- VIT School of Agricultural Innovations and Advanced Learning, Department of Agronomy, Vellore Institute of Technology (VIT), Vellore, India
Recently, the injudicious use of herbicides in agriculture is causing numerous hazards that affect the environment and living organisms. To achieve the SDG 2030 agenda goal 12- “Ensure sustainable consumption and production patterns,” there is an urgent need to shift towards using toxic-free agricultural inputs. Various techniques are widely adopted to control weed growth and development, but farmers mostly rely on herbicide application. Nanotechnology-enabled herbicide formulations are more sustainable and efficient in weed control than traditional sources. The nanoherbicides enable lesser application frequency and minimum quantity requirement, thereby preventing herbicide accumulation in soil and water bodies. Mainly nano-mediated biodegradable carrier-based herbicides possess properties of prolonged release, targeted inhibition, reduced mobility in soil, better adhesion to the plant surface, and retards rapid degradation of active ingredients (AIs), which increase herbicide use efficiency against weeds. Biodegradable carrier materials are cost-effective and readily available from living/non-living organisms and mineral sources, which can be an alternate source for metal/metal-oxide carrier materials. Materials like chitosan, plant derivatives, clay particles, and synthetic polymers are notable carrier materials reported for encapsulating or loading herbicide molecules. Applying nano-mediated herbicides is an innovative methodology for controlling weeds while considering environmental safety. This review focused mainly on recent advances in biodegradable carrier-based herbicide application in agriculture to mitigate the crisis in sustainable weed management. The ultimate objective of this manuscript is to serve as a source of reference material for exploring the nano-based herbicide formulations, their mobility, fate, and future perspectives.
1 Introduction
The global population is projected to increase from over 7 billion to 8.7 billion by 2033, which is expected to directly impact the per capita food consumption (OECD/FAO, 2024). The increasing demand for food production needs better utilization of natural and renewable resources (Fraceto et al., 2016). To mitigate the food demand, agriculture is demanding the application of more inputs, including fertilizers and pesticides. Weeds are renowned for their competitive behavior in agriculture, resulting in yield losses. Therefore, weed management is an essential inter-cultivation practice during crop production. Agriculture often faces challenges in managing the use of plant protection chemicals. Nevertheless, weeds account for the highest proportion of production loss among all other pests in agriculture, with an average yield loss of 34% (Jabran et al., 2015). Crop-weed competition is prevalent in agriculture, and it diminishes the yield of crops (Campos et al., 2023). The foremost cause of competitiveness is the weed’s interest in resources such as light, water, and nutrients (Singh et al., 2022).
Herbicides are a type of formulation that falls under the pesticide category (Periakaruppan et al., 2023; Rajput et al., 2021), which are described as any substance that is used to prevent, destroy, or inhibit the growth of weeds, mainly in agricultural lands (Paul et al., 2024). In 2023, global agricultural pesticides consumption rates were as follows: herbicides > fungicides and bactericides > insecticides > others, with an amount of 1732.3, 816.38, 757.54, and 220.15 thousand metric tons (FAO, 2023). Herbicides are chemical compounds derived from synthetic or naturally available substances. Herbicides are motivated to control or inhibit weed growth in the crop circumstance, which has resulted in a reliable source of controlling weeds, alongside causing anthropogenic pollution such as degrading soil fertility, water, and air quality (Singh et al., 2023; Verma et al., 2022).
Herbicide application in greenhouses and open fields is common in agriculture, leading to occupational exposure among workers (Sameeha, 2023; Abdollahdokht et al., 2022). Herbicides applied, either foliar or soil, may undergo leaching, volatilization, and runoff, affecting biodiversity (Wilms et al., 2020). Unfortunately, it accumulates in soil and water bodies and reaches the food chain, which can cause hazards to human life and the environment for several years (Ghazi et al., 2023). Herbicides such as 2,4-D, oxyfluorfen, glyphosate, atrazine, diuron, tebuthiuron, alachlor, metribuzin, fenuron, monuron, metolachlor, fluometuron, and ametryn are reported as water pollutants in several research findings (Brillas, 2021). For instance, glyphosate is one of the most widely used herbicides throughout the world, and it is identified as an essential factor causing various illnesses such as cancer, autism, infertility, depression, Alzheimer’s, Parkinson’s, and obesity in human society (Anarghou et al., 2024). The herbicides were found to be causing detrimental effects through biological magnification and long-term residual buildup in the ecosystem (Ebube et al., 2024). On the other hand, the target organism does not fully utilize the applied chemical because of various external factors. Over 90% of pesticides, including herbicides, insecticides, and fungicides, are going unutilized after application (Paul et al., 2024). To overcome this peril, the United Nations stipulated its third goal in the SDG 2030 agenda to reduce the disorders caused by chemical contamination in living circumstances (Onyeaka et al., 2024).
In a recent field experiment, the herbicide residue in the post-harvest soil was investigated. When four herbicides (triasulfuron, clopyralid, pyroxasulfone, and chlorsulfuron) were applied to the wheat crop at the mid-tillering stage, the number of nodules, root length, shoot length, and whole plant weight decreased in subsequently cultivated pasture and legume crops (Yates et al., 2024).
In agriculture, synthetic herbicides are considered a cost-effective and efficient method of weed control. But the herbicide resistance is also one of the worries brought by the new generation of herbicides (Kumar et al., 2017). There are 533 herbicide-resistant cases, with 273 weed species (156 dicots and 117 monocots) reported to 168 different herbicides. According to the HRAC (Herbicide Resistance Action Committee), 34 herbicide groups were classified based on mechanisms of action. To support this certainty, a survey has been reported, where the resistance to herbicides has the highest share of 54% in the Poaceae family, followed by Brassicaceae and Asteraceae with 30% (Oreja et al., 2024). Figure 1 depicts the recent data on herbicide resistance that occurs worldwide due to the overuse of herbicides. In a broader sense, herbicide production without a new mode of action will be prone to herbicide resistance in various weed species (Khan et al., 2024). Target-site resistance (TSR) and non-target-site resistance (NTSR) are two mechanisms responsible for resistance in most weed biotypes (Sen et al., 2023). At higher levels, both TSR and NTSR mechanisms can sometimes combine and produce survival mechanisms at an individual level or within a population. In addition, herbicide resistance in a trait is acquired through the pollination nature of the weed species. Notably, different resistance mechanisms to single or multiple herbicides can be present in cross-pollinated weed groups; this process occurs rapidly in cross-pollinated groups compared with self-pollinated groups (Gaines et al., 2020).
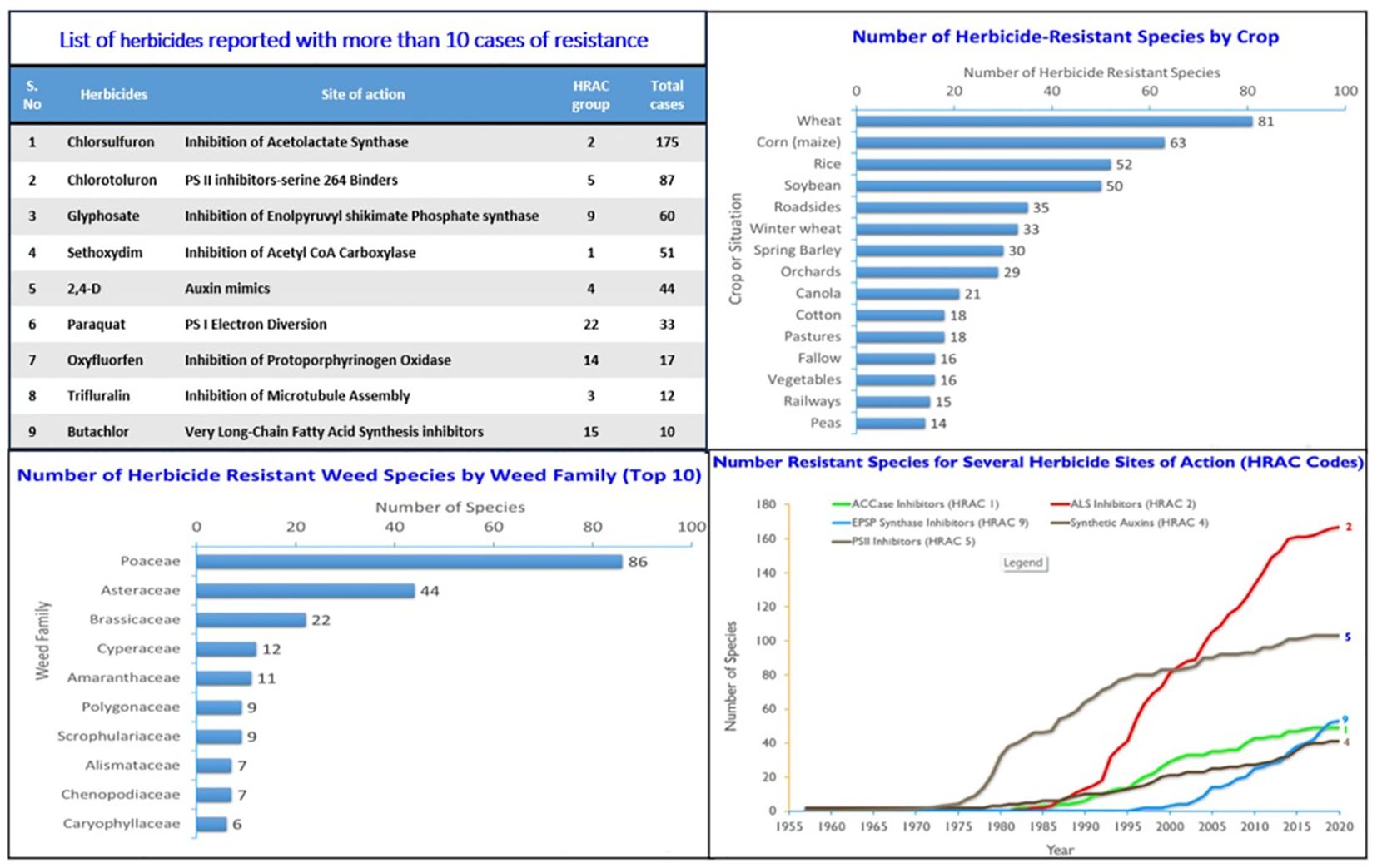
Figure 1. Data on herbicide-resistant weed species sourced from the international survey of herbicide-resistant weeds (Source: Heap I. The International Herbicide-Resistant Weed Database. www.weedscience.org).
TSR mechanism occurs mainly due to 1) gene mutation affecting the herbicide binding site at single or multiple protein targets polymorphism, 2) codon deletion, and 3) gene duplication. NTSR is more complex than TSR in terms of mechanisms of action, including reduced translocation, adsorption, more sequestration, and metabolic degradation. Generally, herbicide efficacy depends on the quantity of herbicide that enters a plant cell and how long it reacts with the target site. GSH S-transferases, cytochromes P450, aryl acylamidase, glucosyl, and other enzymes cause herbicide metabolism (produce noninhibitive by-products) resulting in herbicide resistance (Gaines et al., 2020). The sustainable bioactive chemical release and targeted inhibition of nano-enabled molecules remain long-term in the crop environment. This leads to avoiding frequent herbicide application, which subsequently reduces weed resistance. Hence, the diverse resistance mechanism of weeds can be mitigated by applying nanoherbicides.
After the green revolution, pesticides emerged as an inevitable component of agriculture, but concealing considerable complications in pesticide use must be redefined (Shangguan et al., 2024). Consuming tainted food and water with pesticide residues impacts the general public. These constraints indicate the need for intelligent, responsive, biodegradable, and biocompatible alternatives (Abdollahdokht et al., 2022). Nanotechnology is approved to be a novel technology that can revitalize the farming system and food production. Numerous uses of nanotechnology have been found in various domains, including chemical production, engineering, energy, clothing, beauty care, medical science, material science, food, and agriculture (Khan et al., 2022; Paul et al., 2024).
Combining herbicide molecules with nanoparticles (NPs) increases efficiency by up to 84% (Rajput et al., 2021). Nanotechnology in agriculture is becoming a component of “Precision farming” and “Sustainable agriculture” owing to its priority of increasing food production, with more focus on environmental safety (He et al., 2019). To maximize the crop yield, nanoparticles, and nanocomposite-enabled crop protection agrochemicals provide the advantages of controlled release and increased use efficiency against pests and diseases (Abd-Elsalam, 2024).
Nanotechnology-enabled agriculture practices can be considered a forthcoming green revolution, with reduced threats caused by the contemporary green revolution (Kumar, 2020). Nanotechnology in agriculture involves the production of nano-size inputs in the range of 1-100 nm materials for tackling agricultural constraints and global food shortages (Campos et al., 2023; Lv et al., 2023). The synonym of “nano” is one-billionth of a meter (Nuruzzaman et al., 2019), roughly 1/80,000 the size of a human hair diameter, or approximately the size of 10 hydrogen atoms (Paul et al., 2024). To achieve sustainability, targeted delivery and dose reduction could be an effective approach (Khan et al., 2024; Gayathri et al., 2023). Nano-based biodegradable herbicides open the window for mitigating concerns about controlling weeds on the agricultural field sustainably. Recently, several benefits of polymeric carriers have been studied extensively in the biomedical field to develop drug delivery systems (MaChado et al., 2022).
In agriculture, polymeric nanocarriers are underutilized, and their applications have been explored less in weed control. However, the commercial availability of carrier-based nanoherbicides is less prevalent. The high production cost for perfect infrastructure and lack of multidisciplinary approach in laboratory research with multiple field trials. Thus, nanoherbicide application in agriculture has opened up wider research opportunities in the current decade.
2 Nanoherbicide- an alternative to conventional herbicide
Nano-herbicides are specially fabricated herbicides, and the AI molecules are coated or loaded with nano-size (1-100 nm) carrier materials (Paul et al., 2024). Innovation of new agricultural methods and technologies is a highly paid priority among the global communities to discard hunger and malnutrition (Food and Agriculture Organization., 2018; Xin et al., 2020). Figure 2 represents the chronological evolution of herbicides over the years. In numerous studies, conventional herbicides have demonstrated low water solubility and inconsistent release kinetics. It directs farmers to apply more chemicals in agricultural fields, which leads to high application costs, herbicide resistance, and toxicity buildup in the ecosystem. On the other hand, nano-enabled herbicide delivery writes off pollution concerns and minimizes labor costs for herbicide application with a lesser quantity (An et al., 2022).
Nano-enabled herbicides reduce the overall requirement for herbicide quantity owing to the extended-release of AI molecules without undergoing external losses (de Oliveira et al., 2015; Zargar et al., 2023). Nanoencapsulated atrazine, clodinofop propargyl, and fenoxaprop-p-ethyl used at 10-fold less quantity on weeds provide similar results to commercial formulations (Khan et al., 2022). Nano-encapsulated herbicides obtain a high surface area and subsequently require much less active compounds in their primary field application (Kremer, 2019). Applying nanotechnology-intervened encapsulated herbicide formulations increases weed control efficiency and reduces environmental threats (Kah and Hofmann, 2014). The higher surface area of the particle to the lesser volume ratio guarantees the NPs a powerful carrier tool and makes them a vector for bioactive molecules (Xin et al., 2020).
Nano-herbicides are specifically engineered to tackle several limitations, such as evaporation, volatilization, leaching, runoff, erosion, photochemical degradation, and microbial and chemical degradation (An et al., 2022; Paul et al., 2024). The wettability and dispersibility of herbicide formulations are enhanced when herbicides are loaded into nanomaterials containing a high specific surface area and minute herbicidal active ingredients (AI) (Forini et al., 2022; Manisankar et al., 2022). To optimize the herbicidal effects of nanoherbicides over traditional herbicides, the primary focus of their fabrication is on improving their physiochemical features, such as size, shape, surface chemistry, concentration, etc. The chemical makeup of the nanomaterials determines the interaction of nanoherbicides with weed foliage, roots, and seeds (Forini et al., 2022).
According to the properties and chemical constituents of carrier materials, nanoherbicides are divided into three types: organic, inorganic, and hybrid-based (Forini et al., 2022). Organically available carrier materials such as proteins, lipids, lignocellulosic materials, clays, etc (Su et al., 2019). possess easy degradability, better stability, bio-efficacy, easy availability, and feasibility. The encapsulation technique driven through biodegradable polymers offers various benefits, including enhanced efficacy, sustainable biological compound release, environmental factor protection (Sahoo et al., 2022), and self-life of materials (An et al., 2022; Campos et al., 2023).
On the other hand, metal and metal oxide NPs act as carrier materials for delivering AI molecules owing to their high surface area, higher reactive ability, and optical and electronic properties (Xin et al., 2020). However, the pristine metallic NPs pose negative impacts, such as unaddressed environmental hazards and health risks to organisms (Lead et al., 2018). Even though metal NPs have the potential to act as carrier materials, they exhibit a wide range of toxicity threats in the ecosystem. For example, foliar application of metallic NP suspension (10 to 1000 ppm) resulted in the accumulation of metal NP content in plant (root and shoot) parts ranging from 10 to 70 mg/kg of dry weight) (Su et al., 2019). In conventional agrochemicals, currently accessible carrier materials are derived from petroleum, such as polyurea and polyolefins, exhibiting notable resistance to degradation. Consequently, this characteristic leads to the buildup of detrimental substances within the biogeochemical cycle (MaChado et al., 2022).
Hybrid nanoherbicides are different nanomaterials in a single structure with herbicide molecules. Hybrid nanomaterials contain agrochemicals with two or more carrier materials like natural polymers, fabricated polymers, and inorganic NPs (Khan et al., 2022). Combining the potential chemical interaction of different nanomaterials turns them into outstanding carrier materials. Hybrid nanomaterials promote traceability, targetability, and stimuli-responsive properties like organic and inorganic types. Thus, hybrid nanomaterials pose an excellent source of carrier materials for better affinity and spreadability with plant surfaces and ensure sustained release with a reduced chance of UV irradiation degradation (Forini et al., 2022). Completely biomass-based hybrid materials constitute xylan, starch, cellulose, lignin, etc. For example, a study reported that hybrid xylan-lignin nanomaterials have core-shell structure and amphiphilic properties (Jiang et al., 2020). Natural clay (inorganic mineral) and biopolymers are compatible after making chemical alterations and act as carriers for herbicide molecules (Granetto et al., 2022). Further, the release kinetic and weed control abilities were discussed briefly in the upcoming section of the review.
Since the carrier material’s biodegradability is a high priority among the available sources, we have reviewed recently published biodegradable carrier-based nano herbicide applications for weed control in agriculture in the upcoming sections. Naturally available or synthetically produced biodegradable polymers such as chitosan and alginates are synthesized by the assembly of copolymers, which are scientifically proven to be eco-friendly biopolymers (Muthukrishnan et al., 2019).
Managing weed flora through administering nano-mediated herbicides is perfectly compatible with its controlled-release mechanism (CRM) (Kumar et al., 2017; Paul et al., 2024) and reduces phytotoxicity in the crop ecosystem. For example, crosslinking substances like glutaraldehyde or genipin can enhance the structural integrity and stability of chitosan NPs, ensuring the controlled release of herbicide molecules (Thambiliyagodage et al., 2023). The CRM was ensured by adsorbing, attaching, entrapping, or encapsulating the AI with organic and inorganic nano-based carrier materials (Paul et al., 2024). Furthermore, optimized diuron nanoformulation (ODNF) was synthesized using subabul stem lignin as a matrix for the controlled release of herbicides. The polymer matrix greatly influences the release kinetics and bioefficacy (weed control) of the AI molecules. Notably, the release of diuron was observed under HPLC in all the pH levels (acidic-5.0, neutral-7.0, and basic- 9.0). The ODNF results in a nonlinear biphasic release of AI from the matrix, indicating that initial burst release is followed by controlled release until the 120th day. The release rate was highly influenced by the pH, where the acidic pH was slower than the neutral and basic pH, released at 53%, 62.2%, and 67%, respectively. The initial fast release is due to the diffusion of herbicide molecules from the periphery of the outer matrix. In contrast, the subsequent slow release in a while is due to an increase in diffusion distance towards the inner core of the matrix (presence of diffused herbicide molecule in the depleted zone). This result provides insights into CRM occurrence in the nano-enabled herbicide molecules without compromising weed control (Yearla and Padmashree, 2016). For instance, traditional herbicides are less effective in dryland conditions (Sahoo et al., 2022) due to the dormant weed seed bank in the subsurface soil. If the weed seed gets optimum moisture for its germination, the nondegraded nanoencapsulated herbicide molecules in the soil can restrict its growth and development.
Nanoformulations such as nano-encapsulation, nano-gels, nano-emulsions, etc., have better efficacy of AI molecules and pledge environmental safety (Abdollahdokht et al., 2022). The primary objectives of nano-encapsulation are controlled release and stimulus-responsive release (Ananikov, 2019; Xin et al., 2020). Nano-encapsulated pesticides are developed to promote the smart release (Gomollón-Bel, 2019) of AI molecules under biotic (plant pathogens, insects, and weeds) and abiotic (drought, soil texture, flooding, sunlight, temperature, and salinity) stresses. For instance, paraquat (PQ) is a widely used herbicide that binds to the photosystem I (PSI) site and inhibits electron transport to nicotinamide adenine dinucleotide phosphate (NADP+). PQ exposure to humans is highly toxic and causes acute ingestion, and chronic exposure leads to severe health problems. The compatible, user-friendly, photosensitive PQ formulation was loaded with supramolecular vesicles. The supramolecular vesicles were formulated using hydrophobic PQ as an inner core coated with two hydrophilic layers of an azobenzene derivative, and cucurbit[8]uril forms a 1:1:1 ternary complex. Under sunlight and UV irradiation, the azobenzene derivative changes its trans- to its cis-form, resulting in ternary complex dissociation. Furthermore, the formulation ensures the slow release of AI molecules and suppresses the invasive weed species (Estuca arundinaceae), similar to the free PQ. In addition, an in vivo study on the zebrafish and mouse model ensures an enhanced safety profile of the herbicide formulation (Gao et al., 2018).
Herbicides are lost in several ways, such as volatilization, vapor drift, and leaching of AIs. In most cases, the herbicides undergo volatilization after immediate application to the target area. Subsequently, drift happens due to wind blowing, and low soil adsorption causes the leaching of herbicide molecules. Nanohydrogels are a particular type of formulation that offers a variety of applications for agrochemicals with higher use efficiency and environmental sustainability. The high stability and low solubility of the formulation ensure the sustainable release of AIs and inhibit the target pest without affecting the other organisms (Kashyap et al., 2015; Ghaderpoori et al., 2020; Artusio et al., 2021). The mini emulsion template was formulated using sunflower oil, where the hydrophilic dicamba herbicide was loaded/encapsulated with alginate nanoparticles to form nanohydrogels. The presence of biocompatible sunflower oil and alginate NPs overcomes premature degradation of AIs. In addition, it acts as a toxic-free surfactant to reduce surface tension and as an adjuvant to improve plant uptake (Artusio et al., 2021). Similarly, using the ionic gelification method, chitosan-paraquat (PQ) encapsulated nanohydrogels were produced using sodium triphosphate and xanthan crosslinkers. The above studies resulted in the slow release of herbicide molecules and enhanced herbicidal efficacy (Ghaderpoori et al., 2020).
A nanoemulsion is a colloidal system containing continuous (external phase) and dispersed (internal phase) phases of two immiscible liquids, mainly oil and water. These nonpolar liquids cannot mix, so the nanoscale droplets of one liquid are dispersed into another liquid (e.g., oil in water). The studies reported that oil-in-water (O/W) formulation demonstrated better stability and shelf life. The nanoscale droplet in the formulation provides a high surface-to-volume ratio that enhances the utility of the formulation. The surfactants or emulsifiers are used as a protective coating material for the oil droplets that prevent creaming and deposition or sedimentation over the period. The hydrophobicity of the surfactants increases the electrostatic repulsive force between the nanodroplets to avoid coalescence. The stability depends on the quantity of the surfactant used in the nanoemulsion (increasing quantity yields more stability). It is important to have a surfactant with both hydrophobic and hydrophilic structural groups for better interaction with water and oil (Bamisaye et al., 2023). A study revealed the nanoemulsion containing palm oil and Parthenium hysterophorus L. crude extract (PHCE) for pre-emergence application against slender button (Diodia ocimifolia) weed in Malaysian palm oil cultivation. The optimum quantity of Parthenium hysterophorus L. extract, palm oil, and surfactants was blended using a high-shear homogenizer. The nanoemulsion showed better weed control with a lesser concentration (5 g L-1) than crude extract alone (10 g L-1). The reason could be better adhesion of the formulation with the plant surface, subsequently increasing the uptake. After 60 days of storage, the nanoemulsion exhibits an increase in particle size (droplets), which can be attributed to the Ostwald ripening phenomena (Zainuddin et al., 2019).
In addition, biopolymers’ nano-sized structures act as a shield for herbicide molecules. Hence, biopolymers are biocompatible and biodegradable carrier pesticide materials (Abdollahdokht et al., 2022). The controlled and targeted release of herbicides can be engineered with biodegradable carrier materials such as chitosan (Khan et al., 2023; Li et al., 2023), plant-based NPs (Carvalho et al., 2023; Rodríguez et al., 2022), organoclays (Granetto et al., 2022; Zha et al., 2022), and biodegradable polymers (Schnoor et al., 2018; Mahmoudian et al., 2020; Moore et al., 2022). This review focuses mainly on research related to biodegradable carrier-based herbicides for better weed control while paying more attention to environmental safety. The inevitability of utilizing biodegradable carrier materials in various applications holds significant importance and presents extensive opportunities for developing nanoherbicide formulations. An examination of the crucial role played by sustainable carrier-based nanoherbicide applications in the field of agriculture is provided in this review.
3 Encapsulation of herbicides in biodegradable carrier materials
This part of the review highlights the characteristics and compatibility of biodegradable carrier materials. The concept of nanoencapsulation is to reduce the use of high chemical concentrations without compromising efficiency and environmental safety (He et al., 2019). The advantages of encapsulated nanoherbicides include the properties of reduced soil mobility, genotoxicity, and phytotoxicity, which favor the ecological balance (Liu et al., 2021; Bratovcic et al., 2023). Nanoscale materials are made of organic and inorganic substances to protect AI molecules. The AIs are protected from uncontrolled degradations such as hydrolysis, photolysis, volatilization, leaching, and microbial decomposition of agrochemicals. (Nuruzzaman et al., 2016; Xin et al., 2020). Recently, the availability of clay minerals, polymers, metals, and metal oxides has been widely utilized to produce carrier materials to load the active ingredient for the slow-release mechanism (Paul et al., 2024).
The polymer-based nanoencapsulation has been evoked in the interest of longer storage time and easy handling, leading to the prolonged biological viability of the formulation (Chang et al., 2022). In addition, the polymeric nanoparticles exhibit increased efficacy over traditional herbicides by enabling targeted delivery, prolonged release, and better uptake (Machodo et al., 2022). Figure 3 illustrates the difference between conventional herbicides and nanoencapsulated herbicides. The controlled release of nanoherbicides in the plant and soil systems gives environmental compatibility by getting rid of concerns such as runoff and leaching of hazardous substances into the ecosystem (MaChado et al., 2022). Encapsulating AI molecules in carrier materials rather than as being pure active ingredients, increases the herbicidal activity (Forini et al., 2022). For example, essential oil nanoencapsulation showed superior growth suppression capacity and sustained release of herbicidal compounds against the weed species (Campos et al., 2023).
Herbicides that are nanoencapsulated with biodegradable polymers have a biologically active surface that helps better adsorption and penetration in the plant system (Pan et al., 2023). Biopolymers like chitosan, zein, alginates, and synthetic or naturally available polymers can be produced either in the bottom-up or the top-down approach. The top-down approach uses fewer solvents and other toxic chemicals and delivers both hydrophobic and hydrophilic bioactive compounds, easing production at a large scale. The synthesis techniques fall under two categories viz., 1) hydrophobic (emulsion evaporation and nanoprecipitation or solvent displacement), and 2) hydrophilic (ionic gelation and the double emulsion method), and others like photo-crosslinking. The hydrophobic surface of the polymeric nanoparticles is highly preferred for agriculture utilization. The polymeric nanoformulation synthesis technique relies on the type of polymer, AI, and surfactant chosen for the production. For agriculture purposes, nanoprecipitation or emulsion technique is highly preferred. Both techniques methodologically use organic solvents like alcohol, ethyl acetate, and acetone (Shakiba et al., 2020). On the other hand, ionic gelation, double emulsion, and photo-crosslinking methods were often adopted less due to stability and complications in synthesis. Furthermore, Shakiba et al., 2020 have briefly explained each technique. Nanoencapsulation uses different carrier materials to make herbicide formulations. Such formulations have high loading capacity, effective water dispersibility, improved adhesive properties with leaf surface area, and sustained release (Hao et al., 2020). The organoclay minerals represent an alternative selection of carrier materials, offering novel prospects for precise release mechanisms and extended durability within the soil (Paul et al., 2019).
Likewise, the hybrid nanomaterials are an excellent source of carrier materials. It is worthwhile to mention that hybrid NPs are the composites of organoclay-plant NPs (e.g., K10 Na-montmorillonite/carboxymethyl cellulose) (Granetto et al., 2022), and biopolymer-plant NPs (e.g., chitosan/pectin/sodium tripolyphosphate) (Rashidipour et al., 2019). It also includes combining two different biopolymers (e.g., PLGA/PVA) (Chen and Wang, 2019), and carbon NP-plant NPs (carbon nanotubes (CNT)/gum arabic) (Ke et al., 2021). This combining ability opens up the multidimensional characteristics of NPs and provides an opportunity to develop encapsulated nano-herbicides for sustained release, focused delivery, and escalated affinity on plant parts (Takeshita et al., 2023; MaChado et al., 2022).
The interaction between AI molecules and carrier materials is an important aspect of nano-herbicide production. Van der Waals forces, hydrogen bonding, and hydrophobic interaction drive the affinity between bioactive molecules and carrier materials (Nuruzzaman et al., 2019). The encapsulation of AI molecules using diversified nanomaterials provides control over the release of pesticides, prevents chemical accumulation in the environment, and responds to better weed control (Liu et al., 2021). The AI molecules of plant protectants can be loaded into nanoscale materials. The engineered structures have internal core material (pesticides) and external carrier material (Singh et al., 2023; Wu et al., 2021).
The accelerated or decelerated release of bioactive compounds is believed to be influenced by physical, chemical, and biological degradation, such as hydrolysis, thermolysis, photoresponse, etc (Shakiba et al., 2020). The carrier releases AI on a stimuli-responsive basis, responding to various factors like pH, temperature, enzyme, redox reaction, light intensity, relative humidity, and osmotic pressure (Huang et al., 2018; Camara et al., 2019; Gao et al., 2020; Xin et al., 2020). The overall herbicidal performance of the AIs depends on the release profile of the carrier material.
The dispersion of AIs from the carrier matrix may happen by any release behaviors as follows: Fickian diffusion, swelling or relaxation, and surface or bulk degradation can cause the initial “burst release” of AIs (Kamaly et al., 2016). In an in vitro experiment, pH-responsive release of AI molecules with degradation of carrier NPs at high pH conditions in phloem cells has been confirmed (Xin et al., 2018, 2020). Figure 4 exhibits the available sources of nano-based carrier materials for producing various agricultural nanoscale inputs. The nanoscale carrier materials can be used as a delivery vehicle for beneficial components within plant, human, or animal systems (Patra et al., 2018). The typical smart delivery mechanism of carrier materials, which delivers effective components at site-specific or targeted release in plant systems, is highly appreciable (Liu et al., 2016).
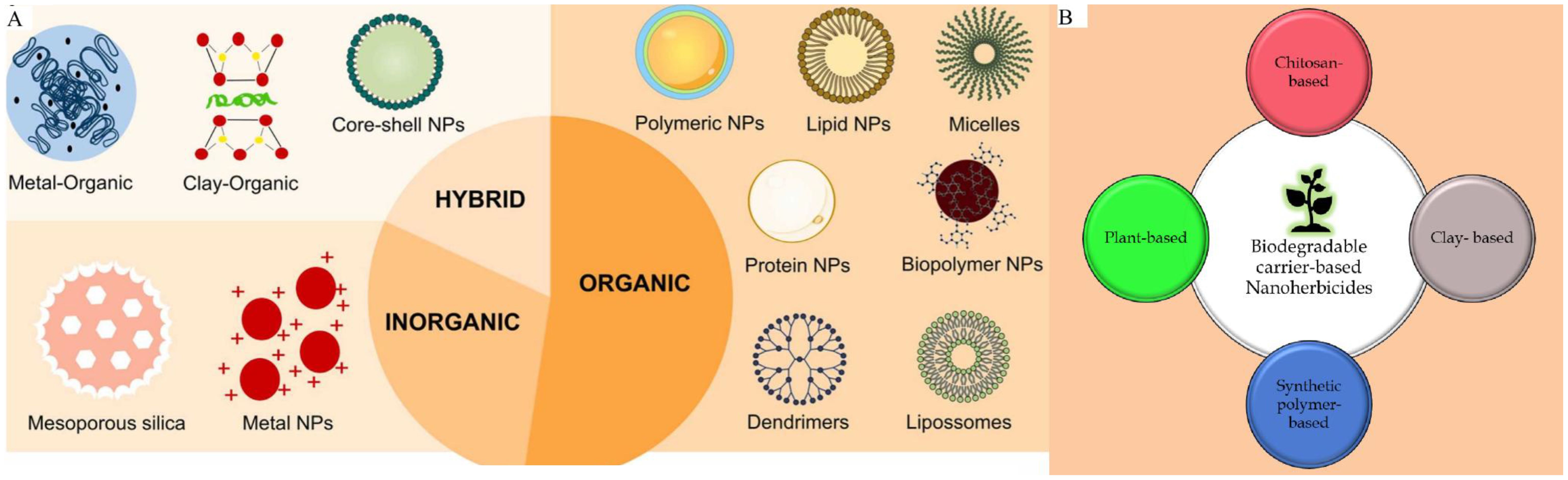
Figure 4. (A) Different sources of nano-enabled carrier materials developed in recent years (Forini et al., 2022). (B) Representing the biodegradable carrier sources for nanoherbicides production.
The amphiphilic nature of the carrier materials results in high affinity with the plant surface, thereby increasing the deposition by decreasing the surface tension of the particles (Shangguan et al., 2024). Thus, biodegradable carrier materials are perceived as a reliable source for producing nanoformulations for agricultural systems by anchoring environmental stability. The information suggested that polymeric nanoparticles promise zero toxicity in plant systems and no negative impact on soil microbiota (Shakiba et al., 2020).
In addition, the biopolymer-encapsulated formulations are less soluble, more stable, and non-volatile (An et al., 2022). Compared with the prodrug delivery system in the medical field, the delivery of nano-based agrochemicals is very complex because of their sinking capacity in the ecosystem, which is highly uncontrollable (Shangguan et al., 2024). Hence, this review focuses on the current application and recent development of biodegradable carrier-based nanoherbicide formulations. The encapsulation provides better uptake and enhanced efficacy of agrochemicals against weed control sustainably. The forthcoming section of the review presents the significant discoveries provided in recent research publications related to formulations of biodegradable carrier-based herbicides. The degradable carrier nanomaterials are discussed in the sequence viz., 1) chitosan-based, 2) plant-based, 3) clay composite-based, and 4) synthetic polymer-based. Table 1 discusses the key properties, such as source, degradability, thermostability, applicability, and advantages and disadvantages of carrier materials, that have been explored in various studies.
3.1 Chitosan based nanoherbicide
Chitosan is an easily available, widely explored biodegradable, biocompatible carrier material derived from chitin in living organisms. Most of the western and eastern countries have accepted the utilization of chitosan as a nutritional supplement and edible additive in the food industries (MaChado et al., 2022). In addition, chitosan has a variety of applications, including coating fertilizers and seeds, as well as frost protection (Khan et al., 2023).
Chitosan is a naturally available source obtained from chitin deacetylation and has free amino groups, which act as a chelating agent (Itodo, 2019). Chitosan is a cost-effective and highly permeable NP that can be used for the development of carrier materials for various pesticides, fertilizers, and genetic materials in agriculture (Choudhary et al., 2019; Xin et al., 2020). Additionally, chitosan has the properties of stimulating defense systems and growth promotion in plant administration (Xin et al., 2020).
In a recent study, chitosan-based mesosulfuron methyl and mesosulfuran methyl + florasulam + MCPA isooctyl herbicides were coated successfully. For dose optimization, nanoformulations were applied at the 3-4 leaf stage in a completely randomized block design that includes seven treatments with a pot size of 20 cm x 16.5 cm, and the pot was maintained with 12 weed seedlings. The herbicide formulation sprayed on the weed population based on each treatment. After two weeks, results show 100% weed control recorded in treatment D2 (nano herbicides at the recommended dose of regular herbicide). In addition, treatment D3 (5-fold lower dose) was recorded with minimum chlorophyll content (5.57%), plant height (2.35cm), fresh biomass (1.08 g), and dry biomass (0.33 g) (Khan et al., 2023).
In another invitro study, the L-carvone-derived 4-methyl-1,2,4-triazole-thioether complexed with nano chitosan. The L-carvone-derived chitosan derivative has been developed with high drug loading capacity and sustainable release of chemical compounds. The introduction of rigid, bulky, and hydrophobic L-carvone moiety with chitosan improves the compatibility and dispersibility of the 5a compound and enables a multi-stage release mechanism. The compounds have been declared targeted as transketolase (TK)-inhibitors, an enzyme present only in plants involved in the oxidative pentose phosphate pathway and the Calvin cycle. Initially, the compounds 5a-5u were tested in the weed species Echinochloa crusgulli L. and Brassica campestris at 10 μg mL−1 and 100 μg mL−1, respectively. Contrastingly, all the compounds (5a-5u) significantly inhibit the growth of dicots (Brassica campestris) but are inferior in controlling monocot weeds (Echinochloa crusgulli L.). The L-carvone-derived nanochitosan facilitates the adsorption of 5a compounds through its loose and porous microstructure. The release of 5a from the chitosan complex gradually increased after exposure to an aqueous medium, with slow dissolving and disintegration of carriers. The 5a (R = C6H5) compound derived from L-carvone-derived 4-methyl-1,2,4-triazole-thioether shows better weed inhibition in the preliminary study than commercial flumioxazin herbicide. The 5a compound was potentially complexed with chitosan compounds such as 7b/5a, 7c/5a, and 7d/5a. The pot experiment revealed that 7b/5a and 7d/5a at the concentration of 100 μg mL−1 controlled weed emergence significantly, which is on par with the result of the 5a compound alone. Furthermore, the Arabidopsis thaliana transketolase (At TK) structure was created by homology modeling and used as a receptor for molecular docking. The Surflex-Dock module of SYBYL X-2.1 has been used to identify the target-site inhibition using molecular docking simulation. Finally, the 5a compound was selected as a representative, and it interacts with receptors and embeds at an active target site of At TK, the protein-ligand complex indicates the binding affinity (Li et al., 2023).
Further, Ghaderpoori et al. and Rashidipour et al. prepared different chitosan-based composite nano herbicide formulations in their respective studies, which had paraquat (PQ) AI molecules and tested them on mustard (Brassica spp) and corn plants (Zea mays). Ghaderpoori et al. revealed that free PQ and chitosan-loaded PQ cause necrosis and fading of leaves in both plants within 48 h. Additionally, the dry weight of corn plants exposed to chitosan composite (sodium tripolyphosphate/xanthan/PQ) was higher than that of free PQ and commercial PQ. In contrast to the corn plants, the mustard plant resulted in less dry weight when exposed to chitosan-loaded PQ over the other two formulations (Ghaderpoori et al., 2020). This implies that encapsulated formulation effectively controls the weed species rather than non-target crops.
Rashidipour et al. reported that composite nano PQ formulation (pectin/chitosan/tripolyphosphate) significantly enhanced herbicidal activity on mustard plants and lower effect on maize plants due to the presence of P-450S glutathione-S-transferase enzyme. The release kinetics was tested in a laboratory condition, where approximately 75% of PQ molecules were discharged after 360 minutes. The nanocomposite formulation shows reduced cytotoxicity in human lung cells (A549 and KB cells) and mutagenetic effects on Salmonella typhimurium and A. nidulans (Rashidipour et al., 2019). Some more studies also successfully engaged chitosan NPs and herbicide molecules, which have been furnished for better understanding in Table 2.
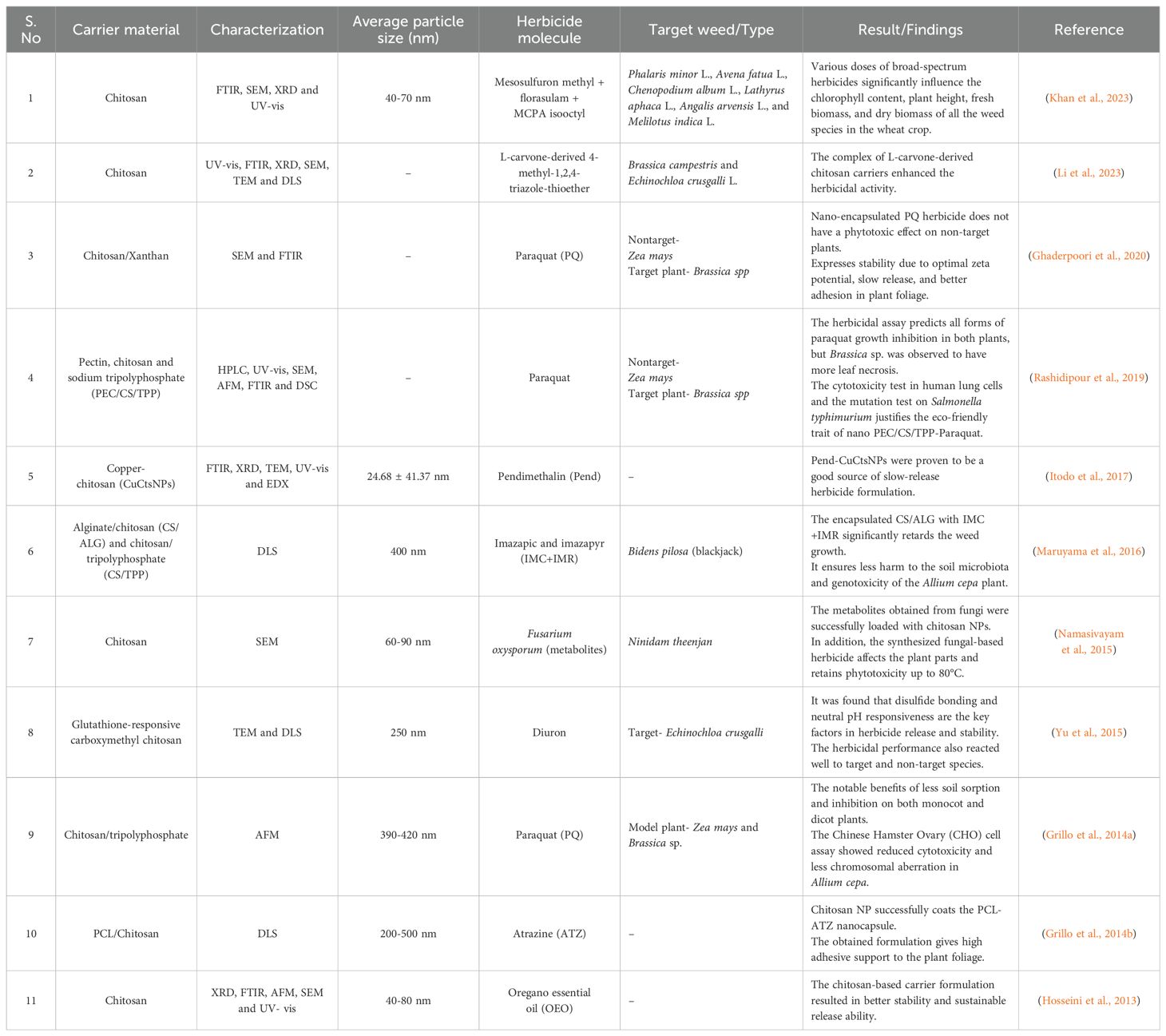
Table 2. Examples of chitosan-based nanoherbicide formulation and their recent findings for weed control.
3.2 Plant-based nanoherbicide
Plant-based natural polymers such as zein, pectin, and lignin, which are non-toxic and biodegradable, can successfully encapsulate herbicide (Qu et al., 2021). Lignin is an abundantly available biopolymer and is easily converted into humus after decomposition under natural conditions (Rahman et al., 2013). Cyclodextrins are identified as carrier materials for host AI molecules in agriculture. The physiochemical properties, lower cost, easy availability, and nontoxicity to nontarget organisms emanate from its use as a carrier material (Rodríguez et al., 2022; Shangguan et al., 2024).
Carvalho et al. successfully coated Atrazine (ATZ) with Zein NPs (ZNP) and demonstrated a greenhouse study to analyze the pre-emergence herbicidal activity of the synthesized nanoformulation. The experiment was designed based on 7 treatments and 5 replications, which evaluated herbicidal activity, soil retention, and mobility with B. juncea as the target and Zea mays as the non-target plant. The results revealed that the preemergence application of an 80-fold lesser dose of ZNP-ATZ resulted in 100% mortality in B. junceae, as ZNP-ATZ has not shown any mortality or herbicidal activity on nonselective maize plants. In addition, (93.34 ± 3.42%) free ATZ and (94.02 ± 1.77%) ZNP-ATZ were retained in the topsoil within 0-4 cm depth. The confocal microscopy results confirmed the accumulation of ZNP-ATZ on B. junceae through the root surface rather than leaves (Carvalho et al., 2023). The results reiterate the significance of ZNP-ATZ based on herbicidal activity, selectivity, retention, and accumulation.
Encapsulated essential oil (EO) with polymer matrices acts as an herbicide formulation for controlling weed species (Campos et al., 2023). In a study, red root amaranth (Amaranthus retroflexus) and radish (Rhaphanus sativus) were treated with starch-encapsulated rosemary essential oil (Rosmarinus officinalis L.) as a pre-emergent herbicide under controlled conditions. When the encapsulated formulation was applied at a rate of 5 g/kg soil, it drastically reduced the germination percentages in amaranth and radish by 91.2% and 84%, respectively. In addition, both weeds have been reduced by 51% and 45% in leaf area and by 46% and 54% in root length. Additionally, Alipour et al. (2019) observed a marginal effect of 33% on chlorophyll content in amaranth and 36% in radish.
A similar study covered nano-formulated essential oil (NEO) with naturally available carbohydrate and protein polymers (Arabic gum-gelatin, apple pectin, and gelatin). The application of NEO (3 ml/L) was compared with the traditional chemical metribuzin (1.75 g/L). The results proved that the pre-emergence application of NEO significantly suppressed weed growth under in vitro conditions in tomato plant circumstances (Taban et al., 2021). Later, in 2020, Taban et al. further investigated the encapsulation of Satureja hortensis essential oil (EO) with Persian gum as wall material. The formulation resulted in better stability after 42 days and suppressed Amaranthus retroflexus weed growth at the optimized concentration of 15 mL/L of water. Aside from that, the NEO limits the physiological and physiochemical activities (including photosynthesis pigments, phenol, flavonoid, starch, fresh weight, electrolyte leakage, and lipid peroxidation) in the amaranth plant (Taban et al., 2020).
Zein is a naturally available plant protein obtained from Zea mays and possesses various properties such as biocompatibility, biodegradability, and hydrophobic surface, which have been highly appreciated for using zein as a biopolymer. For example, Heydari et al. successfully loaded tribenuron-methyl (TM) herbicide molecules in zein nanoparticles (ZNP) and tested their performance against C. arvensis, a prevalent broad-leaf weed in the wheat ecosystem. When compared to the commercial formulation of TM, the results confirmed that half of the dose of the TMZNP formulation greatly turns down the percentage of plant height (77%), dry weight (53%), enzyme activity (82%), and acetolactate synthase (ALS) enzyme activity (82%), as compared to the untreated plot (Heydari et al., 2021). Furthermore, many other studies utilize plant-based nanoparticles as carrier materials, and we have tabulated a few such research findings in Table 3.
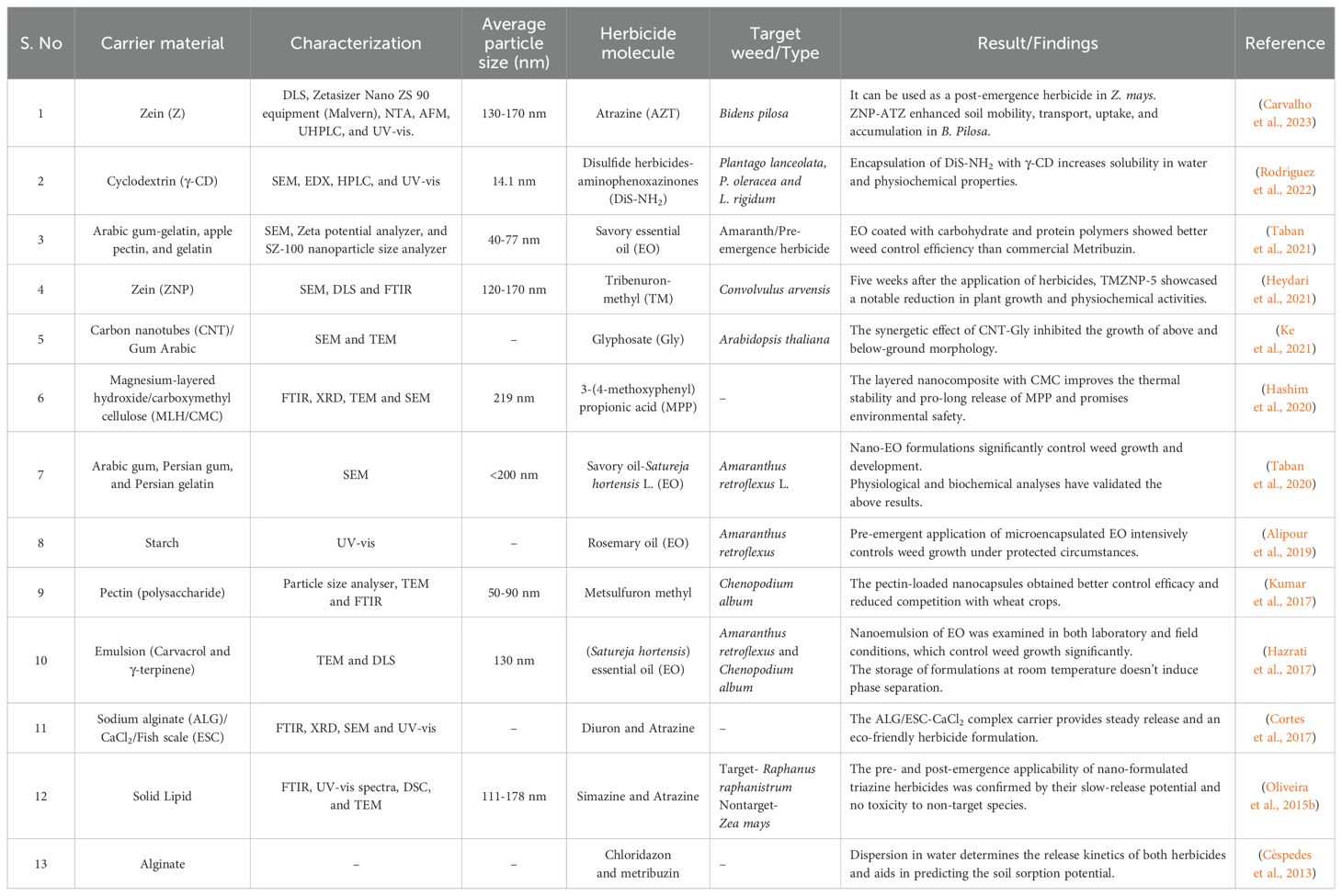
Table 3. Examples of plant-based nanoherbicide formulation and their recent findings for weed control.
3.3 Clay composite-based nanoherbicide
Naturally available clays can function as carrier materials for pesticide delivery. Of course, clays can be used to produce commercial nanoherbicide formulations since clays possess ample characteristics (including being eco-friendly, non-toxic, extensively available, and economically feasible). A study reported that montmorillonite-smectite clay minerals can carry herbicide molecules in their layered structure. Smectite clay has the potential for swelling properties, which regulate the release pattern of the herbicide molecules. Montmorillonite-based 2,4-D nanoformulation obtained better desorption and adsorption capacities at low pH under controlled conditions (Paul et al., 2019).
For instance, thermodynamic and kinetic methods modify 2,4-D herbicide with montmorillonite nanoclay. The results provide scope for using 2,4-D modified nanoformulation as a preferable slow-release herbicide against weed growth (Natarelli et al., 2019). Clay modification as organoclay is achieved by replacing inorganic exchangeable cations with organic quaternary ammonium in the interlayers. Consequently, the change in action from a hydrophilic surface to a hydrophobic surface enhances the herbicide loading capacity of AIs between the layered structures of clays (Paul et al., 2019). In addition, clay-polymer composites ensure the controlled release of herbicide molecules while pretending to be an eco-friendly source of carrier material (Nuruzzaman et al., 2019).
Jia et al. reported the loading of glufosinate ammonium (Glu) in the lumen of halloysite nanotubes (HNTs) and incorporated in the biodegradable poly (butylene adipate-co-terephthalate) (PBAT) and Poly (lactic acid) (PLA) mulch films by blow molding and melt blending techniques. The fabricated composite films have been demonstrated in a pot culture experiment with bristlegrass as a model weed. The overall result shows that PPHG films (PBAT/Poly (lactic acid)/HNT/Glu) significantly controlled the fracture on composite films by up to 51%, reduced water vapor by up to 68%, and reduced the weed incidence by slow release and longer shelf life of the mulch films (Jia et al., 2023).
Garnetto et al. reported that K10-montmorillonite was loaded with commercial dicamba herbicide and coated with carboxymethyl cellulose (CMC), a biodegradable polymer. The K10-CMC-based herbicide formulation was applied to Solanum nigrum and Amaranthus retroflexus in pot culture under greenhouse conditions. The result concluded that the nanoformulation greatly restricts the volatilization loss and mobility of herbicides in the subsoil. Furthermore, the K10-CMC herbicide formulation obtained lesser biomass on both S. nigrum and A. retroflexus, with a minimal requirement of 100 g AI/ha and 50 g AI/ha, respectively (Granetto et al., 2022).
In a comparative study, layered double hydroxide (LDH) anionic clay, and Cloisite10A (Clo 10A) cationic organoclay were used as carriers for imazamox (IMZ) herbicide. The herbicide molecules are partially stuffed between the internal layers and stocked on the surface of the clay nanoparticles. Both formulations resulted in a maximum water release of 73 to 98%, after the immediate release of 67 to 93%, which is comparatively lower than the free IMZ (>98%) herbicide release. Additionally, the soil leaching was reduced by 10 to 35% when compared to commercial formulations. The nanoIMZ herbicide molecules are present in the topsoil layer in the range of 5-15 cm, which can be assumed to be a rhizosphere zone. Furthermore, the clay nanoformulation significantly suppressed the growth of the aerial and root systems of B. nigra weed species (Khatem et al., 2019). Table 4 displays some recently reported research findings regarding synthetic polymer-based nanoherbicide.
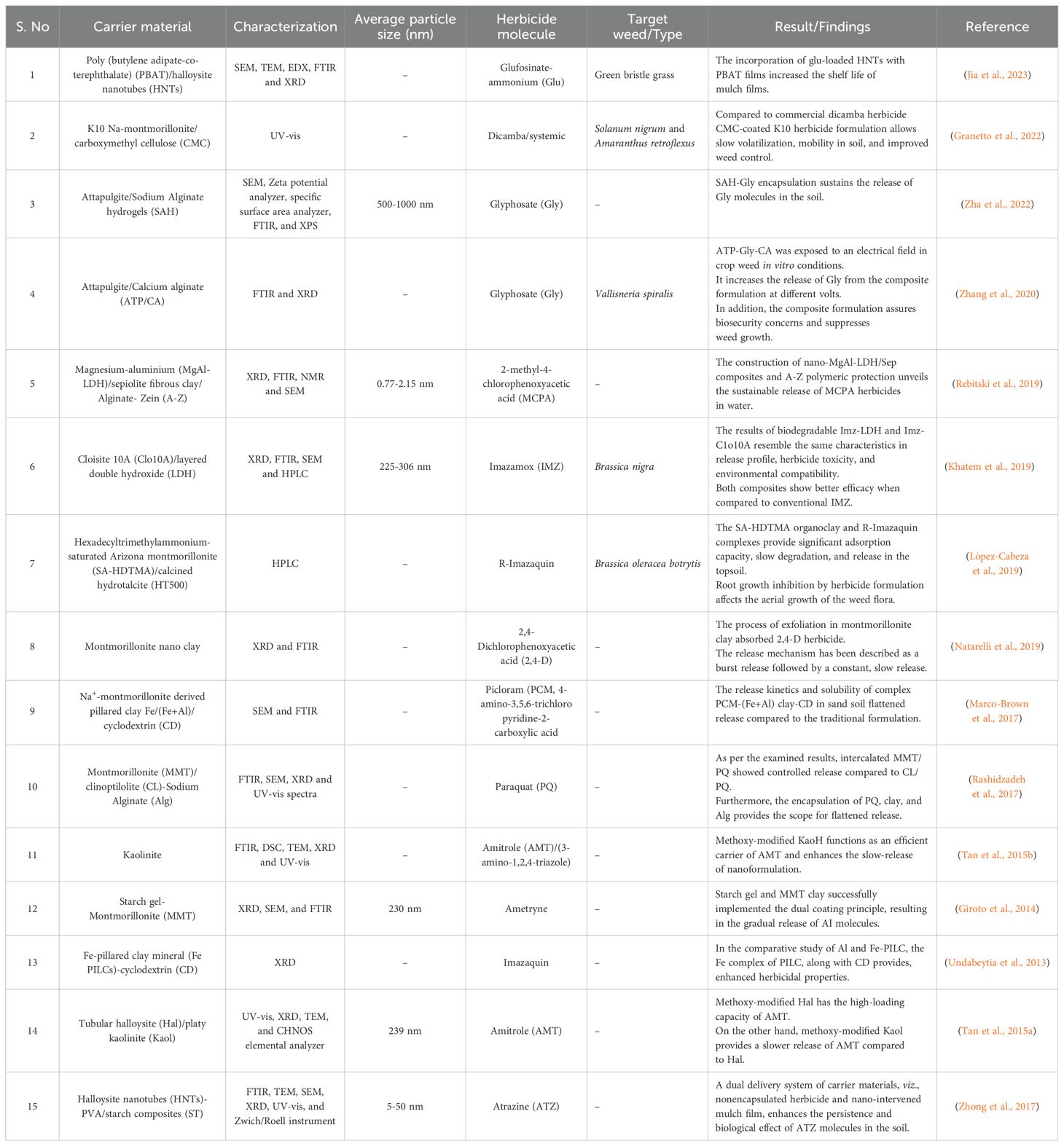
Table 4. Examples of clay-based nanoherbicide formulation and their recent findings for weed control.
3.4 Synthetic polymer-based nanoherbicide
FDA has approved synthetic polymers like PLGA and PLA for human drug delivery, which indicates their minimal environmental risks (Shakiba et al., 2020). The surface modification of AI molecules with polymer NPs retards the washing off, aggregation, and deposition of NPs, subsequently increasing the uptake and transportation ability of the NPs. The polymeric coating of NPs has better adhesion to the plant surface because of its hydrophobic surface (Su et al., 2019). Moreover, surface-modified NPs exhibited greater penetration potential on the waxy leaf surface (Yang et al., 2015).
Moore et al. reported that PCL-coated nanoatrazine (ATZ) was highly reactive and harmful to human cells. To justify the result, Moore et al. experimented with nano-ATZ, PCL capsules, and ATZ alone in human lung cells (immortalized alveolar type 1-like epithelial cell model: TT1 cells). Nano-ATZ significantly influenced lactate dehydrogenase and cytoplasmic accumulation and co-localized in the Golgi structure, which was confirmed by using a confocal microscope with fluorescent labeling in the herbicide formulations (Moore et al., 2022). Most studies indicate that applying nano-formulated herbicides may reduce environmental toxicity and increase herbicidal activity. So, moderation in the dosage of nanoformulation is highly desired in agriculture.
De Sousa et al. encapsulated atrazine with PCL NPs, which has been tested as a post-emergence herbicide on Alternanthera tenella (Colla plants), at various doses (200, 500, 1000, and 2000 g a.i. ha-1). According to the meta-analysis of previous research findings with the study on A. tenella, the nano-ATZ formulation inhibits photosystem II more efficiently than the commercial formulation. In addition, while applying PCL-ATZ, the response of A. tenella is different from that of other weed species, where the nano-ATZ obtained more control efficiency than the commercial formulation until 48 hours after the application. The meta-analysis shows that the outcome with A. tenella is different from other weed types when it comes to how it responds to nano-ATZ formulation (de Sousa et al., 2022).
In a similar study, Wu et al. compared PCL-coated nanoatrazine with commercial atrazine. They tested both formulations during short-, medium-, and long-term exposure at different concentrations. Lettuce has been a widely used plant for toxicity observation. In this study, the plants were exposed to ATZ and nano-ATZ at nominal concentrations of 0.3, 1.5, and 3 mg per kg of soil. The spectrophotometer readings were observed for all the samples, which indicates the significant influence of nano-ATZ over ATZ in terms of chlorophyll content a and b, carotenoids, hydrogen peroxide production, lipid peroxidation, total protein, and antioxidant enzyme activity. Overall, the ATZ formulation controls the weed growth in the initial phase, but the nano-ATZ shows potential on a long-term basis. Finally, the engagement of both formulations in their respective treatments confirmed nutrient displacement in the soil (Wu et al., 2021).
Takeshita et al. developed an herbicide formulation based on the PCL-metribuzin (MTZ) combination. MTZ can easily accumulate due to the rapid desorption of AI molecules in water. However, the polymer-coated MTZ hasn’t shown greater mobility, subsequently lowering the environmental hazards. In addition, the nano-MTZ suppresses Ipomoea grandifolia weed species by inhibiting PSII activity with a lower quantity of herbicide formulation (48 g a.i. ha-1) (Takeshita et al., 2022b).
In another study, Schnoor et al. successfully loaded atrazine molecules with poly (lactic-co-glycolic acid) (PLGA). PLGA is a reliable source of polymeric nanoparticles with biodegradable properties, particularly because it will undergo degradation after unloading AIs from the polymer matrix. The AIs were released up to 50% after 72 hours of application in the soil. Furthermore, Schnoor et al. found that the chemical interaction of atrazine and PLGA nanoparticles was justified by the formation of hydrogen bonds at 1.9 Å. The lab study on potato plants with nano-formulation exhibited significant growth retardation in root length, shoot length, fresh weight, dry weight, stem length, and reduced number of leaves (Schnoor et al., 2018). In addition, several synthetic polymer-based herbicide applications and their significance are listed in Table 5.
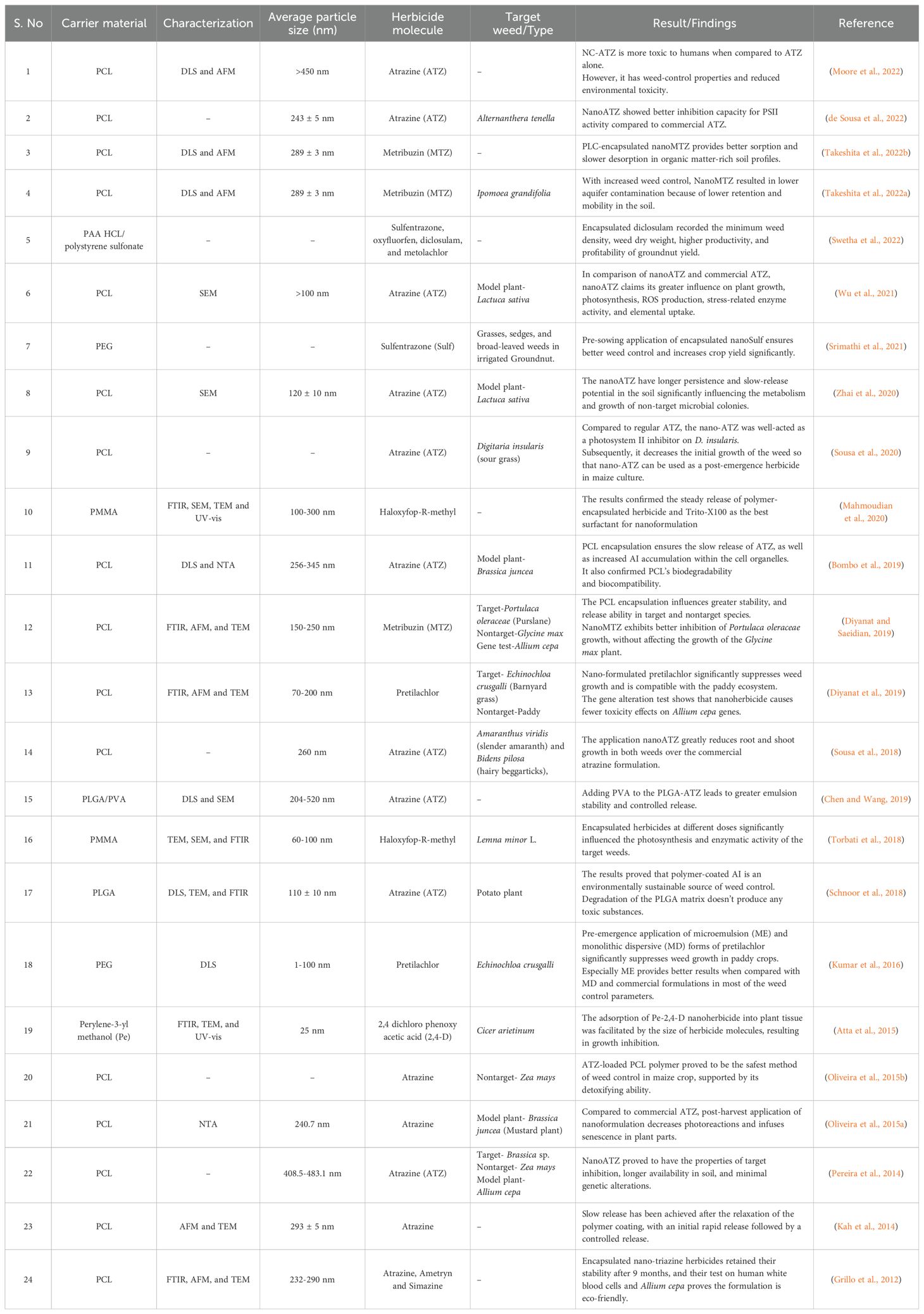
Table 5. Examples of synthetic polymer-based nanoherbicide formulation and their recent findings for weed control.
4 Uptake, transportation, and fate of nanoherbicide in plant systems
Research publications have reported fewer experimental results regarding nanoparticle absorption, mobility, and cellular-level modifications in the plant system. Furthermore, the extent of uptake and mobility of NPs have been determined by the plant canopy conditions and the properties of NPs, including size, shape, and charge, along with the morphology of the plant (Singh et al., 2023). The vascular structure transports the NPs to other parts after they penetrate a specific area through the leaf or root surface (Judy et al., 2012; Lead et al., 2018). Figure 5 illustrates the movement of herbicide molecules from the leaf surface to the vascular tissue.
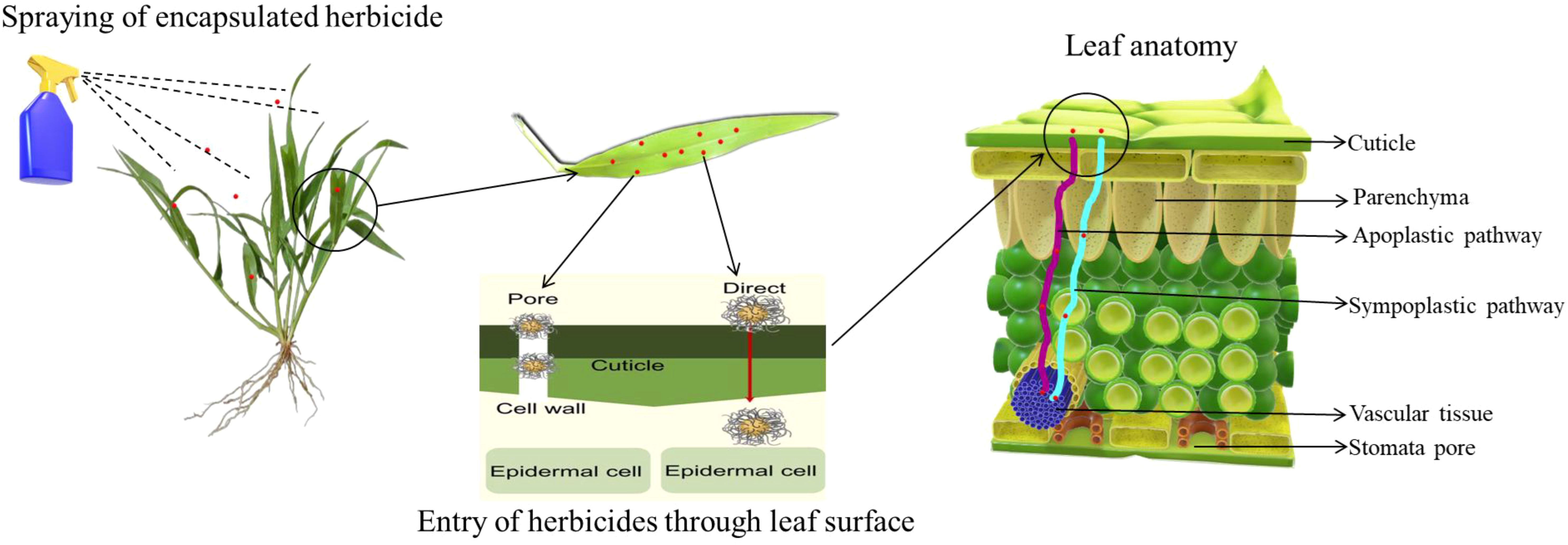
Figure 5. Transport of nanoherbicide from the leaf surface to the vascular tissue (Modified source: Forini et al., 2022).
According to Rajput et al. (2020) and Tripathi et al. (2017), nanoparticles can be translocated from root to aerial parts through xylem (soil application) and aerial parts to root through phloem (foliar application). The foliar application showed better uptake efficiency than the soil application in the plant system. The uptake of NPs in plants takes place in three steps, viz., 1) deposition of NP on the surface of the root, leaf, or stem, 2) NP penetration via epidermis and cuticle, and 3) NP translocation within the plant system. Plant species have similar phenotypical features in their leaves, with distinguished arrangements in an epidermis having stomata, mesophyll, and vascular tissue based on the environmental habitat and plant species’ evolution over the years. In addition, the cuticular pathway acts as a secondary barrier, which is resistant to allowing any particle larger than 5 nm (Su et al., 2019; Ali et al., 2021).
Stomatal opening size ranges on the micrometer scale, which is another way for the NPs to penetrate the leaf surface; it occupies more than 5% of the total leaf surface area. But the opening of stomata differs in each plant in response to external factors like carbon dioxide concentration, RH, light intensity, and temperature (Su et al., 2019). Minuscule unenclosed parts in the plant leaf such as stomata, hydathodes, cuticles, and trichomes, facilitate the entry of NPs into the plant cell (Singh et al., 2023). On the other hand, dissolved NPs in water can reach the root indirectly by capillary movement and enter the root tissue through osmotic pressure (Chen, 2018). The roots are another mode of entry for NPs inside the plant system, where a higher root surface area results in better uptake potential for NPs. In addition, the root system’s micromorphology consists of an endodermis, pericycle, cortex (solid and lacunate), and vascular bundle in the center. However, the solid cortex and endodermal casparian strips make it hard for NPs to move through the apoplastic because of their compacted intercellular spaces (Su et al., 2019).
It is worth mentioning that variations in the structural morphology of leaves and roots determine the penetration and restriction of NPs. The NPs can enter the xylem vessels through the symplastic or apoplastic pathway and then accumulate in the cellular and subcellular organelles (Tripathi et al., 2017) through trailing transportation (root tissues, endocytosis, protein carrier, plasmodesmata, or through pore formation) (Pérez-de-Luque, 2017). Once NPs reach the xylem, the upward movement of NPs takes place, whereas the downward movement of NPs takes place through the phloem cells, respectively (Su et al., 2019). Extremely small-sized NPs can penetrate the cell organelles or membranes of plants and be absorbed directly with their diffusive capability (Xin et al., 2020).
The effects of nanoherbicides on lipid peroxidation, chlorophyll content, protein content (Pontes et al., 2021; Wu et al., 2021; Oliveira-Pinto et al., 2022), electrolyte leakage, chromosomal aberration (Diyanat et al., 2019), reactive oxygen species (ROS) production (Pontes et al., 2021), carbon dioxide assimilation rate (Takeshita et al., 2021), and photosystem I and II yields (Pontes et al., 2021) have been investigated (Forini et al., 2022). The lipid envelope exchange penetration (LEEP) property of chitosan NPs eases penetration into the cell organelles through the nanoscale size and surface charge of the NPs (MaChado et al., 2022). It is emphasized that the hydrophobic-hydrophobic interaction of nonpolar molecules found on plant surfaces and composite nanoformulation promotes the effective adhesion of nanopesticides in plant foliage (Hao et al., 2020; Forini et al., 2022).
The soil application of NPs translocates the AI molecules to other parts of the plant with the help of vascular tissues (MaChado et al., 2022). The secretion of negatively charged substances from the root surface, such as mucus or organic acids resulted in the absorption of positively charged chemical inputs (NPs) from the soil (Lv et al., 2019). The intracellular movement, or protoplast pathway, is the primary movement of NPs and is influenced by plasmodesmata, which have a diameter of 2-20 nm (Kaphle et al., 2018). To remove the barriers to understanding the mobility and uptake of nano-pesticides, labeling of polymeric NPs with fluorescent markers would act as a tracker system for the indication of the translocation pathway (Proença et al., 2022; Shakiba et al., 2020; Takeshita et al., 2023).
The permeability of bioactive NPs into plant cell walls has not been characterized, it also depends on the size and hydrophobicity of the NP (Avellan et al., 2019). NPs with amphiphilic properties and about 40 nm in size can move through cells more easily than NPs that are hydrophilic and large-sized (Santana et al., 2020). To confirm the translocation ability and targeted delivery of NPs in plant organelles, hydrophilic quantum dots (QD) coated with amphiphilic β-cyclodextrin NPs were used to facilitate translocation through leaf cell wall pores. The modified QDs are injected into the Arabidopsis thaliana leaf in 3-4 weeks, successfully delivered by peptide-guided targeted delivery at chloroplast cells. The fluorescence intensity of QDs in chloroplast cells was imaged through confocal microscopy (Santana et al., 2020). Once again, the fluorescent labeling of NPs shows successful tracking of uptake and translocation pathways, which is supported by the results of foliar-applied PCL NPs (Bombo et al., 2019) and root-applied Zein NPs (Prasad et al., 2017). Isothiocyanate and rhodamine are widely used fluorescent probes for nano-constructed polymeric NPs (Proença et al., 2022). The poly(ϵ-caprolactone) (PCL) nanocapsules containing atrazine (ATZ) herbicide successfully coated with chitosan (CS) polymer. The PCL/CS+ATZ nanoformulation inhibits the PSII system, and the relative electron transport rate significantly controls the growth of the Bidens pilosa plant. Additionally, the endogenous atrazine quantity at the plant tissues’ leaves, stems, and roots was estimated using Carvalho et al.’s methodology with slight changes (Carvalho et al., 2023). The PCL/CS+ATZ and commercial ATZ quantity on plant tissues fluctuated according to the exposure duration viz., 2, 4, 8, 12, 24, 36, 48, and 72 hours. The root contains the same amount of free ATZ and PCL/CS+ATZ after 72 hours @ 17 ± 2 μg/g of root tissue. Whereas PCL/CS+ATZ recorded the highest quantity after 24 hours @ 14 ± 2 μg/g of stem tissue, it was comparatively lesser than free ATZ quantity. The leaf tissues are recorded as having the highest accumulation of both formulations compared to the quantity of stem and root tissues. After 72 hours, leaf tissues contain 25 ± 2 μg/g of ATZ released from PCL/CS+ATZ formulation (Sousa et al., 2024).
5 Future perspective and development
To balance the food crisis in the context of the burgeoning population, climate fluctuation, water shortage, and reduction in arable land, the need for cutting-edge technology is urgent to ensure global food security (Xin et al., 2020). The use of NPs in agriculture is inevitable, although it has several advantages and disadvantages. It was anticipated that NPs could reduce production-impairing chemicals that pose a severe risk to human health and the soil-plant system (Rajput et al., 2020). The nanoencapsulated herbicides are a good choice for achieving sustainable agriculture, reducing the dependence on conventional herbicides and toxicity. The properties of traditional pesticides would have to be masked by synthesizing polymeric nanoformulations with enhanced colloidal stability and lesser polydispersity, along with successful entrapping or encapsulating of biologically active chemical compounds (Shakiba et al., 2020). However, the disintegration of AI molecules from polymer matrix has been observed in certain instances. The nano-encapsulation with organic polymers has encountered difficulties, including formulation instability and the formation of acid monomers due to polymer degradation (Abdollahdokht et al., 2022). Thus, the nanoformulation should be highly stable 1) until it reaches destiny (target inhibition), 2) as compared to commercial formulations, and 3) without compromising environmental safety.
Conventional pesticide formulations are mixtures of inert substances like alcohol, ketones, and benzenes with active chemical compounds. Due to their extreme toxicity and potential for severe poisoning, all of these substances are dipolar solvents and highly hazardous to human health (Paul et al., 2024). To address this concern, nanoencapsulated biodegradable herbicide formulations should be produced as a much-needed priority. The producers need to pay attention to the selection of polymers and surfactants available with a high surface charge that creates a high electrostatic repulsive force among the particles, which can be confirmed by the zeta potential value (higher or lower than 30 and -30 mV) (Hans and Lowman, 2002; Shakiba et al., 2020). For instance, Zwitterionic surfactants are less hazardous and an excellent choice for environmentally friendly surfactants since they possess cationic and anionic characteristics (Paul et al., 2024). Integrating Zwitterionic surfactants may also enhance the formulation stability, yielding a longer shelf life.
Surface-engineered biodegradable NPs may change their surface potential and electrostatic interaction due to the adhesion of NPs inside the plant system. We can clearly understand that the stability of NPs inside plant systems highly depends on the solute composition of the plant system (Rodrigues et al., 2017). It may result in the aggregation of NPs, which causes poor transportation of NPs in the plant system. The importance of surface coatings is receiving more attention, and further efforts are needed to produce a stable wall matrix until it reaches the target site.
A survey of recent articles on nano-encapsulated biodegradable herbicides shows that the size of NPs ranges more than 100-1000 nm. The plant uptake of NPs is well documented; it indicates superior translocation occurred when the particles are at 1-100 nm nanoscale. Nano-sized particles are known for better uptake and transportation in the plant system. So, future studies on nanomaterials should focus more on particle sizes that fall under the nanoscale range.
Few studies have been reported on the nanoencapsulation of essential oils (EO), but impressive results were provided in controlling weeds. The encapsulation has improved herbicidal capabilities with an improvement in viability, persistence, and targeted delivery against weed species (Campos et al., 2023). Essential oils are derivatives of plant materials, providing an option for producing bioactive herbicides. Encapsulating EO with carrier material is a better strategy to widen the production of EO-based herbicide formulations. However, nanotechnology has been considered a novel technology in various fields. Nevertheless, risk assessment methods must be developed for future endeavors relying on nanotechnology. Thus, future research must be conducted on understudied materials to produce readily biodegradable and non-toxic products.
Few studies have investigated the quantification and translocation of herbicide molecules in plant anatomy. Therefore, more focus should be placed on framing the quantification methodology to estimate the accumulation of bioactive compounds in plant tissues. However, injudicious usage of nano-herbicides also causes lethal environmental and human hazards. The researchers have to focus on dose optimization and the persistence of herbicide molecules. The cohort study on the organisms involved could assist in gaining more knowledge on the fate and effect of NPs.
Even though herbicide encapsulation shows enormous benefits, slow release is the top priority. In weed control, timely germination inhibition and growth restriction must be done without fail; otherwise, crop-weed competition will lead to yield loss. The encapsulated formulation may limit the release of AIs from the core-shell matrix at the critical weed growth period. Likewise, instead of solving one problem, we should not create another problem by encapsulation or loading the bioactive compounds. The studies experimented with the release kinetics were based on the invitro conditions, where factors like RH, temperature, moisture, and solar radiation are uncontrollable in field conditions. So, the nano-encapsulated herbicides must be trailed under different environmental conditions, which can direct optimization of the formulation according to adverse conditions.
6 Conclusion
The current trends in agriculture are heading towards sustainable agriculture. “Green herbicides” with zero toxicity are highly desirable in modern agriculture. In this context, the research community highly recommends nano-enabled biodegradable herbicides to ensure global food security. Furthermore, the study highlights the significance of nano herbicide application for weed control. This review will convey the benefits and limitations of nanoencapsulated herbicide application. Consequently, we have formulated the immediate need for attention to nanoherbicide application. The unfathomable benefits of using nano-herbicides enhance the interest in unveiling the new horizons of nanotechnology. The studies have to focus on the mode of action of herbicide formulations. The studies should consider the following suggestions: 1) commercial production is obsolete because of the need for open field trails rather than laboratory or controlled conditions; 2) studies on risk assessment and tracking of NPs were subjected to a lower-than-anticipated count; 3) selection of compatible carrier materials; 4) focusing more on different crop ecosystem to analyze nontarget effects. Lawmakers, research communities, and manufacturing industries should focus more on the abovementioned limitations. This review will bring clarity and confidence about toxic-free herbicide production and application in the agricultural ecosystem. In addition, the focal point of this review is the biodegradable carrier-based nanoherbicides, which gives insights to researchers, manufacturers, and students on how to take this topic to further advancement.
Author contributions
RJ: Conceptualization, Investigation, Methodology, Resources, Writing – original draft, Writing – review & editing. PR: Conceptualization, Investigation, Resources, Supervision, Validation, Writing – review & editing.
Funding
The author(s) declare financial support was received for the research, authorship, and/or publication of this article. This research was funded by Vellore Institute of Technology, Vellore, India.
Acknowledgments
We thank VIT University for providing technical support throughout the manuscript preparation.
Conflict of interest
The authors declare that the research was conducted in the absence of any commercial or financial relationships that could be construed as a potential conflict of interest.
Publisher’s note
All claims expressed in this article are solely those of the authors and do not necessarily represent those of their affiliated organizations, or those of the publisher, the editors and the reviewers. Any product that may be evaluated in this article, or claim that may be made by its manufacturer, is not guaranteed or endorsed by the publisher.
Abbreviations
UV-vis, Ultraviolet-visible spectra; ATZ, Atrazine; AI, Active ingredient; DLS, Dynamic light scattering; NTA, Nanoparticle tracking analysis; AFM, Atomic force microscopy; UHPLC, Ultra-High Performance Liquid Chromatography; HPLC, High-Performance Liquid Chromatography; NP, Nanoparticles; XRD, X-ray diffraction analysis; TEM, Transmission electron microscopy; SEM, Scanning electron microscopy; EDX, Energy Dispersive X-ray spectroscopy; PBAT, Poly (butylene adipate-co-terephthalate); PCL, Poly(epsilon-caprolactone); PAA HCL, Polyallyl amine hydrochloride; PLGA, Poly (lactic-co-glycolic acid); PLA, Polylactic acid; PVA, Polyvinyl alcohol; PMMA, Poly (methyl methacrylate); XPS, X-ray photoelectron spectroscopy; PEG, Polyethylene glycol; ROS, Reactive Oxygen Species; LDH, Layered Double Hydroxide; NMR, Nuclear Magnetic Resonance spectroscopy; CRM, Controlled release mechanism; FDA, Food and Drug Administration.
References
Abdollahdokht D., Gao Y., Faramarz S., Poustforoosh A., Abbasi M., Asadikaram G., et al. (2022). Conventional agrochemicals towards nano-biopesticides: an overview on recent advances. Chem. Biol. Technol. Agric. 9, 13. doi: 10.1186/s40538-021-00281-0
Ali M., Mir S., Atanase L. I., Kazi M. (2024). Chitosan–PVA–PVP/nano-clay composite: A promising tool for controlled drug delivery. RSC Adv. 14, 15777–15790. doi: 10.1039/D4RA02959C
Ali S., Mehmood A., Khan N. (2021). Uptake, translocation, and consequences of nanomaterials on plant growth and stress adaptation. J. Nanomater. 2021, 6677616. doi: 10.1155/2021/6677616
Alipour M., Saharkhiz M. J., Niakousari M., Damyeh M. S. (2019). Phytotoxicity of encapsulated essential oil of rosemary on germination and morphophysiological features of amaranth and radish seedlings. Sci. Hortic. 243, 131–139. doi: 10.1016/j.scienta.2018.08.023
An C., Sun C., Li N., Huang B., Jiang J., Shen Y., et al. (2022). Nanomaterials and nanotechnology for the delivery of agrochemicals: strategies towards sustainable agriculture. J. Nanobiotechnol. 20, 11. doi: 10.1186/s12951-021-01214-7
Ananikov V. P. (2019). Organic–inorganic hybrid nanomaterials. Nanomaterials 9 (9), 1197. doi: 10.3390/nano9091197
Anarghou H., Malqui H., Ihbour S., Laaroussi M., Essaidi O., Fetoui H., et al. (2024). Impact of glyphosate-based herbicide exposure through maternal milk on offspring’s antioxidant status, neurodevelopment, and behavior. Naunyn-Schmiedeberg's Arch. Pharmacol., 1–19. doi: 10.1007/s00210-024-03035-5
Artusio F., Casà D., Granetto M., Tosco T., Pisano R. (2021). Alginate nanohydrogels as a biocompatible platform for the controlled release of a hydrophilic herbicide. Processes 9 (9), 1641. doi: 10.3390/pr9091641
Atta S., Bera M., Chattopadhyay T., Paul A., Ikbal M., Maiti M. K., et al. (2015). Nano-pesticide formulation based on fluorescent organic photoresponsive nanoparticles: for controlled release of 2, 4-D and real time monitoring of morphological changes induced by 2, 4-D in plant systems. RSC Adv. 5, 86990–86996. doi: 10.1039/C5RA17121K
Avellan A., Yun J., Zhang Y., Spielman-Sun E., Unrine J. M., Thieme J., et al. (2019). Nanoparticle size and coating chemistry control foliar uptake pathways, translocation, and leaf-to-rhizosphere transport in wheat. ACS Nano 13, 5291–5305. doi: 10.1021/acsnano.8b09781
Bamisaye A., Adegoke K. A., Alli Y. A., Bamidele M. O., Idowu M. A., Ogunjinmi O. E. (2023). Recent advances in nanoemulsion for sustainable development of farm-to-fork systems. J. Clean. Prod. 429, 139226. doi: 10.1016/j.jclepro.2023.139226
Bombo A. B., Pereira A. E. S., Lusa M. G., De Medeiros Oliveira E., De Oliveira J. L., Campos E. V. R., et al. (2019). A mechanistic view of interactions of a nanoherbicide with target organism. J. Agric. Food Chem. 67, 4453–4462. doi: 10.1021/acs.jafc.9b00806
Bratovcic A., Hikal W. M., Mehdizadeh M., Al Ahl H. A., Omidi A., Adetunji C. O., et al. (2023). Application of nanotechnology in agroecosystems: nanoparticles for improving agricultural production. Rev. Agric. Sci. 11, 291–309. doi: 10.7831/ras.11.0_291
Brillas E. (2021). Recent development of electrochemical advanced oxidation of herbicides: A review on its application to wastewater treatment and soil remediation. J. Clean. Prod. 290, 125841. doi: 10.1016/j.jclepro.2021.125841
Camara M. C., Campos E. V. R., Monteiro R. A., do Espirito Santo Pereira A., de Freitas Proença P. L., Fraceto L. F. (2019). Development of stimuli-responsive nano-based pesticides: emerging opportunities for agriculture. J. Nanobiotechnol. 17, 1–19. doi: 10.1186/s12951-019-0533-8
Campos E. V., Ratko J., Bidyarani N., Takeshita V., Fraceto L. F. (2023). Nature-based herbicides and micro-/nanotechnology fostering sustainable agriculture. ACS Sustain. Chem. Eng. 11, 9900–9917. doi: 10.1021/acssuschemeng.3c02282
Carvalho L. B., Godoy I. S., Preisler A. C., de Freitas Proença P. L., Saraiva-Santos T., Verri W. A., et al. (2023). Pre-emergence herbicidal efficiency and uptake of atrazine-loaded zein nanoparticles: a sustainable alternative to weed control. Environ. Sci. Nano 10, 1629–1643. doi: 10.1039/d2EN01064J
Céspedes F. F., García S. P., Sánchez M. V., Pérez M. F. (2013). Bentonite and anthracite in alginate-based controlled release formulations to reduce leaching of chloridazon and metribuzin in a calcareous soil. Chemosphere 92, 918–924. doi: 10.1016/j.chemosphere.2013.03.001
Chang Y., Harmon P. F., Treadwell D. D., Carrillo D., Sarkhosh A., Brecht J. K. (2022). Biocontrol potential of essential oils in organic horticulture systems: From farm to fork. Front. Nutr. 8. doi: 10.3389/fnut.2021.805138
Chen X. T., Wang T. (2019). Preparation and characterization of atrazine-loaded biodegradable PLGA nanospheres. J. Integr. Agric. 18, 1035–1041. doi: 10.1016/S2095-3119(19)62613-4
Chen H. (2018). Metal based nanoparticles in agricultural system: behavior, transport, and interaction with plants. Chem. Speciat. Bioavailab 30(1), 123–134. doi: 10.1080/09542299.2018.1520050
Choudhary R. C., Kumari S., Kumaraswamy R. V., Sharma G., Kumar A., Budhwar S., et al. (2019). Chitosan nanomaterials for smart delivery of bioactive compounds in agriculture. Nanoscale Eng. Agric. Manage., 124–139. Available at: https://www.researchgate.net/publication/331044154.
Cortes G. K. D. R., Vieira E. F., Oliveira D. M., Cestari A. R., Maria de Lara P. D. M. (2017). A new environmentally safe formulation and of low cost for prolonged release system of atrazine and diuron. Orbital Electron. J. Chem., 181–187. doi: 10.17807/orbital.v9i3.994
de Oliveira J. L., Campos E. V. R., Goncalves da Silva C. M., Pasquoto T., Lima R., Fraceto L. F. (2015). Solid lipid nanoparticles co-loaded with simazine and atrazine: preparation, characterization, and evaluation of herbicidal activity. J. Agric. Food Chem. 63, 422–432. doi: 10.1021/jf5059045
de Sousa B. T., Santo Pereira A. D. E., Fraceto L. F., Oliveira H. C., Dalazen G. (2022). Post-emergence herbicidal activity of nanoatrazine against Alternanthera tenella Colla plants compared to other weed species. Heliyon 8 (7). doi: 10.1016/j.heliyon.2022.e09902
Diyanat M., Saeidian H. (2019). The metribuzin herbicide in polycaprolactone nanocapsules shows less plant chromosome aberration than non-encapsulated metribuzin. Environ. Chem. Lett. 17, 1881–1888. doi: 10.1007/s10311-019-00912-x
Diyanat M., Saeidian H., Baziar S., Mirjafary Z. (2019). Preparation and characterization of polycaprolactone nanocapsules containing pretilachlor as a herbicide nanocarrier. Environ. Sci. pollut. Res. 26, 21579–21588. doi: 10.1007/s11356-019-05257-0
Ebube O. C., Amira G., Ngozi-Cynthia C. (2024). Future prospect in nano technology for herbicides production. Direct Res. J. Agric. Food Sci. 12 (1). doi: 10.26765/DRJAFS342006782
FAO. (2023). “Pesticides use and trade 1990–2021,” in FAOSTAT Analytical Briefs Series no. 70. (Rome, Italy: Food and Agriculture Organization of the United Nations).
Food and Agriculture Organization of the United Nations (FAO) (2018). Food outlook. Available online at: http://www.fao.org/worldfoodsituation/en/ (Accessed 1 December 2019).
Forini M. M., Pontes M. S., Antunes D. R., de Lima P. H., Santos J. S., Santiago E. F., et al. (2022). Nano-enabled weed management in agriculture: From strategic design to enhanced herbicidal activity. Plant Nano Biol. 1, 100008. doi: 10.1016/j.plana.2022.100008
Fraceto L. F., Grillo R., de Medeiros G. A., Scognamiglio V., Rea G., Bartolucci C. (2016). Nanotechnology in agriculture: which innovation potential does it have? Front. Environ. Sci. 4. doi: 10.3389/fenvs.2016.00020
Gaines T. A., Duke S. O., Morran S., Rigon C. A., Tranel P. J., Küpper A., et al. (2020). Mechanisms of evolved herbicide resistance. J. Biol. Chem. 295, 10307–10330. doi: 10.1074/jbc.REV120.013572
Gao C., Huang Q., Lan Q., Feng Y., Tang F., Hoi M. P. (2018). A user-friendly herbicide derived from photo-responsive supramolecular vesicles. Nat. Commun. 9 (1), 2967. doi: 10.1038/s41467-018-05437-5
Gao Y., Xiao Y., Mao K., Qin X., Zhang Y., Li D., et al. (2020). Thermoresponsive polymer-encapsulated hollow mesoporous silica nanoparticles and their application in insecticide delivery. Chem. Eng. J. 383, 123169. doi: 10.1016/j.cej.2019.123169
Gayathri K., Bhaskaran M., Selvam C., Thilagavathi R. (2023). Nano formulation approaches for curcumin delivery—a review. J. Drug Deliv. Sci. Technol. 82, 104326. doi: 10.1016/j.jddst.2023.104326
Ghaderpoori M., Jafari A., Nazari E., Rashidipour M., Nazari A., Chehelcheraghi F., et al. (2020). Preparation and characterization of loaded paraquat-polymeric chitosan/xantan/tripolyphosphate nanocapsules and evaluation for controlled release. J. Environ. Health Sci. Eng. 18, 1057–1066. doi: 10.1007/s40201-020-00527-3
Ghazi R. M., Yusoff N. R. N., Halim N. S. A., Wahab I. R. A., Ab Latif N., Hasmoni S. H., et al. (2023). Health effects of herbicides and its current removal strategies. Bioengineered 14, 2259526. doi: 10.1080/21655979.2023.2259526
Giroto A. S., de Campos A., Pereira E. I., Cruz C. C., Marconcini J. M., Ribeiro C. (2014). Study of a nanocomposite starch–clay for slow-release of herbicides: Evidence of synergistic effects between the biodegradable matrix and exfoliated clay on herbicide release control. J. Appl. Polym. Sci. 131 (23). doi: 10.1002/app.41188
Gomollón-Bel F. (2019). Ten chemical innovations that will change our world: IUPAC identifies emerging technologies in chemistry with potential to make our planet more sustainable. Chem. Int. 41, 12–17. doi: 10.1515/ci-2019-0203
Granetto M., Serpella L., Fogliatto S., Re L., Bianco C., Vidotto F., et al. (2022). Natural clay and biopolymer-based nanopesticides to control the environmental spread of a soluble herbicide. Sci. Total Environ. 806, 151199. doi: 10.1016/j.scitotenv.2021.151199
Grillo R., dos Santos N. Z. P., Maruyama C. R., Rosa A. H., de Lima R., Fraceto L. F. (2012). Poly (ε-caprolactone) nanocapsules as carrier systems for herbicides: physico-chemical characterization and genotoxicity evaluation. J. Hazard. Mater. 231, 1–9. doi: 10.1016/j.jhazmat.2012.06.019
Grillo R., Pereira A. E., Nishisaka C. S., de Lima R., Oehlke K., Greiner R., et al. (2014a). Chitosan/tripolyphosphate nanoparticles loaded with paraquat herbicide: an environmentally safer alternative for weed control. J. Hazard. Mater. 278, 163–171. doi: 10.1016/j.jhazmat.2014.05.079
Grillo R., Rosa A. H., Fraceto L. F. (2014b). Poly (ϵ-caprolactone) nanocapsules carrying the herbicide atrazine: effect of chitosan-coating agent on physico-chemical stability and herbicide release profile. Int. J. Environ. Sci. Technol. 11, 1691–1700. doi: 10.1007/s13762-013-0358-1
Hans M. L., Lowman A. M. (2002). Biodegradable nanoparticles for drug delivery and targeting. Curr. Opin. Solid State Mater. Sci. 6, 319–327. doi: 10.5650/jos.ess18209
Hao L., Gong L., Chen L., Guan M., Zhou H., Qiu S., et al. (2020). Composite pesticide nanocarriers involving functionalized boron nitride nanoplatelets for pH-responsive release and enhanced UV stability. Chem. Eng. J. 396, 125233. doi: 10.1016/j.cej.2020.125233
Hashim N., Misuan N. S., Isa I. M., Bakar S. A., Mustafar S., Mamat M., et al. (2020). Carboxymethylcellulose-coated magnesium-layered hydroxide nanocomposite for controlled release of 3-(4-methoxyphenyl) propionic acid. Arab. J. Chem. 13, 3974–3987. doi: 10.1016/j.arabjc.2019.04.004
Hazrati H., Saharkhiz M. J., Niakousari M., Moein M. (2017). Natural herbicide activity of Satureja hortensis L. essential oil nanoemulsion on the seed germination and morphophysiological features of two important weed species. Ecotoxicol. Environ. Saf. 142, 423–430. doi: 10.1016/j.ecoenv.2017.04.041
He X., Deng H., Hwang H. M. (2019). The current application of nanotechnology in food and agriculture. J. Food Drug Anal. 27, 1–21. doi: 10.1016/j.jfda.2018.12.002
Heap I. The international survey of herbicide resistant weeds. Available online at: http://www.weedscience.org (Accessed 23 October 2024).
Heydari M., Yousefi A. R., Nikfarjam N., Rahdar A., Kyzas G. Z., Bilal M. (2021). Plant-based nanoparticles prepared from protein containing tribenuron-methyl: fabrication, characterization, and application. Chem. Biol. Technol. Agric. 8, 1–11. doi: 10.1186/s40538-021-00254-3
Hosseini S. F., Zandi M., Rezaei M., Farahmandghavi F. (2013). Two-step method for encapsulation of oregano essential oil in chitosan nanoparticles: preparation, characterization and in vitro release study. Carbohydr. Polym. 95, 50–56. doi: 10.1016/j.carbpol.2013.02.031
Huang B., Chen F., Shen Y., Qian K., Wang Y., Sun C., et al. (2018). Advances in targeted pesticides with environmentally responsive controlled release by nanotechnology. Nanomaterials 8, 102. doi: 10.3390/nano8020102
Itodo H. U. (2019). Controlled release of herbicides using nano-formulation: A review. J. Chem. Rev. 1, 130–138. doi: 10.33945/SAMI/JCR.2019.2.4
Itodo H. U., Nnamonu L. A., Wuana R. A. (2017). Green synthesis of copper chitosan nanoparticles for controlled release of pendimethalin. Asian J. Chem. Sci. 2, 1–10. doi: 10.9734/ajocs/2017/32937
Jabran K., Mahajan G., Sardana V., Chauhan B. S. (2015). Allelopathy for weed control in agricultural systems. Crop Prot. 72, 57–65. doi: 10.1016/j.cropro.2015.03.004
Jia X., Yan Y., Zhang K., Wang C., You X., Yang S., et al. (2023). Glufosinate ammonium-loaded halloysite nanotubes for slow-release weeding polymer mulch films. ACS Appl. Nano Mater. 6, 6186–6196. doi: 10.1021/acsanm.3c00436
Jiang Y., Chen Y., Tian D., Shen F., Wan X., Xu L., et al. (2020). Fabrication and characterization of lignin–xylan hybrid nanospheres as pesticide carriers with enzyme-mediated release property. Soft Matter 16, 9083–9093. doi: 10.1039/d0sm01402h
Judy J. D., Unrine J. M., Rao W., Wirick S., Bertsch P. M. (2012). Bioavailability of gold nanomaterials to plants: importance of particle size and surface coating. Environ. Sci. Technol. 46, 8467–8474. doi: 10.1021/es3019397
Kah M., Hofmann T. (2014). Nanopesticide research: current trends and future priorities. Environ. Int. 63, 224–235. doi: 10.1016/j.envint.2013.11.015
Kah M., Machinski P., Koerner P., Tiede K., Grillo R., Fraceto L. F., et al. (2014). Analysing the fate of nanopesticides in soil and the applicability of regulatory protocols using a polymer-based nanoformulation of atrazine. Environ. Sci. pollut. Res. 21, 11699–11707. doi: 10.1007/s11356-014-2523-6
Kamaly N., Yameen B., Wu J., Farokhzad O. (2016). Degradable controlled-release polymers and polymeric nanoparticles: Mechanisms of controlling drug release. Chem. Rev. 116, 2602–2663. doi: 10.1021/acs.chemrev.5b00346
Kaphle A., Navya P. N., Umapathi A., Daima H. K. (2018). Nanomaterials for agriculture, food and environment: applications, toxicity and regulation. Environ. Chem. Lett. 16, 43–58. doi: 10.1007/s10311-017-0662-y
Kashyap P. L., Xiang X., Heiden P. (2015). Chitosan nanoparticle-based delivery systems for sustainable agriculture. Int. J. Biol. Macromol. 77, 36–51. doi: 10.1016/j.ijbiomac.2015.02.039
Ke M., Ye Y., Zhang Z., Gillings M., Qu Q., Xu N., et al. (2021). Synergistic effects of glyphosate and multiwall carbon nanotubes on Arabidopsis thaliana physiology and metabolism. Sci. Total Environ. 769, 145156. doi: 10.1016/j.scitotenv.2021.145156
Khan B. A., Nadeem M. A., Iqbal M., Yaqoob N., Javaid M. M., Maqbool R., et al. (2023). Chitosan nanoparticles loaded with mesosulfuron methyl and mesosulfuron methyl+ florasulam+ MCPA isooctyl to manage weeds of wheat (Triticum aestivum L.). Green Process. Synth. 12, 20228152. doi: 10.1515/gps-2022-8152
Khan B., Nadeem M., Javaid M., Maqbool R., Ikram M., Oraby H. (2022). Chemical synthesis, characterization, and dose optimization of chitosan-based nanoparticles of clodinofop propargyl and fenoxaprop-p-ethyl for management of Phalaris minor (little seed canary grass): First report. Green Process. Synth. 11, 1118–1127. doi: 10.1515/gps-2022-0096
Khan B. A., Nadeem M. A., Najeeb Alawadi H. F., Ayub M. A., Mahmood A., Abbas T., et al. (2024). An overview of the role of nanoherbicides in tackling challenges of weed management in wheat: A novel approach. Green Process. Synth. 13, 20240021. doi: 10.1515/gps-2024-0021
Khan Y., Sadia H., Ali Shah S. Z., Khan M. N., Shah A. A., Ullah N., et al. (2022). Classification, synthetic, and characterization approaches to nanoparticles, and their applications in various fields of nanotechnology: A review. Catalysts 12 (11), 1386. doi: 10.3390/catal12111386
Khatem R., Celis R., Hermosín M. C. (2019). Cationic and anionic clay nanoformulations of imazamox for minimizing environmental risk. Appl. Clay Sci. 168, 106–115. doi: 10.1016/j.clay.2018.10.014
Kremer R. J. (2019). Bioherbicides and nanotechnology: Current status and future trends. Nano-Biopesticides Today Future Perspect., 353–366. doi: 10.1016/B978-0-12-815829-6.00015-2
Kumar P. (2020). Role of nanotechnology in slow release of herbicide and season long weed control: A review. J. Pharmacogn. Phytochem. 9, 1882–1887.
Kumar S., Bhanjana G., Sharma A., Dilbaghi N., Sidhu M. C., Kim K. H. (2017). Development of nanoformulation approaches for the control of weeds. Sci. Total Environ. 586, 1272–1278. doi: 10.1016/j.scitotenv.2017.02.138
Kumar N., Kumar R., Shakil N. A., Das T. K. (2016). Nanoformulations of pretilachlor herbicide: preparation, characterization and activity. J. Sci. Ind. Res. 75, 676–680.
Lead J. R., Batley G. E., Alvarez P. J. J., Croteau M. N., Handy R. D., McLaughlin M. J., et al. (2018). Nanomaterials in the environment: behavior, fate, bioavailability, and effects—an updated review. Environ. Toxicol. Chem. 37, 2029–2063. doi: 10.1002/etc.4147
Li B., Duan W., Lin G., Cui Y., Wen R., Liu C., et al. (2023). Rational design and synthesis of L-carvone-derived 4-methyl-1, 2, 4-triazole-thioether/nanochitosan complexes as potent nanopesticides for sustainable and efficient herbicidal application. Environ. Sci.: Nano 10, 3317–3328. doi: 10.1039/d3EN00573A
Liu D., Yang F., Xiong F., Gu N. (2016). The smart drug delivery system and its clinical potential. Theranostics 6, 1306. doi: 10.7150/thno.14858
Liu C., Zhou H., Zhou J. (2021). The applications of nanotechnology in crop production. Molecules 26 (23), 7070. doi: 10.3390/molecules26237070
López-Cabeza R., Poiger T., Cornejo J., Celis R. (2019). A clay-based formulation of the herbicide imazaquin containing exclusively the biologically active enantiomer. Pest Manage. Sci. 75, 1894–1901. doi: 10.1002/ps.5296
Lv J., Christie P., Zhang S. (2019). Uptake, translocation, and transformation of metal-based nanoparticles in plants: recent advances and methodological challenges. Environ. Sci.: Nano 6, 41–59. doi: 10.1039/C8EN00645H
Lv X., Sha H., Ye Z., Wang Y., Mao B. (2023). Nanomaterials in plant management: functions, mechanisms and prospects. Environ. Sci.: Nano 10, 3232–3252. doi: 10.1039/D3EN00014A
MaChado T. O., Grabow J., Sayer C., de Araújo P. H., Ehrenhard M. L., Wurm F. R. (2022). Biopolymer-based nanocarriers for sustained release of agrochemicals: A review on materials and social science perspectives for a sustainable future of agri-and horticulture. Adv. Colloid Interface Sci. 303, 102645. doi: 10.1016/j.cis.2022.102645
Mahmoudian M., Torbati S., AliMirzayi N., Nozad E., Kochameshki M. G., Shokri A. (2020). Preparation and investigation of poly (methylmethacrylate) nano-capsules containing haloxyfop-R-methyl and their release behavior. J. Environ. Sci. Health B 55, 301–309. doi: 10.1080/03601234.2019.1692614
Maliki S., Sharma G., Kumar A., Moral-Zamorano M., Moradi O., Baselga J., et al. (2022). Chitosan as a tool for sustainable development: A mini review. Polymers 14, 1475. doi: 10.3390/polym14071475
Manisankar G., Ghosh P., Malik G. C., Banerjee M. (2022). Recent trends in chemical weed management: A review. Pharma Innov. 11, 745–753.
Marco-Brown J. L., Undabeytia T., Torres Sanchez R. M., dos Santos Afonso M. (2017). Slow-release formulations of the herbicide picloram by using Fe–Al pillared montmorillonite. Environ. Sci. pollut. Res. 24, 10410–10420. doi: 10.1007/s11356-017-8699-9
Maruyama C. R., Guilger M., Pascoli M., Bileshy-José N., Abhilash P. C., Fraceto L. F., et al. (2016). Nanoparticles based on chitosan as carriers for the combined herbicides imazapic and imazapyr. Sci. Rep. 6, 19768. doi: 10.1038/srep19768
Moore A. J., Dean L. S., Yam A. N., de Lima R., Fraceto L. F., Tetley T. D. (2022). Bioreactivity of a novel poly (epsilon-caprolactone) nanocapsule containing atrazine with human lung alveolar epithelial cells. Environ. Sci.: Nano 9, 2134–2148. doi: 10.1039/d1en01068a
Muthukrishnan S., Murugan I., Selvaraj M. (2019). Chitosan nanoparticles loaded with thiamine stimulate growth and enhances protection against wilt disease in Chickpea. Carbohydr. Polym. 212, 169–177. doi: 10.1016/j.carbpol.2019.02.037
Namasivayam S. K. R., Tony B. R. U. C. E., Bharani R. A., Raj F. R. (2015). Herbicidal activity of soil isolate of Fusarium oxysporum free and chitosan nanoparticles coated metabolites against economic important weedninidam theenjan. Asian J. Microbiol. Biotechnol. Environ. Sci. 17, 1015–1020. Available at: https://www.researchgate.net/publication/299511505.
Natarelli C. V. L., Claro P. I. C., Miranda K. W. E., Ferreira G. M. D., de Oliveira J. E. (2019). 2, 4-Dichlorophenoxyacetic acid adsorption on montmorillonite organoclay for controlled release applications. SN Appl. Sci. 1, 1–13. doi: 10.1007/s42452-019-1235-4
Nuruzzaman M., Liu Y., Rahman M. M., Dharmarajan R., Duan L., Uddin A. F. M. J., et al. (2019). Nanobiopesticides: Composition and preparation methods. Nano-biopesticides Today Future Perspectives., 69–131. doi: 10.1016/B978-0-12-815829-6.00004-8
Nuruzzaman M. D., Rahman M. M., Liu Y., Naidu R. (2016). Nanoencapsulation, nano-guard for pesticides: a new window for safe application. J. Agric. Food Chem 64(7), 1447–1483. doi: 10.1021/acs.jafc.5b05214
OECD/FAO. (2024). OECD-FAO agricultural outlook 2024-2033 (Paris and Rome: Organisation for Economic Co-operation and Development). doi: 10.1787/4c5d2cfb-en
Oliveira H. C., Stolf-Moreira R., Martinez C. B. R., Grillo R., de Jesus M. B., Fraceto L. F. (2015a). Nanoencapsulation enhances the post-emergence herbicidal activity of atrazine against mustard plants. PloS One 10, e0132971. doi: 10.1371/journal.pone.0132971
Oliveira H. C., Stolf-Moreira R., Martinez C. B., Sousa G. F., Grillo R., De Jesus M. B., et al. (2015b). Evaluation of the side effects of poly (epsilon-caprolactone) nanocapsules containing atrazine toward maize plants. Front. Chem. 3. doi: 10.3389/fchem.2015.00061
Oliveira-Pinto P. R., Mariz-Ponte N., Gil R. L., Cunha E., Amorim C. G., Montenegro M. C. B. S. M., et al. (2022). Montmorillonite nanoclay and formulation with Satureja montana essential oil as a tool to alleviate Xanthomonas euvesicatoria load on Solanum lycopersicum. Appl. Nano 3, 126–142. doi: 10.3390/applnano3030009
Onyeaka H., Ghosh S., Obileke K., Miri T., Odeyemi O. A., Nwaiwu O., et al. (2024). Preventing chemical contaminants in food: Challenges and prospects for safe and sustainable food production. Food Control 155, 110040. doi: 10.1016/j.foodcont.2023.110040
Oreja F. H., Moreno N., Gundel P. E., Vercellino R. B., Pandolfo C. E., Presotto A., et al. (2024). Herbicide-resistant weeds from dryland agriculture in Argentina. Weed Res. 64, 89–106. doi: 10.1111/wre.12613
Pan X., Guo X., Zhai T., Zhang D., Rao W., Cao F., et al. (2023). Nanobiopesticides in sustainable agriculture: developments, challenges, and perspectives. Environ. Sci.: Nano 10, 41–61. doi: 10.1039/d2en00605g
Patra J. K., Das G., Fraceto L. F., Campos E. V. R., Rodriguez-Torres M. D. P., Acosta-Torres L. S., et al. (2018). Nano based drug delivery systems: recent developments and future prospects. J. Nanobiotechnol. 16, 1–33. doi: 10.1186/s12951-018-0392-8
Paul S. K., Mazumder S., Naidu R. (2024). Herbicidal weed management practices: History and future prospects of nanotechnology in an eco-friendly crop production system. Heliyon 10 (5). doi: 10.1016/j.heliyon.2024.e26527
Paul S. K., Nuruzzaman M., Correa T. C., Naidu R. (2019). Aluminosilicate nano-skeleton to firm the structure and properties of nano-herbicide formulations. Proceedings of the 8th International Contaminated Site Remediation Conference, CRC CARE Pty. Ltd., CleanUp Conference 2019, Adelaide, Australia. Available online at: https://www.researchgate.net/publication/376954609 (Accessed September 8 –12, 2019).
Pereira A. E., Grillo R., Mello N. F., Rosa A. H., Fraceto L. F. (2014). Application of poly (epsilon-caprolactone) nanoparticles containing atrazine herbicide as an alternative technique to control weeds and reduce damage to the environment. J. Hazard. Mater. 268, 207–215. doi: 10.1016/j.jhazmat.2014.01.025
Pérez-de-Luque A. (2017). Interaction of nanomaterials with plants: what do we need for real applications in agriculture? Front. Environ. Sci. 5. doi: 10.3389/fenvs.2017.00012
Periakaruppan R., Romanovski V., Thirumalaisamy S. K., Palanimuthu V., Sampath M. P., Anilkumar A., et al. (2023). Innovations in modern nanotechnology for the sustainable production of agriculture. ChemEngineering 7, 61. doi: 10.3390/chemengineering7040061
Pontes M. S., Antunes D. R., Oliveira I. P., Forini M. M. L., Santos J. S., Arruda G. J., et al. (2021). Chitosan/tripolyphosphate nanoformulation carrying paraquat: insights on its enhanced herbicidal activity. Environ. Sci. Nano 8, 1336–1351. doi: 10.1039/D0EN01128B
Prasad A., Astete C. E., Bodoki A. E., Windham M., Bodoki E., Sabliov C. M. (2017). Zein nanoparticles uptake and translocation in hydroponically grown sugar cane plants. J. Agric. Food Chem. 66, 6544–6551. doi: 10.1021/acs.jafc.7b02487
Proença P. L., Carvalho L. B., Campos E. V., Fraceto L. F. (2022). Fluorescent labeling as a strategy to evaluate uptake and transport of polymeric nanoparticles in plants. Adv. Colloid Interface Sci. 305, 102695. doi: 10.1016/j.cis.2022.102695
Qu R. Y., He B., Yang J. F., Lin H. Y., Yang W. C., Wu Q. Y., et al. (2021). Where are the new herbicides? Pest Manage. Sci. 77, 2620–2625. doi: 10.1002/ps.6285
Rahman M. M., Tsukamoto J., Rahman M. M., Yoneyama A., Mostafa K. M. (2013). Lignin and its effects on litter decomposition in forest ecosystems. Chem. Ecol. 29, 540–553. doi: 10.1080/02757540.2013.790380
Rajput V., Minkina T., Mazarji M., Shende S., Sushkova S., Mandzhieva S., et al. (2020). Accumulation of nanoparticles in the soil-plant systems and their effects on human health. Ann. Agric. Sci. 65, 137–143. doi: 10.1016/j.aoas.2020.08.001
Rajput V. D., Singh A., Minkina T. M., Shende S. S., Kumar P., Verma K. K., et al. (2021). Potential applications of nanobiotechnology in plant nutrition and protection for sustainable agriculture. Nanotechnology Plant Growth Promotion Protection: Recent Adv. Impacts, 79–92. doi: 10.1002/9781119745884.ch5
Rashidipour M., Maleki A., Kordi S., Birjandi M., Pajouhi N., Mohammadi E., et al. (2019). Pectin/chitosan/tripolyphosphate nanoparticles: efficient carriers for reducing soil sorption, cytotoxicity, and mutagenicity of paraquat and enhancing its herbicide activity. J. Agric. Food Chem. 67, 5736–5745. doi: 10.1021/acs.jafc.9b01106
Rashidzadeh A., Olad A., Hejazi M. J. (2017). Controlled release systems based on intercalated paraquat onto montmorillonite and clinoptilolite clays encapsulated with sodium alginate. Adv. Polym. Technol. 36, 177–185. doi: 10.1002/adv.21597
Rebitski E. P., Darder M., Aranda P. (2019). Layered double hydroxide/sepiolite hybrid nanoarchitectures for the controlled release of herbicides. Beilstein J. Nanotechnol 10(1), 1679–1690. doi: 10.3762/bjnano.10.163
Rodrigues S. M., Demokritou P., Dokoozlian N., Hendren C. O., Karn B., Mauter M. S., et al. (2017). Nanotechnology for sustainable food production: promising opportunities and scientific challenges. Environ. Sci. Nano 4, 767–781. doi: 10.1039/c6en00573j
Rodríguez Mejías F. J., Carrasco Á., García Durán A., González Molinillo J. M., Macías Domínguez F. A., Chinchilla Salcedo N. (2022). On the formulation of disulfide herbicides based on aminophenoxazinones: polymeric nanoparticle formulation and cyclodextrin complexation to combat crop yield losses. doi: 10.1002/ps.7327
Sahoo U., Malik G. C., Banerjee M., Sahoo B., Maitra S. (2022). Application of nanotechnology in agriculture in India. Environ. Nanotechnol. 4, 317–348.
Sameeha M. S. (2023). Herbicides And Human Health - An Overview. iCliniq. https://www.icliniq. com/articles/healthy-living-wellness-and-prevention /herbicides-and-human-health
Santana I., Wu H., Hu P., Giraldo J. P. (2020). Targeted delivery of nanomaterials with chemical cargoes in plants enabled by a biorecognition motif. Nat. Commun. 11, 2045. doi: 10.1038/s41467-020-15731-w
Schnoor B., Elhendawy A., Joseph S., Putman M., Chacón-Cerdas R., Flores-Mora D., et al. (2018). Engineering atrazine loaded poly (lactic-co-glycolic acid) nanoparticles to ameliorate environmental challenges. J. Agric. Food Chem. 66, 7889–7898. doi: 10.1021/acs.jafc.8b01911
Sen M. K., Bhattacharya S., Bharati R., Hamouzová K., Soukup J. (2023). Comprehensive insights into herbicide resistance mechanisms in weeds: A synergistic integration of transcriptomic and metabolomic analyses. Front. Plant Sci. 14. doi: 10.3389/fpls.2023.1280118
Shakiba S., Astete C. E., Paudel S., Sabliov C. M., Rodrigues D. F. (2020). Emerging investigator series: polymeric nanocarriers for agricultural applications: synthesis, characterization, and environmental and biological interactions. Environ. Sci. Nano 7, 37–67. doi: 10.1039/C9EN01127G
Shangguan W., Huang Q., Chen H., Zheng Y., Zhao P., Cao C., et al. (2024). Making the complicated simple: A minimizing carrier strategy on innovative nanopesticides. Nano-Micro Lett. 16, 1–16. doi: 10.1007/s40820-024-01413-5
Singh M., Kukal M. S., Irmak S., Jhala A. J. (2022). Water use characteristics of weeds: A global review, best practices, and future directions. Front. Plant Sci. 12. doi: 10.3389/fpls.2021.794090
Singh A., Rajput V. D., Varshney A., Ghazaryan K., Minkina T. (2023). Small tech, big impact: Agri-nanotechnology journey to optimize crop protection and production for sustainable agriculture. Plant Stress, 100253. doi: 10.1016/j.stress.2023.100253
Sousa B. T., Carvalho L. B., Preisler A. C., Saraiva-Santos T., Oliveira J. L., Verri W. A. Jr., et al. (2024). Chitosan coating as a strategy to increase postemergent herbicidal efficiency and alter the interaction of nanoatrazine with Bidens pilosa plants. ACS Appl. Mater. Interfaces. doi: 10.1021/acsami.4c03800
Sousa G. F., Gomes D. G., Campos E. V., Oliveira J. L., Fraceto L. F., Stolf-Moreira R., et al. (2018). Post-emergence herbicidal activity of nanoatrazine against susceptible weeds. Front. Environ. Sci. 6. doi: 10.3389/fenvs.2018.00012
Sousa B. T., Pereira S., do Espírito A., Fraceto L. F., de Oliveira H. C., Dalazen G. (2020). Effectiveness of nanoatrazine in post-emergent control of the tolerant weed Digitaria insularis. J. Plant Protect. Res., 185–192. doi: 10.24425/jppr.2020.133311
Srimathi T. K., Chinnamuthu C. R., Marimuthu S., Senthil A. (2021). Optimizing time and dose of newly synthesised Nano encapsulated sulfentrazone herbicide formulation for weed management in irrigated groundnut (Arachis hypogaea). Pharma Innov. J. 10, 546–549. Available at: http://www.thepharmajournal.com.
Su Y., Ashworth V., Kim C., Adeleye A. S., Rolshausen P., Roper C., et al. (2019). Delivery, uptake, fate, and transport of engineered nanoparticles in plants: a critical review and data analysis. Environ. Sci. Nano 6, 2311–2331. doi: 10.1039/c9en00461k
Swetha S., Chinnamuthu C. R., Arthanari P. M., Marimuthu S., Senthil A. (2022). Novel method to manage weeds in the rainfed groundnut (var. VRI 8 and TMV 14) using nano encapsulated herbicide formulations. J. Appl. Nat. Sci. 14, 1341–1350. doi: 10.31018/jans.v14i4.3920
Taban A., Saharkhiz M. J., Kavoosi G. (2021). Development of pre-emergence herbicide based on Arabic gum-gelatin, apple pectin and savory essential oil nano-particles: A potential green alternative to metribuzin. Int. J. Biol. Macromol. 167, 756–765. doi: 10.1016/j.ijbiomac.2020.12.007
Taban A., Saharkhiz M. J., Khorram M. (2020). Formulation and assessment of nano-encapsulated bioherbicides based on biopolymers and essential oil. Ind. Crops Prod. 149, 112348. doi: 10.1016/j.indcrop.2020.112348
Takeshita V., Campos E. V., Rodrigues J. S., Fraceto L. F. (2023). Opinion: Hybrid nanoparticle systems–Two-way delivery approach for agriculture. Plant Nano Biol. 6, 100053. doi: 10.1016/j.plana.2023.100053
Takeshita V., Carvalho L. B., Galhardi J. A., Munhoz-Garcia G. V., Pimpinato R. F., Oliveira H. C., et al. (2022a). Development of a preemergent nanoherbicide: From efficiency evaluation to the assessment of environmental fate and risks to soil microorganisms. ACS Nanoscience Au 2, 307–323. doi: 10.1021/acsnanoscienceau.1c00055
Takeshita V., de Sousa B. T., Preisler A. C., Carvalho L. B., Pereira A., do E. S., et al. (2021). Foliar absorption and field herbicidal studies of atrazine-loaded polymeric nanoparticles. J. Hazard. Mater. 418, 126350. doi: 10.1016/j.jhazmat.2021.126350
Takeshita V., Munhoz-Garcia G. V., Werk Pinácio C., Cardoso B. C., Nalin D., Tornisielo V. L., et al. (2022b). Availability of metribuzin-loaded polymeric nanoparticles in different soil systems: an important study on the development of safe nanoherbicides. Plants 11 (23). doi: 10.3390/plants11233366
Tan D., Yuan P., Annabi-Bergaya F., Dong F., Liu D., He H. (2015a). A comparative study of tubular halloysite and platy kaolinite as carriers for the loading and release of the herbicide amitrole. Appl. Clay Sci. 114, 190–196. doi: 10.1016/j.clay.2015.05.024
Tan D., Yuan P., Annabi-Bergaya F., Liu D., He H. (2015b). Methoxy-modified kaolinite as a novel carrier for high-capacity loading and controlled-release of the herbicide amitrole. Sci. Rep. 5, 8870. doi: 10.1038/srep08870
Thambiliyagodage C., Jayanetti M., Mendis A., Ekanayake G., Liyanaarachchi H., Vigneswaran S. (2023). Recent advances in chitosan-based applications—a review. Materials 16, 2073. doi: 10.3390/ma16052073
Torbati S., Mahmoudian M., Alimirzaei N. (2018). Nanocapsulation of herbicide Haloxyfop-R-methyl in poly (methyl methacrylate): phytosociological effects of pure herbicide and its nanocapsule form on duckweed as a model macrophyte. Turk. J. Chem. 42, 132–145. doi: 10.3906/kim-1705-70
Tripathi D. K., Singh S., Singh S., Pandey R., Singh V. P., Sharma N. C., et al. (2017). An overview on manufactured nanoparticles in plants: uptake, translocation, accumulation and phytotoxicity. Plant Physiol. Biochem. 110, 2–12. doi: 10.1016/j.plaphy.2016.07.030
Undabeytia López T., Galán Jiménez C., Gómez-Pantoja E., Vázquez Cabello J., Casal B., Annaby-Bergaya F., et al. (2013). Fe-pillared clay mineral-based formulations of imazaquin for reduced leaching in soil. Appl. Clay Sci. 80, 382–389. doi: 10.1016/j.clay.2013.07.001
Verma K. K., Song X. P., Joshi A., Rajput V. D., Singh M., Sharma A., et al. (2022). Nanofertilizer possibilities for healthy soil, water, and food in future: An overview. Front. Plant Sci. 13. doi: 10.3389/fpls.2022.865048
Wilms W., Woźniak-Karczewska M., Syguda A., Niemczak M., Ławniczak Ł., Pernak J., et al. (2020). Herbicidal ionic liquids: A promising future for old herbicides? Review on synthesis, toxicity, biodegradation, and efficacy studies. J. Agric. Food Chem. 68, 10456–10488. doi: 10.1021/acs.jafc.0c02894
Wu J., Zhai Y., Monikh F. A., Arenas-Lago D., Grillo R., Vijver M. G., et al. (2021). The differences between the effects of a nanoformulation and a conventional form of atrazine to lettuce: physiological responses, defense mechanisms, and nutrient displacement. J. Agric. Food Chem. 69, 12527–12540. doi: 10.1021/acs.jafc.1c01382
Xin X., He Z., Hill M. R., Niedz R. P., Jiang X., Sumerlin B. S. (2018). Efficiency of biodegradable and pH-responsive polysuccinimide nanoparticles (PSI-NPs) as smart nanodelivery systems in grapefruit: in vitro cellular investigation. Macromol. Biosci. 18, 1800159. doi: 10.1002/mabi.201800159
Xin X., Judy J. D., Sumerlin B. B., He Z. (2020). Nano-enabled agriculture: from nanoparticles to smart nanodelivery systems. Environ. Chem. 17, 413–425. doi: 10.1071/EN19254
Yang C., Powell C. A., Duan Y., Shatters R., Zhang M. (2015). Antimicrobial nanoemulsion formulation with improved penetration of foliar spray through citrus leaf cuticles to control citrus huanglongbing. PloS One 10 (7). doi: 10.1371/journal.pone.0133826
Yates R. J., Steel E. J., Edwards T. J., Harrison R. J., Hackney B. F., Howieson J. G. (2024). Adverse consequences of herbicide residues on legumes in dryland agriculture. Field Crops Res. 308, 109271. doi: 10.1016/j.fcr.2024.109271
Yearla S. R., Padmasree K. (2016). Exploitation of subabul stem lignin as a matrix in controlled release agrochemical nanoformulations: A case study with herbicide diuron. Environ. Sci. pollut. Res. Int. 23, 18085–18098. doi: 10.1007/s11356-016-6983-8
Yu Z., Sun X., Song H., Wang W., Ye Z., Shi L., et al. (2015). Glutathione-responsive carboxymethyl chitosan nanoparticles for controlled release of herbicides. Mater. Sci. Appl. 6, 591–604. doi: 10.4236/msa.2015.66062
Zaaba N. F., Jaafar M. (2020). A review on degradation mechanisms of polylactic acid: Hydrolytic, photodegradative, microbial, and enzymatic degradation. Polym. Eng. Sci. 60, 2061–2075. doi: 10.1002/pen.25511
Zainuddin N. J., Ashari S. E., Salim N., Asib N., Omar D., Lian G. E. C. (2019). Optimization and characterization of palm oil-based nanoemulsion loaded with Parthenium hysterophorus crude extract for natural herbicide formulation. J. Oleo Sci. 68, 747–757. doi: 10.5650/jos.ess18209
Zargar M., Bayat M., Saquee F. S., Diakite S., Ramzanovich N. M., Akhmadovich K. A. (2023). New advances in nano-enabled weed management using poly(epsilon-caprolactone)-based nanoherbicides: A review. Agriculture 13, 2031. doi: 10.3390/agriculture13102031
Zha X., Hou X., Li Q., Nan H., Ge F., Liu Y., et al. (2022). Loading glyphosate in attapulgite and sodium alginate hydrogels to construct pH-responsive controlled release microsphere for enhanced soil sustained release. ACS Agric. Sci. Technol. 2, 1090–1100. doi: 10.1021/acsagscitech.2c00195
Zhai Y., Abdolahpur Monikh F., Wu J., Grillo R., Arenas-Lago D., Darbha G. K., et al. (2020). Interaction between a nano-formulation of atrazine and rhizosphere bacterial communities: atrazine degradation and bacterial community alterations. Environ. Sci. Nano 7, 3372–3384. doi: 10.1039/d0en00638f
Zhang L., Chen C., Zhang G., Liu B., Wu Z., Cai D. (2020). Electrical-driven release and migration of herbicide using a gel-based nanocomposite. J. Agric. Food Chem 68(6), 1536–1545. doi: 10.1021/acs.jafc.9b07166
Keywords: nanotechnology, herbicide, biodegradable, weed, encapsulation, slow-release, sustainable, carrier-based
Citation: Jayasoorya R and Kumar P (2024) Utilization of biodegradable carrier-based nano herbicide formulations for sustainable weed management in agriculture. Front. Agron. 6:1497041. doi: 10.3389/fagro.2024.1497041
Received: 16 September 2024; Accepted: 06 November 2024;
Published: 27 November 2024.
Edited by:
Simerjeet Kaur, Punjab Agricultural University, IndiaReviewed by:
Muthuraman Yuvaraj, Tamil Nadu Agricultural University, IndiaDr Harshdeep Kaur, Punjab Agricultural University, India
Copyright © 2024 Jayasoorya and Kumar. This is an open-access article distributed under the terms of the Creative Commons Attribution License (CC BY). The use, distribution or reproduction in other forums is permitted, provided the original author(s) and the copyright owner(s) are credited and that the original publication in this journal is cited, in accordance with accepted academic practice. No use, distribution or reproduction is permitted which does not comply with these terms.
*Correspondence: Pradeesh Kumar, cHJhZGVlc2hrdW1hci50QHZpdC5hYy5pbg==