- 1Tobacco Research Institute of Hubei Province, Wuhan, China
- 2Microelement Research Centre, Huazhong Agricultural University, Wuhan, China
- 3Hubei Tobacco Company Shiyan City Company, Shiyan, China
- 4Honghong, Wengfu (Group) Co., Ltd., Guiyang, China
Introduction: This study investigated the combined effects of molybdenum (Mo) and nitrogen (N) fertilization on N metabolism and amino acid content in the leaves of flue-cured tobacco (Nicotiana tabacum L.) during its mature stage through a pot experiment.
Methods: Different application levels of Mo (0, 0.15, 0.30 mg/kg soil) and N (0.06, 0.24, 0.42 g/kg soil) were set to observe and analyze changes in leaf quality, N, and amino acid content in the tobacco plants.
Results: The results revealed that the N fertilizer application level exhibited a primary effect on regulating the total nitrogen, nitrate nitrogen, soluble protein, and amino acid nitrogen concentrations within tobacco leaves, while the effectiveness of Mo fertilization was influenced by the level of N applied. Specifically, under the conditions of 0.24 g/kg soil N and 0.30 mg/kg soil Mo application, the N content, N accumulation, and dry matter mass of the tobacco plants increased significantly by 110%, 204%, and 48%, respectively. Concurrently, nitrate reductase activity increased by 107-fold, and the nitrate nitrogen content was relatively low, contributing to enhanced tobacco yield and safety. Moreover, this treatment led to a notable (170%) increase in free amino acid nitrogen content, with minimal impact on total amino acids and soluble proteins. Notably, it effectively increased the content of free amino acids beneficial to the sensory quality of tobacco (such as histidine, arginine, aspartic acid, isoleucine, and glutamic acid) without reducing the total amino acid content, while simultaneously reducing other amino acids that might affect quality. Therefore, the combined application of 0.30 mg/kg soil Mo and 0.24 g/kg soil N specifically optimized the amino acid composition in tobacco leaves, positively impacting overall quality and market competitiveness.
Discussion: This study provides a theoretical basis for the rational application of Mo fertilizer in Mo-deficient areas to improve the yield and quality of flue-cured tobacco.
1 Introduction
N is an indispensable nutritional element for plant growth and development, actively participates in vital physiological mechanisms, including carbon and N metabolism (Mahboob et al., 2023). Its appropriate application ensures the coordinated progression of plant life, maintaining a balanced carbon- N ratio, and notably augmenting both the yield and quality of crops (Rebouh et al., 2023). In recent years, China has witnessed a consistent rise in N fertilizer usage across its agricultural lands (Zhang et al., 2015; Lu and Tian, 2017; Zuo et al., 2018). However, despite this surge, the efficiency of N utilization stands at a mere 30%, a stark contrast to the efficiency rates observed in North America and Europe, ranging between 52-68% (Grahmann et al., 2014). This discrepancy highlights the inefficiency of N uptake by Chinese crops and underscores the need for alternative strategies to improve N use efficiency. The excessive remnants of N fertilizers in these farmlands have triggered severe soil acidification, consequently diminishing the soil’s efficacy in retaining essential trace elements such as Mo (Yahaya et al., 2023). Reports indicate that approximately 44.50 million hectares of Chinese soil currently suffer from decreased Mo effectiveness (Zou et al., 2008). Therefore, the pressing concern revolves around exploring methodologies aimed at enhancing crop N utilization through the application of trace elements, forming a critical focal point in contemporary agricultural research and practice.
Mo serves as an indispensable micronutrient for plants, acting as a fundamental constituent of nitrate reductase and playing a pivotal role in the assimilation of plant nitrate N, thereby influencing plant N metabolism (Zimmer and Mendel, 1999; Nandety and Missaoui, 2020; Banerjee and Nath, 2022; Bursakov et al., 2023). Previous studies have demonstrated the benefits of Mo fertilization on crop growth and yield. For instance, the application of Mo fertilizer can significantly enhance nitrate reductase activity and N content in crops such as soybeans, corn, and potatoes, leading to improved N utilization efficiency and increased yield (Elrys et al., 2018; Oliveira et al., 2022). Furthermore, Mo profoundly influences crop quality. For instance, its application significantly elevated the concentration of free amino acids, proline, soluble proteins, and total soluble sugars in winter wheat, thereby augmenting wheat yield (Imran et al., 2019). Additionally, the application of Mo fertilizer substantially increases the crude protein and lipid content in soybeans (Latifinia and Eisvand, 2022). However, Chatterjee’s research found that under 170 mg/L N levels, Mo deficiency or the addition of 10 mg/L of Mo significantly reduced nitrate reductase activity, protein content, and other quality indicators in wheat leaves and seeds (Chatterjee and Nautiyal, 2001). This suggested that the interactions between varying levels of Mo and N may have different effects on crop N uptake, conversion, and N metabolism, which require further clarification.
Tobacco, a widely cultivated crop in China, has high demands for both Mo and N. The critical Mo requirement threshold for tobacco plants stands at 0.13 mg/kg, which many soils fail to meet. This deficiency can significantly impact tobacco yield and quality. Therefore, investigating the combined effects of Mo and N fertilization on tobacco growth and quality is crucial for improving N utilization efficiency and enhancing crop quality. This study focuses on the K326 tobacco variety, a widely grown cultivar in China. By systematically investigating the combined effects of Mo and N fertilization on N uptake, conversion, N metabolism, and quality parameters, we aim to gain insights into the optimal fertilization regime for tobacco production in Mo-deficient regions. Understanding the interactions between Mo and N in tobacco plants is crucial for developing scientific fertilization strategies that can enhance N utilization efficiency, improve tobacco yield, and enhance the sensory quality of tobacco leaves.
2 Materials and methods
2.1 Experimental materials and design
In this study, the flue-cured tobacco variety K326, provided by the Jiangxi Tobacco Research Institute in China, was utilized. The soil used for testing was acidic yellow-brown soil collected from Wuhan City, Hubei Province, which was identified as severely deficient in Mo. The specific soil properties are presented in Supplementary Table S1. The experiments were conducted using polyethylene plastic barrels, each containing 12 kg of soil. A pot experiment was designed with three levels of Mo (0, 0.15, and 0.30 mg/kg Mo in soil, henceforth abbreviated as Mo0, Mo0.15, and Mo0.30) and three levels of N (0.06, 0.24, and 0.42 g/kg N in soil; abbreviated as N0.06, N0.24, and N0.42), resulting in a total of nine treatments. Each treatment was replicated four times.
Prior to transplanting the tobacco seedlings, potassium dihydrogen phosphate (P2O5 at 0.24 g/kg) and potassium sulfate (K2O at 0.48 g/kg) were applied as basal fertilizers. Ammonium molybdate and urea were used as sources for Mo and N treatments, respectively. All fertilizer solutions were administered to the soil simultaneously before transplanting. The plants were irrigated with ultrapure water during the growth period. The potting field was equipped with a fiberglass tile rainproof shelter to prevent rainwater from washing the soil, and timely soil loosening, weeding, and pesticide spraying were carried out. Each pot was transplanted with one tobacco seedling, and leaves were harvested from various parts at the mature stage after 90 days of transplantation. The biomass was recorded for the middle leaves (middle 6–14 leaves), as they are more representative of the entire tobacco plant’s foliage. The leaf samples were washed with ultrapure water, treated at 105°C for 30 minutes, dried to a constant weight at 60°C, and finally ground into powder after recording the dry weight.
2.2 Soil and plant nutrient analysis
Soil pH was determined in a 1:2.5 water suspension (distilled water) with a pH meter (Mettler Toledo, China) (Ng et al., 2022). Soil organic matter was analyzed according to the methods of Chen et al. (Chen et al., 2022). Soil alkaline-hydrolyzable nitrogen was determined by the alkaline-hydrolysis diffusion method (1 mol/L NaOH and 2% H3BO3) (Drescher et al., 2020). Soil available phosphorus was extracted with 0.50 mol/L NaHCO3 and determined by spectrophotometer at 700 nm (Alewell et al., 2020) (Metash UV−5200, China).
Initially, the leaves were accurately weighed using a precision scale. Subsequently, they were transferred into a digestion tube, where 5 mL of H2SO4 and an appropriate volume of H2O2 were added. The exact volume of H2O2 was determined based on the specific protocol or the amount of sample being digested. The digestion tube was then heated in a digestion block to a temperature of 180°C for a specified period, typically several hours, to break down the organic matter in the leaves and release the nitrogen for analysis. After digestion, the sample was allowed to cool and was diluted with distilled water to a suitable volume for analysis. The total N content of the digested sample was then analyzed using a flow injection analyzer (FIAstar5000, Sweden), which measured the nitrogen content by detecting the amount of ammonia produced during the digestion process. The N content was calculated based on the measured ammonia concentration and the sample weight, and was expressed in appropriate units such as percentage.
2.3 Determination of nitrate nitrogen content
1 g of fresh tobacco leaves was weighed, added with 10 mL of pure water and boiled for 30 min. The sample was then filtered, and the volume was set to 25 mL. Then, 0.1 mL of filtrate was taken and added with 0.40 mL 5% (m/v) sulfuric acid-salicylic acid, followed by the addition of 9.5 mL 8% (m/v) NaOH, and colorimetric measurement by spectrophotometer at 410 nm after cooling (Salehzadeh et al., 2020).
2.4 Leaves nitrate reductase assay
The determination of nitrate reductase (NR) was carried out as described previously by (Baki et al., 2000). 1 g leaves were ground in a chilled mortar with 4 mL of extraction buffer which contained 100 mM Hepes-KOH (pH 7.5), 3% (w/v) polyethylene pyrrolidone, 1 mM EDTA, and 7mM cysteine. Add 1.2 mL of 0.10 M KNO3 phosphate buffer and 0.40 mL of 2 mg/mL NADH solution, and finally add 6 mM MgCl2 to determine nitrate reductase actual activity.
The mixture was then thoroughly mixed and placed in a 25°C water bath for 30 minutes. Immediately after the incubation period, 1 mL of 1% (w/v) sulfonamide solution was added to terminate the enzymatic reaction, followed by the addition of 1 mL of 0.20% (w/v) α-naphthylamine solution. The mixture was centrifuged, and the nitrite concentration was determined calorimetrically using a spectrophotometer at 540 nm.
2.5 Determination of soluble protein content
Bradford (Bradford, 1976) spectrophotometric method was used to determine the total content of soluble protein in leaves. In brief, 10 µL of the standards (aqueous solution of bovine serum albumin, BSA) or samples were mixed with 200 µL of diluted dye (acidic solution of Coomassie Brilliant Blue G-250). Five minutes later, the absorbance was measured at 595 nm using a spectrophotometer at room temperature.
2.6 Determination of free amino acid nitrogen content
0.50 g fresh sample was weighed, followed by the addition of 5 mL 10% C2H4O2 grinding homogenate, dilution with distilled water to 50 mL, and filtering. About 1 mL of filtrate was taken and put into a 20-mL dry tube, followed by the addition of 1 mL of sterile distilled water, 0.5 mL of ascorbic acid, and 3 mL of ninhydrin hydrate, and then bathed in boiling water for 15 min. When the solution turned to blue-purple, the volume was fixed to 20 mL with 60% C2H6O, and the absorbance value was determined by spectrophotometer at 570 nm after mixing (Mustafa et al., 2007).
2.7 Determination of individual amino acids contents
1 g of dry tobacco leaf sample was taken into a hydrolysis tube, which was then added with 10 mL of 6 mol/L HCl, installed with a hydrolysis tube on the vacuum pump for degassing. The sample was then hydrolyzed with a 110°C thermostat for 22-24 h, cooled, mixed, and filtered. Then, an appropriate amount of filtrate was taken into a rotary evaporation dish or concentrator, vacuumed at 60°C, evaporated to dryness, added with 3-5 mL of pH 2.2 sodium citrate buffer and centrifuged well. The supernatant was taken to be determined on an amino acid analyzer (Hitachi 835-50, Japan) (Mustafa et al., 2007).
2.8 Determination of individual free amino acids contents
0.50 g of dry tobacco leaf sample was weighed and put in a triangular flask, which was then added with 20 mL of distilled water and boiled on an electric furnace for 15 min. The sample was then transferred to a volumetric flask with a fixed volume of 50 mL after cooling. 1 mL of filtrate was taken and evaporated on a rotary evaporator, and then dissolved with 0.02 mol/L HCl. The aqueous solution was directly determined on an amino acid analyzer (Mustafa et al., 2007).
2.9 Data analysis
All data were first tested for normality to ensure their suitability for statistical analysis. Subsequently, the data were statistically analyzed using SPSS 20.0 (IBM Corp., Armonk, USA) and Excel 2019 (Microsoft Corp., Redmond, USA). The Tukey test was used to determine significant variance at p < 0.05. Additionally, Origin 2021 (OriginLab Corp., Northampton, USA) was used for plotting the results. In the principal component analysis, Y1 represents the score of the principal component, and F represents the regression model coefficient.
3 Results
3.1 The impact of molybdenum and nitrogen co-application on tobacco leaf biomass
At the same Mo level, tobacco leaf biomass exhibited a gradual increase as N application increased. Specifically, compared to the N 0.06 treatment, tobacco leaf biomass significantly increased by 29.8% (N 0.24) and 47.4% (N 0.42) at the Mo 0 level; by 37.5% (N 0.24) and 63.1% (N 0.42) at the Mo 0.15 level; and by 48.3% (N 0.24) and 93.6% (N 0.42) at the Mo 0.3 level, respectively. Conversely, under the same N treatment, the trend of tobacco leaf biomass varied inconsistently with increasing Mo application. Specifically, under the N 0.06 treatment, tobacco leaf biomass exhibited no significant variation. However, under the N 0.24 treatment, Mo application significantly increased tobacco leaf biomass by 12.0% (Mo 0.15) and 12.3% (Mo 0.3), respectively. Similarly, under the N 0.42 treatment, tobacco leaf biomass significantly increased by 17.1% (Mo 0.15) and 29.2% (Mo 0.3), respectively. These findings indicate that effective enhancement of tobacco leaf biomass is only achieved with molybdenum application at moderate and high nitrogen levels.
3.2 Effect of molybdenum and nitrogen combined application on nitrogen content and accumulation in tobacco leaves
At the same Mo level, tobacco leaf N content and accumulation exhibited significant increases with increasing N application (Figure 1). Specifically, compared to the N 0.06 treatment, tobacco leaf N content increased by 1.4-2 times under the N 0.24 and N 0.42 treatments, respectively (Figure 1A). Furthermore, with increasing Mo application, tobacco leaf N content demonstrated a decreasing trend under the N 0.06 treatment, while it remained stable under the N 0.24 and N 0.42 treatments (Figure 1A). Notably, tobacco leaf N accumulation significantly increased with increasing Mo application under the N 0.24 and N 0.42 treatments. Specifically, under the N 0.24 treatment, tobacco leaf N accumulation increased by 25.43% (Mo 0.15) and 26.51% (Mo 0.3), respectively, while under the N 0.42 treatment, it increased significantly by 15.97% (Mo 0.15) and 36% (Mo 0.3) (Figure 1B). These results indicated that Mo application may decrease tobacco leaf N content under low N conditions but significantly enhance leaf N accumulation under moderate and high N conditions.
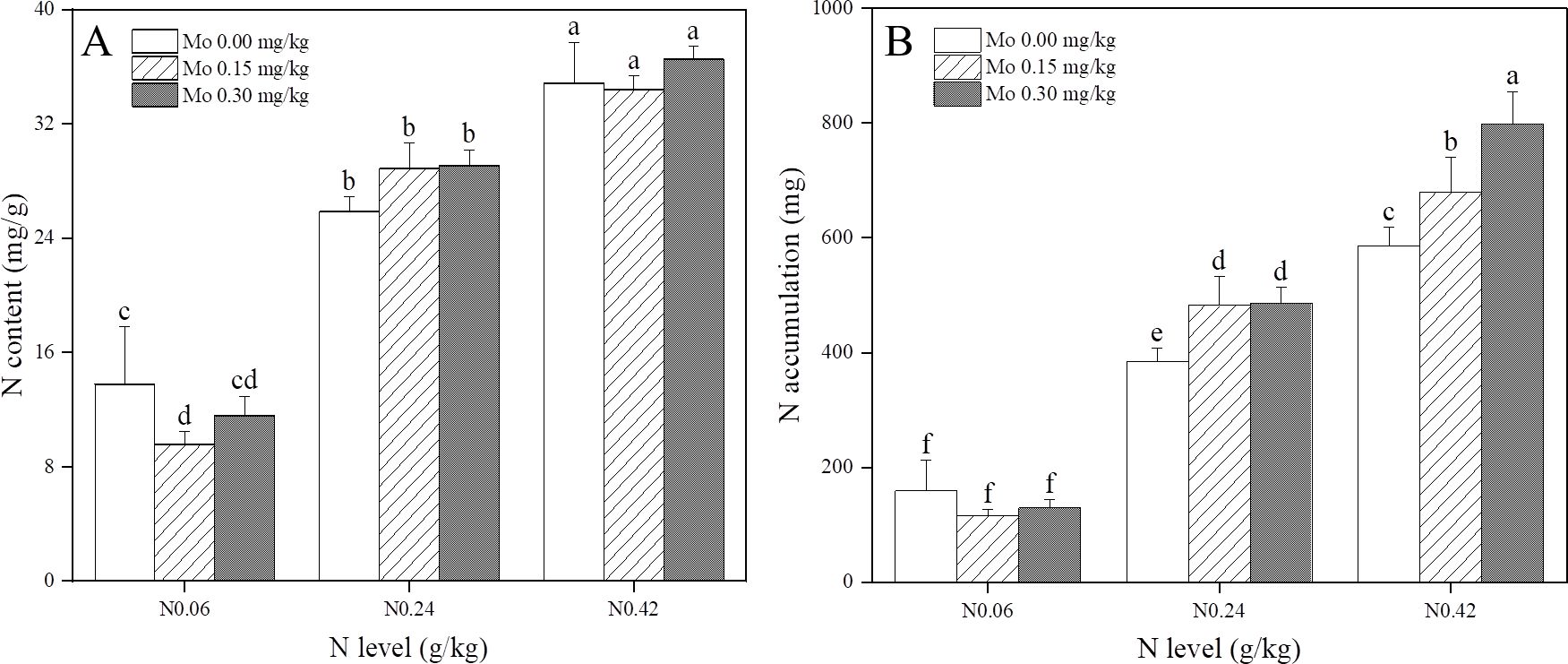
Figure 1. Effect of Mo and N application on (A) N content and (B) N accumulation in tobacco leaves at the mature stage of flue-cured tobacco. The data are the average of four biological replicates, error bars represent standard deviation, values followed by the same letters are not significantly different and different letters indicate that Tukey test is significantly different at P < 0.05.
3.3 Effects of molybdenum and nitrogen application on nitrate nitrogen content and nitrate reductase activity in tobacco leaves
Supplementary Table S2 demonstrated that both the main effects and interaction effects of Mo and N significantly influenced the nitrate nitrogen content in tobacco leaves. Specifically, with increasing N application, the NO3–N content exhibited an increasing trend across all three Mo levels. Compared to the N 0.06 treatment, the NO3–N content in tobacco leaves significantly increased by 131% (N 0.24) and 537% (N 0.42) at the Mo 0 level; by 49.15% (N 0.24) and 389.45% (N 0.42) at the Mo 0.15 level; and by 7.06% (N 0.24) and 373.54% (N 0.42) at the Mo 0.3 level (Figure 2A). Furthermore, with increasing Mo application, the NO3–N content in leaves showed no significant change under the N 0.06 treatment but exhibited a decreasing trend under the N 0.24 and N 0.42 treatments. Compared to the no Mo application level, the NO3–N content in leaves significantly decreased by 56.37% (N 0.24) and 30.05% (N 0.42) at the Mo 0.3 level (Figure 2A). These results indicated that Mo application significantly reduced tobacco leaf NO3–N content only under moderate and high N treatments, with the magnitude of reduction increasing with Mo level.
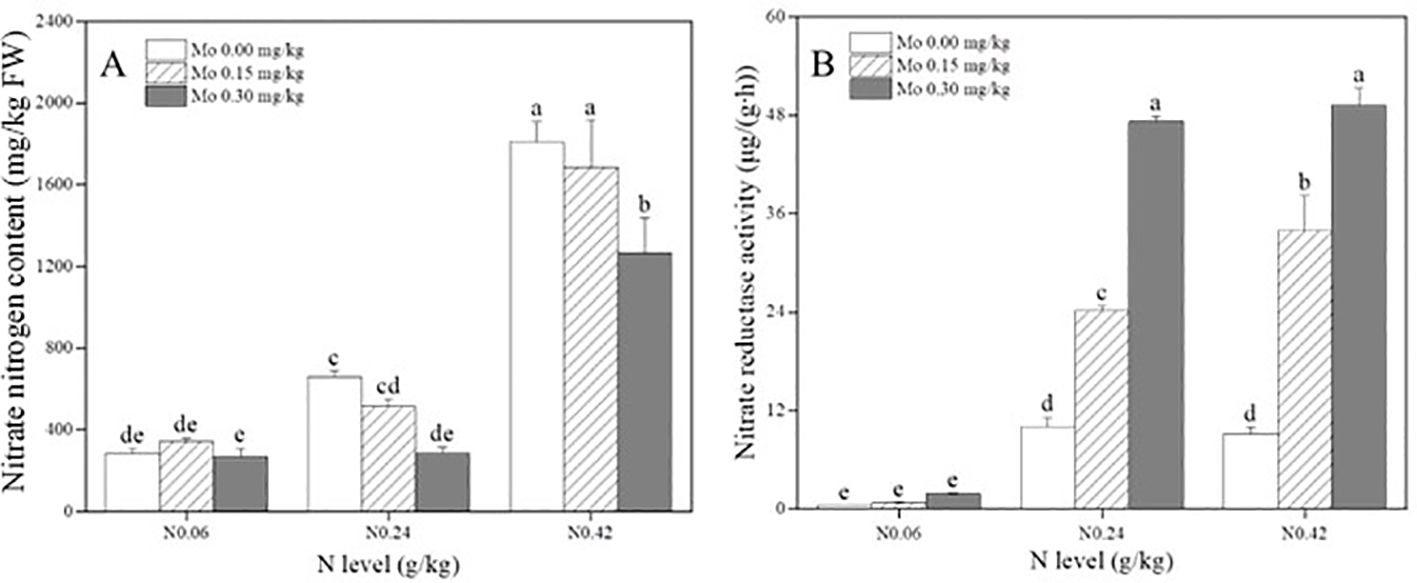
Figure 2. Effect of Mo and nitrogen application on (A) nitrate nitrogen content and (B) nitrate reductase activity in the middle leaves of flue-cured tobacco. The data are the average of four biological replicates, error bars represent standard deviation, values followed by the same letters are not significantly different and different letters indicate that Tukey test is significantly different at P < 0.05.
Additionally, Supplementary Table S2 revealed that both the main effects and interaction effects of Mo and N significantly influenced the nitrate reductase enzyme activity in tobacco leaves. With increasing N application, the nitrate reductase enzyme activity exhibited an increasing trend across all three Mo levels. Compared to the N 0.06 treatment, the nitrate reductase enzyme activity in tobacco leaves significantly increased by 22 times (N 0.24) and 20 times (N 0.42) at the Mo 0 level; by 31 times (N 0.24) and 44 times (N 0.42) at the Mo 0.15 level; and by 25 times (N 0.24) and 26 times (N 0.42) at the Mo 0.3 level (Figure 2B). Moreover, with increasing Mo application, the nitrate reductase enzyme activity in leaves showed no significant change under the N 0.06 treatment but exhibited an increasing trend under the N 0.24 and N 0.42 treatments. Compared to the no Mo application level, the nitrate reductase enzyme activity in leaves significantly increased by 143.37% (N 0.24) and 270.45% (N 0.42) at the Mo 0.15 level and by 374.67% (N 0.24) and 438.76% (N 0.42) at the Mo 0.3 level (Figure 2B). These findings suggested that Mo application significantly increased tobacco leaf nitrate reductase enzyme activity only under moderate and high N treatments, with the magnitude of increase escalating with Mo level.
3.4 Effect of molybdenum and nitrogen application on soluble protein content and free amino acid nitrogen content in tobacco leaves
Analysis of Supplementary Table S2 revealed that both the main effects of Mo and N significantly impacted the soluble protein content in tobacco leaves. Specifically, compared to the N 0.06 treatment, the N 0.24 and N 0.42 treatments led to a significant reduction in soluble protein content in leaves. The reductions were 35.45% (Mo 0), 38.26% (Mo 0.15), and 21.37% (Mo 0.3) under the N 0.24 treatment, and 25.68% (Mo 0), 29.13% (Mo 0.15), and 32.44% (Mo 0.3) under the N 0.42 treatment, as depicted in Figure 3A. Under the N 0.06 treatment, Mo 0.15 significantly increased the soluble protein content in leaves by 12.62%, whereas Mo 0.3 had no significant effect. Conversely, under the N 0.24 and N 0.42 treatments, Mo levels did not significantly affect the soluble protein content in leaves (Figure 3A). These findings suggested that increasing N fertilizer application reduces soluble protein content in leaves, and only moderate Mo application under low N treatment significantly enhances soluble protein content.
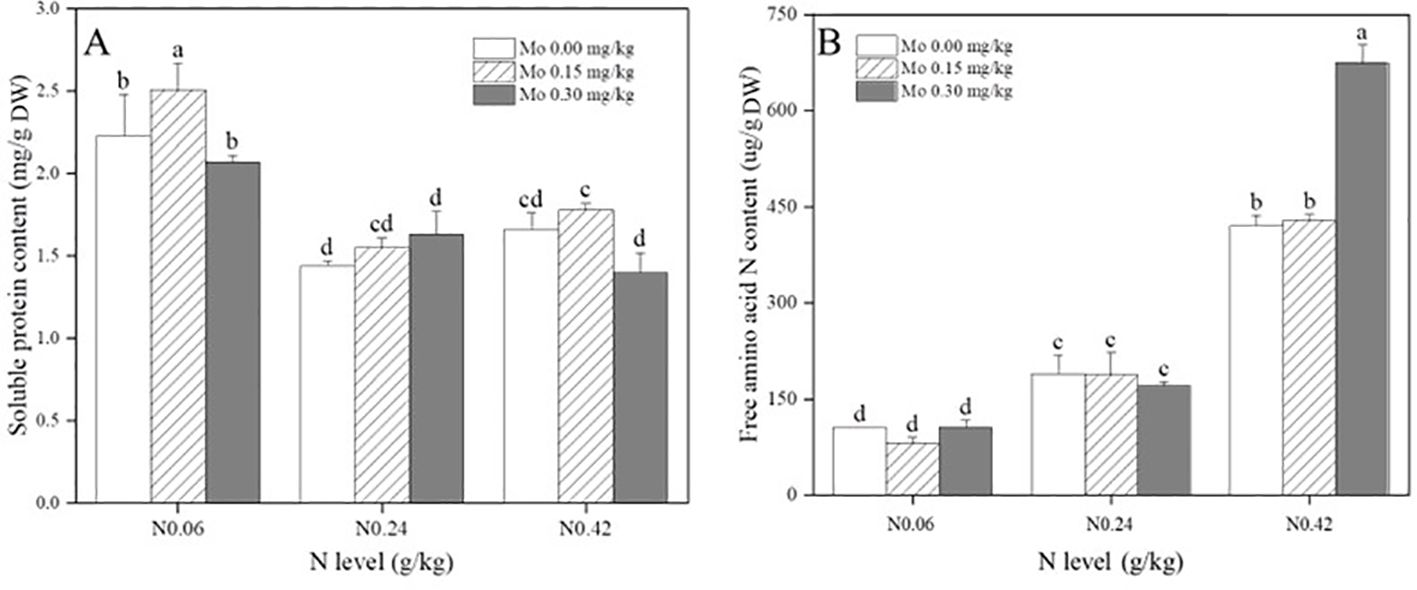
Figure 3. Effect of Mo and N application on soluble protein content (A) and free amino acid N content (B) in leaves at the mature stage of fluecured tobacco. The data are the average of four biological replicates, error bars represent standard deviation, values followed by the same letters are not significantly different and different letters indicate that Tukey test is significantly different at P < 0.05.
Furthermore, Supplementary Table S2 demonstrated that both the main effects and interaction effects of Mo and N significantly influenced the free amino acid N content in tobacco leaves. As N application increased, the free amino acid N content in leaves gradually increased. Compared to the N 0.06 treatment, the N 0.24 and N 0.42 treatments significantly increased the free amino acid N content in leaves, with increments of 78.98% (Mo 0), 130% (Mo 0.15), and 61.43% (Mo 0.3) under the N 0.24 treatment, and 297% (Mo 0), 423% (Mo 0.15), and 539% (Mo 0.3) under the N 0.42 treatment, as illustrated in Figure 3B. Under the N 0.06 and N 0.24 treatments, Mo application did not significantly affect the free amino acid N content in leaves. However, under the N 0.42 treatment, Mo 0.3 significantly increased the free amino acid N content in leaves by 60.54% (Figure 3B). These results indicated that increasing N fertilizer application significantly enhances the free amino acid N content in leaves, but only Mo application under high N treatment further augments the free amino acid N content in leaves.
3.5 Effect of molybdenum and nitrogen application on total amino acid content and individual amino acids contents in tobacco leaves
Analysis of Supplementary Table S3 revealed that only the main effect of N treatment significantly impacted the total amino acid content in tobacco leaves. Specifically, compared to the N 0.06 treatment, the N 0.24 and N 0.42 treatments led to a significant increase in total amino acid content in leaves. The increases were 147% (Mo 0), 217% (Mo 0.15), and 130% (Mo 0.3) under the N 0.24 treatment, and 206% (Mo 0), 313% (Mo 0.15), and 216% (Mo 0.3) under the N 0.42 treatment, as presented in Figure 4. Under the N 0.06 treatment, Mo 0.15 significantly decreased the total amino acid content in leaves by 21.76%. These findings indicated that increasing nitrogen fertilizer application enhances the total amino acid content in leaves, but moderate Mo application under low nitrogen treatment decreases the total amino acid content in leaves.
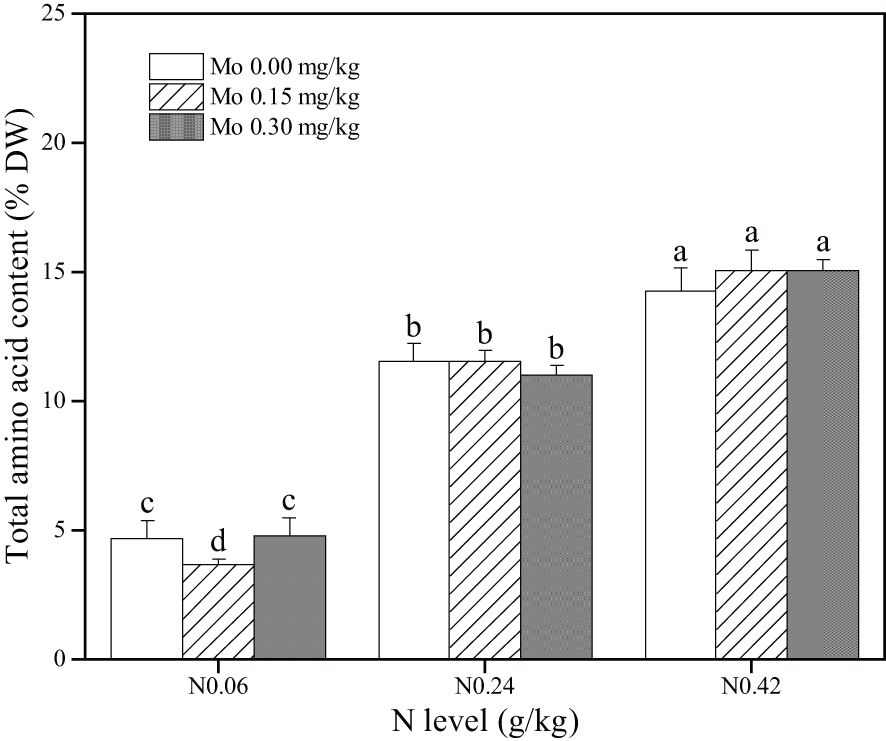
Figure 4. Effect of Mo and N application on the total amount of amino acids in the middle leaves of flue-cured tobacco. The data are the average of four biological replicates, error bars represent standard deviation, values followed by the same letters are not significantly different and different letters indicate that Tukey test is significantly different at P < 0.05.
Furthermore, the results in Supplementary Table S3 indicated that the main effect of N treatment significantly influenced the content of all amino acids in tobacco leaves. As shown in Table 1, with increasing N treatment, the content of all amino acids in leaves exhibited an increasing trend. Under the N 0.24 treatment, Mo application did not significantly affect the content of all amino acids in leaves. However, under the N 0.06 treatment, Mo 0.15 significantly decreased the content of isoleucine (Ile), methionine (Met), tyrosine (Tyr), leucine (Leu), and phenylalanine (Phe). Conversely, under the N 0.42 treatment, Mo 0.15 significantly increased the content of arginine (Arg), histidine (His), proline (Pro), and aspartic acid (Asp), while significantly decreasing the content of Tyr, Leu, Phe, and valine (Val).
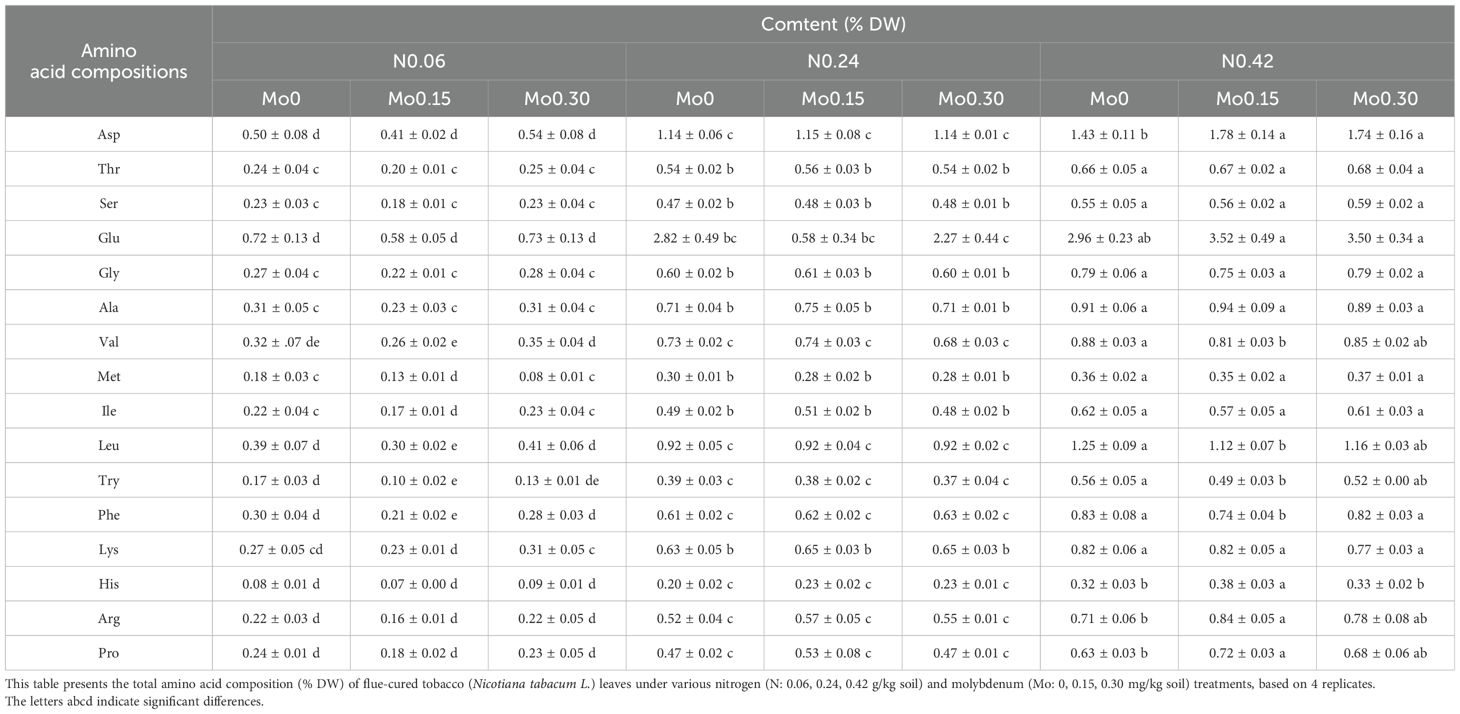
Table 1. Total amino acid composition of flue-cured tobacco leaves (Nicotiana tabacum L.) under different nitrogen and molybdenum treatments (n=4 replicates).
3.6 Effects of molybdenum and nitrogen application on the total free amino acid content and individual free amino acids contents in tobacco leaves
Analysis of Supplementary Table S4 revealed that both the main effects and interaction effects of Mo and N significantly influenced the total free amino acid content in tobacco leaves. Specifically, compared to the N 0.06 treatment, the N 0.24 and N 0.42 treatments led to a significant increase in total free amino acid content in leaves. The increases were 221% (Mo 0), 311% (Mo 0.15), and 143% (Mo 0.3) under the N 0.24 treatment, and decreases of 403% (Mo 0), 1027% (Mo 0.15), and 480% (Mo 0.3) under the N 0.42 treatment, as presented in Figure 5. Under the N 0.06 treatment, Mo 0.15 significantly decreased the total free amino acid content in leaves by 23.1%. Conversely, under the N 0.42 treatment, Mo 0.15 and Mo 0.3 significantly increased the total free amino acid content in leaves by 72.57% and 28.78%, respectively. These findings indicated that increasing nitrogen fertilizer application enhances the total free amino acid content in leaves, and Mo application under high N treatment further augments the total free amino acid content in leaves, but Mo application under low N treatment may decrease the total free amino acid content in leaves.
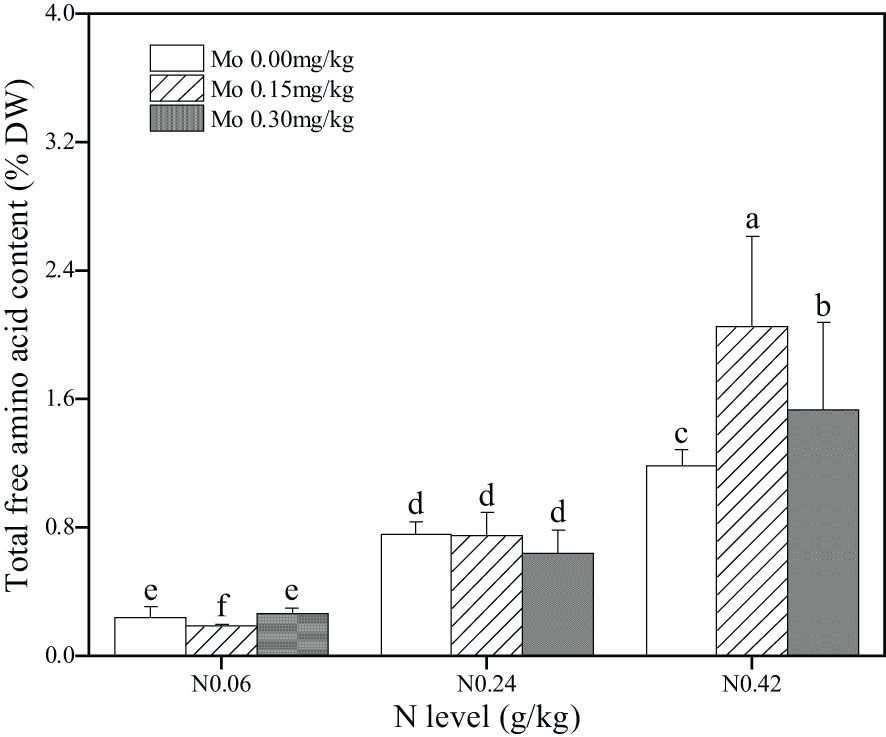
Figure 5. Effect of Mo and N application on the total free amino acids content in the middle leaves of flue-cured tobacco. The data are the average of four biological replicates, error bars represent standard deviation, values followed by the same letters are not significantly different and different letters indicate that Tukey test is significantly different at P < 0.05.
Furthermore, the results presented in Supplementary Table S4 demonstrated that the main effect of N treatment significantly influenced the content of all free amino acids in tobacco leaves. As shown in Table 2, with increasing N treatment, the content of all free amino acids in leaves exhibited an increasing trend. Under the N 0.24 treatment, Mo 0.3 significantly increased the content of histidine (His) in leaves and decreased the content of Tyr and Glu. Under the N 0.42 treatment, Mo 0.15 significantly increased the content of His, Arg, Asp, Ile, Phe, Glu, Ala, Pro, and Thr, and decreased the content of Ser. This indicated that under low and moderate nitrogen treatments, Mo has minimal effect on the free amino acid content in leaves, while under high N treatment, the Mo 0.15 level showed a significant enhancing effect on the free amino acid content.
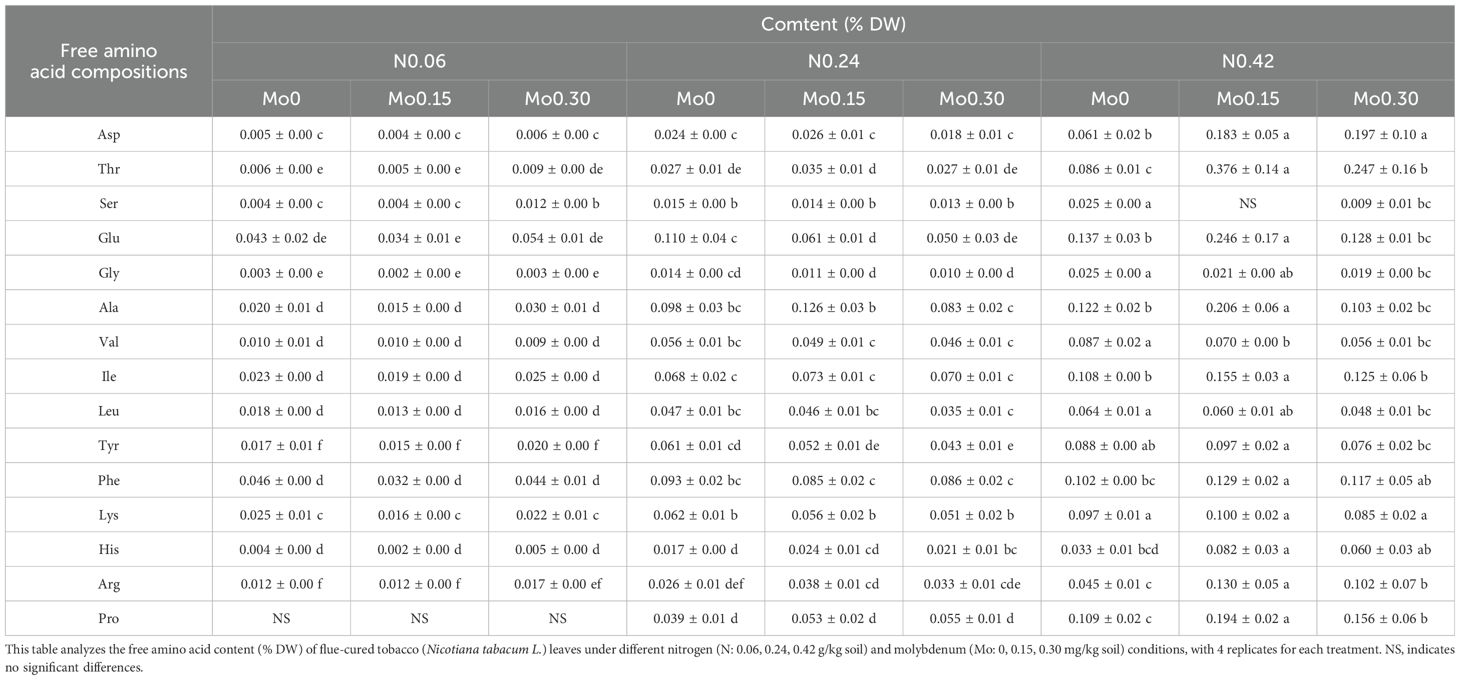
Table 2. Free amino acid content analysis of flue-cured tobacco leaves (Nicotiana tabacum L.) under varying nitrogen and molybdenum conditions (n=4 replicates).
4 Discussion
This study found that the combined treatment of 0.24 g/kg soil N and 0.30 mg/kg soil Mo had a significant positive impact on N metabolism and amino acid content in tobacco plants, outperforming all other tested fertilizer treatments. The fundamental reason for this remarkable enhancement in tobacco growth and quality lies in the synergistic effect of N and Mo, which has been previously observed in prior studies (Liu et al., 2017; dos Santos et al., 2019; Imran et al., 2021) but was further validated and elaborated in our research.
Notably, this study observed that Mo application significantly increased tobacco biomass only under moderate and high N levels, an important finding with practical implications for fertilizer application strategies. This specificity arises from several underlying factors: Firstly, N is an indispensable nutrient for plant growth and development, playing a crucial role in photosynthesis, nutrient uptake, and biomass accumulation (Below, 2001; Lawlor et al., 2001; Leghari et al., 2016). When N supply is adequate, plants can effectively utilize this nutrient for growth and development, facilitating biomass accumulation. Conversely, under N deficiency, plant growth is restricted, and Mo application alone cannot significantly boost biomass due to the limiting role of N. Furthermore, Mo, as an essential micronutrient, is integral to N metabolism, particularly as a cofactor in nitrate reductase, directly involved in converting nitrate N to ammonium N (Zimmer and Mendel, 1999; Kovács et al., 2015; Imran et al., 2019; Wen et al., 2019). At moderate and high N levels, adequate Mo supply significantly enhances NR activity (Figure 2B), accelerating nitrate reduction and utilization, thereby promoting N uptake and assimilation, leading to enhanced growth and biomass accumulation. Additionally, Mo may affect plant growth through other mechanisms, such as regulating hormone synthesis and signaling pathways, and contributing to the plant’s antioxidant system (Khan et al., 2018; Nazir et al., 2020). Our study also revealed significant changes in nitrate reductase activity and NO3–N content in response to varying N and Mo fertilization treatments. To understand these changes, this study analyzed the following mechanisms: NR, a key enzyme in plant N metabolism, directly determines the efficiency of nitrate conversion to ammonium (Liao et al., 2023; Zayed et al., 2023; Li et al., 2024). The results showed that NR activity significantly increased with Mo application under moderate and high N conditions (Figure 2B). This can be attributed to Mo being a cofactor of NR, and its adequate supply promotes NR synthesis and function, accelerating nitrate reduction. This process not only improves N use efficiency but also reduces nitrate accumulation in plants, enhancing crop quality and safety. The nitrate content was inversely related to NR activity, with higher NR activity leading to lower nitrate accumulation, especially under high N conditions. Under low N conditions, nitrate supply is limited, and even with Mo application, NR activity enhancement was constrained by N availability, resulting in no significant decrease in nitrate content.
Furthermore, this study found that combined Mo and N application significantly increased specific free amino acids beneficial to tobacco sensory quality, particularly histidine (His), arginine (Arg), aspartic acid (Asp), isoleucine (Ile), and glutamic acid (Glu) under the 0.24 g/kg N + 0.3 mg/kg Mo treatment. These amino acids contribute to tobacco’s aroma, sweetness, and umami taste, significantly improving tobacco’s sensory profile (Liu et al., 2023). Specifically, our findings indicated that combined application of 0.30 mg/kg Mo and 0.24 g/kg N optimized tobacco leaf amino acid composition, enhancing overall quality. Based on these findings, this study recommended the following fertilization practices: accurately assessing soil Mo and N levels to identify deficiencies; supplementing Mo, particularly in Mo-deficient soils, using sources like ammonium molybdate; and scientifically calculating and applying N fertilizer, while concurrently adding Mo to leverage their synergistic effects. This approach not only improved N use efficiency but also optimized amino acid composition, enhancing tobacco quality and sensory attributes. Moreover, the treatment with 0.24 g/kg N and 0.30 mg/kg Mo not only increased tobacco biomass (Figure 6) but also reduced potentially carcinogenic nitrosamine synthesis by decreasing nitrate N content in leaves (Figure 2), thereby enhancing tobacco safety and aligning the final product with public health requirements. This approach offered a novel perspective for the tobacco industry by improving tobacco leaf quality and safety through scientific fertilization strategies. From the perspective of the N-Mo synergy, this study further elucidated the interaction mechanism between trace elements and major nutrients in tobacco leaf growth and quality formation (Supplementary Tables S2–S4). These interactions not only influenced N metabolism pathways but also optimized amino acid composition, crucial factors affecting tobacco leaf quality (Yang et al., 2022; Li et al., 2023). Principal Component Analysis (PCA) validated our results, further amplifying treatment differences and comprehensively evaluating the effects of varying Mo and N concentrations on tobacco quality attributes (biomass, N content, N accumulation, nitrate N, nitrate reductase activity, soluble protein, free amino acid N, total amino acids). The treatment efficacy ranking was N0.24 Mo0.3 > N0.42 Mo0.3 > N0.42 Mo0.15 > N0.42 Mo0 > N0.24 Mo0.15 > N0.06 Mo0.15 > N0.24 Mo0 > N0.06 Mo0.3 > N0.06 Mo0 (Supplementary Table S5), emphasizing the optimal combination of 0.24 g/kg N and 0.30 mg/kg Mo.
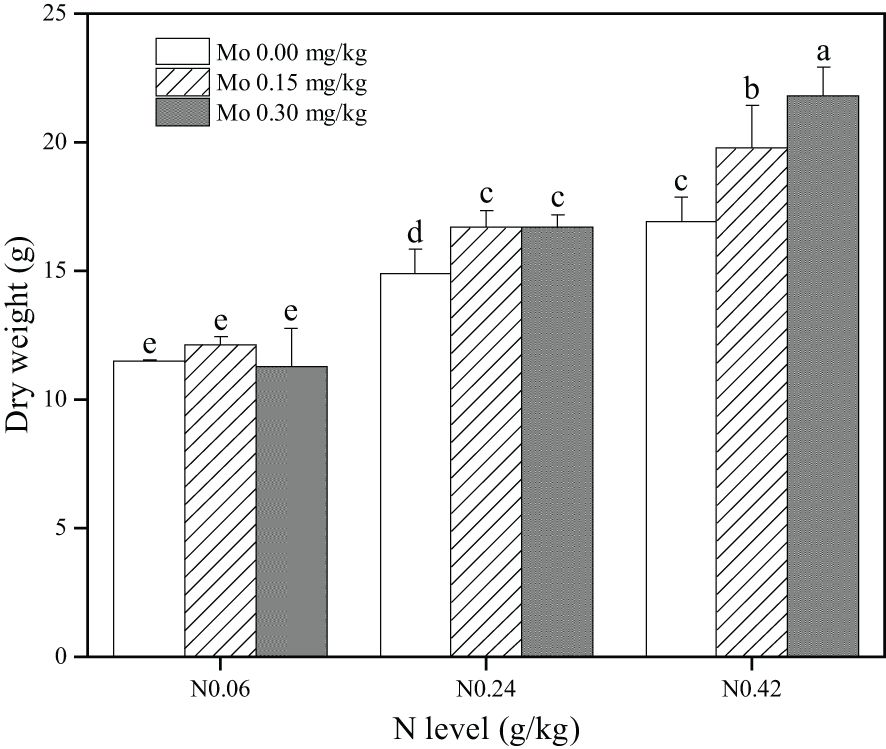
Figure 6. Effect of Mo and N application on dry weight at the mature stage of flue-cured tobacco. The data are the average of four biological replicates, error bars represent standard deviation, values followed by the same letters are not significantly different and different letters indicate that Tukey test is significantly different at P < 0.05.
In this study, an intriguing observation was made: under low N conditions, an increase in Mo application resulted in a decrease in N content in tobacco leaves. This phenomenon underscores the complex physiological interactions between N and Mo, warranting further investigation. We speculate that this may be related to the regulation of N uptake and translocation mechanisms. Under low N conditions, plants prioritize the allocation of limited N resources to vital life processes such as photosynthesis and respiration to ensure basic survival requirements (Nord et al., 2011; Simontacchi et al., 2015; Zhang et al., 2020). Therefore, excessive Mo application may disturb the equilibrium of N distribution within the plant, leading to reduced N accumulation in leaves. Mo serves as a critical component of nitrate reductase, a key enzyme in N metabolism. However, under low N conditions, nitrate reductase activity may be constrained by the limited N supply. Consequently, even with increased Mo availability, the activity of nitrate reductase and overall N metabolism efficiency may not be significantly enhanced. Instead, excess Mo may exert an unforeseen influence on other enzymes or regulatory factors involved in N metabolism pathways, thereby impacting N absorption and utilization. Furthermore, we cannot rule out the possibility of an antagonistic relationship between Mo and N under certain conditions, though such interactions are relatively uncommon in plant nutrition. This potential antagonism could affect plant N uptake, translocation, and assimilation processes, ultimately contributing to the observed decrease in N content. To gain a deeper understanding of the underlying physiological mechanisms, we recommend that future research focus on the following aspects: 1) investigating the absorption, translocation, and distribution patterns of N and Mo within plants; 2) examining the impact of Mo on key enzyme activities and gene expression in N metabolism pathways; and 3) assessing the combined effects of varying N and Mo concentrations on plant physiological and biochemical characteristics. Through these efforts, we aim to uncover the intricate interactions between N and Mo, providing a more scientific basis for rational fertilization practices and high-yield, high-quality crop cultivation. In conclusion, by adjusting fertilization ratios, particularly by appropriately adding Mo based on N application, the overall quality of tobacco leaves can be significantly improved, enhancing aroma and smoking taste. This study provides a scientific basis for optimizing N and trace element fertilizer use in tobacco production and serves as a reference for nutrient management in other crops, promoting sustainable agricultural development and public health.
5 Conclusions
In summary, the application of N fertilizer was found to be the decisive factor in determining the total nitrogen, nitrate nitrogen, soluble protein, and amino acid nitrogen content in tobacco leaves. Specifically, the combined application of Mo and N at moderate levels (0.24 g/kg soil N and 0.30 mg/kg soil Mo) yielded the most significant improvements. This treatment combination significantly increased N content, accumulation, and dry matter mass by 110.88%, 204.90%, and 48.81%, respectively. Furthermore, nitrate reductase activity surged by 107.36-fold, leading to a notable reduction in nitrate nitrogen content and an increase in free amino acid nitrogen content by 170.66%. Importantly, this optimized amino acid composition, specifically enhancing histidine, arginine, aspartic acid, isoleucine, and glutamic acid, which positively impacted the sensory quality of tobacco leaves.
Data availability statement
The raw data supporting the conclusions of this article will be made available by the authors, without undue reservation.
Author contributions
JS: Writing – review & editing. YZ: Methodology, Writing – original draft. XQ: Conceptualization, Writing – review & editing. ZH: Investigation, Writing – review & editing. JL: Investigation, Writing – review & editing. YG: Investigation, Writing – review & editing. GS: Investigation, Writing – review & editing. ZC: Investigation, Writing – review & editing. HH: Investigation, Writing – review & editing. CH: Project administration, Writing – review & editing. XS: Funding acquisition, Writing – review & editing.
Funding
The author(s) declare financial support was received for the research, authorship, and/or publication of this article. This work was supported by the Project of China National Tobacco Corporation Hubei Province Company (027Y2022-003), the Project of Guizhou Provincial Tobacco Company Qianxinan Branch (2021-05) and Company Key Project of China National Tobacco Corporation (110201902005).
Acknowledgments
We express our gratitude to Chai Liguang and Tian Huiwen for providing the experimental facilities that were essential for this study.
Conflict of interest
Author LC was employed by Shiyan Tobacco Company of Hubei Province Corporation. Author YG was employed by Guizhou Provincial Tobacco Company Qianxinan Branch. Author HH was employed by Honghong, Wengfu Group Co., Ltd.
The remaining authors declare that the research was conducted in the absence of any commercial or financial relationships that could be construed as a potential conflict of interest.
Publisher’s note
All claims expressed in this article are solely those of the authors and do not necessarily represent those of their affiliated organizations, or those of the publisher, the editors and the reviewers. Any product that may be evaluated in this article, or claim that may be made by its manufacturer, is not guaranteed or endorsed by the publisher.
Supplementary material
The Supplementary Material for this article can be found online at: https://www.frontiersin.org/articles/10.3389/fagro.2024.1427571/full#supplementary-material
Abbreviations
Mo, molybdenum; N, nitrogen; C, Carbon; NR, nitrate reductase; Phe, phenylalanine; Tyr, tyrosine; Glu, glutamic acid; Ala, Alanine; Pro, proline; Asp, aspartic acid; Arg, arginine; Val, valine; Gly, glycine; His, histidine; Ile, isoleucine; Met, methionine; Ser, serine; Thr, threonine; Leu, leucine; Lys, lysine
References
Alewell C., Ringeval B., Ballabio C., Robinson D. A., Panagos P., Borrelli P. (2020). Global phosphorus shortage will be aggravated by soil erosion. Nat. Commun. 11, 4546. doi: 10.1038/s41467-020-18326-7
Baki G. K. A.-E., Siefritz F., Man H.-M., Weiner H., Kaldenhoff R., Kaiser W. M. (2000). Nitrate reductase in Zea mays L. under salinity. Plant Cell Environ. 23, 515–521. doi: 10.1046/j.1365-3040.2000.00568.x
Banerjee P., Nath R. (2022). Prospects of molybdenum fertilization in grain legumes-A review. J. Plant Nutr. 45, 1425–1440. doi: 10.1080/01904167.2021.2020831
Below F. E. (2001). Nitrogen metabolism and crop productivity[M]/Handbook of plant and crop physiology. CRC Press 407–428.
Bradford M. M. (1976). A rapid and sensitive method for the quantitation of microgram quantities of protein utilizing the principle of protein-dye binding. Analytical Biochem. 72, 248–254. doi: 10.1016/0003-2697(76)90527-3
Bursakov S. A., Kroupin P. Y., Karlov G. I., Divashuk M. G. (2023). Tracing the element: the molecular bases of molybdenum homeostasis in legumes. Agronomy 13, 2300. doi: 10.3390/agronomy13092300
Chatterjee C., Nautiyal N. (2001). Molybdenum stress affects viability and vigor of wheat seeds. J. Plant Nutr. 24, 1377–1386. doi: 10.1081/PLN-100106988
Chen S., Klotzbücher T., Lechtenfeld O. J., Hong H., Liu C., Kaiser K., et al. (2022). Legacy effects of sorption determine the formation efficiency of mineral-associated soil organic matter. Environ. Sci. Technol. 56, 2044–2053. doi: 10.1021/acs.est.1c06880
dos Santos R. L., Freire F. J., de Oliveira E. C. A., Freire M.B.G.d., West J. B., Barbosa J.de A., et al. (2019). Nitrate reductase activity and nitrogen and biomass accumulation in sugarcane under molybdenum and nitrogen fertilization. Rev. Bras. Ciênc. Solo 43, e0180171. doi: 10.1590/18069657rbcs20180171
Drescher G. L., Da silva L. S., Sarfaraz Q., Molin G. D., Marzari L. B., Lopes A. F., et al. (2020). Alkaline hydrolyzable nitrogen and properties that dictate its distribution in paddy soil profiles. Pedosphere 30, 326–335. doi: 10.1016/S1002-0160(20)60012-1
Elrys A. S., Abdo A. I. E., Desoky E. S. M. (2018). Potato tubers contamination with nitrate under the influence of nitrogen fertilizers and spray with molybdenum and salicylic acid. Environ. Sci. pollut. Res. 25, 7076–7089. doi: 10.1007/s11356-017-1075-y
Grahmann K., Verhulst N., Buerkert A., Ortiz-Monasterio I., Govaerts B. (2014). Nitrogen use efficiency and optimization of nitrogen fertilization in conservation agriculture. CABI Rev. 2013, 1–19. doi: 10.1079/PAVSNNR20138053
Imran M., Sun X., Hussain S., Ali U., Rana M. S., Rasul F., et al. (2019). Molybdenum-induced effects on nitrogen metabolism enzymes and elemental profile of winter wheat (Triticum aestivum L.) under different nitrogen sources. Int. J. Mol. Sci. 20, 3009. doi: 10.3390/ijms20123009
Imran M., Sun X., Hussain S., Rana M. S., Saleem M. H., Riaz M., et al. (2021). Molybdenum supply increases root system growth of winter wheat by enhancing nitric oxide accumulation and expression of NRT genes. Plant Soil 459, 235–248. doi: 10.1007/s11104-020-04765-0
Khan D. A., Ali Z., Iftikhar S., Amraiz D., Zaidi N.-S. S., Gul A., et al. (2018). “Role of Phytohormones in Enhancing Antioxidant Defense in Plants Exposed to Metal/Metalloid Toxicity,” in Plants Under Metal and Metalloid Stress: Responses, Tolerance and Remediation. Eds. Hasanuzzaman M., Nahar K., Fujita M. (Springer, Singapore), 367–400. doi: 10.1007/978-981-13-2242-6_14
Kovács B., Puskás-Preszner A., Huzsvai L., Lévai L., Bódi É. (2015). Effect of molybdenum treatment on molybdenum concentration and nitrate reduction in maize seedlings. Plant Physiol. Biochem. 96, 38–44. doi: 10.1016/j.plaphy.2015.07.013
Latifinia E., Eisvand H. R. (2022). Soybean physiological properties and grain quality responses to nutrients, and predicting nutrient deficiency using chlorophyll fluorescence. J. Soil Sci. Plant Nutr. 22, 1942–1954. doi: 10.1007/s42729-022-00785-0
Lawlor D. W., Lemaire G., Gastal F. (2001). “Nitrogen, Plant Growth and Crop Yield,” in Plant Nitrogen. Eds. Lea P. J., Morot-Gaudry J.-F. (Springer, Berlin, Heidelberg), 343–367. doi: 10.1007/978-3-662-04064-5_13
Leghari S. J., Wahocho N. A., Laghari G. M., HafeezLaghari A., MustafaBhabhan G., HussainTalpur K., et al. (2016). Role of nitrogen for plant growth and development: a review. Adv. Environ. Biol. 10, 209–219. Available at: link.gale.com/apps/doc/A472372583/AONE?u=anon~878ead3&sid=bookmark-AONE&xid=0bed94a3
Li G., Guo X., Sun W., Hou L., Wang G., Tian R., et al. (2024). Nitrogen application in pod zone improves yield and quality of two peanut cultivars by modulating nitrogen accumulation and metabolism. BMC Plant Biol. 24, 48. doi: 10.1186/s12870-024-04725-1
Li N., Yu J., Yang J., Wang S., Yu L., Xu F., et al. (2023). Metabolomic analysis reveals key metabolites alleviating green spots under exogenous sucrose spraying in air-curing cigar tobacco leaves. Sci. Rep. 13, 1311. doi: 10.1038/s41598-023-27968-8
Liao G., Yang Y., Xiao W., Mo Z. (2023). Nitrogen modulates grain yield, nitrogen metabolism, and antioxidant response in different rice genotypes. J. Plant Growth Regul. 42, 2103–2114. doi: 10.1007/s00344-022-10684-4
Liu L., Xiao W., Li L., Li D.-M., Gao D.-S., Zhu C., et al. (2017). Effect of exogenously applied molybdenum on its absorption and nitrate metabolism in strawberry seedlings. Plant Physiol. Biochem. 115, 200–211. doi: 10.1016/j.plaphy.2017.03.015
Liu L., Zhao Y., Lu S., Liu Y., Xu X., Zeng M. (2023). Metabolomics investigation on the volatile and non-volatile composition in enzymatic hydrolysates of Pacific oyster (Crassostrea gigas). Food Chemistry: X 17, 100569. doi: 10.1016/j.fochx.2023.100569
Lu C., Tian H. (2017). Global nitrogen and phosphorus fertilizer use for agriculture production in the past half century: shifted hot spots and nutrient imbalance. Earth System Sci. Data 9, 181–192. doi: 10.5194/essd-9-181-2017
Mahboob W., Yang G., Irfan M. (2023). Crop nitrogen (N) utilization mechanism and strategies to improve N use efficiency. Acta Physiol. Plant 45, 52. doi: 10.1007/s11738-023-03527-6
Mustafa A., Åman P., Andersson R., Kamal-Eldin A. (2007). Analysis of free amino acids in cereal products. Food Chem. 105, 317–324. doi: 10.1016/j.foodchem.2006.11.044
Nandety A., Missaoui A. M. (2020). “Micronutrients: advances in understanding molybdenum in crop production,” in Achieving sustainable crop nutrition (Burleigh Dodds Science Publishing).
Nazir F., Fariduddin Q., Khan T. A. (2020). Hydrogen peroxide as a signalling molecule in plants and its crosstalk with other plant growth regulators under heavy metal stress. Chemosphere 252, 126486. doi: 10.1016/j.chemosphere.2020.126486
Ng J. F., Ahmed O. H., Jalloh M. B., Omar L., Kwan Y. M., Musah A. A., et al. (2022). Soil nutrient retention and pH buffering capacity are enhanced by calciprill and sodium silicate. Agronomy 12, 219. doi: 10.3390/agronomy12010219
Nord E. A., Shea K., Lynch J. P. (2011). Optimizing reproductive phenology in a two-resource world: a dynamic allocation model of plant growth predicts later reproduction in phosphorus-limited plants. Ann. Bot. 108, 391–404. doi: 10.1093/aob/mcr143
Oliveira S. L., Crusciol C. A. C., Rodrigues V. A., Galeriani T. M., Portugal J. R., Bossolani J. W., et al. (2022). Molybdenum foliar fertilization improves photosynthetic metabolism and grain yields of field-grown soybean and maize. Front. Plant Sci. 13. doi: 10.3389/fpls.2022.887682
Rebouh N. Y., Khugaev C. V., Utkina A. O., Isaev K. V., Mohamed E. S., Kucher D. E. (2023). Contribution of eco-friendly agricultural practices in improving and stabilizing wheat crop yield: A review. Agronomy 13, 2400. doi: 10.3390/agronomy13092400
Salehzadeh H., Maleki A., Rezaee R., Shahmoradi B., Ponnet K. (2020). The nitrate content of fresh and cooked vegetables and their health-related risks. PloS One 15, e0227551. doi: 10.1371/journal.pone.0227551
Simontacchi M., Galatro A., Ramos-Artuso F., Santa-María G. E. (2015). Plant survival in a changing environment: the role of nitric oxide in plant responses to abiotic stress. Front. Plant Sci. 6. doi: 10.3389/fpls.2015.00977
Wen X., Hu C., Sun X., Zhao X., Tan Q. (2019). Research on the nitrogen transformation in rhizosphere of winter wheat (Triticum aestivum) under molybdenum addition. Environ. Sci. pollut. Res. 26, 2363–2374. doi: 10.1007/s11356-018-3565-y
Yahaya S. M., Mahmud A. A., Abdullahi M., Haruna A. (2023). Recent advances in the chemistry of nitrogen, phosphorus and potassium as fertilizers in soil: A review. Pedosphere 33, 385–406. doi: 10.1016/j.pedsph.2022.07.012
Yang R., Yang J., Yu J., Wang S., Yang C., Xu F. (2022). Effects of different nitrogen application rates on the quality and metabolomics of cigar tobacco. Agron. J. 114, 1155–1167. doi: 10.1002/agj2.20983
Zayed O., Hewedy O. A., Abdelmoteleb A., Ali M., Youssef M. S., Roumia A. F., et al. (2023). Nitrogen journey in plants: from uptake to metabolism, stress response, and microbe interaction. Biomolecules 13, 1443. doi: 10.3390/biom13101443
Zhang X., Davidson E. A., Mauzerall D. L., Searchinger T. D., Dumas P., Shen Y. (2015). Managing nitrogen for sustainable development. Nature 528, 51–59. doi: 10.1038/nature15743
Zhang J., He N., Liu C., Xu L., Chen Z., Li Y., et al. (2020). Variation and evolution of C:N ratio among different organs enable plants to adapt to N-limited environments. Global Change Biol. 26, 2534–2543. doi: 10.1111/gcb.14973
Zimmer W., Mendel R. (1999). Molybdenum metabolism in plants. Plant Biol. (Stuttg) 1, 160–168. doi: 10.1055/s-2007-978502
Zou C., Gao X., Shi R., Fan X., Zhang F. (2008). “Micronutrient Deficiencies in Crop Production in China,” in Micronutrient Deficiencies in Global Crop Production. Ed. Alloway B. J. (Springer Netherlands, Dordrecht), 127–148. doi: 10.1007/978-1-4020-6860-7_5
Keywords: molybdenum, nitrogen, combined application, total nitrogen, nitrate nitrogen, free amino acids
Citation: Sun J, Zhao Y, Qin X, Hu Z, Li J, Guo Y, Sun G, Chen Z, Huang H, Hu C and Sun X (2024) Combined effect of molybdenum and nitrogen fertilization on nitrogen metabolism and amino acid content in tobacco leaves. Front. Agron. 6:1427571. doi: 10.3389/fagro.2024.1427571
Received: 04 May 2024; Accepted: 19 August 2024;
Published: 15 October 2024.
Edited by:
Ioanna Kakabouki, Agricultural University of Athens, GreeceCopyright © 2024 Sun, Zhao, Qin, Hu, Li, Guo, Sun, Chen, Huang, Hu and Sun. This is an open-access article distributed under the terms of the Creative Commons Attribution License (CC BY). The use, distribution or reproduction in other forums is permitted, provided the original author(s) and the copyright owner(s) are credited and that the original publication in this journal is cited, in accordance with accepted academic practice. No use, distribution or reproduction is permitted which does not comply with these terms.
*Correspondence: Xuecheng Sun, c3hjY25AbWFpbC5oemF1LmVkdS5jbg==