- 1Department of Soil Resource and Watershed Management, College of Agriculture and Natural Resource, Bonga University, Bonga, Ethiopia
- 2Department of Natural Resource Management, College of Agriculture and Environmental Science, Bahir Dar University, Bahir Dar, Ethiopia
Ethiopia's dominant maize production relies on rain, but growing water scarcity challenges dry season irrigation efforts. This necessitates smarter irrigation techniques to maximize water use efficiency. This study optimizes water use efficiency in maize production through deficit irrigation in Gazhen-Fuafuat kebele, Fogera woreda, Ethiopia. A field experiment was conducted during the 2019/20 dry season, comparing four irrigation levels: 55%, 70%, 85%, and 100% of crop water requirements (ETc). Findings revealed that while higher irrigation levels generally enhanced plant growth and grain yield, irrigation water use efficiency was optimized at 70% ETc. Deficit irrigation at 55% ETc proved to be suboptimal, leading to significant reductions in crop growth and grain production. Conversely, applying 70% ETc resulted in a 30% reduction in irrigation water use without compromising yield. Compared to full irrigation, deficit irrigation at 85% ETc, 70% ETc, and 55% ETc resulted in yield reductions of 8%, 13.5%, and 33.1%, respectively. However, these reductions were accompanied by water savings of 15%, 30%, and 45%, respectively, leading to corresponding increases in water use efficiency of 8%, 23.4%, and 21.9%. These results suggest that deficit irrigation practices can be effectively employed to improve water use efficiency in maize production, especially in the study area facing water scarcity. This study provides valuable insights into the potential of deficit irrigation to improve maize production in Ethiopia while conserving water resources. Therefore, by implementing deficit irrigation strategies and supporting farmers with appropriate training and resources, Ethiopia can enhance its agricultural productivity and ensure food security in the face of increasing water scarcity.
1 Introduction
Agriculture forms the cornerstone of the Ethiopian economy, significantly impacting national income, employment, foreign exchange earnings, and overall Gross Domestic Product (GDP) (Makombe et al., 2011; Awulachew et al., 2010). Currently, Ethiopia relies heavily on rain-fed agriculture, with limited irrigation practices (Belete, 2006). This dependence on unpredictable rainfall presents a significant vulnerability to food security and economic stability.
To address these challenges, Ethiopia is increasingly implementing irrigation development strategies. These initiatives aim to enhance agricultural productivity and diversify food and raw material production for agro-industries (Ayana, 2011). Recognizing the critical role of water resource management, the government has prioritized water harvesting and small-scale irrigation projects (Hagosa et al., 2010; Awulachew and Ayana, 2011). Deficit irrigation offers a promising solution in areas with limited water resources. This practice involves strategically under-irrigating crops to optimize water consumption while minimizing yield reductions due to water stress (Dağdelen et al., 2006). Deficit irrigation strategies can significantly improve water use efficiency (WUE) in agriculture, potentially allowing for the cultivation of additional land (Ali et al., 2007; Patel and Rajput, 2013; Narayanan and Seid, 2015).
Maize stands as a leading global cereal crop, playing a vital role in global food security (Shiferaw et al., 2011). It constitutes a staple food source for billions worldwide (Ignaciuk and Mason-D'Croz, 2014) and holds immense importance in Ethiopia, ranking first in both production and area coverage (CSA (Central Statically Agency), 2017). Ethiopian farmers primarily cultivate maize for subsistence, with a large portion consumed by farming households themselves (CSA, 2012). Ethiopian farmers primarily cultivate maize for subsistence, with a large portion consumed by farming households themselves (CSA, 2012).
Rising irrigation costs and dwindling global water resources necessitate the development of efficient irrigation methods like deficit irrigation. This approach aims to maximize WUE and minimize water use (FAO (Food and Agricultural Organization), 1996). While the study area isn’t prone to drought, winter seasons experience uncertain irrigation water supplies. To sustain their livelihoods, farmers heavily rely on irrigation, leading to water scarcity and unequal water allocation. The escalating demand for water due to the expansion of irrigated agriculture has resulted in significant water scarcity in the study area. Deficit irrigation presents a potential solution, involving the strategic application of controlled water stress to maize crops during specific growth stages to optimize water use efficiency. The water scarcity results in unequal irrigation water allocation and raises conflicts among the irrigators. Certain farmers located in close proximity to irrigation sources may be inadvertently over-irrigating their fields. The others who have land far from the source cannot get enough irrigation water or sometimes no Water at all. Therefore, deficit irrigation is one technique of managing limited water resources through exposing the crop to a certain level of water stress during a particular period or the whole growing period, but it must be identified with the level of irrigation that minimize water demand with minimal impact on yield. This research was also conducted considering the above facts and the sensitivity of maize to moisture stress. The objectives of this study were: (1) To analyze primary evaluation of deficit irrigation on maize growth and yield. (2) To determine the water use efficiency of different levels of irrigation water application. (3) To identify the optimal irrigation regime that maximizes water use efficiency while ensuring acceptable crop production under deficit irrigation conditions in the Gazhen-Fuafuat Kebele, Northwest Ethiopia.
2 Materials and methods
2.1 Description of the study area
The experiment was conducted in Gazhen-Fuafuat kebele, Fogera woreda, Amhara National Regional State, Ethiopia. Situated approximately 26 kilometers south of Woreta town, the study site is characterized by favorable conditions for agriculture and livestock rearing, with an altitude ranging from 1774 to 2410 meters (Figure 1). The two major rivers, Gumara and Reb, play a crucial role in the local economy, particularly for irrigation during the dry season. These rivers support the cultivation of horticultural crops, primarily vegetables, in the surrounding kebeles. The selection of this study site was influenced by its accessibility, irrigation water availability, and the supportive local community (Fogera Woreda Agricultural Office (FWAO), unpublished data).
The study area is characterized by a semi-arid climate with a bimodal rainfall regime. This climatic pattern is characterized by two distinct periods of precipitation throughout the year. The average annual rainfall is 1215 mm, ranging from 1100 to 1340 mm. According to data from the Bahir Dar meteorological weather station, the annual rainfall in the Kebele varies between 1163.0 and 1684.7 mm. The area also experiences a monthly mean maximum and minimum temperatures of 30.7°C (April) and7.6°C (January), respectively. Figure 2 provides a detailed breakdown of temperature and rainfall during the experimental period.
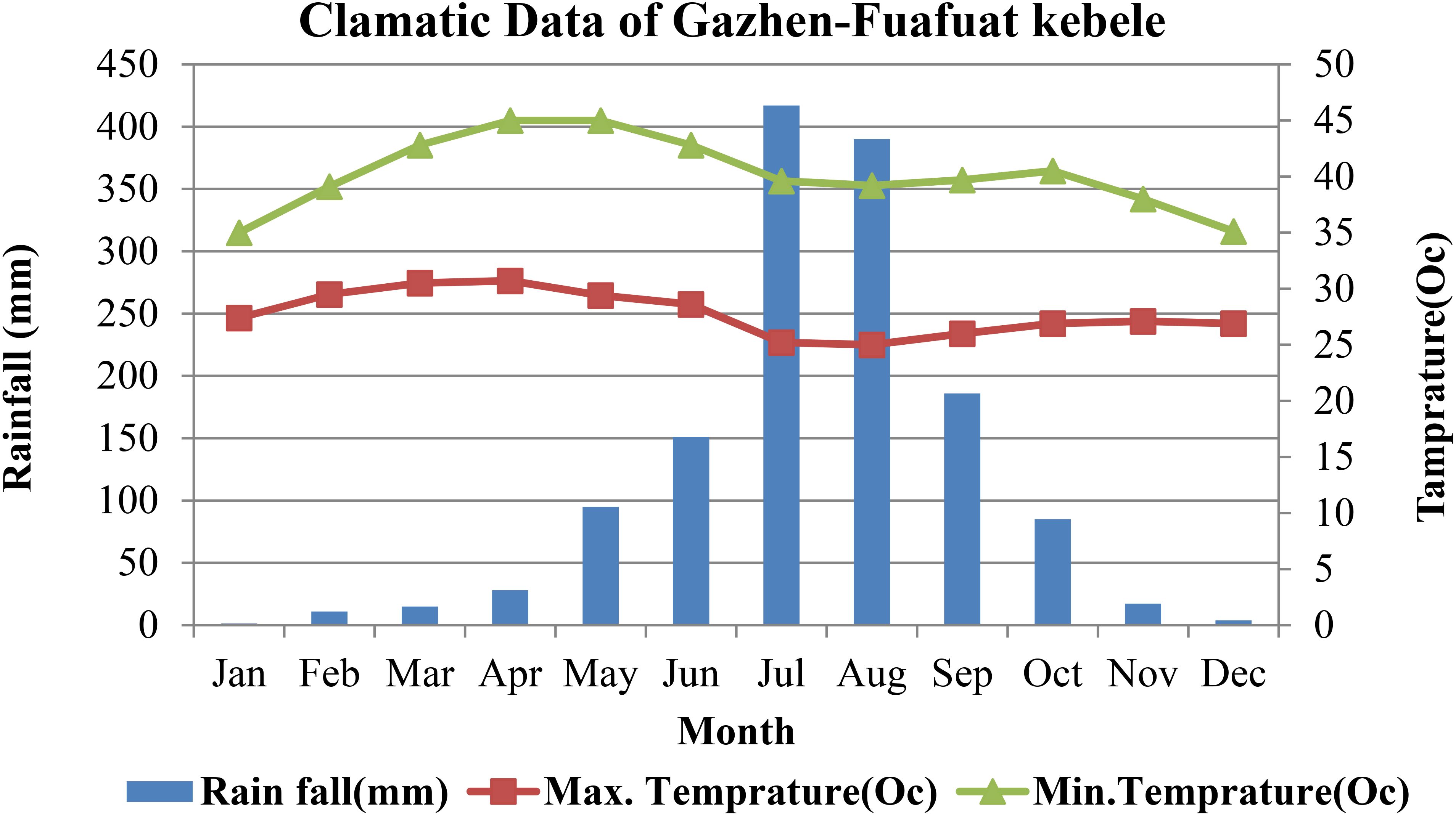
Figure 2. Mean monthly rainfall and temperature of the study area (2008-2018); (Source: National Metrological Agency, Bahir Dar Branch).
2.2 Soil type, topography and irrigation practice in the study area
Irrigation practices in the study area have undergone a significant transformation in recent years. Traditionally reliant on small-scale gravitational irrigation systems, the region has witnessed a substantial expansion of irrigated agriculture through the diversion of the Gumara River using motor pumps. The construction of irrigation canals, facilitated by oxen, has enabled the efficient distribution of water to agricultural fields. A variety of irrigation methods, including basin, furrow, and border irrigation, are employed in the study area. The primary crops cultivated under irrigation are maize and teff, which serve as food for people and feed for the livestock. While vegetable production is currently limited by transportation constraints, the region’s fertile alluvial soils, deposited by annual floods, offer considerable potential for diversified crop cultivation.
Gazhen-Fuafuat kebele’s predominantly flat topography, coupled with its proximity to water sources, provides favorable conditions for irrigation development. However, waterlogging can be a challenge in certain areas, particularly in the plains. The Kebele’s soils exhibit distinct characteristics, with black clay soils (ferric vertisols) dominating the lowlands and orthic luvisols prevalent in the higher altitudes. The alluvial vertisols deposited by nearby rivers in the lower plains are renowned for their fertility and agricultural productivity, provided that flooding is managed effectively (Fogera Woreda Agricultural Office (FWAO), unpublished data).
2.3 Experimental design
A completely randomized block design (RCBD) was implemented to evaluate the impact of varying irrigation regimes on maize growth. This design incorporated four irrigation treatments replicated three times. The treatments consisted of:
1. Full Irrigation (100%ETc): This treatment aimed to satisfy the crop’s evapotranspiration (ETc) demand entirely through a combination of effective rainfall and applied irrigation water.
2. Deficit Irrigation: Three levels of deficit irrigation were included, providing 85%, 70%, and 55% of the full irrigation amount (100% ETc).
The experiment utilized a designated field area. The gross area encompassed 575 m² (57.5 m x 10 m), while the net experimental area dedicated to planting was 315.25 m² (48.5 m x 6.5 m). Individual treatment plots measured 6.5 m x 3.75 m, resulting in an area of 24.375 m². Buffer zones of 1.5 m separated plots within blocks, while 2m buffer zones were implemented between blocks themselves. A further 2m buffer zone separated the experimental area from neighboring fields. Maize planting employed a spacing of 75 cm between rows and 25 cm within rows, establishing 5 rows per plot. This optimized plant distribution and facilitated efficient resource utilization. Full irrigation was designated as the control treatment, serving as a reference point for evaluating the performance of the deficit irrigation strategies. Throughout the growing season, irrigation application was meticulously monitored to ensure that all plots achieved field capacity (FC). The total irrigation water applied for each treatment over the season was meticulously documented to quantify water use efficiency under varying irrigation regimes.
2.4 Sowing and other cultural practices
Field experiments were conducted in the dry season starting from December, 02, 2019 to April, 20, 2020 after the summer cereal collected from farm land. Maize (Zea mays l.) variety BH-540 was used as a test crop where two seeds per hill were planted by hand with a spacing of 25 cm between hills and 75 cm between rows on a net plot size of 6 m long by 3 m wide. The total plant population was about 69444 plants ha-1. After the crop fully germinated, seedlings were thinned to one plant per hill to obtain a population of 34722 plants ha-1. Urea fertilizer was also applied based on the local practice of the study area near to the flowering stage. Crop management during the growing season included dibbling once and weeding twice to control weeds and create favorable growing conditions. Additionally, a botanical insecticide application targeted insect pests and specifically the stalk borer, Busseola fusca.
2.5 Data collection
Following the acquisition of climatic and soil data, researchers conducted a comprehensive assessment of crop growth and yield. This assessment included the measurement of growth parameters (plant height, leaf area index, and above-ground biomass) throughout the growing season. Additionally, at harvest, grain yield and yield components (number of ears per plant, number of grains per ear, ear length, and 1000-grain weight) were meticulously evaluated.
2.6 Soil sampling and analysis
Composite soil samples were collected randomly from the experimental field at three depth intervals: 0-30 cm, 30-60 cm, and 60-100 cm. These samples were subsequently analyzed at the Amhara Designs Supervision Work Enterprise Soil Laboratory to determine key soil chemical and physical properties.
2.6.1 Soil texture analysis
Soil texture, a critical factor influencing plant growth, cultivation practices, hydraulic conductivity, and soil strength, was assessed using the hydrometer method. This widely accepted laboratory technique, originally introduced by Bouyoucos, 1927 and refined by Day (1965) and the American Society for Testing and Materials (1972), involves suspending a measured soil sample in water and measuring the suspension density over time as particles settle. The resulting data are used to calculate the percentage of each particle size class (sand, silt, and clay).
2.6.2 Electrical conductivity, pH, and organic matter
The electrical conductivity (EC) of the soil was assessed through the analysis of saturated soil paste extracts, adhering to the methodology outlined by van Reeuwijk (1992). Soil pH was determined potentiometrically using a 1:2.5 soil-to-water suspension, following established protocols. Organic carbon content was quantified using the wet combustion procedure described by Walkley and Black (1934). Consequently, the organic matter content was estimated by multiplying the measured organic carbon value by a conversion factor of 1.724.
2.6.3 Soil water properties
Undisturbed soil samples were collected using a core sampler at three depths (0-30 cm, 30-60 cm, and 60-100 cm) within the experimental field. Soil water retention characteristics, including field capacity (FC) and permanent wilting point (PWP), were determined using a pressure plate and pressure membrane apparatus, following the methodology of Klute (1965). The total available soil water content was calculated as the difference between FC and PWP moisture contents (Hillel, 1982). Soil bulk density was determined as the ratio of oven-dried soil mass to the bulk volume of the soil core (Blake, 1986).
2.6.4 Moisture content calculation
The moisture content at each soil water property (FC and PWP) was expressed on a gravimetric basis. The gravimetric water content was calculated using the standard equation, as outlined in the literature (FAO (Food and Agricultural Organization), 1989; Jury et al., 1991; Evans et al., 1996). The gravimetric water content was calculated using the following equation:
2.7 Growth, yield and yield parameters measurement
2.7.1 Leaf area index
Measurements were obtained at physiological maturity from nine randomly selected plants in each plot. Leaf area was estimated by multiplying leaf length and maximum width, followed by the application of a correction factor of 0.75 (Francis et al., 1969) to account for non-planar leaf surfaces. LAI represents the total one-sided leaf area per unit ground area occupied by the crop canopy.
2.7.2 Plant height
Plant height (cm) was measured at physiological maturity (end of March) from the base of the plant to the tip of the panicle using a meter tape on nine randomly selected plants within each plot.
2.7.3 Growth and yield parameters
Following harvest, destructive sampling was employed on nine randomly chosen plants from each plot. These plants were sun-dried for two weeks to determine aboveground biomass, grain yield, and 1000-grain weight.
● Aboveground Biomass: Nine plants were randomly selected post-harvest and sun-dried for a period of two weeks to determine above-ground biomass from each plot.
● Grain Yield: Weight (kg) of harvested grain from nine randomly selected plants in each plot.
● 1000-Grain Weight: Average dry weight (g) of 1000 individual grains from each plot, measured using a digital balance.
● Number of Ears per Plant: Counted on nine randomly selected maize plants within each plot.
● Number of Grains per Ear: Determined by counting the grains from nine randomly selected ears per plot.
● Ear Length: Average length (cm) of nine ears measured from each plot.
2.8 Data analysis
2.8.1 Determination of crop water requirement and irrigation requirement
The daily reference evapotranspiration (ET0) was calculated using the FAO’s CROPWAT 8.0 software (Smith, 1992). The crop water requirement was subsequently determined by multiplying ET0 by the corresponding crop coefficient (Kc). Following this, irrigation requirements were calculated. The actual irrigation depth was calculated as the difference between the crop water requirement and the effective precipitation depth.
2.8.2 Irrigation water application
River water was diverted from the main channel into an irrigation canal and subsequently distributed to individual furrows within treatment plots (Table 1). The Water flow was carefully regulated to prevent over-irrigation. The volume of irrigation water applied to each plot was calculated using the following formula (Doorenbos and Pruitt, 1992):
Irrigation water discharge was measured using the float method (Bessembinder et al., 2005). A tennis ball was allowed to drift along a 20-meter straight section of the irrigation canal, and its travel time was recorded using a stopwatch. This measurement was repeated three times to ensure accuracy. The average velocity of the water flow was calculated and adjusted using a correction factor of 0.85 to account for channel irregularities. The width and depth of the irrigation canal were measured at ten points along the 20-meter section. The average values were used in the discharge calculation. The discharge was calculated using the following formulas (Bessembinder et al., 2005):
The time required to apply the desired water depth to each plot was calculated using the following relationship, as suggested by Jensen (1982):
2.9 Calculation of harvest index and water use efficiency
Harvest index (HI%) can be calculated as the ratio of grain yield (Y) and the total above ground biomass (B) at maturity (Huehn, 1993). Irrigation water use efficiency is the yield harvested in kilograms per total water used. Irrigation water use efficiency was calculated as follows (Payero et al., 2008). Irrigation water use efficiency IWUE (kg/m3) is the grain yield (kg/ha) divided by seasonal irrigation water applied (m3/ha).
2.10 Statistical analysis
The collected data were subjected to a one-way analysis of variance (ANOVA) using the General Linear Model (GLM) procedure within SAS version 9.2 software (Der and Everitt, 2008). This statistical technique enabled the assessment of significant differences among the various deficit irrigation treatments. Following the ANOVA, a Least Significant Difference (LSD) test with a significance level of α = 0.05 was employed for post-hoc comparisons. This test facilitated the identification of specific treatment combinations that differed significantly in terms of their impact on the measured parameters (plant height, yield, water use efficiency). The results of the ANOVA and LSD tests are presented in tables within the main body of the report, providing a clear and concise overview of the statistical analyses.
3 Results and discussion
A comprehensive assessment of the effects of deficit irrigation on maize growth, yield, and water use efficiency was undertaken. This evaluation employed a dual approach: Direct Measurement of Growth and Yield Parameters: Quantitative data on various plant growth and yield characteristics were collected throughout the experiment. These parameters included plant height, number of ears per plant, grain yield, and other relevant metrics. Indirect Assessment of Water Use Efficiency the CROPWAT software was utilized to estimate crop water requirements under different irrigation scenarios. By comparing the water applied with the estimated water needs, water use efficiency was indirectly determined. This indirect approach provided insights into the efficiency with which maize utilized available water under various irrigation regimes. Combining the direct and indirect evaluation methods, the study aimed to achieve a holistic understanding of the relationship between deficit irrigation strategies, plant growth performance, crop yield, and water use efficiency.
3.1 Crop and irrigation water requirement
The experiment involved the cultivation of maize (Zea mays L.) during the period of December 2019 to April 2020. Notably, the study region experienced negligible precipitation throughout this period. This resulted in a pronounced water deficit, necessitating irrigation for successful crop production (Table 2).
3.2 Influence of soil depth on selected soil physico-chemical properties
Laboratory analysis of soil samples from the study site revealed a clay loam soil texture, comprising 36.33% sand, 32.33% silt, and 31.33% clay. The soil exhibited a slightly acidic pH, ranging from 6.21 to 6.04 at depths of 0-30 cm, 30-60 cm, and 60-100 cm respectively. Additionally, the soil’s electrical conductivity (EC), organic carbon content, and organic matter content were determined to be within the following ranges: EC: 0.049-0.062 dS/m, Organic Carbon: 1.09-1.80%, and Organic Matter: 1.88-3.03%. These soil properties, summarized in (Table 3), provide valuable insights into the physical and chemical characteristics of the experimental site and their potential influence on crop growth and water retention.
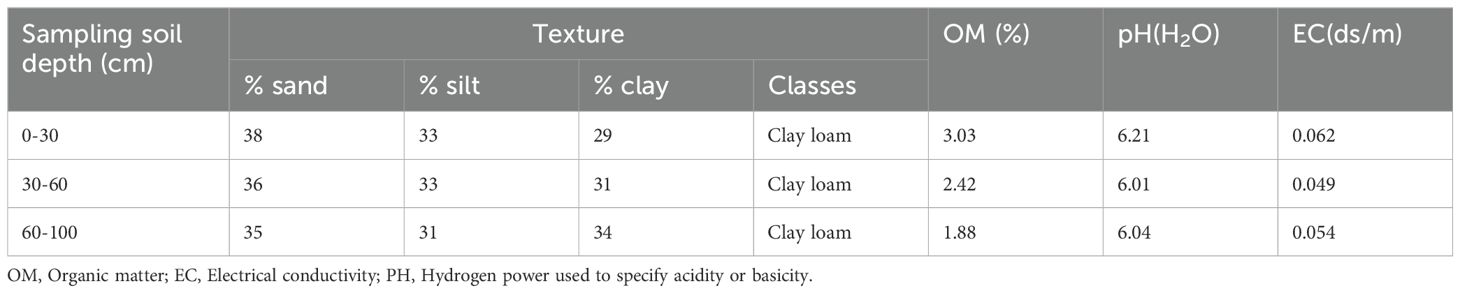
Table 3. Influence of soil depth on selected soil physico-chemical properties at the experimental site.
3.3 Experimental site soil characteristics
The experimental site has soil moisture content at field capacity ranged from 29.98% to 33.03% and soil moisture content the permanent wilting point ranged from 17.84% to 20.47%. Bulk density and total available water ranges from 1.34-1.41 g/m3 and 131.18 -203.51 mm/m, respectively (Table 4).
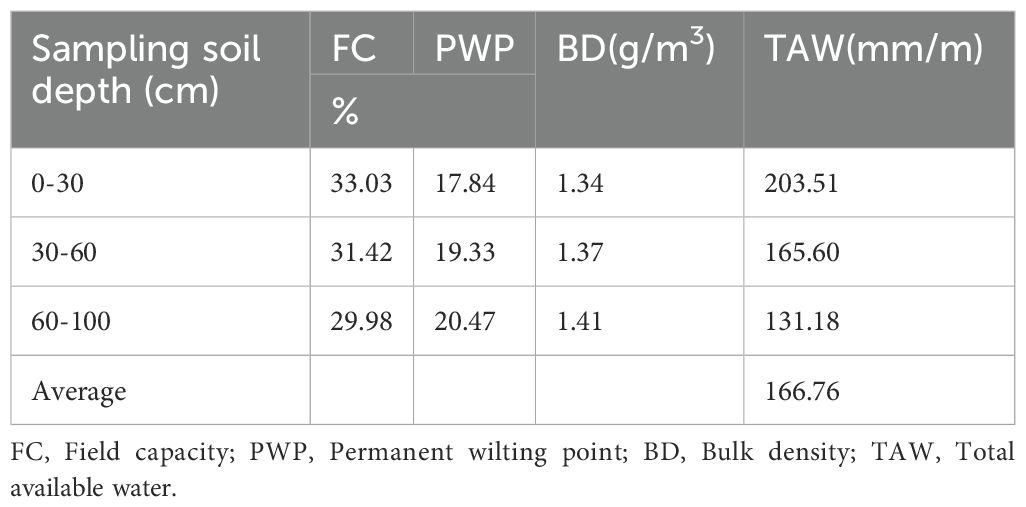
Table 4. Soil moisture content and bulk density of the soil profile at different depths of the experimental site.
3.4 Growth components of maize
3.4.1 Effects of irrigation on plant height
The analysis of variance (ANOVA) revealed a statistically significant (p < 0.01) effect of irrigation level on plant height, as detailed in Table 5. Plants receiving full irrigation (100% ETc) exhibited the greatest average height, followed by those under 85% Etc. Importantly, no significant difference in plant height was observed between these two treatments. Similarly, the 70% ETc treatment produced plants with heights statistically indistinguishable from the 85% ETc group. Conversely, the 55% irrigation level resulted in the lowest average plant height. These findings align with the established trend that plant height generally increases with greater water availability. This observation is corroborated by the works of Bozkurt et al. (2006); Cakir (2004); Istanbulluoglu et al. (2002); Otegui et al. (1995), and Pandey et al. (2000), who all reported that maize under full irrigation achieved the highest average heights. Further support for this notion comes from El-Noemani et al. (2009) and Admasu et al. (2017), who suggested a proportional relationship between plant growth and irrigation level.
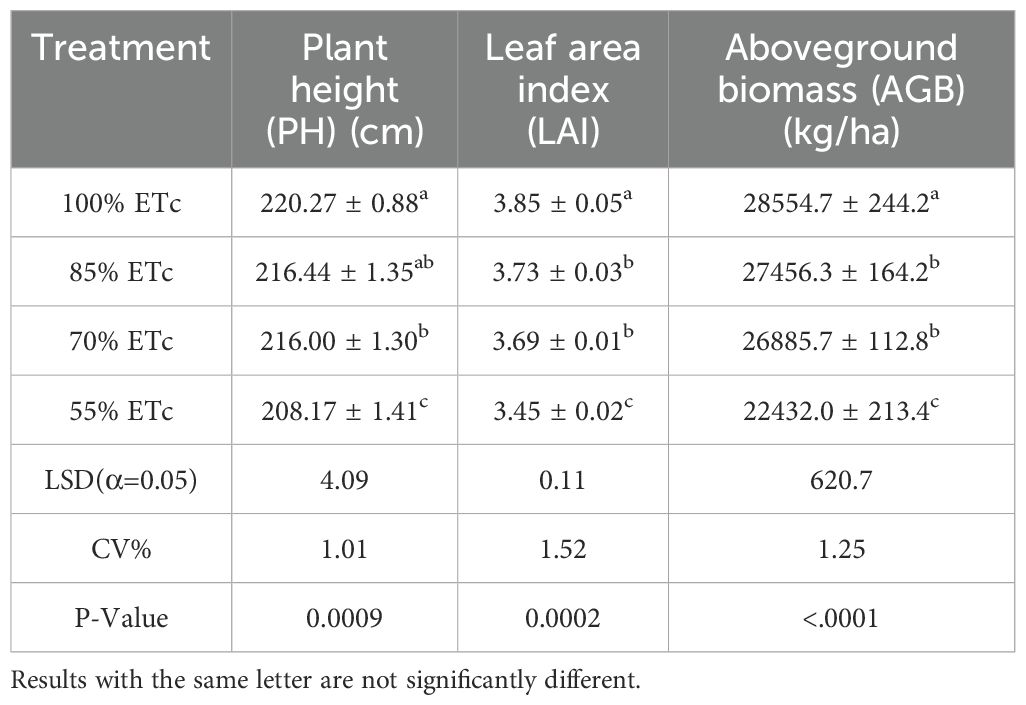
Table 5. Growth Parameters of the Plants, Including Plant Height (PH), Leaf Area Index (LAI), and Aboveground Biomass (AGB).
However, it is important to acknowledge contrasting findings from Furgassa (2017) and Gebreigziabher (2020), who reported no significant impact of the irrigation level on maize plant height. These discrepancies indicate that the influence of irrigation on plant height may be contingent on additional factors beyond water availability, potentially including specific environmental conditions or the maize cultivar employed in the study.
3.4.2 Impact of irrigation on biomass accumulation
A statistically significant difference (p < 0.01) was observed in aboveground biomass accumulation based on the water supplied (Table 5). Plants subjected to full irrigation exhibited the highest biomass, followed by those receiving 85% of their crop water requirement. The lowest biomass was recorded in the treatment, receiving only 55% of its evapotranspiration (ETc) needs throughout the growing season. These findings suggest a direct correlation between the irrigation level and aboveground biomass production. These results align with previous research conducted by Yenesew and Tilahun (2009), who reported the highest biomass yield under 100% ETc irrigation throughout the growing season. Similarly, Ullah et al. (2003) confirmed that varying irrigation levels significantly affect biological yield, which is closely linked to aboveground biomass. Ayana (2011) further corroborated these findings by demonstrating that maximum biomass was achieved with 100% ETc irrigation. Additionally, Moser et al. (2006) reported a reduction in biomass under moisture stress conditions, further supporting the observed relationship.
3.4.3 Influence of irrigation on maize leaf area index
A statistically significant difference was observed in the leaf area index (LAI) among the various moisture stress treatments applied. As shown in Table 5, the 100% ETc irrigation level resulted in the highest LAI, while the lowest value was recorded at the 55% ETc stress level. This aligns with established literature, where maize LAI during the grain filling period typically falls within a range of 2-6 (Tollenaar, 1986). The findings of this study further support this established range, as evidenced by the data presented in (Table 5). Similarly, previous research by Gonzalez et al. (2005) reported a maximum LAI range of 2.9-7.14, which aligns with the current study’s observations with the exception of the 55% ETc treatment (Table 5). In corroborating these findings, Azarpanah et al. (2013) demonstrated a significant effect of irrigation regimes on LAI, highlighting a decrease in leaf surface area with reduced irrigation levels. Likewise, Greaves and Wang (2017) reported a statistically significant impact of irrigation treatments on maize leaf area index (LAI), with a mean value of 5.94.
3.5 Impact of irrigation on yield and yield components of maize
3.5.1 Grain yield
This study investigated the relationship between irrigation water availability and grain yield. Results revealed a statistically significant (p < 0.01) difference in grain yield among moisture stress treatments (Table 6). The control treatment (100% ETc), representing non-water-stressed conditions, produced the highest yield (Table 6). Grain yield progressively decreased with increasing moisture stress levels (85% ETc, 70% ETc, and 55% ETc), with statistically significant differences observed between treatments (Table 6).
These findings corroborate previous research by Mansouri-Far et al. (2010) who demonstrated a negative impact of the irrigation water reduction on grain yield. Similarly, Ullah et al. (2003) reported a significant positive correlation between irrigation level and grain yield. Our results further support the established body of knowledge documented by Nadanam and Morachan (1974); Hiraoka et al. (1976); Lazarov et al. (1976); Warrick and Gardner (1983); Karlen and Camp (1985), and van Averbeke and Marais (1992), all of whom observed a direct association between increased irrigation and enhanced grain yield.
3.5.2 Number of ear per plant
A study investigating the influence of irrigation on corn yield revealed a statistically significant effect (p < 0.05)) on the number of ears produced per plant. While no significant differences were observed between most irrigation treatments, plants receiving only 55% of their potential evapotranspiration (ETc) exhibited a marked decrease in ear number compared to those receiving full irrigation (100% ETc). These findings support previous research by Cakir (2004); Karasu et al. (2015), and Pandey et al. (2000), who all demonstrated that water stress negatively impacts ear production in corn.
3.5.3 Number of grain per ear
The number of grains per ear was significantly influenced by the irrigation level at (p<0.01). The maximum grain number per ear was achieved with a 100% ETc irrigation level, followed by 85% ETc, with no significant difference between them. Similarly, there was no significant difference between 85% ETc and 70% ETc moisture deficist (Table 6). The minimum number of grains per ear was recorded at 55% Etc. This finding aligns with Ertek and Kara (2013), who reported that deficit irrigation reduced the number of grains per ear. Ullah et al. (2003) supported this result, concluding that varying irrigation levels significantly impacted the number of grains per ear. These findings have also been documented in other studies for maize (Aydinsakir et al., 2013; Karasu et al., 2015; Moosavi, 2012). Conversely, Elzubeir and Mohamed (2011) found that the amount of irrigation water did not affect the number of kernels per ear.
3.5.4 Ear length
The level of irrigation had a highly significant impact on the ear length of maize at (p< 0.01) (Table 6). These findings indicated that the ear length of maize was greater at 100% ETc and shorter at 55% ETc irrigation levels. Among the treatments, 85% ETc and 70% ETc showed no significant difference. This outcome is consistent with the findings of Ertek and Kara (2013), who demonstrated that ear length, was influenced by varying irrigation water levels and reported a decrease in ear length with reduced water application. Contrary to this result, Tabatabaei and Dadashi (2013) found that irrigation levels had no significant effect on ear length.
3.5.5 Impact of irrigation on 1000 grain weight of maize
Irrigation significantly impacted maize 1000-grain weight. As shown in Table 6, the highest 1000-grain weight was observed under 100% ETc irrigation, followed by 85% Etc. No significant difference was found between these treatments or between 85% ETc and 70% Etc. Conversely, the lowest 1000-grain weight was recorded under 55% ETc irrigation. These results corroborate the existing literature. Ullah et al. (2003) and Mansouri-Far et al. (2010) previously reported a positive correlation between irrigation levels and 1000-grain weight. Similarly, Aydinsakir et al. (2013); Cakir (2004), and Karam et al. (2003) observed a decrease in 1000-grain weight due to water deficits. However, some studies, such as those by Elzubeir and Mohamed (2011) and Yazar et al. (2009) did not find a significant association between irrigation water amounts and grain weight.
3.6 Irrigation water use efficiency and harvest index
3.6.1 Effect of irrigation level on harvest index
A highly significant effect (p<0.01) of the irrigation level on the maize harvest index was observed through an analysis of variance (Table 7). Plants receiving 100% of their reference evapotranspiration (ETc) exhibited the greatest harvest index, while those under 55% ETc displayed the lowest. This progressive decrease in harvest index with decreasing irrigation level suggests a strong dependence of grain formation on soil moisture content. These findings align with those of Ullah et al. (2003) and Toor (1990), who reported significant impacts of irrigation levels on harvest index. However, Furgassa (2017) observed no significant difference in the harvest index under varying irrigation, suggesting the potential influence of additional factors in specific contexts.
3.6.2 Effect of irrigation level on maize yield and irrigation water use efficiency
Variance analysis indicated that the irrigation level had a highly significant (p<0.01) impact on the irrigation water use efficiency of maize (Table 7). The maximum irrigation water use efficiency was achieved with the 70% ETc treatment, followed by 55% ETc, with no significant difference between the two. This finding suggests that the 70% ETc irrigation level is preferable over the 55% ETc, 85% ETc, and 100% ETc levels for water conservation without a notable yield reduction, allowing for additional land production. Conversely, the lowest water productivity was observed at 100% Etc. The results indicate that irrigation water use efficiency decreased with increasing water supply, except at the 70% ETc level. In support of this, Bozkurt and Yazar (2011) reported that irrigation water use efficiency values increased with decreasing seasonal irrigation amounts. These findings align with those of Yenesew and Tilahun (2009); Lee et al. (2011); Karrou et al. (2012); Narayanan and Seid (2015); Admasu et al. (2017), and Furgassa (2017), who demonstrated that irrigation water use efficiency significantly increased as irrigation levels were reduced. However, Payero et al. (2006) showed that applying deficit irrigation to boost water productivity might not be a beneficial strategy. In this context, limited irrigation of maize is not a viable practice. In this study, 55% ETc reduced yield but had lower irrigation water use efficiency compared to the 70% ETc level.
As shown in Table 8, deficit irrigation at 85% ETc resulted in an 8% yield penalty to save 15% irrigation water, with a corresponding 8% increase in irrigation water use efficiency. Deficit irrigation at 70% ETc led to a 13.5% yield penalty, increasing irrigation water use efficiency by 23.4% and saving 30% water. Deficit irrigation at 55% ETc saved 45% water and increased irrigation water use efficiency by 21.9%, but resulted in a 33.1% yield loss. The study results indicate that among the deficit treatments, deficit irrigation at 70% ETc can save water and increase irrigation water use efficiency without significant yield reduction compared to the other treatments.
3.7 Correlation
The analysis of treatment variables in Table 8 revealed statistically significant correlations (p < 0.01) between most variables. Notably, all correlations were positive except for irrigation water use efficiency (WUE), which exhibited a significant negative association. Grain yield demonstrated the strongest positive correlation (R = +0.98) with both aboveground biomass and ear length. Conversely, WUE displayed the weakest negative correlation (R = -0.75) with 1000-seed weight. These findings corroborate prior research by Ilker (2011); Hasyan et al. (2012), and Kumar et al. (2014), who reported significant correlations between maize yield and related traits.
4 Conclusion
This study examined the efficacy of deficit irrigation in optimizing water utilization and maize yield in Ethiopia, where maize reigns supreme as the leading food crop. Water scarcity poses a significant challenge to maize productivity, prompting the exploration of deficit irrigation strategies for improved water management. The results demonstrated a statistically significant influence of varying irrigation levels on all measured parameters, encompassing growth parameters, yield components, harvest index, and water use efficiency. Notably, a significant difference in yield was observed between applying 55% and 100% of the reference evapotranspiration (ETc). While the highest water use efficiency was achieved with 70% ETc irrigation without compromising growth or yield significantly, full irrigation (100% ETc) yielded the highest values for growth parameters, yield components, and harvest index. Conversely, the most severe deficit irrigation (55% ETc) resulted in the lowest values for these parameters. These findings suggest that deficit irrigation can be a valuable tool in water-scarce environments. Strategic reductions in irrigation water use can facilitate the cultivation of additional land. The study demonstrates the potential to maintain significant grain yield even under deficit irrigation. Compared to full irrigation, deficit irrigation at 85% ETc, 70% ETc, and 55% ETc resulted in yield reductions of 8%, 13.5%, and 33.1%, respectively. However, these reductions were accompanied by water savings of 15%, 30%, and 45%, respectively, leading to corresponding increases in water use efficiency of 8%, 23.4%, and 21.9%. In conclusion, this study provides compelling evidence that strategically implementing deficit irrigation strategies can enhance both water use efficiency and grain production in maize cultivation. This approach offers a promising solution for optimizing maize production in water-scarce environments like Ethiopia.
Data availability statement
The raw data supporting the conclusions of this article will be made available by the authors, without undue reservation.
Author contributions
AB: Formal analysis, Methodology, Writing – original draft, Writing – review & editing. TM: Data curation, Formal analysis, Methodology, Software, Supervision, Writing – original draft, Writing – review & editing. WJ: Formal analysis, Methodology, Software, Writing – original draft, Writing – review & editing.
Funding
The author(s) declare that no financial support was received for the research, authorship, and/or publication of this article.
Conflict of interest
The authors declare that the research was conducted in the absence of any commercial or financial relationships that could be construed as a potential conflict of interest.
Publisher’s note
All claims expressed in this article are solely those of the authors and do not necessarily represent those of their affiliated organizations, or those of the publisher, the editors and the reviewers. Any product that may be evaluated in this article, or claim that may be made by its manufacturer, is not guaranteed or endorsed by the publisher.
References
Admasu R., Tadesse M., Shimbir T. (2017). Effect of growth stage moisture stress on maize (Zea Mays L.) yield and water use efficiency at West Wellaga, Ethiopia. J. Biology Agric. Healthcare 7, 98–103.
Ali M. H., Hoque M. R., Hassan A. A., Khair A. (2007). Effects of deficit irrigation on yield, water productivity, and economic returns of wheat. Agric. Water Manage. 92, 151–161. doi: 10.1016/j.agwat.2007.05.010
American Society for Testing and Material (1972). Standard method for particle-size analysis of soils. Amer. Sot. Testing Materials. D422-63 and approved 1972.
Awulachew S. B., Ayana M (2011). Performance of irrigation: An assessment at different scales in Ethiopia. Exp. Agric. 47, 57–69.
Awulachew S. B., Erkossa T., Namara R. E. (2010). Irrigation Potential in ETHIOPIA– Constraints and Opportunities for Enhancing the System (Colombo, Sri Lanka: International Water Management Institute).
Ayana M. (2011). Deficit irrigation practices as alternative means of improving water use efficiencies in irrigated agriculture: Case study of maize crop at Arba Minch, Ethiopia. Afr. J. Agric. Res. 6, pp.226–pp.235.
Aydinsakir K., Erdal S., Buyuktas D., Bastug R., Toker R. (2013). The influence of regular deficit irrigation applications on water use, yield, and quality components of two corn (Zea mays L.) genotypes. Agric. Water Manage. 128, 65–71. doi: 10.1016/j.agwat.2013.06.013
Azarpanah A., Alizadeh O., Dehghanzadeh H., Zare M. (2013). The effect of irrigation levels in various growth stages on morphological characteristics and yield components of Zea mays (L.). Tech. J. Eng. Appl. Sci. 3, 1447–1459.
Belete A. (2006). Determinants of farmers willingness to pay for the conservation of the National parks: The case of semen Mountains National park. Thesis submitted to Haramaya University. MSc.
Bessembinder J., Leffelaar P., Dhindwal A., Ponsioen T. (2005). Which crop and which drop, and the scope for improvement of water productivity. Agric. Water Manage. 73, 113–130. doi: 10.1016/j.agwat.2004.10.004
Bouyoucos G. J. (1927). The hydrometer as a new method for the mechanical analysis of soils. Soil Sci. 23, 343–354. doi: 10.1097/00010694-192705000-00002
Bozkurt S., Yazar A. (2011). Effects of different drip irrigation levels on yield and some agronomic characteristics of raised bed planted corn. Afr. J. Agric. Res. 6, 5291–5300.
Bozkurt Y., Yazar A., Gençel B., Sezen M. S. (2006). Optimum lateral spacing for drip- irrigated corn in the Mediterranean Region of Turkey. Agric. Water Manage. 85, 113–120. doi: 10.1016/j.agwat.2006.03.019
Cakir R. (2004). Effect of water stress at different development stages on vegetative and reproductive growth of corn. Field Crops Res. 89, 1–16. doi: 10.1016/j.fcr.2004.01.005
CSA (2012). “Agricultural Sample Survey 2011-2012,” in Area and Production of Crops, vol. I. (Central Statistical Agency, Ethiopia).
CSA (Central Statically Agency) (2017). Area and production of crops in 2016/2017 cropping season, CSA, Bulletin 584 Vol. I (Addis Ababa, Ethiopia).
Dağdelen N., Yılmaz E., Sezgin F., Gürbüz T. (2006). Water-yield relation and water use efficiency of cotton (Gossypiumhirsutum L.) and second crop corn (Zea mays L.) in western Turkey. Agric. Water Manage. 82, 63–85.
Day P. (1965). “Particle fractionation and particle-size analysis,” in Methods of Soil Analysis, Part 1. Ed. Black C. A. (Amer. Sot. Of Agron., Inc.).
Doorenbos J., Pruitt W. O. (1992). Calculation of crop water requirements. FAO irrigation drainage paper, 1–65.
El-Noemani A. A., Aboamera M. A., Aboellil A. A., Dewedar O. M. (2009). Growth, yield, quality and water use efficiency of pea (Pisumsativum L.) plants as affected by evapo-transpiration and sprinkler height. Minufiya J. Agric. Res. 34, 1445–1466.
Elzubeir A. O., Mohamed A. E. (2011). Irrigation scheduling for maize (Zea mays L.) under desert area conditions-North of Sudan.
Ertek A., Kara B. (2013). Yield and quality of sweet corn under deficit irrigation. Agric. Water Manage. 129, 138–144. doi: 10.1016/j.agwat.2013.07.012
Evans R., Cassel D. K., Sneed R. E. (1996). Measuring soil water for irrigation scheduling: monitoring methods and devices North Carolina Cooperative Extension Service. Available online at: http://www.bae.ncsu.edu/programs//evans (Accessed 29 September 2011).
FAO (Food and Agricultural Organization) (1989). “Guidelines for Designing and Evaluating Surface Irrigation Systems,” in Irrigation and Drainage paper (FAO, Rome, Italy). No. 45.
FAO (Food and Agricultural Organization) (1996). “Deficit irrigation scheduling program for supplementary irrigation,” in Irrigation Scheduling: From theory to practice(Rome, Italy).
Francis C. A., Rutger J. N., Palmer A. F. E. (1969). A rapid method for plant leaf area estimation in maize (Zea mays L.) 1. Crop Sci. 9, 537–539. doi: 10.2135/cropsci1969.0011183X000900050005x
Furgassa Z. S. (2017). The effect of deficit irrigation on maize crop under conventional furrow irrigation in Adami Tulu Central Rift Valley of Ethiopia. Appl. Eng. 1, 1–12.
Gebreigziabher E. T. (2020). Effect of deficit irrigation on yield and water use efficiency of maize at Selekleka District (Ethiopia).
Gonzalez A. D. B., Kiniry J. R., Maas S. J., Tiscareno M. L., Jaime M. C., Mendoza J. L., et al. (2005). Agronomic modeling. Large-Area maize yield forecasting using leaf area index based yield model. Am. Soc. Agron. J. 97, 418–425.
Greaves G. E., Wang Y. M. (2017). Yield response, water productivity, and seasonal water production functions for maize under deficit irrigation water management in southern Taiwan. Plant Production Sci. 20, 353–365. doi: 10.1080/1343943X.2017.1365613
Hagosa F., Makombe G., Namara R. E., Awulachew S. B. (2010). Importance of irrigated agriculture to the Ethiopian economy: capturing the direct net benefis of irrigation Vol. 128 (Colombo, Sri Lanka: IWMI), 37.
Hasyan R. M., Moualla Y. M., Ahmad A. A. S. (2012). Potence ratio and path coefficient analysis for some quantitative traits of maize (Zea mays L.) hybrids developed in Syria. Jordan J. Agric. Sci. 8, 557–565.
Hiraoka H., Sasiprapa V., Piyawongsombon. W. (1976). “Irrigation effect on maize and soyabean,” in Tech. Bulletin, vol. 20. (Tropical Agricultural Research Center, Japan), 28–34.
Huehn M. (1993). Harvest index versus grain/straw-ratio. Theoretical comments and experimental results on the comparison of variation. Euphytica 68, 27–32. doi: 10.1007/BF00024151
Ignaciuk A., Mason-D'Croz D. (2014). “Modelling Adaptation to Climate Change in Agriculture,” in OECD Food, Agriculture and Fisheries Papers (OECD Publishing). No. 70.
Ilker E. (2011). Correlation and coefficient analysis in sweet corn. Turkish J. Field Crops 16, 105–107.
Istanbulluoglu A., Kocaman I., Konukcu F. (2002). Water use-production relationship of maize under Tekirdag conditions in Turkey. Pakistan J. Biol. Sci. 5, 287–291.
Jensen M. E. (1982). Design and operation of farm irrigation systems. Agric. Water Manage. 5, 269–270. doi: 10.1016/0378-3774(82)90048-8
Jury W. A., Gardner W. R., Gardner W. H. (1991). Soil physics. 5th ed (New York: John Wiley and Sons).
Karam F., Breidy J., Stephan C., Rouphael J. (2003). Evapotranspiration, yield and water use efficiency of drip irrigated corn in the Bekaa Valley of Lebanon. Agric. Water Manage. 63, pp.125–pp.137.
Karasu A., Kuşcu H., Mehmet Ö.Z., Bayram G. (2015). The effect of different irrigation water levels on grain yield, yield components and some quality parameters of silage Maize (Zea mays indentata Sturt.). Notulae Botanicae Horti Agrobotanici Cluj- Napoca 43, 138–145. doi: 10.15835/nbha4319602
Karlen D. C., Camp C. R. (1985). Row spacing, plant population and water management effects in the Atlantic coastal plains. Agron. J. 77, 393–398. doi: 10.2134/agronj1985.00021962007700030010x
Karrou M., Oweis T., El Enein R. A., Sherif M. (2012). Yield and water productivity of maize and wheat under deficit and raised bed irrigation practices in Egypt. Afr. J. Agric. Res. 7, 1755–1760.
Klute A. (1965). “Water holding capacity,” in Methods of soil analysis (Agron. Madison, Wisconsin, USA), 273–2278.
Kumar G. P., Prashanth Y., Reddy V. N., Sudheer S., Rao V. P. (2014). Character association and path coefficient analysis in maize (Zea mays L.). Int. J. Appl. Biol. Pharm. Technol. 5, 257–260.
Lazarov R., Mekhandzhieva A., Ug rchinski S. (1976). Irrigation of maize with reduced irrigation norms. Rasteniev dni Nauki. 13, 40–50.
Lee T. S., Salemi. H., Soom. M. A., Yusoff M. K., Ahmed D. (2011). Effects of deficit irrigationon water productivity and maize yields in arid regions of Iran. Pertanika J. Trop. Sci. 34, 207–216.
Makombe G., Namara R., Hagos F., Awulachew S. B., Ayana M., Bossio D. (2011). A comparative analysis of the technical efficiency of rain-fed and smallholder irrigation in Ethiopia (Colombo, Sri Lanka: International Water Management Institute), 37.
Mansouri-Far C., Sanavy S. A. M. M., Saberali S. F. (2010). Maize yield response to deficit irrigation during low-sensitive growth stages and nitrogen rate under semi-arid climatic conditions. Agric. Water Manage. 97, 12–22. doi: 10.1016/j.agwat.2009.08.003
Moosavi S. G. (2012). The effect of water deficit stress and nitrogen fertilizer levels on morphology traits, yield and leaf area index in maize. Pakistan J. Bot. 44, 1351–1355.
Moser S. B., Feil B., Jampatong S., Stamp P. (2006). Effect of pre-anthesis drought nitrogen fertilizer rate and variety on grain yield, yield components and harvest index of tropical maize. Agric. Water Manage 81, 41–58. doi: 10.1016/j.agwat.2005.04.005
Nadanam M., Morachan Y. B. (1974). Effect of soil moisture on the yield and yield components of maize. Madras Agric. J. 61, 371–375. doi: 10.29321/MAJ.10.A03413
Narayanan K., Seid M. M. (2015). Effect of deficit irrigation on maize under conventional, fixed and alternate furrow irrigation systems at Melkassa, Ethiopia. Int. J. Eng. Res. Technol. (IJERT) 4, 119–126. doi: 10.17577/IJERTV4IS110178
Otegui M. E., Andrade F. H., Suero E. E. (1995). Growth, water use, and kernel abortion of maize subjected to drought at silking. Field Crops Res. 40, 87–94. doi: 10.1016/0378-4290(94)00093-R
Pandey R. K., Maranville J. W., Admou A. (2000). Deficit irrigation and nitrogen effects on maize in a Sahelian environment: I. Grain yield and yield components. Agric. Water Manage. 46, 1–13. doi: 10.1016/S0378-3774(00)00073-1
Patel N., Rajput T. B. S. (2013). Effect of deficit irrigation on crop growth, yield and quality of onion in subsurface drip irrigation. Int. J. Plant Prod 7, 417–436.
Payero J. O., Melvin S. R., Irmak S., Tarkalson D. (2006). Yield response of corn to deficit irrigation in a semiarid climate. Agric. Water Manage. 84, 101–112. doi: 10.1016/j.agwat.2006.01.009
Payero J. O., Tarkalson D. D., Irmak S., Davison D., Petersen J. L. (2008). Effect of irrigation amounts applied with subsurface drip irrigation on corn evapo- transpiration, yield, water use efficiency, and dry matter production in a semiarid climate. Agric. Water Manage. 95, 895–908. doi: 10.1016/j.agwat.2008.02.015
Shiferaw B., Prasanna B. M., Hellin J., Bänziger M. (2011). Crops that feed the world 6. Past successes and future challenges to the role played by maize in global food security. Food Secur. 3, 307–327. doi: 10.1007/s12571-011-0140-5
Smith M. (1992). CROPWAT: A computer program for irrigation planning and management (Food & Agriculture Org). (No. 46).
Tabatabaei S. H., Dadashi M. (2013). Effect of different water levels on the yield of corn with trickle irrigation method (T-tape) in Moghan. Int. J. Scientificand Eng. Res. 4, 1275–1281.
Tollenaar M. (1986). “Effect of assimilate partitioning during the grain-filling period of maize on dry matter accumulation,” in Crenshaw (Allan R. Liss, New York), 551–556.
Toor M. S. (1990). Effect of NPK application on the growth and yield of new maize genotype planted in the two geometrical patterns. M.Sc. (Hons.) (Faisalabad: Agri. Thesis, Dept. Agron. Univ. Agric).
Ullah S., Maqsood M., Farooq M., Hussain and A. Habib. S. (2003). Effect of planting patterns and different irrigation levels on yield and yield component of maize (Zea mays L.). Int. J. Agric. Biol. 5, 64–66.
van Averbeke W., Marais J. N. (1992). Maize response to plant population and soil water supply. I. Yield of grain and total above ground biomass. South Afr. J. Plant Soil. 9, 186–192.
van Reeuwijk L. P. (1992). Procedure for soil analysis. 3rd ed Vol. 371 (The Netherlands, Wageningen: International Soil Reference Center Wageningen. (ISRIC).
Walkley A., Black I. A. (1934). An examination of the Degtjareff method for determining soil organic matter, and a proposed modification of the chromic acid titration method. Soil Sci. 37, 29–38. doi: 10.1097/00010694-193401000-00003
Warrick A. W., Gardner W. R. (1983). Crop yield as affected by spatial variations of soil and irrigation. Water Resour. Res. 19, 181–186. doi: 10.1029/WR019i001p00181
Yazar A., Gökçel F., Sezen M. S. (2009). Corn yield response to partial rootzone drying and deficit irrigation strategies applied with drip system. Plant Soil Environ. 55, 494–503. doi: 10.17221/96/2009-PSE
Keywords: maize, water use efficiency, deficit irrigation, water management, yield
Citation: Melkie T, Jemberu W and Bitew A (2024) Optimizing water use efficiency in maize (Zea mays L.) production through deficit irrigation in Gazhen-Fuafuat Kebele, Northwest Ethiopia. Front. Agron. 6:1490423. doi: 10.3389/fagro.2024.1490423
Received: 04 September 2024; Accepted: 04 November 2024;
Published: 26 November 2024.
Edited by:
Juana Paul Moiwo, Njala University, Sierra LeoneReviewed by:
Vanaja Kankarla, Florida Gulf Coast University, United StatesMohamed Blango, Njala University, Sierra Leone
Copyright © 2024 Melkie, Jemberu and Bitew. This is an open-access article distributed under the terms of the Creative Commons Attribution License (CC BY). The use, distribution or reproduction in other forums is permitted, provided the original author(s) and the copyright owner(s) are credited and that the original publication in this journal is cited, in accordance with accepted academic practice. No use, distribution or reproduction is permitted which does not comply with these terms.
*Correspondence: Amare Bitew, YW1hcmViaXRldzIwMTlAZ21haWwuY29t