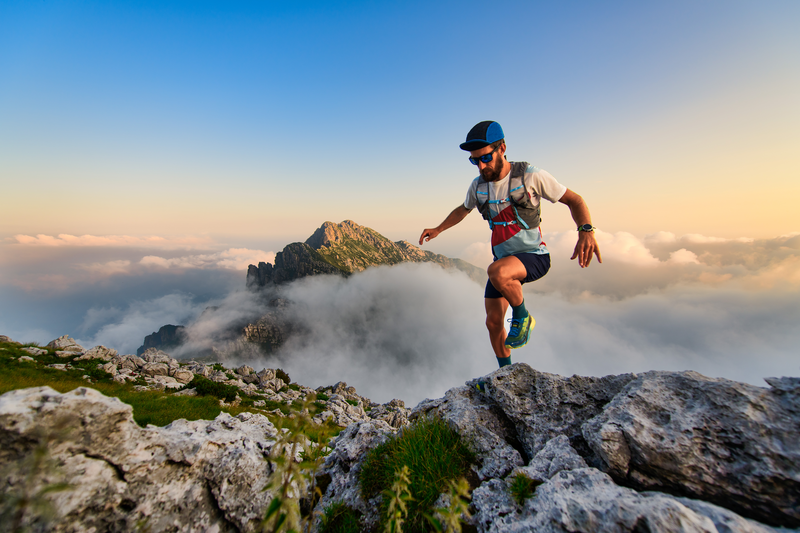
95% of researchers rate our articles as excellent or good
Learn more about the work of our research integrity team to safeguard the quality of each article we publish.
Find out more
ORIGINAL RESEARCH article
Front. Agron. , 10 December 2024
Sec. Plant-Soil Interactions
Volume 6 - 2024 | https://doi.org/10.3389/fagro.2024.1492505
Agricultural productivity in semi-arid regions is constrained by soil and groundwater salinity. This study quantified the desalinization potential of cut-soiler-constructed preferential shallow sub-surface drainage (PSSD) and its impact on crop performance in saline agroecologies. The study was conducted at the Indian Council of Agricultural Research (ICAR)-Central Soil Salinity Research Institute, Karnal, India, from 2019-2021. The rice residue-filled PSSD was simulated manually (60 cm depth) in a split-split plot experiment with saline and normal soil under saline water irrigation (4, 8, and 12 dS m-1). Cut-soiler PSSD were constructed in the middle of the lysimeter using rice residue (equivalent to 6 Mg ha-1) as the filling material with an outlet to quantify salt and water outflow. Pearl millet [Pennisetum glaucum L. (R. Br)], variety HHB-197, and mustard (Brassica juncea L.), variety CS-58, were grown in the rainy (Kharif) and winter (Rabi) seasons, respectively. The soil salinity profile decreased by 23.3%-58.5% with cut-soiler PSSD in different soils. The decrease in salinity was associated with increased plant height, dry matter accumulation (DMA), net assimilation rate (NAR), crop growth rate (CGR), relative growth rate (RGR), leaf area (LA), and leaf area Index (LAI) in both crops. The pearl millet and mustard yield increases in the two soil types were 11.6%-43.3% and 26%-36%, respectively using saline water up to 12 dS m-1. This study concludes that cut-soiler PSSD can effectively mitigate salinity stress and utilize saline water at the farm scale in salt-affected semi-arid agro-eco-regions.
Food and water security are under threat in many parts of the world from frequent and intense extreme weather events causing drought, floods, and associated loss of vital natural ecosystems (Cooper, 2023; Shrivastava and Kumar, 2015). Approximately 351.5 million hectares (M ha) of saline and 581.0 M ha of sodic soils have given rise to a total of 932.5 M ha of salt-affected soils across the globe (Szabolcs, 1989; FAO, 2017; Pessarakli and Szabolcs, 2019). Globally, US$12 to 27.3 billion are lost annually due to salinity-associated reductions in crop productivity (Qadir et al., 2014; Wang, 2021). Agricultural and agroecosystem productivity, especially in semi-arid and arid regions worldwide, is challenged by a scarcity of fresh water and secondary salinization (Lakhdar et al., 2009). In India, approximately 6.73 M ha of land is affected by different levels of salinity and sodicity (Sharma et al., 2015). Furthermore, at present, approximately 32%-84% of groundwater in various states is affected by salinity and/or sodicity (Choudhary and Kharche, 2018). The projected effects of climate change also have a direct impact on soil salinization because of upward salt movement under increased temperature conditions. The situation is further worsened in different regions due to the use of poor-quality groundwater for irrigation (Minhas, 1996). Arid to semi-arid climatic conditions and irrigation with saline groundwater without provision of adequate drainage result in an accumulation of salts in the soil profile and pose risks to sustainable crop production (Qadir et al., 2009), environmental health, and financial welfare (Diaz et al., 2021; Wei et al., 2021). Root zone salinity adversely alters the soil solution composition, adversely affecting the nutrient supply capacity and productivity of the soil (Soni et al., 2021; Fu et al., 2020; Dong et al., 2008).
Pearl millet-mustard is one of the important cropping systems in arid and semi-arid regions (Choudhary et al., 2017). Pearl millet (Pennisetum glaucum L.), being a drought- and salinity-tolerant, highly vigorous, and quick-growing crop, serves as a staple diet for the vast majority of the population and is an important fodder for livestock in these regions (Krishnamurthy et al., 2007). Mustard [Brassica juncea (L.) Czern and Coss.], (2n=36) is also a very important oilseed crop, mainly cultivated for edible oil, in addition to being used as a spice, consumed as a leafy vegetable, and used for condiments and fodder. Nevertheless, the productivity of these two crops is far below their potential in salt-affected conditions in semi-arid regions. A high soil salinity (10 dS m-1) is reported to cause up to ~31% decrease in seed setting and grain yield (GY) (Prakash et al., 2021). The stresses due to the salinity of soil and water contribute to greater yield losses in both seed and oil yields (Singh and Sharma, 2016). Salinity stress significantly reduces plant height and a high Na/K ratio in pearl millet and wheat (Yadav et al., 2020). Similarly, irrigation with saline water of 12 dS m-1 led to a 38.7% decrease in pearl millet grain yield (Heidari and Jamshidi, 2011). Salinity stress interferes with plant metabolism through decreased nutrient uptake, impaired photosynthetic machinery, a reduction in the photosynthetic rate, suppressed assimilated partitioning, translocation of photosynthates from the source to the sink, and pre-mature leaf senescence, causing appreciable decreases in crop growth and yield (Wahid et al., 2005; Tripathi et al., 2010; Soni et al., 2021). Managing root zone salinity within a critical range for the crops by promoting downward salt flux is key to the successful cultivation of moderately salt-tolerant crops in regions underlined by saline groundwater (Rai et al., 2022).
Root zone salinity management primarily focuses on agronomic practices that retard upward flux during the crop season such as mulching, deficit saline water irrigation, and conjunctive use of saline water with good-quality water (Soni et al., 2023). In all these approaches, salts remain in the profile and reappear in the root zone depending upon the evaporative flux. The shallow residue filled cut-drains prepared using a cut-soiler machine were observed to reduce soil salinity and increase cotton and paddy yields after 1 year of operation in salt-affected irrigated fields in Uzbekistan and paddy fields in Japan (Okuda et al., 2018). This residue-filled preferential shallow sub-surface drainage (PSSD) has also shown promise in increasing the yield of the pearl millet and mustard crops in saline soils under irrigation with saline waters in the semi-arid monsoonal climate of India (Neha et al., 2022a, b). These findings suggest that cut-soiler (machine) based residue-filled preferential sub-surface drainage can serve as a remunerative strategy for the sustainable management of saline soil and improve crop production in semi-arid regions where saline groundwater is the only source of irrigation. However, information on the effect of cut-soiler-constructed PSSD on the build-up of salinity in the soil profile and crop growth in a monsoonal climate with different soil and water salinities is scarce. Therefore, we evaluated cut-soiler-constructed PSSD to (i) quantify the effect of cut-soiler PSSD on the changes in soil salinity and moisture content in the soil profile and (ii) assess the effect of change in soil salinity and moisture availability on the physiological processes, growth, and yield of pearl millet-mustard cropping system under saline groundwater irrigation.
The experiment was conducted in semi-controlled lysimeters during 2019 to 2021 at the ICAR- Central Soil Salinity Research Institute, Karnal, Haryana, India (29°42' N latitude and 76°57' E longitude). The site has a semi-arid subtropical monsoonal climate with an average annual rainfall of 757.6 mm, hot summers (May-June), cold winters (December-January), and a distinct rainy (monsoon) season (June-September). The maximum and minimum mean temperature ranges from 32.7°C to 42.8°C in May-June and 3.4°C to 10.8°C in December-January, respectively. The seasonal weather conditions of the two experimental years are provided in Figure 1.
Figure 1. Average weekly weather during 2019, 2020, and 2021 (RF, rainfall; Temp.Max., maximum temperature; Temp.Min., minimum temperature).
The study was conducted in a split-split plot design. The treatments comprised two main plots (cut-soiler PSSD and without cut-soiler PSSD), two sub-plots [saline (sandy loam; ECe 6.0 dS m-1) and non-saline (silty clay loam; ECe 3.02 dS m-1) soils], and three levels of irrigation water salinity (4, 8, and 12 dS m-1) in sub-sub plots. In total, these 12 treatment combinations (2 x 2 x 3) were replicated twice in a total of 24 lysimeters of 2.0 m x 2.0 m x 3.0 m (L x W x H), and the layout is depicted in Figure 2. Each lysimeter was separated by a 10 cm thick reinforced cement and concrete (RCC) wall and a polythene sheet lining the wall and the bottom. Pearl millet [Pennisetum glaucum L. (R. Br)] variety HHB-197 and mustard (Brassica juncea L.) variety CS-58 were grown in the rainy (Kharif) and winter (Rabi) seasons, respectively. The pearl millet was sown on 29 July and 14 July in 2019 and 2020, respectively. A uniform seed rate of 4 kg ha-1 of pearl millet was used with an inter-row spacing of 45 cm. Sowing was done manually using a hand plow. Thinning was done 20 days after sowing (DAS) to maintain a single plant with a plant-to-plant spacing of 15 cm to avoid competition. CS-58 is a high-yielding variety of Indian mustard that is tolerant of salt (Singh et al., 2019). CS-58 is recommended for saline soils with a salinity level (ECe) of up to 11.0 dS m-1 (ICAR-CSSRI::Central Soil Salinity Research Institute). Sowing of mustard was done on 02 November and 23 October in 2019 and 2020, respectively, with a row-to-row distance of 45 cm and a uniform seed rate of 5 kg ha-1. Thinning was done 3-4 weeks after sowing to maintain a plant-to-plant distance of 10-15 cm. One hoeing is done 3-4 weeks after sowing. N: P2O5: K2O at a ratio of 80:40:30 in pearl millet and 80:60:40 kg ha-1 in mustard were applied using urea, diammonium phosphate (DAP), and muriate of potash. A full dose of phosphorus (P) and potassium (K), and half dose of nitrogen were applied as the basal dose at the time of sowing and the remaining nitrogen was applied as top dressing 30 days after sowing (vegetative stage) in both crops. The plots were maintained weed-free (by hand weeding and hoeing as and when required) throughout. For the pearl millet, the fungicide Tilt (Propiconazole 25% EC) and Monocrotophos 36% SL insecticide were applied to control fungal diseases and insect pests. In the mustard crop, the Ridomil Gold (Metalaxyl-M 4% + Mancozeb 64% WP) fungicide and the Rogor (Dimethoate 30% EC) insecticide were applied to control the insect pests. Irrigation was scheduled for the identified critical growth stages for mustard and as per the lifesaving water requirement for pearl millet. Saline water irrigation as per treatments were applied pre-sowing and during the flowering stages of the pearl millet. In the mustard, in addition to pre-sowing irrigation, three irrigations were applied at the vegetative, flowering, and siliqua-formation stages.
Figure 2. Layout of the semi-controlled lysimeter experiment to quantify the desalinization effect of cut-soiler PSSD with a control.
The lysimeters were filled with saline sandy loam and non-saline silty clay loam soil collected from representative sites in Nain, Panipat (29°19′7.09′′ to 29°19′10.0′′N latitude and 76°47′30.0′′to 76°48′0.0′′E) and Sitamai, Karnal (29°68′ N and 76°99′E), respectively. Cut-soiler-based residue-filled V shape preferential drains were constructed manually to simulate cut-soiler PSSD at a depth of 60 cm below the soil surface. The cut-soiler PSSD was filled with 2.4 kg/lysimeter rice residue (equivalent to 6 Mg ha-1). The residue-filled preferential shallow sub-surface drains were connected to the outlet provided in a gallery at the same depth (60 cm) in the lysimeter for this purpose. Nylon and gravel filter material were used at the drain outlet connection point of the pipe to avoid clogging. Another outlet at the bottom of the lysimeter maintained the hydraulic head and the water table at the desired depth. The details of the function of cut-soiler PSSD are provided in Neha et al. (2022a). Soil samples were collected 50 cm from the cut-soiler PSSD line in each lysimeter plot at the beginning of the experiment for the initial soil physico-chemical properties such as ECe, pH, texture, hydraulic conductivity, bulk density, and soil moisture (Table 1). The changes in the salinity of the aqueous soil saturation extract (ECe) under different treatments were measured at the beginning and end of each growing season as per the method described by Richards (1954). The initial soil salinity (ECe) up to 90 cm (0-15, 15-30, 30-60, and 60-90 cm) ranged from 5.05 ± 1.49 to 6.75 ± 1.18 in the saline soil and 2.38 ± 1.84 to 3.63 ± 1.82 dS m-1 in the non-saline silty clay loam soil (Table 1). Water with 4, 8, and 12 dS m-1 salinity levels was prepared by mixing the non-saline tap water (<0.5 dS m-1) with underground water of 4 and 17 dS m-1 collected from Nain (Panipat), India. The EC of the irrigation water was estimated at 25°C using a portable EC Testr11 meter.
The height (cm) of three randomly selected plants was measured at the vegetative, flowering, and harvesting stages for the pearl millet and at 30, 60, 90, and 120 DAS for the mustard. Dry matter accumulation (g m-2) of fresh plant samples was estimated by oven drying at 65 ± 5°C until the attainment of a constant weight. The crop growth rate (CGR) (g m-2 day-1), relative growth rate (RGR) (mg g-1 day-1), and net assimilation rate (NAR) (mg m-2 day-1) were calculated as per the formulae given by Radford (1967). The leaf area (LA, cm2 plant-1) of the detached fully expanded flag leaves was measured using a leaf area meter (CI-203 Wand Leaf Area Meter, WA USA). The leaf area index (LAI) was measured by Sun Scan during mid-day (Tillack et al., 2014) using a CI-203 handheld Laser Leaf Area Meter. Likewise, the average test weight (g) and grain yields (t ha-1) were estimated separately for each treatment. Soil samples were collected at 0-15, 15-30, 30-60, and 60-90 cm depths before sowing and after harvest of each crop and analyzed for ECe and gravimetric moisture content.
The data generated were subjected to the statistical analyses of variance (ANOVA) technique for split-split plot design using SAS 9.2 software (SAS Institute, 2001). The normality and heterogeneity of the variance were tested using Shapiro–Wilk’s and Bartlett’s tests, respectively, before performing the ANOVA. Tukey’s test at p≤ 0.05 was used for pairwise comparisons of different treatments.
The cut-soiler PSSD continuously decreased ECe from the harvest of the first pearl millet crop in October 2019 to the harvest of the second mustard crop in April 2021 in both soil types (Figure 3). Soil salinity declined by 23.3% to 58.5% in both soil types from its initial values (~6.0 dS m-1 in the saline soil and ~3.02 dS m-1 in the normal soil) in different soil layers after the pearl millet harvest in October 2019. The decline in salinity was greater in the surface soil (0-15 cm) and layers adjacent (30-60 and 60-90 cm) to the cut-soiler PSSD depth (Figure 3A). In April 2021, after the harvest of the mustard crop, the decline in soil salinity was even greater, 38.88% to 62.92 % in both the soil types with a maximum decrease at the 60-90 cm depth (just below the cut-soiler PSSD depth) (Figure 3D). The soil salinity gradually decreased with time and it was greater in 2020 compared to 2021 in all the soil layers (Figure 3B).
Figure 3. Change in soil salinity at different soil depths after the harvest of pearl millet under (A) cut-soiler PSSD x soil type, (B) year x soil type, and (C) Cut-soiler PSSD x irrigation water salinity; and after the harvest of mustard under (D) cut-soiler PSSD x soil type, (E) year x soil type, and (F) cut-soiler PSSD x irrigation water salinity. Bars with standard error at each depth are significantly different (P ≤ 0.05); the capped line on each bar is the standard error. CS, cut-soiler PSSD; WCS, control without cut-soiler PSSD; data is the mean of 2 years. The letter (a,b,c...) on the value bar of figure indicate significance of differences based on LSD.
The interaction effect of cut-soiler PSSD and soil type was significant for soil ECe at the 0-15, 15-30, 30-60, and 60-90 cm depths after the harvest of pearl millet (Figure 3). The treatments without cut-soiler PSSD in the saline soil recorded significantly higher ECe (3.77, 4.49, 5.17, and 5.56 dS m-1) at 0-15, 15-30, 30-60, and 60-90 cm soil depths, respectively, in comparison to that of the cut-soiler PSSD treatment observed after harvest of both the crops. Again, the without cut-soiler PSSD and the saline sandy loam soil treatment recorded significantly higher ECe (6.43, 6.06, and 5.53 dS m-1) at the 15-30, 30-60, and 60-90 cm soil depths (Figure 3D).
Cut-soiler PSSD and the salinity of the irrigation water also had a significant interaction effect on the observed soil salinity after both crops were harvested (Figures 3C, F). In general, the soil salinity was lowest with cut-soiler PSSD with the application of 4 dS m-1; ECe increased progressively with an increase in irrigation water salinity at all the soil depths (0-15, 15-30, 30-60, and 60-90 cm) and was the highest in the 12 dS m-1 ECiw without the cut-soiler PSSD treatment. The changes in gravimetric soil moisture content (%) were significantly higher before sowing the pearl millet and mustard crops than at their harvest (Figure 4). Relatively lower soil moisture content was recorded in the cut-soiler PSSD treatments compared to the treatments without cut-soiler PSSD at each sampling i.e., before sowing and after harvest of the pearl millet crop (19.38% and 14.26%) and the mustard crop (18.17% and 12.96%) (Figure 4).
Figure 4. Effect of cut-soiler PSSD on soil moisture content (%) before sowing and after harvesting of pearl millet and mustard. Bars with standard error at each depth are significantly different (P ≤ 0.05); the capped line on each bar is the standard error. CS, cut-soiler PSSD; WCS, control without cut-soiler PSSD; data is the mean of 2 years. The letter (a,b...) on the value bar of figure indicate significance of differences based on LSD.
The soil salinity negatively affected the growth and dry matter accumulation (DMA) of both crops. Likewise, increasing the salinity of the irrigation water also decreased the plant height and DMA of the pearl millet and mustard. However, the cut-soiler PSSD moderated the negative impact of salinity and the plant height of the pearl millet was greater at the vegetative, flowering, and harvesting stages in the cut-soiler PSSD treatment (Table 2). Similarly, the mustard plant height at 30, 60, 90, and 120 DAS, and at harvest was also significantly increased by 7% to 30 % in the cut-soiler PSSD plots compared to those without cut-soiler PSSD. The DMA of the pearl millet also significantly increased by 12.07%, 19.45%, 21.75%, and 23.75% at all four studied stages, respectively, (Table 3) with cut-soiler PSSD compared to without cut-soiler PSSD. Similarly, the mustard crop also had greater a DMA at all the growth stages in the cut-soiler PSSD plots compared to those without cut-soiler PSSD.
Table 2. Effect of cut-soiler PSSD, soil type, and irrigation water salinity on the plant height (cm) of pearl millet and mustard crops at various growth stages.
Table 3. Effect of cut-soiler PSSD, soil type, and irrigation water salinity on the dry matter accumulation (g m-2) of pearl millet and mustard crops at various growth stages.
The NAR of both crops at different growth stages was low in the saline soil and was significantly decreased with an increase in irrigation water salinity (Table 4). The cut-soiler PSSD improved the NAR of both crops and a relatively increased NAR at all growth stages was recorded in the pearl millet with cut-soiler PSSD in comparison to that without cut-soiler PSSD (control). However, the cut-soiler PSSD effect was apparent only at the vegetative to flowering stage of the mustard. Similarly, the effect of soil type on NAR was apparent only at the flowering to reproductive stage with higher values for the non-saline soil. The effect of irrigation water salinity was evident only for 12 dS m-1 at the vegetative to flowering stage (Table 4).
Table 4. Effect of cut-soiler PSSD, soil type, and irrigation water salinity on the net assimilation rate (g m-2 d-1) of pearl millet and mustard crops at various growth stages.
The cut-soiler PSSD plots recorded higher CGR for the pearl millet at all three growth stages (Figure 5A). A higher CGR of the crop at all three successive growth stages was observed in the non-saline soil compared to the saline soil (Figure 5B). Among the different irrigation water salinity treatments, the maximum CGR was found in the 4 dS m-1 treatment, followed by 8 dS m-1 and 12 dS m-1 (Figure 5C). Similarly, the cut-soiler PSSD plots had a significantly higher CGR for the mustard crop compared to those without cut-soiler PSSD (Figure 5A). The non-saline soil had a higher CGR compared to the saline soil (Figure 5B). The CGR was significantly decreased with an increase in irrigation water salinity (Figure 5C). Similar to CGR, the RGR at different growth stages of pearl millet had higher values in the cut-soiler PSSD treatments compared to those without cut-soiler PSSD. The non-saline soil recorded a higher RGR at all three growth stages compared to the saline soil (Figure 5E). Among the different irrigation water salinity treatments, increasing the water salinity significantly reduced the RGR by 3.87%, 7.43%, and 1.03% under 8 dS m-1 ECiw and by 7.93%, 10.03%, and 1.45 % under 12 dS m-1 ECiw in the respective growth stages (Figure 5F). The mustard crop also recorded similar results for cut-soiler PSSD, non-saline soil, and irrigation water salinity (Figures 5D–F).
Figure 5. Effect of cut-soiler PSSD, soil type, and irrigation water salinity on crop growth rate (CGR) (A–C) and relative growth rate (RGR) (D–F) of pearl millet and mustard crops at various growth stages. Bars with standard error at each depth are significantly different (P ≤ 0.05); the capped line on each bar is the standard error. CS, cut-soiler PSSD; WCS, control without cut-soiler PSSD; data is the mean of 2 years. The letter (a,b...) on the value bar of figure indicate significance of differences based on LSD.
The cut-soiler PSSD significantly increased pearl millet LA by 27.15% and 23.55 % in the vegetative and reproductive stages, respectively. Of the two soil types, the non-saline soil had significantly higher LA compared to the saline soil at both the studied stages (Table 5). A progressive increase in irrigation water salinity, from 4 dS m-1 to 12 dS m-1, decreased LA significantly. In the mustard crop, cut-soiler PSSD resulted in a significant increase of 10.72% and 13.65% in LA in both the studied stages respectively compared to the treatment without cut-soiler PSSD (Table 5). Similarly, of the two soil types, the non-saline soil had significantly higher LA compared to the saline soil in the vegetative (100.30 and 88.97 cm-2) and reproductive (140.99 and 137.79 cm-2) stages. The LA decreased significantly with increasing salinity of irrigation water. The cut-soiler PSSD and non-saline soil plots recorded a higher LAI than those without cut-soiler PSSD and saline soil (Table 5). However, increasing the salinity of irrigation water reduced the LAI of both crops.
Table 5. The effect on leaf area and leaf area index of pearl millet and mustard crops of cut-soiler PSSD, soil type, and irrigation water salinity at various growth stages.
The cut-soiler PSSD treatment caused a significant decrease in the Na:K ratio in pearl millet grain and stover compared to the treatment without cut-soiler PSSD (Figure 6). An increase in irrigation water salinity significantly increased the Na:K ratio in the grain and stover of the pearl millet (Figure 6). Similarly, in the mustard, the Na:K ratio in the seeds was lower in the cut-soiler PSSD treatment compared to the treatment without cut-soiler PSSD. The non-saline soil had a lower Na:K ratio in seeds compared to the saline soil. However, increasing the salinity level of the irrigation water significantly increased the Na:K ratio in seeds. A similar Na:K ratio trend was observed in mustard straw.
Figure 6. Effect of cut-soiler PSSD, soil type, and irrigation water salinity on Na:K ratio of pearl millet and mustard crops. Bars with standard error at each depth are significantly different (P ≤ 0.05); the capped line on each bar is the standard error. CS, cut-soiler PSSD; WCS, control without cut-soiler PSSD; data is the mean of 2 years.
The grain/seed yield of both crops significantly increased in the treatment with cut-soiler PSSD and non-saline soil but decreased with an increase in the salinity of the irrigation water. The combined interactive effect of all three factors was also evident during the two study years. Cut-soiler PSSD, in both the saline and non-saline soils, resulted in an increased yield of pearl millet by 26.08% and 20.27% (2019) and 43.26% and 11.62% (2020), and of mustard by 36% and 26% (both years), respectively. The highest pearl millet grain yield (4.61 t ha-1) was recorded in a cut-soiler PSSD treatment in non-saline soil in 2020 and the lowest (2.53 t ha-1) was in a treatment without cut-soiler PSSD in saline soil in 2019 (Figure 7A). The interaction effect of cut-soiler PSSD, soil type, and irrigation water salinity was also found positive (Figure 7B). The highest pearl millet grain yield (4.72 t ha-1) was obtained in a cut-soiler PSSD plot with non-saline soil and irrigation with 4 dS m-1 salinity water and declined with increment in soil and irrigation water salinity. The lowest yield (2.24 t ha-1) was recorded in treatment without cut-soiler PSSD with saline soil and the application of 12 dS m-1 salinity irrigation water. The effect of saline water irrigation treatments was also found significant (P=<0.0001), as the pearl millet yield decreased with increased salinity level of the applied irrigation water. In the case of the mustard crop, the interaction effect of cut-soiler PSSD and irrigation water salinity was significant for seed yield (Figure 7D). Seed yield was the highest (3.01 t ha-1) in a cut-soiler PSSD plot with an irrigation water salinity level of 4 dS m-1 while it was the lowest (2.0 t ha-1) in a treatment without cut-soiler PSSD and a 12 dS m-1 salinity level for the irrigation water (Figure 7D). Similarly, cut-soiler PSSD and soil type also had a positive interaction effect on the seed yield of mustard (Figure 7E). The treatment with cut-soiler PSSD in non-saline soil produced the highest seed yield (2.89 t ha-1) and the treatment without cut-soiler PSSD in saline soil resulted in the lowest (1.98 t ha-1) seed yield.
Figure 7. Interaction effect of cut-soiler PSSD x soil type x irrigation water salinity on the grain/seed yield of pearl millet and mustard crops at various growth stages. Bars with standard error at each depth are significantly different (P ≤ 0.05); the capped line on each bar is the standard error. CS, cut-soiler PSSD; WCS, control without cut-soiler PSSD; data is the mean of 2 years. The letter (a,b...) on the value bar of figure indicate significance of differences based on LSD.
A significantly higher test weight for pearl millet was recorded in the cut-soiler PSSD plots (9.98 g) than in the control (9.33 g) and in non-saline soil (9.80 g) compared to the saline soil (9.51 g) (Figure 8). Heavier grains were produced under an ECiw of 4 dS m-1 (9.82 g) followed by an ECiw of 8 dS m-1 (9.65 g) and an ECiw of 12 dS m-1 (9.49 g). For the mustard crop, a significantly higher test weight was recorded in the cut-soiler PSSD plots (5.20 g) compared to those without cut-soiler PSSD (4.86 g), and in non-saline soil (5.15 g) compared to saline soil (4.90 g). Among the different irrigation water salinity levels, an ECiw of 4 dS m-1 produced heavier grains (5.11 g) followed by an ECiw of 8 dS m-1 (5.03 g), and an ECiw of 12 dS m-1 (4.95 g).
Figure 8. Effect of cut-soiler PSSD, soil type, and irrigation water salinity on test weight (g) of pearl millet and mustard crops. Bars with standard error at each depth are significantly different (P ≤ 0.05); the capped line on each bar is the standard error. CS, cut-soiler PSSD; WCS, control without cut-soiler PSSD; data is the mean of 2 years. The letter (a,b...) on the value bar of figure indicate significance of differences based on LSD.
The growth traits were associated either positively or negatively among themselves and with yield traits and ECe (Figure 9). In the pearl millet, grain yield had a strong positive correlation with plant growth traits such as plant height, dry matter accumulation, crop growth rate, relative growth rate, net assimilation rate, leaf area index, leaf area, and thousand-seed weight (r = 0.63-0.85), while it was negatively correlated with the Na:K ratio (r = -0.63). Similarly, thousand-seed weight was also positively correlated with plant height, dry matter accumulation, crop growth rate, relative growth rate, net assimilation rate, leaf area index, and leaf area (r = 0.61-0.97), while it was negatively correlated with the Na:K ratio (r = -0.57) The soil salinity was negatively correlated with pearl millet grain yield (r = -0.64) and all other growth parameters and positively associated with the Na:K ratio (r = 0.71).
Figure 9. Pearson’s correlation coefficients among the growth and yield traits of pearl millet and mustard. The color of the boxes reflects the strength of the correlation. The correlation coefficients represent the mean value of pooled measurements from two growing seasons; PH, plant height; DM, dry matter accumulation; CGR, crop growth rate; RGR, relative growth rate; NAR, net assimilation rate; LAI, leaf area index; LA, leaf area; 1000 wt, 1000-grain weight; Na/K, Na:K ratio; GY, Grain Yield; ECe, soil salinity.
Also, there was a strong correlation between growth and yield traits in the mustard crop. The grain yield was positively correlated with plant height, dry matter accumulation, crop growth rate, relative growth rate, net assimilation rate, leaf area index, leaf area, and thousand-seed weight (r = 0.67-0.95), but was negatively correlated with the Na:K ratio (r = -0.77). Likewise, thousand-seed weight also showed a positive correlation with plant height, dry matter accumulation, crop growth rate, relative growth rate, net assimilation rate, leaf area index, and leaf area (r = 0.64-0.93), while it was negatively correlated with the Na:K ratio (r = -0.86). The soil salinity was negatively correlated with mustard grain yield (r = -0.81) and all other growth parameters and positively associated with the Na:K ratio (r = 0.92) (Figure 9).
Salinity stress largely increases through the interplay of inherent soil salinity, drainage congestion, salinity of irrigation water, and the sensitivity of any crop and its growth stages (Minhas, 1996; Okuda et al., 2018; Yadav et al., 2020; Chakraborty et al., 2016). Hydraulic conductivity, the range of moisture retention, and the moisture release characteristics of any textural class of soil govern its drainage function and leaching fraction (Feng et al., 2019). Whereas the total salt accumulation in the soil profile is a consequence of its drainage function and leaching fraction, and the quality of irrigation water or rainfall available for crop production (Sharma and Tyagi, 2004). The balance of the upward and downward salt flux is the result of salt accumulation in the soil solution and the associated proportionate osmotic stress (Lu et al., 2018). The osmotic stress affects the water and nutrient uptake (Yadav et al., 2007; Sarker and Oba, 2020), plant cellular constituents (Silva et al., 2015), physiological processes (Manjili et al., 2012), and growth and production of crops (Alzahrani et al., 2019). The salt movement equilibria in any agroclimatic conditions are modified by the introduction of management practices to tilt the equilibria towards low osmotic stress and better plant-growing conditions. The installation of properly designed subsurface drainage systems can effectively manage the adverse effects of salinity stress (Bundela et al., 2016; Islam et al., 2022). However, such approaches involve a huge cost and require a community approach for large-scale installation. Residue-filled cut-soiler-constructed PSSD has been found to be effective in removing salt from the surface soil (Neha et al., 2022a, b; Okuda et al., 2018). Our results from a present study also showed an appreciable decrease in surface salinity through the lateral flow of salt from the drainage line. In the semi-arid monsoonal climate of the western IGP, salt moves upward and downward depending on the rainfall and evaporative flux (Soni et al., 2021). The use of PSSD in these soils provides an outlet for the net removal of salt through drainage effluents. The repeated cycle of salt movement in the surface soil followed by leaching with rain or irrigation water leads to a decrease in the salinity of the soil profile. This was evident from the decrease in salinity in the soil up to 90 cm in depth and the associated effects on the growth and biomass production of both crops in a pearl millet-mustard cropping system. The salinity of the soil profile is the outcome of salt addition and loss from the system. This was the reason for slightly greater salinity in the soil profile of the PSSD plots irrigated with water with higher salinity. The associated improvement in the soil solution composition, nutrient availability, and plant rhizosphere conditions also resulted in higher growth and production for both crops. This magnitude of reduction in the soil salinity of the root zone was equivalent to a 44%-49% reduction observed in the third year after the installation of pipe SSD (Bundela et al., 2016; Islam et al., 2022; Raju et al., 2016). Similarly, Lu et al. (2018) also reported that maize stalk-filled SSD was quite efficient in removing salt and water from the crop’s root zone.
Relatively less soil moisture was observed in the cut-soiler PSSD plots in both seasons. Drainage water outflow carrying soluble salts that lower soil salinity in both types of soil under different levels of irrigation water salinity leads to the lowering of the water potential because of its low osmotic potential (Yadav et al., 2007). Therefore, the plant-utilizable water content that was in the field capacity range and permanent wilting point was relatively greater in the PSSD plots compared to the non-PSSD plots under both soil conditions due to the removal of extra water by PSSD through lateral drainage (Singh and Sharma, 2016). Rice residue-filled cut-soiler PSSD caused higher conducive soil moisture with lower salinity stress in both textural class soils and at different salinity levels of irrigation water. Such an improvement in the aeration and maintenance of optimum moisture during germination and the growth cycle of both crops increased crop germination, root growth, metabolic activities, growth, and overall performance (Neha et al., 2022a). The soil effect on the plant performance was mainly associated with higher hydraulic conductivity and a narrower range of moisture retention/release constants. The moisture constant at the field capacity and permanent wilting point of sandy loam saline soil and silty clay loam soil were 22.1 ± 3.2 and 8.3 ± 0.6%, and 26.2 ± 2.8 and 15.6 ± 1.4%, respectively. This led to a relatively higher reduction in soil salinity (up to 43.42%) but a lower soil moisture content in the sandy loam compared to the silty clay loam soil. Kahlown et al. (2009) found that soil EC and ESP increased by 2.2 dS m-1 and 14.8% in coarse, and 9.0 dS m-1 and 26.3% in fine-textured soil when high SAR (16) saline water (6.2 dS m-1) was used for irrigation in a mustard crop. Similarly, Ragab et al. (2008) and Chaudhry et al. (2001) recorded a progressive increase in soil EC with increasing levels of irrigation water salinity. Such favorable conditions under PSSD increased crop germination, root growth, metabolic activities, growth, and overall performance (Neha et al., 2022a, b). A progressive increase in the leaching of salts under cut-soiler PSSD during consecutive rainy seasons resulted in a relative decrease in salinity with every season, leading to an increased yield of both crops in the second year (Okuda et al., 2018; Chiba et al., 2012; Kaneko et al., 2002). The use of cut-soiler PSSD in saline sandy loam soils in India and shallow-cut drains in salt-affected fields in Uzbekistan have been reported to result in consistent salt removal and consequently better growth and yield of pearl millet, mustard, and cotton (Neha et al., 2022c, d; Okuda et al., 2018).
Excess soluble salts in the soil profile induce an adverse osmotic effect that reduces the uptake of water (Yadav et al., 2007) with a lower but selective accumulation of ions and nutrients (Sarker and Oba, 2020). Salinity stress also leads to a progressive increase in excess accumulation of Na+ in the cytosol (Sarker and Oba, 2020) and a reduction in the biosynthesis of the chlorophyll protein-lipid complex and photosynthesis efficiency (Manjili et al., 2012). The application of higher salinity irrigation water also causes more negative osmotic/water potential in the soil profile, which in turn decreases the uptake of both water and nutrients, thereby causing an ionic imbalance and toxicity in plants. All these factors cause impairment of different physiological and biochemical processes, which ultimately cause reduced plant growth and production (Yadav et al., 2020; Soni et al., 2021). Increasing the salinity of irrigation water increased the Na:K ratio and decreased the growth, yield, and their attributes in both crops as was also reported previously in Brassica juncea (Kumar et al., 2005) and pearl millet (Mostafa et al., 2012). The residue-filled cut-soiler PSSD in this study reduced salinity stress and lowered the Na:K ratio in plants of both crops. The inhibitory effect of higher salinity levels of irrigation water on yield was caused mainly by osmotic shock (Nadaf et al., 2010), ion toxicity (Makarana et al., 2017), and nutritional imbalance (Yadav et al., 2020) which reduced photosynthetic activity and other physiological processes (Kumar et al., 2017; Ali and Awan, 2004). The poor seed yield in the saline environment could be also due to salt-induced shrinkage and even complete damage of chloroplasts (Manjili et al., 2012), a decrease in photosynthates in the phloem (Flowers et al., 1991), and water deficiency in the pearl millet-mustard growing regions (Singh and Panda, 2011). However, rice residue-filled cut-soiler PSSD improved the drainage of excess water and salts from the root zone, thus increasing the grain/seed test weight and finally the yield of both crops (Figure 7). Therefore, this study illustrated the efficiency of rice residue-filled cut-soiler PSSD in mitigating the negative effect of increased irrigation water salinity on soil salinization and the consequent adverse impact on various growth and yield parameters in pearl millet and mustard.
The rice residue-filled cut-soiler-constructed preferential shallow sub-surface drainage system removed excess salts from the root zone with percolating water, especially during the rainy season. Continued leaching of the salt from the layer above the cut-soiler line maintained a lower salinity in the soil profile with improved soil moisture conditions for plant growth even under saline water irrigation. Furthermore, it increased dry matter accumulation, net assimilation rate, CGR, RGR, leaf area, and LAI, as well as the production of both pearl millet and mustard crops in saline semi-arid conditions. This study demonstrated the effectiveness of rice residue-filled cut-soiler PSSD in reducing the salinity of the soil profile and preventing salt accumulation under saline water irrigation. This study also showed its agronomic potential to mitigate root zone salinity to improve the productivity of pearl millet and mustard crops in saline groundwater or saline irrigation agroecologies.
The raw data supporting the conclusions of this article will be made available by the authors, without undue reservation.
N: Data curation, Formal analysis, Methodology, Validation, Writing – original draft. GY: Data curation, Formal analysis, Methodology, Validation, Writing – original draft, Conceptualization, Funding acquisition, Project administration, Supervision, Visualization, Writing – review & editing. RY: Conceptualization, Funding acquisition, Methodology, Project administration, Resources, Supervision, Validation, Visualization, Writing – review & editing. AK: Investigation, Methodology, Writing – review & editing. AR: Investigation, Methodology, Writing – review & editing. GP: Methodology, Writing – review & editing. SK: Investigation, Writing – review & editing. BN: Investigation, Methodology, Writing – review & editing. JO: Conceptualization, Investigation, Methodology, Writing – review & editing. KO: Conceptualization, Funding acquisition, Writing – review & editing. SC: Conceptualization, Supervision, Writing – review & editing.
The author(s) declare financial support was received for the research, authorship, and/or publication of this article. This research was funded by the Indian Council of Agricultural Research (ICAR)-Japan International Research Center for Agricultural Sciences (JIRCAS) collaborative research project.
We thank the director of ICAR-CSSRI for providing the necessary logistics/facilities, CCS HAU for PhD studentship under ICAR-CSSRI-CSS HAU MoU, and JIRCAS for funding support.
The authors declare that the research was conducted in the absence of any commercial or financial relationships that could be construed as a potential conflict of interest.
All claims expressed in this article are solely those of the authors and do not necessarily represent those of their affiliated organizations, or those of the publisher, the editors and the reviewers. Any product that may be evaluated in this article, or claim that may be made by its manufacturer, is not guaranteed or endorsed by the publisher.
Ali Y., Awan A. R. (2004). Influence of salinity at seedling stage and on yield and yield components of different rice lines. Int. J. Biol. Biotechnol. 1, 175–179.
Alzahrani Y., Kuşvuran Ş., Kuşvuran A. (2019). Exogenously applied proline alleviates salt-induced oxidative stress in strawberry plants by enhancing antioxidant enzyme activity and maintaining chlorophyll content. Hortic. Sci. 46, 142–150.
Bundela D. S., Kaledhonkar M. J., Gupta S. K., Lal M., Kamra S. K., Sharma D. K., et al. (2016). Cost estimation of sub surface drainage systems for reclamation of waterlogged saline lands. J. Soil Salinity Water Quality. 8, 131–143.
Chakraborty K., Sairam R. K., Bhaduri D. (2016). Effects of different levels of soil salinity on yield attributes, accumulation of nitrogen, and micronutrients in Brassica spp. J. Plant Nutr. 39, 1026–1037. doi: 10.1080/01904167.2015.1109105
Chaudhry M. R., Iqbal M., Subhani K. M. (2001). Management of brackish water: Impact on soil and crops. Pakistan J. Soil Sci. (Pakistan). 20, 33–38. Available online at: http://localhost:80/xmlui/handle/123456789/18749.
Chiba K., Kato T., Togashi C., Kanmuri H. (2012). Effectiveness of desalinization by infiltration of water and desalinization of tsunami-hit farmlands in Miyagi Prefecture. Water Land Environ. Eng. 80, 3–6.
Choudhary M., Rana K. S., Bana R. S., Ghasal P. C., Choudhary G. L., Jakhar P., et al. (2017). Energy budgeting and carbon footprint of pearl millet–mustard cropping system under conventional and conservation agriculture in rainfed semi-arid agro-ecosystem. Energy 141, 1052–1058. doi: 10.1016/j.energy.2017.09.136
Choudhary O. P., Kharche V. K. (2018). Soil salinity and sodicity. Soil science: an intro. 12, 353–384.
Cooper N. (2023). Available online at: https://www.weforum.org/agenda/2023/03/the-ipcc-just-published-its-summary-of-5-years-of-reports-here-s-what-you-need-to-know/ (accessed March 2023).
Diaz C. G., Secanellas S. A., Caro G. M., Velayos J. J., Carrascosa H., Hernández M. G., et al. (2021). Thermal Conductivity measurements of macroscopic frozen Salt Ice analogs of Jovian Icy moons in support of the planned JUICE mission. Month. Notices R. Astronom. Society. 510, 4166–4179. doi: 10.48550/arXiv.2112.05697
Dong H., Li W., Tang W., Zhong D. (2008). Furrow seeding with plastic mulching increases stand establishment and lint yield of cotton in saline field. Agron. J. 100, 1640–1646. doi: 10.2134/agronj2008.0074
Feng G., Zhang Z., Zhang Z. (2019). Evaluating the sustainable use of saline water irrigation on soil water-salt content and grain yield under subsurface drainage condition. Sustainability 11, 6431. doi: 10.3390/su11226431
Flowers T. J., Hajibagherp M. A., Yeo A. R. (1991). Ion accumulation in the cell walls of rice plants growing under saline conditions: evidence for the Oertli hypothesis. Plant Cell Environ. 14, 319–325. doi: 10.1111/j.1365-3040.1991.tb01507.x
Food and Agriculture Organization of the United Nations (FAO) (2017). Expert meeting on climate change, land use and food security (Rome, Italy: Intergovernmental Panel on Climate Change (IPCC)). Available at: http://www.ipcc.ch/about/about.htm.
Fu Z., Wang P., Sun J., Lu Z., Yang H., Liu J., et al. (2020). Composition, seasonal variation, and salinization characteristics of soil salinity in the Chenier Island of the Yellow River Delta. Global Ecol. Conserv. 24, e01318. doi: 10.1016/j.gecco.2020.e01318
Heidari M., Jamshidi P. (2011). Effects of salinity and potassium application on antioxidant enzyme activities and physiological parameters in pearl millet. Agric. Sci. China. 10, 228–237. doi: 10.1016/S1671-2927(09)60309-6
ICAR-CSSRI::Central Soil Salinity Research Institute. Technology Published. Available online at: https://cssri.res.in/technology/.
Islam M. N., Bell R. W., Barrett-Lennard E. G., Maniruzzaman M. (2022). Shallow surface and subsurface drains alleviate waterlogging and salinity in a clay-textured soil and improve the yield of sunflower in the Ganges Delta. Agron. Sustain. Dev. 42, 1–17. doi: 10.1007/s13593-021-00746-4
Kahlown M. A., Akram M., Soomro Z. A., Kemper W. D. (2009). Prospectus of growing barley and mustard with saline groundwater irrigation in fine and coarse-textured soils of Cholistan desert. Irrigation and Drainage. J. Int. Commission Irrig. Drain. 58, 469–481. doi: 10.1002/ird.v58:4
Kaneko T., Murakawa M., Kozai N., Mitsugi K. (2002). Desalting technique for illuviated salts soil at paddy field by using underdrainage. J. Japan. Soc. Irrig. Drain. Reclam. Eng. (Japan). 70, (7).
Krishnamurthy L., Serraj R., Rai K. N., Hash C. T., Dakheel A. J. (2007). Identification of pearl millet [Pennisetum glaucum (L.) R. Br.] lines tolerant to soil salinity. Euphytica 158, 179–188. doi: 10.1007/s10681-007-9441-3
Kumar R., Goyal V., Kuhad M. S. (2005). Influence of fertility salinity interactions on growth, water status and yield of Indian mustard (Brassica juncea). Indian Plant Physiol. 10, 139–144.
Kumar A., Lata C., Krishnamurthy S. L., Kumar A., Prasad K. R. K., Kulshreshtha N. (2017). Physiological and biochemical characterization of rice varieties under salt and drought stresses. J. Soil Salinity Water Quality. 9, 167–177.
Lakhdar A., Rabhi M., Ghnaya T., Montemurro F., Jedidi N., Abdelly C. (2009). Effectiveness of compost use in salt-affected soil. J. Hazard Mater. 171, 29–37. doi: 10.1016/j.jhazmat.2009.05.132
Lu P., Zhang Z., Feng G., Huang M., Shi X. (2018). Experimental study on the potential use of bundled crop straws as subsurface drainage material in the newly reclaimed coastal land in Eastern China. Water 10, 31. doi: 10.3390/w10010031
Makarana G., Yadav R. K., Kumar R., Soni P. G., Yadav T., Yadav M. R., et al. (2017). Fodder yield and quality of pearl millet (Pennisetum glaucum L.) Genotypes as influenced by salinity of irrigation water in north western India. Indian J. Anim. Nutr. 34, 56–63. doi: 10.5958/2231-6744.2017.00009.3
Manjili F. A., Sedghi M., Pessarakli M. (2012). Effects of phytohormones on proline content and antioxidant enzymes of various wheat cultivars under salinity stress. J. Plant Nutr. 35, 1098–1111. doi: 10.1080/01904167.2012.671411
Minhas P. S. (1996). Saline water management for irrigation in India. Agric. Water manage. 30, 1–24. doi: 10.1016/0378-3774(95)01211-7
Mostafa A. Z., Amato M., Galal Y. G. M., Hamdi A., Lotfy S. M. (2012). Effects of Irrigation with saline water and soil type on germination and seedling growth of sweet maize (Zea Mays L.). Arab J. Nucl. Sci. Applic. 45, 537–547.
Nadaf S. K., Al-Hinai S. A., Al-Farsi S. M., Al-Lawati A. H., Al-Bakri A. N., Ahmed M., et al. (2010). “Differential response of salt tolerant pearl millet genotypes to irrigation water salinity,” in Management of Salt-Affected Soils and Water for Sustainable Agriculture. Eds. Mushtaque A., Al-Rawahi S. A., Hussain N. (Sultan Qaboos University, Oman), 47–60.
Neha, Yadav G., Yadav R. K., Kumar A., Rai A. K., Onishi J. (2022d). Effect of Cut-soiler PSSD on growth, fodder yield and quality of pearl millet crop using saline irrigation water. Forage Res. 47, 441–447.
Neha, Yadav G., Yadav R. K., Kumar A., Rai A. K., Onishi J., et al. (2022b). Salt Removal through Residue Filled Cut-Soiler Simulated Preferential Shallow Subsurface Drainage Improves Yield, Quality and Plant Water Relations of Mustard (Brassica juncea L.). Sustainability 14, 4146. doi: 10.3390/su14074146
Neha, Yadav G., Yadav R. K., Kumar A., Rai A. K., Onishi J., et al. (2022c). “Response of saline water irrigated mustard crop growth and yield under Cut-soiler PSSD salinity management,” in AMA, Agricultural Mechanization in Asia, Africa and Latin America. Farm Machinery Industrial Research Crop, vol. 53, 6025–6037.
Neha, Yadav G., Yadav R. K., Kumar A., Rai A. K., Sehwag M., et al. (2022a). Desalinization effect of simulated Cut-soiler based residue filled preferential shallow sub surface drainage improves physiology and yield of rainfed pearl millet in saline semiarid regions. Land Degrad. Dev. 33, 1758–1770. doi: 10.1002/ldr.4237
Okuda Y., Onishi J., Shirokova Y. I., Kitagawa I., Kitamura Y., Fujimaki H. (2018). Salt removal technology by shallow subsurface drainage in combination with a cut-drain. J. Arid Land Stud. 28, 127–130. doi: 10.14976/jals.28.S_127
Pessarakli M., Szabolcs I. (2019). Soil salinity and sodicity as particular plant/crop stress factors. In Handbook of Plant and Crop Stress, Fourth Edition. CRC press. pp. 3–21.
Prakash R., Singh V., Diwedi A., Popat R. C., Kumari S., Kumar N., et al. (2021). Sewage sludge impacts on yields, nutrients and heavy metals contents in pearl millet–wheat system grown under saline environment. Int. J. Plant Product. 15, 93–105. doi: 10.1007/s42106-020-00122-4
Qadir M., Noble A. D., Qureshi A. S., Gupta R. K., Yuldashev T., Karimov A. (2009). Salt-induced land and water degradation in the Aral Sea basin: A challenge to sustainable agriculture in Central Asia Vol. 33 (Natural Resources Forum Oxford, UK: Blackwell Publishing Ltd), 134–149.
Qadir M., Quillerou E., Nangia V., Murtaza G., Singh M., Thomas R. J., et al. (2014). Economics of salt-induced land degradation and restoration. Nat. Resour. Forum. 38, 282–295. doi: 10.1111/1477-8947.12054
Radford P. J. (1967). Growth analysis formulae-their use and abuse. Crop Sci. 7, 171–175. doi: 10.2135/cropsci1967.0011183X000700030001x
Ragab A. A. M. M., Hellal F. A., Abd El-Hady M. (2008). “Irrigation water salinity effects on some soil water constants and plant,” in Twelfth International Water Technology Conference Proceedings, Vol. 12. 1–14 (Alexandria, Egypt: IWTC).
Rai A. K., Basak N., Soni P. G., Kumar S., Sundha P., Narjary B., et al. (2022). Bioenergy sorghum as balancing feedback loop for intensification of cropping system in salt-affected soils of the semi–arid region: Energetics, biomass quality and soil properties. Eur. J. Agron. 134, 126452. doi: 10.1016/j.eja.2021.126452
Raju R., Singh K., Kumar P., Kumar S., Tripathi R. S. (2016). Reclamation of saline soils through subsurface drainage technology in Haryana–an economic impact analysis. J. Soil Salinity Water Quality. 8, 194–201.
Richards L. A. (1954). “Diagnosis and improvement of saline and alkali soils,” in USDA Agriculture Handbook, vol. 60. (Washington, D.C: United States Department of Agriculture), 160.
Sarker U., Oba S. (2020). The response of salinity stress-induced A. tricolor to growth, anatomy, physiology, non-enzymatic and enzymatic antioxidants. Front. Plant Sci. 11, 559876. doi: 10.3389/fpls.2020.559876
Sharma D. K., Thimmppa K., Chinchmalatpure A. R., Mandal A. K., Yadav R. K., Choudhari S. K., et al. (2015). Assessment of Production and Monetary Losses from Salt-affected Soils in India (Karnal: ICAR-Central Soil Salinity Research Institute). Technical Bulletin: ICAR-CSSRI/ Karnal/Tech.Bulletin/2015/.
Sharma D. P., Tyagi N. K. (2004). On-farm management of saline drainage water in arid and semi-arid regions. Irrig. drainage. 53, 87–103. doi: 10.1002/ird.v53:1
Shrivastava P., Kumar R. (2015). Soil salinity: A serious environmental issue and plant growth promoting bacteria as one of the tools for its alleviation. Saudi J. Biol. Sci. 22, 123–131. doi: 10.1016/j.sjbs.2014.12.001
Silva E. N., Ribeiro R. V., Ferreira-Silva S. L., Viégas R. A., Silveira J. A. G. (2015). Salt stress-induced damages on the photosynthesis of Physalis angulata associated with the induction of oxidative stress. Plant Physiol. Biochem. 86, 126–137. doi: 10.1590/S0103-90162011000100010
Singh A., Panda A. S. (2011). Effect of saline irrigation water on mustard (Brassica juncea) crop yield and soil salinity in a semi-arid area of North India. Exp. Agricult. 48, 99–110. doi: 10.1017/S0014479711000780
Singh J., Sharma P. C. (2016). Comparative effects of soil and water salinity on oil quality parameters of Brassica juncea. J. Oilseed Brassica. 7, 29–37.
Singh J., Sharma P. C., Singh V., Sharma D. K., Sharma S. K., Singh Y. P., et al. (2019). CS58: new high yielding, salt and alkaline tolerant cultivar of Indian mustard. Crop Breed. Appl. Biotechnol. 19, 451–455. doi: 10.1590/1984-70332019v19n4c63
Soni P. G., Basak N., Rai A. K., Sundha P., Chandra P., Yadav R. K. (2023). “Occurrence of salinity and drought stresses: status, impact, and management,” in Salinity and Drought Tolerance in Plants: Physiological Perspectives (Springer Nature Singapore, Singapore), 1–28.
Soni P. G., Basak N., Rai A. K., Sundha P., Narjary B., Kumar P., et al. (2021). Deficit saline water irrigation under reduced tillage and residue mulch improves soil health in sorghum-wheat cropping system in semi-arid region. Sci. Rep. 11, 1–3. doi: 10.1038/s41598-020-80364-4
Statistical Analysis System. (2001). User’s Guide: Statistics, Version 8.2 (SAS Institute, NC, USA).
Tillack A., Clasen A., Kleinschmit B., Förster M. (2014). Estimation of the seasonal leaf area index in an alluvial forest using high-resolution satellite-based vegetation indices. Remote Sens. Environ. 141, 52–63. doi: 10.1016/j.rse.2013.10.018
Tripathi M. K., Chaturvedi S., Shukla D. K., Mahapatra B. S. (2010). Yield performance and quality in Indian mustard (Brassica juncea) as affected by integrated nutrient management. Indian J. Agron. 55, 138–142. doi: 10.59797/ija.v55i2.4731
Wahid A., Rasul E., Rao R., Iqbal R. (2005). Photosynthesis in leaf, stem, flower and fruit. Handb. photosynth. 2, 479–497.
Wang F. (2021). Characterize soil salinity at multiple depth using electromagnetic induction and remote sensing data with random forests: A case study in Tarim River Basin of southern Xinjiang, China. Sci. Total Environ. 754, 142030. doi: 10.1016/j.scitotenv.2020.142030
Wei Y., Ding J., Yang S., Wang F., Wang C. (2021). Soil salinity prediction based on scale-dependent relationships with environmental variables by discrete wavelet transform in the Tarim Basin. Catena 196, 104939. doi: 10.1016/j.catena.2020.104939
Yadav T., Kumar A., Yadav R. K., Yadav G., Kumar R., Kushwaha M. (2020). Salicylic acid and thiourea mitigate the salinity and drought stress on physiological traits governing yield in pearl millet–wheat. Saudi J. Biol. Sci. 27, 2010–2017. doi: 10.1016/j.sjbs.2020.06.030
Keywords: cut-soiler PSSD, salinity, drainage, pearl millet [Pennisetum glaucum (L.)], mustard [Brassica juncea (L)]
Citation: Neha, Yadav G, Yadav RK, Kumar A, Rai AK, Prasad G, Kumar S, Narjary B, Onishi J, Omori K and Chaudhari SK (2024) Cut-soiler-constructed residue-filled preferential shallow sub-surface drainage improves the performance of mustard-pearl millet cropping system in saline soils of semi-arid regions. Front. Agron. 6:1492505. doi: 10.3389/fagro.2024.1492505
Received: 07 September 2024; Accepted: 08 November 2024;
Published: 10 December 2024.
Edited by:
Subhash Babu, ICAR-Indian Agricultural Research Institute, IndiaReviewed by:
Amit Anil Shahane, Central Agricultural University, IndiaCopyright © 2024 Neha, Yadav, Yadav, Kumar, Rai, Prasad, Kumar, Narjary, Onishi, Omori and Chaudhari. This is an open-access article distributed under the terms of the Creative Commons Attribution License (CC BY). The use, distribution or reproduction in other forums is permitted, provided the original author(s) and the copyright owner(s) are credited and that the original publication in this journal is cited, in accordance with accepted academic practice. No use, distribution or reproduction is permitted which does not comply with these terms.
*Correspondence: Gajender Yadav, Z2FqZW5kZXIuaWNhckBnbWFpbC5jb20=; Rajender Kumar Yadav, cmsueWFkYXZAaWNhci5nb3YuaW4=
†These authors have contributed equally to this work and share first authorship
‡ORCID: Neha, orcid.org/0009-0000-7473-0434
Disclaimer: All claims expressed in this article are solely those of the authors and do not necessarily represent those of their affiliated organizations, or those of the publisher, the editors and the reviewers. Any product that may be evaluated in this article or claim that may be made by its manufacturer is not guaranteed or endorsed by the publisher.
Research integrity at Frontiers
Learn more about the work of our research integrity team to safeguard the quality of each article we publish.