- 1Department of Crop, Soil and Environmental Sciences, Auburn University, Auburn, AL, United States
- 2National Soil Dynamics Laboratory, Agricultural Research Service, U.S. Department of Agriculture, Auburn, AL, United States
- 3Department of Entomology and Plant Pathology, Auburn University, Auburn, AL, United States
Cover crops are increasingly adopted to suppress weed growth and reduce reliance on chemical herbicides. A greenhouse experiment was conducted to evaluate the emergence and growth response of troublesome southeastern weeds to various cereal rye (Secale cereale L.) residue levels. Trays planted with Palmer amaranth (Amaranthus palmeri S. Watson), sicklepod (Senna obtusifolia L.), ivyleaf morningglory (Ipomoea hederacea), and large crabgrass (Digitaria sanguinalis L.) seeds mixed with soil were covered uniformly by four different levels of cereal rye biomass. The following field experiment was conducted at two locations in Alabama in a split-plot design, with the main plot factor being four seeding rates of cereal rye to obtain various cereal rye biomass. Subplot factors were preemergence herbicide flumioxazin and non-treated (NT) check. The greenhouse results demonstrated reduced seed emergence and lower weed biomass for Palmer amaranth, sicklepod, and large crabgrass in plots with higher cereal rye residue biomass compared to those with lower biomass. In both greenhouse and field conditions, the emergence of ivyleaf morningglory was not affected by the increasing biomass of cereal rye residue. Palmer amaranth seed emergence was the most sensitive to increasing biomass residue due to its small seed size. Cereal rye biomass and Palmer amaranth counts were strongly negatively correlated with a Pearson’s coefficient (r) of 0.83 while weakly negatively correlated for ivyleaf morningglory with 0.49. In conclusion, increasing the biomass of cereal rye residue is effective in suppressing Palmer amaranth seed emergence but not ivyleaf morningglory. The flumioxazin treatment demonstrated 95%–90% control for Palmer amaranth and ivyleaf morningglory, while the NT check exhibited 50% control of Palmer amaranth and 30% control of ivyleaf morningglory by cereal rye biomass alone. In conclusion, a sufficient amount of cereal rye biomass can effectively suppress the emergence and growth of weeds, particularly Palmer amaranth.
1 Introduction
Palmer amaranth (Amaranthus palmeri S. Watson), morningglory (Ipomoea spp.), sicklepod (Senna obtusifolia L.), and large crabgrass (Digitaria sanguinalis L.) are among the most troublesome weeds threatening cropping systems in the Southern USA (Webster and Nichols, 2012). Furthermore, the development of herbicide-resistant weeds is one of the major challenges due to the continuous use of similar types of chemical herbicides in the field. Considering the challenges of herbicide-resistant weed species, specifically glyphosate-resistant Palmer amaranth, and consistent public pressure to reduce herbicide utilization, there is an urgent need for integrated weed management (IWM) strategies (Norsworthy et al., 2012; Price et al., 2011). Herbicide-resistance management programs should incorporate all existing cultural, mechanical, and chemical methods for efficient and sustainable weed control. According to Norsworthy et al. (2012), implementing the best management practices is necessary, with principal attention on understanding the biology of the weeds. Furthermore, Zeidali et al. (2021) suggested that it is essential to understand the influence of management practices on seed emergence to establish IWM practices.
Conservation tillage, which involves high-residue cover crops, is a well-established strategy in IWM (Norsworthy et al., 2011; Price et al., 2016). Cover crop residue offers physical suppression of weed emergence and establishment by blocking light, competing for nutrient resources (Teasdale and Mohler, 2000), and releasing allelochemicals to retard weed growth (Sturm et al., 2018). Previous research has found that high-residue cover crops in no-till or strip-till cropping systems were effective in facilitating weed suppression through the mulching effect (Kumari et al., 2023a, 2023b; Price and Norsworthy, 2013; Price et al., 2021), specifically cereal rye (Secale cereale L.) (Kumari et al., 2024a, 2024b). Additionally, cover crops provide soil health benefits such as increasing soil organic matter, conserving soil moisture, and preventing soil erosion losses in the Southeastern USA (Farmaha et al., 2022). Cereal rye is the most widely adopted cover crop in the Southeastern USA and dominates as a winter cover crop species in the subtropical and temperate regions across the country (CTIC, 2017). Furthermore, many growers prefer a cereal rye cover crop, as it produces high biomass residue due to its vigorous vegetative growth and winter hardiness. Moreover, a high C:N ratio and slower decomposition rate of cereal rye residue after termination (SARE, 2007) provide season-long weed control. However, the weed-suppressing ability of cover crops depends on various factors such as weed type, amount of cover crop biomass, allelopathic effect, and shading effect (Teasdale, 1996). According to Schomberg et al. (2006), the cover crop biomass production depends on region, weather conditions, and applied management practices. Hence, evaluation of cover crop performance across diverse environmental conditions is needed.
Additionally, Palmer amaranth is becoming resistant to nearly all available postemergence herbicide options. The integration of soil residual herbicide with cover crops to manage glyphosate-resistant Palmer amaranth is an increasing trend for growers. Field research found that combining high-residue cover crops such as cereal rye with preemergence herbicide to control Palmer amaranth and other troublesome weeds could be an effective strategy for weed management (Kumari et al., 2024a, 2024b). Therefore, we conducted this study with the objective of evaluating the influence of different biomass of cereal rye residue and soil-applied herbicide on the emergence and growth of ivyleaf morningglory and Palmer amaranth. In the greenhouse study, we determined the influence of different biomass residues of cereal rye on the emergence and growth of troublesome southeastern weeds including Palmer amaranth, ivyleaf morningglory, sicklepod, and large crabgrass.
2 Materials and methods
2.1 Greenhouse experiment
A greenhouse experiment was conducted in the Plant Sciences Center at Auburn University, Auburn, AL. The experiment was conducted two times from June to August 2021. Five replications of each treatment were placed in a randomized block design in each run. The dimensions of soil flats were 55.88 × 29.21 × 3.81 cm. Weed seeds were planted at 70.69, 12.25, 12.25, and 64.32 million seeds ha−1 for Palmer amaranth, ivyleaf morningglory, sicklepod, and large crabgrass, respectively. Weed seeds were thoroughly mixed with organic soil and placed over the top of the soil (sandy loam)-filled flats. Cereal rye biomass was harvested by hand from a field in May 2021, oven-dried for 3 days at 60°C, and then trimmed to match the length of the soil flat. Then, soil flats were covered uniformly by four different biomass levels of rye straw (2,800 kg ha−1 as low, 5,600 kg ha−1 as medium, 8,400 kg ha−1 as high, and 11,200 kg ha−1 as highest) and a check with no residue added.
2.2 Field experiment
A field experiment was conducted at E.V. Smith (EVS) Auburn University Research and Extension Center (Field Crops Unit; 32.4417°N, 85.8974°W) near Shorter, Alabama, and Wiregrass Research and Experimental Station (WREC) Headland, AL (31°30′ N, 85°17′ W) from late October 2022 through August 2023. The soil characteristics at the EVS site were Compass sandy loam (coarse-loamy, siliceous, subactive, thermic Paleudults) with pH 6.2 and 0.8% organic matter. At the WREC site, the soil was a Dothan fine sandy loam (fine-loamy, siliceous, thermic Plinthic Paleudult) with pH 6.0 and 1.1% organic matter. The soil sampling depth of pH and organic matter was up to 10 cm.
The experiment was conducted in a split-plot design with six replications; the main plots were four seeding rates (45, 90, 135, and 180 kg ha−1) of cereal rye and a winter fallow check. Plot size measured 20 by 12 feet. In the subplot, flumioxazin herbicide and a non-treated (NT) check were considered. The cereal rye ‘Wrens Abruzzi’ was planted utilizing JD 7730 and a Great PlainsR no-till drill (Great Plains Salina, KS 67401) with GreenStar GPS in the third week of November 2022 at both locations. The cover crop was mechanically rolled via a three-section bar roller-crimper to level the biomass residue on the soil surface in the third week of April 2023. After the mechanical rolling of cover crops, burndown was performed with an application of glyphosate (Roundup Powermax®; Monsanto Company, St. Louis, MO, USA) 0.91 kg ae ha−1 and glufosinate (Liberty; Bayer Crop Science, Research Triangle Park, NC, USA) 0.57 kg ai ha−1. Flumioxazin (Valor®, Valent U.S.A. Corporation, Walnut Creek, CA, USA) at 71.5 g ai ha−1 was applied approximately 3–4 weeks after cover crop termination. The herbicide was applied using a CO2-pressurized backpack sprayer equipped with TTI 11004 nozzles (TeeJet® Technologies, Glendale Heights, IL, USA) at 276-kPa calibration to deliver 280 L ha−1. At the EVS location, precipitation was received during the week of herbicide application (Figure 1); however, at the WREC location, approximately 12 mm of irrigation was provided within 24 hours of herbicide application.
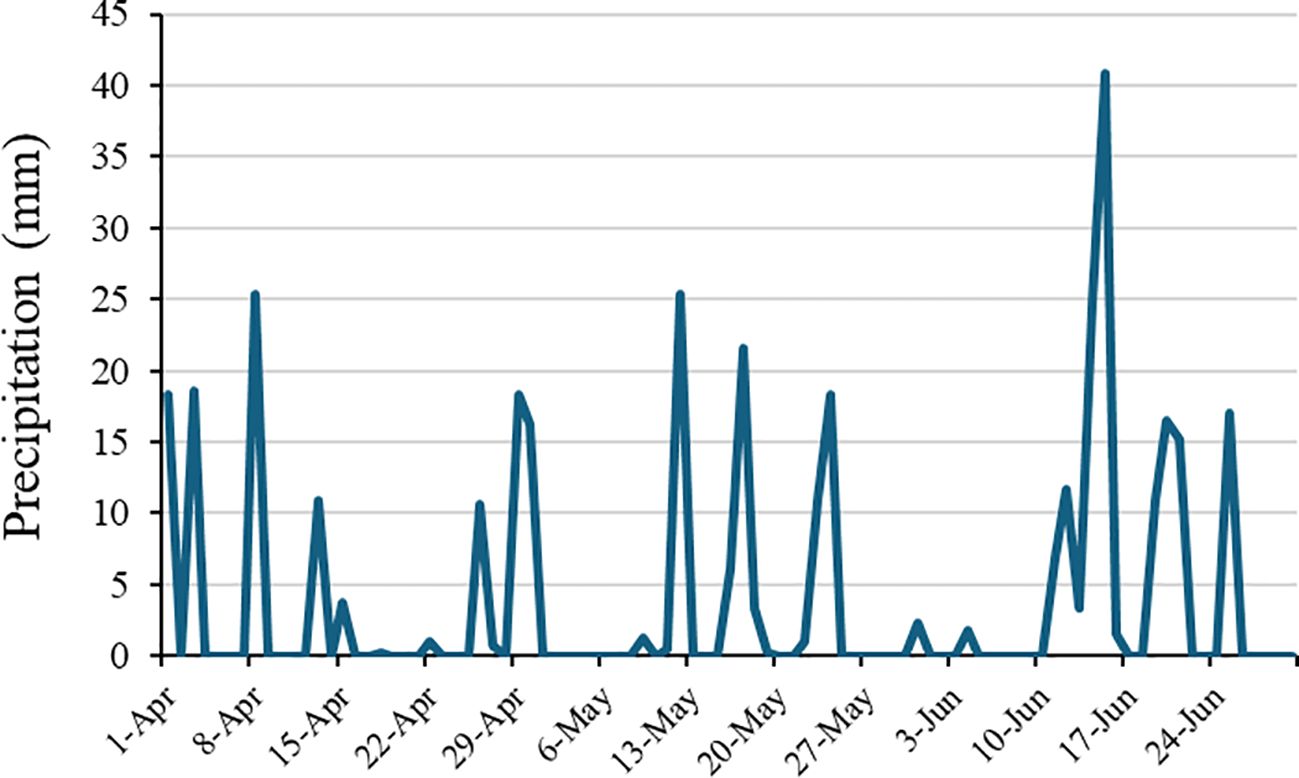
Figure 1. Precipitation data (mm) at E.V. Smith (EVS) location, Auburn, AL, during 2023 growing season.
2.3 Data collection and analysis
In the greenhouse experiment, data collection such as weed counts and weed biomass was performed every 10 days for 30 days. Mostly, the emergence of ivyleaf morningglory and sicklepod occurred within 10 days after planting. However, emergence for Palmer amaranth and large crabgrass was also observed at 20 and 30 days after planting, with weed emergence decreasing over time. Subsequently, all weed counts were summed to show the total counts for each weed species in the graphs.
In the field study, visual weed control ratings on a scale of 0–100 (0 = no control and 100 = complete control), weed counts for each species, and weed biomass collection between 3 and 4 weeks after herbicide application based on randomly selected 0.25-m2 quadrats per plot at each location was performed. In both greenhouse and field experiments, weed biomass collected samples were placed into a drier at 65°C for 72 h, and then the dry weight of weed biomass was recorded.
Data were analyzed using the PROC GLIMMIX model, and means were separated using Tukey’s honestly significant difference (HSD) post-hoc test at α < 0.05 in the SAS statistical software version 9.4 (SAS Institute, Cary, NC, USA). Normality was checked using the qq plot and Shapiro–Wilk test. There was no statistically significant difference between the two runs in the greenhouse; hence, data were pooled. The fixed effects were cereal rye biomass and herbicide treatment, while replication was considered a random effect. The SigmaPlot software (version 13.0; Systat Software, San Jose, CA, USA) was used for curve-fitting regressions and to estimate coefficient values and coefficient of determination (R2), which were utilized to assess the fitness of each regression curve.
3 Results and discussion
3.1 Greenhouse study
3.1.1 Ivyleaf morningglory
There was no significant effect (p > 0.05) of biomass treatments on weed counts and weed biomass reduction of ivyleaf morningglory. Under all biomass treatments, approximately 8.6 to 9.3 million ha−1 weed counts and 420 to 477 kg ha−1 weed biomass were observed (Figures 2A, B). Due to the large seed size of ivyleaf morningglory, its physical suppression by cereal rye residue biomass was not anticipated.
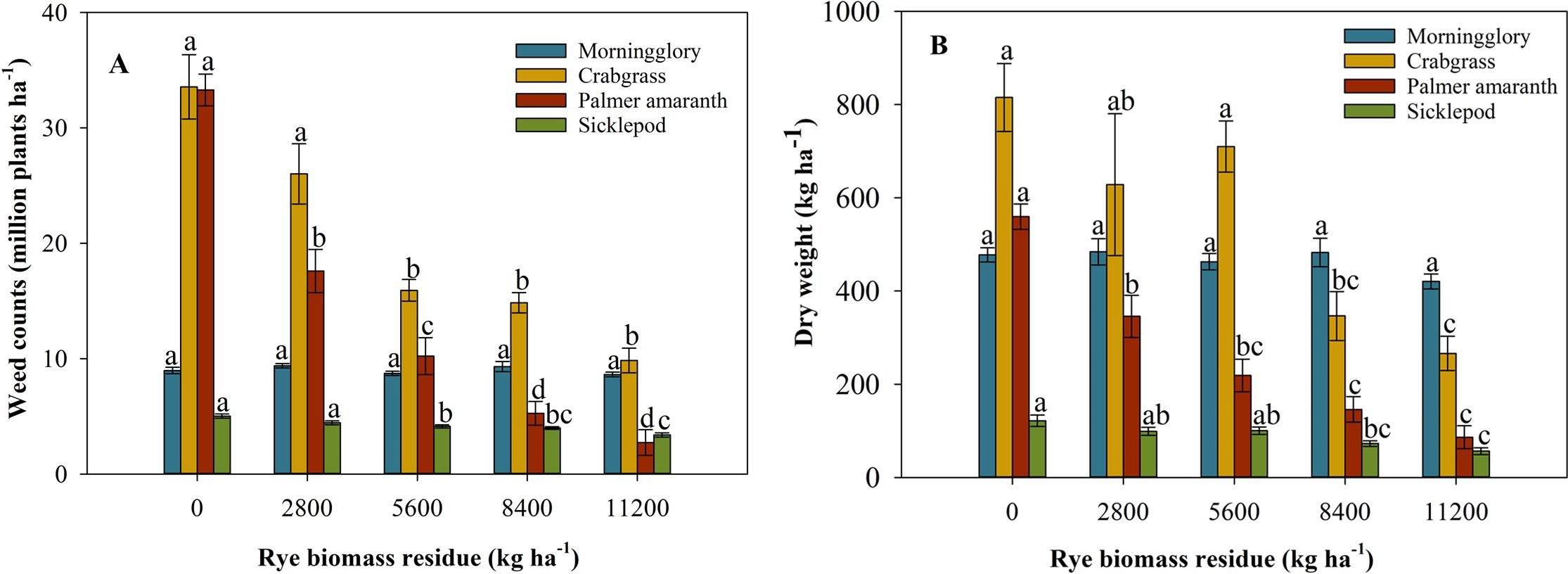
Figure 2. Weed counts (A) and dry weight of weed biomass (B) including Palmer amaranth, ivyleaf morningglory, sicklepod, and large crabgrass under different treatments of cereal rye biomass in the greenhouse condition. Means followed by the same letter within a weed species are not statistically different.
Seed size is a major attribute of its quality because large seeds favor vigor emergence, faster establishment, and growth rate due to more stored resources (Ellis, 1992; Sanderson et al., 2002).
3.1.2 Palmer amaranth
A significant effect (p < 0.001) under various biomass treatments in terms of weed suppression and weed biomass reduction was observed. The low, medium, high, and highest cereal rye biomass reduced the weed counts by 47%, 69%, 84%, and 92%, respectively, compared to the fallow treatment (Figure 2A). Furthermore, low, medium, high, and highest biomass of cereal rye residue decreased the weed biomass by 38%, 61%, 74%, and 85%, respectively, compared to the fallow treatment (Figure 2B). The biomass of the cereal rye residue effectively suppressed Palmer amaranth seeds by inhibiting their growth, which was attributed to its small size. Wiggins et al. (2016) found that cereal rye residue was more consistent in the suppression of Palmer amaranth and provided 59% to 80% control. A previous study has illustrated that cereal rye offered the highest emergence inhibition of Palmer with 83% as compared to fallow checks (Palhano et al., 2018). It is well-known that cover crop cereal rye has the potential to provide several advantages, such as scavenging nutrients, contributing to soil organic matter, preventing runoff losses, and leaching in agricultural crop production systems. Moreover, cover crops could be a part of herbicide resistance mitigation strategy because they can decrease weed density and weed growth, thus lowering seed production, which decreases the possibility of development of herbicide resistance (Owen et al., 2014; Riar et al., 2013).
3.1.3 Sicklepod
A significant effect (p < 0.05) of various biomass levels on reducing weed counts and biomass was found. The highest and high cereal rye biomass reduced the weed counts by 33% and 21%, respectively, compared to the fallow treatment (Figure 2A). The biomass residue including the highest and high treatments decreased the weed biomass by 54% and 40%, respectively, compared to the fallow treatment (Figure 2B). Sicklepods have also large seed sizes, and previous research studies have suggested that the amount of cover crop residues has more impact on suppressing small-seeded weed species compared to large-seeded weed species (Bhowmik and Inderjit, 2003; Teasdale and Mohler, 2000).
3.1.4 Large crabgrass
A significant effect (p < 0.05) of various biomass treatments on reducing weed counts and biomass was observed. The highest, high, and medium biomass of cereal rye residue reduced the weed counts by 70%, 56%, and 53%, respectively, compared to the fallow treatment (Figure 2A). The highest and high biomass treatment decreased the weed biomass by 71% and 56%, respectively, compared to the fallow treatment (Figure 2B). For the suppression of large crabgrass, sufficient biomass is required to suppress its emergence. According to Pittman et al. (2020), large crabgrass required 5,570 kg ha−1 and 11,440 kg ha−1 biomass at termination to achieve 50% suppression at 6 and 8 weeks after termination, respectively. Haramoto and Pearce (2019) claimed that there were variations in annual grass suppression by cover crop residues. Large crabgrass emergence was either the same or decreased following cereal rye residue compared to fallow plots (Brainard et al., 2016).
3.2 Non-linear regression curve
A three-parameter logistic model was used for fitting weed counts and weed biomass for each species individually against cereal rye biomass.
where y is the weed counts and weed biomass, x0 represents the inflection point, b is the slope of the curve or growth rate, α is the asymptote, and x depicts the amount of biomass residue.
All coefficients were found to be statistically significant (p < 0.05), indicating a robust relationship of weed counts and weed biomass with cereal rye residue in the case of Palmer amaranth with R2 = 0.99 for both regressions (Figures 3A, B; Tables 1, 2). Based on the logistic curve, reducing the Palmer amaranth counts by 50%, 75%, and 90% compared to the fallow treatment required 3,112, 6,520, and 10,490 kg ha−1, respectively, of cereal rye biomass. It was observed that weed counts and weed biomass of Palmer amaranth decreased with increasing biomass of cereal rye residue. A previous study by Ryan et al. (2011) also claimed that increasing cereal rye biomass residue suppressed weeds and decreased weed biomass, including pigweeds (Amaranthus spp.).
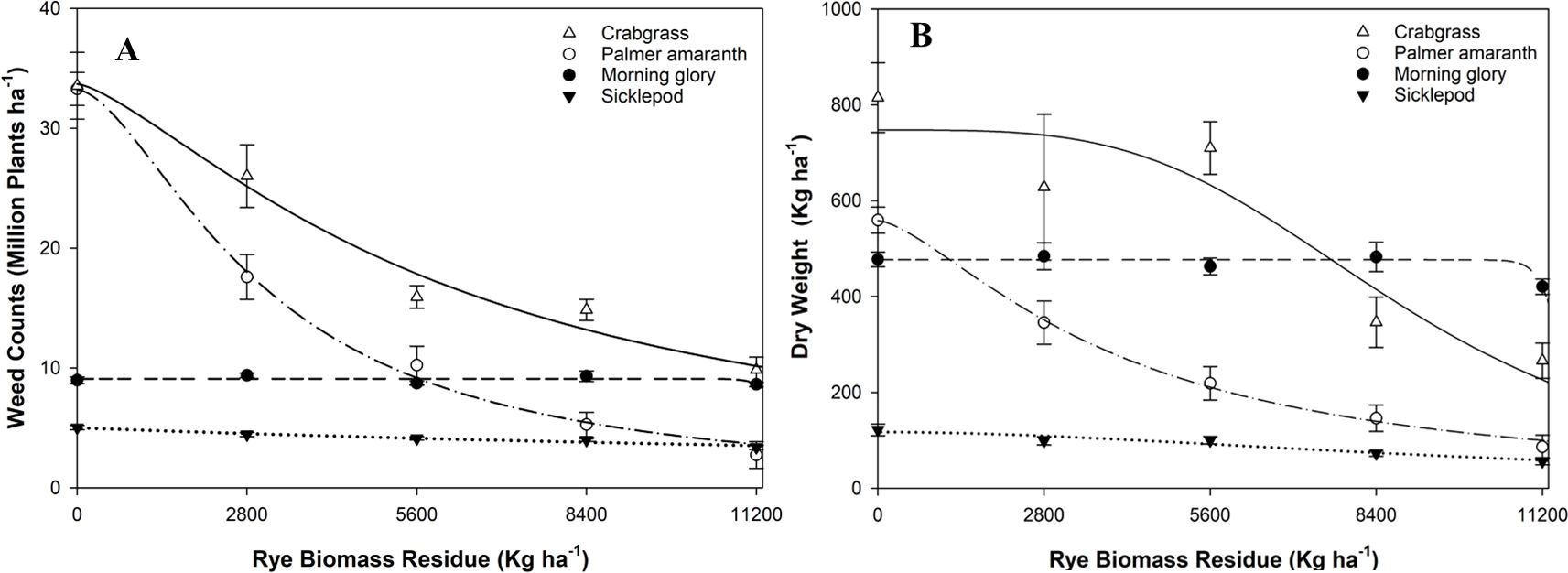
Figure 3. A three-parameter logistic regression curve was fitted to evaluate the effect of cereal rye biomass residue on weed seedling counts (A) and dry weight of weed biomass for Palmer amaranth, morningglory, sicklepod, and crabgrass in the greenhouse condition (B).
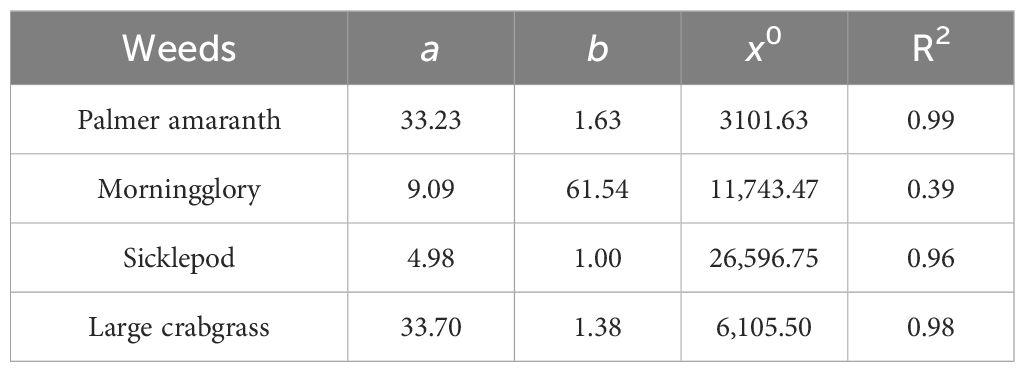
Table 1. Estimated values of three-parameter logistic model used for fitting weed counts against cereal rye residue biomass in the greenhouse condition.
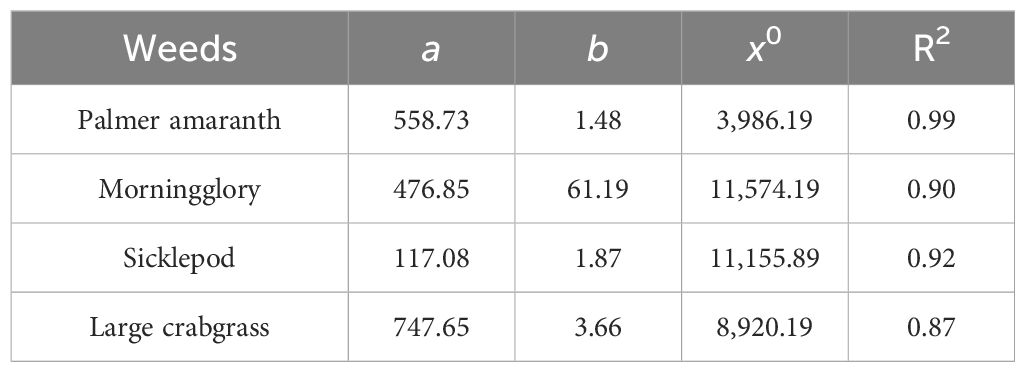
Table 2. Estimated values of three-parameter logistic model used for fitting dry weight of weeds against cereal rye residue biomass in the greenhouse condition.
However, the slope was non-significant (p > 0.05) for weed counts and weed biomass of ivyleaf morningglory with R2 = 0.39 for counts and R2 = 0.90 for weed biomass. The slope was non-significant for sicklepod (p > 0.05) with R2 = 0.96 for weed counts and R2 = 0.92 for weed biomass. Hence, there was no relationship between increasing cereal rye biomass with the reduction of weed counts and weed biomass for ivyleaf morningglory and sicklepod. A decreasing trend in crabgrass counts and biomass was observed with increasing cereal rye biomass, showing R2 values of 0.98 for weed counts and 0.87 for biomass.
3.3 Field experiment
There was a significant effect of seeding rate on cover crop biomass production; however, the effect of location and their interaction was not significant. The seeding rate of 90 kg ha−1 of cereal rye provided significantly greater cover crop biomass as compared to the 180 kg ha−1 seeding rate (Figure 4).
Palmer amaranth and ivyleaf morningglory were the dominant weeds throughout the field studies at both locations. A logistic three-parameter curve was fitted on weed counts of Palmer amaranth and ivyleaf morningglory and biomass of Palmer amaranth against cereal rye biomass residue.
where y represents counts and biomass of Palmer amaranth and counts of ivyleaf morningglory, x0 represents the inflection point, b represents the slope of the curve or growth rate, α represents the asymptote, and x depicts the amount of biomass residue.
For the morningglory biomass, the Gompertz equation was the best fit and was fitted against cereal rye biomass residue.
where y represents weed biomass for ivyleaf morningglory, x0 represents the inflection point, b represents the slope of the curve or growth rate, α represents the asymptote, and x depicts the amount of biomass residue.
3.3.1 Palmer amaranth
All coefficients were found to be statistically significant (p < 0.05), indicating a robust relationship of cereal rye residue with Palmer amaranth counts and biomass with R2 = 0.85 and 0.76, respectively (Figures 5A, B; Table 3). The results from both greenhouse and field studies suggested that as cereal rye biomass increased, there was a significant trend of decreasing Palmer amaranth counts as well as weed biomass. Previous literature has also claimed that the extent of early season weed suppression is strongly influenced by the cover crop biomass accumulation, having greater weed suppression with higher cover biomass (MacLaren et al., 2019; Osipitan et al., 2019). However, weed suppression by cover crops depends on the production of ground cover biomass as well as the persistence of the residue on the ground. According to Palhano et al. (2018), the higher C:N ratio of cereal rye, which is linked to slow residue decomposition, enables it to remain present for most of the crop growing season. This persistence has been shown to suppress Palmer amaranth until 8 weeks after planting.
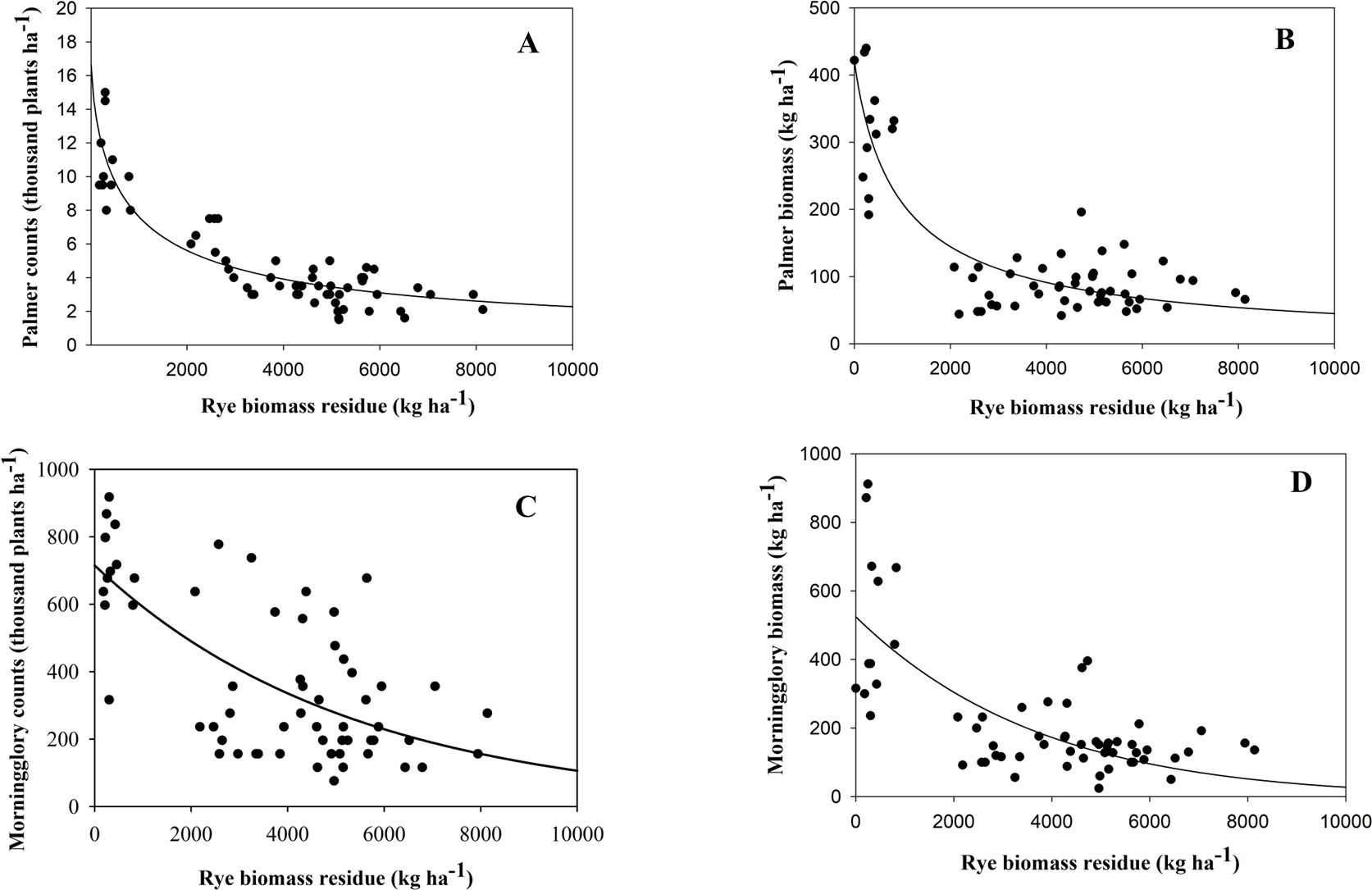
Figure 5. The three-parameter sigmoidal regression curve was fitted to estimate the trend of cereal rye biomass residue on counts (A) and biomass (B) of Palmer amaranth; counts (C) and biomass (D) of ivyleaf morningglory field condition at 7 weeks after cover crop termination.
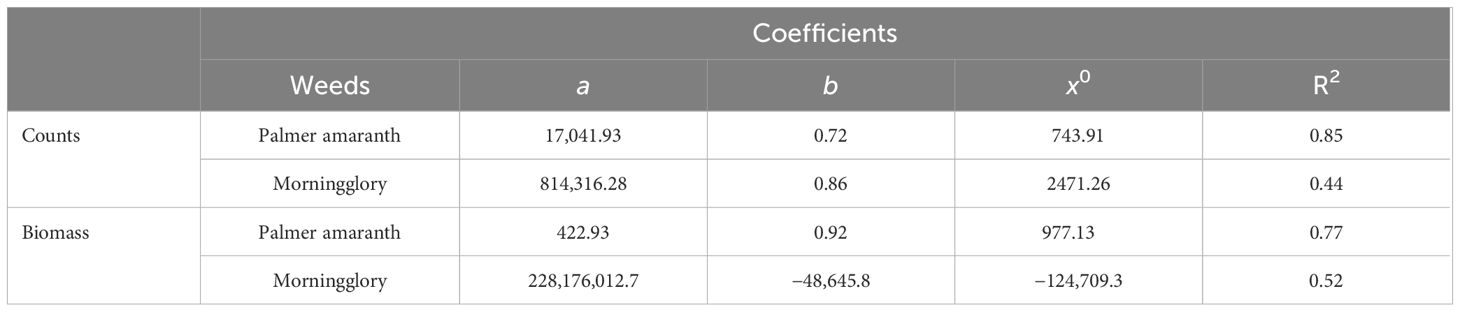
Table 3. Estimated values of three-parameter sigmoidal curve used for fitting counts and biomass of Palmer amaranth and ivyleaf morningglory against cereal rye residue biomass under field conditions.
The results from the field experiment suggested that, for a 10% and 50% relative reduction in Palmer amaranth density, approximately 1,300 and 2,600 kg ha−1 of cereal rye biomass, respectively, were required. Moreover, based on the predicted curve, a 75% maximum reduction in relative Palmer density was observed, and approximately 7,100 kg ha−1 biomass was required to achieve this suppression.
Therefore, if growers are seeking weed suppression in the absence of herbicides, approximately 7,100 kg ha−1 biomass of cereal rye is required to attain 75% suppression of Palmer amaranth. Previous literature has also found that to maintain full-season weed control without any herbicide application, the cover crop biomass threshold should be approximately 8,000 kg ha−1 (Mirsky et al., 2013; Reberg-Horton et al., 2012). According to Ryan et al. (2011), weed suppression, including pigweeds, increases with higher biomass levels of residue, and weeds were totally suppressed above 15,000 kg ha−1 of cover crop biomass. We also found that an increase in the amount of cereal rye residue shows a decreasing trend of Palmer amaranth counts and weed biomass under both greenhouse and field conditions. However, achieving aboveground biomass of a cover crop exceeding 10,000 kg ha−1 is challenging, and farmers may need to incur additional costs for fertilization and earlier planting to enhance cover crop biomass. Managing weed suppression while balancing the cost of fertilization and the timing of planting and termination of cover crops is crucial. It requires finding a balance between achieving significant weed suppression and obtaining optimum residue biomass without incurring extra costs. This balance is essential for the decision-making process. However, research studies and growers have observed significant variability in cover crop biomass production among sites and even between different years. Therefore, to make site-specific decisions, other management practices and the inclusion of herbicides should be tested for each soil type.
3.3.2 Ivyleaf morningglory
The slope of the regression curve was found to be non-significant (p > 0.05) for both counts and biomass with R2 = 0.44 and 0.51, respectively (Figures 5C, D; Table 3).
In other words, this suggests that the effect of increasing cover crop biomass on suppressing weed counts and biomass was not effective. Following the same trend as the greenhouse experiment, counts and biomass of ivyleaf morningglory were not responsive to reducing emergence with increasing biomass residue of cereal rye due to their large seed size, as discussed above.
3.4 Correlation
The correlation between cereal rye biomass, visual control rating, weed biomass, and weed counts of morningglory and Palmer amaranth was estimated and represented in a correlation graph (Figure 6). In the graph, the size of the circle and the color intensity in the circle were used to depict the correlation strength, with bigger circles and darker colors indicating stronger correlations between variables. The blue color indicates a positive correlation, while the red color shows a negative correlation between variables. Furthermore, the correlation is represented from 1 to −1, in which 1 means a positive correlation, −1 means a negative correlation, and 0 means no correlation between variables.
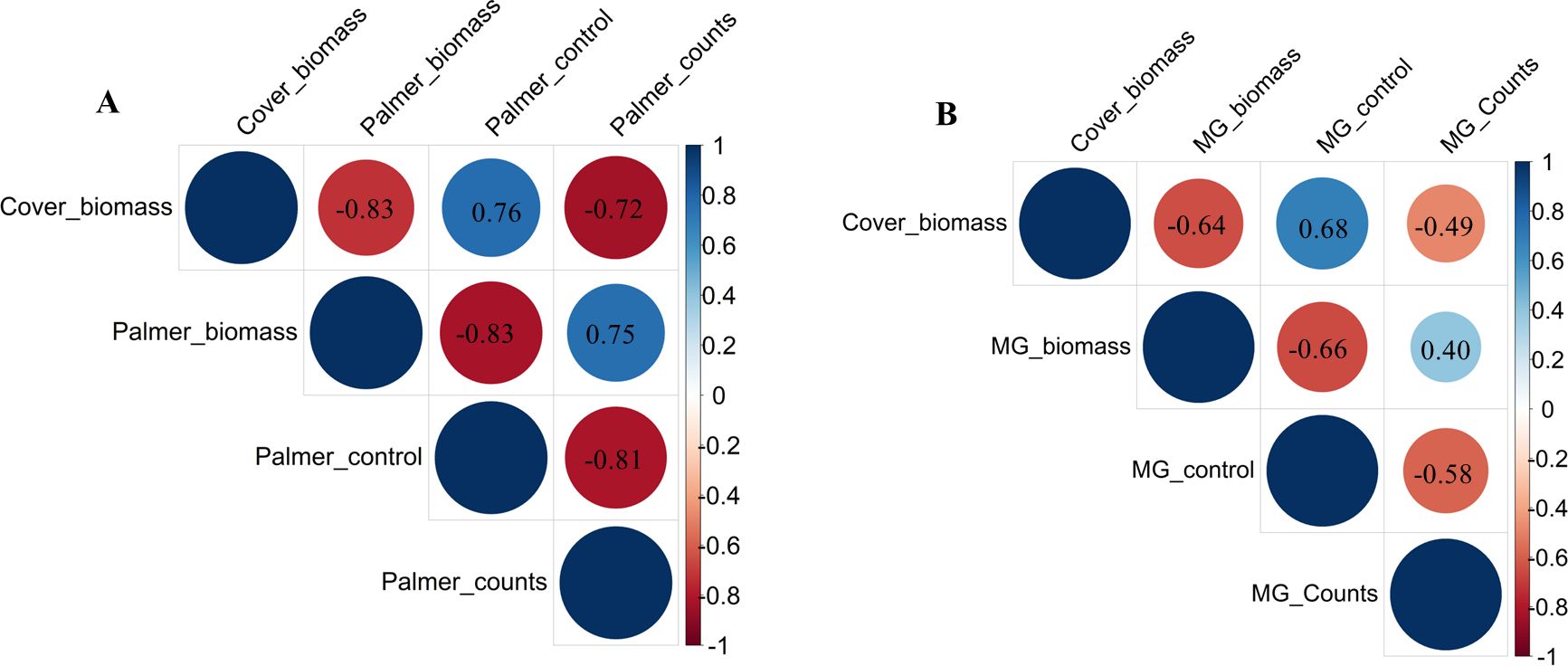
Figure 6. Pearson’s correlation coefficients between variables for the Palmer amaranth (A) and ivyleaf morningglory (depicted as MG) (B) under field conditions.
3.4.1 Palmer amaranth
There was a strong negative correlation of cover biomass with weed counts and weed biomass with a value of −0.83 and −0.72, respectively, showing higher cover biomass, which means more suppression of Palmer and more weed biomass reduction (Figure 6A). As anticipated, cover biomass positively correlated with a visual control rating of 0.76, which means the greater the cover biomass, the better the visual weed control effect. The visual control rating, which exhibited strong negative correlations with weed counts and weed biomass, resulted in values of −0.81 and −0.83, respectively. Weed counts and weed biomass showed a positive correlation of 0.75. Previous literature has also claimed that cover crop biomass was positively correlated with weed suppression (Finney et al., 2016; Florence et al., 2019).
3.4.2 Ivyleaf morningglory
There was a negative correlation of cover biomass with weed counts and weed biomass with values of −0.49 and −0.64, respectively (Figure 6B). It suggested that cereal rye residue may not reduce the emergence of ivyleaf morningglory significantly but could negatively impact the growth of ivyleaf morningglory. Furthermore, cover biomass was positively correlated with a visual control rating of 0.68. Visual control rating was negatively correlated with weed counts and weed biomass, with correlations of −0.58 and −0.66, respectively. A weak positive correlation of 0.40 between weed counts and weed biomass was found.
3.5 Flumioxazin effect
We examined the interaction of seeding rate with herbicide treatments regarding weed control. The results showed that across all seeding rates of cover crops, the effect of weed control was similar for the herbicide treatment. Furthermore, biomass residue was grouped into categories (<2,000 kg ha−1 as A, 2,000–4,000 kg ha−1 as B, 4,000–6,000 kg ha−1 as C, and >6,000 kg ha−1 as D) to assess the interaction of herbicide with levels of biomass residue for weed control; however, no significant effect was found. Herbicide wash-off from cover crop residue is expected due to precipitation/irrigation after herbicide application, leading to its release into the soil. The overall effect of herbicide was found significant (p < 0.01). It has been observed that Palmer amaranth control was 95% when treated with flumioxazin herbicide, while the biomass residue of NT checks only provided approximately 50% control of Palmer amaranth at 7 weeks after cover crop termination (Table 4). However, 4 weeks after cereal rye termination, it was observed that cereal rye provided up to 70% control of Palmer amaranth (data not shown). Significantly lesser counts of Palmer amaranth were found in herbicide-treated plots compared to the NT check. Therefore, herbicide is still required to achieve excellent control of this troublesome weed. In the case of the flumioxazin-treated check, we found that it worked well regardless of cover crop biomass. According to Reeves et al. (2005), at 60 days after planting, weed control achieved using cereal rye with preemergence herbicide was comparable to that achieved in winter fallow checks using both preemergence and postemergence herbicides.
Ivyleaf morningglory control was 90% when treated with flumioxazin while 30% under NT check. Similarly, fewer counts of ivyleaf morningglory were observed in plots that received flumioxazin herbicide compared to the NT check. Previous research studies have also found that preemergence-applied flumioxazin controls many broadleaf weed species such as morningglories and pigweeds (Cranmer et al., 2000; Wilcut et al., 2000). Specifically, flumioxazin as preemergence showed high efficacy and provided 94% control of Palmer amaranth at 4 weeks after application (Whitaker et al., 2010).
The incorporation of cereal rye into the cropping system would not only provide weed suppression but also offer significant soil health benefits, given the importance of cover crops. Furthermore, the integration of cereal rye with preemergence-applied flumioxazin herbicide could be an effective strategy to control glyphosate-resistant Palmer amaranth, which is a major challenge for growers in the Southern USA. According to Palhano et al. (2018), the incorporation of a highly productive cover crop system in combination with preemergence herbicides not only offers early-season weed control but also provides flexibility in scheduling postemergence herbicide application. Additionally, a previous research study at the same site has also demonstrated that cereal rye, in combination with herbicides, provided greater weed control compared to winter fallow with herbicide treatment (Kumari et al., 2024b). Hence, adopting a cereal rye cover crop would be a better tool for weed management.
Data availability statement
The original contributions presented in the study are included in the article/supplementary material. Further inquiries can be directed to the corresponding author.
Author contributions
AK: Formal analysis, Investigation, Methodology, Software, Visualization, Writing – original draft, Writing – review & editing. AP: Conceptualization, Investigation, Methodology, Project administration, Resources, Supervision, Writing – review & editing. SL: Conceptualization, Investigation, Methodology, Project administration, Resources, Supervision, Writing – review & editing. AG: Resources, Supervision, Writing – review & editing. AJ: Resources, Supervision, Writing – review & editing.
Funding
The author(s) declare that no financial support was received for the research, authorship, and/or publication of this article.
Acknowledgments
We wish to thank the station personnel at the E.V. Smith and Wiregrass Research and Extension Centers for their technical assistance in conducting this study.
Conflict of interest
The authors declare that the research was conducted in the absence of any commercial or financial relationships that could be construed as a potential conflict of interest.
Generative AI statement
The author(s) declare that no Generative AI was used in the creation of this manuscript.
Publisher’s note
All claims expressed in this article are solely those of the authors and do not necessarily represent those of their affiliated organizations, or those of the publisher, the editors and the reviewers. Any product that may be evaluated in this article, or claim that may be made by its manufacturer, is not guaranteed or endorsed by the publisher.
References
Bhowmik P. C., Inderjit (2003). Challenges and opportunities in implementing allelopathy for natural weed management. Crop Prot. 22, 661–671. doi: 10.1016/S0261-2194(02)00242-9
Brainard D. B., Bryant A., Noyes D. C., Haramoto E. R., Szendrei Z. (2016). Evaluating pest regulating services under conservation agriculture: a case study in snap beans. Agric. Ecosyst. Environ. 235, 142–154. doi: 10.1016/j.agee.2016.09.032
Cranmer J. R., Altom J. V., Braun J. C., Pawlak J. A. (2000). Valor™ herbicide: a new herbicide for weed control in cotton, peanuts, soybeans, and sugarcane. Proc. South Weed Sci. Soc 53, 158.
CTIC (2017). Report of the 2016-17 National Cover Crop Survey (West Lafayette, IN: Conservation Technology Information Center, North Central Region Sustainable Agriculture Research and Education Program, American Seed Trade Association).
Ellis R. H. (1992). Seed and seedling vigor in relation to crop growth and yield. Plant Growth Regul. 11, 249–255. doi: 10.1007/BF00024563
Farmaha B. S., Sekaran U., Franzluebbers A. J. (2022). Cover cropping and conservation tillage improve soil health in the southeastern United States. Agron. J. 114, 296–316. doi: 10.1002/agj2.v114.1
Finney D. M., White C. M., Kaye J. P. (2016). Biomass production and carbon/nitrogen ratio influence ecosystem services from cover crop mixtures. Agron. J. 108, 39–52. doi: 10.2134/agronj15.0182
Florence A. M., Higley L. G., Drijber R. A., Francis C. A., Lindquist J. L. (2019). Cover crop mixture diversity, biomass productivity, weed suppression, and stability. PloS One 14, e0206195ju. doi: 10.1371/journal.pone.0206195
Haramoto E. R., Pearce R. (2019). Cover crop termination treatment impacts weed suppression potential. Weed Sci. 67, 91–102. doi: 10.1017/wsc.2018.75
Kumari A., Price A. J., Gamble A., Li S., Jacobson A. (2024a). Integrating cover crops and herbicides for weed control in soybean. Weed Technol. 38, e38. doi: 10.1017/wet.2024.24
Kumari A., Price A. J., Gamble A., Li S., Jacobson A. (2024b). Synergistic effect of cover crops residue and herbicides for effective weed management in Southern U. S Cotton Prod. Syst. Weed Technol. 38, e73. doi: 10.1017/wet.2024.49
Kumari A., Price A. J., Korres N. E., Gamble A., Li S. (2023a). Influence of a cereal rye cover crop on the critical period for weed control in soybean. Weed Technol. 37, 25–33. doi: 10.1017/wet.2022.100
Kumari A., Price A. J., Korres N. E., Gamble A., Li S. (2023b). Effect of crimson clover on the critical period of weed control in conservation tillage corn. Front. Agron. 4, 1068365. doi: 10.3389/fagro.2022.1068365
MacLaren C., Swanepoel P., Bennett J., Wright J., Dehnen-Schmutz K. (2019). Cover crop biomass production is more important than diversity for weed suppression. Crop Sci. 59, 733–748. doi: 10.2135/cropsci2018.05.0329
Mirsky S. B., Ryan M. R., Teasdale J. R., Curran W. S., Reberg-Horton C. S., Spargo J. T., et al. (2013). Overcoming weed management challenges in cover crop–based organic rotational no-till soybean production in the eastern United States. Weed Technol. 27, 193–203. doi: 10.1614/WT-D-12-00078.1
Norsworthy J. K., McClelland M., Griffith G., Bangarwa S. K., Still J. (2011). Evaluation of cereal and Brassicaceae cover crops in conservation-tillage, enhanced, glyphosate resistant cotton. Weed Technol. 25, 6–13. doi: 10.1614/WT-D-10-00040.1
Norsworthy J. K., Ward S. M., Shaw D. R., Llewellyn R. S., Nichols R. L., Webster T. M., et al. (2012). Reducing the risks of herbicide resistance: best management practices and recommendations. Weed Sci. 60, 31–62. doi: 10.1614/WS-D-11-00155.1
Osipitan O. A., Dille J. A., Assefa Y., Radicetti E., Ayeni A., Knezevic S. Z. (2019). Impact of cover crop management on level of weed suppression: a meta-analysis. Crop Sci. 379 59, 833–842. doi: 10.2135/cropsci2018.09.0589
Owen M. D., Beckie H. J., Leeson J. Y., Norsworthy J. K., Steckel L. E. (2014). Integrated pest management and weed management in the US and Canada. Pest Manage. Sci. 71, 357–376. doi: 0.1002/ps.3928
Palhano M. G., Norsworthy J. K., Barber T. (2018). Cover crops suppression of Palmer amaranth (Amaranthus palmeri) in cotton. Weed Technol. 32, 60–65. doi: 10.1017/wet.2017.97
Pittman K. B., Barney J. N., Flessner M. L. (2020). Cover crop residue components and their effect on summer annual weed suppression in corn and soybean. Weed Sci. 68, 301–310. doi: 10.1017/wsc.2020.16
Price A. J., Balkcom K. S., Culpepper S. A., Kelton J. A., Nichols R. L., Schomberg H. (2011). Glyphosate-resistant Palmer amaranth: a threat to conservation agriculture. J. Soil Water Conserv. 66, 265–275. doi: 10.2489/jswc.66.4.265
Price A. J., Monks C. D., Culpepper A. S., Duzy L. M., Kelton J. A., Marshall M. W., et al. (2016). High residue cover crops alone or with strategic tillage to manage glyphosate-resistant Palmer amaranth (Amaranthus palmeri) in southeastern cotton (Gossypium hirsutum). J. Soil Water Cons. 71, 1–11. doi: 10.2489/jswc.71.1.1
Price A. J., Nichols R. L., Morton T. A., Balkcom K. S., Grey T. L., Li S. (2021). Effect of cover-crop biomass, strip-tillage residue disturbance width, and PRE herbicide placement on cotton weed control, yield, and economics. Weed Technol. 35, 385–393. doi: 10.1017/wet.2021.8
Price A. J., Norsworthy J. K. (2013). Cover crops for weed management in southern reduced tillage vegetable cropping systems. Weed Technol. 27, 212–217. doi: 10.1614/WT-D-12-00056.1
Reberg-Horton S. C., Grossman J. M., Kornecki T. S., Meijer A. D., Price A. J., Place G. T., et al. (2012). Utilizing cover crop mulches to reduce tillage in organic systems in the southeastern USA. Renew. Agric. Food Syst. 27, 41–48. doi: 10.1017/S1742170511000469
Reeves D. W., Price A. J., Patterson M. G. (2005). Evaluation of three winter cereals for weed control in conservation-tillage nontransgenic cotton. Weed Technol. 19, 731–736. In review 18. doi: 10.1614/WT-04-245R1.1
Riar D. S., Riar D. S., Norsworthy J. K., Steckel L. E., Stephenson D. O., Eubank T. W., et al. (2013). Adoption of best management practices for herbicide407 resistant weeds in midsouthern United States cotton, rice and soybean. Weed Technol. 27, 788–797. doi: 10.1614/WT-D-13-00087.1
Ryan M. R., Mirsky S. B., Mortensen D. A., Teasdale J. R., Curran W. S. (2011). Potential synergistic effects of cereal rye biomass and soybean planting density on weed suppression. Weed Sci. 59, 238–246. doi: 10.1614/WS-D-10-00110.1
Sanderson M. A., Skinner R. H., Elwinger G. F. (2002). Seedling development and field performance of prairie grass, grazing bromegrass, and orchard grass. Crop Sci. 42, 224–414 230. doi: 10.2135/cropsci2002.2240
SARE Sustainable Agriculture Research and Education (2007). Managing cover crops Profitably. 3rd edition. Ed. Clark A. (College Park, MD: SARE).
Schomberg H. H., McDaniel R. G., Mallard E., Endale D. M., Fisher D. S., Cabrera M. L. (2006). Conservation tillage and cover crop influences on cotton production on a southeastern US coastal plain soil. Agron. J. 98, 1247–1256. doi: 10.2134/agronj2005.0335
Sturm D. J., Peteinatos G., Gerhards R. (2018). Contribution of allelopathic effects to the overall weed suppression by different cover crops. Weed Res. 58, 331–337. doi: 10.1111/wre.2018.58.issue-5
Teasdale J. R. (1996). Contribution of cover crops to weed management in sustainable agricultural systems. J. Prod. Agric. 9, 475–479. doi: 10.2134/jpa1996.0475
Teasdale J. R., Mohler C. L. (2000). The quantitative relationship between weed emergence and the physical properties of mulches. Weed Sci. 48, 385–392. doi: 10.1614/0043-1745(2000)048[0385:TQRBWE]2.0.CO;2
Webster T. M., Nichols R. L. (2012). Changes in the prevalence of weed species in the major agronomic crops of the Southern United States: 1994/1995 to 2008/2009. Weed Sci. 60, 145–157. doi: 10.1614/WS-D-11-00092.1
Whitaker J. R., York A. C., Jordan D. L., Culpepper A. S. (2010). Palmer amaranth (Amaranthus palmeri) control in soybean with glyphosate and conventional herbicide systems. Weed Technol. 24, 403–410. doi: 10.1614/WT-D-09-00043.1
Wiggins M. S., Hayes R. M., Steckel L. E. (2016). Evaluating cover crops and herbicides for glyphosate-resistant Palmer amaranth (Amaranthus palmeri) control in cotton. Weed Technol. 30, 415–422. doi: 10.1614/WT-D-15-00113.1
Wilcut J. W., Askew S. D., Price A. J., Scott G. H., Cranmer J. (2000). Valor™—a new weed management option for cotton. Proc. South Weed Sci. Soc 53, 159–160. In review 19.
Keywords: cereal rye residue, weed seed emergence, weed suppression, cover crop, herbicide
Citation: Kumari A, Price AJ, Li S, Gamble A and Jacobson A (2025) Effects of cereal rye residue biomass and preemergence herbicide on the emergence of troublesome southeastern weed species. Front. Agron. 6:1502864. doi: 10.3389/fagro.2024.1502864
Received: 27 September 2024; Accepted: 30 December 2024;
Published: 22 January 2025.
Edited by:
Shibu Jose, University of Missouri, United StatesReviewed by:
Muhammad Saqlain Zaheer, Khwaja Fareed University of Engineering and Information Technology (KFUEIT), PakistanSujeewa Rathnayake, NSW Government, Australia
Copyright © 2025 Kumari, Price, Li, Gamble and Jacobson. This is an open-access article distributed under the terms of the Creative Commons Attribution License (CC BY). The use, distribution or reproduction in other forums is permitted, provided the original author(s) and the copyright owner(s) are credited and that the original publication in this journal is cited, in accordance with accepted academic practice. No use, distribution or reproduction is permitted which does not comply with these terms.
*Correspondence: Annu Kumari, YXprMDEzMkBhdWJ1cm4uZWR1