- 1Department of Crop Science, Muni University, Arua, Uganda
- 2Nematology Department, Ghent University, Ghent, Belgium
- 3Pest and Disease Suppressive and Enhance Production Department, Bioversity International, Kampala, Uganda
- 4Department of Bio Molecular Resources and Bio Lab Sciences, School of Biosecurity Biotechnical & Laboratory Sciences, Makerere University, Kampala, Uganda
- 5Pest and Disease Suppressive and Enhance Production Department, Bioversity International, Addis Ababa, Ethiopia
- 6Department of Sciences and Vocational Studies, Lira University, Lira, Uganda
Introduction: The management of root-knot nematodes has predominantly been based on use of chemicals, which are detrimental to the environment and human health. Biological control provides alternative management. This study evaluated the potential of using Pleurotus ostreatus, an edible mushroom species to control Meloidogyne spp. in eggplants.
Methods: In vitro, the mortality of juveniles (J2) of Meloidogyne spp. were assessed i) P. ostreatus - water suspension with actively growing mycelia, and ii) different dilutions of P. ostreatus PDB broth culture filtrates. In the screen house nematicidal potential of P. ostreatus was tested on eggplants using artificially inoculated soils in a screen house. To attain this, juveniles (J2) of Meloidogyne spp. were inoculated at the base of plants in pots containing P. ostreatus colonized millet grains mixed in 3 kg of soil. The galling index (GI) (scale of 0 to 5), root growth and nematode populations in the different treatments were assessed.
Results and discussion: Mortality of nematodes in the P. ostreatus - water suspension significantly increased with time, reaching over 88% at 48 h and 95% at 72 h. Mortality in undiluted filtrate was consistently significantly higher than the diluted filtrates and control without P. ostreatus. Mortality in the undiluted filtrate increased to 95% at 48 h. When 50 g of P. ostreatus-millet culture was mixed with 3 kg autoclaved pot soil, a GI of 0.95 was observed, dropping to 0.70 when the inoculum was doubled to 100 g. For the treatment without P. ostreatus, a high GI of 2.4 was scored. A significant difference in eggplant root growth and nematode population at (p = 0.02) was found across the treatments. The findings from this study for both in-vitro and pot assay suggest that P. ostreatus and its substrate are potential biological control agents for plant parasitic nematodes in eggplants.
1 Introduction
Eggplant (Solanum melongena L.) is an important vegetable crop of the Solanaceae family (Taher et al., 2017). Eggplants are rich in calcium, magnesium, iron, amino acids, vitamins, and antioxidants that are essential for human health (Sharma and Kaushik, 2021). Eggplant ranks as the fifth most economically important solanaceous crop after potato (Solanum tuberosum), tomatoes (Solanum lycopersicum), pepper (Capsicum annuum), and tobacco (Nicotiana tabacum) (Taher et al., 2017). In 2021, the global eggplant trade was estimated at over USD 10 billion with a production capacity of about 56 million tons, a 32% increase from 2010 (FAOSTAT, 2022).
The production of eggplant is greatly constrained by root-knot nematodes (RKN, Meloidogyne spp.). RKN are notorious, sedentary endoparasites that can cause up to 60% yield loss (Khan et al., 2021). Infection by RKN starts with root penetration of second-stage juveniles (J2) after hatching in soil from eggs laid by the females and stored in egg masses on the infected roots. During root penetration, the second stage juveniles (J2) which are the damaging stage of Meloidogyne spp pierces through the plant root tissues using their protrusible stylet. The penetration points created by these nematodes also act as entry avenues for other secondary pathogens which affects the plant quality and so reduction in yield. Once inside the root system, the J2 establishes a feeding site (galls) and starts feeding. The established galls hinders water and nutrient uptake by the plant resulting in yield reduction (Coyne et al., 2018).
Generally, several management options have been designed to address the RKN challenge. The use of Meloidogyne spp.-resistant cultivars could provide an effective strategy to reduce the damaging impact of RKN. However, a few resistant cultivars are commercially available, and resistance may be overcome by emerging RKN spp (Xiang et al., 2018; Hajihassani et al., 2019). Grafting onto RKN resistant rootstock such as Solanum torvum and S. palinacanthum, is one of the most sustainable strategies for managing RKN (Goodell, 2010; Gisbert et al., 2011; Murata et al., 2022). Nevertheless, this practice is still restricted to some high value production systems in Europe and Asia due to its prohibitive costs (Goodell, 2010). Other cultural control practices such as intercropping or rotating with non-host crops, fallowing, weed control, soil solarization, application of organic amendments, destruction of infested crop roots after harvesting and use of clean plantlets can reduce nematode populations (Goodell, 2010; Noling, 2014). While chemical control is the most common short-term management strategy against RKN (Coyne et al., 2018), it poses environmental and human health concerns. Thus, the development of environmentally sound and sustainable alternative control measures for RKN remains a priority. The use of biological control agents (BCA) in the management of plant pests and diseases offer an environmentally sound alternative due to their safety to humans and non-target organisms (Lacey et al., 2001; Lopez-Llorca et al., 2008). Several BCA against plant parasitic nematodes including nematophagous fungi and bacteria, plant growth promoting Rhizobacteria, Arbuscular Mycorrhizal Fungi and predatory nematodes have been reported (Abd-Elgawad, 2016; Atif et al., 2023). At present effective and commercially available BCA for successful control of nematodes under farmers conditions are lacking. In this study we explore the potential as BCA of the edible mushroom species Pleurotus ostreatus and its wastes in the management of RKN in eggplants.
Some edible mushrooms including Pleurotus ostreatus (oyster mushroom) that belongs to the genus Pleurotus, family Pleurotacea, order Agaricale, and class Basidiomycete have been reported to suppress plant parasitic nematodes in diverse crops such as maize (Zea mays), lettuce (Lactuca sativa), tomatoes, and soybean (Glycine max) (Okorie et al., 2011; Wille et al., 2019). The mode of action of these mushrooms include predation, direct penetration of the nematode by the fungal hyphae as well as production of toxins (Barron and Thorn, 1987; Kwok et al., 1992; Zouhar et al., 2013; Hahn et al., 2019). In a study conducted by Genier et al. (2015), P. ostreatus (PO) were found to affect the larvae of Panagrellus sp., a free-living nematode species by directly capturing and entangling on them using their fungal hyphae and having nematicidal effects through their proteases. In vitro studies conducted by Heydari et al. (2006) showed that PO and other Pleurotus spp. produced tiny droplets that were toxic and aided colonization of juveniles of Meloidogyne javanica within 24 to 48 hours. The authors also observed the filtrates of P. ostreatus to have nematicidal activity against the nematode. In another in vitro study by Kanaujiya et al. (2022), PO was found to paralyze and kill different species of plant parasitic nematodes with M. incognita the most affected. Thus, PO could also be nematicidal to RKN in eggplants. Other than being nematicidal, through their effects on decomposition of organic wastes, Pleurotus spp. may also promote plant growth (Hahn et al., 2019; Ocimati et al., 2021; Youssef and El-Nagdi, 2021). Mushroom substrates could thus potentially be deployed for the control of nematodes in a wide range of crops.
2 Materials and methods
2.1 Fungal strain, nematodes, and chemicals
A pure culture of P. ostreatus was obtained from the Mushroom Incubation Center, National Agricultural Research Laboratories (NARL)-Kawanda. The fungus was maintained by repeatedly sub-culturing every 12 days on half-strength solid media of potato dextrose agar (PDA) at 28°C. Juveniles of Meloidogyne spp. were extracted from a highly infested red cabbage (Brassica oleracea) plant using a modified Baermann funnel method (Coyne and Ross, 2014) and multiplied on a susceptible tomato variety grown in pots containing autoclaved soils in the screen house for subsequent studies. All chemicals, unless otherwise stated, were purchased from Sigma-Aldrich (Steinheim, Germany).
2.2 In vitro assessment of P. ostreatus effects on survival of Meloidogyne spp. juveniles
In the laboratory, the effect of P. ostreatus on the survival of Meloidogyne spp. juveniles were assessed using; i) a suspension of P. ostreatus mycelia in water, and ii) a filtrate of the potato dextrose broth (PDB) - P. ostreatus culture. The P. ostreatus suspension in water aimed at determining the effect of both active predation by mycelia and P. ostreatus metabolites whereas, the filtered PDB – P. ostreatus culture aimed at removing all actively growing mycelia and spores to only assess the potential effect of secondary metabolites.
2.2.1 Effect of Pleurotus ostreatus suspension in water on survival of Meloidogyne spp. juveniles
To prepare the P. ostreatus-water suspension, an actively growing 7-day old culture of P. ostreatus mycelia covering the entire surface of a 90 mm diameter Petri plate containing PDA was gently scraped off using a sterile scalpel blade and transferred to a sterile mortar. The mycelia were gently and thoroughly ground with a pestle until a smooth paste was formed. 20 mL of distilled water was then added to the smooth paste, to form a suspension of mycelia. 2 mL of the mycelia suspension was separately pipetted into four 30 mm diameter Petri dishes and each Petri dish inoculated with 20 Meloidogyne spp. juveniles (J2), the infective and damaging stage. Each Petri dish acted as a replicate. Petri30 mm Petri dishes in which 2 mL of sterile water was inoculated with J2 served as controls. The experiment was repeated four times.
The mortality of the J2 in the P. ostreatus hyphae suspension and distilled water was determined at 3, 6, 12, 24, 48, and 72 h after treatment application. Mortality of the J2 was confirmed using the method described by Thoden et al. (2009). The J2 nematodes, which appeared immobile and static at each time interval, were washed 3 times with distilled water, transferred into fresh water and re-observed after 24 h at x40 objective magnification under the Primostar microscope (Zeiss microscopy GmbH, Germany). A nematode was only confirmed dead if it did not recover after the treatment with distilled water. Percentage mortality rate was determined as described by Youssef and El-Nagdi (2021) below:
2.2.2 Effect of Pleurotus ostreatus filtrate on survival of Meloidogyne spp. juveniles
Pleurotus ostreatus filtrate was prepared by culturing P. ostreatus in PDB media contained in sterile 500 mL conical flasks. To achieve this, 250 mL of sterile broth medium was added into 500 mL conical flasks and inoculated with a 1 cm3 block of P. ostreatus mycelia cut from 7-day old pure culture of P. ostreatus actively growing on a PDA Petri plate. The inoculated culture was sealed and incubated at room temperature on a rotary shaker at 121 rpm for 14 days.
The broth was then filtered using a sterile cheese cloth to get rid of all the fungal mycelial fragments and remain with media containing only the metabolites produced by P. ostreatus during growth. The filtrate was further filtered using 0.23 µm diameter micro filters to ensure its sterility and removal of fungi fragments and spores. This filtrate was taken as the original concentration (i.e., 100 of the undiluted) and subsequently serially diluted up to the fourth dilution (10-4). Three treatments of P. ostreatus filtrate were prepared as: i) original concentration (100), ii) second dilution (10-2), and iii) fourth dilution (10-4). Then, 2 mL of each of the three dilutions were pipetted separately to four 30 mm diameter Petri dishes. A suspension of 20 Meloidogyne juveniles was added to each Petri dish. Petri plates to which 2 mL of PDB only and 20 juveniles were added served as controls. The activity levels of the J2 were observed and the number of dead and live J2 in the P. ostreatus filtrate and control treatment (distilled water) recorded at 3, 6, 12, 24, 48, and 72 h after treatment application. Mortality of the J2 was determined after being treated as described in the section above.
2.3 Screen house assessment of Pleurotus ostreatus effects on survival of Meloidogyne spp. juveniles
2.3.1 Raising eggplants
Seeds of Solanum melongena (eggplant, var. ‘Purple long’) were purchased from Simlaw Seeds Company Limited, Kampala, Uganda. The seeds were raised in a seedling pallet for one month, where they were watered daily in the morning and the evening. The seedlings were then subjected to different treatments as described in the sections below.
2.3.2 Preparation of Pleurotus ostreatus inoculum
To prepare the P. ostreatus inoculum, millet grains were washed under clean running tap water to remove dirt and dust. The millet grains were subsequently rinsed with distilled water and soaked overnight to ensure that they are soft enough for easy colonization by the fungus. The millet grains were then transferred to clear autoclaving bags in quantities of 500 g and autoclaved for 90 min at a temperature of 121 °C and pressure of 15 psi. The millet grains were then allowed to cool under a thoroughly disinfected and running laminar flow hood. Actively growing 7-day old P. ostreatus mycelia grown on PDA culture plate was scraped off and inoculated onto the millet grains in transparent autoclavable bags. A total of two culture plates were used per 500 g of millet grain. The millet grains were thoroughly mixed to ensure uniform distribution of the fungus mycelia, sealed and incubated at 28 °C for 14 days. The culture was stirred after every 3 days, to ensure uniform distribution of the growing fungus.
2.3.3 Soil preparation
The soil substrate for the screen house trial was composed of a mixture of loamy soil, poultry manure and sand, in a ratio of 3:3:1. The sterile soils were thoroughly mixed to ensure a uniform mixture and transferred into 3 kg plastic pots that were subjected to treatment as described in the following section. The 3 kg pot is large enough to allow adequate development and establishment of egg plant roots over the study period.
2.3.4 Experimental design
A pot experiment with a complete randomized design (CRD) was set up with five treatments. These included eggplants planted in soil; i) Inoculated with 50 g of millet grain colonized by P. ostreatus and nematodes, ii) Inoculated with 100 g of millet grain colonized by P. ostreatus and nematodes, iii) Inoculated with 50 g of millet grain colonized by P. ostreatus and no nematodes, iv) Without P. ostreatus but with nematodes (positive control), and vi) Without P. ostreatus and no nematodes (negative control-untreated). Each treatment had four replicates of 12 plants each. For treatments with P. ostreatus, the P. ostreatus colonized millet grains were mixed thoroughly in the 3 kg pots of soil at the time of transplanting (at one month) and given two weeks to establish. For treatments with Meloidogyne juveniles, a suspension containing a total of 720 juveniles were inoculated around each plant by drenching through three holes made carefully to minimize interference with the roots. Each drenched hole was thereafter covered with soil. The 720 juveniles used in the experiment falls within the range reported in the literature (Ayeni et al., 2022; Frimpong et al., 2022). Quantification of nematodes for inoculation was performed as described by Coyne and Ross (2014). The experiment was repeated two times, and each terminated after two months.
2.4 Data collection
At termination vertical root length (cm), and root weight (g) were recorded using a measuring tape and precision weighing scale. The nematode growth parameters collected at termination included number of galls per plant, population of plant parasitic nematodes per 5 g of roots, population of plant parasitic and non-parasitic nematodes per 100 g of soil, and the galling index. Galling index was scored using a scale of 0 to 5 as described by Khun-In et al. (2015), where, 0 = no galls; 1 = 1 to 5 galls; 2 = 6 to 20 small galls; 3 = more than 20 galls homogeneously distributed in the root system; 4 = reduced and deformed root system with some large galls; and 5 = completely deformed root system with few, but large galls. To determine the population of plant parasitic nematodes per 5g of roots, tomato roots were sliced into small pieces of between 1 to 3 cm and mixed well on a piece of aluminum foil. Five (5) grams of roots were weighed using a precision laboratory weighing scale and nematodes extracted using a modified Bermann method. For extraction of nematodes from the soil, 1500 g of soil was collected and mixed well in the laboratory before weighing off 100 g for use in nematode extraction. Nematodes were extracted using the same method as for the roots above.
2.5 Data analysis and visualization
Data were analyzed and visualized using R statistical package, v.4.0.2 (R Development Core Team, 2024). Prior to statistical analysis, the data were inspected to ensure homoscedasticity and normality (Kozak and Piepho, 2018). Comparisons were made between treatments and the control. Statistical significance was declared at p ≤ 0.05. Significant differences among treatments and the control were tested using analysis of variance (ANOVA), and subsequent post hoc analysis using Tukey’s Honest Significant Difference (Tukey HSD) test at p value of 0.05. Where the ANOVA assumption was not fulfilled, Kruskal -Wallis a non-parametric test was used and means compared using the Wilcoxon non parametric test at p ≤ 0.05. All data are expressed as mean ± standard error of the mean.
3 Results
3.1 In-vitro effect of Pleurotus ostreatus on juveniles of Meloidogyne species
3.1.1 Effect of Pleurotus ostreatus-water suspension
The P. ostreatus hyphae suspension started to turn turbid after the first 6 h, with the highest turbidity observed at 72 h. Upon introduction of the nematodes into the hyphae suspension, the nematode movement was normal in the first 3 to 6 h of exposure. The nematodes were seen moving freely within the suspension avoiding contact with the fungal mycelia. However, with increased time of exposure, both mobility and activity reduced, with no visible movement at 72 h, with exception of a few nematodes after careful observation at the tail region. Nematodes in contact with the fungal mycelia were immobilized and colonized. In some cases, the fungal hyphae were seen to colonize and grow through the nematode tissue.
There was a significant difference (p value= 0.02) in mortality rate of nematodes across the different time of exposure. Mortality of Meloidogyne juveniles was observed in P. ostreatus suspensions from as early as 3 h, with mortality increasing with increase in time of exposure. At 3 h a mortality of 3% was observed, increasing to 95% at 72 h. (Figure 1A). No nematode mortality was registered in the control (distilled water) at all exposure times.
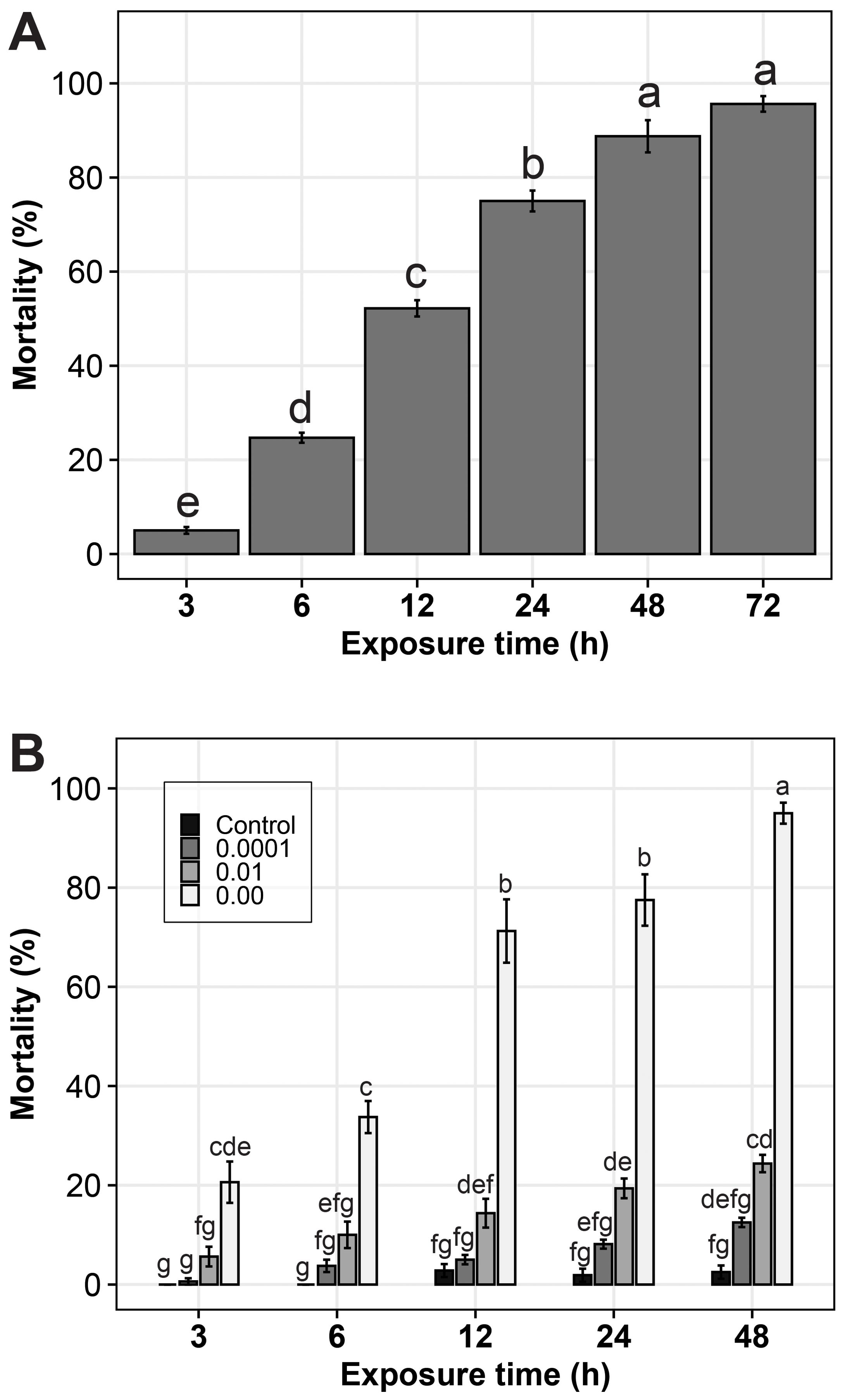
Figure 1. Mortality (%) of Meloidogyne spp. juvenile in; (A) Pleurotus ostreatus- water suspension and (B) different concentrations of a filtrate of P. ostreatus culture in PDB, at different exposure times. The error bars are standard errors. The treatments or treatment combinations with the same letters (‘a’ to ‘g’) above the error bars are not significantly different at 5% Tukey’s Honest Significant Difference.
3.1.2 Effect of Pleurotus ostreatus filtrate
The different concentrations of P. ostreatus filtrate were observed to cause mortality in the Meloidogyne spp. juveniles. Nematode mortality rates were significantly different (p < 0.001) across the different P. ostreatus filtrate concentrations. The original concentration of P. ostreatus filtrate showed very high efficiency in causing nematode mortality at all exposure time intervals. As soon as the nematodes were introduced to the original P. ostreatus filtrate concentration, they were seen to move rapidly. After the vigorous movement, especially by the end of 12 h, most of them were immobile and appeared straight in shape. For those nematodes which were mobile, mobility was observed after gently stroking the nematode tail or head region with a picking needle. At the end of the 24 h, more than 70% of the nematodes remained straight and immobile.
For the 10-2 and 10-4 concentrations, the rate of mortality was lower, and it increased slowly with prolonged exposure time. At the end of 48 h, most of the juvenile nematodes were still moving, but with lower mobility compared to the PDB control treatment, an indication that the dilution reduced the effectiveness of the filtrate activity (Figure 1B).
3.2 Effect of Pleurotus ostreatus on nematode damage in potted eggplants
Pleurotus ostreatus reduced the population of Meloidogyne spp. and boosted the growth of the egg plants at different concentrations. The population of nematodes varied significantly (p = 0.02) across the treatments. The positive control (soil inoculated with nematodes only) had on average over 250 nematodes per 5 g of root tissue compared to less than 50 nematodes per 5 g of root tissue and no nematodes per 5 g of root tissue in soils inoculated with the 50 g and 100 g of millet substrate colonized by P. ostreatus inoculum, respectively (Figure 2A). In the soils, the positive control had on average over six Meloidogyne spp. nematodes per 100 g of soil, compared to about 0.2 and none in soils treated with 50 g and 100 g of millet substrate colonized by P. ostreatus inoculum, respectively (Figure 2B). The application of P. ostreatus was observed to enhance the population of the non-parasitic nematodes, in the soil relative to the positive and negative controls (Figure 2C).
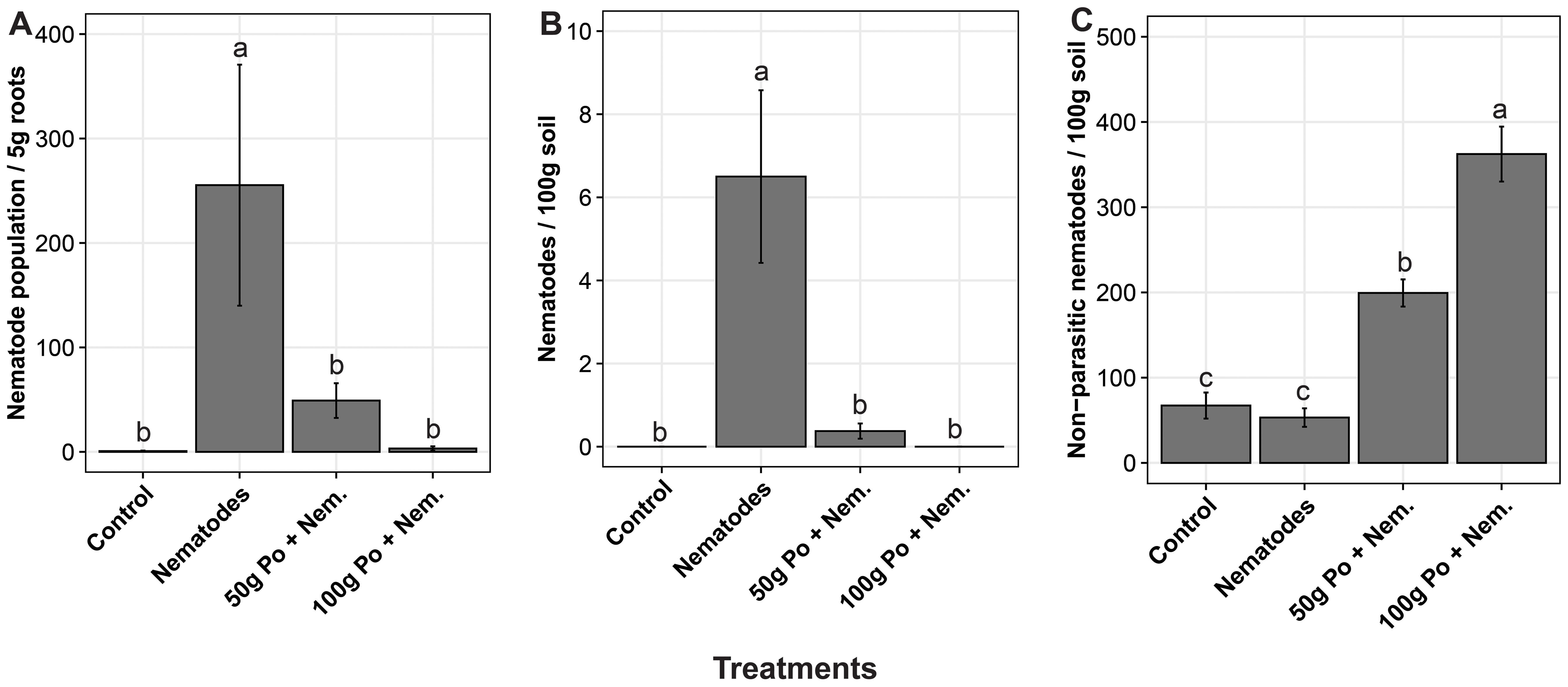
Figure 2. The effect of different concentrations of P. ostreatus inoculum on the population of; (A) the plant parasitic nematode, Meloidogyne spp., in 5g of eggplant root tissues, (B) Meloidogyne spp. in 100g of soil sample, and (C) non-parasitic nematodes in 100g the soil sample. The error bars denote the standard errors. The treatments with the same letters (‘a’, ‘b’ and ‘c’) above the error bars are not significantly different at 5% Tukey’s Honest Significant Difference.
The positive control (i.e., nematodes applied without P. ostreatus) had a high number of galls (mean galling index (GI) of 2.4) compared with a minimal number of galls (mean GI < 1) in the 50 g and 100g P. ostreatus-millet substrate treatment (Figures 3A, B). No galls (GI = 0) were observed in the negative control (no P. ostreatus and no nematodes). The plants inoculated with nematodes only had a significantly (p = 0.02) lower root biomass and root length (Figures 3C, D). Root length in the negative control was comparable to that in the P. ostreatus treated plots whereas the P. ostreatus treated plants had a higher root weight.
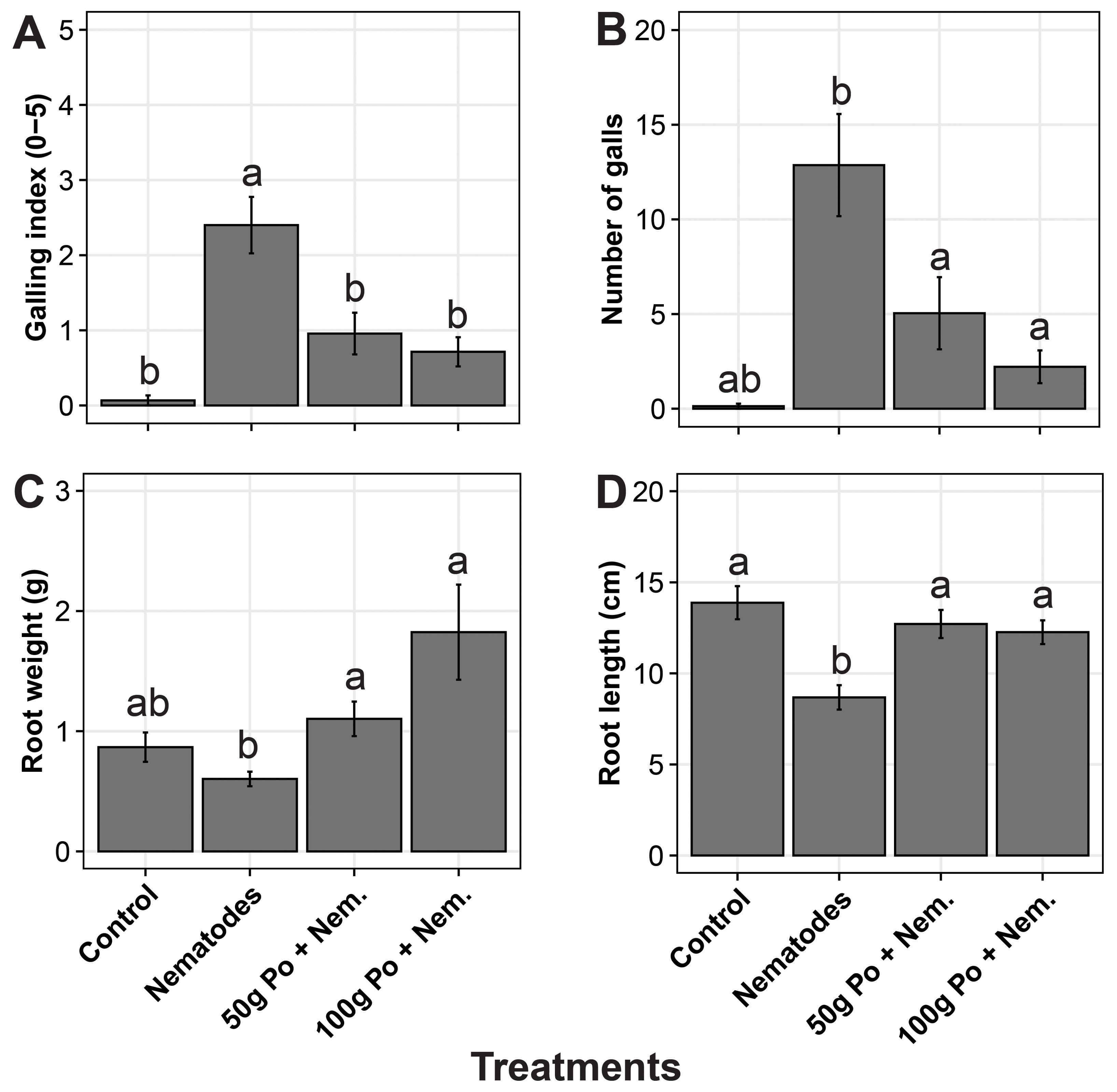
Figure 3. (A) Galling index, (B) number of galls, (C) root fresh weight, and (D) root length of eggplants subjected to different treatments. The treatments included i) a negative control to which no Pleurotus ostreatus substrate and no nematodes were added, ii) a positive control to which nematodes were added without P. ostreatus, ii) a treatment to which P. ostreatus was cultured on 50g of millet substrate and a treatment to which P. ostreatus cultured on 100g of was added. The error bars denote the standard errors. The treatments with the same letters (‘a’ and ‘b’) above the error bars are not significantly different at 5% Tukey’s Honest Significant Difference.
4 Discussion
P. ostreatus was observed to exhibit nematicidal properties and suppress Meloidogyne spp. in vitro and in potted eggplants within a screen house. In-vitro suppression of Meloidogyne juveniles (J2) by pure cultures of P. ostreatus hyphae and filtrate can be attributed to both active predation on the nematodes and immobilization by the secondary metabolites produced by P. ostreatus, a Basidiomycetes. Basidiomycetes have been reported to be nematophagus with the potential to produce toxins as a unique identifying characteristic. Satou et al. (2008) identified toxins such as tridecanoic, tetradecanoic, linolelaidei, and trans 2-decenodioc acid, a peroxide of linoleic acid in these fungi. Amongst all these toxins, trans 2 decanoic acid was observed as the most active toxin in exhibiting nematicidal properties (Kwok et al., 1992). This compound is produced as tiny droplets at the tip of the fungal hyphae and once the nematode gets in contact with it, the nematode is rendered immobile or motionless (Heydari et al., 2006; Castañeda-Ramírez et al., 2020). In the current study, the juvenile Meloidogyne spp. became immobile and died after a few hours of interacting with both the fungal culture and the filtrate. The death could have thus been caused by the penetration of nematicidal compounds such as 2-decanoic acid into the nematode tissues, affecting the osmotic pressure of the inner membrane, resulting in necrosis of the nervous system (Castañeda-Ramírez et al., 2020). In a study on C. elegans, it was found that the head of the dead nematodes appeared to be shrunken and the esophagus displaced (Lee et al., 2020). Once the nematode was immobilized, fungal hyphae and mycelia were seen surrounding it and later penetrating the nematode tissue through the body orifices, an observation similar to that in Palizi et al. (2009). Genier et al. (2015) reported that the penetration and colonization of nematode bodies by P. ostreatus is facilitated by proteases. Proteases are secondary metabolites released by P. ostreatus during its growth. The proteases facilitate the disintegration of the proteaceous nematode protection barrier allowing the penetration of the fungal hyphae (Inácio et al., 2015).
Pleurotus ostreatus also caused a reduction in the nematode population, root galling, and improved eggplant root growth in potted plants, an observation similar to that of Youssef and El-Nagdi (2021). The reduced nematode population can be attributed to the effect of the toxic metabolites and direct predation on the nematodes and eggs as described above and as observed in the in-vitro studies Genier et al. (2015) and Hussain et al. (2020) reported the hydrolytic enzymes (largely the proteases) released by P. ostreatus to have the potential to hydrolyze the nematode eggs and juveniles, thus altering their reproduction and reducing their populations. The Trans 2-decenoic acid, a secondary metabolite largely produced by P. ostreatus, also directly immobilizes the nematodes causing their death (Castañeda-Ramírez et al., 2020), thus also explaining the reduction in nematode populations. The reduction in nematode populations ultimately translated into reduced galling and improved eggplant growth.
Hyphae of P. ostreatus have been reported to colonize the roots of okra plants, thereby hindering access, attachment, and penetration of root tips by Meloidogyne spp. juveniles (Bordallo et al., 2002). This effect on roots could also partially explain the observed reduction in the number of galls and galling index in treatments having P. ostreatus compared to the untreated controls. This mechanism of action was also reported in a number of nematophagus fungi, mainly Trichoderma harzianum (Kleifeld and Chet, 1992; Yedidia et al., 1999).
In addition to the protection against the nematodes, the improved eggplant root growth in shown by a higher root weight in treatments having P. ostreatus could have been because of the saprophytic nature of the fungi, P. ostreatus, leading to better decomposition of organic matter including the millet substrate and an increased nutrient availability. The improved nutrition also boosts plant growth and tolerance to RKN infections.
A large population of free-living nematodes were also observed in the soil of treatments having P. ostreatus compared to those without. This could have been due to the role of free living nematodes in decomposition of organic matter (Yadav et al., 2018). This could further explain the improved root growth in eggplant treated with P. ostreatus compared to the controls without P. ostreatus inoculum.
The above findings show that P. ostreatus has got a very high potential in the management of RKN in eggplants. P. ostreatus cultivation and consumption is currently being embraced widely. Its promotion for nematode management can thus be easily adopted and of great benefit in addressing nematode constraints in agricultural production systems. The fungus would be a great alternative since it’s environmentally safe with no harmful effects in the soil and on beneficial soil microbes. With the advances in technology, the active nematicidal compounds produced by the fungus could be isolated and mass produced synthetically as nematode bio-control agents making them readily available to the farmers.
The current study relied on colonized millet substrates. The potential of spent mushroom substrates that are more readily available and cheaper for managing the nematodes in eggplants needs to be explored. The use of P. ostreatus as a bio-control approach in the management of RKN should also be explored under different field conditions to evaluate its performance under natural environments. While doing the field evaluations, cultural nematode management strategies should also be employed alongside the P. ostreatus to assess how the integration of the different strategies goes a long way in addressing the production challenges related to RKN.
Data availability statement
The original contributions presented in the study are included in the article/supplementary material. Further inquiries can be directed to the corresponding author.
Ethics statement
The manuscript presents research on animals that do not require ethical approval for their study.
Author contributions
BN: Conceptualization, Data curation, Formal analysis, Investigation, Methodology, Writing – original draft, Writing – review & editing. WO: Conceptualization, Funding acquisition, Project administration, Resources, Supervision, Writing – review & editing, Validation. AT: Conceptualization, Methodology, Writing – review & editing. GB: Funding acquisition, Writing – review & editing. AA: Writing – review & editing. FO: Conceptualization, Methodology, Project administration, Resources, Supervision, Writing – review & editing, Validation.
Funding
The author(s) declare financial support was received for the research, authorship, and/or publication of this article. The authors are grateful to the CGIAR Initiative on Plant Health, the CGIAR Trust Fund: www.cgiar.org/funders/ for financing some of the staff time and the publication cost of the study. The authors also appreciate Muni University for financing staff time, giving BN study leave to attend Nematology training and NEMEDUSA project (EU: 618814-EPP-1-2020-1-BE-EPPKA2-CBHE-JP) for funding training of BN in Nematology and supporting this research.
Acknowledgments
Authors acknowledge the contributions of Erasmus+ Capacity Building in Higher Education: Nematology Education in Sub-Saharan Africa (NEMEDUSSA) Project. -and Ghent University through capacity building. We are grateful to Ms. Inge Dehennin, Dora Scott and Prof. Wim Bert of Ghent University, Faculty of Sciences, and Nematology Research Unit for training BN in aspects of Nematology which provided her with necessary knowledge and skills for completing this research.
Conflict of interest
The authors declare that the research was conducted in the absence of any commercial or financial relationships, therefore there is no conflict of interest.
Publisher’s note
All claims expressed in this article are solely those of the authors and do not necessarily represent those of their affiliated organizations, or those of the publisher, the editors and the reviewers. Any product that may be evaluated in this article, or claim that may be made by its manufacturer, is not guaranteed or endorsed by the publisher.
References
Abd-Elgawad M. M. M. (2016). Biological control agents of plant-parasitic nematodes. Egypt. J. Biol. Pest Control 26, 423–429.
Atif A. M., Elzamik F. I., Mohamed G. M., Al-Quwaie D. A., Ashkan M F., Alqahtani F. S., et al. (2023). Biological control of the root-knot nematode (Meloidogyne incognita) on eggplants with various chitinase-producing Streptomyces strains. Eur. J. Plant Pathol. 167, 371–394. doi: 10.1007/s10658-023-02718-8
Ayeni B. P., Claudius-Cole A. O., Abberton M., Coyne D. (2022). Host status of Bambara groundnut accessions to Meloidogyne incognita using conventional screenhouse and novel seedling pouch methods. Nematol 24, 543–558. doi: 10.1163/15685411-bja10150
Barron G. L., Thorn R. G. (1987). Destruction of nematodes by species of Pleurotus. Can. J. Bot. 65, 774–778. doi: 10.1139/b87-103
Bordallo J. J., Lopez-Llorca L. V., Jansson H. B., Salinas J., Persmark L., Asensio L. (2002). Colonization of plant roots by egg-parasitic and nematode-trapping fungi. New Phyto. 154, 491–499. doi: 10.1046/j.1469-8137.2002.00399.x
Castañeda-Ramírez G. S., Torres-Acosta J. F. D. J., Sánchez J. E., Mendoza-De-Gives P., González-Cortázar M., Zamilpa A., et al. (2020). The possible biotechnological use of edible mushroom bioproducts for controlling plant and animal parasitic nematodes. Biomed. Res. Int. 1-12. doi: 10.1155/2020/6078917
Coyne D., Ross J. L. (2014). Protocol for nematode resistance screening: root knot nematodes. Meloidogyne, 27. Available at: https://hdl.handle.net/10568/87912.
Coyne D. L., Cortada L., Dalzell J. J., Claudius-Cole A. O., Haukeland S., Luambano N., et al. (2018). Plant-parasitic nematodes and food security in Sub-Saharan Africa. Annu. Rev. Phytopathol. 56, 381–403. doi: 10.1146/annurev-phyto-080417-045833
FAOSTAT. (2022). World Food and Agriculture – Statistical Yearbook 2022 (Rome:FAO Statistics). doi: 10.4060/cc2211en7544
Frimpong M., Osei K., Prempeh R. N. A. (2022). Yield potential and root-knot nematode (Meloidogyne incognita) resistance of six tomato (Solanum lycopersicum L.) genotypes. Int. J. Phyto. Res. 2, 30–33.
Genier H. L. A., de Freitas Soares F. E., de Queiroz J. H., de Souza Gouveia A., Araújo J. V., Braga F. R., et al. (2015). Activity of the fungus Pleurotus ostreatus and of its proteases on Panagrellus sp. larvae. Afri. J. Biotechnol. 14, 1496–1503. doi: 10.5897/AJB2015.14447
Gisbert C., Prohens J., Raigón M. D., Stommel J. R., Nuez F. (2011). Eggplant relatives as sources of variation for developing new rootstocks: Effects of grafting on eggplant yield and fruit apparent quality and composition. Sci. Hortic. 128, 14–22. doi: 10.1016/j.scienta.2010.12.007
Goodell P. B. (2010). University of California IPM Pest Management Guidelines: Eggplant (UC ANR Publication). Available online at: https://ipm.ucanr.edu/agriculture/eggplant/nematodes/gsc.tab=0 (Accessed Sept. 03, 2024).
Hahn M. H., De Mio L. L. M., Kuhn O. J., Duarte H. D. S. S. (2019). Nematophagous mushrooms can be an alternative to control Meloidogyne javanica. Biol. Control 138, 104024. doi: 10.1016/j.biocontrol.2019.104024
Hajihassani A., Davis R. F., Timber P. (2019). Evaluation of selected non fumigant nematicides on increasing inoculation densities of Meloidogyne incognita on Cucumber. Plant Dis. 103, 3161–3165. doi: 10.1094/PDIS-04-19-0836-RE
Heydari R., Pourjam E., Goltapeh E. M. (2006). Antagonistic effect of some species of Pleurotus on the root-knot nematode, Meloidogyne javanica in vitro. Plant Pathol. J. 5, 173–177. doi: 10.3923/ppj.2006.173.177
Hussain M., Manasova M., Zouhar M., Ryšánek P. (2020). Comparative virulence assessment of different nematophagous fungi and chemicals against northern root-knot nematodes, Meloidogyne hapla, on carrots. Pakistan J. Zool. 52, 199. doi: 10.17582/journal.pjz/2020.52.1.199.206
Inácio F. D., Ferreira R. O., Araujo C. A. V. D., Brugnari T., Castoldi R., Peralta R. M., et al. (2015). Proteases of wood rot fungi with emphasis on the genus Pleurotus. BioMed. Res. Int. 2015, 290161. doi: 10.1155/2015/290161
Kanaujiya D. K., Gupta S. K., Kumar G., Pathak R., Kumar Y. (2022). Ability of Pleurotus ostreatus in vitro to kill different plant parasitic nematodes. Pharma Innov. J. 11, 911–913.
Khan A., Aiman S. I., Baber Y., Hassan F., Usman H. M., Sohail M. A., et al. (2021). An overview of root-knot nematodes and their management. J. Ento. Zool. Stud. 9, 35–40.
Khun-In A., Sukhakul S., Chamswarng C., Tangkijchote P., Sasnarukkit A. (2015). Culture filtrate of Pleurotus ostreatus isolate poa3 effect on egg mass hatching and juvenile 2 of Meloidogyne incognita and its potential for biological control. J. Int. Soc Southeast Asian Agric. Sci. 21, 46–54.
Kleifeld O., Chet I. (1992). Trichoderma harzianum-interaction with plants and effect on growth response. Plant Soil 144, 267–272. doi: 10.1007/BF00012884
Kozak M., Piepho H. P. (2018). What’s normal anyway? Residual plots are more telling than significance tests when checking ANOVA assumptions. J. Agron. Crop Sci. 204, 86–98. doi: 10.1111/jac.2018.204.issue-1
Kwok O. C. H., Plattner R., Weisleder D., Wicklow D. T. (1992). A nematicidal toxin from Pleurotus ostreatus NRRL 3526. J. Chem. Ecol. 18, 127–136. doi: 10.1007/BF00993748
Lacey L. A., Frutos R., Kaya H. K., Vail P. (2001). Insect pathogens as biological control agents: Do they have a future? Biol. Control 21, 230–248. doi: 10.1006/bcon.2001.0938
Lee C. H., Chang H. W., Yang C. T., Wali N., Shie J. J., Hsueh Y. P. (2020). Sensory cilia as the Achilles heel of nematodes when attacked by carnivorous mushrooms. Proc. Natl. Acad. Sci. 117, 6014–6022. doi: 10.1073/pnas.1918473117
Lopez-Llorca L. V., Maciá-Vicente J. G., Jansson H. B. (2008). “Mode of action and interactions of nematophagous fungi,” in Integrated management and biocontrol of vegetable and grain crops nematodes (Springer Netherlands, Dordrecht), 51–76. doi: 10.1007/978-1-4020-6063-2_3
Murata G., Uehara T., Lee H. J., Mizutani M., Kadota Y., Shinmura Y., et al. (2022). Solanum palinacanthum Dunal as a potential eggplant rootstock resistant to root-knot nematodes. J. Phytopathol. 170, 185–193. doi: 10.1111/jph.v170.3
Noling J. W. (2014). Nematode management in tomatoes, peppers, and eggplant. Available online at: https://worldveg.tind.io/record/55809/ (Accessed September 03, 2024).
Ocimati W., Were E., Tazuba A. F., Dita M., Zheng S.-J., Blomme G. (2021). Spent Pleurotus ostreatus substrate has potential for managing Fusarium wilt of banana. J. Fungi 7, 946. doi: 10.3390/jof7110946
Okorie C. C., Ononuuj C. C., Okwujiako I. A. (2011). Management of Meloidogyne incognita with Pleurotus ostreatus and P. tuberregium in soybean. Int. J. Agric. Biol. 13, 401–405.
Palizi P., Goltapeh E. M., Pourjam E., Safaie N. (2009). Potential of oyster mushrooms for the biocontrol of sugar beet nematode (Heterodera schachtii). J. Plant Prot. Res. 49, 27–33. doi: 10.2478/v10045-009-0004-6
R Core Team. (2024). R: A language and environment for statistical computing (Vienna, Austria: R Foundation for Statistical Computing). Available at: https://www.R-project.org/.
Satou T., Kaneko K., Li W., Koike K. (2008). The toxin produced by Pleurotus ostreatus reduces the head size of nematodes. Biol. Pharm. Bull. 31, 574–576. doi: 10.1248/bpb.31.574
Sharma M., Kaushik P. (2021). Biochemical composition of eggplant fruits: A Review. Appl. Sci. 11, 7078. doi: 10.3390/app11157078
Taher D., Solberg S. Ø., Prohens J., Chou Y.-Y., Rakha M., Wu T.-H. (2017). World Vegetable Center eggplant collection: origin, composition, seed dissemination and utilization in breeding. Front. Plant Sci. 8. doi: 10.3389/fpls.2017.01484
Thoden T. C., Boppré M., Hallmann J. (2009). Effects of pyrrolizidine alkaloids on the performance of plant-parasitic and free-living nematodes. Pest Manage. Sci. 65, 823–830. doi: 10.1002/ps.1764
Wille C. N., Gomes C. B., Minotto E., Nascimento J. S. (2019). Potential of aqueous extracts of basidiomycetes to control root-knot nematodes on lettuce. Hortic. Bras. 37, 54–59. doi: 10.1590/s0102-053620190108
Xiang N., Lawrence K. S., Donald P. A. (2018). Biological control potential of plant growth-promoting rhizobacteria suppression of Meloidogyne incognita on cotton and Heterodera glycines on soybean: A Review. J. Phytopathol. 166, 449–458. doi: 10.1111/jph.12712
Yadav S., Patil J., Kanwar R. S. (2018). The role of free living nematode population in the organic matter recycling. Int. J. Curr. Microb. Appl. Sci. 7, 2726–2734. doi: 10.20546/ijcmas.2018.706.321
Yedidia I., Benhamou N., Chet I. (1999). Induction of defense responses in cucumber plants (Cucumis sativus L.) by the biocontrol agent Trichoderma harzianum. Appl. Envir. Microb. 65, 1061–1070. doi: 10.1128/aem.65.3.1061-1070.1999
Youssef M. M. A., El-Nagdi W. M. A. (2021). New approach for biocontrolling root-knot nematode, Meloidogyne incognita on Cowpea by commercial fresh Oyster mushroom (Pleurotus ostreatus). Jordan J. Biol. Sci. 14, 173–177. doi: 10.54319/jjbs/140122
Keywords: biological, Meloidogyne spp., Pleurotus ostreatus, eggplants, mushroom substrate
Citation: Nyangwire B, Ocimati W, Tazuba AF, Blomme G, Alumai A and Onyilo F (2024) Pleurotus ostreatus is a potential biological control agent of root-knot nematodes in eggplant (Solanum melongena). Front. Agron. 6:1464111. doi: 10.3389/fagro.2024.1464111
Received: 13 July 2024; Accepted: 04 October 2024;
Published: 25 October 2024.
Edited by:
Omer Frenkel, Agricultural Research Organization (ARO), IsraelReviewed by:
Hipolito Cortez-Madrigal, National Polytechnic Institute (IPN), MexicoMehtap Alkan, Ankara University, Türkiye
Copyright © 2024 Nyangwire, Ocimati, Tazuba, Blomme, Alumai and Onyilo. This is an open-access article distributed under the terms of the Creative Commons Attribution License (CC BY). The use, distribution or reproduction in other forums is permitted, provided the original author(s) and the copyright owner(s) are credited and that the original publication in this journal is cited, in accordance with accepted academic practice. No use, distribution or reproduction is permitted which does not comply with these terms.
*Correspondence: Francis Onyilo, ZnJhbmNpcy5vbnlpbG9AZ21haWwuY29t