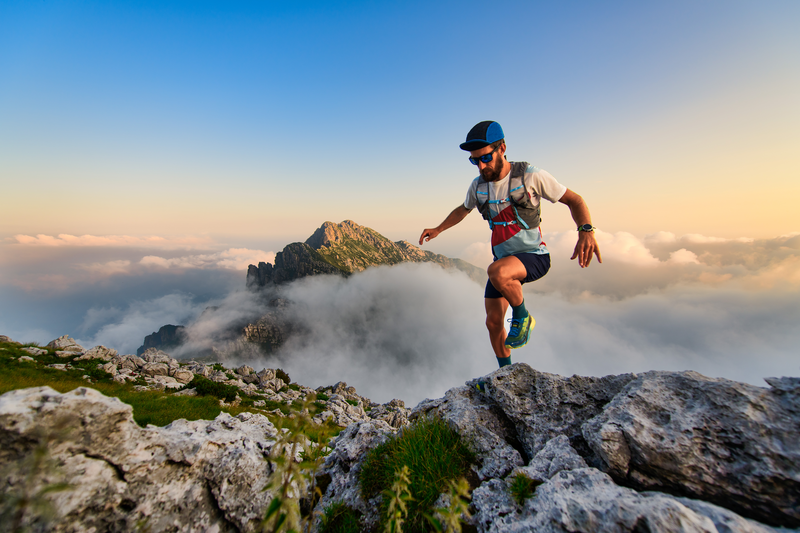
94% of researchers rate our articles as excellent or good
Learn more about the work of our research integrity team to safeguard the quality of each article we publish.
Find out more
ORIGINAL RESEARCH article
Front. Agron. , 02 January 2025
Sec. Weed Management
Volume 6 - 2024 | https://doi.org/10.3389/fagro.2024.1496841
This article is part of the Research Topic Innovative Technology and Techniques for Effective Weed Control View all 6 articles
Introduction: Ambrosia artemisiifolia, a highly invasive weed species, poses significant challenges to agriculture and human health. This study investigated the germination thresholds and physical properties of A. artemisiifolia populations from diverse regions in Europe, encompassing Serbia, Croatia, Italy, and France.
Results: Results revealed intriguing variations in germination thresholds among the populations. The Italian population exhibited the lowest base temperature (Tb) of 0.58°C, closely followed by the Croatian population (1.49°C), statistically similar to the Serbian (1.46°C) and French (2.74°C) populations. In contrast, the Serbian population displayed the lowest base water potential (Ψb) of −1.44 MPa, followed by the French population (−1.23 MPa), with no significant differences observed between the Italian (−0.78 MPa) and Croatian (−0.80 MPa) populations. Analysis of physical seed properties unveiled notable disparities in size, weight, and shape. The Italian population boasted the smallest, lightest, and most spherical seeds, while the French population harbored the largest and most elongated seeds. Interestingly, the seeds of the Croatian population were the heaviest.
Conclusions: This study underscores the adaptability of A. artemisiifolia populations to diverse climatic conditions, showcasing varied responses across regions. These findings elucidate the intricate interplay between environmental factors and seed traits, offering valuable insights for the development of effective weed management strategies.
Among the vast array of plant species within the Ambrosia genus, Ambrosia artemisiifolia L. emerges as a particularly troublesome species (Goeden and Andrès, 1999; Taylor, 2019). Originating in North America, this invasive plant from the Asteraceae family has rapidly expanded its reach, becoming a ubiquitous presence in diverse ecosystems around the world (Smith et al., 2013; Montagnani et al., 2017). Characterized by its robust vigor, superior adaptability, high seed yields, and rapid dispersal, A. artemisiifolia presents an important menace capable of significantly altering the dynamics of native flora and fauna (Essl et al., 2015). Over the last two centuries, A. artemisiifolia has spread in 80 countries, disrupting ecological balances, diminishing biodiversity, and threatening the production of valuable farmland, thereby reducing crop yields (Dong et al., 2020). The adaptability potential of A. artemisiifolia takes various forms, including genetic diversity and phenological variations. Studies have consistently highlighted the high genetic variability of this species, pointing to its capacity to adapt to diverse environments (Genton et al., 2005; Li et al., 2019; Sun et al., 2020). Beyond genetic diversity, also the phenological variations are further accentuating the species’ ability to thrive across varied ecological niches (Buttenschøn et al., 2009; Leskovšek et al., 2012; Scalone et al., 2016). This adaptability assumes even greater significance considering predictions of A. artemisiifolia’s continued expansion fueled by global warming (Cunze et al., 2013; Skálová et al., 2017; Sun et al., 2020). In addition to its disruptive impact on natural ecosystems, A. artemisiifolia has proven to be a significant agricultural weed. Changes in agricultural practices, especially the shift towards more intensive farming systems, have provided a suitable environment for the accelerated expansion of this invasive species (Kiss and Béres, 2006). Studies indicate that high populations of A. artemisiifolia can result in substantial yield losses, reaching up to 75% in soybean and 80% in maize, compared to estimated weed-free yields (Weaver, 2001; Cowbrough et al., 2003). Various control measures including chemical, physical and biological techniques have been explored to mitigate the impact of A. artemisiifolia (Buttenschøn et al., 2009; Milakovic et al., 2014; Milakovic and Karrer, 2016). However, eradication of this species has proven to be a formidable challenge. Notably, A. artemisiifolia exhibits resistance to air and soil pollution (Pichtel et al., 2000; Ziska, 2002), and in some cases it remarkably retains the ability to reproduce even after damage from defoliation and mowing (Brandes and Nitzsche, 2006; Gard et al., 2013). Presently, control of this invasive weed species is feasible in major crops through chemical, mechanical, or combined measures (Scruggs et al., 2019; Máčajová et al., 2022; Wang et al., 2022). However, there is a concern over the potential development of herbicide resistance over time (Saint-Louis et al., 2005). Understanding the distribution and spread of this species is challenging. Its introduction to Europe was tied to trade, resulting in different introduction points at different times and contributing to genetic variations. Populations already present in Europe further propagated in a similar manner, fostering hybridization and population variations (Csontos et al., 2010; Chapman et al., 2016; van Boheemen et al., 2017; Afonin et al., 2018). Despite existing studies on the introduction and distribution of A. artemisiifolia in the origin areas of the studied populations (Chauvel et al., 2006; Csontos et al., 2010; Galzina et al., 2010; Gentili et al., 2017), pinpointing the exact introduction pathway and population evolution remains a difficult, if not impossible, task. Given the species’ great adaptability potential and diversification (Battlay et al., 2023), it might be more meaningful to explore the responses of different populations to climatic conditions.
To address this critical issue and improve the control of A. artemisiifolia, an in-depth study of its behavior and physiology is essential, particularly during crucial stages such as germination. Germination and subsequently the emergence are the most critical periods in weed species life cycle, as they can largely influence the future competition with the crops and damage to the yield (Forcella et al., 2000). These critical periods mostly depend on the temperature and water availability needed for germination processes. In this regard it was discovered that seeds have temperature and water thresholds below which germination is not possible, these thresholds are defined as base temperature (Tb) and base water potential (Ψb) respectfully (Arnold, 1959; Dahal and Bradford, 1994). This knowledge can inform the development of emergence models, aiding in predicting growth dynamics and suggesting optimal timing for control operations, thereby reducing herbicide use and airborne pollen levels (Masin et al., 2005, 2010, 2014; Colbach et al., 2007). Given the high variability of A. artemisiifolia, that reflects in both a wide range of temperatures and water availability needed for germination (Leiblein and Lösch, 2011; Dinelli et al., 2013; Knolmajer et al., 2024), it is crucial to determine if populations from different regions exhibit similar behavior. Considering that there are only a few articles found in the literature that examined these parameters (Guillemin et al., 2013), in this study, we compared four A. artemisiifolia populations, examining seed parameters and determining base temperature (Tb) and base water potential (Ψb) essential for germination, as well as different seed physical properties. By discovering the germination dynamics of diverse populations, our research aims to contribute to a broader understanding of A. artemisiifolia behavior. Insights gained can potentially inform the development of effective control strategies that are appropriate to specific populations, ultimately mitigating the ecological, agricultural, and health impacts of this invasive species.
Experiments were conducted in 2018 at the University of Padova, Department of Agronomy, Food, Natural Resources, Animals and Environment (DAFNAE). The mature, ripe seeds (achenes) of A. artemisiifolia were hand collected in 2017 from four different locations: Dijon in eastern France −47°20’43’’N 5°06’05’’E (FR), Turin in northwestern Italy –45°07’27’’N 7°31’04’’E (IT), Zagreb in central Croatia –45°44’02’’N 15°54’23’’E (CRO) and Šabac in northwestern Serbia –44°45’49’’N 19°36’12’’E (SRB); in each case the seeds were collected from an agricultural environment (Figure 1). The decision to collect seeds from these sites, very far apart from one another was based on previous studies (Scalone et al., 2016; Song et al., 2023; Putra et al., 2024), which determined the absence or very low variability between the close populations, even when separated by several kilometers from one another. In addition, this species shows niche-filling tendencies, meaning that there are little to no statistically significant differences between the populations that occupy a single niche, for example in a study conducted in Australia the expansion of the range of A. artemisiifolia was seen only in the case of introduction of this species populations from different continents (Song et al., 2023; Putra et al., 2024). After harvesting, all seeds underwent a two-step cleaning procedure. The seeds were firstly manually cleaned by rubbing them against a wooden tablet laced with rubber to avoid damaging the seeds. In this way, different impurities present were ground reducing their weight. After this initial step, a seed blower was used to separate the seeds from impurities blowing them away as they were lighter than the seeds. Once the cleaning was completed the seeds were stored in a dry environment at 4°C until utilized. In all the trials the original seeds collected at different sites were used.
Figure 1. The overview map (top-right) shows the European continent, with a yellow box marking the region where the seed populations originated. The main map provides a detailed view of this region, highlighting the specific locations where seeds were collected.
Since the geographical distribution of the examined populations is quite diverse it is reasonable to assume that certain parameters are influenced by the climate. To account for this, temperature and precipitation data were sourced from Climate-Data.org, a platform with confirmed reliability, that has been widely used in previous studies (Rodriguez and D’Alessandro, 2019; Stradford et al., 2021; Gardin et al., 2023). According to the Köppen-Geiger classification, Zagreb, Šabac and Turin are categorized as Cfa with warm temperatures, humid and hot summers (Kottek et al., 2006). Dijon is classified as Cfb, which indicates a warm summer, high humidity and warm temperature. The average annual temperature in Zagreb is 11°C. July is the warmest month with a maximum temperature of 27.4°C, while January is the coldest at −2.8°C. The average annual precipitation is 930 mm. Dijon has a similar average annual temperature of 11°C. July has the highest temperatures at 24.7°C, while February is the lowest at −0.3°C. The average annual precipitation is 982 mm. In Turin, the average temperature rises slightly to 12°C. July remains the warmest month with a maximum temperature of 27.3°C, and the lowest average temperature is −1.7°C in January. The city records an average annual precipitation of 1002 mm. In Šabac, the average annual temperature rises to 12.9°C. August is the warmest month with a maximum temperature of 29.1°C, while January has the lowest temperature at −2.6°C. The average annual precipitation is 714 mm.
The data presented is the average on a monthly basis, spanning a 30-year period from 1991 to 2021 (Figure 2).
Figure 2. Average monthly precipitations, minimum, mean and maximum temperatures in Dijon (A), Turin (B), Zagreb (C) and Šabac (D) for a 30-year period from 1991 to 2021 (source: Climate-Data.org). Tmax, maximum temperature; Tmean, mean temperature; Tmin, minimum temperature.
To determine the temperature thresholds for germination, climate chambers were set at the following temperatures: 1, 3, 6, 9, 12, 15, 18, 21, 24, 27 and 30°C. Four replicates of 50 seeds each were tested for each temperature and for each population of A. artemisiifolia, following an already established protocol in different similar works (Puteh et al., 2010; Loddo et al., 2013, 2018). The seeds were sown on filter paper (Whatman No. 1, Whatman, Maidstone, UK) in 9 cm-diameter Petri dishes with 4 ml of distilled water. After sowing, the Petri dishes were sealed with parafilm and placed inside the climate chambers (W87R, KW Apparecchi Scientifici SRL, via della Resistenza 119, 53035 Monteriggioni, Italy) set to the predetermined temperature, and with a photoperiod of 12 h light and 12 h dark; water was added if needed. Germination was monitored every 2–3 days, and was considered concluded once all seeds had germinated or after 10 days had elapsed without further germination, as proposed by (Onofri et al., 2010; Baskin and Baskin, 2014b). All germinated seeds were counted and removed.
The water potential threshold was determined using the same seed of the populations previously described. Polyethylene glycol (PEG) 6000 (Sigma-Aldrich Chemie GmbH 25322-68-3, St. Louis, MO, USA) was used to simulate different conditions of water availability. Following the formula proposed by Kaufmann and Michel (1973), specific quantities of PEG-6000 were added to distilled water to obtain solutions of −0.05, −0.10, −0.30, −0.50, −0.70, −1.00, −1.50, −2.00 MPa; controls consisted in distilled water (0.00 MPa) alone. The seeds were sown on filter paper and placed in plastic containers with diameter and height of 10 cm and 7 cm, respectively, as described by Šoštarčić et al. (2021a) with the specific PEG-6000 solution; the liquid level was kept below the seeds to prevent them from being immersed in the solution. The experiment consisted of four replicates of 50 seeds each, for each population of A. artemisiifolia. After sowing, the plastic containers were closed and placed in climate chambers at 24°C with a photoperiod of 12 h light and 12 h dark. Germination was monitored every 2–3 days and was considered concluded after 10 days had elapsed without further germination (Onofri et al., 2010; Baskin and Baskin, 2014b). All germinated seeds were counted and removed.
Considering the diverse origins of the tested populations and in order to characterize them, the seed physical properties were also analyzed. Seed physical properties, such as size, weight, and shape, are critical traits that can significantly influence germination processes and plant performance under varying environmental conditions (Leishman, 2001; Norden et al., 2009). For example, variations in seed size and mass have been shown to affect germination metrics such as germination time (Murali, 1997) and germination percentage (Van Mölken et al., 2005). These characteristics indirectly impact plant distribution and abundance, contributing to the ability of species to establish across different habitats (Silveira et al., 2012). Importantly, seed size can vary both within and between plant species, sometimes by several orders of magnitude (Leishman et al., 1995; Silvertown and Bullock, 2003; Moles and Westoby, 2006). Such variability in seed traits is often correlated with differences in germination parameters, which may enhance a species capacity to colonize diverse habitats and expand its geographic range (Ranieri et al., 2012). Understanding these variations is therefore essential for interpreting inter-population differences and predicting weed emergence dynamics. For this, various metrics, including total area, perimeter, circularity, and weight, were measured and compared. To obtain high-resolution images, 100 seeds were randomly selected from each population and scanned using an Expression 1100 XL scanner (EPSON, Suwa, Nagano, Japan). An object with known dimensions was included during scanning for subsequent analysis. The obtained images were processed using the ImageJ open-source software (https://imagej.net/ij/index.html) chosen for its established reliability, precision, and user-friendliness in previous studies (Schneider et al., 2012; Schroeder et al., 2021). Prior to measurements, the images were converted into black and white 8-byte images, assigning values exclusively to the seeds while excluding any extraneous elements. Calibration of the software was performed using the object with known dimensions scanned alongside the seeds, converting values from pixels to millimeters. To determine seed weight, four groups of 100 seeds from each population were measured using a Mettler PM100 laboratory precision balance (Mettler Toledo, Columbus, Ohio, USA).
All statistical analyses were performed in the R environment (version 4.3.2) (RStudio Team, 2023).
Tb and Ψb were assessed with the drc and drcSeedGerm packages using the methods proposed by Mesgaran (2019).
A thermal time model with sub-optimal temperatures was used to determine the base temperature, as in the work of Nikolić and Masin (2024) and Oveisi et al. (2024). The model first fits a sigmoid nonlinear regression to the cumulative data from each temperature:
Where:
G(t) = cumulative germination over time, t.
Gmax = maximum germination as t approaches infinity.
b = slope around the inflection point.
t50 = time at which germination is half Gmax.
It then calculates the time to germination:
Where:
t(g) = time to a given germination percentage.
g = germination percentage.
The model then calculates the germination rate (rapidity), GR, which is simply the reciprocal of the time to a given germination percentile:
And finally, it investigates the relationship between GR and temperature, T, for each g using a linear regression model of the form:
Where:
Tb = the base temperature (°C).
b = the slope.
A similar procedure was used to calculate the base water potential (Ψb), substituting the different temperatures with the different levels of water potential (from 0 to −2 MPa), while the temperature was a constant 24°C.
To determine whether there were significant differences in seed dimensions and weight, a one-way ANOVA was performed followed by Tukey’s HSD test (p <0.05) for post hoc analysis. Differences between different base temperatures (Tb) and base water potentials (Ψb), were analyzed using Students T test.
Base temperatures and base water potentials of the studied populations of A. artemisiifolia are shown in Table 1.
Table 1. Estimated base temperature and base water potential for different populations of A. artemisiifolia, the letters indicate a significant difference p-value < 0.05.
The analysis showed distinct trends in Tb across the tested populations (Table 1). Notably, the Italian population exhibited the lowest Tb and was significantly different from the Serbian and French populations. Differently, the French population displayed the highest Tb, but was not statistically different from the Serbian and Croatian populations. The Croatian population had noteworthy variability, as evident from the standard error values.
The base water potentials (Ψb) among A. artemisiifolia populations exhibited more pronounced variations than the observed differences in Tb (Table 1). The Ψb of the Italian and Croatian populations were the highest, with no significant difference between them. Conversely, the Serbian and French populations displayed considerably lower Ψb values, with the lowest being the one of the Serbian population.
The results of the one-way ANOVA on the physical properties are presented in Table 2.
Table 2. Results of the one-way ANOVA for different properties of the four A. artemisiifolia populations tested.
There were significant differences between the populations for every physical property tested (Figure 3). The seeds belonging to the French population were the largest, followed by the Croatian population and then by the Serbian and Italian, with seeds of a similar size. It can also be seen that the results concerning seed perimeter confirm the size comparison. However, although similar in area, it can be observed that the perimeter is different between the seeds of Serbian and Italian population of this weed species.
Figure 3. Seed area (A), seed perimeter (B), seed circularity (C), and the weight of 100 seeds (D) of different ecotypes of A. artemisiifolia. The letters indicate a significant difference p-value < 0.05. FR, France; IT,Italy; CRO, Croatia; SRB, Serbia.
Seed circularity is an insightful parameter offering information about seed shape. A value closer to 1 indicates a shape more akin to a perfect circle, while a value closer to 0 signifies a more elongated form. It is noteworthy that none of the tested populations exhibits perfectly round seeds. Among them, the Italian population comes closest to a circular shape, while the French population showcases the most elongated seeds.
Finally, the results show a distinctive seed weight for each population. Surprisingly, the seeds of the Croatian population, despite not being the largest, emerge as the heaviest among the populations. In contrast, the seeds of the French population, which are the largest in size, rank third in terms of weight. Remarkably, the lightest seeds are from the Italian population, aligning with the smaller size characteristic of this population’s seeds.
The results revealed disparities in both germination thresholds and physical properties among various populations of A. artemisiifolia. This aligns with prior studies highlighting the weed species considerable genetic and phenotypic variability (Leiblein-Wild and Tackenberg, 2014; Martin et al., 2016; Li et al., 2019). Rapid adaptation to new environments is a common trait among invasive weed species (Prentis et al., 2008; Clements and Jones, 2021; Gong et al., 2022). The introduction of A. artemisiifolia to Europe from its native habitat employed an adaptation strategy, establishing it as a formidable invasive species globally. Studies on A. artemisiifolia adaptation underscore its exceptionally high potential, with adaptations occurring rapidly, contributing significantly to its invasiveness (Battlay et al., 2023). These findings are in accordance with the results obtained in this study, revealing interesting differences and similarities among the studied populations.
The influence of climate and climate change on the behavior of A. artemisiifolia is well-documented (Hamaoui-Laguel et al., 2015; Case and Stinson, 2018; Gentili et al., 2019; Xian et al., 2023), consistent with the results obtained in this study. Analyzing meteorological data on minimum, maximum, and mean temperatures in the four areas (Figure 2) reveals that Turin and Zagreb experience similar temperatures. Conversely, Šabac tends to have higher temperatures in the spring-autumn period, while Dijon tends to have lower temperatures during the same period compared to Turin and Zagreb. This may explain the similarities in Tb for the Turin and Zagreb populations, although the Croatian population shows significant variability, indicating a more diverse response. Notably, the spring temperatures for Zagreb intersect with those of other places, potentially explaining its wide germination range.
For the Serbian and French populations, Šabac experiences very low winter temperatures that rise rapidly in spring, remaining high until autumn. While the temperatures in Dijon are consistently lower than in other areas, the consistently high minimum temperatures may explain why this population has the highest Tb. This is in accordance with different studies conducted on this matter, explaining how Tb is closely linked with the temperature of the habitat, indicating that the plants, even of the same species growing under different temperature regimes will have different Tb, ergo plants growing in colder climates will have lower Tb compared with those growing in warmer climates (Trudgill et al., 2000; Bao et al., 2019). This finding highlights important considerations for effective weed control strategies. For instance, in Dijon, the combination of consistently high and steady minimum temperatures during the early months corresponds to a population with a higher Tb. This likely delays seedling emergence compared to populations from warmer regions, as emergence depends on the accumulation of sufficient thermal time above Tb (Grundy, 2003; Baraibar et al., 2018). Such delayed emergence may help A. artemisiifolia in Dijon avoid frost damage or other unfavorable early-season conditions, allowing optimal emergence and development during warmer months. This pattern aligns with adaptive strategies observed in other weed species (Ramesh et al., 2017). However, this delayed emergence has practical implications for weed management, necessitating adjustments in the timing of control measures to coincide with peak seedling emergence. Emergence predictive models can play a crucial role in this regard. In contrast, populations with lower Tb might emerge earlier, leading to differences in developmental stages across regions, further emphasizing the need for region-specific management strategies. Examining the Ψb of the studied species and observing precipitation data in Figure 2, it is evident that Turin receives the most precipitation in April and May, aligning with June precipitation in Zagreb. This explains the high Ψb of the Italian population and its similarity to the Croatian one. In contrast, Šabac receives significantly less precipitation during the same period, establishing it as the driest area compared to others. This likely accounts for the low Ψb of the Serbian A. artemisiifolia population, indicating adaptation to drought conditions. As for Tb, similar studies were conducted on the relationship between the Ψb of plants and water availability in different habitats. These studies also concluded that the seeds developing in more arid conditions will have a more negative Ψb compared to those, even of the same species, growing in more humid conditions (Ceri and Etherington, 1991; Baskin and Baskin, 2014a; Bao et al., 2022). Interestingly, despite high precipitation in the Dijon area, its Ψb is the second lowest. This discrepancy may be attributed to lower temperatures in this region compared to others, emphasizing the crucial interaction between temperature and precipitation for germination thresholds. A similar finding can also be observed in the work by Dürr et al. (2015), who observed that species growing in cool conditions were more able to germinate in dry conditions than those growing in warmer conditions.
It is important to emphasize that the seeds used in these trials were the original seeds collected from their native sites and were not reproduced in a common garden or uniform environment. This approach was chosen to capture the seeds local responses, reflecting the base temperature (Tb) and base water potential (Ψb) specific to their place of origin. By including both external (climatic) and internal (genetic/maternal) effects influencing these parameters, this work aimed to provide results that are representative of real-world conditions. This is particularly critical given that emergence predictive models are designed for specific areas and require calibration to ensure their accuracy and efficiency in those environments (Masin et al., 2010, 2012, 2014; Šoštarčić et al., 2021b).
The results regarding the physical properties of the seeds are consistent with studies on invasive weed species, demonstrating high variability among different populations (Fenollosa et al., 2021). These findings parallel the results of germination thresholds and can be interpreted as an adaptation to different climatic conditions. Qiu et al. (2010) found a positive correlation between high precipitation and low temperatures, influencing seed size. This aligns with our results, as French seeds turned out to be the largest. The same authors also noted that larger seeds typically have lower temperature requirements for germination, contradicting our findings. However, our results align with those of Ge et al. (2020), who discovered that some larger seeds might have higher thermal requirements for germination.
Several studies investigating the influence of the origin of A. artemisiifolia have highlighted temperature as a major driver of seed variability between different populations. Typically, seeds from higher latitudes and lower temperatures produce larger seeds with lower germination potential compared to populations from lower latitudes and higher temperatures (Li et al., 2015; Zhou et al., 2021), consistent with the results obtained in this study.
In discussing the generalizability of our findings across different populations of A. artemisiifolia, it is important to consider the reasons behind the selection of the four populations used in this study. Previous research has shown that populations of A. artemisiifolia exhibit very low variability when situated within the same geographic niche, even when separated by several kilometers (Scalone et al., 2016; Song et al., 2023; Putra et al., 2024). A similar niche effect was also found by Farooq et al. (2019), according to the authors, this effect is a consequence of ecological adaptations to prevailing climatic conditions in the area. This consistency in population characteristics supports our decision to focus on populations from distinctly different regions across Europe. Additionally, the niche-filling tendencies of this species imply that significant variations are more likely to arise from populations that are very far apart or exposed to very different conditions, differences and similarities between the populations uncovered in this work are clear evidence in support of this claim. This context is crucial as it not only underpins the reliability of our data, suggesting that the observed differences in germination thresholds and seed properties are likely attributable to regional climatic conditions rather than mere geographic proximity. But it also highlights one of the main limitations of emergence predictive models—‘one model cannot fit all’—emphasizing the need to develop specific models tailored to different areas.
The results presented here broaden our understanding of the behavior of this important invasive species, but can also be considered as an important contribution to effective control strategies. As it was mentioned previously, different control strategies for A. artemisiifolia are present, including chemical, mechanical and biological methods, that can also be combined, with varying degrees of effectiveness (Buttenschøn et al., 2009; Gerber et al., 2011; Essl et al., 2015). Considering that the chemical weed control methods are often the most effective ones, it is no surprise that they are the most used ones, also for the control of this species. However, the need to control A. artemisiifolia also outside of the strictly agricultural context, both effective and environmentally friendly control becomes particularly challenging (Essl et al., 2015; Wang et al., 2022).
Using the temperature and water potential thresholds determined in this work, it could be possible to create emergence prediction models for the tested areas, to assist the farmers and authorities in choosing the best timing for control operations, thus maximizing the effectiveness and reducing the excessive use of herbicides (Masin et al., 2010, 2012). Moreover, the same principle could also be applied for mechanical control methods and integrated weed management (IWM) strategies, providing the species emergence percentage in time. An example of the usefulness of choosing the right time for weed control operations can be observed in the work of Beam et al. (2021), who found that the effectiveness of A. artemisiifolia control using IWM strategies strongly depends on the time of their application. Having in mind that today the timing of control operations against this weed species is decided arbitrarily, an emergence predictive model based on the data obtained in this study could significantly improve this weed management strategy.
This study sheds light on the germination thresholds and diversity among different European populations of A. artemisiifolia, offering crucial insights into the variability of this invasive species. The results demonstrated significant differences in germination thresholds, with the Italian population exhibiting the lowest base temperature (0.58°C), while the French population demonstrated the highest (2.74°C), and the Serbian population showing the lowest base water potential (−1.44 MPa), while the Italian one exhibited the highest (−0.78). Additionally, substantial disparities were also observed in seed physical properties, where the Italian population had the smallest and lightest seeds, while the French population had the largest and most elongated seeds. Given its high adaptability and the associated threats to human and animal health, as well as agricultural production, the findings presented in this research carry substantial importance.
By addressing the gap in knowledge regarding the differentiation of A. artemisiifolia at a European level, considering diverse climatic conditions and geographic origins, this study contributes valuable information for the effective management of this weed species. The germination thresholds obtained can serve as foundational data for constructing emergence predictive models or enhancing existing ones, which are vital for timely control measures against this agricultural and health hazard in Europe.
Moreover, understanding the levels of seed variability provides a basis for refining control strategies and mitigating the spread of this invasive species. While this study offers valuable insights, further research is needed, including more populations from various European countries. Future studies, particularly those simulating the effects of climate change, will contribute to a more comprehensive understanding of A. artemisiifolia’s adaptability. Such insights are crucial for anticipating the species’ impact on agricultural production and the overall well-being of European communities.
The original contributions presented in the study are included in the article/supplementary material. Further inquiries can be directed to the corresponding author.
NN: Conceptualization, Data curation, Formal analysis, Investigation, Methodology, Resources, Software, Validation, Writing – original draft. VŠ: Conceptualization, Data curation, Investigation, Methodology, Resources, Validation, Writing – review & editing. MŠ: Conceptualization, Resources, Supervision, Visualization, Writing – review & editing. RM: Conceptualization, Formal analysis, Project administration, Resources, Supervision, Visualization, Writing – review & editing.
The author(s) declare that financial support was received for the research, authorship, and/or publication of this article. Open Access funding provided by Università degli Studi di Padova | University of Padua, Open Science Committee.
The authors are grateful to UMR1347 Agroècologie (INRA Dijon) for providing us with the seeds of the French population of A. artemisiifolia.
The authors declare that the research was conducted in the absence of any commercial or financial relationships that could be construed as a potential conflict of interest.
All claims expressed in this article are solely those of the authors and do not necessarily represent those of their affiliated organizations, or those of the publisher, the editors and the reviewers. Any product that may be evaluated in this article, or claim that may be made by its manufacturer, is not guaranteed or endorsed by the publisher.
Afonin A. N., Luneva N. N., Fedorova Y. A., Kletchkovskiy Y. E., Chebanovskaya A. F. (2018). History of introduction and distribution of common ragweed (Ambrosia artemisiifolia L.) in the European part of the Russian Federation and in the Ukraine. EPPO. Bull. 48, 266–273. doi: 10.1111/epp.12484
Arnold C. Y. (1959). The determination and significance of the base temperature in a linear heat unit system. Proc. Am. Soc. Hortic. Sci. 74, 430–445.
Bao G., Song M., Wang Y., Saikkonen K., Wang H. (2019). Interactive effects of Epichloë fungal and host origins on the seed germination of Achnatherum inebrians. Symbiosis 79, 49–58. doi: 10.1007/s13199-019-00636-0
Bao G., Zhang P., Wei X. X., Zhang Y., Liu W. (2022). Comparison of the effect of temperature and water potential on the seed germination of five Pedicularis kansuensis populations from the Qinghai–Tibet plateau. Front. Plant Sci. 13. doi: 10.3389/fpls.2022.1052954
Baraibar B., Mortensen D. A., Hunter M. C., Barbercheck M. E., Kaye J. P., Finney D. M., et al. (2018). Growing degree days and cover crop type explain weed biomass in winter cover crops. Agron. Sustain. Dev. 38. doi: 10.1007/s13593-018-0543-1
Baskin C. C., Baskin J. M. (2014a). Biogeographical and evolutionary aspects of seed dormancy. Seeds. 1005–1073. doi: 10.1016/B978-0-12-416677-6.00012-3
Baskin C. C., Baskin J. M. (2014b). “Ecologically meaningful germination studies,” in Seeds (Elsevier, Amsterdam, The Netherlands), 5–35. doi: 10.1016/B978-0-12-416677-6.00002-0
Battlay P., Wilson J., Bieker V. C., Lee C., Prapas D., Petersen B., et al. (2023). Large haploblocks underlie rapid adaptation in the invasive weed Ambrosia artemisiifolia. Nat. Commun. 14. doi: 10.1038/s41467-023-37303-4
Beam S. C., Cahoon C. W., Haak D. C., Holshouser D. L., Mirsky S. B., Flessner M. L. (2021). Integrated weed management systems to control common ragweed (Ambrosia artemisiifolia L.) in soybean. Front. Agron. 2. doi: 10.3389/fagro.2020.598426
Brandes D., Nitzsche J. (2006). Biology, introduction, dispersal, and distribution of common ragweed (Ambrosia artemisiifolia L.) with special regard to. Nachrichtenbl. Deut. Pflanzenschutzd. 58, 286–291.
Buttenschøn R. M., Waldispühl S., Bohren C. (2009). Guidelines for management of common ragweed, Ambrosia artemisiifolia, 27_SMS. Available at: http:/www.EUPHRESCO.org.
Case M. J., Stinson K. A. (2018). Climate change impacts on the distribution of the allergenic plant, common ragweed (Ambrosia artemisiifolia) in the eastern United States. PloS One 13, 1–12. doi: 10.1371/journal.pone.0205677
Ceri E., Etherington J. (1991). The effect of soil water potential on seedling growth of some British plants. New Phytol. 118, 571–579. doi: 10.1111/j.1469-8137.1991.tb00998.x
Chapman D. S., Makra L., Albertini R., Bonini M., Páldy A., Rodinkova V., et al. (2016). Modelling the introduction and spread of non-native species: international trade and climate change drive ragweed invasion. Glob. Change Biol. 22, 3067–3079. doi: 10.1111/gcb.13220
Chauvel B., Dessaint F., Cardinal-Legrand C., Bretagnolle F. (2006). The historical spread of Ambrosia artemisiifolia L. @ in France from herbarium records. J. Biogeogr. 33, 665–673. doi: 10.1111/j.1365-2699.2005.01401.x
Clements D. R., Jones V. L. (2021). Rapid evolution of invasive weeds under climate change: present evidence and future research needs. Front. Agron. 3. doi: 10.3389/fagro.2021.664034
Colbach N., Chauvel B., Gauvrit C., Munier-Jolain N. M. (2007). Construction and evaluation of ALOMYSYS modelling the effects of cropping systems on the blackgrass life-cycle: From seedling to seed production. Ecol. Modell. 201, 283–300. doi: 10.1016/j.ecolmodel.2006.09.018
Cowbrough M. J., Brown R. B., Tardif F. J. (2003). Impact of common ragweed (Ambrosia artemisiifolia) aggregation on economic thresholds in soybean. Weed. Sci. 51, 947–954. doi: 10.1614/02-036
Csontos P., Vitalos M., Barina Z., Kiss L. (2010). Early distribution and spread of Ambrosia artemisiifolia in Central and Eastern Europe. Bot. Helv. 120, 75–78. doi: 10.1007/s00035-010-0072-2
Cunze S., Leiblein M. C., Tackenberg O. (2013). Range expansion of ambrosia artemisiifolia in europe is promoted by climate change. ISRN. Ecol. 2013, 1–9. doi: 10.1155/2013/610126
Dahal P., Bradford K. J. (1994). Hydrothermal time analysis of tomato seed germination at suboptimal temperature and reduced water potential. Seed. Sci. Res. 4, 71–80. doi: 10.1017/S096025850000204X
Dinelli G., Marotti I., Catizone P., Bosi S., Tanveer A., Abbas R., et al. (2013). Germination ecology of Ambrosia artemisiifolia L. and Ambrosia trifida L. biotypes suspected of glyphosate resistance. Open Life Sci. 8. doi: 10.2478/s11535-013-0135-z
Dong H., Song Z., Liu T., Liu Z., Liu Y., Chen B., et al. (2020). Causes of differences in the distribution of the invasive plants Ambrosia artemisiifolia and Ambrosia trifida in the Yili Valley, China. Ecol. Evol. 10, 13122–13133. doi: 10.1002/ece3.6902
Dürr C., Dickie J. B., Yang X. Y., Pritchard H. W. (2015). Ranges of critical temperature and water potential values for the germination of species worldwide: Contribution to a seed trait database. Agric. For. Meteorol. 200, 222–232. doi: 10.1016/j.agrformet.2014.09.024
Essl F., Biró K., Brandes D., Broennimann O., Bullock J. M., Chapman D. S., et al. (2015). Biological flora of the british isles: ambrosia artemisiifolia. J. Ecol. 103, 1069–1098. doi: 10.1111/1365-2745.12424
Farooq S., Onen H., Ozaslan C., Baskin C. C., Gunal H. (2019). Seed germination niche for common ragweed (Ambrosia artemisiifolia L.) populations naturalized in Turkey. South Afr. J. Bot. 123, 361–371. doi: 10.1016/j.sajb.2019.03.031
Fenollosa E., Jené L., Munné-Bosch S. (2021). Geographic patterns of seed trait variation in an invasive species: how much can close populations differ? Oecologia 196, 747–761. doi: 10.1007/s00442-021-04971-2
Forcella F., Benech Arnold R. L., Sanchez R., Ghersa C. M. (2000). Modeling seedling emergence. F. Crop Res. 67, 123–139. doi: 10.1016/S0378-4290(00)00088-5
Galzina N., Baric K., Šcepanovic M., Goršic M., Ostojic Z. (2010). Distribution of invasive weed Ambrosia artemisiifolia L. @ in Croatia. Agric. Conspec. Sci. 75, 75–81.
Gard B., Bretagnolle F., Dessaint F., Laitung B. (2013). Invasive and native populations of common ragweed exhibit strong tolerance to foliar damage. Basic. Appl. Ecol. 14, 28–35. doi: 10.1016/J.BAAE.2012.10.007
Gardin A., Otero O., Réveillac E., Lafitte A., Valentin X., Lapalus F., et al. (2023). Seasonality and growth in tropical freshwater ectotherm vertebrates: Results from 1-year experimentation in the African gray bichir, giraffe catfish, and the West African mud turtle. Ecol. Evol. 13, 1–17. doi: 10.1002/ece3.9936
Ge W., Bu H., Wang X., Martinez S. A., Du G. (2020). Inter- and intra-specific difference in the effect of elevation and seed mass on germinability of eight Allium species. Glob. Ecol. Conserv. 22, e01016. doi: 10.1016/j.gecco.2020.e01016
Gentili R., Asero R., Caronni S., Guarino M., Montagnani C., Mistrello G., et al. (2019). Ambrosia artemisiifolia L. temperature-responsive traits influencing the prevalence and severity of pollinosis: A study in controlled conditions. BMC Plant Biol. 19, 1–9. doi: 10.1186/s12870-019-1762-6
Gentili R., Gilardelli F., Bona E., Prosser F., Selvaggi A., Alessandrini A., et al. (2017). Distribution map of Ambrosia artemisiifolia L. (Asteraceae) in Italy. Plant Biosyst. 151, 381–386. doi: 10.1080/11263504.2016.1176966
Genton B. J., Shykoff J. A., Giraud T. (2005). High genetic diversity in French invasive populations of common ragweed, Ambrosia artemisiifolia, as a result of multiple sources of introduction. Mol. Ecol. 14, 4275–4285. doi: 10.1111/J.1365-294X.2005.02750.X
Gerber E., Schaffner U., Gassmann A., Hinz H. L., Seier M., Müller-Schärer H. (2011). Prospects for biological control of Ambrosia artemisiifolia in Europe: Learning from the past. Weed. Res. 51, 559–573. doi: 10.1111/j.1365-3180.2011.00879.x
Goeden R. D., Andrès L. A. (1999). Biological control of weeds in terrestrial and aquatic environments. Handb. Biol. Control. 6, 871–890. doi: 10.1016/b978-012257305-7/50081-3
Gong W., Wang Y., Chen C., Xiong Y., Zhou Y., Xiao F., et al. (2022). The rapid evolution of an invasive plant due to increased selection pressures throughout its invasive history. Ecotoxicol. Environ. Saf. 233. doi: 10.1016/j.ecoenv.2022.113322
Grundy A. C. (2003). Predicting weed emergence: a review of approaches and future challenges. Weed. Res. 43, 1–11. doi: 10.1046/j.1365-3180.2003.00317.x
Guillemin J.-P., Gardarin A., Granger S., Reibel C., Munier-Jolain N. M., Colbach N. (2013). Assessing potential germination period of weeds with base temperatures and base water potentials. Weed. Res. 53, 76–87. doi: 10.1111/wre.12000
Hamaoui-Laguel L., Vautard R., Liu L., Solmon F., Viovy N., Khvorostyanov D., et al. (2015). Effects of climate change and seed dispersal on airborne ragweed pollen loads in Europe. Nat. Clim. Chang. 58 5, 766–771. doi: 10.1038/nclimate2652
Kaufmann M., Michel B. (1973). The osmotic potential of polyethylene glycol 6000. Plant Physiol. 51, 914–916. doi: 10.1104/pp.51.5.914
Kiss L., Béres I. (2006). Anthropogenic factors behind the recent population expansion of common ragweed (Ambrosia artemisiifolia L.) in Eastern Europe: Is there a correlation with political transitions? [3. J. Biogeogr. 33, 2156–2157. doi: 10.1111/j.1365-2699.2006.01633.x
Knolmajer B., Jócsák I., Taller J., Keszthelyi S., Kazinczi G. (2024). Common ragweed—Ambrosia artemisiifolia L.: A review with special regards to the latest results in biology and ecology. Agronomy 14. doi: 10.3390/agronomy14030497
Kottek M., Grieser J., Beck C., Rudolf B., Rubel F. (2006). World map of the Köppen-Geiger climate classification updated. Meteorol. Z. 15, 259–263. doi: 10.1127/0941-2948/2006/0130
Leiblein M. C., Lösch R. (2011). Biomass development and CO2 gas exchange of Ambrosia artemisiifolia L. under different soil moisture conditions. Flora. Morphol. Distrib. Funct. Ecol. Plants 206, 511–516. doi: 10.1016/j.flora.2010.09.011
Leiblein-Wild M. C., Tackenberg O. (2014). Phenotypic variation of 38 European Ambrosia artemisiifolia populations measured in a common garden experiment. Biol. Invasions. 16, 2003–2015. doi: 10.1007/s10530-014-0644-y
Leishman M. R. (2001). Oikos - 2003 - Leishman - Does the seed size number trade-off model determine plant community structure An assessment of.pdf. OIKOS 93, 294–302. doi: 10.1034/j.1600-0706.2001.930212.x
Leishman M. R., Westoby M., Jurado E. (1995). Correlates of seed size variation: A comparison among five temperate floras. J. Ecol. 83, 517. doi: 10.2307/2261604
Leskovšek R., Datta A., Knezevic S. Z., Simončič A. (2012). Common ragweed (Ambrosia artemisiifolia) dry matter allocation and partitioning under different nitrogen and density levels. Weed. Biol. Manage. 12, 98–108. doi: 10.1111/J.1445-6664.2012.00439.X
Li X. M., She D. Y., Zhang D. Y., Liao W. J. (2015). Life history trait differentiation and local adaptation in invasive populations of Ambrosia artemisiifolia in China. Oecologia 177, 669–677. doi: 10.1007/s00442-014-3127-z
Li F., van Kleunen M., Li J., Liu X., Gao K., Zhu J., et al. (2019). Patterns of genetic variation reflect multiple introductions and pre-admixture sources of common ragweed (Ambrosia artemisiifolia) in China. Biol. Invasions. 21, 2191–2209. doi: 10.1007/s10530-019-01966-2
Loddo D., Ghaderi-Far F., Rastegar Z., Masin R. (2018). Base temperatures for germination of selected weed species in Iran. Plant Prot. Sci. 54, 60–66. doi: 10.17221/92/2016-PPS
Loddo D., Sousa E., Masin R., Calha I., Zanin G., Fernández-Quintanilla C., et al. (2013). Estimation and Comparison of Base Temperatures for Germination of European Populations of Velvetleaf (Abutilon theophrasti) and Jimsonweed (Datura stramonium). Weed. Sci. 61, 443–451. doi: 10.1614/ws-d-12-00162.1
Máčajová P., Tóthová M., Krchňavá V., Týr Š., Tóth P. (2022). Herbicide control of Ambrosia artemisiifolia in sunflower, soybean and maize. Agric. 68, 110–118. doi: 10.2478/agri-2022-0010
Martin M. D., Olsen M. T., Samaniego J. A., Zimmer E. A., Gilbert M. T. P. (2016). The population genomic basis of geographic differentiation in North American common ragweed (Ambrosia artemisiifolia L.). Ecol. Evol. 6, 3760–3771. doi: 10.1002/ece3.2143
Masin R., Cacciatori G., Zuin M. C., Zanin G. (2010). AlertInf: Emergence predictive model for weed control in maize in Veneto. Ital. J. Agrometeorol. 5.
Masin R., Loddo D., Benvenuti S., Otto S., Zanin G. (2012). Modeling weed emergence in italian maize fields. Weed. Sci. 60, 254–259. doi: 10.1614/WS-D-11-00124.1
Masin R., Loddo D., Gasparini V., Otto S., Zanin G. (2014). Evaluation of weed emergence model alertInf for maize in soybean. Weed. Sci. 62, 360–369. doi: 10.1614/WS-D-13-00112.1
Masin R., Zuin M. C., Archer D. W., Forcella F., Zanin G. (2005). WeedTurf: a predictive model to aid control of annual summer weeds in turf. Weed. Sci. 53, 193–201. doi: 10.1614/WS-04-066R1
Mesgaran M. (2019).Modeling weed seed germination and seedling emergence. In: RPubs - Seed Germination Emerg. Available online at: https://rpubs.com/mbmesgaran/495385 (Accessed February 10, 2021).
Milakovic I., Fiedler K., Karrer G. (2014). Management of roadside populations of invasive Ambrosia artemisiifolia by mowing. Weed. Res. 54, 256–264. doi: 10.1111/wre.12074
Milakovic I., Karrer G. (2016). The influence of mowing regime on the soil seed bank of the invasive plant Ambrosia artemisiifolia L. NeoBiota 28, 39–49. doi: 10.3897/neobiota.28.6838
Moles A. T., Westoby M. (2006). Seed size and plant strategy across the whole life cycle. Oikos 113, 91–105. doi: 10.1111/j.0030-1299.2006.14194.x
Montagnani C., Gentili R., Smith M., Guarino M. F., Citterio S. (2017). The worldwide spread, success, and impact of ragweed (Ambrosia spp.). CRC. Crit. Rev. Plant Sci. 36, 139–178. doi: 10.1080/07352689.2017.1360112
Murali K. S. (1997). Patterns of seed size, germination and seed viability of tropical tree species in Southern India. Biotropica 29, 271–279. doi: 10.1111/j.1744-7429.1997.tb00428.x
Nikolić N., Masin R. (2024). Exploring germination requirements of Iva xanthiifolia: Implications for potential spread in Europe. Plant Stress 11, 100312. doi: 10.1016/j.stress.2023.100312
Norden N., Daws M. I., Antoine C., Gonzalez M. A., Garwood N. C., Chave J. (2009). The relationship between seed mass and mean time to germination for 1037 tree species across five tropical forests. Funct. Ecol. 23, 203–210. doi: 10.1111/j.1365-2435.2008.01477.x
Onofri A., Gresta F., Tei F. (2010). A new method for the analysis of germination and emergence data of weed species. Weed. Res. 50, 187–198. doi: 10.1111/j.1365-3180.2010.00776.x
Oveisi M., Alizadeh H., Lorestani S. A., Esmaili A., Sadeghnejad N., Piri R., et al. (2024). Triangle area model (TAM) for predicting germination: An approach to enhance hydrothermal time model applications. Curr. Plant Biol. 39. doi: 10.1016/j.cpb.2024.100356
Pichtel J., Kuroiwa K., Sawyerr H. T. (2000). Distribution of Pb, Cd and Ba in soils and plants of two contaminated sites. Environ. pollut. 110, 171–178. doi: 10.1016/S0269-7491(99)00272-9
Prentis P. J., Wilson J. R. U., Dormontt E. E., Richardson D. M., Lowe A. J. (2008). Adaptive evolution in invasive species. Trends Plant Sci. 13, 288–294. doi: 10.1016/j.tplants.2008.03.004
Puteh A. B., Rosli R., Mohamad R. B. (2010). Dormancy and cardinal temperatures during seed germination of five weedy rice (oryza spp.) strains. Pertanika. J. Trop. Agric. Sci. 33, 243–250.
Putra A. R., Hodgins K. A., Fournier-Level A. (2024). Assessing the invasive potential of different source populations of ragweed (Ambrosia artemisiifolia L.) through genomically informed species distribution modelling. Evol. Appl. 17, 1–13. doi: 10.1111/eva.13632
Qiu J., Bai Y., Fu Y. B., Wilmshurst J. F. (2010). Spatial variation in temperature thresholds during seed germination of remnant Festuca hallii populations across the Canadian prairie. Environ. Exp. Bot. 67, 479–486. doi: 10.1016/j.envexpbot.2009.09.002
Ramesh K., Matloob A., Aslam F., Florentine S. K., Chauhan B. S. (2017). Weeds in a changing climate: Vulnerabilities, consequences, and implications for future weed management. Front. Plant Sci. 8. doi: 10.3389/fpls.2017.00095
Ranieri B. D., Pezzini F. F., Garcia Q. S., Chautems A., França M. G. C. (2012). Testing the regeneration niche hypothesis with Gesneriaceae (tribe Sinningiae) in Brazil: Implications for the conservation of rare species. Austral Ecol. 37, 125–133. doi: 10.1111/j.1442-9993.2011.02254.x
Rodriguez C. M., D’Alessandro M. (2019). Indoor thermal comfort review: The tropics as the next frontier. Urban. Clim. 29, 100488. doi: 10.1016/j.uclim.2019.100488
RStudio Team (2023). RStudio | Open source & professional software for data science teams - RStudio (RStudio Inc). Available online at: https://rstudio.com/ (Accessed February 10, 2021).
Saint-Louis S., DiTommaso A., Watson A. K. (2005). A common ragweed (Ambrosia artemisiifolia) biotype in southwestern québec resistant to linuron. Weed. Technol. 19, 737–743. doi: 10.1614/wt-04-276.1
Scalone R., Lemke A., Štefanić E., Kolseth A. K., Rašić S., Andersson L. (2016). Phenological variation in ambrosia artemisiifolia L. Facilitates near future establishment at northern latitudes. PloS One 11, 1–15. doi: 10.1371/journal.pone.0166510
Schneider C. A., Rasband W. S., Eliceiri K. W. (2012). NIH Image to ImageJ: 25 years of image analysis. Nat. Methods 9, 671–675. doi: 10.1038/nmeth.2089
Schroeder A. B., Dobson E. T. A., Rueden C. T., Tomancak P., Jug F., Eliceiri K. W. (2021). The ImageJ ecosystem: Open-source software for image visualization, processing, and analysis. Protein Sci. 30, 234–249. doi: 10.1002/pro.3993
Scruggs E., Sciences E., Tech V., Tech V. (2019). Common ragweed (Ambrosia artemisiifolia) control in soybeans. Blacksburg, VA: Virginia Cooperative Extension SPES143NP.
Silveira F. A. O., Negreiros D., Araújo L. M., Fernandes G. W. (2012). Does seed germination contribute to ecological breadth and geographic range? A test with sympatric Diplusodon (Lythraceae) species from rupestrian fields. Plant Species. Biol. 27, 170–173. doi: 10.1111/j.1442-1984.2011.00342.x
Silvertown J., Bullock J. M. (2003). Do seedlings in gaps interact? A field test of assumptions in ESS seed size models. Oikos 101, 499–504. doi: 10.1034/j.1600-0706.2003.12247.x
Skálová H., Guo W. Y., Wild J., Pyšek P. (2017). Ambrosia artemisiifolia in the Czech Republic: History of invasion, current distribution and prediction of future spread. Preslia 89, 1–16. doi: 10.23855/preslia.2017.001
Smith M., Cecchi L., Skjøth C. A., Karrer G., Šikoparija B. (2013). Common ragweed: A threat to environmental health in Europe. Environ. Int. 61, 115–126. doi: 10.1016/j.envint.2013.08.005
Song X. J., Liu G., Qian Z. Q., Zhu Z. H. (2023). Niche Filling Dynamics of Ragweed (Ambrosia artemisiifolia L.) during Global Invasion. Plants 12, 1–16. doi: 10.3390/plants12061313
Šoštarčić V., Masin R., Loddo D., Brijačak E., Šćepanović M. (2021a). Germination parameters of selected summer weeds: Transferring of the alertinf model to other geographical regions. Agronomy 11. doi: 10.3390/agronomy11020292
Šoštarčić V., Masin R., Loddo D., Svečnjak Z., Rubinić V., Šćepanović M. (2021b). Predicting the emergence of echinochloa crus-galli (L.) p. beauv. in maize crop in Croatia with hydrothermal model. Agronomy 11. doi: 10.3390/agronomy11102072
Stradford J., Sakhare A., Ravichandran R., Schroeder E. T., Michener L. A., Pa J. (2021). Conducting a VR clinical trial in the era of COVID-19. Front. Virtual. Real. 2. doi: 10.3389/frvir.2021.639478
Sun Y., Bossdorf O., Grados R. D., Liao Z. Y., Müller-Schärer H. (2020). Rapid genomic and phenotypic change in response to climate warming in a widespread plant invader. Glob. Change Biol. 26, 6511–6522. doi: 10.1111/gcb.15291
Taylor R. A. J. (2019). Plants (Elsevier: Taylor’s Power Law), 103–142. doi: 10.1016/B978-0-12-810987-8.00006-9
Trudgill D. L., Squire G. R., Thompson K. (2000). A thermal time basis for comparing the germination requirements of some British herbaceous plants. New Phytol. 145, 107–114. doi: 10.1046/j.1469-8137.2000.00554.x
van Boheemen L. A., Lombaert E., Nurkowski K. A., Gauffre B., Rieseberg L. H., Hodgins K. A. (2017). Multiple introductions, admixture and bridgehead invasion characterize the introduction history of Ambrosia artemisiifolia in Europe and Australia. Mol. Ecol. 26, 5421–5434. doi: 10.1111/mec.14293
Van Mölken T., Jorritsma-Wienk L. D., Van Hoek P. H. W., De Kroon H. (2005). Only seed size matters for germination in different populations of the dimorphic Tragopogon pratensis subsp. pratensis (Asteraceae). Am. J. Bot. 92, 432–437. doi: 10.3732/ajb.92.3.432
Wang H., Liu T., Zhao W., Liu X., Sun M., Su P., et al. (2022). Reduced invasiveness of common ragweed (Ambrosia artemisiifolia) using low-dose herbicide treatments for high-efficiency and eco-friendly control. Front. Plant Sci. 13. doi: 10.3389/fpls.2022.861806
Weaver S. E. (2001). Impact of lamb’s-quarters, common ragweed and green foxtail on yield of corn and soybean in Ontario. Can. J. Plant Sci. 81, 821–828. doi: 10.4141/P01-057
Xian X., Zhao H., Wang R., Huang H., Chen B., Zhang G., et al. (2023). Climate change has increased the global threats posed by three ragweeds (Ambrosia L.) in the Anthropocene. Sci. Total. Environ. 859, 160252. doi: 10.1016/j.scitotenv.2022.160252
Zhou L., Yu H., Yang K., Chen L., Yin W., Ding J. (2021). Latitudinal and longitudinal trends of seed traits indicate adaptive strategies of an invasive plant. Front. Plant Sci. 12. doi: 10.3389/fpls.2021.657813
Keywords: invasive species, germination thresholds, seed properties, climate adaptation, weed management strategies
Citation: Nikolić N, Šoštarčić V, Šćepanović M and Masin R (2025) Exploring germination thresholds and seed properties of Ambrosia artemisiifolia populations from different European regions for improving control strategies. Front. Agron. 6:1496841. doi: 10.3389/fagro.2024.1496841
Received: 15 September 2024; Accepted: 09 December 2024;
Published: 02 January 2025.
Edited by:
Thomas R. Butts, Purdue University, United StatesReviewed by:
Fernando H. Oreja, Oregon State University, United StatesCopyright © 2025 Nikolić, Šoštarčić, Šćepanović and Masin. This is an open-access article distributed under the terms of the Creative Commons Attribution License (CC BY). The use, distribution or reproduction in other forums is permitted, provided the original author(s) and the copyright owner(s) are credited and that the original publication in this journal is cited, in accordance with accepted academic practice. No use, distribution or reproduction is permitted which does not comply with these terms.
*Correspondence: Nebojša Nikolić, bmVib2pzYS5uaWtvbGljQHVuaXBkLml0
Disclaimer: All claims expressed in this article are solely those of the authors and do not necessarily represent those of their affiliated organizations, or those of the publisher, the editors and the reviewers. Any product that may be evaluated in this article or claim that may be made by its manufacturer is not guaranteed or endorsed by the publisher.
Research integrity at Frontiers
Learn more about the work of our research integrity team to safeguard the quality of each article we publish.