- 1Environmental Systems Graduate Group, University of California, Merced, Merced, CA, United States
- 2Department of Life and Environmental Sciences, University of California, Merced, Merced, CA, United States
Growing interest in human-excreta derived fertilizers requires more information on their agronomic relevance. In this study, we measured the nitrogen (N) and phosphorus (P) mineralization from fresh urine, stored urine, urine-enriched biochar prepared with either fresh or stored urine, and feces-derived compost application in a 90-day aerobic loam soil incubation. Soils were extracted for available N at days 0, 5, 10, 20, 30, 60, and 90, while soils were extracted for four biologically relevant P pools at days 0, 30, 60, and 90. We found that N in urine applied alone was immediately bioavailable, supplying nearly all the 200 kg-N ha-1 applied, while urine-enriched biochar supplied approximately half of the N applied. Feces-derived compost application led to a slow release of mineral N. Feces-derived compost application stimulated substantial native soil P mining, while urine-P was likely rapidly immobilized. These results are relevant to container-based sanitation and other source-separated sanitation endeavors, and researchers and producers interested in human excreta-derived fertilizers. Future research should explore, among other things, different urine-enriched biochar preparations and the co-application of urine-based fertilizers and feces-derived compost.
1 Introduction
The growing human population coupled with the exacerbating effects of agricultural practices on climate change point to the need for a paradigm shift towards more sustainable global nutrient management (Rockström et al., 2020). Additionally, the increasing scarcity, climate change impact, and price volatility of mineral fertilizers has increased interest in alternative approaches to fertilization (Crespi et al., 2022; Krein et al., 2023). Human excreta have long been considered as primarily a waste product, with treatment focused on reducing public and environmental health risks (Trimmer et al., 2019). However, we excrete most of the plant-essential nutrients that we consume once we reach adulthood (Jönsson et al., 2004). This means that large quantities of nitrogen (N), phosphorus (P), and potassium (K) are emitted with our waste, with a lack of widespread emphasis on resource recovery (Harder et al., 2020). Most of the N and approximately half of the P and K we excrete is present in urine. The remaining N, P, and K is present in feces, which is also rich in organic matter (Harder et al., 2019). Reuse of human excreta in agriculture was a practice common to most ancient cultures, which was largely lost with the Industrial and subsequent Green Revolution (Ashley et al., 2011). However, recent research and policy are shifting back towards circular approaches to sanitation and agriculture, often termed Ecological Sanitation (or EcoSan) (Langergraber and Muellegger, 2005). Many EcoSan technologies are concerned with the source separation of urine and feces, as a way to increase nutrient recovery efficiencies and reduce environmental impacts (Larsen et al., 2013).
An emerging application of EcoSan, largely practiced in low-income urban settlements in the Global South, is container-based sanitation. Container-based sanitation employs the separate collection of urine and feces in sealable containers, which are transported offsite for treatment and resource recovery (Russel et al., 2019). Thermophilic co-composting is a common approach to fecal waste management in container-based sanitation systems, as a way to create a nutrient-rich organic fertilizer that reduces pathogens and greenhouse gas emissions (Preneta et al., 2013; Ryals et al., 2019; McNicol et al., 2020). Source-separated urine can be applied alone as a fertilizer, with demonstrated equivalences to N-rich synthetic fertilizers (Martin et al., 2021). Urine nutrients can also be recovered through adsorption to biochar, the carbon-rich product of pyrolyzed biomass (Masrura and Khan, 2022). Soil application of biochar has the added benefit of soil carbon sequestration, a key climate change mitigation tool (Lehmann et al., 2006). While there is growing interest in the use of human excreta-derived fertilizers, there is a need for more information on their agronomic relevance. Specifically, understanding the mineralization of N and P from such fertilizers is crucial. Comprehensive insights into mineralization dynamics of any fertilizer are essential for recommending optimal application rates that maximize plant uptake and minimize environmental losses (Stanford, 1973). Recent research on human excreta-derived fertilizer mineralization has shed light on certain knowledge gaps (Kelova et al., 2021; Martin et al., 2021; Rumeau et al., 2023). However, it is important to study such fertilizers across various agroecological contexts to provide suitable recommendations for farmers.
In this study, we examined six human excreta-derived fertilizers relevant to container-based sanitation or other source-separated sanitation systems: fresh urine, stored urine (urea hydrolyzed), urine-enriched biochar (UEBC) prepared with either fresh or stored urine, and feces-derived composts (FDC) from two prominent container-based sanitation organizations. Our objective was to quantify the N and P mineralization of human excreta-derived fertilizers over the course of a typical 90 day cropping cycle length in an aerobic incubation method. We hypothesized that urine N and P would be immediately plant available from urine applied alone, and that that UEBC N and P would release slowly over the course of the experiment. We also hypothesized that N and P in FDCs would release slowly, with higher availability of P than N.
2 Materials and methods
2.1 Amendment preparation
A 90-day aerobic amended soil incubation was conducted to assess the N and P mineralization of six human-excreta derived amendments. Fresh and stored urine, two UEBC preparations, and two FDCs were tested. Each FDC was applied on a total N and potentially available N basis. An unfertilized control was also incubated, for a total of nine treatments. Each treatment was replicated three times.
Urine was collected from willing participants over age 18 (IRB number UCM2020-171), aggregated, and refrigerated at -4°C until use. Fresh urine had an electrical conductivity of ~11.4± 1.47 mS cm-1 and pH of ~6.77 ± 0.05, typical of fresh urine values (Ray et al., 2018) (Bischak et al., 2024). A sample of fresh urine was analyzed for ammonium (NH4+). Urease (CAS 9002-13-5, Fisher Scientific) was applied to a subsample of fresh urine at 0.533 g/L to determine urea content by analysis as NH4+. Fresh urine-N content was considered as the combined urea and NH4+ content. To prepare the stored urine, 0.533 g/L of urease was added based on methods used by (Ray et al., 2018), shaken for 30 minutes at 180 RPM, and left at 25°C in a sealed container until all urea was hydrolyzed. Urea hydrolysis was considered complete when the electrical conductivity stabilized at ~22.0 ± 1.67 mS cm-1 and pH at 9.37 ± 0.09, approximately one day later. Stored urine-N content was considered as NH4+ content, assuming all N was present as NH4+ after complete urea hydrolysis. Urine-N, in both stored and fresh urine, was determined by NH4+ analysis using the microplate colorimetric salicylate-nitroprusside method (Agilent BioTek Gen5 Microplate Reader, Agilent Technologies, Santa Clara, CA, USA).
Urine-enriched biochars were prepared with a walnut shell biochar pyrolyzed at 350°C (NextChar, Amherst, MA) mixed with fresh urine (hereafter Fresh UEBC), and stored urine (hereafter Stored UEBC). Urine and biochar were mixed at a 200 g biochar: 1 L urine ratio, allowed to saturate in a sealed container at room temperature for 48 hours, and drained freely over a 53 μm sieve. An effluent sample from each UEBC was filtered through a 0.45 μm polypropylene syringe filter for later analysis to determine the N adsorbed to biochar (Qe) using the following equation:
Where Qe is the mass of urine-N adsorbed to biochar (mg urine-N g-biochar-1), Co is the urine-N concentration in solution before adsorption (mg L-1) and Ce is the urine-N concentration in solution after adsorption (mg L-1), V is the volume of urine (L), and M is the mass of biochar (g). Urease was applied to a subsample of the Fresh-UEBC effluent to correct for urea content, which was negligible. The same equation was used to calculate P sorption to UEBCs.
Feces-derived composts were sourced from two prominent container-based sanitation organizations, Sustainable Organic Integrated Livelihoods (SOIL) in Cap-Haïtien, Haiti, and Sanergy in Nairobi, Kenya. Both SOIL and Sanergy use thermophilic co-composting to transform fecal matter into nutrient-rich compost while disinfecting fecal pathogens, a process in which compost piles are maintained at greater than 55°C for approximately ten weeks (Berendes et al., 2015; Tarpeh et al., 2023). The SOIL compost, Konpos Lakay, is hereafter referred to as SOIL Konpos Lakay. The Sanergy compost, Evergrow, is hereafter referred to as Sanergy Evergrow. Amended soils are hereafter referred to by the name of their treatment. For example, soil amended with fresh urine is referred to as Fresh Urine.
2.2 Amendment application and incubation
The amendments were mixed with a gravelly loam soil mapped in the Redding series (Fine, mixed, active, thermic Abruptic Durixeralfs). Relevant soil properties are shown in Table 1. Soil was collected from four sampling locations from 0-15 cm in Merced, CA, and stored at 4°C until use. Six 10 g soil subsamples were oven dried at 105°C to determine the initial moisture content. Soils were sieved to 4 mm to remove gravel prior to mixing with amendments. Fresh soil equivalent to 200 g oven dry soil was added to each 473 mL polypropylene incubation jar.
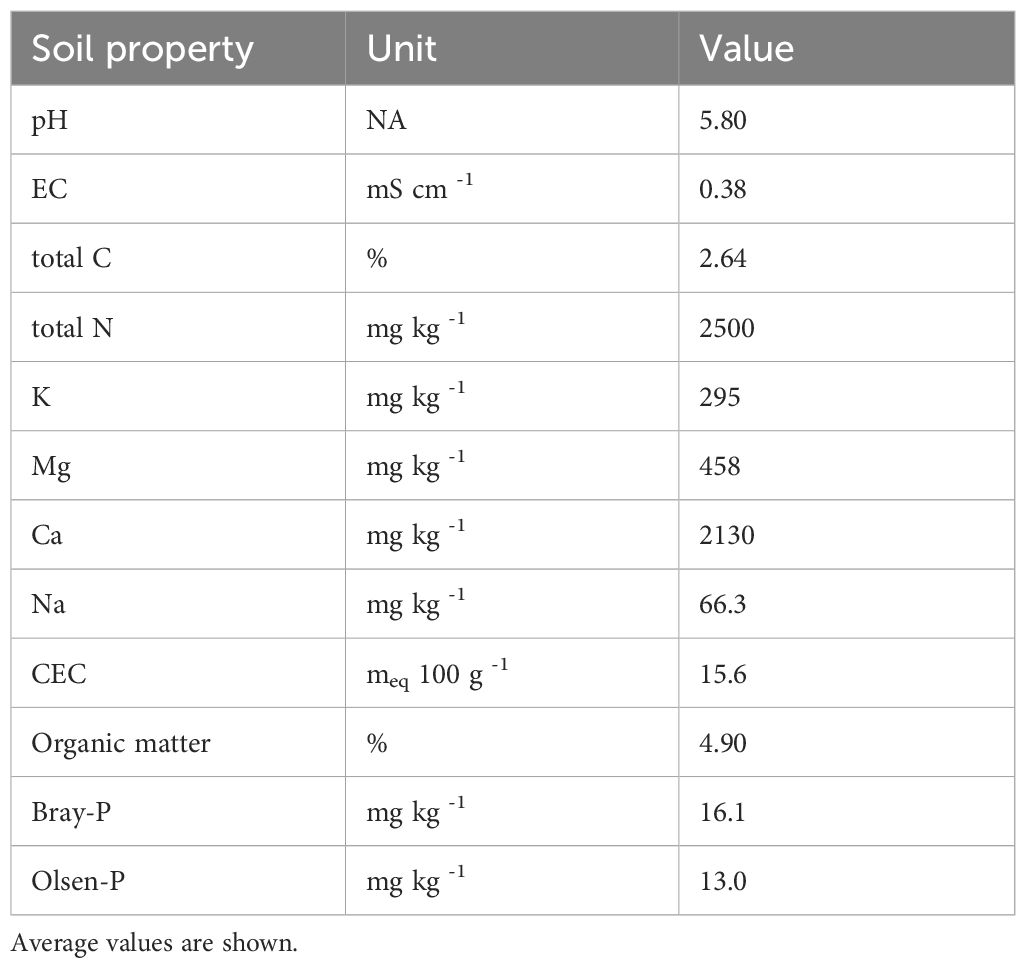
Table 1 Relevant properties of Redding gravelly loam soil collected from 0-15 cm in Merced, CA, USA.
All amendments were applied to soil at a rate of 200 kg-N ha-1. Each FDC was also applied at a rate of 2860 kg-N ha-1. This rate assumes that 7% of compost-N is bioavailable (Ryals et al., 2021), and is referred to hereafter as potentially available nitrogen (PAN). Feces-derived compost was otherwise applied on a total N basis. Approximately 14 times as much N and P were applied with FDC PAN treatments as with FDC total N treatments. The amount of N and P applied for each amendment are shown in Table 2. Since amendments were applied on an N basis, the P applied varies across amendments. Urine-enriched biochars were applied on a urine-N basis (Qe from Equation 1), assuming that biochar-N mineralization was negligible over the course of the study. Urine-only treatments were applied on a total N basis assuming all urine-N is available. Amendment application corrected for the moisture content of the given amendment. All soil + amendment mixtures were homogenized at the beginning of the experiment. Jars were adjusted to 60% of field capacity (approximately 22% moisture) with deionized water and maintained between 19% and 26% moisture throughout the study. Field capacity was determined by preparing three jars of approximately 200 g of soil. The initial soil moisture content was analyzed and the initial jar weight was noted. Jars were saturated with water until they began to leach. They were allowed to drain freely and weighed three days later to ensure leaching was finished. The percentage soil moisture at field capacity was considered as the difference between the initial and final weight divided by the final weight, multiplied by 100. Incubation jars had perforated lids to ensure adequate aeration. All jars were kept in the dark to prevent plant growth throughout the study. Each treatment was incubated in triplicate at 24°C.
2.3 Soil N and P extractions
Amended soils were extracted for available N (NO3- and NH4+) seven times throughout the study: on days 0, 5, 10, 20, 30, 60, and 90. We chose these times points to capture spikes in plant-available nitrogen shortly after amendment. Five grams of amended soil was shaken with 25 mL of 2M KCl for 1 hour at 200 RPM. Three KCl-only samples were prepared per extraction time point. Extracts were filtered using Whatman #1 filter paper. Samples were stored at -20°C until analysis for available NH4+and NO3- using the sodium nitroprusside and vanadium chloride spectrophotometric method, respectively (Mulvaney, 1996) (Agilent BioTek Gen5 Microplate Reader, Agilent Technologies, Santa Clara, CA, USA). The average absorbance from KCl-only samples was subtracted from analysis data to correct for background absorptivity, per time point.
Amended soils were also extracted for four biologically relevant pools of P using the biologically based-P (BBP) method (DeLuca et al., 2015) at four times during the study: on days 0, 30, 60, and 90. One gram of amended soil was shaken with 10 mL of each extractant. 0.01M Calcium chloride (CaCl2) was used to extract soluble and weakly adsorbed inorganic P, 0.01M citric acid (C6H8O7) was used to extract active inorganic P pool sorbed to clay particles or weakly bound in inorganic precipitates, 0.02 EU ml-1 phosphatase enzyme solution in 50 mM sodium acetate buffer was used to extract organic P readily attacked by acid phosphatase enzymes, and 1M hydrochloric acid (HCl) was used to extract soluble, active, and moderately stable inorganic P adsorbed to mineral surfaces or present in inorganic precipitates. These extractants are hereafter referred to as CaCl2, Citric Acid, Enzyme, and HCl, respectively. Three extractant-only samples were prepared per extractant and extraction time point. Extracts were shaken for 3 hours at 200 RPM and allowed to settle in a refrigerator at -4°C for 30 minutes. Extracts were filtered using Whatman #1 filter paper. Samples were stored at -20°C until orthophosphate analysis using the Malachite green method (Ohno and Zibilske, 1991). The average absorbance from extractant-only samples was subtracted from analysis data to correct for background absorptivity, per extractant and time point.
2.4 Statistical analysis and modeling
The percent of N mineralized was calculated as follows for each time point (Nmint), adapted from methods used by Lazicki et al. (2020):
The total mineral N extracted from the unfertilized control was subtracted from the total mineral N for a treatment at day t, divided by the total N applied for said treatment (Ntotal), and multiplied by 100. Nmint at day 0 represents the percent of N initially in mineral form. The same approach was used for P mineralized (Pmint) for each BBP reagent. For example, PmintHCl was calculated as follows:
All statistical analyses were performed in R Statistical Software (v 4.1.3; R Core Team, 2022). Linear models were used to compare the amount of each extracted analyte at each extraction date using the lm function. Pairwise Tukey’s Honestly Significant Difference (Tukey HSD) post hoc comparisons between treatments at each extraction date were performed using the emmeans function in the emmeans package. The same statistical analyses were performed on Nmint and Pmint data. Standard error of Nmint and Pmint by time point was propagated using the crossing function in R to calculate the uncertainty space for all combinations of N or P mineralizedtreatment - N or P mineralizedcontrol. All references to statistical significance consider p< 0.05. As most discussion of statistical significance is of multiple pairwise comparisons, exact p values are not reported in the main text.
3 Results
3.1 Nitrogen
Net N mineralization varied over time and between treatments (Figure 1; Supplementary Table S1). Most N mineralization occurred within the first five days for all treatments. Stored Urine and Fresh Urine treatments had the highest amounts of extracted mineral N in this period, followed by UEBCs, and composts applied on a PAN basis. For much of the 90 days, extractable N from compost amended soils did not differ significantly from unfertilized controls, aside from initially available mineral N from PAN composts and a significant N release from total N composts on day 60. Soil mineral N accumulation was not observed during the experiment, implying N volatilization, N immobilization in microbial biomass after mineralization, and/or N sorption to organic matter. The bulk of the extracted mineral N from all amendments was NH4+ rather than NO3- (Supplementary Figures S1, S2). Nmint is calculated with Equation 2. The statistics for Nmint remain largely the same as for the extracted mineral N data (Supplementary Table S2; Supplementary Figure S3). The exception is Nmin0 for composts applied on a PAN basis, which do not differ significantly from the control due to the large amount of N applied. We chose not to express our results as cumulative N mineralized, since we cannot be certain what mineral N was newly mineralized from one extraction day to the next, what N was volatilized, and what N remained in the system immobilized in microbial biomass.
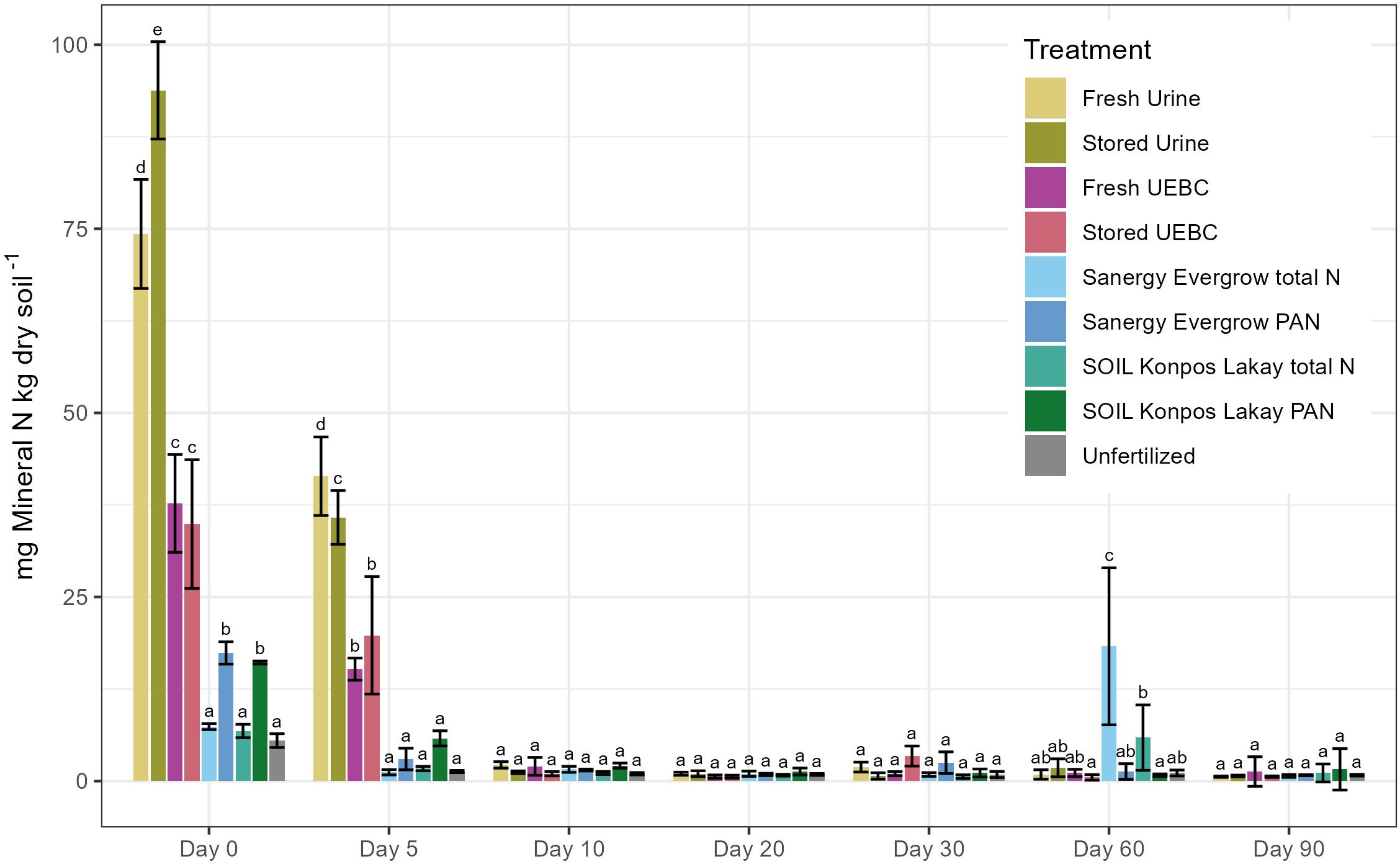
Figure 1 Extracted mineral N of human excreta-derived fertilizer amended soils over the course of a 90-day aerobic incubation. Letters show significant differences from a post hoc Tukey’s HSD test at p< 0.05 between treatments for each extraction date. If treatments share a letter, they do not differ significantly. Error bars represent ± standard deviation (n = 3). These data are also expressed in Supplementary Table S1.
On day 0, 93.8 ± 6.61 mg mineral-N kg dry soil -1 was extracted from Stored Urine, significantly more than any other treatment. This accounts for approximately 88% of the N applied (Supplementary Figure S3). The second-most was extracted from Fresh Urine, at 74.3 ± 7.39 mg mineral-N kg dry soil -1, or approximately 69% of the N applied, significantly higher than all other treatments. Fresh UEBC and Stored UEBC treatments were significantly lower on day 0 than urine-only treatments and significantly higher than all FDC treatments and the unfertilized control, with 37.7 ± 6.64 mg mineral-N kg dry soil -1 (approximately 32% of N applied) and 34.9 ± 8.74 mg mineral-N kg dry soil -1 extracted (approximately 29% of N applied), respectively. 16.1 ± 0.21 mg mineral-N kg dry soil -1 (approximately 0.7% of N applied) and 17.4 ± 1.52 mg mineral-N kg dry soil -1 (approximately 0.8% of N applied) were extracted from SOIL Konpos Lakay PAN and Sanergy Evergrow PAN, respectively. SOIL Konpos Lakay total N and Sanergy Evergrow total N did not differ significantly from the unfertilized control at day 0. Significantly more NO3- was extracted from the unfertilized control than all treatments except Fresh and Stored Urine on day 0, indicative of an initial NO3- depression period (Supplementary Figure S2).
On day 5, Fresh Urine mineralized significantly more N than all other treatments, with 41.4 ± 5.33 mg mineral-N kg dry soil -1 extracted, approximately 40% of N applied. Store Urine had the second most, with 35.8 ± 3.65 mg mineral-N kg dry soil -1 extracted (approximately 35% of N applied), significantly higher than all other treatments. This is followed by UEBCs, significantly higher than all FDCs and the unfertilized control, with 15.2 ± 1.51 mg mineral-N kg dry soil -1 extracted (approximately 14% of N applied) for Fresh UEBC and 19.8 ± 7.98 mg mineral-N kg dry soil -1 (approximately 19% of N applied) for Stored UEBC.
From days 10-30, extractable mineral N declined across treatments. No treatments differed significantly from each other or the unfertilized control on days 10, 20, or 30. A significant mineral N release was observed for SOIL Konpos Lakay and Sanergy Evergrow total N on day 60 compared to all other treatments. 18.3 ± 10.66 mg mineral-N kg dry soil -1 (approximately 17% of N applied) was extracted from Sanergy Evergrow total N, and 5.9 ± 4.44 mg mineral-N kg dry soil -1 (approximately 5% of N applied) from SOIL Konpos Lakay total N. No treatments differed significantly on day 90.
3.2 Phosphorus
Phosphorus mineralization also varied over time and between treatments (Figure 2; Supplementary Table S3). The most P was generally extracted with HCl for all treatments and time points, followed by Citric Acid, Enzyme, and CaCl2. HCl extracted-P increased, and Enzyme extracted-P decreased over time for SOIL Konpos Lakay PAN and Sanergy Evergrow PAN. There is a spike of CaCl2 extractable-P for FDCs applied on a PAN basis at day 60. Significantly more P was extracted from PAN FDCs compared to all other treatments at nearly every time point for each P extractant (Figure 2; Supplementary Table S3).
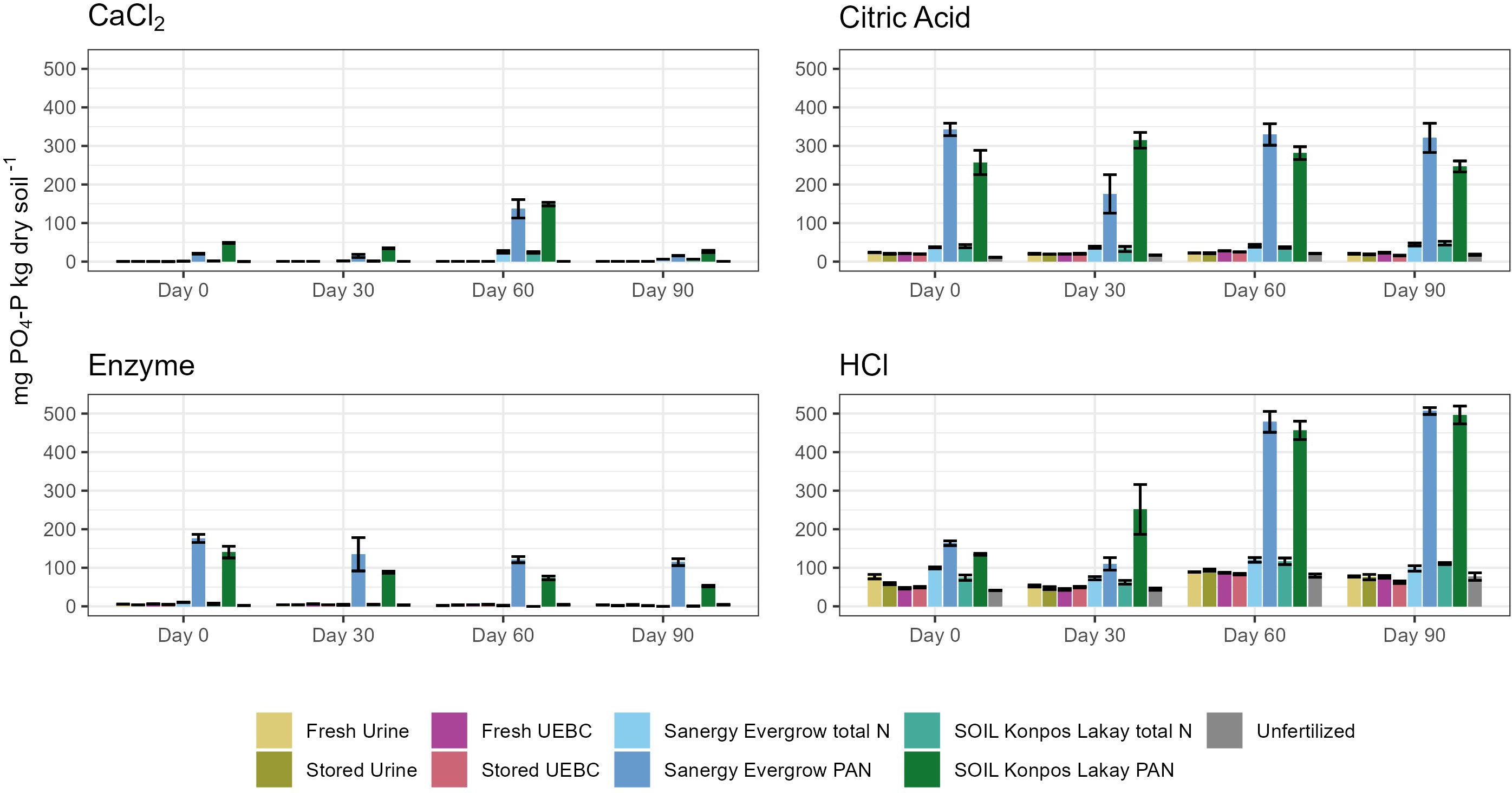
Figure 2 Extracted PO4-P of human excreta-derived fertilizer amended soils over the course of a 90-day aerobic incubation. Error bars represent ± standard deviation (n = 3). These data and significant differences between treatments by extraction date and extractant type are shown in Supplementary Table S3.
The most significant differences between treatments were observed for HCl-extracted soils (Supplementary Table S3). On day 0, HCl Sanergy Evergrow PAN was significantly higher than other treatments, with 164 ± 15 mg PO4-P kg dry soil -1. HCl SOIL Konpos Lakay PAN was significantly higher than Stored Urine, Fresh UEBC, and Stored UEBC, and the control, with 135 ± 5 mg PO4-P kg dry soil -1. On day 30, significantly more P was extracted from HCl SOIL Konpos Lakay PAN than any other treatment, with 252 ± 158 mg PO4-P kg dry soil -1. HCl Sanergy Evergrow PAN, saw 110 ± 40 mg PO4-P kg dry soil -1 extracted, which was significantly higher than Stored Urine, Fresh UEBC, and the control. On day 60 the HCl PAN FDCs were significantly higher than all others, with an average of 468 mg PO4-P kg dry soil -1 extracted for both. The same trend held for day 90, with an average of 501 mg PO4-P kg dry soil -1 extracted for both. For the CaCl2, Citric Acid, and Enzyme extractions, the PAN FDCs treatments were the only treatments that differed significantly from the control, which was true at each extraction date.
Pmint is calculated with Equation 3. The statistics for Pmint remain largely the same when compared to the extracted mineral P data (Supplementary Table S4; Supplementary Figure S4). PmintHCl Fresh urine is not statistically significant at any date, due to the relatively large amount of P applied compared to P extracted. However, all FDCs treatments are significantly higher than other treatments at all dates for all BBP extractants, due to the relatively small amount of P applied compared to large amount of P extracted (Supplementary Table S4). Substantial mining of native soil P is evident for these treatments. This was most evident for total N composts, as a small amount of P was applied (0.34 mg PO4-P kg dry soil -1 for SOIL Konpos Lakay and 0.19 mg PO4-P kg dry soil -1 for Sanergy Evergrow), and a comparatively large amount of P was extracted. As for the N data, we chose not to express our results as cumulative P mineralized, since we cannot be certain what mineral P was newly mineralized from one extraction day to the next, and what P was immobilized in microbial biomass as the BBP method does not account for microbial biomass P (DeLuca et al., 2015). As there is also overlap between the P extraction pools (DeLuca et al., 2015), we cannot sum them to indicate total P availability of each amendment.
4 Discussion
4.1 Nitrogen availability
4.1.1 Urine only
Our results show that N is more available from urine-derived fertilizers compared to FDCs. Extracted mineral N was much higher for urine-derived fertilizers, with the highest from Stored Urine in the first five days of the experiment (Figure 1). The lack of soil mineral N accumulation over the course of the study for urine-only treatments implies that N was either volatilized, sorbed, mineralized and subsequently immobilized, or leached. Because soils were maintained at field capacity and not allowed to drain, the possibility of leaching can be excluded.
Nitrogen volatilization as NH3 has been shown to be high from urine application as evidenced by Martin et al. (2023), who found that stored urine applied at a rate of 145 kg-N ha -1 to the surface of a loamy haplic Luvisol resulted in 34% N volatilization of the total urine-N applied under field conditions. Rumeau et al. (2023) modeled NH3 volatilization from stored urine applied at 170 kg-N ha−1 to a calcareous loamy clay soil and found that between 57% and 67% urine-N applied would be lost to NH3 volatilization. The lack of substantial NO3- accumulation from urine-only treatments (Supplementary Figure S2) implies potentially high volatilization of NH3 from Fresh Urine and Stored Urine in our study. Effort should be made when applying urine alone as a fertilizer to prevent volatilization. The Rich Earth Institute, a prominent urine nutrient recovery research institute, recommends applying urine in a furrow and covering it with soil, tilling after application, irrigating after application, or diluting urine before application (Rich Earth Institute, 2019). Urea hydrolysis appeared to happen within the first five days of the experiment for Fresh Urine. Stored Urine had the highest extracted mineral N at day 0, and Fresh Urine the highest at day 5. These results imply a brief period of urea hydrolysis mediated by soil microbes, by which Fresh Urine urea-N is hydrolyzed to NH4+-N. This is consistent with literature on urea hydrolysis, which is shown to be a rapid, first-order reaction in soils (Chin and Kroontje, 1963).
It is possible that mineral urine-N that was not volatilized was immobilized in microbial biomass for urine-only treatments. Ma et al. (2021) found preferential immobilization of fertilizer NH4+-N in microbial biomass compared to fertilizer NO3–N, particularly for treatments with low carbon availability, in an incubated agricultural Andosol with a pH of 6.55. As most urine-N in our study was in ammoniacal form, and negligible carbon was added with urine-only treatments, some urine-N may have been similarly immobilized. Christie and Wasson (2001) found similarly low immobilization rates of NH4+-N without carbon addition in an incubated clay loam grassland soil with a pH of 6.0, which they attribute in part to non-microbial fixation of NH4+-N as nonexchangeable NH4+-N. Thus, NH4+-N fixation is another possible explanation for the lack of mineral N accrual over the course of our study. It is likely that NH4+-N from urine-derived fertilizers in our study may have sorbed to clay minerals, rendering it non-extractable with a KCl solution (Mulvaney, 1996). Urine-NH3 may have also chemisorbed to soil organic matter in either of the urine-only treatments, following urea hydrolysis in the Fresh Urine treatment (Nommik and Vahtras, 1982; Johansson, 1998).
4.1.2 Urine-enriched biochar
Urine-enriched biochar-N was most available within the first five days of our aerobic incubation, though the activity of plant roots would likely stimulate further desorption and thus bioavailability in planted soils. A slight slow-release effect was observed for Stored UEBC, with a small, significant amount of extractable NO3- on day 30 (Supplementary Figure S2). While some of the aforementioned N fates may be the same for the UEBCs as for the urine-only treatments, there are also likely some differences. The negligible urea content in Fresh-UEBC effluent suggests urea hydrolysis happened during the 48-hour UEBC saturation period. This implies that urine-N in Fresh UEBC likely sorbed as NH4+ (after hydrolysis) and urea (before hydrolysis). Previous research demonstrates the entrapment of urea molecules in biochar pores in N-enriched biochars (Bakshi et al., 2021; Castejón-del Pino et al., 2023). This urine-urea-N may have been desorbed, hydrolyzed by microbes, and microbially assimilated or adsorbed to clay or organic surfaces over the course of the incubation. It also could have remained entrapped in the biochar pores, and thus microbially inaccessible. If urea-urine-N remained entrapped in biochar pores, it could explain the lack of any significant slow release behavior in Fresh UEBC compared to Stored UEBC.
As urea hydrolysis was catalyzed with urease for the stored urine used to prepare the Stored UEBC, all urine-N in this treatment can be assumed as NH4+. Cai et al. (2016) demonstrated NH4+ desorption ratios lower than 10% for biochar pyrolyzed at 200°C and 300°C, and a desorption ratio of around 30% for biochar pyrolyzed at 400°C, for biochars produced from corn cob, pomelo peel, and banana stalk. Our walnut shell biochar was pyrolyzed at 350°C, suggesting a NH4+ desorption ratio potentially similarly low. This would explain the nonsignificant extractable mineral N for both Stored and Fresh UEBCs after day 5. Regardless, this dynamic would likely differ in the presence of plant roots, as roots may stimulate desorption from N-enriched biochar fertilizers by building ion gradients (Rasse et al., 2022). This explains the previously observed slow-release effect of walnut shell UEBCs when used as fertilizer for tomatoes (Bischak et al., 2024).
It is also possible that mineral N extracted from UEBC treatments at days 0 and 5 was from urine absorbed in large biochar pores or floating in the biochar slurry. The UEBC was drained over a 0.53-μm sieve to retain small biochar particles, based on prior research that demonstrated the importance of small biochar particles to retain urine-N (Bischak et al., 2024). This small sieve size created a wet UEBC product that was more difficult to handle than our prior preparations which used a 500-μm sieve (Bischak et al., 2024). The wetness of the UEBCs may have contributed to some NH3 volatilization from UEBC treatments as well. Further research on UEBC should seek to optimize the retention of urine-N in UEBC, while making it practical and safe to handle. It is important to note that while we did not see a large slow release effect with UEBC, its application may lead to soil N accrual and subsequent release with repeated applications, or release N when the biochar weathers (Haider et al., 2020). As noted with urine-only treatments, the lack of mineral N accrual in UEBC treatments could be due to microbial immobilization or binding to clay or organic surfaces.
4.1.3 Feces-derived composts
Nitrogen was less available from FDCs compared to urine-derived fertilizers. On day 0, the third-most mineral N was extracted from PAN FDCs, while total N FDCs did not differ significantly from the control. From days 5 – 30, mineral N extracted from all compost treatments did not differ significantly from the control (Figure 1). This is likely due to either the N in FDC being relatively stable, and thus not readily decomposed, or N mineralization and subsequent volatilization, sorption to organic matter, and/or sorption to clay minerals as discussed in previous sections. Kelova et al. (2021) found low N mineralization rates after 90 days of soil incubation for a mixture of fecal matter, sanitary bark, urine, and water composted at 38°C, with a 2.6% increase in extractable mineral N. The C:N ratio of the FDCs also likely explains the lower N mineralization when compared to urine-derived fertilizers. Sanergy Evergrow had a C:N of 20, while SOIL Konpos Lakay had a C:N of 6.7 (Table 2). Though these are quite different ratios, they both supplied more labile organic matter than the urine-derived fertilizers. The C content of urine alone is negligible, and while the C content of the UEBCs was quite high, biochar C is generally assumed to be marginally labile (Mašek et al., 2013). The significant release of NH4+ from both total N FDCs on day 60 is likely due to the well-documented slow release nature of compost as a nitrogen fertilizer (Amlinger et al., 2003). These results are also consistent with Kelova et al. (2021), who saw a notable N release from FDCs after 60 days. Though the extractable mineral N was low from FDC treatments in our study, a single compost application is shown to increase soil N years after addition (Ryals et al., 2014), and increase soil N availability (Sullivan et al., 2003).
Over the course of the study, the amount of extracted mineral N from PAN FDCs was not proportional to the amount of N applied (Supplementary Table S5). Since we assumed 7% of the N in compost was bioavailable, PAN FDCs supplied more than 14 times the amount of N as total N FDCs. However, on day 0, approximately 2.4 times more N was extracted from the PAN FDCs compared to the total N FDCs (Supplementary Table S5). At no point throughout the incubation did the N release from PAN FDCs become proportional to the amount of N applied. This may be due to N mineralization and subsequent sorption to organic matter, as the PAN FDCs supplied a large amount of organic matter. Our results suggest that less than 7% of FDC-N is initially bioavailable for total N FDCs, as Nmint did not exceed 2% until day 60 when Nmint was 17.2% for Sanergy Evergrow, and Nmint was 4.8% for SOIL Konpos Lakay. Nmint did not exceed 1% for PAN FDCs at any time point (Supplementary Table S2). Negative Nmint values at various time points for most FDCs treatments also suggest some immobilization of FDC-N throughout the growing season (Supplementary Table S2).
4.2 Phosphorus availability
4.2.1 Urine only and urine-enriched biochar
Our results show that FDCs stimulate more P mineralization than urine-derived fertilizers, even though more P was applied with urine-derived fertilizers. The most P was applied with UEBCs, with 9.11 mg PO4-P kg dry soil -1 for Fresh UEBC and 6.64 mg PO4-P kg dry soil -1 for Stored UEBC. However, only Stored UEBC was significantly higher than the control at day 30 for HCl extracted soils (Supplementary Table S3). Fresh UEBC never differed significantly from the control for any date or extractant (Supplementary Table S3). This implies that though urine-P was concentrated in UEBCs compared to urine applied alone, urine-P desorption was negligible. The Qe values for P were low compared to Qe for N (Supplementary Table S6). Low P sorption to biochar is consistent with other studies, likely due to negatively charged biochar surface functional groups (Hale et al., 2013; Takaya et al., 2016).
A relatively large amount of P was also applied with Fresh and Stored Urine alone, with 5.57 mg PO4-P kg dry soil -1 and 3.19 mg PO4-P kg dry soil -1, respectively. However, extracted P for either treatment never differed significantly from the control for any extractant or extraction time point (Supplementary Table S3). This implies that urine-P may be rapidly assimilated into microbial biomass. The BBP method notably does not extract for microbial biomass P (DeLuca et al., 2015), meaning that this P pool is not accounted for in our study. Inorganic phosphate comprises nearly all of the P in urine (Bonvin et al., 2015). Rapid microbial inorganic P uptake (within the first 24 hours of an isotope dilution experiment) in permanent grassland soils has been demonstrated (Bünemann et al., 2012). Day 0 extractions were performed within 24 hours of amendment application, which may account for the apparent rapid immobilization of urine-P. The soil used in our study was collected from a naturalized grassland managed for cattle grazing in Merced, CA.
While applied urine-P may have been rapidly immobilized, a potential explanation for low levels of extractable P from Stored Urine is that while high P concentrations were analyzed from small, well-mixed urine samples, only a small amount of urine-P may have actually been applied. Phosphorus precipitation as struvite is well documented as being triggered by urea hydrolysis in stored urine, and can reach maximum concentrations in a few hours (Udert et al., 2003). These precipitates settle to the bottom of a container (Tilley et al., 2008), and thus may have not been present in representative quantities in the pipette tip when urine was applied (though the container was also well-mixed before application). From a practical standpoint, this makes recovery of P-rich precipitates difficult when applying stored urine alone as a fertilizer. Different recovery pathways should be prioritized to recover P from stored urine, such as struvite precipitation with magnesium addition, sorption, or membrane separation (Harder et al., 2019).
4.2.2 Feces-derived composts
The high Pmint values (Supplementary Table S4; Supplementary Figure S4) indicate FDC application led to large stores of native soil P becoming available during the incubation. These data indicate that even small applications of FDC simulate mineralization of soil P reserves. While the Olsen-P and Bray-P tests used to evaluate the soil P status indicated moderate native soil P availability (Table 1), these tests may underestimate soil P status, particularly in previously fertilized soils (Barrow et al., 2022). Both Citric Acid and HCl extracted more P from unfertilized soils at day 0 than the Olsen-P or Bray-P values (Figure 2; Supplementary Table S3). The pool isolated with these extractants is P adsorbed to clay minerals or present in inorganic precipitates (Crain et al., 2018). Our soil was acidic, with an initial pH of 5.8 (Table 1). Acidic soil conditions tend to favor P precipitation or adsorption to iron or aluminum (Doydora et al., 2020). It is also possible that P was precipitated as hydroxyapatite, due to the large amount of calcium native to the soil (Table 1) (Doydora et al., 2020). The organic matter content of FDCs, in combination with the relatively high organic matter content of 4.9% of our soil (Table 1) (Hiederer and Köchy, 2011), may have stimulated soil P mining. There is a demonstrated positive correlation between soil organic matter content and P availability, suggesting that organic matter molecules may prevent P diffusion into micropores and thus increase P availability (Hawkins et al., 2022; Vermeiren et al., 2022). This may explain the high Pmint values for all FDCs (Supplementary Table S4), and the significant amount of extracted available P for PAN FDCs across extractants and time points (Figure 2; Supplementary Table S3). We also observed a decline in Enzyme-extractable P over the course of the incubation, and an increase in HCl-extractable P for compost treatments. As Enzyme-extractable P represents the organic P fraction (DeLuca et al., 2015), this suggests that some organic P was mineralized over the course of the incubation and adsorbed to mineral surfaces or incorporated in inorganic precipitates. This is consistent with literature on the decomposition of organic P (Taylor et al., 1978; Jalali and Ranjbar, 2009; Gagnon et al., 2012).
4.3 Implications for human excreta-derived amendment application
Our results have implications for the application of human excreta-derived fertilizers. We demonstrate that urine and UEBC are a significant source of plant available N, while FDCs are a significant source of plant available P. Urine-N is a relatively fast cycling pool of N, while FDC-P is a fast cycling pool of P. FDC also provides a moderate amount of slow cycling N, and urine-P appears to be a much slower cycling pool that was unavailable over the course of our study.
Stored Urine applied alone results in nearly the entire crop N demand being met immediately, with 187.6 kg-N ha−1 available on day 0 (Supplementary Table S1). Similarly, 148.6 kg-N ha−1 was available for Fresh Urine on day 0. On day 5, slightly more of the crop N demand was met with Fresh Urine, with 82.8 kg-N ha−1 available compared to 71.6 kg-N ha−1 for Stored Urine. This implies that Stored or Fresh Urine applied alone is an excellent source of plant available N at the start of the growing season, with a slight preference for immediate availability from Stored Urine. This is consistent with high mineral fertilizer equivalencies observed from urine fertilizers (Martin et al., 2021). As noted previously, N volatilization was likely high from Fresh and Stored Urine, potentially depleting their fertilization values. We recommend that urine should be well incorporated into the soil when applied alone to reduce volatilization risk. Urine could be used similarly to synthetic fertilizer as a preplant or sidedress to meet specific crop N demand throughout the growing season. UEBC prepared with a low pyrolysis temperature, lignocellulosic biochar supplied approximately half of the available N as Fresh or Stored Urine applied alone, with 75.4 kg-N ha−1 from Fresh UEBC and 69.8 kg-N ha−1 from Stored UEBC on day 0, and 30.4 kg-N ha−1 from Fresh UEBC and kg-N ha−1 and 39.6 kg-N ha−1 from Stored UEBC at day 5. Like urine-only treatments, N was mainly available from UEBCs within the first five days. For this reason, we recommend applying UEBC to crops with a high initial N demand, with adequate soil incorporation to prevent volatilization. Based on our results, we recommend applying similar UEBC preparations at a rate of 400 kg-N ha−1 to provide approximately 200 kg-N ha−1 However, as noted previously, UEBC-N desorption dynamics likely differ with plant root gradients and biochar weathering. We observed a slow release of N for FDCs applied on a total N basis in our study, with 36.6 kg-N ha−1 from Sanergy Evergrow total N and 11.8 kg-N ha−1 from SOIL Konpos Lakay total N on day 60. Applying FDCs on a PAN basis did not result in proportional N release, with approximately 34 kg-N ha−1 available from each at the start of the growing season, but declining availability thereafter. For this reason, we recommend applying smaller amounts of FDC as an N fertilizer, though applying larger quantities may result in long-term soil health benefits (Courtney and Mullen, 2008). Additionally, the carry-over effect of PAN FDC application on crop yield and soil carbon accrual has been demonstrated (Ryals et al., 2021).
Though we did not normalize amendment application on a P basis, we demonstrated that even low rates of FDC-P application stimulate significant native P mining, likely from inorganic precipitates or P adsorbed to clay minerals, in an acidic soil with a relatively high organic matter content of 4.9% (Hiederer and Köchy, 2011). Indeed, FDCs applied on a total N basis supplied an average of 0.53 kg-P ha−1 (Supplementary Table S3) but stimulated an average of 174 kg-PO4-P ha−1 extracted with 1M HCl at day 0. Practically, this means that while FDC supplies low rates of P when applied on an N basis, considerable soil P mining may be expected for soils with legacy P. Available P was high across BBP extractants for PAN FDCs, particularly for Citric Acid and HCl extractions. As a P fertilizer, applying FDC on a PAN basis may be expected to stimulate approximately 1000 kg-PO4-P ha−1 at the end of a growing season, compared to the approximately 7.5 kg-PO4-P ha−1 applied (Supplementary Table S3). We also demonstrate that urine-P is potentially rapidly immobilized after application and not liberated over the course of the growing season, implying that urine alone is not a reliable source of P fertilizer. Our research points to the need for BBP extraction from soils amended with human excreta-derived fertilizers across soil types with various site histories to better elucidate the results of this study. This work provides valuable insight into the potential of human-excreta derived amendments to meet specific crop N and P demand over the course of a typical growing season, which may be applied to a diversity of agroecosystems with different fertilization strategies and cropping systems.
Future work should address key knowledge gaps in this research area. The mineralization of K from human excreta has been understudied and should be prioritized. Isotopic studies of N and P uptake from human excreta-derived fertilizers should also be undertaken. We used a 0.53-μm sieve to prepare the UEBC in this study, which resulted in a wet urine-biochar slurry that was difficult to handle. Future UEBC research should focus on optimizing N and P recovery and plant availability while creating a drier product that is easy and safe to handle and reduces the mass of the product. Additionally, the co-application of P-rich FDC and N-rich urine or UEBC should be studied, to determine if mineralization dynamics differ and if total crop N and P demand can be met through human excreta alone.
5 Conclusion
This research demonstrates that human excreta-derived fertilizers are a good source of plant available N and P. Our results suggest that comparatively more available N is present in urine-derived fertilizers, and more available P is present in FDCs in a simulated 90-day cropping cycle. Urine-N was available during the first five days of a simulated cropping cycle, with nearly the entire N demand met from Stored Urine and slightly less from Fresh Urine. Approximately half of the urine-N in UEBC was plant available, also within the first five days. Urine-P was rapidly immobilized. FDC applied on a total N and PAN basis stimulated substantial mining of legacy P incorporated in inorganic precipitates or adsorbed to clay minerals. Feces-derived-N stimulates a slow release of mineral N. These results are relevant to container-based sanitation and other source-separation based sanitation organizations, and researchers and producers interested in human excreta-derived fertilizers as a novel source of plant-available N and P.
Data availability statement
The original contributions presented in the study are included in the article/Supplementary Material. Further inquiries can be directed to the corresponding author.
Ethics statement
The studies involving humans were approved by The Office of Research Compliance and Integrity at UC Merced. The studies were conducted in accordance with the local legislation and institutional requirements. The participants provided their written informed consent to participate in this study.
Author contributions
EB: Conceptualization, Data curation, Formal analysis, Funding acquisition, Investigation, Methodology, Project administration, Resources, Software, Supervision, Validation, Visualization, Writing – original draft, Writing – review & editing. TG: Conceptualization, Methodology, Supervision, Validation, Writing – review & editing. RR: Conceptualization, Funding acquisition, Methodology, Resources, Supervision, Validation, Writing – review & editing.
Funding
The author(s) declare financial support was received for the research, authorship, and/or publication of this article. This work was supported in part by the Rotary District 5220.
Acknowledgments
The authors thank Isabella Segarra, Jess Saad, Evelyn Perez-Agredano, Ariadne Castaneda, and Dounya Moukhlis for helping conduct the experiment.
Conflict of interest
The authors declare that the research was conducted in the absence of any commercial or financial relationships that could be construed as a potential conflict of interest.
Publisher’s note
All claims expressed in this article are solely those of the authors and do not necessarily represent those of their affiliated organizations, or those of the publisher, the editors and the reviewers. Any product that may be evaluated in this article, or claim that may be made by its manufacturer, is not guaranteed or endorsed by the publisher.
Supplementary material
The Supplementary Material for this article can be found online at: https://www.frontiersin.org/articles/10.3389/fagro.2024.1425461/full#supplementary-material
References
Amlinger F., Götz B., Dreher P., Geszti J., Weissteiner C. (2003). Nitrogen in biowaste and yard waste compost: dynamics of mobilisation and availability—a review. Eur. J. Soil Biol. 39, 107. doi: 10.1016/S1164-5563(03)00026-8
Ashley K., Cordell D., Mavinic D. (2011). A brief history of phosphorus: From the philosopher’s stone to nutrient recovery and reuse. Chemosphere 84, 737–746. doi: 10.1016/j.chemosphere.2011.03.001
Bakshi S., Banik C., Laird D. A., Smith R., Brown R. C. (2021). Enhancing biochar as scaffolding for slow release of nitrogen fertilizer. ACS Sustain. Chem. Eng. 9, 8222–8231. doi: 10.1021/acssuschemeng.1c02267
Barrow N. J., Roy D., Debnath A. (2022). Evaluating the benefits of legacy phosphate. Plant Soil 480, 561–570. doi: 10.1007/s11104-022-05601-3
Berendes D., Levy K., Knee J., Handzel T., Hill V. R. (2015). Ascaris and Escherichia coli inactivation in an ecological sanitation system in Port-au-Prince, Haiti. PloS One 10, 1–14. doi: 10.1371/journal.pone.0125336
Bischak E., Fusi S. C., Jeliazovski J., Beheshtian K., Ryals R. (2024). Urine-enriched biochar: Coupling sustainability in sanitation and agriculture. Elem Sci. Anth 12, 118. doi: 10.1525/elementa.2022.00118
Bonvin C., Etter B., Udert K. M., Frossard E., Nanzer S., Tamburini F., et al. (2015). Plant uptake of phosphorus and nitrogen recycled from synthetic source-separated urine. Ambio 44, 217–227. doi: 10.1007/s13280-014-0616-6
Bünemann E. K., Oberson A., Liebisch F., Keller F., Annaheim K. E., Huguenin-Elie O., et al. (2012). Rapid microbial phosphorus immobilization dominates gross phosphorus fluxes in a grassland soil with low inorganic phosphorus availability. Soil Biol. Biochem. 51, 84–95. doi: 10.1016/j.soilbio.2012.04.012
Cai Y., Qi H., Liu Y., He X. (2016). Sorption/desorption behavior and mechanism of NH4+ by biochar as a nitrogen fertilizer sustained-release material. J. Agric. Food Chem. 64, 4958–4964. doi: 10.1021/acs.jafc.6b00109
Castejón-del Pino R., Cayuela M. L., Sánchez-García M., Sánchez-Monedero M. A. (2023). Nitrogen availability in biochar-based fertilizers depending on activation treatment and nitrogen source. Waste Manage. 158, 76–83. doi: 10.1016/j.wasman.2023.01.007
Chin W., Kroontje W. (1963). Urea hydrolysis and subsequent loss of ammonia. Soil Sci. Soc. Am. J. 27, 316. doi: 10.2136/sssaj1963.03615995002700030030x
Christie P., Wasson E. A. (2001). Short-term immobilization of ammonium and nitrate added to a grassland soil. Soil Biol. Biochem. 33, 1277–1278. doi: 10.1016/S0038-0717(00)00237-6
Courtney R. G., Mullen G. J. (2008). Soil quality and barley growth as influenced by the land application of two compost types. Bioresour. Technol. 99, 2913–2918. doi: 10.1016/j.biortech.2007.06.034
Crain G. M., McLaren J. R., Brunner B., Darrouzet-Nardi A. (2018). Biologically available phosphorus in biocrust-dominated soils of the chihuahuan desert. Soil Syst. 2, 56. doi: 10.3390/soilsystems2040056
Crespi J. M., Hart C., Pudenz C. C., Schulz L. L., Wongpiyabovorn O., Zhang W., et al. (2022). An Examination of Recent Fertilizer Price Changes (Ames, Iowa: Iowa State University).
DeLuca T. H., Glanville H. C., Harris M., Emmett B. A., Pingree M. R. A., De Sosa L. L., et al. (2015). A novel biologically-based approach to evaluating soil phosphorus availability across complex landscapes. Soil Biol. Biochem. 88, 110–119. doi: 10.1016/j.soilbio.2015.05.016
Doydora S., Gatiboni L., Grieger K., Hesterberg D., Jones J. L., McLamore E. S., et al. (2020). Accessing legacy phosphorus in soils. Soil Syst. 4, 74. doi: 10.3390/soilsystems4040074
Gagnon B., Demers I., Ziadi N., Chantigny M. H., Parent L.-É, Forge T. A., et al. (2012). Forms of phosphorus in composts and in compost- amended soils following incubation. Can. J. Soil Sci. 92 (5), 711–721. doi: 10.4141/cjss2012-032
Haider G., Joseph S., Steffens D., Müller C., Taherymoosavi S., Mitchell D., et al. (2020). Mineral nitrogen captured in field-aged biochar is plant-available. Sci. Rep. 10, 13816. doi: 10.1038/s41598-020-70586-x
Hale S. E., Alling V., Martinsen V., Mulder J., Breedveld G. D., Cornelissen G. (2013). The sorption and desorption of phosphate-P, ammonium-N and nitrate-N in cacao shell and corn cob biochars. Chemosphere 91, 1612–1619. doi: 10.1016/j.chemosphere.2012.12.057
Harder R., Wielemaker R., Larsen T. A., Zeeman G., Öberg G. (2019). Recycling nutrients contained in human excreta to agriculture: Pathways, processes, and products. Crit. Rev. Environ. Sci. Technol. 49 (8), 695–743. doi: 10.1080/10643389.2018.1558889
Harder R., Wielemaker R., Molander S., Öberg G. (2020). Reframing human excreta management as part of food and farming systems. Water Res. 175, 115601. doi: 10.1016/j.watres.2020.115601
Hawkins J. M. B., Vermeiren C., Blackwell M. S. A., Darch T., Granger S. J., Dunham S. J., et al. (2022). The effect of soil organic matter on long-term availability of phosphorus in soil: Evaluation in a biological P mining experiment. Geoderma 423, 115965. doi: 10.1016/j.geoderma.2022.115965
Hiederer R., Köchy M. (2011). Global soil organic carbon estimates and the harmonized world soil database (Luxembourg: European Commission Joint Research Centre Institute for Environment and Sustainability, LU).
Jalali M., Ranjbar F. (2009). Rates of decomposition and phosphorus release from organic residues related to residue composition. J. Plant Nutr. Soil Sci. 172, 353–359. doi: 10.1002/jpln.200800032
Johansson G. (1998). Chemical fixation of ammonia to soil organic matter after application of urea. Acta Agriculturae Scandinavica Section B — Soil Plant Sci. 48, 73–78. doi: 10.1080/09064719809362482
Jönsson H., Stintzing A. R., Vinnerås B., Salomon E. (2004). Guidelines on the use of urine and faeces in crop production, Stockholm Environment Institute (Sweden: Stockholm).
Kelova M. E., Eich-Greatorex S., Krogstad T. (2021). Human excreta as a resource in agriculture – Evaluating the fertilizer potential of different composting and fermentation-derived products. Resources Conserv. Recycling 175, 105748. doi: 10.1016/j.resconrec.2021.105748
Krein D. D. C., Rosseto M., Cemin F., Massuda L. A., Dettmer A. (2023). Recent trends and technologies for reduced environmental impacts of fertilizers: a review. Int. J. Environ. Sci. Technol 20, 12903–12918. doi: 10.1007/s13762-023-04929-2
Langergraber G., Muellegger E. (2005). Ecological Sanitation - A way to solve global sanitation problems? Environ. Int. 31, 433–444. doi: 10.1016/j.envint.2004.08.006
Larsen T. A., Udert K. M., Lienert J. (2013). Source Separation and Decentralization for Wastewater Management, Source Separation and Decentralization for Wastewater Management (London, UK: IWA Publishing). doi: 10.2166/9781780401072
Lazicki P., Geisseler D., Lloyd M. (2020). Nitrogen mineralization from organic amendments is variable but predictable. J. Environ. Qual. 49, 483–495. doi: 10.1002/jeq2.20030
Lehmann J., Gaunt J., Rondon M. (2006). Bio-char sequestration in terrestrial ecosystems - A review. Mitigation Adaptation Strategies Global Change 11, 403–427. doi: 10.1007/s11027-005-9006-5
Ma Q., Zheng J., Watanabe T., Funakawa S. (2021). Microbial immobilization of ammonium and nitrate fertilizers induced by starch and cellulose in an agricultural soil. Soil Sci. Plant Nutr. 67, 89–96. doi: 10.1080/00380768.2020.1843072
Martin T. M. P., Levavasseur F., Dion C., Vidal M., Genermont S., Carozzi M., et al. (2023). High fertilizing value but potentially high volatilization of urine based fertilizers. Nutr. Cycling Agroecosyst. 127, 265–284. doi: 10.1007/s10705-023-10304-x
Martin T. M. P., Levavasseur F., Dox K., Tordera L., Esculier F., Smolders E., et al. (2021). Physico-chemical characteristics and nitrogen use efficiency of nine human urine-based fertilizers in greenhouse conditions. J. Soil Sci. Plant Nutr. 21, 2847–2856. doi: 10.1007/s42729-021-00571-4
Mašek O., Brownsort P., Cross A., Sohi S. (2013). Influence of production conditions on the yield and environmental stability of biochar. Fuel 103, 151–155. doi: 10.1016/j.fuel.2011.08.044
Masrura S. U., Khan E. (2022). “Biochar for nutrient recovery from source-separated urine,” in Biochar in Agriculture for Achieving Sustainable Development Goals (Amsterdam, The Netherlands: Elsevier), 263–272. doi: 10.1016/B978-0-323-85343-9.00007-0
McNicol G., Jeliazovski J., François J. J., Kramer S., Ryals R. (2020). Climate change mitigation potential in sanitation via off-site composting of human waste. Nat. Climate Change 10, 545–549. doi: 10.1038/s41558-020-0782-4
Mulvaney R. L. (1996). “Nitrogen—Inorganic forms,” in Methods of Soil Analysis: Part 3-Chemical Methods. Eds. Sparks D. L., Page A. L., Helmke P. A., Loeppert R. H., Soltanpour P. N., Tabatabai M. A., Johnston C. T., Sumner M. E. (Madison, WI: Soil Science Society of America, Inc., American Society of Agronomy, Inc.), 1123–1184.
Nommik H., Vahtras K. (1982). “Retention and fixation of ammonium and ammonia in soils,” in Nitrogen in Agricultural Soils (Madison, WI: John Wiley & Sons, Ltd), 123–171. doi: 10.2134/agronmonogr22.c4
Ohno T., Zibilske L. M. (1991). Determination of low concentrations of phosphorus in soil extracts using malachite green. Soil Sci. Soc. Am. J. 55, 892–895. doi: 10.2136/sssaj1991.03615995005500030046x
Preneta N., Kramer S., Magloire B., Noel J. M. (2013). Thermophilic co-composting of human wastes in Haiti. J. Water Sanitation Hygiene Dev. 3, 649–654. doi: 10.2166/washdev.2013.145
R Core Team. (2022). R: A language and environment for statistical computing. (Vienna, Austria: R Foundation for Statistical Computing). Available at: https://www.R-project.org/.
Rasse D. P., Weldon S., Joner E. J., Joseph S., Kammann C. I., Liu X., et al. (2022). Enhancing plant N uptake with biochar-based fertilizers: limitation of sorption and prospects. Plant Soil 2022, 1–24. doi: 10.1007/s11104-022-05365-w
Ray H., Saetta D., Boyer T. H. (2018). Characterization of urea hydrolysis in fresh human urine and inhibition by chemical addition. Environ. Science: Water Res. Technol. 4, 87–98. doi: 10.1039/C7EW00271H
Rich Earth Institute (2019). Guide to Starting a Community-scale Urine Diversion Program. (Brattleboro, VT: Rich Earth Institute).
Rockström J., Edenhofer O., Gaertner J., DeClerck F. (2020). Planet-proofing the global food system. Nat. Food 1, 3–5. doi: 10.1038/s43016-019-0010-4
Rumeau M., Marsden C., Ait-Mouheb N., Crevoisier D., Pistocchi C. (2023). Fate of nitrogen and phosphorus from source-separated human urine in a calcareous soil. Environ. Sci. pollut. Res. 30, 65440–65454. doi: 10.1007/s11356-023-26895-5
Russel K. C., Hughes K., Roach M., Auerbach D., Foote A., Kramer S., et al. (2019). Taking container-based sanitation to scale: opportunities and challenges. Front. Environ. Sci. 7. doi: 10.3389/fenvs.2019.00190
Ryals R., Bischak E., Porterfield K. K., Heisey S., Jeliazovski J., Kramer S., et al. (2021). Toward zero hunger through coupled ecological sanitation-agriculture systems. Front. Sustain. Food Syst. 5. doi: 10.3389/fsufs.2021.716140
Ryals R., Kaiser M., Torn M. S., Berhe A. A., Silver W. L. (2014). Impacts of organic matter amendments on carbon and nitrogen dynamics in grassland soils. Soil Biol. Biochem. 68, 52–61. doi: 10.1016/j.soilbio.2013.09.011
Ryals R., McNicol G., Porder S., Kramer S. (2019). Greenhouse gas fluxes from human waste management pathways in Haiti. J. Clean. Prod. 226, 106–113. doi: 10.1016/j.jclepro.2019.04.079
Stanford G. (1973). Rationale for optimum nitrogen fertilization in corn production. J. Environ. Qual. 2, 159–166. doi: 10.2134/jeq1973.00472425000200020001x
Sullivan D. M., Bary A. I., Nartea T. J., Myrhe E. A., Cogger C. G., Fransen S. C. (2003). Nitrogen availability seven years after a high-rate food waste compost application. Compost Sci. Utilization 11, 265–275. doi: 10.1080/1065657X.2003.10702133
Takaya C. A., Fletcher L. A., Singh S., Anyikude K. U., Ross A. B. (2016). Phosphate and ammonium sorption capacity of biochar and hydrochar from different wastes. Chemosphere 145, 518–527. doi: 10.1016/j.chemosphere.2015.11.052
Tarpeh W. A., Clark B. D., Nelson K. L., Orner K. D. (2023). “Reimagining excreta as a resource: recovering nitrogen from urine in Nairobi, Kenya,” in Introduction to Development Engineering: A Framework with Applications from the Field. Eds. Madon T., Gadgil A. J., Anderson R., Casaburi L., Lee K., Rezaee A. (Springer International Publishing, Cham), 429–462. doi: 10.1007/978-3-030-86065-3_16
Taylor J. M., Sikora L. J., Tester C. F., Parr J. F. (1978). Decomposition of sewage sludge compost in soil: II. Phosphorus and Sulfur transformations. J. Environ. Qual. 7, 119–123. doi: 10.2134/jeq1978.00472425000700010024x
Tilley E., Atwater J., Mavinic D. (2008). Recovery of struvite from stored human urine. Environ. Technol. 29, 797–806. doi: 10.1080/09593330801987129
Trimmer J. T., Miller D. C., Guest J. S. (2019). Resource recovery from sanitation to enhance ecosystem services. Nat. Sustainability 2, 681–690. doi: 10.1038/s41893-019-0313-3
Udert K. M., Larsen T. A., Biebow M., Gujer W. (2003). Urea hydrolysis and precipitation dynamics in a urine-collecting system. Water Res. 37, 2571–2582. doi: 10.1016/S0043-1354(03)00065-4
Keywords: ecological sanitation, nitrogen, phosphorus, organic fertilizer, sustainable agriculture, container-based sanitation, compost, urine
Citation: Bischak E, Ghezzehei TA and Ryals R (2024) Nitrogen and phosphorus mineralization dynamics in human excreta-derived fertilizers. Front. Agron. 6:1425461. doi: 10.3389/fagro.2024.1425461
Received: 29 April 2024; Accepted: 25 June 2024;
Published: 09 July 2024.
Edited by:
Tajamul Hussain, Oregon State University, United StatesCopyright © 2024 Bischak, Ghezzehei and Ryals. This is an open-access article distributed under the terms of the Creative Commons Attribution License (CC BY). The use, distribution or reproduction in other forums is permitted, provided the original author(s) and the copyright owner(s) are credited and that the original publication in this journal is cited, in accordance with accepted academic practice. No use, distribution or reproduction is permitted which does not comply with these terms.
*Correspondence: Elena Bischak, ZWJpc2NoYWtAdWNtZXJjZWQuZWR1