- 1Laboratory of Dry Land Farming and Oasis Cropping, Institute of Arid Regions (IRA), Médenine, Tunisia
- 2National Institute of Agronomy of Tunisia (INAT), University of Carthage, Tunis, Tunisia
Crops’ water requirement is generally higher than the annual average precipitation in arid environments characterized by scarce freshwater resources. While using saline water for irrigation can help sustain agriculture in water-stressed regions, several challenges arises concerning productivity and soil salinization. However, adoption of efficient irrigation techniques such as drip irrigation, irrigation scheduling, and deficit irrigation can help optimize water productivity and mitigate salinity problems in irrigated agriculture. In southern Tunisia, potato is considered among the main cultivated horticultural crops due to its high economic value while it is considered as a crop sensitive to salinity. This crop (cv. Spunta) was the subject of long-term studies (2002–2020) conducted during the fall period in the arid region of Médenine. The crop response to full and deficit irrigation with saline water was assessed for several seasons under contrasting climatic conditions. Scheduling using the soil water balance (SWB) method consisted of the total and/or partial replacement of accumulated crop evapotranspiration (ETc), as derived from climatic data and crop coefficients. The impact of decreasing amounts of irrigation waters on crop yield and soil salinity with waters having a salinity ranging between 3 and 7 dS m−1 was evaluated. Results showed improvements in yield (30% to 37%) obtained with the SWB strategy under actual farming conditions, supporting the use of this strategy for irrigation. Appropriate scheduling also seems to be a key element in saving water (15%–22%) and in reducing risks of soil salinization. In the dry environment of southern Tunisia, optimum supply seems to correspond to a replacement of 100% to approximately 70%–80% of ETc. Applying such irrigation levels resulted in a lower salinity buildup in the root zone and higher crop water productivity. Natural salt leaching seems to be more effective under a more humid soil profile. Yield decreases and soil salinity increases almost linearly (r2 = 0.60) with decreasing irrigation water amounts. Future work should focus on the integration of management practices when using saline water. Investigating the relationship and interaction between irrigation amounts, cultivar, fertilizer supply, and salt leaching will help in resolving productivity and environmental issues.
1 Introduction
The recent development of irrigation in the arid regions of Tunisia using shallow groundwater resulted in overexploitation, the effect of which started to be felt by farmers. Therefore, innovations in irrigation management are needed, which should be carried out more efficiently, aiming at saving water and maximizing its productivity in order to mitigate the increasing water scarcity situation. The adoption of good irrigation management practices is required to optimize water use and improve farmers’ practices. Many studies have reported substantial increases in crop yields as a result of suitable irrigation management, including studies under saline conditions (Ali et al., 2007; Nagaz et al., 2013; Irfan et al., 2014; and Ahmed et al., 2023). In the absence of significant rainfall events that can improve natural leaching, irrigated farming in arid lands is exposed to accumulation of salts in the soil.
Good management should take into consideration the effect of irrigation on the crop yield and, at the same time, on the environment, particularly the risk of soil salinization. Both quantity and quality of water to be used and their effects on farm productivity need to be precisely determined. Considerable research has been directed towards evaluating the effects of salts on crop growth and development (Maas, 1990; Shalhevet, 1994; Wang et al., 2023 and Ebrahimian et al., 2023). The current practices in arid regions show the importance of potato crops in the annual crops’ rotation (El Mokh et al., 2017; Si et al., 2024). With such practices, various crops are grown over different seasons in order to optimize the use of water and manure. Thus, crops with a short cycle such as potato are used for their high economic value and their high water productivity especially when cultivated during the rainy season. However, the management and the amount of irrigation water used by local farmers remains empirical (El Mokh, 2016; El Mokh et al., 2022a). Large water losses induced by the current methods of irrigation or the scheduling used by farmers could be avoided by providing them with simple tools and techniques.
One way to improve water productivity is to adopt efficient irrigation systems, such as drip irrigation. However, field surveys show that even with such efficient equipment, low productivity is observed by most farmers due to inadequate scheduling and overirrigation. In order to make efficient use of this irrigation system, adequate irrigation scheduling techniques that totally [full irrigation (FI)] or partially [deficit irrigation (DI)] meet crop requirements should be developed and promoted among farmers. FI consists of applying 100% of the crop water requirement (ETc) and seeks maximum yield. DI provides a means of reducing water consumption by applying a fraction of ETc while minimizing adverse effects on yield (Fereres and Soriano, 2007; Shammout et al., 2018; Fitsum et al., 2023; Xu et al., 2024) and seeks to maximize water productivity. However, when saline water is used, reduction of the amount of irrigation presents the risk of soil salinization and can be successful only if some measures are taken to avoid this process. The irrigation from private shallow wells constitutes an advantage for the farmers in the region. They have the option to choose the time and to control the amount of irrigation with more flexibility without the constraints imposed to farmers in the irrigated public perimeters (Smith, 1985). This flexibility could facilitate the development and management of the DI program in private farms under arid conditions. In the absence of drainage systems and under conditions of high evaporative demand and low amounts of rainfall, techniques based on DI scheduling with reduced impact on yield should be developed and tested.
A good understanding and assessment of the long-term impact of DI strategy on water consumptive use, crop productivity, and salt accumulation in the soil is also necessary before its recommendation among farmers. The grower should have prior knowledge of the crop yield responses to DI. In this context, a series of field experiments were conducted within the farmer’s fields in the arid region of southern Tunisia in order to evaluate farmer practices and investigate the response of potato crops to different water supplies under the local farmer’s conditions.
2 Materials and methods
2.1 Experimental site, design, and climate
Several irrigation-scheduling experiments were carried out for 8 years between 2002 and 2020 in fields situated in southeastern Tunisia. Every autumn season, potato crops (Solanum tuberosum L., cv. Spunta) were cultivated in order to evaluate their response to different irrigation regimes with saline water, focusing on soil salinity, yield, and water productivity. The rainfall received during the cropping periods ranged between 0 and 112 mm; during the 2012 season, no rainfall was received since it was a dry year (Figure 1).
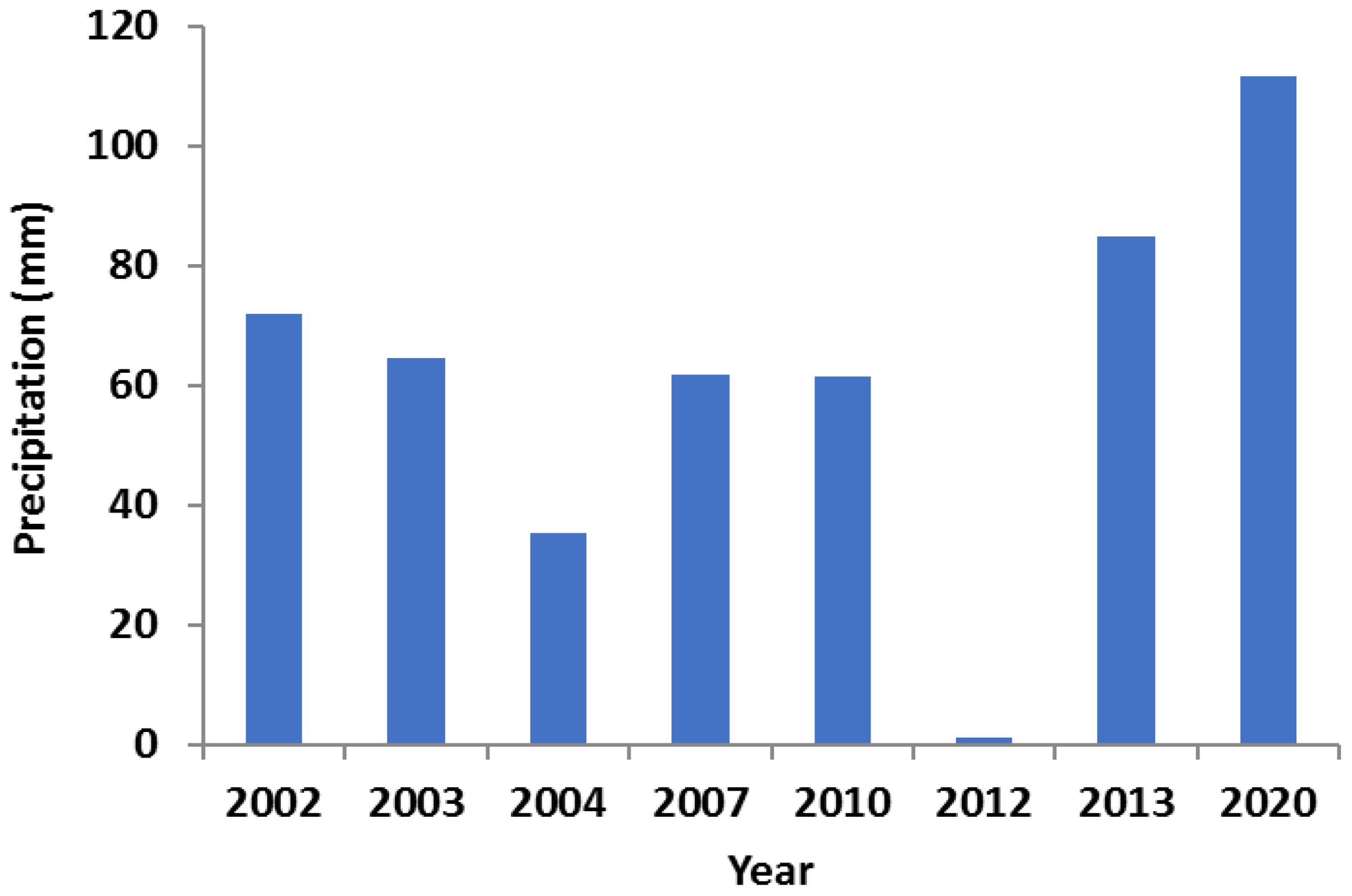
Figure 1. Rainfall received by the potato crop during September–December for the 8 years of field experimentation.
The soils of the experimental fields are sandy and characterized with low organic matter ranging from 5 to 10 g/kg. The average value of total available water in soil (TAW) taken as the difference between volumetric soil moisture at field capacity, θFC (0.179–0.188 cm3 cm−3), and wilting point, θWP (0.043–0.066 cm3 cm−3), was approximately 75 mm for a maximum potato root depth of 0.60 m. Before each planting date, approximately 17 t/ha of organic manure was supplied and 300, 300, and 200 kg/ha of nutrients N, P, and K, respectively, were applied. After the tuber initiation, 120 kg/ha of potassium nitrate was applied. These amounts are assumed based on farmer practices in the region. Planting of potato crops took place between September 1 and 20 for the contrasting seasons 2002–2004, 2007, 2010, 2012–2013, and 2020.
2.2 Crop and irrigation management
Different irrigation strategies were applied to test crop response to FI and DI by decreasing amounts of applied water in relation to maximum crop evapotranspiration (ETc). FI, taken as the control treatment, received 100% of accumulated ETc. Deficit treatments (DI80, DI70, DI60, DI40, and DI30) were irrigated at the same frequency as the control, but with quantities representing respectively 80%, 70%, 60%, 40%, and 30% of accumulated ETc. Farmer’s irrigation method (FM), which consists of applying water with fixed doses and intervals over the cropping season, was considered in some seasons in order to evaluate its performance.
For all treatments, irrigation was applied when soil water content in the root zone was depleted by the crop to a specific fraction of the total available water defined as readily available water, RAW (35% of TAW). The irrigation scheduling FI was determined based on the soil SWB method according to FAO guideline no. 56 (Allen et al., 1998). This model (SWB) consists of computing soil water depletion (Dr) on a daily basis and recommends irrigation when 35% of the TAW in the rootzone is depleted. The root depth starts with a value of 0.15 m at planting and increases linearly with the potato crop coefficient (Kc) up to 0.60 m.
Crop evapotranspiration (ETc) was estimated with a daily time step by using reference evapotranspiration (ETo) and the potato crop coefficient (Kc). The ETo was estimated from daily climatic data collected from meteorological stations, located near the experimental sites by means of the FAO-56 Penman-Monteith method given by Allen et al. (1998). The potato crop coefficient (Kc) was computed using the dual crop coefficient approach, considering the soil evaporation coefficient (Ke) and the basal crop coefficient (Kcb) that may be affected by the soil water stress coefficient (Ks). Such an approach provides a means to estimate transpiration and soil evaporation separately:
During the cropping period of potato, the values of Kcb, Ke, and Ks that are dependent on available soil water were computed following the procedure described in the FAO-56 guidelines (Allen et al., 1998).
A randomized complete block design was adopted with three replicates for each experiment. Each plot included four to six rows depending on the season. All plots were irrigated from a well with water having an ECiw ranging from 3 to 7 dS m−1 using 4 l/h drippers with an operating pressure of 1 bar. Each row was equipped with a dripline including an emitter spaced 40 cm. Each experimental field was equipped with a water meter and a gate valve in addition to a mini-valve for each row to control water supply (Figure 2).
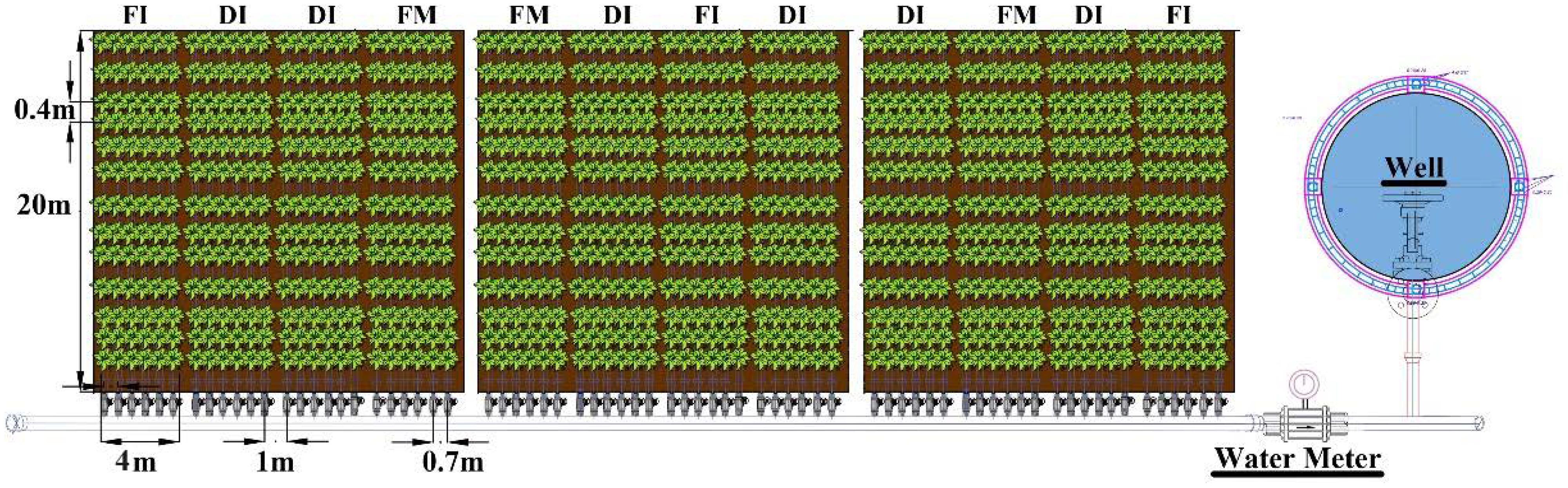
Figure 2. Experimental field design. FI represents the treatment based on the SWB method; FM, farmer method; DI, deficit irrigation (restriction treatments depend on the studied years).
2.3 Measured parameters
Soil samples were taken after harvest, for three depths below the emitter 0–20, 20–40, and 40–60 cm using a 4-cm auger. Samples were air-dried and ground to pass a mesh of 2 mm size and were analyzed to determine the electrical conductivity of saturated paste extract (ECe).
For each season, 20 plants per row within each plot were manually harvested between the last week of December and the first week of January to determine the fresh tuber yield (t/ha).
Irrigation water productivity (IWP, kg m−3) was defined as the yield (kg ha−1) obtained per unit of applied irrigation water (IWS, m3 ha−1) from planting to harvest and was calculated as follows:
Total water productivity (TWP) was defined the same way but considering total water supply (TWS) including both rainfall and irrigation.
In order to assess the effects of different irrigation treatments on crop yields, water productivity (WP), and soil salinity, analysis of variance (ANOVA) was performed using Statgraphics v.18 (www.statgraphics.com). Post-hoc analysis was carried out using the LSD test at a significance level of 5% to evaluate the differences between treatment means.
3 Results and discussion
3.1 Soil salinity
Figure 3 illustrates the salt accumulation in the soil, as indicated by the electrical conductivity of the soil-paste extract (ECe), measured at harvest across different irrigation water supplies (IWS) and seasons. For IWS values within the range of 250 to 350 mm corresponding to FI treatments with the SWB method, salt accumulation in the soil seems to be moderate with ECe values below 3 dS m−1. However, ECe values exceed 3 dS m−1 in some years as a result of using irrigation water with higher salinity levels (ECiw in the range 5.2–7.6 dS m−1). In contrast, deficit treatments led to higher salt accumulation, except for DI40 during the 2002 season characterized by high precipitation (72 mm) and relatively low ECiw (3.2 dS m−1). Additionally, DI70 appeared to benefit from heavy rain (85 and 112 mm) received, respectively, during cropping periods 2013 and 2020, despite the high salinity of irrigation water (6 dS m−1). The highest values of final ECe were observed for all treatments in 2012 as a result of the absence of rainfall events during the cropping season. The most restrictive irrigation method (DI30) exhibited the highest salt accumulation levels compared to the other deficit treatments and was significantly (p < 0.05) higher than the SWB treatment for the 2010 season (Table 1). In fact, the use of SWB techniques resulted in ECe values of 3.8 dS m−1 compared to DI30 with 5.4 dS m−1. However, this value remained lower than the ECiw, which could be attributed to the better distribution of rainfall during the cropping period for the 2010 season (61 mm). Toumi et al. (2024) indicated that salt accumulation for winter-season crops is significantly lower than that for summer-season crops. Their study, which focused on the seasonal variation of soil salinity under peach orchards cultivated in arid environments, underlines the impact of climatic conditions on salt accumulation dynamics. Therefore, the adoption of the DI30 strategy in dry seasons could negatively impact soil sustainability.
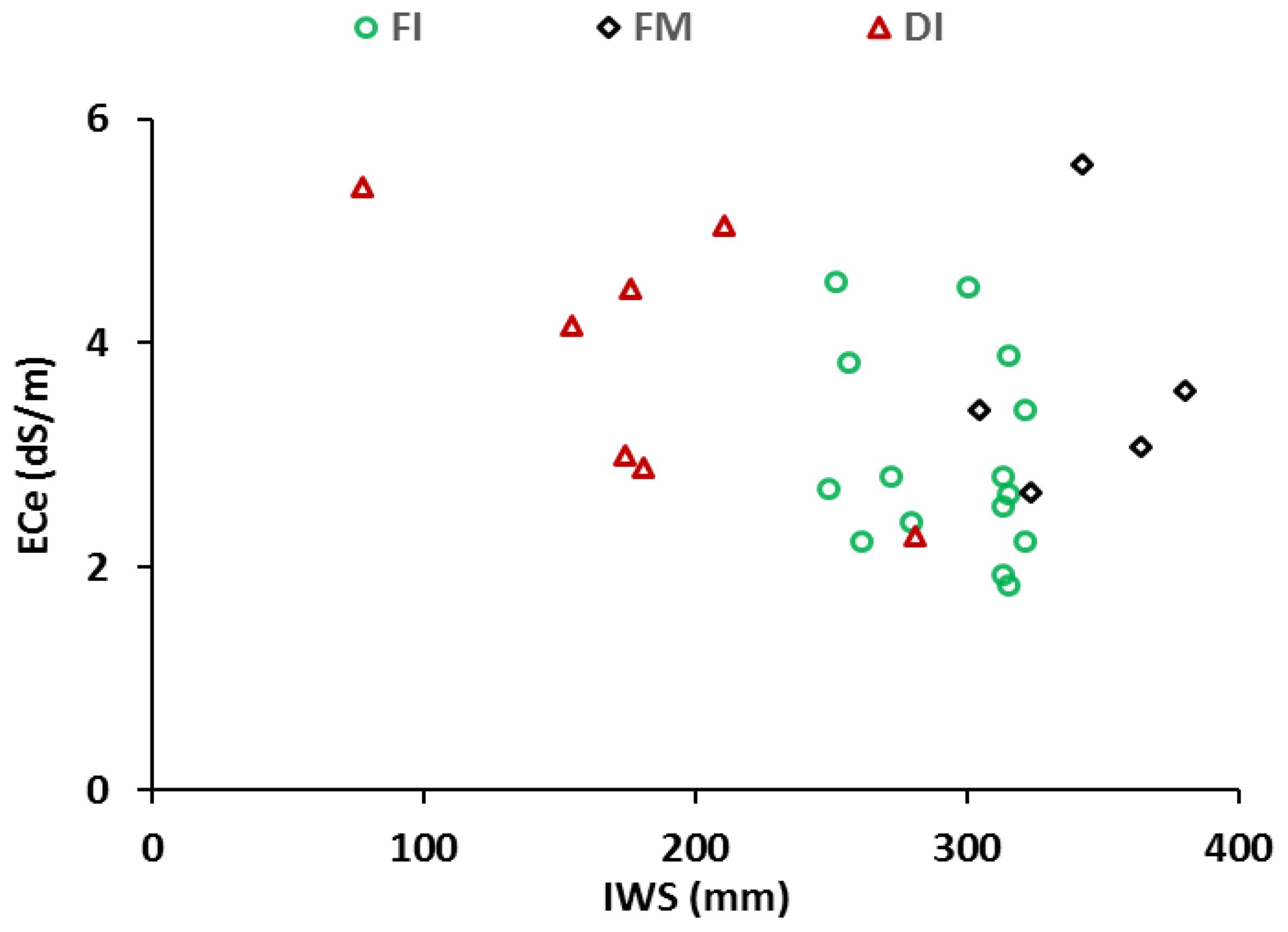
Figure 3. Soil ECe measured during potato harvest, irrigated with saline water in southern Tunisia, with full (FI), deficit (DI), and farmers’ (FM) strategies supplying different amounts of irrigation water (IWS) (2002–2020).

Table 1. Least significant difference test at 5% (LSD) of average treatment values under different seasons for ECe, yield, and WP.
The final ECe values under FM are comparable to and significantly higher (Table 1) for a certain season (2012) than those observed under the SWB method, despite the higher irrigation water applied (300–380 mm) using this method. Hence, the irrigation practices employed by farmers may not always align with the specific needs of the crops and the variations in climate and may cause salt accumulation in the root zone.
Based on these results, salt accumulation does not exhibit uniformity concerning irrigation supply or irrigation water quality in field conditions. Melgar et al. (2009) and Wiesman et al. (2004) have reported that salt accumulation is not solely dependent on irrigation water amount, but is influenced by various factors such as climate conditions, water salinity level, soil salinity, and characteristics. Rainfall occurrences within each season and their distribution notably influenced salt accumulation, particularly for the SWB method and moderate DI (DI80), which did not present a significant difference with FI (p < 0.05) (Table 1). For these treatments, even a low rainfall event during the cropping season seems to cause salt leaching.
The results reported by El Mokh et al. (2020) emphasized that under certain treatments, such as FI and moderate DI, there is a higher chance of leaching, and the ECe at harvest decreased compared to ECiw for carrot crop grown on sandy soil. This process is particularly observed in sandy soils, with high infiltration rates that allow freshwater to move easier in the soil profile and carry dissolved salts downwards. Yang et al. (2023) confirmed this phenomenon by demonstrating that the amount of water required for leaching in coarse soils, such as sandy soils, is less than that required in fine soils due to the lower capillarity force in coarse soils. Thus, even small rainfall events in sandy soils can effectively flush excess salts from the soil profile, helping to maintain soil fertility and productivity.
3.2 Crop yield
The findings indicate that higher potato yields, ranging between 20 and 30 t/ha, were achieved with the SWB method (FI) and DI involving the reduction of supply by 20% (DI80) and 30% (DI70) (Figure 4). Optimal crop productions (20–30 t/ha) were observed with an irrigation water supply ranging between 250 and 350 mm. However, yield variations within this range can be attributed to several factors, including initial soil salinity, water salinity, and primarily the amount of rainfall received during the cropping season. Indeed, potential yields exceeding 25 t/ha were achieved during seasons characterized by lower initial soil salinity and relatively low ECiw in most cases. Thus, ECe is accountable for the initial crop establishment. El Mokh et al. (2022b) indicated that the initial soil conditions, particularly salinity, have a pronounced effect on barley crop productivity in arid environments. However, for the 2010 season, starting with a relatively high initial ECe of 5.9 dS m−1 and with a relatively high ECiw of 7 dS m−1, reductions in potato yields were respectively 20%, 40%, and 60% under FI, DI60, and DI30, compared to the average yield of 25 t/ha. In fact, the FI treatment appeared to highly benefit from a 61-mm rainfall received during the cropping period. Yields produced under this treatment were significantly higher than those under DI60 and DI30 (p < 0.05) (Table 1). Along with the increased yields, the final soil salinity decreased to 3.8 dS m−1. This suggests that the combination of FI and rainfall helped to leach salts from the soil, reducing its salinity level. The tuber yields were inversely correlated with the final ECe values with a reasonable coefficient of determination r² of 0.60 (Figure 5).
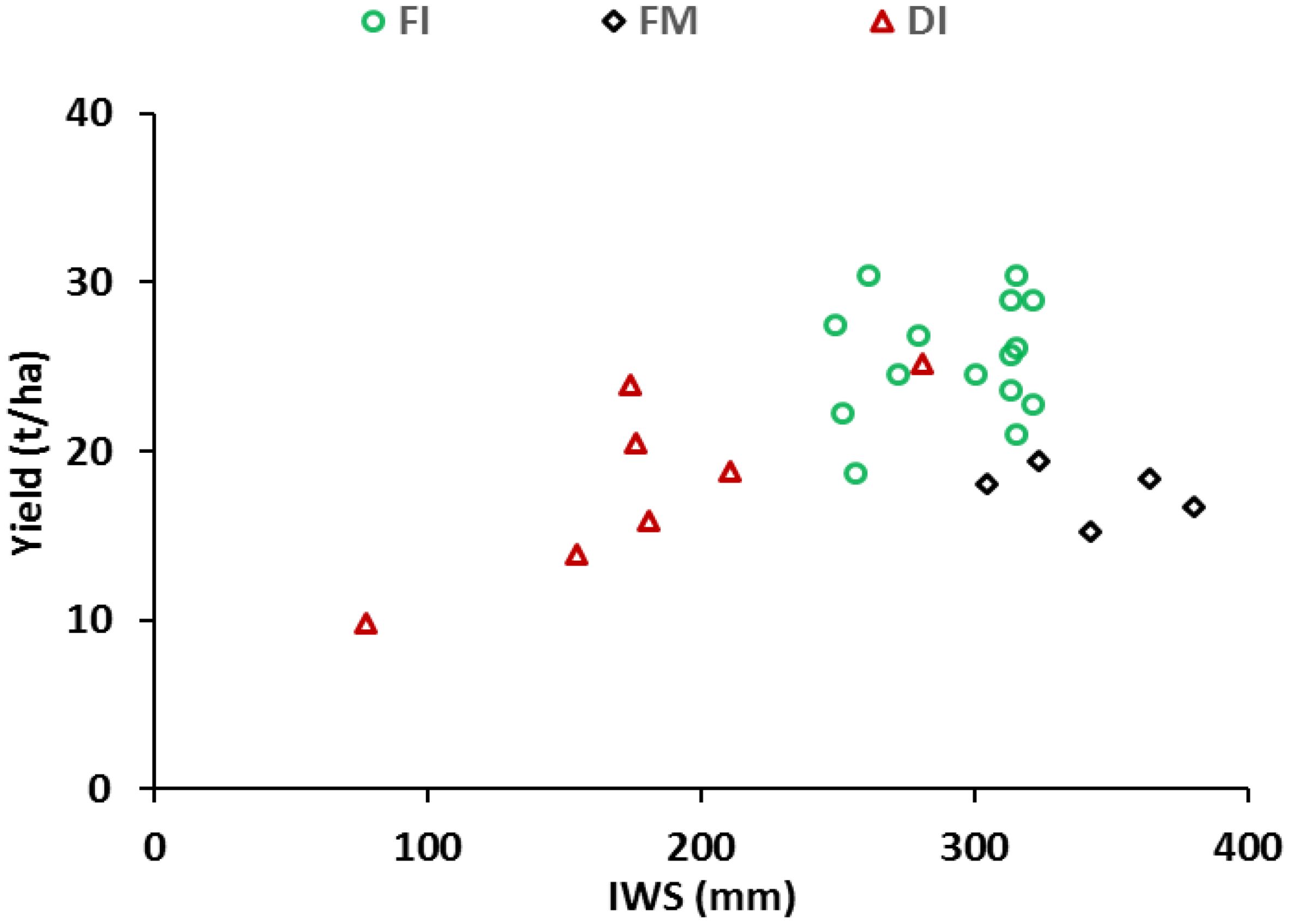
Figure 4. Yields of potato, irrigated with saline water in southern Tunisia, obtained under full (FI), deficit (DI), and farmers’ (FM) irrigation regimes supplying different amounts of water (IWS) (2002–2020).
Throughout most of the studied seasons, the final soil salinity values were lower than the initial ones except for the dry seasons. This outcome can be attributed to the interplay of numerous factors, including the adoption of drip irrigation. Several studies have emphasized the significance of employing drip irrigation for enhancing crop growth and yield compared to other systems such as furrow irrigation mainly in saline conditions (Nagaz et al., 2008; Jha et al., 2017).
The deficit treatments resulted in comparable yields to full treatments mainly for DI80 and DI70 and for the seasons that benefited from natural leaching. However, during dry seasons, it caused salt accumulation, particularly in the 2012 season, which is characterized by the absence of rainfall events that ensure natural leaching. On the other hand, with more water applied (300–380 mm) using the farmer’s method, the tuber yield was significantly reduced (Table 1). Interestingly, most farmers believed that adding more water would result in higher yields.
Thus, there might be a complex interplay between climate and soil conditions, water management, and crop responses that needs to be carefully considered to optimize yield. Exploring the specific conditions within different contexts would offer valuable insights into refining water application strategies, especially concerning the impact of DI.
3.3 Water productivity
IWP indicates the amount of crop yield produced per unit of irrigation water supplied (IWS). The amount of IWS during the potato cropping period ranges between 77 and 380 mm and TWS including rainfall ranges between 138 and 444 mm. The farmers’ practices resulted in the highest irrigation amounts, followed by the SWB method and the deficit treatment, which varied depending on restriction levels (Figure 6).
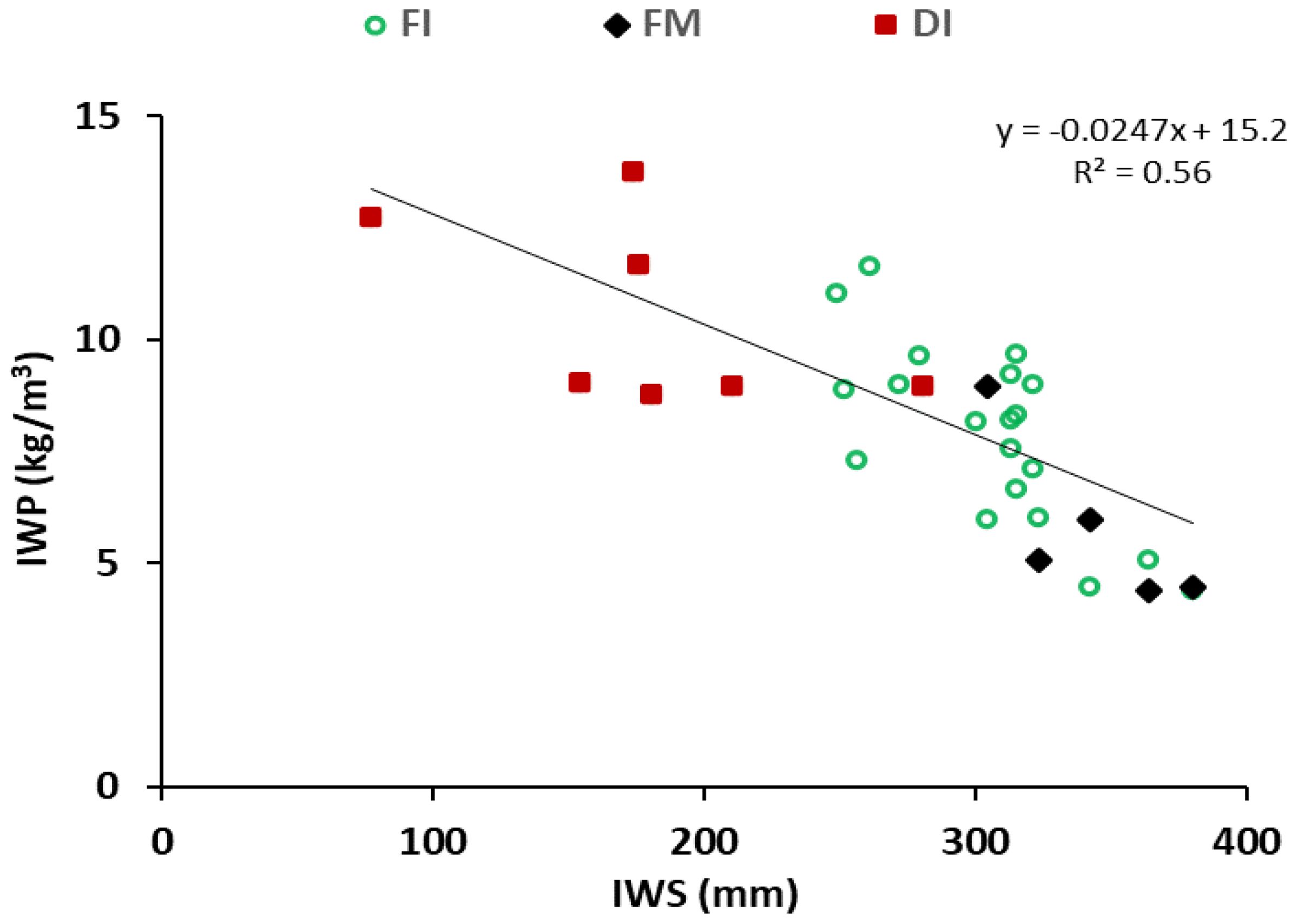
Figure 6. Irrigation water productivity of potato, grown in southern Tunisia under full (FI), deficit (DI), and farmers’ (FM) irrigation regimes supplying different amounts of irrigation water (IWS) (2002–2020).
Figure 6 shows that IWP is relatively correlated with irrigation water supply with a coefficient of determination r2 of 0.56. In fact, the relationship between water supply and water productivity is not linear as production is not possible under a minimum value of water supply. This is illustrated in Figure 7, which shows that TWP average levels decrease when total water supply is taken into account and that the envelop curve is parabolic. For potato cultivated during the autumn season, optimum values of TWP are in the range 7–9 kg/m3 obtained under FI and moderate DI regimes with a total water supply ranging from 250 to 350 mm.
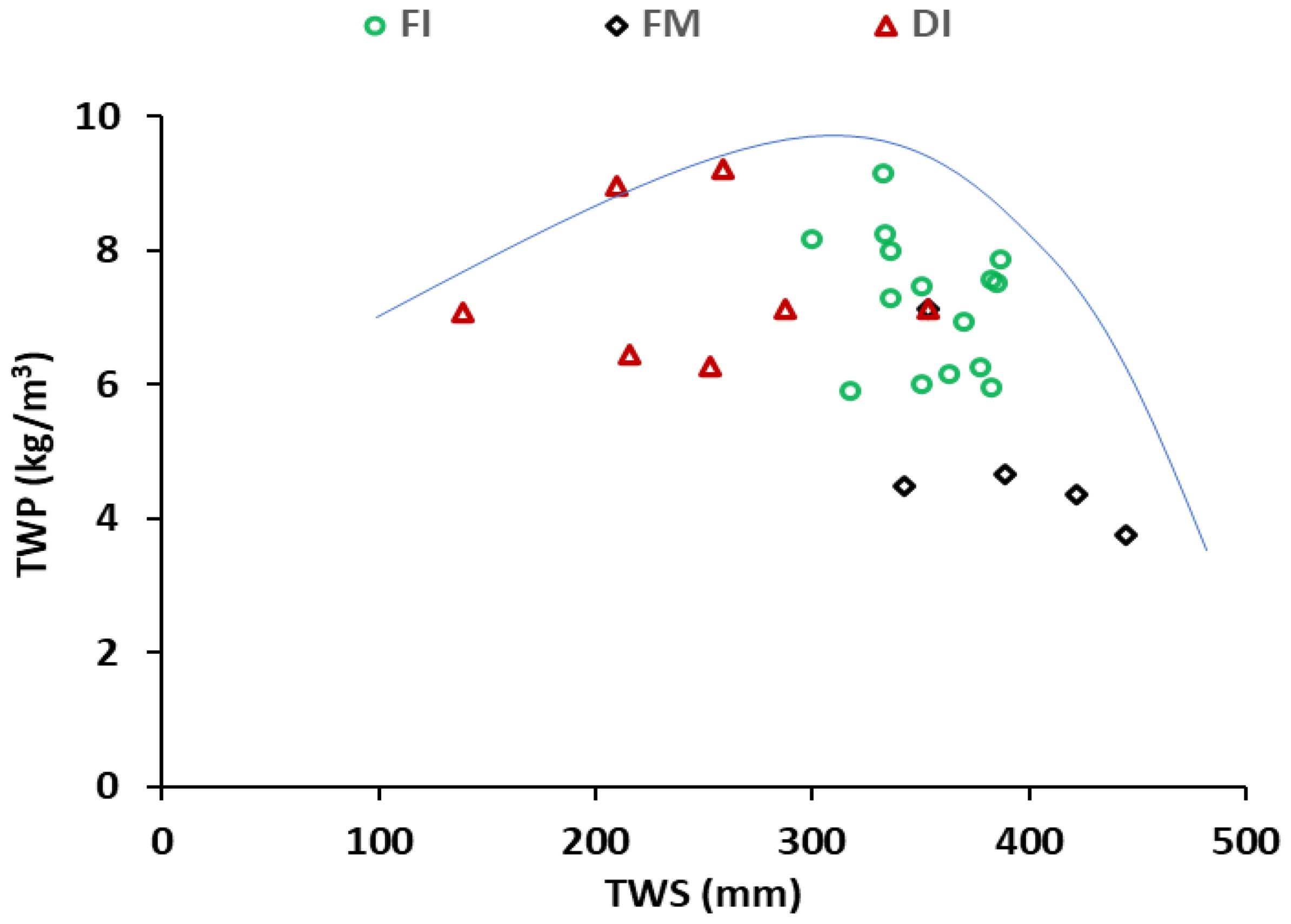
Figure 7. Total water productivity of potato, grown in southern Tunisia under full (FI), deficit (DI), and farmers’ (FM) irrigation regimes. TWS refers to total water supply by rainfall and irrigation (2002–2020).
DI led to a significant increase in IWP (Table 1), particularly in seasons where crop yields were less affected by water and salinity stresses, such as DI70 for the 2013 and 2020 seasons. These seasons received sufficient rainfall events, 85 and 112 mm, respectively, for 2013 and 2020, which potentially mitigated stresses during the sensitive growth periods of potato. Even though the DI30 treatment (season 2010) resulted in a considerable decrease in yield (p < 0.05), the IWP remained significantly high (Table 1). This is attributed to the fact that the reduction in yield (50%) was less than the 70% reduction in water supply. Consequently, while this treatment (DI30) ensured water savings of 179 mm, it resulted in relatively higher yield reduction and salt accumulation. The deficit treatments, including DI80, DI70, DI60, and DI40 applied during the 2002, 2010 and 2012 seasons, resulted in IWP values comparable to those of FI treatment, falling within the range of 8 to 10 kg/m3.
Under local conditions, the use of DI with severe water restriction (40% to 70%) could affect farmers’ income through the decrease of crop production and present a risk of salt accumulation in the root zone. The limited leaching associated with these techniques can intensify the process of soil salinization, further compromising land productivity and sustainability of the entire production system, whereas techniques with moderate water restrictions can serve as viable alternatives in terms of production, water conservation, and increased leaching, particularly during wet seasons. Many studies have advocated the use of these techniques under conditions of water scarcity and salinity variability (Ali et al., 2007; El Mokh, 2016; Alshami et al., 2023) and have demonstrated their beneficial effects on farmers’ net income. The higher amount of water applied by farmers (FM) resulted in lower values of water productivity. Under the same water resources availability, adoption of FI and DI by farmers will allow substantial water savings that can be used to irrigate more land and cultivate more crops. Farmers can therefore increase their income while limiting the risk of soil salinization through adequate management of water.
4 Conclusion
Irrigation scheduling plays a crucial role in enhancing crop water productivity and minimizing the environmental impact of saline water. The assessment of the effects of DI on production and soil salinity using long-term field experimentation (8 years) is investigated in this study. The results show a substantial yield improvement ranging between 30% and 40% using SWB techniques for all seasons compared to farmers’ practices. The application of moderate DI, with 20%–30% (DI80–DI70) reduction, caused small yield reduction ranging from 8% to 20% over the eight seasons. The application of moderate DI also led to a relatively small increase in soil salinity. However, the ECe values observed at harvest under DI remain comparable to those obtained with the SWB method. These irrigation strategies improved water productivity and can be a good alternative in similar conditions especially when used in rainy-season crops. They resulted in important water savings (10%–30%) and yield increase that can be used to improve farmer incomes.
Strategies involving severe reduction of irrigation water (60%–70%) (DI40–DI30) certainly increased IWP but resulted in significant yield reduction and increased soil salinity. The use of these strategies could impact sustainability of the vulnerable production system particularly in dry seasons where the effective leaching management with saline waters should be investigated. Current irrigation practices used by farmers, which consist of applying fixed doses over the season, resulted in lower productivity due to the higher water supply compared to the SWB method. In fact, the long-term use of these strategies may threaten the sustainability of the farmer’s field.
Based on this long-term study, we can note that seasonal rainfall has a crucial role in alleviating the impact of using saline water on crop and soil. Therefore, growing potato during the autumn season in order to align the cropping season with the rainy period can be an effective strategy that help mitigate salinity stress caused by irrigation with saline water. However, these practices remain highly dependent on climatic conditions particularly in the arid regions. Therefore, it is essential to assess their viability under future climate change scenarios.
Data availability statement
The original contributions presented in the study are included in the article/supplementary material. Further inquiries can be directed to the corresponding author.
Author contributions
FE: Writing – original draft, Writing – review & editing. KN: Writing – review & editing. MM: Writing – review & editing.
Funding
The author(s) declare financial support was received for the research, authorship, and/or publication of this article. Financial support was received from WADIS-MAR project funded by EU for the realization of field studies.
Acknowledgments
The authors would like to express their gratitude to the farmers for their collaboration and support during field experiment monitoring.
Conflict of interest
The authors declare that the research was conducted in the absence of any commercial or financial relationships that could be construed as a potential conflict of interest.
Publisher’s note
All claims expressed in this article are solely those of the authors and do not necessarily represent those of their affiliated organizations, or those of the publisher, the editors and the reviewers. Any product that may be evaluated in this article, or claim that may be made by its manufacturer, is not guaranteed or endorsed by the publisher.
References
Ahmed Z., Gui D., Murtaza G., Yunfei L., Ali S. (2023). An overview of smart irrigation management for improving water productivity under climate change in drylands. Agronomy 13, 2113. doi: 10.3390/agronomy13082113
Ali M. H., Hoque M. R., Hassan A. A., Khair M. A. (2007). Effects of deficit irrigation on yield, water productivity, and economic returns of wheat. Agric. Water Manage. 92, 151–161. doi: 10.1016/j.agwat.2007.05.010
Allen R. G., Perreira L. S., Raes D., Smith M. (1998). Crop evapotranspiration: Guidelines for computing crop water requirements. Irrigation and Drainage Paper N° 56 (Rome, Italy: FAO). 300p.
Alshami A. K., El-Shafei A., Al-Omran A. M., Alghamdi A. G., Louki I., Alkhasha A. (2023). Responses of tomato crop and water productivity to deficit irrigation strategies and salinity stress in greenhouse. Agronomy 13, 3016. doi: 10.3390/agronomy13123016
Ebrahimian H., Fujimaki H., Toderich K. (2023). Parameterization of the response function of sesame to drought and salinity stresses. Agriculture 13, 1516. doi: 10.3390/agriculture13081516
El Mokh F. (2016). Irrigation management with saline water in dry environment. Institut National Agronomique de Tunisie, Tunisia, 164.
El Mokh F., Nagaz K., Alva A. K., Masmoudi M. M., Ben Mechlia N. (2022a). Effect of irrigation regimes using saline water on faba bean (Vicia faba L.) yield and water productivity in an arid environment. Irrig. Drain 71, 81–93. doi: 10.1002/ird.2635
El Mokh F., Nagaz K., Masmoudi M. M., Ben Mechlia N. (2020). Saline water use for vegetable crops production in smallholders farms. J. Agric. Environ. Int. Dev. (JAEID) 114, 85–101. doi: 10.12895/jaeid.20202.1406
El Mokh F., Nagaz K., Masmoudi M. M., Mechlia N. B. (2017). Irrigation management to improve smallholder’s incomes for saline conditions. Int. J. Crop Sci. Technol. 3, 51–58. doi: 10.26558/ijcst.347891
El Mokh F., Nagaz K., Masmoudi M. M., Mechlia N. B. (2022b). Management practices assessment using Aquacrop model for growing barley under saline conditions of the arid regions of Tunisia. J. Anim. Plant Sci. 32, 1053. doi: 10.36899/JAPS.2022.4.0509
Fereres E., Soriano M. A. (2007). Deficit irrigation for reducing agricultural water use. J. Exp. Bot. 58, 147–159. doi: 10.1093/jxb/erl165
Fitsum T., Haimanote B., Bruce S., Yiannis A., Gerrit H., Aditya S. (2023). Exploring deficit irrigation as a water conservation strategy: Insights from field experiments and model simulation. Agric. Water Manage. 289, 108490. doi: 10.1016/j.agwat.2023.108490
Irfan M., Arshad M., Shakoor A., Anjum L. (2014). Impact of irrigation management practices and water quality on maize production and water use efficiency. J. Anim. Plant Sci. 24, 1518–1524.
Jha G., Choudhary O. P., Sharda R. (2017). Comparative effects of saline water on yield and quality of potato under drip and furrow irrigation. Cogent Food Agric. 3, 1369345. doi: 10.1080/23311932.2017.1369345
Maas E. V. (1990). “Crop salt tolerance,” in Agricultural Salinity Assessment and Management, ASCE Manuals and Reports on Engineering Practice No. 71. Ed. Tanji K. K. (American Society of Civil Engineers, New York), 262–304.
Melgar J. C., Mohamed Y., Serrano N., García-Galavís P. A., Navarro C., Parra M. A., et al. (2009). Long term responses of olive trees to salinity. Agri. Water Manage. 96, 1105–1113. doi: 10.1016/j.agwat.2009.02.009
Nagaz K., Masmoudi M. M., Ben Mechlia N. (2013). “Field studies on the use of saline water for deficit irrigation in arid Tunisia,” in Irrigation Management, Technologies and Environmental Impact. Ed. Ali M. H. (Nova Science Publishers, Inc., New York), 87–128.
Nagaz K., Toumi I., Masmoudi M. M., Ben Mechlia N. (2008). Comparative effects of drip and furrow irrigation with saline water on the yield and water use efficiency of potato (Solanum tuberosum L.) in arid conditions of Tunisia. Agric. J. 3, 272–277.
Shalhevet J. (1994). Using water of marginal quality for crop production: major issues. Agric. Water Manage. 25, 233–269. doi: 10.1016/0378-3774(94)90063-9
Shammout M. W., Qtaishat T., Rawabdeh H., Shatanawi M. (2018). Improving water use efficiency under deficit irrigation in the Jordan valley. Sustainability 10, 4317. doi: 10.3390/su10114317
Si J., Wang L., Zhang K., Li L., Fudjoe S. K., Luo Z. (2024). Irrigation as an effective way to increase potato yields in Northern China: A meta-analysis. Agronomy 14, 448. doi: 10.3390/agronomy14030448
Smith M. (1985). Irrigation scheduling and water distribution (Paris, France: Actes de Conférence Internationale sur Les besoins en eau des cultures, INRA), 497–514.
Toumi I., Ghrab M., Zarrouk O., Nagaz K. (2024). Impact of deficit irrigation strategies using saline water on soil and peach tree yield in an arid region of Tunisia. Agriculture 14, 377. doi: 10.3390/agriculture14030377
Wang H., Zheng C., Ning S., Cao C., Li K., Dang H., et al. (2023). Impacts of long-term saline water irrigation on soil properties and crop yields under maize-wheat crop rotation. Agric. Water Manage. 286, 108383. doi: 10.1016/j.agwat.2023.108383
Wiesman Z., Itzhak D., Ben Dom N. (2004). Optimization of saline water level for sustainable Barnea olive and oil production in desert conditions. Sci. Hortic. 100, 257–266. doi: 10.1016/j.scienta.2003.08.020
Xu Q., Dong X., Huang W., Li Z., Huang T., Song Z., et al. (2024). Evaluating the effect of deficit irrigation on yield and water use efficiency of drip irrigation cotton under film in Xinjiang based on meta-analysis. Plants 13, 640. doi: 10.3390/plants13050640
Keywords: potato, yield, saline water, deficit irrigation, water productivity, arid
Citation: El Mokh F, Nagaz K and Masmoudi MM (2024) Long-term potato response to different irrigation scheduling methods using saline water in an arid environment. Front. Agron. 6:1426034. doi: 10.3389/fagro.2024.1426034
Received: 30 April 2024; Accepted: 19 August 2024;
Published: 16 September 2024.
Edited by:
Hamed Ebrahimian, University of Tehran, IranReviewed by:
Zhen Wang, China Institute of Water Resources and Hydropower Research, ChinaJunya Onishi, Japan International Research Center for Agricultural Sciences (JIRCAS), Japan
Copyright © 2024 El Mokh, Nagaz and Masmoudi. This is an open-access article distributed under the terms of the Creative Commons Attribution License (CC BY). The use, distribution or reproduction in other forums is permitted, provided the original author(s) and the copyright owner(s) are credited and that the original publication in this journal is cited, in accordance with accepted academic practice. No use, distribution or reproduction is permitted which does not comply with these terms.
*Correspondence: Fathia El Mokh, ZWxtb2toLmZhQGdtYWlsLmNvbQ==