MPP I succeeded in supplying fertilizers and basic extension messages to farmers with a high potential of 280 weredas (World Bank, 1979).
Recognizing the need to expand to more smallholders, MPP II (1981-1985) aimed to increase productivity through fertilizer, improved seeds and soil and water conservation across 440 woredas in six major agroecological zones (Ibrahim, 2004). It introduced a non-fertilizer package with innovations such as row planting, better land preparation, weed/pest control, mulch and manure use and soil and water conservation. Under MPP I, the distribution of farm inputs to farmers in the project area was handled mainly by the EPID through its marketing centers, but in MPP II, the EPID was terminated, and the peasant associations emerged as forces for development in the rural areas. In essence, the MPP transitioned from a localized fertilizer-focused approach to a nationwide program integrating improved seeds, agronomic practices and catchment-based soil and water conservation for a wider reach to smallholders.
3.4.3 Peasant agricultural development programme (1986-1995)
The Peasant Agricultural Development Program (PADEP) was designed to support the continuation of the largely successful MPP-II that was phased out in 1985. The program aimed to provide smallholder farmers of rainfed agriculture, organized into approximately 2,900 farmer service cooperatives, with agricultural inputs, credit facilities, and extension services following the training and visit extension system approach (World Bank, 1988). Although the Government’s Ten-Year Perspective Plan (TYPP, 1985-1994), which was issued in 1984, emphasized the importance of the peasant sector and the implementation of the PADEP, smallholders were left out of agricultural growth due to producers’ cooperatives receiving most of the extension services and input supplies during the Derg regime (Ibrahim, 2004).
Previous projects focused on conventional seed-fertilizer packages with the aim of increasing crop yields, mostly in high-potential zones of rainfed agriculture. Recognizing that different agroecological zones need location-specific interventions, the PADEP classified the country into eight development zones. However, only the programs for Gojjam, Gonder, Wellega, Ilibabur, Kefa, Arsi, Bale, and Central Shewa secured funding and were implemented. To avoid the exclusion of low-potential, degraded highlands from PADEP, an alternative conservation-based development strategy was developed based on the findings of the Ethiopian Highland Reclamation Study (EHRS) (World Bank, 1988). Thus, two types of PADEPs were identified: those in the high-potential areas (Type I) and those in the low-potential and low-food deficit areas (Type II). In the degraded highlands, activities, including stone terraces, soil bunds, tree planting, gully rehabilitation and closure of hillsides, were undertaken by campaigns involving peasant associations under a food for work system.
3.4.4 Participatory small-scale irrigation development programme
The PASIDP was implemented from 2008 to 2015 to improve the food security, family nutrition, and income of poor rural households living in drought-affected and food-deficit areas in Tigray, Amhara, Oromia, and the Southern Nations, Nationalities and Peoples Region (SNNPR) of Ethiopia through a sustainable farmer-owned and farmer-managed system of small-scale irrigated agriculture. The PASIDP I project constructed a total of 121 irrigation schemes (i.e., 12,000 ha) and benefitted approximately 62,000 households (Garbero et al., 2016). The project comprises three main components: (a) small-scale irrigation scheme development, (b) agricultural development and (c) institutional capacity development. Although the agricultural development component involved activities such as watershed-based soil and water conservation, no emphasis was given to the ecological/environmental aspects of the project (Garbero et al., 2016).
The PASIDP II (2017–2024) has an overall goal of increasing prosperity and improving resilience to shocks in food insecure areas of Ethiopia. The project achieves its goal and objective through the implementation of three components: the use of irrigation infrastructure, the adoption of watershed management practices and the participation of farmers in capacity-building activities. The project was designed based on the assumption that poor farmers who are provided with access to a secure irrigation production base and access to markets and services will be able to produce and market greater volumes of crops in a profitable manner. The watersheds adjacent to the irrigation schemes are also included in the project to improve their productive capacity and enhance their resilience. The PASIDP II envisages the development of approximately 18,400 ha of small-scale irrigation.
The PASIDP follows a landscape approach to watershed management where both irrigation command areas and rainfed upstream areas adjacent to irrigation schemes are managed (Garbero et al., 2016). The PASIDP has demonstrated its relevance, particularly through the use of irrigation infrastructure as an effective climate risk management strategy, capacity building, the establishment of more than 175 water user associations, the adoption of biophysical watershed management measures and the adoption of different climate-smart agriculture (CSA) practices, such as agroforestry, integrated soil fertility, conservation agriculture and forage development and the construction of alternative water sources for adjacent upstream micro watersheds. Alternative sources of water are constructed to benefit upper stream communities and some households living adjacent to irrigation plans. An alternative source of water will enhance the agricultural productivity of farmers and avoid conflicts between upper stream communities and WUAs.
3.4.5 Agricultural growth program
The Agricultural Growth Programs (AGP I and II) from 2011–2020 aimed to increase agricultural productivity and market access for key crop and livestock products. AGP I covered 96 woredas in high-potential areas of the Amhara, Oromia, SNNP and Tigray regions, while AGP II expanded to 167, including the woredas of Gambela, Benishangul Gumuz, Harar and Dire Dawa prioritized based on market access, natural resource endowment, and irrigation potential (World Bank, 2017). The focus was on generating appropriate technologies through research, developing smallholder irrigation, and improving agricultural marketing and value chains. The key investments included small-scale irrigation development, water harvesting, microirrigation, irrigation management, and watershed management. As a result, the AGP supported irrigation and drainage services across 26,528 ha through small-scale schemes and microirrigation. Another 10,190ha received improved or rehabilitated irrigation and drainage treatments. Watershed management practices were adopted across 217,000 ha of land under the project (World Bank, 2017). In essence, AGP facilitated market-oriented agricultural growth in high-potential areas through strategic investments in smallholder irrigation, natural resource management, and value chain development over a decade.
The AGP encountered several significant challenges, including frequent organizational instability within government agencies, an absence of quality control mechanisms for small-scale irrigation projects, inadequate government support for private and public water work enterprises, and ineffective contract management practices. These challenges could result in the development of unsustainable irrigation infrastructure. Furthermore, the program neglected rainfed agricultural areas, despite its stated goal of promoting agricultural growth. By overlooking the majority of small-holder farmers who rely on rainfed agriculture and who contribute significantly to the nation’s overall food production, the AGP undermined inclusive growth and failed to address the needs of vulnerable rural communities.
3.4.6 Agricultural transformation institute projects
The Agricultural Transformation Institute (ATI), formerly ATA, was established in 2010 to identify constraints in agricultural development and recommend solutions (FAO, 2020; Diriba and Man, 2019). It focuses on the Agricultural Transformation Agenda and Agricultural Commercialization Clusters (ACC) initiative (Louhichi et al., 2019). The ATI has implemented approximately 48 innovative and large-scale strategic projects in the areas of soils, irrigation, mechanization, value chains, seeds, access to markets and rural finance to smallholder farmers and has conducted more than 250 strategy and policy studies, including mechanization, seeds and inputs, irrigation, fertilizer and agricultural strategies (FAO, 2020). These projects are mainly crop-focused or livestock-focused: the Production & Productivity Projects and Agribusiness & Markets Projects. Notable initiatives include the following:
● EthioSIS for localized fertilizer recommendation replacing the usual practice of blanket recommendations.
● ACC for promoting modern inputs such as improved seeds and access to credit/mechanization in 300 woredas for 9 priority crops. (i.e., wheat, maize, sesame, malt barley and horticulture crops – tomato, onion, banana, mango and avocado) (Louhichi et al., 2019).
● Efforts to boost agricultural mechanization through strategies, reducing import duties, and promoting tef row planters.
● Deploying 50 automatic weather stations for advisories through the Agromet project.
While ATI has made progress in various areas, there are concerns about its approach: (1) it has focused mostly on market-oriented, high-potential areas while neglecting the majority of smallholder farmers; (2) through the promotion of the Agri-cultural Commercialization Clusters (ACC), ATI has encouraged unsustainable cropland use by reducing crop rotation practices; and (3) residual moisture-based crops such as grasspea and chickpea, which are important for smallholders, have been completely ignored from research and development interventions. Thus, a more comprehensive strategy is needed, one that can make smallholder farmers productive within their existing rainfed farming practices without requiring capital-intensive options. This strategy should cater to the needs of the large majority of smallholders, promote sustainable cropland use, and include crops that are suitable for residual moisture conditions.
3.4.7 Small and micro irrigation support project
The Small Scale and Micro Irrigation Support Project (SMIS) was implemented in Tigray, Amhara, Oromia and Southern Nations, Nationalities and People`s Region (SNNPR) from 2015–2019 with funding support from the Embassy of the Kingdom of the Netherlands and the Government of Canada. The objective of the SMIS project was “to ensure that the concerned public and private institutions within each of the four regional states have the institutional, human and technical capacity required for gender-responsive identification, planning, design, construction and management support of sustainable SSI systems and micro-irrigation schemes in a coordinated manner and according to the adopted integrated watershed-based approach” (Jebelli, 2021). The main focus of the SMIS was in the capacity development for planning, design, construction and management of gender-responsive SSIs and micro-irrigation schemes.
The SMIS project adopted a watershed-based approach, rather than a scheme-based approach, for identifying and mapping small-scale irrigation sites and evaluating the water balance within the catchment area. Furthermore, 34 guidelines and manuals were developed to address the inconsistencies and variations that existed in the study, design, and implementation procedures for small-scale irrigation (SSI) projects. These disparities were prevalent across regions, among professionals within the same region, and even within the same institution. The primary reason for these discrepancies was the lack of standardized technical guidelines that were widely accepted and followed.
3.5 Pathways to improving green water management in rainfed agriculture
The pathways presented in this section were identified through a combination of literature review and synthesis of the state-of-the-art research on green water management. While some of the pathways were explicitly identified as such in the literature, others were derived from our analysis of the relationships and interactions between different components of green water management. By synthesizing the findings from multiple studies and identifying patterns and trends, we were able to identify key pathways that are critical to effective green water management. These pathways are not necessarily mutually exclusive, and some may overlap or intersect with one another. However, they represent a comprehensive and coherent framework for understanding the complex relationships between green water management and its various components.
3.5.1 Pathway 1: emphasis on improving water productivity
Improving water productivity holds significant potential for enhancing smallholder livelihoods by maximizing soil water availability and improving crop water uptake. Poor land and water management practices are major contributors to low water productivity in the drylands of sub-Saharan Africa, where up to 70-85% of rainfall may be effectively lost and unavailable for crop utilization (Rockström et al., 2007; Liniger et al., 2011). According to Rockström (2003), large yield gaps are not primarily caused by a lack of rainfall itself but rather result from poor land water management practices stemming from a complex interplay of factors. These factors include hydroclimate challenges such as droughts and dry spells, deficiencies in crop management practices, and inadequate crop protection measures against pests, diseases, and weeds (Rockström and Falkenmark, 2000; Rockström, 2003). Additionally, soil constraints such as low infiltrability and water holding capacity limit water availability for crops. These challenges are often exacerbated by human-induced land degradation and poor land use decisions. Addressing these multifaceted challenges requires an integrated approach encompassing improved drought and dry spell management, enhanced soil and water management strategies, and optimized crop management practices to improve water productivity (Rockström, 2003). By tackling these interconnected factors through holistic interventions, it is possible to mitigate the large yield gaps and low water productivity prevalent in the semiarid regions of sub-Saharan Africa.
The implementation of comprehensive measures of increasing water productivity is expected in any Green Water Management (GWM) program implemented in Ethiopia. The focus of most projects has been on reducing soil erosion, and less attention has been given to increasing water productivity. In any efforts of land and water management programs, practices that can enhance water productivity should be emphasized. Surface runoff generated within a watershed should be harvested and used as a valuable resource for agricultural activities through water harvesting techniques and conservation tillage practices. Rather than solely concentrating on soil conservation measures, where excess runoff is seen as a threat and diverted away from farmlands using cutoff drains, the focus should be on beneficially utilizing runoff water for agricultural purposes.
Table 4 shows the yield gap and green water productivity gaps for the three major crops in Ethiopia. The yield gap (Yg) is the difference between the water-limited yield potential (Yw) and the actual yield (Ya). For rainfed crops, although Yw represents the ceiling yield (van Ittersum and Rabbinge, 1997), achieving yields of 80% of Yw is realistic and profitable (Lobell et al., 2009). The exploitable yield gap represents the difference between Ya and 80% of Yw (Figure 4). There is a large yield gap between the potential farm/on-station yield and the actual farm yield. For example, the average yield gaps for maize, wheat, and sorghum were 2.34, 1.96, and 2.11 t ha-1, respectively (Table 4). Rainfed maize has the highest yield potential and largest yield gap, whereas sorghum has the smallest potential and yield gap. High yield gaps indicate that there is scope for improvement before reaching the practical limit of observed yield gaps (i.e., 80% of Yw) in the near future in rainfed agriculture if appropriate land and water management measures are taken. A significant reduction in crop yield gaps is highly required by improving crop yields to meet the growth in food demand and reduce poverty.
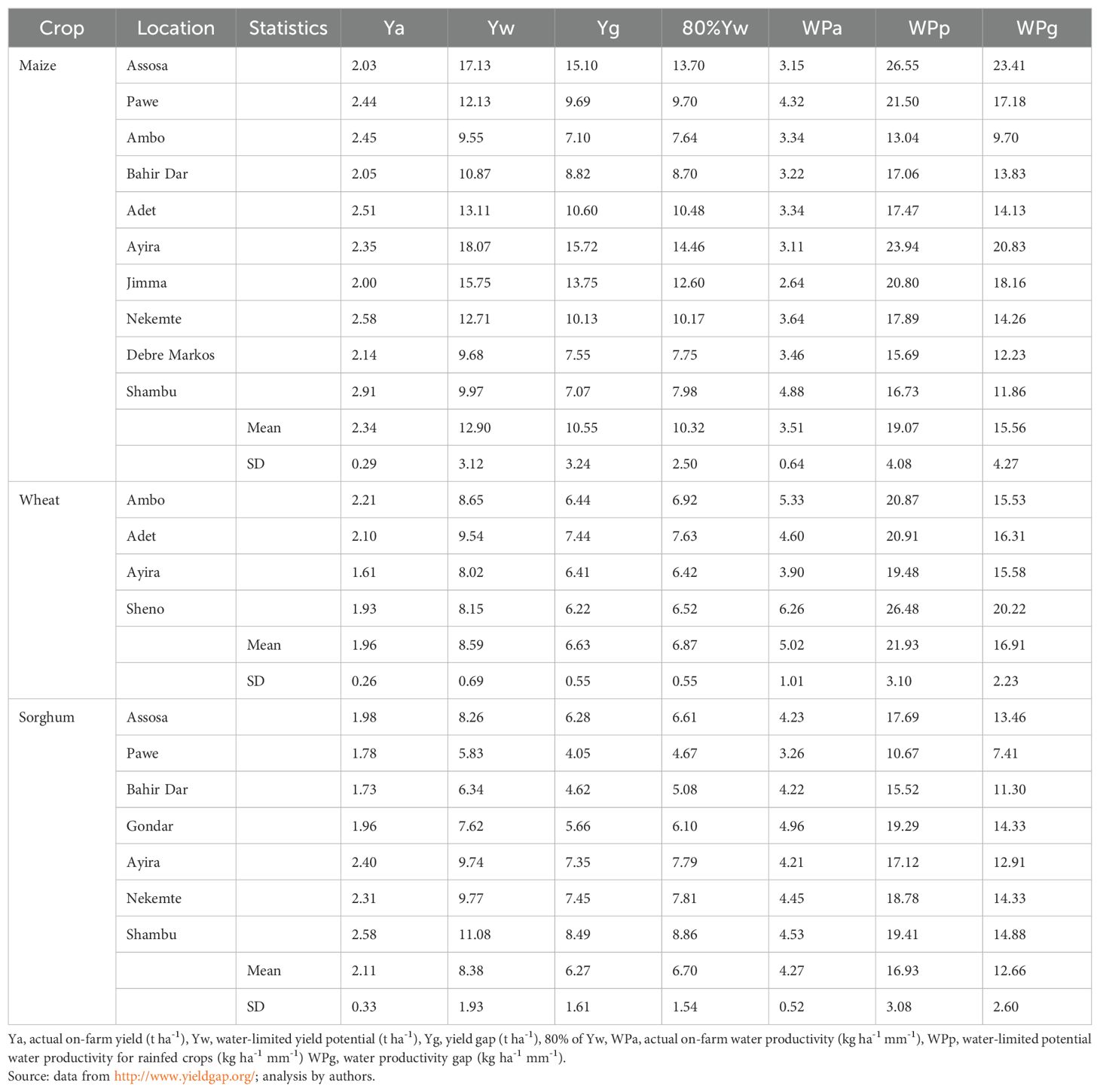
Table 4. Crop Water Productivity (CWP) gap and yield gap for major crops in selected sites in Ethiopia.
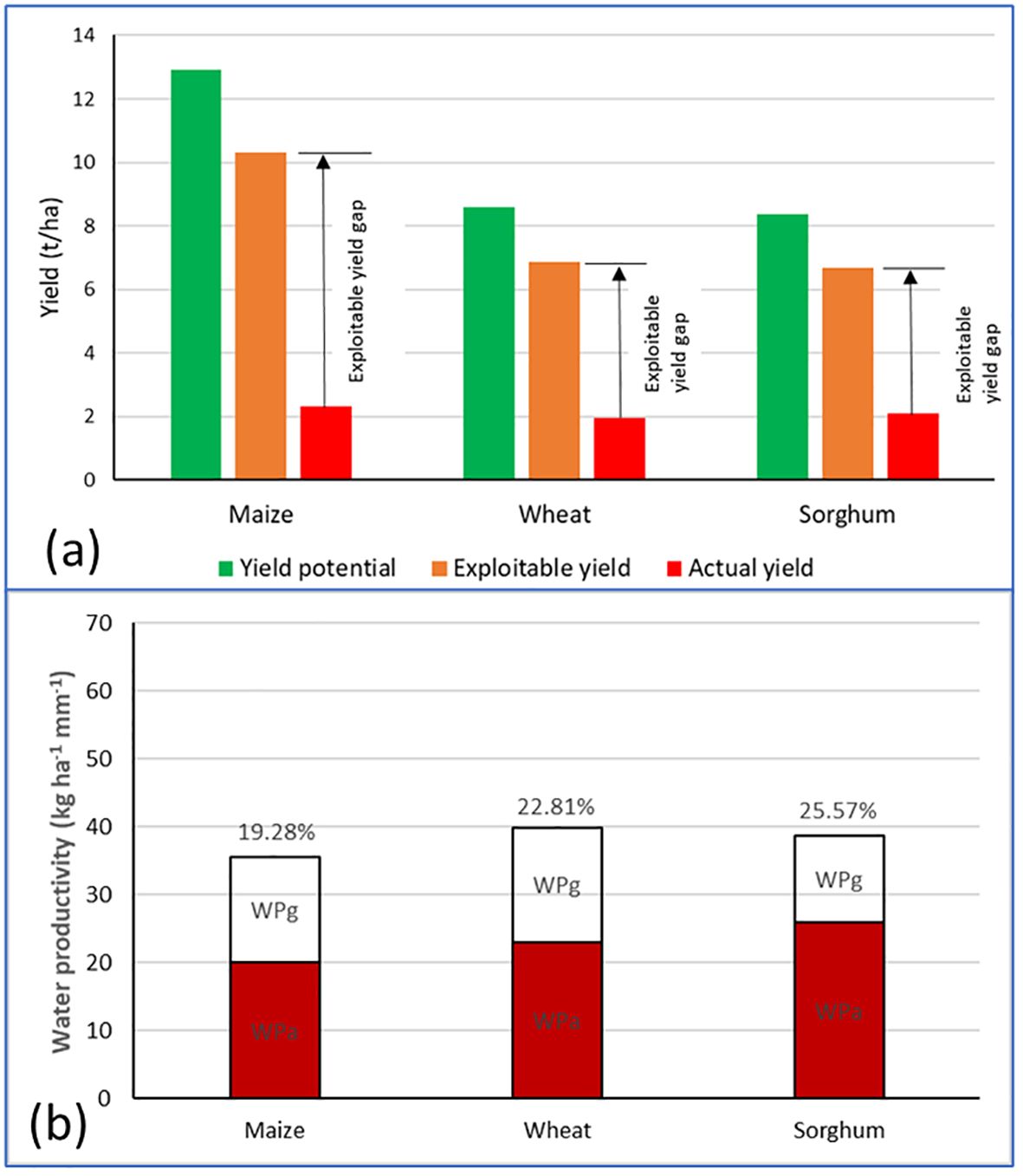
Figure 4. (A) The exploitable yield gap of the three major crops. The difference between the actual yield and 80% of the water-limited yield (Yw) is shown. (B) water productivity gap and actual water productivity. Source: data from http://www.yieldgap.org/; analysis by authors.
As shown in Table 4, the prevailing water productivity of the three major crops (i.e., maize, sorghum, and wheat) is very low, much less than the world average water productivity. The average water productivity of maize, wheat, and sorghum was 3.51 kg/ha/mm (2.64 - 4.88 kg/ha/mm), 5.02 kg/ha/mm (3.9 - 6.26 kg/ha/mm), and 4.27 kg/ha/mm (3.26 - 4.96 kg/ha/mm), respectively. Sadras et al. (2011) compared water productivity values for major crops and found wide ranges, amounting to 6-23 kg/ha per mm for maize, 5-10 kg/ha per mm for wheat, and 5-21 kg/ha per mm for sorghum, indicating considerable potential for improving the water productivity of the major crops in Ethiopia.
The wide range of WP values for the same crop indicates that climatic factors (e.g., evaporative demand of the atmosphere, rainfall pattern) influence water productivity. The highest and lowest WP values for maize were observed at the Shambu Research Station (4.88 kg/ha/mm) and Jimma Research Station (2.64 kg/ha/mm), respectively. Therefore, Shambu’s agro-climate is highly suitable for the production of maize in terms of both grain yield and water productivity. For sorghum, the highest WP was recorded in Gonder (4.96 kg/ha/mm), while the lowest WP was observed in Pawe (3.26 kg/ha/mm). For wheat, the lowest and highest values of WP are observed in Ayira (3.9 kg/ha per mm) and Sheno (6.26 kg/ha per mm), respectively. The highest yields are not necessarily associated with the highest WPs (e.g., wheat and sorghum). However, for maize crops, the Shambu area had the highest average yield of 2.91 t/ha, with the highest water productivity of 4.88 kg/ha per mm.
Figure 5 shows that the WP ranges from ~2 kg/ha per mm at ~ 0.5 t/ha yield to ~5 kg/ha per mm of consumptive green water flow at ~3 t/ha using data collected from 12 Agricultural Research Centers in Ethiopia. This suggests that by increasing crop yields through mechanisms such as soil fertility management, crop selection, and the use of improved tillage, it is possible to increase WP. This concept has also been widely acknowledged by several researchers (e.g., Rockström, 2003). Rockström (2003) revealed the nonlinear relationship between WP and crop yield by considering a yield range of 0.5–10 t/ha from both tropical and temperate regions, where the curve flattens out and the water use efficiency does not increase any further beyond 3 t/ha.
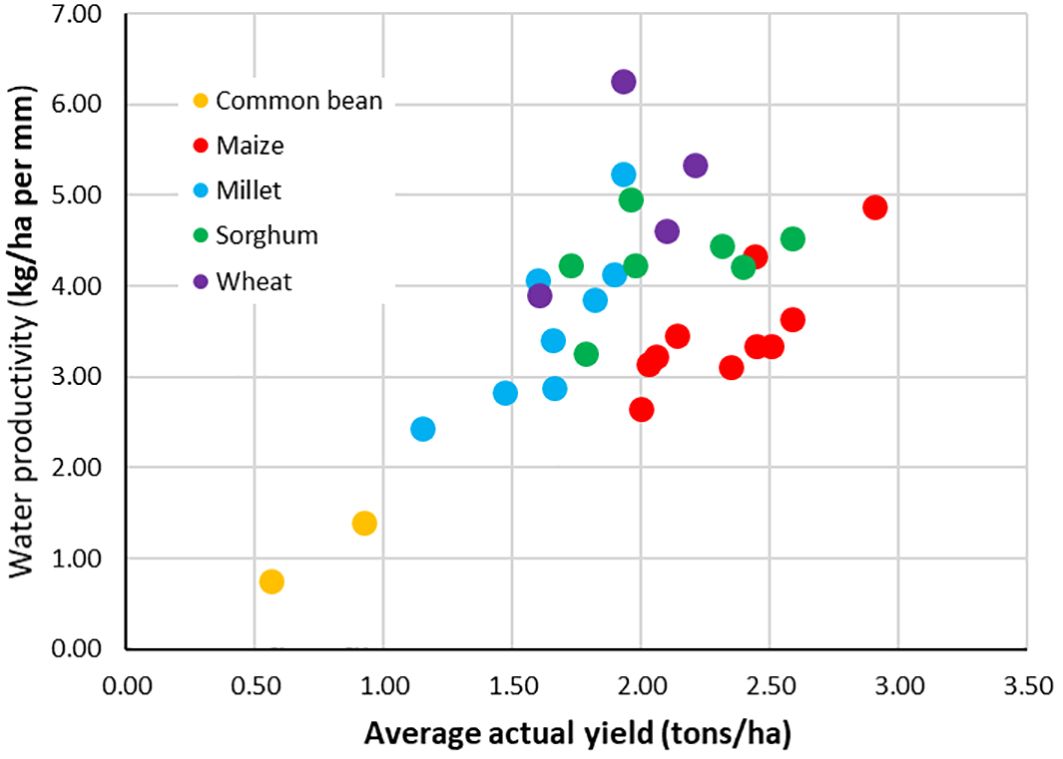
Figure 5. Relationship between actual yield and water productivity. Source: data from http://www.yieldgap.org/; analysis by authors.
3.5.2 Pathway 2: virtual green water transfer
The concept of virtual water trade, where water is essentially exported in virtual form through agricultural commodity trade, has important implications for green water resources (Chapagain et al., 2006; Allan, 2006). Exporting virtual water generates foreign exchange for the exporting country. Indirectly, it also saves water in the importing countries that would otherwise need to use domestic water resources to produce those commodities (Hoekstra and Chapagain, 2008). The virtual water content of crops (m3 of water per ton of crop) depends on the crop water use in the field divided by the yield. Virtual water trade can save water globally under two conditions: (1) if a water-intensive crop is traded from a region with high water productivity to a region with lower productivity and (2) if the virtual water flow saves irrigation water because the exporting country cultivates the crop under rainfed (green water) conditions. Thus, virtual water trade flows related to green water in rainfed agricultural systems present strategic opportunities for generating economic value and saving water globally through more efficient allocation of green water resources across regions.
An analysis of Ethiopia’s water footprint from 1996–2005 revealed that out of the total 77.8 billion m3 of water used annually, a staggering 97% came from green water (soil moisture from rainfall), while only 2% from blue water (surface/groundwater) and 1% from gray water (Table 5). Specifically, for agriculture, approximately 97% of the water footprint was green water, with 75% of the green water footprint going to crop production and 25% to grazing livestock. Crop production alone had a GWF of 56.5 billion m3 per year. In contrast, the blue water footprint was only 2% of the total at 1.85 billion m3 per year, with approximately 64% used for crop irrigation, 34.5% for livestock water supply, and the remaining 1.9% for domestic and industrial uses. This analysis highlights the overwhelming dominance and importance of green water resources, especially soil moisture from rainfall, in meeting Ethiopia’s agricultural and overall water footprint requirements.
Mekonnen and Hoekstra (2014) developed water footprint benchmark values for crop production worldwide. The benchmark water footprint values indicate how efficiently water is used to produce a crop and show the potential for increasing water productivity through better green water (soil moisture) management practices. For Ethiopia’s three major crops—maize, sorghum, and wheat—the analysis revealed that their current total (green+blue) water footprints are very high compared to the respective global 25th percentile benchmarks, indicating low water productivity: maize: 4211 m3/ton actual vs 562 m3/ton benchmark; sorghum: 4968 m3/ton actual vs 1122 m3/ton benchmark; and wheat: similarly high actual vs benchmark (Table 6). This large gap between the actual and benchmark water footprints highlights the significant opportunity in Ethiopia to improve crop water use efficiency and increase yields per unit of water consumed by implementing better green water management techniques. Raising the land and water productivity specifically for these three key crops can contribute greatly to increasing Ethiopia’s food security. Adopting practices that enhance green water utilization and boost crop yields per hectare will lead to more efficient and productive use of the country’s land and water resources. Therefore, research questions such as how various green water management interventions affect the water footprint and what practices are required to reduce the water footprint to at least the 25th per-centile of water footprint values for different crops constitute important research areas (Table 6).
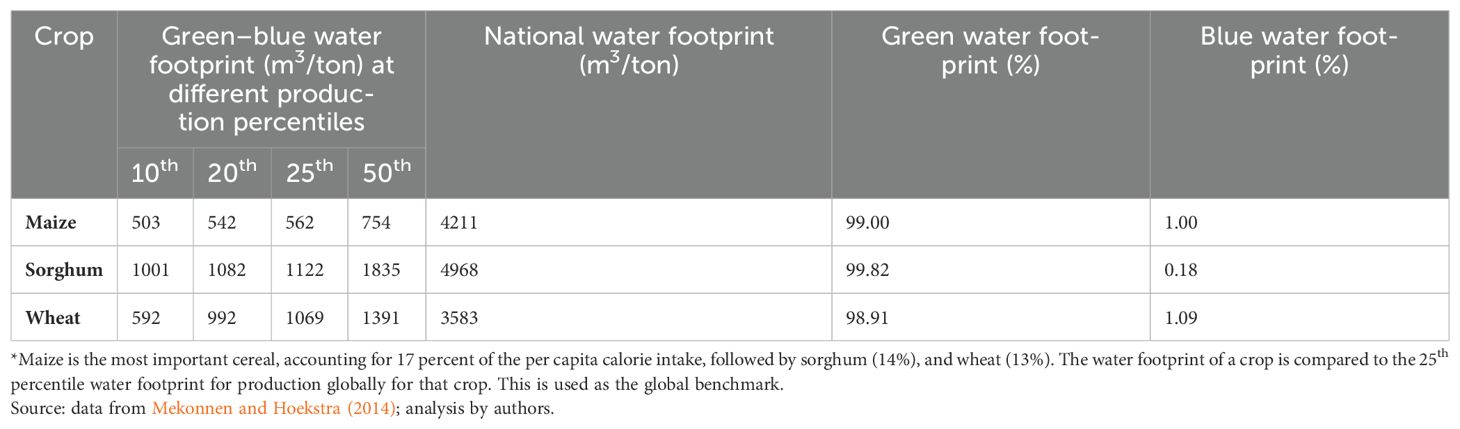
Table 6. Green water footprint for selected crops (three major staples dominating the national food basket in Ethiopia) at different production percentiles.
Ethiopia lags behind other major grain exporters (i.e USA, Canada, France, Australia and Argentina) in terms of green water productivity for key crops, but has an opportunity to change this by optimizing the use of green water resources in its rainfed farming systems. This could unlock export potential while also improving domestic water and food security. However, Ethiopia is a significant importer of wheat from India, USA, Ukraine, Argetina, etc (Figure 6). Most wheat-exporting countries utilize green water efficiently for wheat production. In contrast, Ethiopia, a significant importer of wheat, has not optimized its green water use for wheat production. This inefficiency is reflected in the substantial gap between the actual and benchmark water footprints for wheat in Ethiopia. This gap presents a significant opportunity for the country to enhance crop water use efficiency, increase yields per unit of water consumed, and boost wheat production by adopting better green water management techniques. By improving the low water productivity of wheat, Ethiopia can not only reduce its reliance on imports but also explore the potential of virtual water trade to foster regional economic solidarity and stimulate the growth of its agricultural sector.
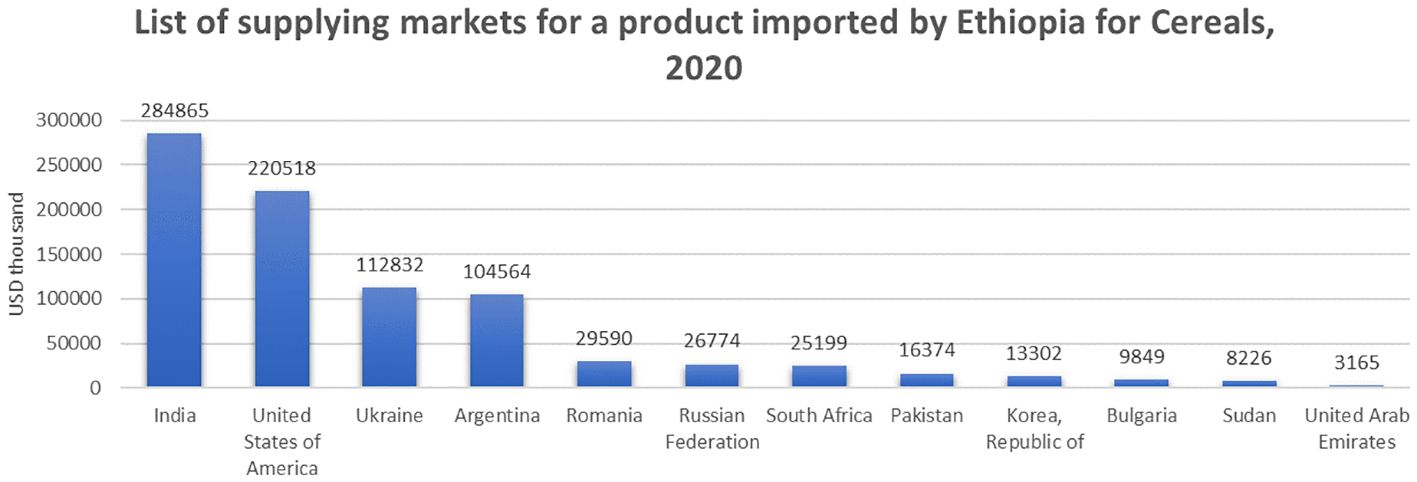
Figure 6. Supplying markets for a product imported by Ethiopia for cereals in 2020. Source: https://www.trademap.org/.
3.5.3 Pathway 3: investing in green water management
While Ethiopian farmers possess extensive knowledge of rainfed agriculture management (since most practices involve rainfed farming), investments in transforming this knowledge into governance, policy, institutional, practical, and technological innovations that support smallholder farmers for managing rainwater and soil moisture in rainfed systems have been very limited. The current practice of not giving proper attention to rainfed agriculture aligns with Ethiopia’s Ten Years Development Plan (Planning and Development Commission, 2020). The development plan aims to shift away from a reliance on rainfed, green water-based agriculture toward increasing irrigation (blue water) infrastructure and expanding large-scale, mechanized farming by smallholders. Interventions usually have a limited focus on rainwater management, which otherwise has a great influence on the sustainability of both surface water and groundwater (Hagos et al., 2011).
There are compelling reasons why investing in green water use (rainfed agriculture) is more important than investing in blue water use (irrigation). First, green water is the main source of water for approximately 80% of the world’s cultivated land and contributes 60-70% of global crop production (Falkenmark and Rockström, 2004; Bruinsma, 2009). In sub-Saharan Africa, food production almost entirely depends on green water (i.e., 95% of the cropland). Irrigated areas, on the other hand, account for 34% of crop production and cover only 24% of all cropland (Siebert and Döll, 2010). Second, in contrast to blue water-based agriculture, which has a high investment cost, green water-based food production is less costly. The high investment costs and failures of many past irrigation projects have made governments and donors cautious about investing more in irrigation projects (Inocencio et al., 2007). Third, unlike green water use, the environmental impacts of blue water use (irrigation) involve water logging, salinization, and soil degradation (Wang, 2004). Soil salinization is a widespread global issue, but it is especially severe in semiarid regions that rely heavily on irrigation and have poor drainage conditions. The combination of using large quantities of irrigation water and inadequate drainage exacerbates the salinization problem in these areas. With increasing concerns over irrigation-induced environmental impacts, upgrading rainfed agriculture is receiving increased attention. Fourth, irrigation alone will not be able to provide the food needed to feed the increasing world population. To properly feed humanity by 2050 compared to that in 2000, an additional 5600 km3/yr of freshwater will be needed, out of which a maximum of 800 km3/yr will come from blue water resources (Falkenmark and Rockström, 2004). This means that a deficit of 4,800 km3/yr will have to be contributed by green water use (e.g., expansion and production increase) in rainfed agriculture. Finally, unlike blue water sources such as rivers and groundwater, which can potentially be reallocated for different uses, green water from soil moisture cannot be easily transferred away from supporting natural vegetation and rainfed crop production in a given area (De Fraiture et al., 2004). Since the use of green water does not significantly compete with that of other water use sectors, green water resources generally have a lower opportunity cost than blue water sources (Hoekstra et al., 2001; Albersen et al., 2003). The limited alternative uses of green water mean that its utilization does not forfeit as many other potential economic opportunities as blue water sources do.
Financing for public infrastructure in the water sector has traditionally prioritized “blue water” development projects, such as extensive irrigation. In contrast, water or agriculture government agencies have not traditionally focused on or had expertise in green water management, which depends on maximizing soil moisture from rainfall. However, studies reveal that enhancing rainfed agriculture carries extraordinarily high potential returns on investment in sub-Saharan Africa’s drylands. The potential gains from improving rainfed farming practices are estimated to be nine times greater than investments in small-scale irrigation projects and a staggering 24 times greater than large-scale irrigation endeavors (Abrams, 2018). In Ethiopia’s extensive rainfed agricultural regions, better management of green water resources offers a significant chance to increase food production and decrease poverty. Implementing green water management strategies such as in-field soil moisture retention and small-scale rainwater harvesting is expected to cost between $250 and $500 per hectare (Abrams, 2018). Despite this promising outlook, green water management in rainfed agriculture has not received adequate attention in terms of water policies, strategies, or existing land rehabilitation programs across the region.
The current practice of not giving proper attention to rainfed agriculture aligns with Ethiopia’s Ten Years Development Plan (Planning and Development Commission, 2020). The plan prioritizes “reducing the reliance on rainfed agriculture by developing irrigation capacity; expanding agricultural mechanization services; and enabling highly productive smallholder farmers to become investors by assisting them to have access to additional land.” This indicates that the development plan aims to shift away from a reliance on rainfed, green water-based agriculture toward increasing irrigation (blue water) infrastructure and expanding large-scale, mechanized farming by smallholders acquiring more land. Little focus has been given to improving the productivity and management of existing rainfed, green water agricultural systems. A paradigm shift is necessary in investment priorities for agricultural water management, one that prioritizes effective integration across the entire water management spectrum, from rainfed to irrigated agriculture. This approach recognizes the critical role of green water in rainfed agriculture and seeks to optimize its productive use. By adopting a holistic perspective that encompasses the full continuum of water management options, investments can be targeted more effectively to improve agricultural productivity and enhance the resilience of smallholder farmers. In Ethiopia, the traditional approach to water resource management has been fragmented, with the Ministry of Water focusing on large-scale irrigation, drinking water supply, and hydropower generation, while the Ministry of Agriculture prioritizes erosion control over water management practices in upper catchment areas. This downstream-oriented approach has led to a narrow focus on blue water, neglecting the complete water balance and the importance of green water flows. However, land use choices have a profound impact on water resources, blurring the lines between irrigated and rainfed agriculture.
3.5.4 Pathway 4: water harvesting and supplemental irrigation for improved rainfed agriculture
Another promising pathway to improving the productivity of rainfed agriculture is to adopt water harvesting and supplemental irrigation techniques, which can significantly boost crop yields and enhance livelihoods. Rainfed areas are characterized by relatively high levels of land degradation and low soil moisture holding capacity. Climate change has exacerbated the existing state of vulnerability and elevated the risks to agricultural production systems. While rainfed lands possess substantial untapped potential for growth, the risks of crop failure, low yields, and insecure livelihoods are high due to rainfall variability. Rainfed agriculture is mainly and negatively impacted by intermittent dry spells during the cropping season, especially at critical crop growth stages. Addressing these challenges in rainfed agricultural areas through supplemental irrigation to overcome dry spells is a crucial strategy for upgrading rainfed agriculture (Wani et al., 2003; Oweis and Hachum, 2009). This approach involves collecting small amounts of surface runoff (blue water) during the rainy season and using this stored water for supplementary irrigation alongside green water to meet crop water requirements (Pathak et al., 2009). Bridging dry spells with blue water enables farmers to invest in improved seeds and fertilizers, utilize labor more efficiently, and conserve resources. Under these enhanced conditions, the economic returns from using external fertilizers and supplemental blue water are high. Combining GWM with the judicious use of collected blue water can significantly boost rainfed agricultural productivity.
4 Conclusion and policy recommendation
Rainfed agriculture in Ethiopia faces significant yield and water productivity gap for major crops such as wheat, sorghum, and maize. However, there is significant potential for improvement through adopting enhanced crop management, soil management, and water management practices. These improvements provide “windows of opportunity” to improve food and water security. Ethiopian rainfed agriculture has much potential for improvement, but there have not been enough investments made to close yield gaps and boost water productivity. Prioritizing techniques such as infield soil moisture retention and small-scale rainwater harvesting increase soil water availability and crop water up-take. The majority of projects have concentrated on reducing soil erosion and expanding large-scale irrigation, with insufficient attention given to rainwater management and green water resources in water policy and land rehabilitation initiatives.
Policies and programs related to water and agriculture need to change from their current narrow focus on controlling soil erosion to an integrated green−blue water management approach. At the government level, more funding and knowledge of green water management are essential. Ethiopia is not as productive with green water for important crops as major grain exporters are. By strategically using virtual water trade, rainfed farming can maximize its use of green water resources, improving domestic food and water security and opening up export opportunities. More data, analyses, and research are required to fully understand how green water management can improve Ethiopia’s food and water security.
Overall, this review highlights that unlocking the potential of green water resources through targeted investments and policy support for rainfed agriculture can significantly contribute to Ethiopia’s water and food security objectives in a cost-effective and environmentally sustainable manner. We need to develop a plan for the complementary use of blue water and green water to maximize synergies and prevent trade-offs between food security and water security.
Data availability statement
The original contributions presented in the study are included in the article/supplementary material. Further inquiries can be directed to the corresponding authors.
Author contributions
ET: Conceptualization, Data curation, Formal analysis, Funding acquisition, Investigation, Methodology, Project administration, Resources, Software, Supervision, Validation, Visualization, Writing – original draft, Writing – review & editing. TK: Conceptualization, Methodology, Writing – review & editing. WB: Conceptualization, Investigation, Methodology, Writing – review & editing. GZ: Conceptualization, Project administration, Writing – review & editing. GO’D: Conceptualization, Formal analysis, Investigation, Methodology, Supervision, Writing – review & editing. CW: Conceptualization, Funding acquisition, Project administration, Resources, Supervision, Writing – review & editing. GA: Conceptualization, Project administration, Resources, Supervision, Writing – review & editing.
Funding
The author(s) declare financial support was received for the research, authorship, and/or publication of this article. This work was supported by the Water Security and Sustainable Development Hub funded by the UK Research and Innovation’s Global Challenges Research Fund (GCRF) (grant number: ES/S008179/1).
Conflict of interest
The authors declare that the research was conducted in the absence of any commercial or financial relationships that could be construed as a potential conflict of interest.
Publisher’s note
All claims expressed in this article are solely those of the authors and do not necessarily represent those of their affiliated organizations, or those of the publisher, the editors and the reviewers. Any product that may be evaluated in this article, or claim that may be made by its manufacturer, is not guaranteed or endorsed by the publisher.
References
Albersen P. J., Houba H. E. D., Keyzer M. A. (2003). Pricing a raindrop in a process-based model: General methodology and a case study of the Upper-Zambezi. Phys. Chem. Earth 28, 183–192. doi: 10.1016/S1474-7065(03)00024-X
Allan J. A. (1993). Fortunately, there are Substitutes for Water Otherwise our Hydro-political Futures Would be Impossible. Priorities for Water Resources Allocation and Management. Priorities Water Resour. Alloc. Manage. 13, 13–26.
Allan J. A. (2006). Virtual water - Part of an invisible synergy that ameliorates water scarcity. Water Cris. Myth Real. Marcelino Botin Water Forum 2004, 131–150. doi: 10.1201/9781439834275.pt3
Asmamaw D. K. (2017). A critical review of the water balance and agronomic effects of conservation tillage under rain-fed agriculture in Ethiopia. L. Degrad. Dev. 28, 843–855. doi: 10.1002/ldr.2587
Assefa B. T., Chamberlin J., Reidsma P., Silva J. V., van Ittersum M. K. (2020). Unravelling the variability and causes of smallholder maize yield gaps in Ethiopia. Food Secur. 12, 83–103. doi: 10.1007/s12571-019-00981-4
Barron J., Rockström J., Gichuki F., Hatibu N. (2003). Dry spell analysis and maize yields for two semi-arid locations in east Africa. Agric. For. Meteorol. 117, 23–37. doi: 10.1016/S0168-1923(03)00037-6
Bruinsma J. (2009). The Resource Outlook to 2050. By How Much do Land, Water and Crop Yields Need to Increase by 2050? FAO Expert Meeting on How to Feed the World in 2050. FAO, Rome.
Burek P., Satoh Y., Fischer G., Kahil M. T., Scherzer A., Tramberend S., et al. (2016). Water Futures and Solution– Fast Track Initiative (Final Report). IIASA, Laxenburg, Austria, IIASA Working Paper.
Central Statistics Agency of Ethiopia (CSA). (2018). Report on Area and Production of Crops (Private Peasant Holdings, Meher Season) (Addis Ababa, Ethiopia: Government of Ethiopia).
Chapagain A. K., Hoekstra A. Y., Savenije H. H. G., Gautam R. (2006). The water footprint of cotton consumption: An assessment of the impact of worldwide consumption of cotton products on the water resources in the cotton producing countries. Ecol. Econ. 60, 186–203. doi: 10.1016/j.ecolecon.2005.11.027
Cosgrove W. J., Rijsberman F. R. (2000). Making Water Everybody’s Business. World Water Vision (London: The World Water Council. Earthscan Ltd.).
De Fraiture C., Cai X., Amarasinghe U., Rosegrant M., Molden D. (2004). Does International Cereal Trade Save Water? The Impact of Virtual Water Trade on Global Water Use, Comprehensive Assessment Research Report 4. (IWMI, Colombo, Sri Lanka: Comprehensive Assessment Secretariat).
Dile Y. T., Tekleab S., Kaba E. A., Gebrehiwot S. G., Worqlul A. W., Bayabil H. K., et al. (2018). Advances in water resources research in the Upper Blue Nile basin and the way forward: A review. J. Hydrol. 560, 407–423. doi: 10.1016/j.jhydrol.2018.03.042
Diriba G., Man C. (2019). Building a Big Tent for Agricultural Transformation in Ethiopia (Washington DC: Center for Strategic and International Studies).
Ercin A. E., Hoekstra A. Y. (2014). Water footprint scenarios for 2050: A global analysis. Environ. Int. 64, 71–82. doi: 10.1016/j.envint.2013.11.019
Falkenmark M., Rockström J. (2004). Balancing water for humans and nature: The new approach in ecohydrology (London: Earthscan). doi: 10.4324/9781849770521
Falkenmark M., Rockström J. (2006). The new blue and green water paradigm: breaking new ground for water resources planning and management. J. Water Resour. Plan. Manage. 132, 129–132. doi: 10.1061/(asce)0733-9496(2006)132:3(129
FAO. (2020). Ten years of the Ethiopian Agricultural Transformation Agency. An FAO evaluation of the Agency’s impact on agricultural growth and poverty reduction (Rome, Italy: FAO). doi: 10.4060/cb2422en
FAO. (2023). The State of Food and Agriculture 2023 – Revealing the true cost of food to transform agrifood systems (Rome: FAO). doi: 10.4060/cc7724en
FDRE. (2013). Ethiopia’s Climate Resilient Green Economy climate resilience strategy agriculture and forestry. Ministry of Agriculture, Addis Ababa.
Garbero A., Brailovskaya V., Giera. B. (2016). Reconstructing the impact of IFAD-supported projects on poverty reduction: Results from 14 IFAD-supported projects using observational data. Working paper (Rome: IFAD).
Gerten D., Heinke J., Hoff H., Biemans H., Fader M., Waha K. (2011). Global water availability and requirements for future food production. J. Hydrometeorol. 12, 885–899. doi: 10.1175/2011JHM1328.1
Giustini D., Boulos M. N. K. (2013). Google Scholar is not enough to be used alone for systematic reviews. Online J. Public Health Inform. 5, 214. doi: 10.5210/ojphi.v5i2.4623
Hagos F., Makombe G., Namara R., Awulachew S. (2011). Importance of irrigated agriculture to the Ethiopian economy: Capturing the direct net benefits of irrigation. Colombo: IWMI Research Report 128. doi: 10.4314/ejdr.v32i1.68597
Hoekstra A. Y. (2019). Green-blue water accounting in a soil water balance. Adv. Water Resour. 129, 112–117. doi: 10.1016/j.advwatres.2019.05.012
Hoekstra A., Hung P. (2002). Virtual Water trade: a quantification of virtual water flows between nations in relation to crop trade. Value of Water Research Report Series. (Delft, The Netherlands: Institute for Water Education), 11.
Hoekstra A. Y., Chapagain A. K. (2008). Globalization of Water: Sharing the Planet’s Freshwater Resources, Globalization of Water: Sharing the Planet’s Freshwater Resources. Oxford, UK: Blackwell Publishing. Interface Focus, 13 (20230012), 9. doi: 10.1002/9780470696224
Hoekstra A. Y., Mekonnen M. M. (2012). The water footprint of humanity. Proc. Natl. Acad. Sci. 109, 3232–3237. doi: 10.1073/pnas.1109936109
Hoekstra A. Y., Savenije H. H. G., Chapagain A. K. (2001). An integrated approach towards assessing the value of water: A case study on the zambezi basin. Integr. Assess. 2, 199–208. doi: 10.1023/A:1013368524528
Hoff H., Falkenmark M., Gerten D., Gordon L., Karlberg L., Rockström J. (2010). Greening the global water system. J. Hydrol. 384, 177–186. doi: 10.1016/j.jhydrol.2009.06.026
Hurni H., Tato K., Zeleke G. (2005). The implications of changes in population, land use, and land management for surface runoff in the Upper Nile Basin Area of Ethiopia. Mt. Res. Dev. 25, 147–154. doi: 10.1659/0276-4741(2005)025[0147:TIOCIP]2.0.CO;2
Ibrahim M. (2004). “Extension experiences in Ethiopia,” in Improving Productivity and Market Success (IPMS) of Ethiopian Farmers project launching conference, 30th June 2003, ILRI (International Livestock Research Institute), Addis Ababa, Ethiopia.
Inocencio A. B. (2007). Costs and Performance of Irrigation Projects: A Comparison of Sub-Saharan Africa and Other Developing Regions (Anand, India: IWMI), 109.
IPCC. (2007). Climate change 2007: impacts, adaptation and vulnerability. Work. Gr.II, IPCC 4th Assess. Report. Available online at: https://www.ipcc.ch/site/assets/uploads/2018/03/ar4_wg2_full_report.pdf (Accessed January 12, 2023).
IWMI. (2000). “World water supply and demand in 2025,” in World Water Scenario Analyses. Ed. Rijsberman F. R. (World Water Council, Marseille, France).
Jebelli J. (2021). Implementing a capacity development initiative to build resilience to better adapt to climate change: A case study in Ethiopia, Africa. Int. J. Environ. Agric. Res. 7, 28–38.
Kassawmar T., Zeleke G., Bantider A., Gessesse G. D., Abraha L. (2018). A synoptic land change assessment of Ethiopia's Rainfed Agricultural Area for evidence-based agricultural ecosystem management. Heliyon 4. doi: 10.1016/j.heliyon.2018.e00914
Keys P. W., Falkenmark M. (2018). Green water and African sustainability. Food Secur. 10, 537–548. doi: 10.1007/s12571-018-0790-7
Liniger H. P., Studer R. M., Hauert C., Gurtner M. (2011). Sustainable land management in practice – guidelines and best practices for Sub-Saharan Africa (TerrAfrica, World Overview of Conservation Approaches and Technologies (WOCAT) and Food and Agriculture Organization of the United Nations (FAO), Rome, Italy.
Lobell D. B., Cassman K. G., Field C. B. (2009). Crop yield gaps: Their importance, magnitudes, and causes. Annu. Rev. Environ. Resour. 34, 179–204. doi: 10.1146/annurev.environ.041008.093740
Louhichi K., Temursho U., Colen L., Gomez Y Paloma S. (2019). Upscaling the productivity performance of the Agricultural Commercialization Cluster Initiative in Ethiopia (Luxembourg: Publications Office of the European Union), ISBN: 978-92-76-12941-7. doi: 10.2760/57450,JRC117562
Mann M. L., Warner J. M. (2017). Ethiopian wheat yield and yield gap estimation: A spatially explicit small area integrated data approach. F. Crop Res. 201, 60–74. doi: 10.1016/j.fcr.2016.10.014
Mekonnen M. M., Hoekstra A. Y. (2011). National water footprint accounts: the green, blue and grey water footprint of production and consumption. Value Water Res. Rep. Ser. No. 50. Hydrol. Earth Syst. Sci. Discuss 8, 763–809. doi: 10.5194/hessd-8-763-2011
Mekonnen M. M., Hoekstra A. Y. (2014). Water footprint benchmarks for crop production: A first global assessment. Ecol. Indic. 46, 214–223. doi: 10.1016/j.ecolind.2014.06.013
Molden D., Oweis T., Steduto P., Bindraban P., Hanjra M. A., Kijne J. (2010). Improving agricultural water productivity: Between optimism and caution. Agric. Water Manage. 97, 528–535. doi: 10.1016/j.agwat.2009.03.023
Oweis T., Hachum A. (2009). “Supplemental irrigation for improved rainfed agriculture in WANA region,” in Rainfed agriculture: unlocking the potential (CABI, Wallingford UK), 182–196.
Pathak P., Sahrawat K. L., Wani S. P., Sachan R. C., Sudi R. (2009). “Opportunities for water harvesting and supplemental irrigation for improving rainfed agriculture in semi-arid areas,” in Rainfed agriculture: unlocking the potential (CABI, Wallingford UK), 197–221.
Petticrew M., Roberts H. (2008). Systematic reviews in the social sciences: A practical guide (Malden; Oxford: Blackwell Pub).
Planning and Development Commission (2020). Ten Years Development Plan: A Pathway to Prosperity 2021-2030 (Addis Ababa, Ethiopia: FDRE Planning and Development Commission).
Rockström J. (1997). On-farm agrohydrological analysis of the Sahelian yield crisis: rainfall partitioning, soil nutrients and water use efficiency of pearl millet. Doctoral dissertation. Stockholm University, Stockholm, 62 pp.
Rockström J. (2003). Water for food and nature in drought-prone tropics: Vapour shift in rain-fed agriculture. Philos. Trans. R. Soc B Biol. Sci. 358, 1997–2009. doi: 10.1098/rstb.2003.1400
Rockström J., Falkenmark M. (2000). Semiarid crop production from a hydrological perspective: Gap between potential and actual yields. CRC. Crit. Rev. Plant Sci. 19, 319–346. doi: 10.1080/07352680091139259
Rockström J., Falkenmark M., Karlberg L., Hoff H., Rost S., Gerten D. (2009). Future water availability for global food production: The potential of green water for increasing resilience to global change. Water Resour. Res. 45. doi: 10.1029/2007WR006767
Rockström J., Lannerstad M., Falkenmark M. (2007). Assessing the water challenge of a new green revolution in developing countries. Proc. Natl. Acad. Sci. 104, 6253–6260. doi: 10.1073/pnas.0605739104
Rost S., Gerten D., Bondeau A., Lucht W., Rohwer J., Schaphoff S. (2008). Agricultural green and blue water consumption and its influence on the global water system. Water Resour. Res. 44. doi: 10.1029/2007WR006331
Sadras V. O., Grassini P., Steduto P. (2011). “Status of water use efficiency of main crops,” in State of World’s Land and Water Resources for Food and Agriculture (SOLAW) Background Thematic Rep, 41. FAO, Rome, Italy.
Savenije H. H. G. (1999). The role of green water in food production in Sub-Saharan Africa (Rome: FAO).
Savenije H. H. G. (2000). Water scarcity indicators; the deception of the numbers. Phys. Chem. Earth Part B Hydrol. Ocean. Atmos. 25, 199–204. doi: 10.1016/S1464-1909(00)00004-6
Schneider C. (2013). Three shades of water: increasing water security with blue, green, and gray water. CSA News 58, 4–9. doi: 10.2134/csa2013-58-10-1
Siebert S., Döll P. (2010). Quantifying blue and green virtual water contents in global crop production as well as potential production losses without irrigation. J. Hydrol. 384, 198–217. doi: 10.1016/j.jhydrol.2009.07.031
Sonneveld B. G. J. S. (2002). Land Under Pressure: The Impact of Water Erosion on Food Production in Ethiopia. Amsterdam University. Shaker Publishing (PhD dissertation), The Netherlands.
Sonneveld B. G. J. S., Keyzer M. A. (2003). Land under pressure: Soil conservation concerns and opportunities for Ethiopia. L. Degrad. Dev. 14, 5–23. doi: 10.1002/ldr.503
Sova C., Flowers K., Man C. (2019). Climate Change and Food Security. Center for Strategic and Studies (CSIS) (Washington, D.C.: Center for Strategic and International Studies).
Teferi E., Bantider A., Zeleke G., Bewket W. (2023). Land Degradation in Ethiopia: An Assessment Using a Composite Land Degradation Index Method (Addis Ababa: Water and Land Resource Centre, Addis Ababa University).
Temesgen M., Hoogmoed W. B., Rockström J., Savenije H. H. G. (2009). Conservation tillage implements and systems for smallholder farmers in semi-arid Ethiopia. Soil Tillage Res. 104, 185–191. doi: 10.1016/j.still.2008.10.026
Tessema W. (2000). “Stakeholder participation in policy processes in Ethiopia,” in Managing Africa's soils No. 17 (Edinburgh, United Kingdom: IIED-Publications).
Van Dijk M., Morley T., Jongeneel R., van Ittersum M., Reidsma P., Ruben R. (2017). Disentangling agronomic and economic yield gaps: An integrated framework and application. Agric. Syst. 154, 90–99. doi: 10.1016/j.agsy.2017.03.004
Van Dijk M., Morley T., van Loon M., Reidsma P., Tesfaye K., van Ittersum M. K. (2020). Reducing the maize yield gap in Ethiopia: Decomposition and policy simulation. Agric. Syst. 183, 102828. doi: 10.1016/j.agsy.2020.102828
Vanham D., Hoekstra A. Y., Bidoglio G. (2013). Potential water saving through changes in European diets. Environ. Int. 61, 45–56. doi: 10.1016/j.envint.2013.09.011
van Ittersum M. K., Rabbinge R. (1997). Concepts in production ecology for analysis and quantification of agricultural input-output combinations. F. Crop Res. 52, 197–208. doi: 10.1016/S0378-4290(97)00037-3
Wang Y. (2004). Environmental degradation and environmental threats in China. Environ. Monit. Assess. 90, 161–169. doi: 10.1023/B:EMAS.0000003576.36834.c9
Wani S. P., Pathak P., Sreedevi T. K., Singh H. P., Singh P. (2003). Efficient management of rainwater for increased crop productivity and groundwater recharge in Asia. In Water productivity in agriculture: Limits and opportunities for improvement. (Wallingford UK: CABI Publishing), 199–215.
World Bank. (1979). Appraisal of Minimum Package Project - Ethiopia - March 2, 1973 – Eastern Africa Regional Office - Agriculture Projects Division - Report Number 84ET (Washington, D.C., United States: World Bank Group Archives).
World Bank. (1988). Ethiopia - Peasant Agricultural Development Project (English) (Washington, DC: The World Bank).
World Bank. (2017). Ethiopia - Agricultural Growth Program Project. Implementation Completion and Results Report (Washington, DC: The World Bank).
World Bank. (2023). World Development Indicators. Available online at: https://databank.worldbank.org/source/world-development-indicators (Accessed February 22, 2023).
WWAP. (2016). The United Nations World Water Development Report 2016: Water and Jobs (Paris, France: The United Nations World Water Development Report).
Keywords: rainfed, agriculture, green water, yield gap, water productivity
Citation: Teferi E, Kassawmar T, Bewket W, Zeleke G, Ayele GT, O’Donnell G and Walsh C (2025) Rainfed agriculture in Ethiopia: a systematic review of green water management pathways to improve water and food security. Front. Agron. 7:1418024. doi: 10.3389/fagro.2025.1418024
Received: 09 September 2024; Accepted: 02 January 2025;
Published: 27 January 2025.
Edited by:
Biswanath Dari, North Carolina Agricultural and Technical State University, United StatesReviewed by:
Katharina Löhr, Leibniz Centre for Agricultural Landscape Research (ZALF) e.V., GermanyRafael Felippe Ratke, Federal University of Mato Grosso do Sul, Brazil
Copyright © 2025 Teferi, Kassawmar, Bewket, Zeleke, Ayele, O’Donnell and Walsh. This is an open-access article distributed under the terms of the Creative Commons Attribution License (CC BY). The use, distribution or reproduction in other forums is permitted, provided the original author(s) and the copyright owner(s) are credited and that the original publication in this journal is cited, in accordance with accepted academic practice. No use, distribution or reproduction is permitted which does not comply with these terms.
*Correspondence: Ermias Teferi, ZXJtaWFzLnRlZmVyaUBhYXUuZWR1LmV0; Greg O’Donnell, Zy5tLm8mI3gyMDE5O2Rvbm5lbGxAbmV3Y2FzdGxlLmFjLnVr